- 1The Institute for Advanced Study of Coastal Ecology, Ludong University, Yantai, Shandong, China
- 2Key Laboratory of Ecological Restoration and Conservation of Coastal Wetlands in Universities of Shandong, Ludong University, Yantai, Shandong, China
Blocking of hydrological connectivity could greatly impact the sediment deposition process and change water and salinity conditions, which in turn affect plant germination, growth, and development in delta wetlands. A 2-year experiment, which included the effects of soil burial, water, and salinity on germination, growth, and production, was conducted to examine the function of hydrological connectivity blocking on the development of Suaeda salsa, a halophyte species. The results demonstrated that soil burial, water, and salinity all had significant effects on seed germination, plant growth, and production (p < 0.05). Seed germination decreased as soil buried depth increased (< 4 cm), and seeds did not germinate successfully when the buried depth was > 4 cm. Seed germination was the highest at 0 cm burial. However, moderate burial was beneficial for seedling emergence; therefore, the survival rate was the lowest when seeds were distributed at the surface (0 cm). Water and salinity both significantly affected the germination, growth, and productivity of S. salsa. Moderate salinity (10–20 g/kg) and fluctuating water (0–10 cm water depth) were suitable for seed germination and plant growth. Low salinity (< 10 g/kg), High salinity (>20 g/kg), drought, and high water levels (long-term flooding with water depth > 10 cm) were not conducive to the growth of S. salsa, and biomass and seed yield were also reduced. As a halophyte, salinity that is too low or too high is unsuitable for S. salsa population. Water and salinity also significantly affected S. salsa population (p < 0.05). In particular, water can offset the hazards of high salt concentrations. Blocking of hydrological connectivity can influence seed germination, yield, and vitality. In this case, S. salsa may have died out from the coastal wetland due to the lack of hydrological connectivity restoration.
Introduction
Hydrologic connectivity is a hydrologic process that supports the transfer of mass and energy between or among different water bodies and/or locations (soils, atmosphere, and vegetation) across a landscape (Lexartza-Artza and Wainwright, 2009; Bracken et al., 2013). Complete hydrological connectivity cannot only promote the cycle of energy and nutrients but also provide important habitats for animals and plants (Covino, 2017; Shao et al., 2019; Zheng et al., 2022). Wetland ecosystems with good hydrological connections can keep nutrients in the water body relatively stable in a mutable external environment and play a significant role in maintaining biodiversity (Noe et al., 2019; Norton et al., 2022). Hydrological connectivity is particularly important in delta wetlands, with intense interactions between saline and fresh water (Liu et al., 2020; Cui et al., 2022). In this context, delta wetlands are not only the habitat of many migratory waterfowl and are important for the protection base of biodiversity, but they also provide a buffer zone to maintain the dynamic balance of sea and land. They play a unique role in the material cycle, energy flow, and information transmission between rivers and oceans (Liu et al., 2021).
Suaeda salsa community is the main vegetation and the only pioneer plant in the salt marsh in the Yellow River Delta (YRD), China. It is mainly distributed in the transitional zone from land to beach, and provides an important habitat for birds and macrobenthos (Yu et al., 2012). Seed germination of S. salsa is highly susceptible to environmental factors such as water depth, salinity, and burial depth (Yu et al., 2012; Wang F. et al., 2015; Müller et al., 2019. Therefore, the distribution and growth of S. salsa may be vulnerable to changes in hydrological connectivity. In recent years, due to climate change and human activities such as roads and dams, the hydrological connectivity of the Yellow River has been seriously affected, the sediment transport process has been blocked, and the water-salt-sediment environment of coastal wetlands has also changed significantly (Wang S. et al., 2015; Saunders et al., 2016; Li et al., 2021). The amount of sediment entering the sea has decreased by 89% over the past 50 years, which has decreased the deposition rate of the YRD. Siltation and erosion in the estuary area have been altered, the amount of fresh water and salt water decreased dramatically, and soil salinity increased accordingly (Wang S. et al., 2015; Xue et al., 2022). Consequently, the salt marsh habitats of the YRD had deteriorated or many vegetation types had dried out (Liu, 2020). At the same time, the area of S. salsa shows a decreasing trend since 2006, and the dominance degree of S. salsa is decreasing continuously and the degree of fragmentation is severe (Zhang et al., 2022). Therefore, analyzing the ecological effects of dialectical hydrological connectivity on the development of S. salsa is of great significance.
Once hydrological connectivity blocking occurs in a delta, its effects on S. salsa community are unpredictable; hence, a greenhouse experiment was conducted to test it. In this experiment, seed germination and growth of S. salsa were subjected to different conditions that represent the situation of hydrological connectivity blocking. The purpose of the study was to solve those questions: (1) Do the hydrological connectivity blocking have negative or positive effect on S. salsa development? (2) What is the way that hydrological connectivity blocking affects S. salsa development? The results can provide valuable implications for the management of hydrological system and the restoration of degraded wetlands in the YRD.
Materials and methods
Site description
The study area is located in the YRD (118°3381811°2081, 37°353781°12378118y Dongying City, Shandong Province, China. The study area has a warm temperate continental monsoon climate, with distinctive seasons and rainy summers. The average annual temperature is 12.1°C. The frost-free period lasts 196 d per year in this region. The annual average rainfall in the study area is 551.6 mm, whereas the annual average evaporation is 1,962 mm. Approximately 70% of the precipitation occurs from June to August. Surface water in this area is mainly affected by precipitation and upriver water, particularly by the water-sediment regulation of the Yellow River at the end of June since 2002. The main soil types are tidal and salt soil. S. salsa and Phragmites australis are dominant species in the YRD (Guan et al., 2020).
Experiment design
Seed preparation
Fully developed seeds of S. salsa local genotypes were collected in October 2019 from the YRD National Natural Reserve. All seeds were kept under dark and dry conditions at 4°C until the start of the germination experiments. Soil for the experiment was collected from a depth of 20 cm near the YRD in 2019. All experiments were conducted in a greenhouse located at Ludong University, China. At the beginning of the experiment, seeds were surface-sterilized in an aqueous solution of 0.1% KMnO4 for 10 min to avoid fungal attack, and subsequently rinsed with distilled water before being used in seed germination experiments.
Experiment 1: Effects of soil burial depth on seed germination in 2019
Germination experiments at different buried depths were conducted in a barrel (radius: 5 cm and height: 15 cm). Seeds were germinated at 0 cm (M0), 1 cm (M1), 2 cm (M2), 4 cm (M4), and 6 cm (M6) buried depths, respectively. The soil was collected from the YRD. The salinity, water content, bulk density, electrical conductivity and pH of the initial experimental soils were 1.12 g/kg, 27.18%, 1.09 g/cm3, 478 μm/cm, and 8.92, respectively. For each treatment, there were three replicates of 50 seeds each. During seed germination in buried soil, seeds were irrigated with distilled water to maintain saturation. All seeds were subjected to an alternating diurnal regime of 12 h of light at 25°C and 12 h of darkness at 15°C for 21 days. Seeds were considered germinated when the radicle protruded 1 mm from seed coat. Germination was recorded daily for 14 days, and seedling survival was recorded at the end of the experiment. The entire experiment lasted 4 weeks.
Experiment 2: Effects of water and salt on germination and growth of Suaeda salsa in 2019
After the burial experiment, S. salsa seeds were planted in a barrel (radius: 15 cm and height: 35 cm) at a depth of 1 cm (according to the result of the soil burial experiment) to investigate the effects of the water-salt gradient on their germination and growth. The experiment began with three water treatments [long-term flooding (5–15 cm, F), periodic flooding (0–10 cm, S), and long-term drought (drought to saturated, D)], and four salinity treatments (i.e., 0, 10, 20, and 30 g/kg) in an orthogonal design. Salinity was controlled by adding a sea salt solution (obtained from seawater), in which Na+ and Cl– were the most important ions (Table 1). Treatment D was carried out every 7 d. Treatment S was injected twice daily, and water was emptied after 2 h of water injection. Water control of the F treatment was performed every 2 d. Percent germination was recorded every day. A seed was considered germinated when the coleoptiles were elongated to 1 mm. The entire experiment lasted 16 weeks, and height, density, biomass, content of Na+ and Cl– in each part were recorded and examined at the end.
Experiment 3: Germination of seeds produced in experiment 2 in 2020
Germination experiments were conducted in a dish (radius: 5 cm). Seeds were germinated under saturated conditions at 10 g/kg salinity (the ideal germination condition). In this experiment, all seeds produced in experiment 2 were used in this experiment without replicates. During seed germination, the seeds were irrigated with distilled water to maintain moisture. The experiment lasted for 21 days. Percent germination was recorded daily. Seeds were considered germinated when the coleoptiles were elongated to 1 mm.
Data analysis
Statistical analyses were performed using SPSS 20 and Origin 9.2. One-way analysis of variance (ANOVA) was used to determine the effects of burial depth, water regime, and salinity on the germination and growth of S. salsa. To meet the assumptions of homoscedasticity, some growth and photosynthetic parameters were log-transformed or square-root transformed. Before the analyses, graphs with residuals were applied to examine the rationality of the model assumptions and the reliability of the data. Multiple comparisons of means were performed using Duncan’s test; different letters in Figures 1, 2 indicate significant differences at a significance level of 0.05. Values represent the mean ± SE of three replicates.
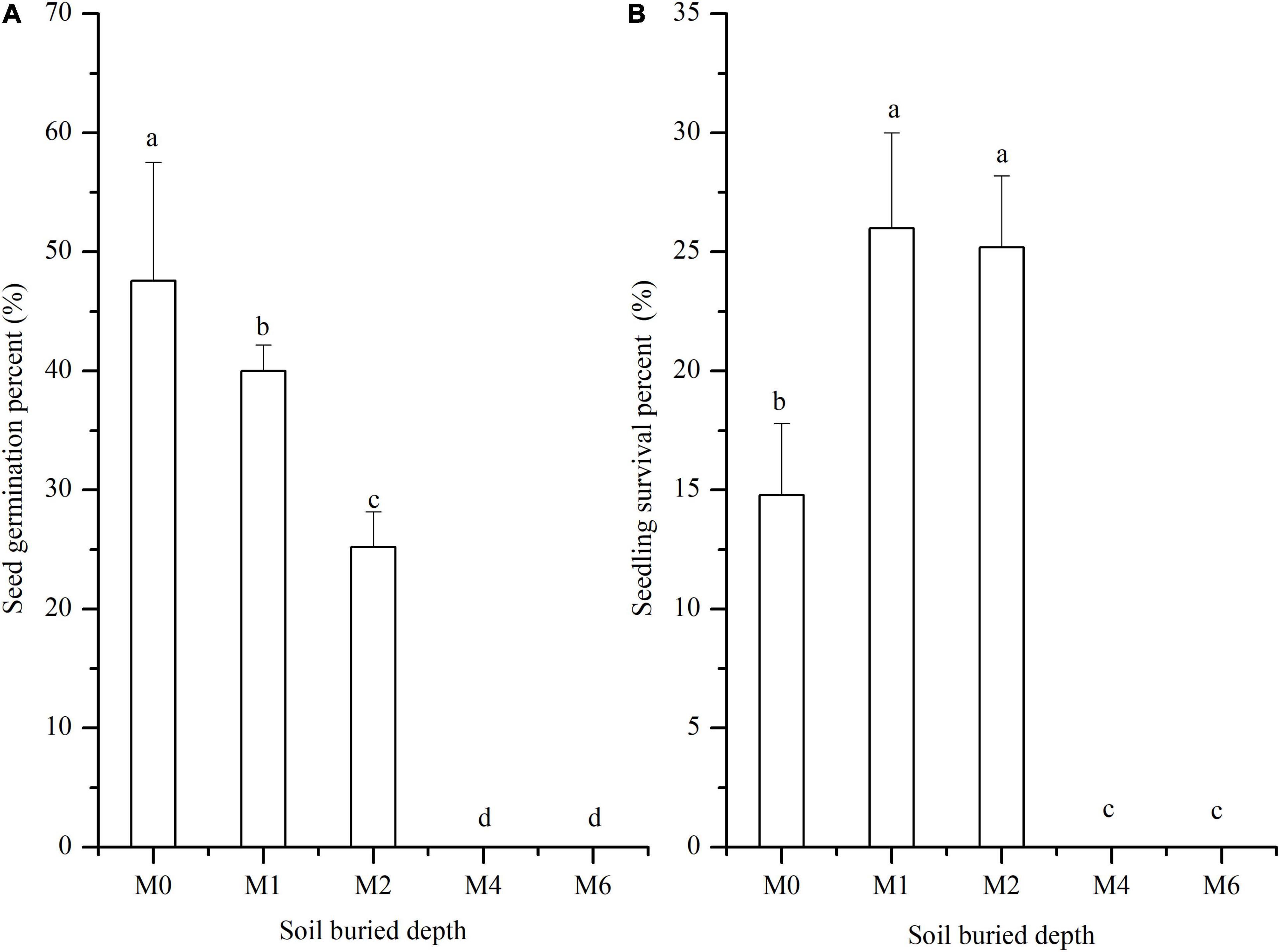
Figure 1. Seed germination of S. salsa subjected to different soil burial depths. (A) Seed germination percent (%) and (B) seedling survival rate subjected to 0 cm (M0), 1 cm (M1), 2 cm (M2), 4 cm (M4), and 6 cm (M6) buried depths. Different letters indicate significant differences in different buried depth. The data was 0 at M4 and M6.
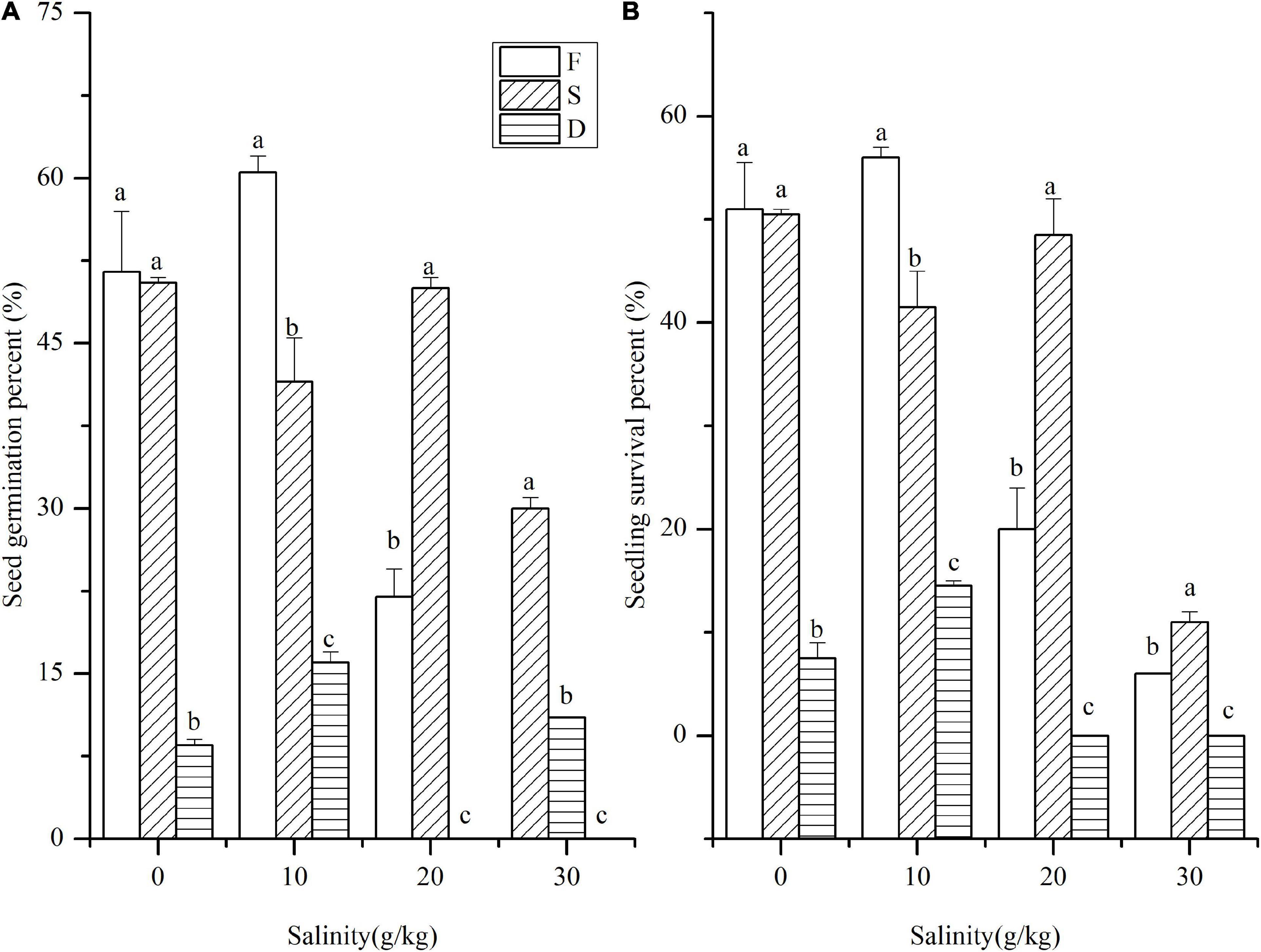
Figure 2. Seed germination of S. salsa subjected to salt and water treatments. (A) Seed germination percent and (B) seedling survival percent subjected to water and salt treatment (long-term flooding (5–15 cm, F), periodic flooding (0–10 cm, S), and long-term drought (drought to saturated, D). Different letters indicate significant differences in different water treatments at the same salinity. The data was 0 at D treatment in salinity values of 20 and 30 (g/kg).
Results
Effects of soil burial on the germination and survival of Suaeda salsa
Analysis of differences indicated that soil burial depth had significant negative effects on germination percent (p < 0.01). As the burial depth increased, the seed germination percent decreased gradually (Figure 1A). Seed germination percent was the highest at M0. When the burial depth was ≥ 4 cm, there was no seed germination. However, the seedling survival percent changed significantly (Figure 1B). The survival percent at M1 was the highest, followed by the germination rates at M2 and M0 treatments. The survival percent were not significantly different between burial depths of M1 and M2.
Effects of water depth and salinity on the germination and survival of Suaeda salsa
Although S. salsa is a halophyte, salinity and water both showed significant effects on its germination and growth (p < 0.01). The germination percent of S. salsa decreased as soil salinity increased, and high salinity was detrimental for seed germination. After 4-week growth, the seedling survival percent was slightly lower than the seed germination percent in all treatments (Figures 2A,B). Water significantly affected germination of S. salsa (p < 0.01). At the period of seed germination, the germination percent was higher in F and S, especially in S. In treatment D, the seed germination percent was the lowest, even at 0 g/kg salinity. In treatment S, except for the 30 g/kg salinity, the seed germination percent were similar. In treatment F, the seed germination percent at 10 g/kg salinity was the highest, even at 30 g/kg salinity, and the germination percent was up to 30%. Subjected to treatments S and D, the seedling survival percent did not decrease much more than the germination percent. The S treatment was the most ideal one for S. salsa under medium and low salinity conditions (salinity was ≤ 20 g/kg). The F treatment (≤ 15 cm) was suitable for S. salsa growth at lower salinity.
Effects of water and salt on the growth of Suaeda salsa
Water and salinity had significant complex effects on the growth of S. salsa. Medium to low salinity (≤ 20 g/kg) and moderate water (periodic flooding at 0–10 cm) were beneficial to the growth of S. salsa (Table 2). Drought and high salinity (salinity was > 30 g/kg) were detrimental to the growth of S. salsa. In the F and D treatment, the height was the highest at 0 g/kg, and the density and total biomass were the highest at 10 g/kg. While in the S treatment, the height and total biomass were the highest at 10 g/kg, and the density was the highest at 0 g/kg. In all treatment, biomass at 10 g/kg salinity was the highest, and biomass was mainly distributed in stems and leaves. In the D treatment, by contrast, root biomass accounted for most of the total biomass. Results of water content were interesting. Salt tolerance gradually increased as water conditions improved. In D treatment, water content was the most at 10 g/kg salinity. In F and S treatment, water content was the most at 20 or 30 g/kg salinity. Multi-way variance analysis indicated that the effects of water are the first priority in salt habitats, and the combined effect of salt and water is also important in regulating S. salsa growth.
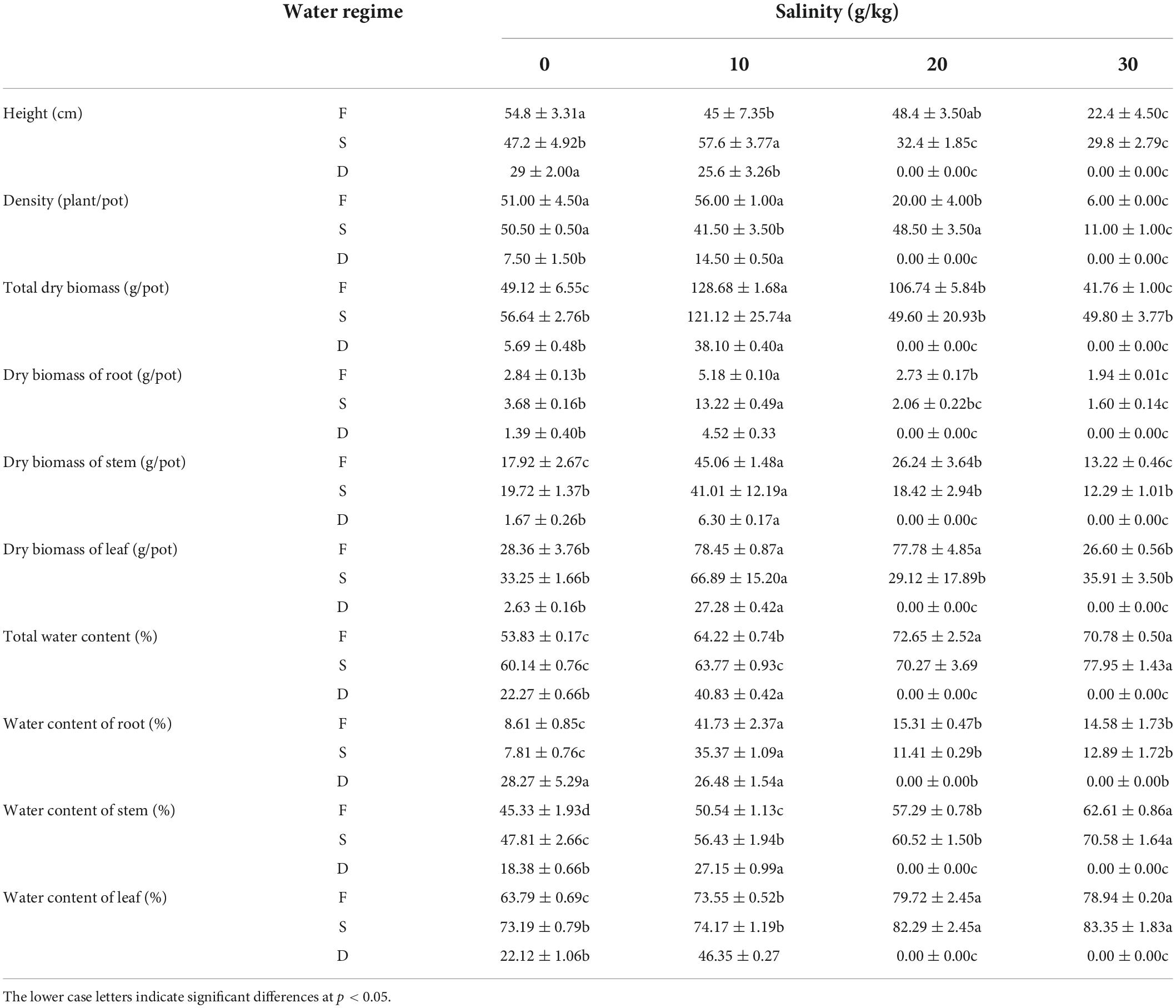
Table 2. Growth characteristics of S. salsa subjected to water [long-term flooding (5–15 cm, F), periodic flooding (0–10 cm, S), and long-term drought (drought to saturated, D)] and salt treatments.
Na+ and Cl– were similarly distributed in each treatment, with the leaf content being the highest, followed by that in the stems and roots (Figures 3A–F). In the 0 salinity treatment, Na+ and Cl– were more evenly distributed in the root-stem-leaf. In the same salinity value, content of Na+ was the highest in the S treatment, followed by that in the stems and roots in F and D treatments, respectively. The difference in Cl– concentration in each treatment was not significant. In the same water regime, contents of Na+ and Cl– increased as salinity increased.
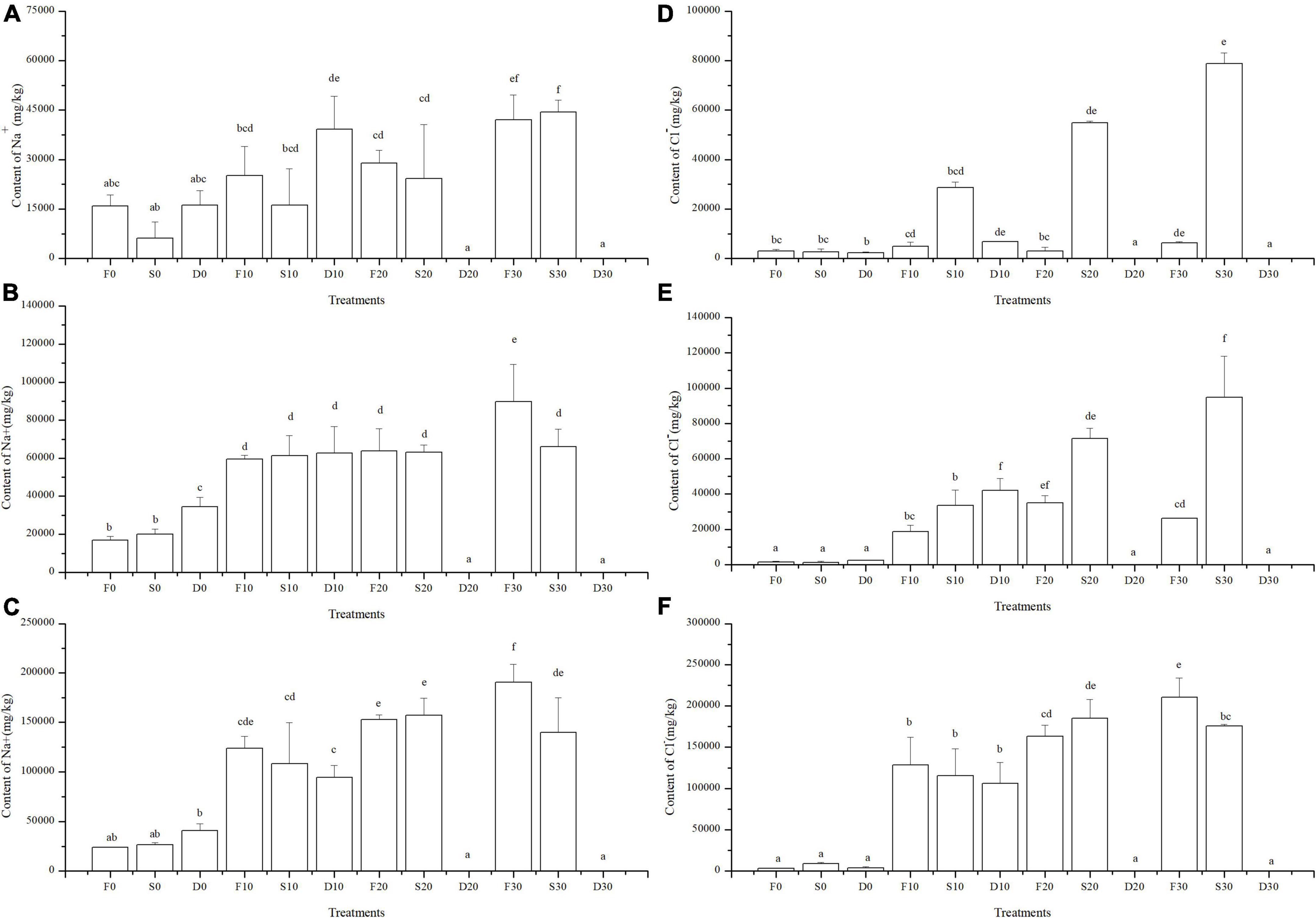
Figure 3. Na+ and Cl– distribution in each part of S. salsa subjected to salt and water treatments. (A) Content of Na+ in the root, (B) Content of Na+ in the stem, (C) Content of Na+ in the root, (D) Content of Cl– in the root, (E) Content of Cl– in the stem, and (F) Content of Cl– in the root. The data was 0 in D20 and D30.
Effects of water-salt treatments on the seed yield and vitality
Seeds are the foundation of the establishment and development of plant communities; therefore, seed yield and vitality are deemed as the preconditions of vegetation establishment and development. There was no seed production in the 0 g/kg and highsalt treatments (except for S30, Table 3). Seeds were produced at F10, S10, D10, F20, and S20 treatment. In the following year, the germination rates of seeds produced in the S10, F10, S20, and F20 treatments were higher than that in the D treatment. Although seeds were produced in the S30 treatment, their germination rate was 0%.
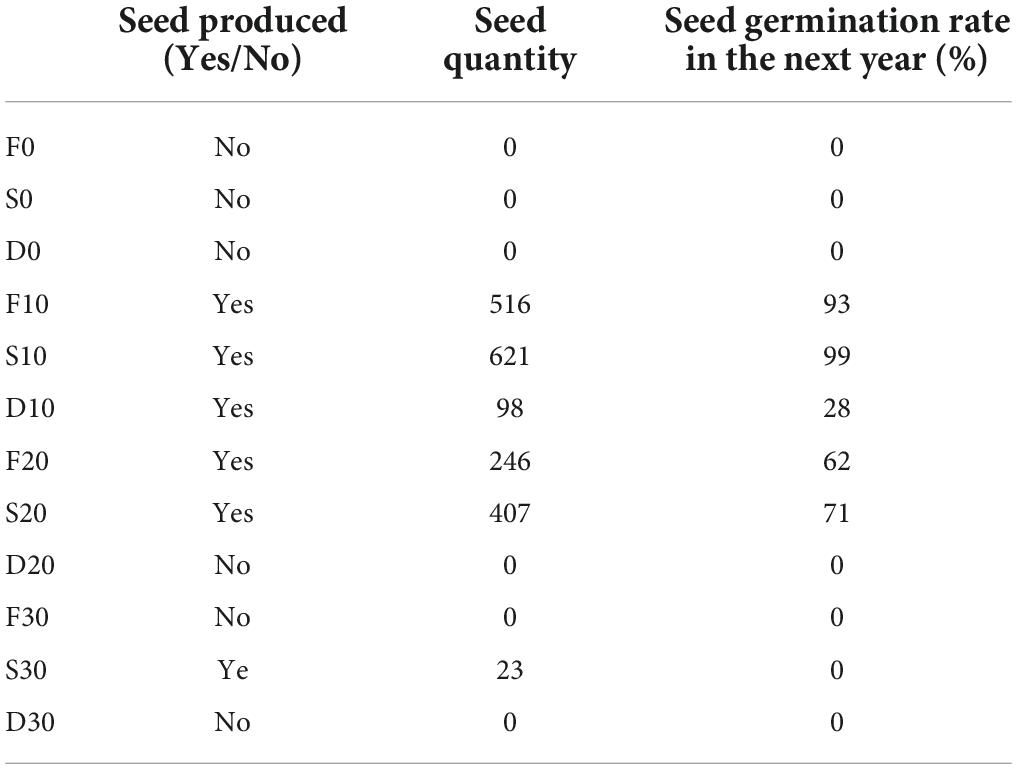
Table 3. Seed production of S. salsa subjected to water [long-term flooding (5–15 cm, F), periodic flooding (0–10 cm, S), and long-term drought (drought to saturated, D)] and salt treatment (0, 10, 20, and 30 g/kg).
Discussion
Germination and seedling emergence are critical stages in the life cycle of plants, particularly for annual halophytes, since they determine whether they can be established in variable environments (salt marshes or deserts) (Merino-Martín et al., 2017; Duan et al., 2018; Müller et al., 2019). In delta wetlands, the mutable deposition process, water regime (timing, duration, and depth), and soil salinity are outstanding environmental problems resulting from lack of hydrological connectivity (Dou et al., 2016). Therefore, adequate hydrological connectivity often determines the growth and distribution of plants. Once hydrological connectivity is blocked, the water cycle process changes, affecting the sediment deposition process and soil salinity, and finally affecting seed germination and plant growth (Wang S. et al., 2015).
Because of the interaction of fresh and saltwater, the sediment thickness in the YRD is variable. Seed germination is regulated by burial depth during the process of deposition (Mou and Sun, 2011). However, the effects were two-sided. Moderate buried depths could generally stimulate more germination and seeding than surface deposition of seed, because burial provides a moist environment around seeds and prevents them from desiccation (Sun et al., 2010). Excessive sediment may prevent seedling sprouting and affect survival because the emergence cannot reach the sediment surface or the seeds are unable to germinate due to lack of oxygen, light, and temperature fluctuation (Sun et al., 2010; Wu et al., 2013). In our study, the initial germination rate decreased and was negatively correlated with burial depth. A large percentage of seedlings emerged at shallow burial depths (≤ 2 cm), but deep burials (> 2 cm) significantly reduced seedling emergence. Similar relationships have also been recorded in other studies. The survival of seedlings is important for plant development. Many studies have indicated that germination rate determines colonization rate. However, many seedling emergences could not successfully colonize the site because of the effects of environmental factors (Duan et al., 2018). In our experiment, although initial germination was the highest at a burial depth of 0 cm, the final survival rate was the lowest. The final survival rate was the highest at 1 cm burial depth, with lower mortality of initial emergences. Mortality was lowest at a buried depth of 2 cm, but the initial germination rate was also low. This result is similar to those of Mou’s findings (Mou and Sun, 2011). The most probable factors that could influence the germination and survival of S. salsa may be the micro-environmental factors surrounding the seeds, such as temperature, humidity, oxygen, and light (Müller et al., 2019). Different burial depths would substantially change these micro-environmental factors and significantly affect seed germination (Limón and Peco, 2016). Under surface conditions, poor water and temperature retention can result in high seedling emergence mortality. Instead, a moderate buried depth could maintain a high seedling emergence survival with suitable influences of micro-environment factors (Soares et al., 2021).
Due to the interaction of fresh water and saline water, water logging and salt content are considered the two key environmental factors for plant establishment, succession, and productivity in the delta wetlands (Hou et al., 2020; Chen et al., 2022; Hussain et al., 2022). Waterlogging stress decreases the availability of carbon dioxide for salt marsh plants while also rendering oxygen levels deficient in the soils. Simultaneously, water influences plant growth through nutrient uptake via restricted transpiration rates and membrane permeability (Wang and Jiang, 2007). Furthermore, excess salinity from seawater affects plant growth via both osmotic stress and ionic toxicity (Wang F. et al., 2015; Louati et al., 2018). In our study, low water and low salinity showed significant positive effects on seed germination, seedling growth, and productivity. High water and salinity negatively affect seed germination and plant growth, and the effects of drought are similar. Our results are agreed with formers’ result, in which no matter single effect or integrated effects of salinity and waterlogging could restricted plant growth, and could reduce plant height and leaf area (Merino-Martín et al., 2017; Lu et al., 2022). As a halophyte, S. salsa thrives in the presence of NaCl. At lower salinity levels, S. salsa can absorb Na+ and Cl– and store them in the vacuoles to lower the plant water potential, thereby improving its ability to absorb water from the soil (Song et al., 2011). In addition, salinity could enhance ATPase and PPase activities, which endows S. salsa with more energy to transfer Na+ and Cl– to vacuoles (Zhang et al., 2010). However, at higher salinity levels, increasing osmotic stress and ionic toxicity would retard the absorption of water and nutrients, and subsequently weaken the photosynthesis and metabolic activity (Li et al., 2022). In this study, Na+ was mainly distributed in the leaves and stems. The Cl– species were mainly distributed in the leaves, and in the 0-salt treatment, Cl– was more evenly distributed in the root-stem-leaf. This is in agreement with Zhang’s study, which indicated that waterlogging can increase Na+ and Cl– concentrations in the leaves.
Studies have shown that salt and waterlogging significantly affect seed yield and quality (Wang F. et al., 2015; Li et al., 2020; Meng et al., 2022). Greater water depth and longer flooding time had a higher impact on the yield, and the yield reduction rate was significantly positively correlated with flooding depth and time (Duan et al., 2018). No significant correlations were found between the seed quality and waterlogging. Salt greatly reduces the seed yield of non-halophytes, particularly of some important cash crops (Yadav et al., 2011) and non-halophytes show significant yield reductions in soils with increasing salinity (Galvan-Ampudia and Testerink, 2011). However, moderate salinity significantly promotes the reproductive growth of halophytes (S. salsa) and increases their number of flowers, number of seeds, and quality (Guo et al., 2018). The seed vitality of halophytes was significantly higher than that under non-saline conditions. In this study, seed yield and vitality at salinities of 10 and 20‰ were better than those at a salinity that was too low or high. Seed yield and vitality in the S and F treatments were better than those in the D treatment.
Conclusion
In delta wetlands, hydrological connectivity blocking could result in the modification of sediment processes, water regimes, and salinity values, which would further alter the germination, seedling, and growth of S. salsa. In this study, we found that blocked ephemeral hydrological connectivity may favor S. salsa germination and growth owing to changes in water and salinity. Blocking short-term hydrological connectivity is suitable for S. salsa growth and development. However, blocking long-term hydrological connectivity (inundation, drought, or excess salinity) is harmful for S. salsa growth. The effects of long-term blocking of hydrological connectivity on the S. salsa population were reflected in the seed yield and quality; long-term blocking sharply reduced the seed yield and vitality. Overall, the findings of this study provide a scientific basis for the protection and restoration of hydrological connectivity and S. salsa habitats in delta wetlands.
Data availability statement
The original contributions presented in this study are included in the article/supplementary material, further inquiries can be directed to the corresponding author.
Ethics statement
Authors state that the research was conducted according to ethical standards.
Author contributions
XW, JSY, and JBY contributed to the conception of the study. YZ and TZ performed the experiment and helped to perform the data analyses. XW and BG contributed significantly to analysis, manuscript preparation, performed the data analyses, and wrote the manuscript. JSY and JBY helped to perform the analysis with constructive discussions. All authors contributed to the article and approved the submitted version.
Funding
This work was supported by the Key Program from the National Natural Science Foundation of China (U2006215 and U1806218) and the National Natural Science Foundation of China (42171111 and 41871087).
Acknowledgments
We thank Editage (www.editage.com) for English language editing.
Conflict of interest
The authors declare that the research was conducted in the absence of any commercial or financial relationships that could be construed as a potential conflict of interest.
Publisher’s note
All claims expressed in this article are solely those of the authors and do not necessarily represent those of their affiliated organizations, or those of the publisher, the editors and the reviewers. Any product that may be evaluated in this article, or claim that may be made by its manufacturer, is not guaranteed or endorsed by the publisher.
References
Bracken, L. J., Wainwright, J., Ali, G. A., Tetzlaff, D., Smith, M. W., Reaney, S. M., et al. (2013). Concepts of hydrological connectivity: Research approaches, pathways and future agendas. Earth Sci. Rev. 119, 17–34. doi: 10.1016/j.earscirev.2013.02.001
Chen, P., Xia, J. B., Ma, H. S., Gao, F. L., Dong, M. M., Xing, X. S., et al. (2022). Analysis of spatial distribution pattern and its influencing factors of the Tamarix chinensis population on the beach of the muddy coastal zone of Bohai Bay. Ecol. Indic. 140:109016. doi: 10.1016/j.ecolind.2022.109016
Covino, T. (2017). Hydrologic connectivity as a framework for understanding biogeochemical flux through watersheds and along fluvial networks. Geomorphology 277, 133–144. doi: 10.1016/j.geomorph.2016.09.030
Cui, Y., Zhang, Y. H., Zhou, S. J., Pan, Y. Y., Wang, R. Q., Li, Z., et al. (2022). Cracks and root channels promote both static and dynamic vertical hydrological connectivity in the Yellow River Delta. J. Clean Prod. 367, 132972.
Dou, P., Cui, B. S., Xie, T., Dong, D. Z., and Gu, B. H. (2016). Macrobenthos Diversity Response to Hydrological Connectivity Gradient. Wetlands 36, 45–55. doi: 10.1007/s13157-014-0580-8
Duan, H. M., Ma, Y. C., Liu, R. R., Li, Q., Yang, Y., and Song, J. (2018). Effect of combined waterlogging and salinity stresses on euhalophyte suaeda glauca. Plant Physiol. Biochem. 127, 231–237. doi: 10.1016/j.plaphy.2018.03.030
Galvan-Ampudia, C. S., and Testerink, C. (2011). Salt stress signals shape the plant root. Curr. Opin. Plant Biol. 14, 296–302. doi: 10.1016/j.pbi.2011.03.019
Guan, B., Zhang, H. X., Wang, X. H., Yang, S. S., Chen, M., Hou, A. X., et al. (2020). Salt is a main factor shaping community composition of arbuscular mycorrhizal fungi along a vegetation successional series in the Yellow River Delta. Catena 185:104318. doi: 10.1016/j.catena.2019.104318
Guo, J. R., Li, Y. D., Han, G. L., Song, J., and Wang, B. S. (2018). NaCl markedly improved the reproductive capacity of the euhalophyte Suaeda salsa. Funct. Plant Biol. 45, 350–361. doi: 10.1071/FP17181
Hou, W. H., Zhang, R. J., Xi, Y. B., Liang, S. X., and Sun, Z. C. (2020). The role of waterlogging stress on the distribution of salt marsh plants in the Liao River estuary wetland. Glob. Ecol. Conserv. 23:e01100. doi: 10.1016/j.gecco.2020.e01100
Hussain, T., Asrar, H., Li, J. S., Feng, X. H., Gul, B., and Liu, X. J. (2022). The presence of salts in the leaf exudate improves the photosynthetic performance of a recreto-halophyte. Tamarix chinensis. Environ. Exp. Bot. 199:104896.
Lexartza-Artza, I., and Wainwright, J. (2009). Hydrological connectivity: Linking concepts with practical implications. Catena 79, 146–152. doi: 10.1016/j.catena.2009.07.001
Li, K. L., Li, Y. D., Guo, J. R., and Wang, B. S. (2020). Preliminary study on na+-mediated improvement of seed yield in euhalophyte Suaeda salsa (in chinese). J. Plant Ecol. 56, 49–56. doi: 10.13592/j.cnki.ppj.2019.0544
Li, Q., Liu, R., Li, Z. H., Fan, H., and Song, J. (2022). Positive effects of NaCl on the photoreaction and carbon assimilation efficiency in Suaeda salsa. Plant Physiol. Bioch. 177, 32–37. doi: 10.1016/j.plaphy.2022.02.019
Li, Y. F., Xu, J. Y., Wright, A., Qiu, C. Q., Wang, C., and Liu, H. Y. (2021). Integrating two aspects analysis of hydrological connectivity based on structure and process to support muddy coastal restoration. Ecol. Indic. 133:108416. doi: 10.1016/j.ecolind.2021.108416
Limón, Á, and Peco, B. (2016). Germination and emergence of annual species and burial depth: Implications for restoration ecology. Acta Oecol. 71, 8–13. doi: 10.1016/j.actao.2016.01.001
Liu, J. K. (2020). Hydrological connectivity and its influence on vegetation in the coast wetland in the yellow river delta. Beijing: Beijing Forestry University.
Liu, J. K., Cui, B. S., Zhang, Z. M., and Zhang, M. X. (2021). Scale effects of structural hydrological connectivity in coastal wetlands in the Yellow River Delta (in chinese). Acta Ecol. Sin. 41, 3745–3754.
Liu, J., Engel, B. A., Zhang, G. F., Wang, Y., Wu, Y. N., Zhang, M. X., et al. (2020). Hydrological connectivity: One of the driving factors of plant communities in the Yellow River Delta. Ecol. Indic. 112:106150. doi: 10.1016/j.ecolind.2020.106150
Louati, D., Majdoub, R., Rigane, H., and Abida, H. (2018). Effects of irrigating with Saline Water on Soil Salinization (Eastern Tunisia). Arabian J. Sci. Eng. 43, 3793–3805. doi: 10.1007/s13369-018-3215-1
Lu, X. B., Liu, R., Liu, H. Q., Wang, T., Li, Z. H., Zhang, L. P., et al. (2022). Experimental evidence from Suaeda glauca explains why the species is not naturally distributed in non-saline soils. Sci. Total Environ. 817:153028. doi: 10.1016/j.scitotenv.2022.153028
Meng, Y., Weng, W. A., Chen, L., Hu, Q., Xing, Z. P., Wei, H. Y., et al. (2022). Effects of water-saving irrigation on grain yield and quality: A meta-analysis. Sci. Agric. Sin. 55, 2121–2134.
Merino-Martín, L., Courtauld, C., Commander, L., Turner, S., Lewandrowski, W., and Stevens, J. (2017). Interactions between seed functional traits and burial depth regulate germination and seedling emergence under water stress in species from semi-arid environments. J. Arid Environ. 147, 25–33. doi: 10.1016/j.jaridenv.2017.07.018
Mou, X. J., and Sun, Z. G. (2011). Effects of sediment burial disturbance on seedling emergence and growth of Suaeda salsa in the tidal wetlands of the Yellow River estuary. J. Exp. Mar. Biol. Ecol. 409, 99–106. doi: 10.1016/j.jembe.2011.08.006
Müller, F. L., Raitt, L. M., Cyster, L. F., Cupido, C. F., Samuels, M. I., Chimphango, S. B. M., et al. (2019). The effects of temperature, water availability and seed burial depth on seed germination and seedling establishment of Calobota sericea (Fabaceae). S. Afr. J. Bot. 121, 224–229. doi: 10.1016/j.sajb.2018.11.012
Noe, G. B., Boomer, K., Gillespie, J. L., Hupp, C. R., Martin-Alciati, M., Floro, K., et al. (2019). The effects of restored hydrologic connectivity on floodplain trapping vs. Release of phosphorus, nitrogen, and sediment along the Pocomoke River, Maryland USA. Ecol. Eng. 138, 334–352. doi: 10.1016/j.ecoleng.2019.08.002
Norton, A. J., Rayner, P. J., Wang, Y.-P., Parazoo, N. C., Baskaran, L., Briggs, P. R., et al. (2022). Hydrologic connectivity drives extremes and high variability in vegetation productivity across Australian arid and semi-arid ecosystems. Remote Sens Environ. 272:112937. doi: 10.1016/j.rse.2022.112937
Saunders, M. I., Brown, C. J., Foley, M. M., Febria, C. M., Albright, R., Mehling, M. G., et al. (2016). Human impacts on connectivity in marine and freshwater ecosystems assessed using graph theory: A review. Mar. Freshw. Res. 67, 277–290. doi: 10.1071/mf14358
Shao, X., Fang, Y., Jawitz, J. W., Yan, J., and Cui, B. S. (2019). River network connectivity and fish diversity. Sci. Total Environ. 689, 21–30. doi: 10.1016/j.scitotenv.2019.06.340
Soares, V. C., Scremin-Dias, E., Felipe, D. L., Damasceno-Junior, G. A., Pott, A., and Lima, L. B. (2021). Fire has little to no effect on the enhancement of germination, but buried seeds may survive in a Neotropical wetland. Flora 278:151801. doi: 10.1016/j.flora.2021.151801
Song, J., Shi, G. W., Gao, B., Fan, H., and Wang, B. S. (2011). Waterlogging and salinity effects on two Suaeda salsa populations. Physiol. Plant. 141, 343–351. doi: 10.1111/j.1399-3054.2011.01445.x
Sun, Z. G., Mou, X. J., Lin, G., Wang, L., Song, H., and Jiang, H. (2010). Effects of sediment burial disturbance on seedling survival and growthof Suaeda salsa in the tidal wetland of the Yellow River estuary. Plant Soil. 337, 457–468. doi: 10.1007/s11104-010-0542-8
Wang, F., Xu, Y. G., Wang, S., Shi, W. W., Liu, R. R., Feng, G., et al. (2015). Salinity affects production and salt tolerance of dimorphic seeds of Suaeda salsa. Plant Physiol. Biochem. 95, 41–48. doi: 10.1016/j.plaphy.2015.07.005
Wang, K. H., and Jiang, Y. W. (2007). Waterlogging tolerance of kentucky bluegrass cultivars. Hort Sci. 42, 386–390.
Wang, S., Fu, B. J., Piao, S. L., Lü, Y. H., Philippe, C., Feng, X. M., et al. (2015). Reduced sediment transport in the Yellow River due to anthropogenic changes. Nat. Geosci. 9, 38–41. doi: 10.1038/ngeo2602
Wu, J., Cui, N. X., and Cheng, S. P. (2013). Effects of sediment anoxia on growth and root respiratory metabolism of. Ecol. Eng. 53, 194–199. doi: 10.1016/j.ecoleng.2012.12.043
Xue, S., Jian, H., Yang, F., Liu, Q., and Yao, Q. (2022). Impact of water-sediment regulation on the concentration and transport of dissolved heavy metals in the middle and lower reaches of the Yellow River. Sci. Total Environ. 806:150535. doi: 10.1016/j.scitotenv.2021.150535
Yadav, S., Irfan, M., Ahmad, A., and Hayat, S. (2011). Causes of salinity and plant manifestations to salt stress: A review. J. Environ. Biol. 32, 667–685.
Yu, J. B., Wang, X. H., Ning, K., Li, Y. Z., Wu, H. F., Fu, Y. Q., et al. (2012). Effects of Salinity and Water Depth on Germination of Phragmites australis in Coastal Wetland of the Yellow River Delta. Clean Soil Air Water 40, 1154–1158. doi: 10.1002/clen.201100743
Zhang, C. Y., Chen, S. L., Li, P., and Liu, Q. L. (2022). Spatiotemporal dynamic remote sensing monitoring of typical wetland vegetation in the current Huanghe River estuary reserve. Acta Oceanol. Sin. 44, 125–136.
Zhang, S. R., Song, J., Wang, H., and Feng, G. (2010). Effect of salinity on seed germination, ion content and photosynthesis of cotyledons in halophytes or xerophyte growing in Central Asia. J. Plant Ecol. 3, 259–267. doi: 10.1093/jpe/rtq005
Keywords: hydrological connectivity blocking, seed germination, seed yield and vitality, Suaeda salsa, the Yellow River Delta
Citation: Wang XH, Zou YH, Zhu T, Guan B, Yang JS and Yu JB (2022) The effects of hydrological connectivity blocking on Suaeda salsa development in the Yellow River Delta, China. Front. Ecol. Evol. 10:1005677. doi: 10.3389/fevo.2022.1005677
Received: 28 July 2022; Accepted: 05 September 2022;
Published: 21 September 2022.
Edited by:
Chuanyu Gao, Northeast Institute of Geography and Agroecology (CAS), ChinaCopyright © 2022 Wang, Zou, Zhu, Guan, Yang and Yu. This is an open-access article distributed under the terms of the Creative Commons Attribution License (CC BY). The use, distribution or reproduction in other forums is permitted, provided the original author(s) and the copyright owner(s) are credited and that the original publication in this journal is cited, in accordance with accepted academic practice. No use, distribution or reproduction is permitted which does not comply with these terms.
*Correspondence: JunBao Yu, eXUuanVuYmFvQGdtYWlsLmNvbQ==