- 1College of Forestry and Landscape Architecture, South China Agricultural University, Guangzhou, China
- 2Guangdong Provincial Key Laboratory of Silviculture, Protection and Utilization, Guangdong Academy of Forestry, Guangzhou, China
- 3Chinese Forest Ecosystem Research Network Guangdong E’huangzhang National Field Observation and Research Station, Yangjiang, China
- 4South China Botanical Garden, Chinese Academy of Sciences, Guangzhou, China
Soil organic carbon (SOC) is a crucial component of the soil carbon pool that regulates fundamental soil properties and water status. In the global context of restoring vegetation, the soil carbon-water coupling relationship has gained attention. In particular, the regulatory mechanism of SOC on soil moisture requires further research. In this study, three typical forests in subtropical China were chosen as restoration sequences to investigate the changes in SOC and soil moisture during subtropical forest restoration and its regulation mechanisms: broadleaf-conifer mixed forest (EF), broad-leaved forest (MF), and old-growth forest (LF). The soil water content (35.71 ± 1.52%), maximum water holding capacity (47.74 ± 1.91%), capillary water holding capacity (43.92 ± 1.43%), and field water holding capacity (41.07 ± 1.65%) in LF were significantly higher than those in EF (p < 0.01). As forest restoration progressed, the amount of litter returning to the soil increased gradually, and the SOC content (0–100 cm) increased from 9.51 ± 1.42 g/kg (EF) to 15.60 ± 2.30 g/kg (LF). The SOC storage increased from 29.49 ± 3.59 to 42.62 ± 5.78 Mg/ha. On one hand, forest restoration led to a change in SOC content, which optimizes the soil structure and enhances soil porosity (path coefficient of 0.537, p < 0.01), further leading to a change in soil water content (path coefficient of 0.940, p < 0.01). On the other hand, the increase in SOC influenced the change in soil nutrient content, i.e., total nitrogen (TN) and total phosphorus (TP) (path coefficient of 0.842, p < 0.01). Changes in SOC and soil nutrients stimulated changes in the stoichiometric ratio, i.e., C:P and N:P (path coefficients of 0.988 and –0.968, respectively, p < 0.01), and the biological activity in soil changed appropriately, which eventually led to a change in soil water content (path coefficient of –0.257, p < 0.01). These results highlight the changes in SOC and soil water content (SWC), as well as the mechanism of SOC controlling SWC as a result of vegetation restoration, which is of tremendous importance for advancing our understanding of the eco-hydrological process of subtropical forest restoration.
Introduction
Forest ecosystems are one of the most important carbon pools and reservoirs in the terrestrial biosphere (Foley et al., 2007; Fahey et al., 2010; Nguyen et al., 2013). Forest soil carbon accounts for 51% of the total ecosystem carbon, and forest soil water storage contributes 73.39% of the total forest ecosystem water retention (Hughes et al., 2002; Wu et al., 2021). Changes in forest soil carbon and water stocks are strongly affected by climatic conditions, soil properties, and plant species (Setälä et al., 2016; Hessburg et al., 2019). At an ecosystem scale, soil carbon and hydrological processes are strongly connected (Sun et al., 2019). Consequently, the functions and processes of forest soil carbon sequestration and water conservation have garnered extensive attention worldwide in the context of widespread vegetation regeneration and increasingly severe climate change effects (DeLuca and Aplet, 2008; Maioli et al., 2021). It is crucial to investigate the carbon and water storage of forest soil and its coupling mechanism to elucidate the production, ecological function formation, and response mechanism of forest ecosystems in the context of vegetation and climate change.
Surface and subsurface biological processes within terrestrial ecosystems rely on soil organic carbon (SOC) as an essential nutrient and energy source (Zhang et al., 2022). In forest ecosystems, land- use changes resulting from natural vegetation restoration can significantly alter carbon storage and fixation (Zhang and Shangguan, 2016). Forest restoration influences the equilibrium between the carbon input and output processes from plant and microbial sources by altering the soil carbon pool (Jastrow et al., 2007; Zhang et al., 2021). According to Erskine et al. (2006), trees can add carbon to the soil, and this process can be affected by the tree species and the external environment. Different vegetation varieties, for instance, have distinct biomass and functional components, and thus distinct decomposition and transformation rates of soil carbon input (Xu et al., 2020b). The physical and chemical properties of soil rely on SOC as a key component (Deng et al., 2013). In a study conducted in northern China, Shao et al. (2019) found that microbial biomass and residues increased during forest restoration. Microorganisms can directly stimulate the creation of SOC (Liang et al., 2017).
Biophysical processes that sustain ecosystem functioning rely on soil moisture as a crucial link between material and energy exchange. It plays a significant role in biogeochemical cycles of ecosystems (Seneviratne et al., 2010; Zhang and Shangguan, 2016). Studies have observed a coupling relationship between soil carbon and water which can affect key soil ecological processes (Kerr and Ochsner, 2020). After the occurrence of severe compounded forest disturbance, the carbon sequestration capacity of the forest and water evaporation dropped dramatically in the forest (Brando et al., 2019). Franzluebbers (2002) hypothesized that changes in SOC content resulting from increased porosity is crucial in regulating water infiltration and the subsequent transfer and storage of water in soil. Soil moisture is not directly affected by SOC, but is mainly affected by soil structure and aggregate composition (Panagea et al., 2021). In a study on the vertical distribution of soil water, Wang et al. (2013) hypothesized that changes in soil porosity and structure caused by SOC would affect the variability of soil water, which would increase with soil depth. Numerous studies have presented the concept of carbon-water coupling. However, a majority of these studies have focused on the effect of soil moisture on soil SOC (Zhang et al., 2015; Singh et al., 2021; Zhao et al., 2021). Research on the mechanism by which organic carbon regulates soil moisture in the context of the natural restoration of forest ecosystems is still limited.
Xinfengjiang National Forest Park is located in Guangdong Province, China. Since 1984, when the Chinese government implemented quota-based cutting for trees, deforestation in this region has reduced, allowing the forest to be gradually restored naturally over the next three decades. In addition, the study site was located in humid subtropical China, where soil erosion is a persistent issue (Fang et al., 2017; Zheng et al., 2021). Therefore, it is an excellent location for monitoring changes in soil moisture during the restoration of subtropical forests (Huang et al., 2018). This region is comprised of forest types at different stages of restoration. We selected three typical forest stands, broadleaf-conifer mixed forest (EF), broad-leaved forest (MF), and old-growth forest (LF), to represent a sequence of subtropical forest restoration. In this study, we anticipated that the soil water content (SWC) in subtropical China would be altered as a result of long-term natural forest restoration, and that this change would be related to the change in SOC. We intended to answer the following questions: First, how does soil moisture change as forest restoration progresses? Second, how does the SOC change during forest restoration? Third, what is the mechanism by which SOC affects soil moisture during forest restoration?
Materials and methods
Site description
This study was conducted in Xinfengjiang National Forest Park (23°40′30′′−24°46′30′′N, 114°30′33′′−114°36′30′′E), China, which has a subtropical monsoon climate, with an annual average temperature of 21.2°C. The total area of the park is 4,479.47 hm2. The average annual precipitation is approximately 1,420 mm, primarily from April to September, with an average relative humidity of 76%. The predominant forms of vegetation are evergreen broad-leaved forests, evergreen coniferous forests, and broadleaf-conifer mixed forests, and 78% of the land is covered by forest. The geological foundation primarily consists of granite, basalt, and sand shale. The zonal soils of this region are predominantly loess and laterite, predominantly medium loam and heavy loam (Huang et al., 2018).
Six 50 m × 50 m plots with equal altitudes, slopes, and climates were created within the three main forest types in the study area (Figure 1). The EF was mostly distributed in the forest margins of the natural forest, and was rapidly undergoing restoration to a broad-leaved forest, and the dominant tree species were Pinus massoniana, Heptapleurum heptaphyllum, and Cunninghamia lanceolata. The main tree species in MF were Castanopsis chinensis, H. heptaphyllum, Machilus chinensis, and Schima superba. The LF is located in the central area with little disturbance and can be considered a well-developed climax community during the late-restoration period. Cinnamomum parthenoxylon, Neolitsea chui, and S. superba are the most abundant tree species. These three forest types constituted a restoration sequence. Under natural conditions, the direction of restoration is as follows: broadleaf-conifer mixed forest–broad-leaved forest–old-growth forest (Huang et al., 2018).
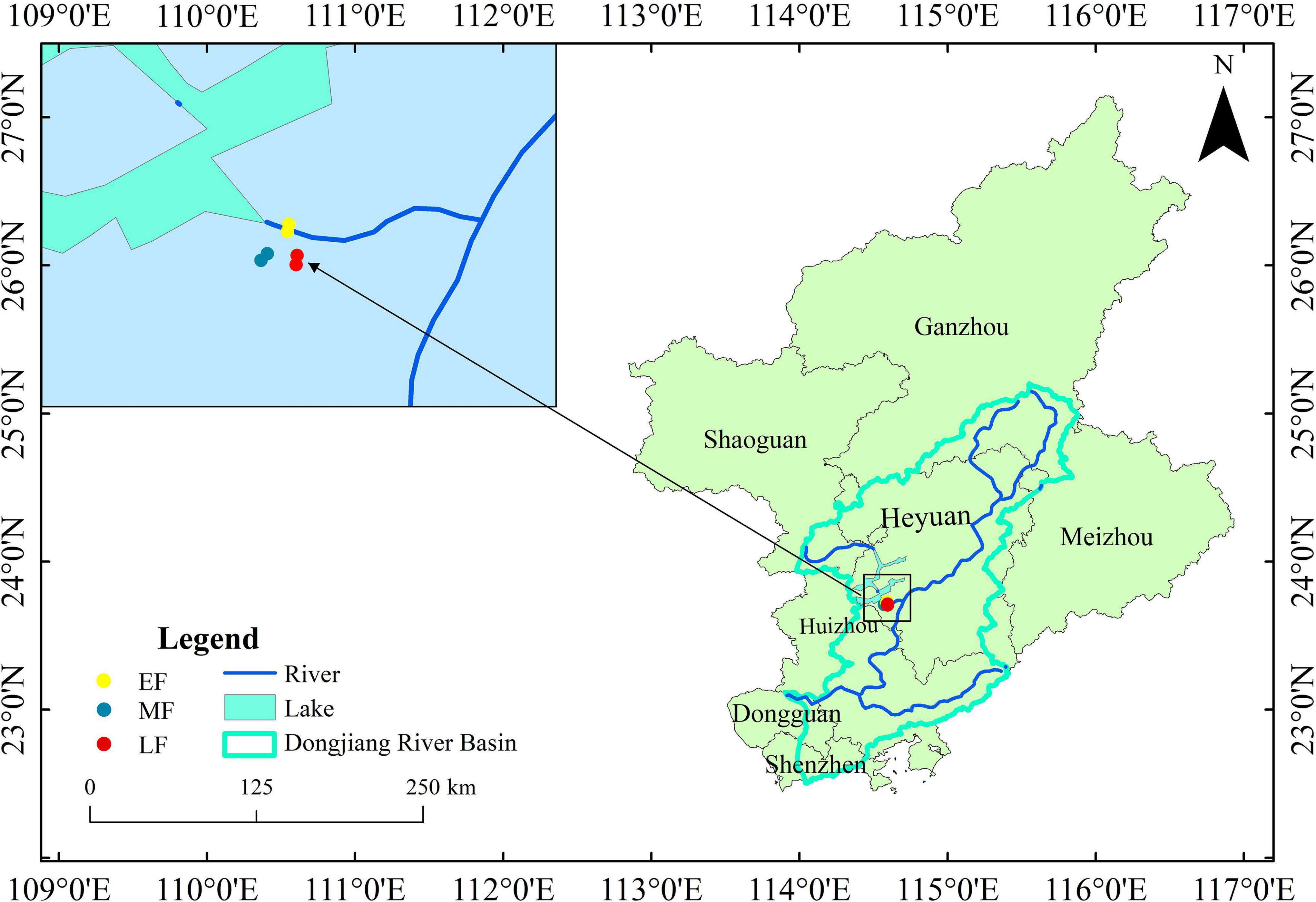
Figure 1. Location of the study area at the Xinfengjiang National Forest Park, Guangdong Province, China. EF, broadleaf-conifer mixed forest; MF, broad-leaved forest; LF, old-growth forest.
Soil sampling
Three 10 m × 10 m replicate sub-samples were randomly set up in each 50 m × 50 m plot. Two holes were taken from each quadrat, with a size of approximately 1.3 m × 1 m. Soil samples from 0–25, 25–50, 50–75, and 75–100 cm soil layers were used to determine soil physical properties [bulk density (BD), soil porosity, and soil moisture index] and chemical properties [SOM, SOC, total nitrogen (TN), and total phosphorus (TP)]. Undisturbed soil cores were collected using 100 cm3 stainless steel cutting rings for each soil layer (three replicates) (Zhang et al., 2019). A 3.5 cm diameter auger was used to collect disturbed soil samples at five points of the soil sampling site, including the four corners and the center of each plot, and the soil samples at five points of each layer were thoroughly mixed to form a composite sample with a weight of approximately 1 kg.
Measurements
Soil water content was determined using the drying method, and indicators such as BD and porosity were determined using the soil cutting ring soaking method (Xia et al., 2017). Before sampling, the weight of a 100 cm3 empty stainless-steel cutting ring was recorded as m0. After sampling, the weight of the cutting ring containing the fresh soil sample (m1) and the weight of the cutting ring after soaking in water with unmoved soil for 12–14 h (m2) were weighed. The cutting ring containing soil samples were drained for 2 h (m3) and 72 h (m4), respectively. The specific formulae used are as follows:
Where SWC is the soil water content (%), md is the dry soil weight (g), MWHC is the maximum water holding capacity (%), CWHC is the capillary capacity (%), and FWC is the field capacity (%).
Prior to analysis, a mixed soil sample from each soil layer (removing plant roots, debris, and gravel) was passed through a 0.25 mm mesh screen to determine soil organic matter (SOM), TN, and TP (Zhao et al., 2022). SOM content was determined by oil bath-K2CrO7 titration (Wang et al., 2009). The conversion of SOM to SOC was achieved using the reciprocal of the van Bemmelen factor (1.724) (Bierer et al., 2021). TN content was determined using the semi-micro Kjeldahl method (Shi et al., 2013). TP content was determined via colorimetry following digestion with perchloric acid and sulfuric acid (Zhang and Shangguan, 2018).
Statistical analysis
We calculated the descriptive statistical parameters of the collected data, i.e., the mean and standard error. All procedures were performed using Excel 2010 and IBM SPSS Statistics 22.0. Before performing statistical data analysis, we examined the normality of the data using the Kolmogorov–Smirnov test (p = 0.05). One-way ANOVA was performed to assess the variations in soil properties between the different vegetation types and soil layers. When the F value was significant (p < 0.05), Duncan’s test was performed to compare the differences in soil variables. Principal Component Analysis (PCA) was used to extract the variables (soil porosity PCA1, soil chemical properties PCA1, and soil chemical properties PCA2) formed by soil bulk density, porosity, soil nutrients, and stoichiometric ratio indices, according to the weight of each factor. Based on the results of the principal component analysis’s dimensionality reduction, the path analysis method was utilized to explore how soil SOC directly or indirectly affects soil properties to further affect soil SWC. All data were plotted using Origin 2021.
Results
Changes in soil moisture with forest restoration
With increasing soil depth, the soil water retention capacity of each forest gradually decreased (Figure 2). SWC is an important index of soil moisture conditions that can reflect the water holding and water supply capacity of the soil and influence nutrient movement in the soil (Zhao et al., 2017). Across the 0–100 cm layer in each forest, SWC demonstrated an increasing trend during forest restoration (Figure 2A), similar to LF (35.71 ± 1.52%) > MF (26.27 ± 1.90%) > EF (17.28 ± 0.91%). With the recovery of the forest, the MWHC (47.74 ± 1.91%) of LF was significantly higher than that of the other forests (32.63 ± 1.39% in EF and 36.44 ± 1.80% in MF) at an average depth of 0–100 cm (Figure 2B). When the average value was taken at a depth of 0–100 cm, the soil CWHC increased from 25.71 ± 1.15% (EF) to 43.92 ± 1.43% (LF) (Figure 2C). The FWC is considered to have the highest soil water content which can be stably maintained. The content of FWC at a depth of 0–100 cm in the forest was LF (41.07 ± 1.65%) > MF (31.40 ± 1.87%) > EF (24.62 ± 1.11%) (Figure 2D).
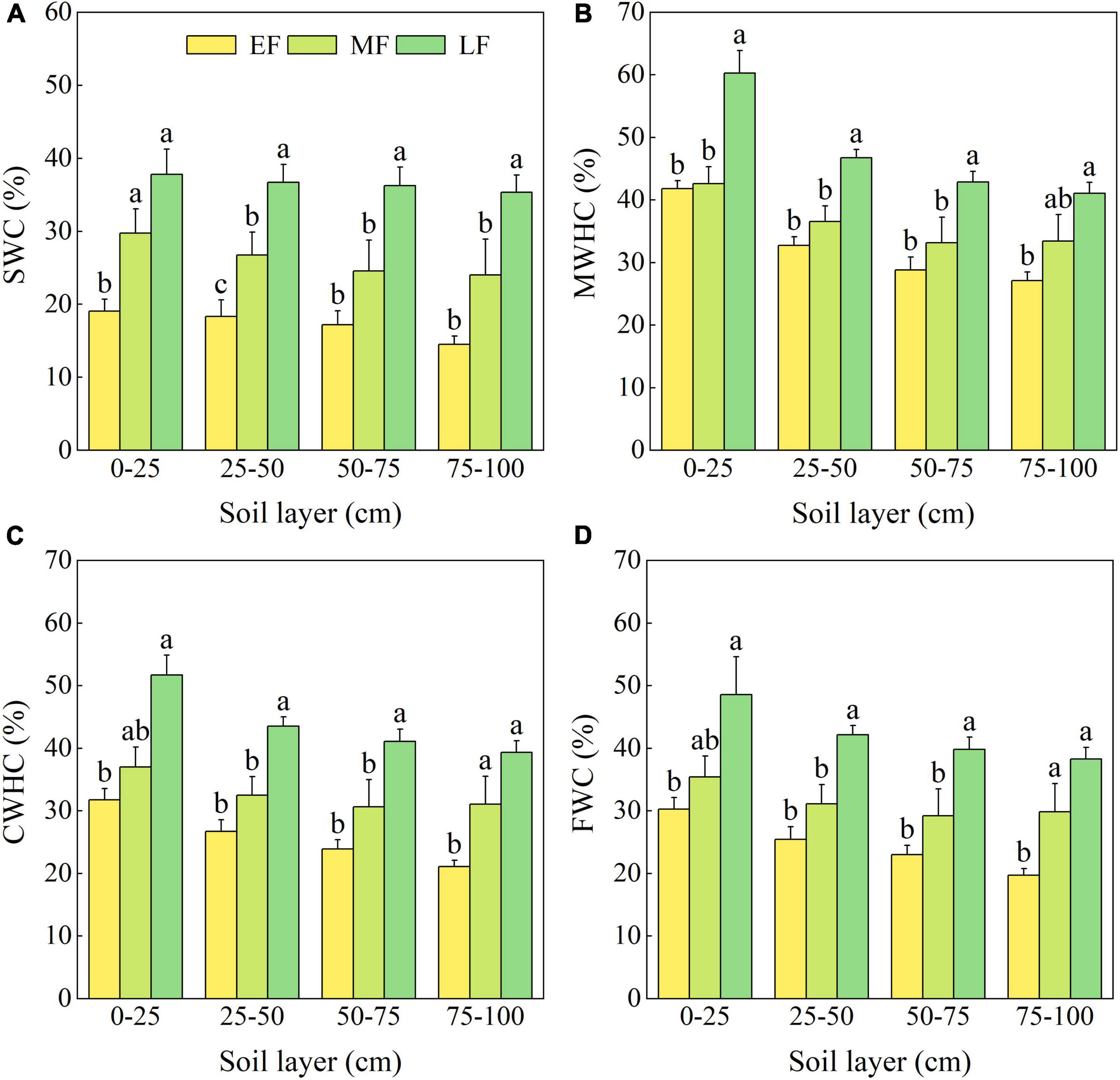
Figure 2. Changes in soil SWC (A), MWHC (B), CWHC (C), and FWC (D) in the 0–100 cm soil layer of different forests. Values are the means ± SE (n = 6). Lowercase letters above the columns represent statistically significant differences among stand types for the same soil layer (Duncan’s tests, p < 0.05). SWC, soil water content; MWHC, maximum soil water holding capacity; CWHC, capillary water holding capacity; FWC, field capacity; EF, broadleaf–conifer mixed forest; MF, broad-leaved forest; LF, old-growth forest.
Changes in soil organic carbon and organic carbon storage with forest restoration
A significant surface enrichment of the soil profile was observed in the SOC content of the three forests (Figure 3A). As the restoration progressed, the SOC content in the topsoil (0–25 cm) in LF was significantly higher than that in EF and MF with a value of 32.70 ± 3.54 g/kg (p < 0.01), and the soil organic carbon storage (SOCS) of the 0–25 cm layer in LF was highest (81.69 ± 12.62 Mg/ha) among three forests. The SOC content between the 0–100 cm depth in three forests was in the order LF (15.60 ± 2.30 g/kg) > MF (10.27 ± 1.40 g/kg) > EF (9.51 ± 1.42 g/kg), and the value of LF was significantly higher than those of EF and MF (p < 0.05). The change in SOCS content (Figure 3B) showed the same pattern as the change in SOC content, which increased from 29.49 ± 3.59 Mg/ha (EF) to 42.62 ± 5.78 Mg/ha (LF).
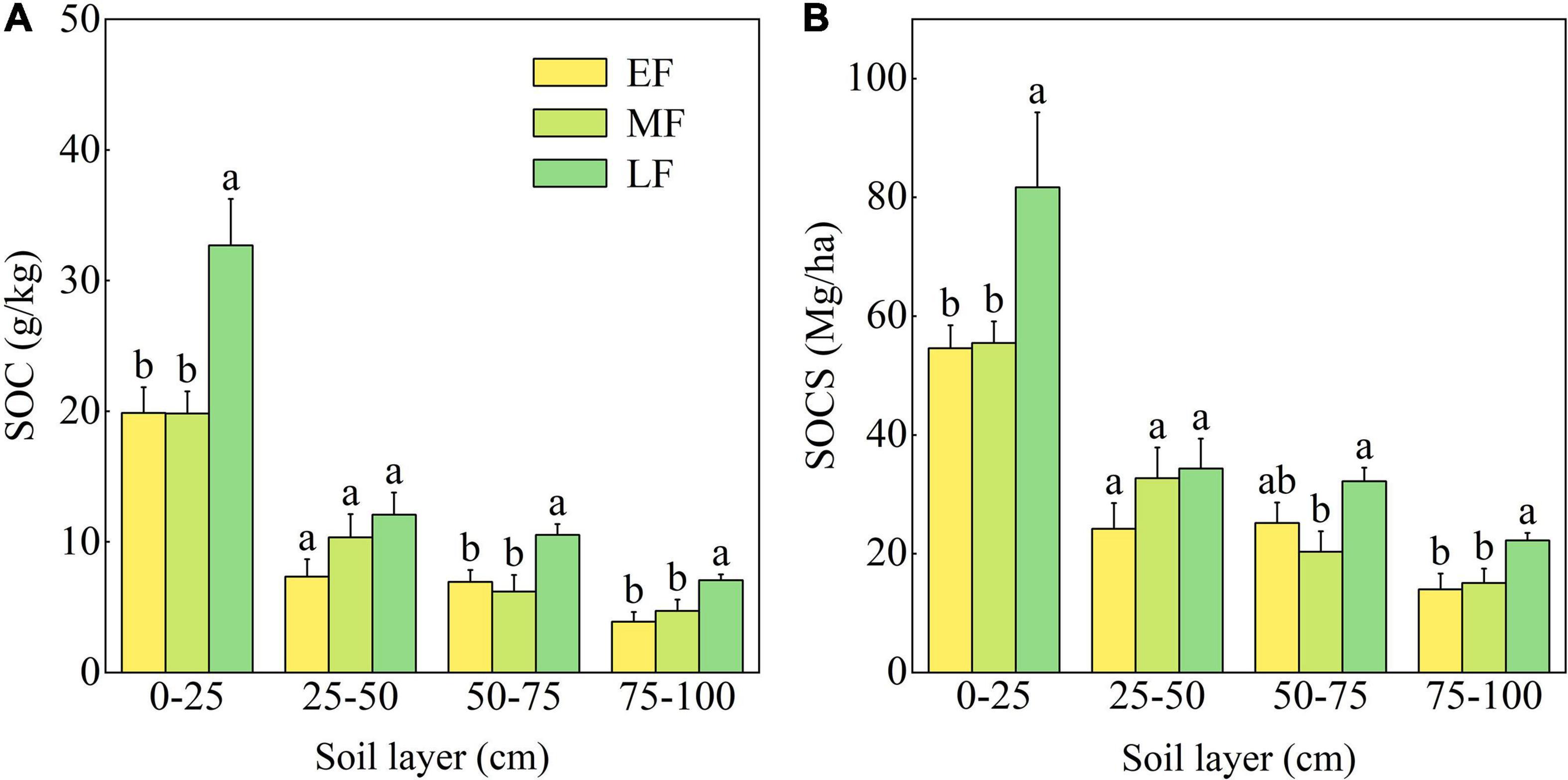
Figure 3. The content of soil organic carbon (SOC) (A) and SOCS (B) in the 0–100 cm soil layer of different forests. Values are the means ± SE (n = 6). Lowercase letters above the columns represent statistically significant differences among stand types for the same soil layer (Duncan’s tests, p < 0.05). SOC, soil organic carbon; SOCS, soil organic carbon storage; EF, broadleaf–conifer mixed forest; MF, broad-leaved forest; LF, old-growth forest.
The coupling relationship between soil moisture content and soil physical and chemical properties
The results demonstrated a relationship between SWC and soil physio-chemical properties at each restoration stage in the 0–100 cm soil layer (Figure 4). Positive correlations were found between SWC and CP (0.943), TSP (0.906), SOC (0.385), TN (0.499), and TP (0.825) (p < 0.01), whereas SWC had a negative effect on BD (–0.655), C:P (–0.310), and N:P (–0.373) (p < 0.01). There was a negative correlation between soil SWC and C:N (–0.044) in the 0–100 cm soil layer during natural forest restoration, although this was not significant (p > 0.05). The soil physicochemical properties affected the coupling of soil SOC and SWC (Table 1). Except for C:N, which had no significant effect on soil SWC, the other indicators showed significant effects on soil SOC and SWC (p < 0.05).
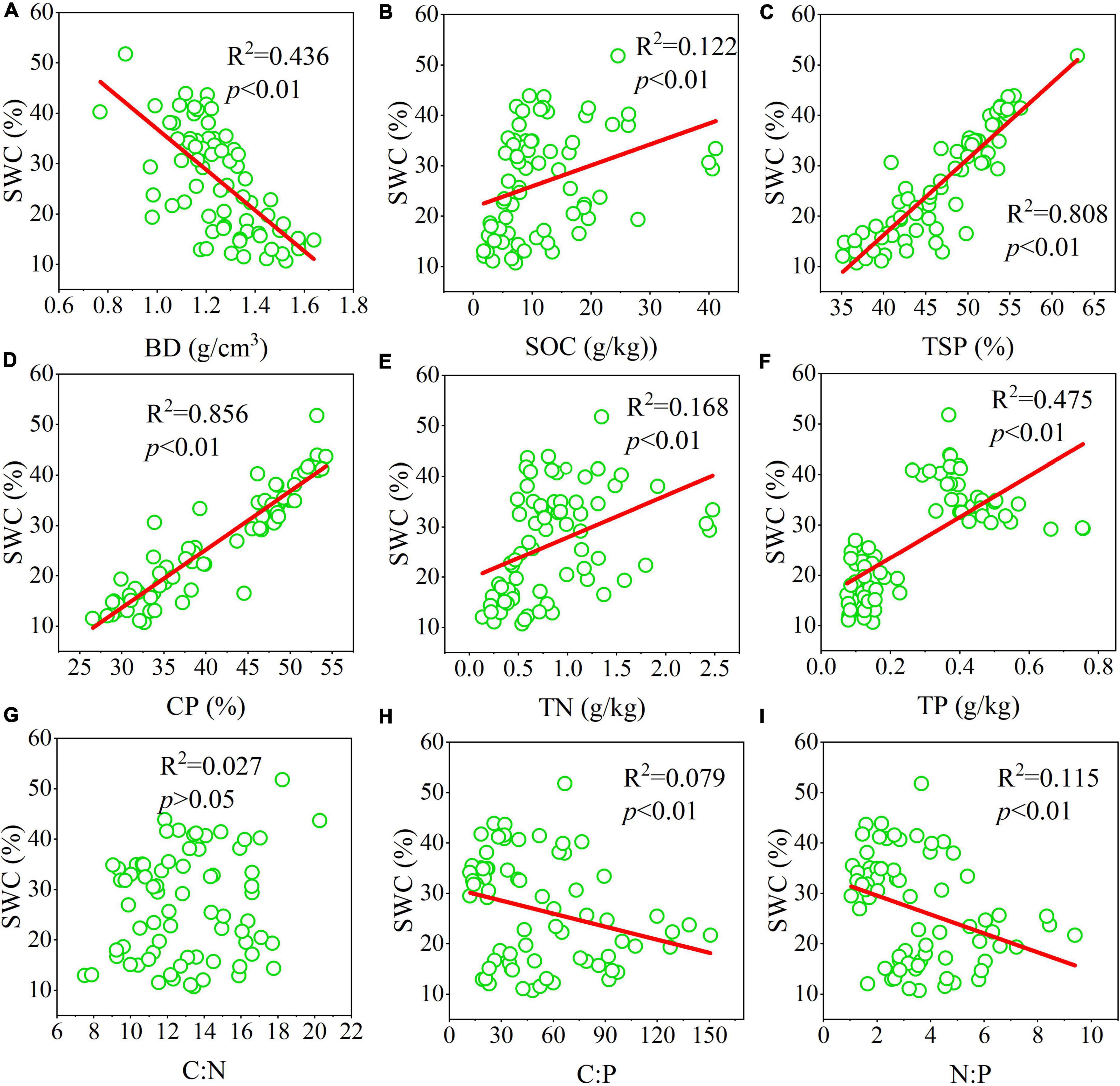
Figure 4. The relationship between SWC and soil physico-chemical properties i.e., BD (A), SOC (B), TSP (C), CP (D), TN (E), TP (F), C:N (G), C:P (H), and N:P (I). BD, bulk density; SOC, soil organic carbon; TSP, total soil porosity; CP, capillary porosity; TN, soil total nitrogen; TP, soil total phosphorus; C:N, SOC:TN; C:P, SOC:TP; N:P, TN:TP.

Table 1. Pearson’s correlation between soil organic carbon (SOC), soil water content (SWC) and soil properties in 0–100 cm soil layer.
The principal component of the soil porosity index (soil porosity PCA) was extracted according to a characteristic value greater than one, including the soil bulk density, capillary porosity (CP), and total porosity. The cumulative variance contribution rate was 82.82% (Appendix 1). At the same time, the principal components of soil chemical properties, soil chemical properties PCA1 (the highest scoring indicators were C:P and N:P), and soil chemical properties PCA2 (the highest scoring indicators were TN and TP) were extracted, and the cumulative variance contribution rate was found to be 95.05%.
Path analysis was used to understand the influence mechanism of SOC on soil SWC (Figure 5). SOC affected soil porosity PCA1 (standardized path coefficient value was 0.537 > 0, p < 0.01), which in turn affected SWC (standardized path coefficient value was 0.940 > 0, p < 0.01). SOC also affected soil SWC by affecting soil chemical properties PCA1 (standardized path coefficient value of 0.988 > 0, p < 0.01) and PCA2 (standardized path coefficient value of 0.842 > 0, p < 0.01). SOC further affected soil chemical property PCA1 by affecting soil chemical property PCA2 (standardized path coefficient value of –0.968 < 0, p < 0.01). The change in the chemical properties of PCA1 ultimately affected the change in soil SWC (the value of the standardized path coefficient was –0.257 < 0, p < 0.01).
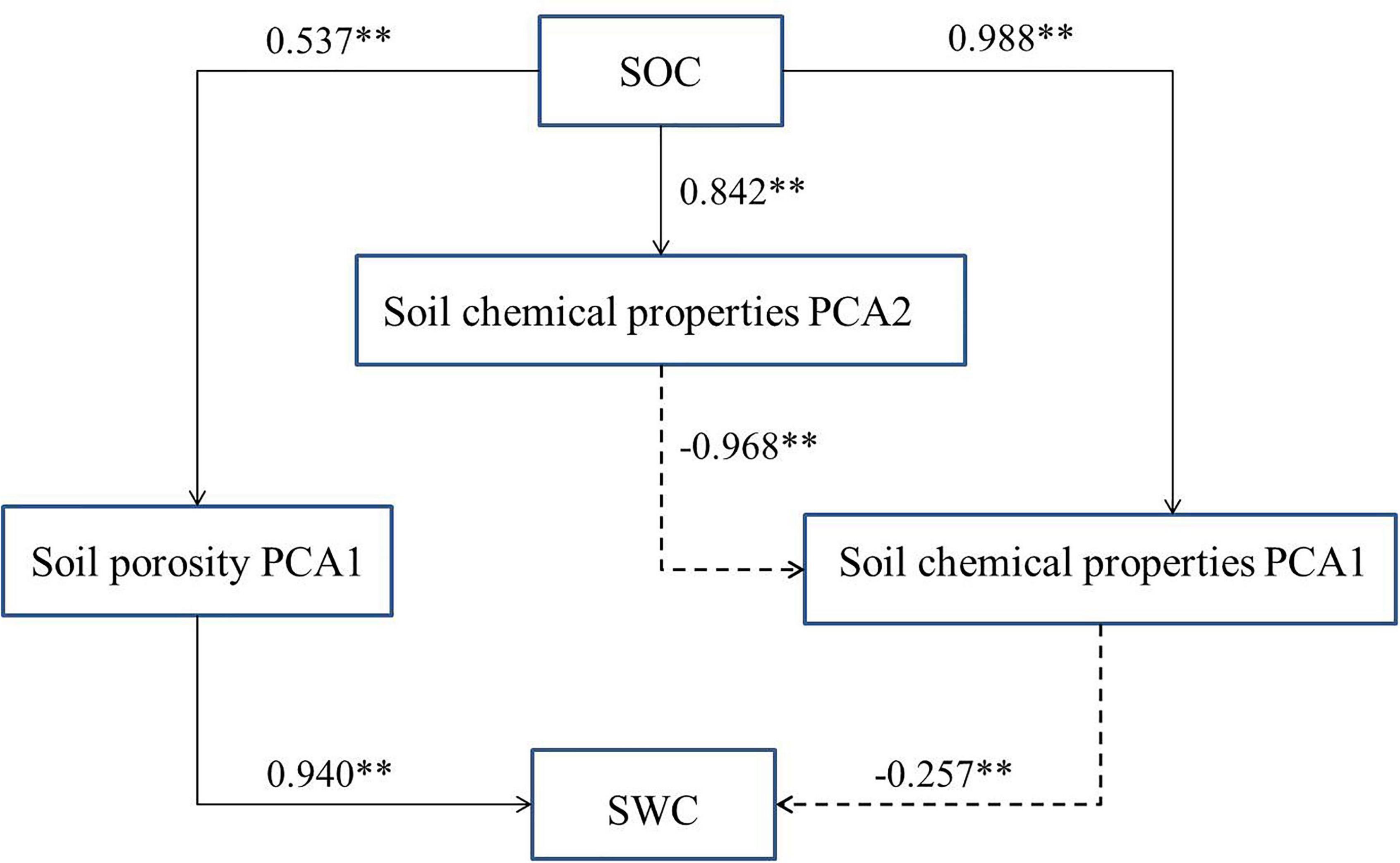
Figure 5. The path analysis of the influence mechanism of soil organic carbon (SOC) on soil SWC. The numbers adjacent to the arrows are standardized path coefficients and * signs indicate significance (**p < 0.01). Positive standardized path coefficients are indicated by continue arrows, whereas negative standardized path coefficients are indicated by dashed arrows.
Discussion
Changes in soil moisture during forest restoration
Forest ecosystems significantly contribute to the preservation of water and soil (Niu et al., 2012; Li et al., 2021). Water storage capacity is a crucial indicator for determining the water regulation of forest soils. Owing to the limited water absorption by the forest canopy and litter layer, the understory soil is primarily responsible for forest water retention, storage, and transmission (Wang et al., 2021; Francis et al., 2022). Therefore, soil is the most essential reservoir of a forest ecosystem, and its storage capacity directly affects the ability of the forest ecosystem to regulate precipitation (Zhang et al., 2011). With the restoration process, the composition and structure of the above-ground vegetation become more complex, the characteristics of soil hydrologic and physical properties improve to a certain extent, and the water storage capacity of soil may gradually increase (Yinglan et al., 2019). Numerous studies have demonstrated a relationship between forest restoration and soil (Wu et al., 2017; Pereira et al., 2021; Qiu et al., 2022). Increasing canopy interception in the LF reduces the impact of high-intensity rainfall on the soil and reduces surface runoff (Zhao et al., 2022). Vegetation restoration changes soil structure by promoting root growth, and roots secrete organic acids, which further reduces soil BD, improves soil anti-scouribility, soil shearing strength, and soil water infiltration capacity, thus further improving soil water storage capacity (Li et al., 2015; Wu et al., 2016). In addition, forest restoration changes plant residues, enhances the soil microclimate, and promotes soil bioactivity. Under the effects of microbial and SOM breakdown, soil particles fragment, thereby reducing BD and enhancing SWC and water retention capacity (Zhang et al., 2018b; Pastore et al., 2020).
Changes in organic carbon during forest restoration
In this study, the content of SOC in LF was higher than that in EF, which may be due to the lower accumulation of SOC in the coniferous forest than that in the broad-leaved forest (Jia et al., 2005). Soil properties may be affected by plant cover (Srivastava et al., 2020; Wang et al., 2020). SOC is primarily derived from tree litter and roots in forest environments, and both litter quantity and quality and living roots are influenced by plant characteristics (De Deyn et al., 2008; Olsson et al., 2019). Evergreen trees usually retain their leaves throughout the year, and a permanently shaded evergreen forest canopy facilitates SOC accumulation because the detrimental effects of high temperature and precipitation on SOC retention are reduced (Olsson et al., 2019). In addition, forward regeneration of the forest increased the amount of returning litter (Xu et al., 2020a). Moreover, the priming effect of broadleaf litter is the most significant. Thus, the decomposition rate of broad-leaf litter and the associated organic matter mineralization rate are faster than those of coniferous litter, which is conducive to SOC addition (Hou et al., 2020). The SOC formed by litter and root secretions and their decomposition by microorganisms first enters the top-soil, which causes the SOC content of forestland soil to have a “surface aggregation” (Yang et al., 2021). It has been demonstrated that root biomass is significantly and positively correlated with SOC (Berhongaray et al., 2019; Liu et al., 2019). As broad-leaf species generally have larger and deeper root systems, they also have higher underground biomass than conifers (Li et al., 2020). Root mass and turnover rate are greater in evergreen broadleaf forests (Ding et al., 2015). Therefore, in LF, evergreen broad-leaved roots drive an increase in SOC input.
The controlling mechanism of organic carbon on soil moisture during forest restoration
The primary and most direct effect of SOC in enhancing the physical qualities of soil is to improve the soil structure and stimulate the formation of agglomerated structures (Wang et al., 2022). An increase in SOC promotes the growth of roots and the activity of soil microorganisms, and the chemical conditions provided by the secretion of organic acids by roots and microorganisms promote the decomposition of soil particles, leading to a reduction in soil BD (Marschner et al., 2011; Pastore et al., 2020). The input of SOC promoted root development, which provides soil porosity through decomposition. Coarse roots can significantly increase soil porosity by increasing inter-aggregate pore space (Bodner et al., 2014; Naveed et al., 2014; Hao et al., 2020). The increase in soil porosity further improved the water-holding capacity and water-storage capacity of the soil. A significant positive association was found between the soil TN, TP, and SOC. This is due to the fact that an increase in SOC makes additional carbon sources accessible to the soil microorganisms (Kramer and Gleixner, 2008). Simultaneously, the energy required for microbial activities is fully guaranteed, which stimulates the decomposition of microorganisms, thereby leading to an increase in the soil TN and TP content (Fontaine et al., 2007; Zhang et al., 2018a). Additionally, soil C:P and N:P stoichiometries were significantly associated with SOC (Table 1). Consistent with the findings of Tian et al. (2018), the change in soil nutrient stoichiometry also depended, to a certain extent, on SOC dynamics. Changes in SOC and microbial biomass caused by litter input can further alter the stoichiometry of soil nutrients (Aponte et al., 2010; Tian et al., 2018). Therefore, changes in SOC content and soil nutrient concentrations during forest restoration can result in alterations in soil biological activity (Zhang et al., 2006). The increase in SOC and nutrient supply improves soil pore connectivity and enables the soil to have better water holding and storage capacities in the LF (Naveed et al., 2014).
Conclusion
Our findings revealed that forest restoration in lower subtropical China had a substantial effect on soil moisture, which was a result of alterations in the SOC content. In the natural forest restoration process, SOC was strongly linked with SWC, and soil BD, CP, TSP, TN, TP, C:P, and N:P were the major elements that might influence the coupling interaction between SWC and SOC in the forest restoration stage. With the progression of forest restoration, the amount of litter returned increased, SOC increased steadily, and the surface accumulation phenomenon became evident. Changes in soil physical structure and soil nutrients caused by the increase in SOC intake had additional effects on soil water content and water-holding capacity. By examining soil moisture and soil physicochemical properties during forest restoration, our study provided new evidence on the improvement mechanism of soil moisture during subtropical forest restoration, which is of great significance for evaluating the eco-hydrological function of subtropical forests in southern China and optimizing forest management programs.
Data availability statement
The original contributions presented in the study are included in the article/supplementary material, further inquiries can be directed to the corresponding authors.
Author contributions
XZ, WZ, and YF: data processing and writing. QM and YS: data processing and data collection. BN: language correction. CQ: site maintenance and data collection. XG: conceptualization and data collection. XL: experimental design and review. All authors contributed to the article and approved the submitted version.
Funding
This work was supported by the Forestry Science and Technology Innovation Project of Guangdong Province, China (2021KJCX003 and 2022KJCX015) and Forestry Science and Technology Innovation Platform Project of Guangdong Province, China (2022-KYXM-09). Science and Technology Projects in Guangzhou (202201010640), Project for Distinctive Innovation of Ordinary Universities of Guangdong Province (2022KTSCX016), and Operation Subsidy Program of Technology Innovation Platform of National Forestry and Grassland Administration (2021132084 and 2021132085).
Acknowledgments
We gratefully acknowledge the support of the Guangdong Dongjiangyuan Forest Ecosystem National Positioning Observation and Research Station, and Guangdong Nanling Forest Ecosystem National Positioning Observation and Research Station.
Conflict of interest
The authors declare that the research was conducted in the absence of any commercial or financial relationships that could be construed as a potential conflict of interest.
Publisher’s note
All claims expressed in this article are solely those of the authors and do not necessarily represent those of their affiliated organizations, or those of the publisher, the editors and the reviewers. Any product that may be evaluated in this article, or claim that may be made by its manufacturer, is not guaranteed or endorsed by the publisher.
References
Aponte, C., Marañón, T., and García, L. V. (2010). Microbial C, N and P in soils of Mediterranean oak forests: influence of season, canopy cover and soil depth. Biogeochemistry 101, 77–92. doi: 10.1007/s10533-010-9418-5
Berhongaray, G., Cotrufo, F. M., Janssens, I. A., and Ceulemans, R. (2019). Below-ground carbon inputs contribute more than above-ground inputs to soil carbon accrual in a bioenergy poplar plantation. Plant Soil 434, 363–378. doi: 10.1007/s11104-018-3850-z
Bierer, A. M., Leytem, A. B., Rogers, C. W., and Dungan, R. S. (2021). Evaluation of a microplate spectrophotometer for soil organic carbon determination in south-central Idaho. Soil Sci. Soc. Am. J. 85, 438–451. doi: 10.1002/saj2.20165
Bodner, G., Leitner, D., and Kaul, H.-P. (2014). Coarse and fine root plants affect pore size distributions differently. Plant Soil 380, 133–151. doi: 10.1007/s11104-014-2079-8
Brando, P. M., Silvério, D., Maracahipes Santos, L., Oliveira Santos, C., Levick, S. R., Coe, M. T., et al. (2019). Prolonged tropical forest degradation due to compounding disturbances: implications for CO2 and H2O fluxes. Glob. Change Biol. 25, 2855–2868. doi: 10.1111/gcb.14659
De Deyn, G. B., Cornelissen, J. H. C., and Bardgett, R. D. (2008). Plant functional traits and soil carbon sequestration in contrasting biomes. Ecol. Lett. 11, 516–531. doi: 10.1111/j.1461-0248.2008.01164.x
DeLuca, T. H., and Aplet, G. H. (2008). Charcoal and carbon storage in forest soils of the Rocky Mountain West. Front. Ecol. Environ. 6:18–24. doi: 10.1890/070070
Deng, L., Wang, K., Chen, M., Shangguan, Z., and Sweeney, S. (2013). Soil organic carbon storage capacity positively related to forest succession on the Loess Plateau, China. Catena 110, 1–7. doi: 10.1016/j.catena.2013.06.016
Ding, J., Zhang, Y., Wang, M., Sun, X., Cong, J., Deng, Y., et al. (2015). Soil organic matter quantity and quality shape microbial community compositions of subtropical broadleaved forests. Mol. Ecol. 24, 5175–5185. doi: 10.1111/mec.13384
Erskine, P. D., Lamb, D., and Bristow, M. (2006). Tree species diversity and ecosystem function: can tropical multi-species plantations generate greater productivity? For. Ecol. Manage. 233, 205–210. doi: 10.1016/j.foreco.2006.05.013
Fahey, T. J., Woodbury, P. B., Battles, J. J., Goodale, C. L., Hamburg, S. P., Ollinger, S. V., et al. (2010). Forest carbon storage: ecology, management, and policy. Front. Ecol. Environ. 8:245–252. doi: 10.1890/080169
Fang, N., Wang, L., and Shi, Z. (2017). Runoff and soil erosion of field plots in a subtropical mountainous region of China. J. Hydrol. 552, 387–395. doi: 10.1016/j.jhydrol.2017.06.048
Foley, J. A., Asner, G. P., Costa, M. H., Coe, M. T., DeFries, R., Gibbs, H. K., et al. (2007). Amazonia revealed: forest degradation and loss of ecosystem goods and services in the Amazon Basin. Front. Ecol. Environ. 5:25–32.
Fontaine, S., Barot, S., Barré, P., Bdioui, N., Mary, B., and Rumpel, C. (2007). Stability of organic carbon in deep soil layers controlled by fresh carbon supply. Nature 450, 277–280. doi: 10.1038/nature06275
Francis, J. R., Wuddivira, M. N., and Farrick, K. K. (2022). Exotic tropical pine forest impacts on rainfall interception: canopy, understory, and litter. J. Hydrol. 609:127765. doi: 10.1016/j.jhydrol.2022.127765
Franzluebbers, A. J. (2002). Water infiltration and soil structure related to organic matter and its stratification with depth. Soil Tillage Res. 66, 197–205. doi: 10.1016/S0167-1987(02)00027-2
Hao, H., Wei, Y., Cao, D., Guo, Z., and Shi, Z. (2020). Vegetation restoration and fine roots promote soil infiltrability in heavy-textured soils. Soil Tillage Res. 198:104542. doi: 10.1016/j.still.2019.104542
Hessburg, P. F., Miller, C. L., Parks, S. A., Povak, N. A., Taylor, A. H., Higuera, P. E., et al. (2019). Climate, environment, and disturbance history govern resilience of western North American forests. Front. Ecol. Evol. 7:239. doi: 10.3389/fevo.2019.00239
Hou, G., Delang, C. O., Lu, X., and Gao, L. (2020). Grouping tree species to estimate afforestation-driven soil organic carbon sequestration. Plant Soil 455, 507–518. doi: 10.1007/s11104-020-04685-z
Huang, F., Zhang, W., Gan, X., Huang, Y., Guo, Y., and Wen, X. (2018). Changes in vegetation and soil properties during recovery of a subtropical forest in South China. J. Mt. Sci. 15, 46–58. doi: 10.1007/s11629-017-4541-6
Hughes, R. F., Kauffman, J. B., and Cummings, D. L. (2002). Dynamics of aboveground and soil carbon and nitrogen stocks and cycling of available nitrogen along a land-use gradient in Rondônia, Brazil. Ecosystems 5, 244–259. doi: 10.1007/s10021-001-0069-1
Jastrow, J. D., Amonette, J. E., and Bailey, V. L. (2007). Mechanisms controlling soil carbon turnover and their potential application for enhancing carbon sequestration. Clim. Change 80, 5–23. doi: 10.1007/s10584-006-9178-3
Jia, G., Cao, J., Wang, C., and Wang, G. (2005). Microbial biomass and nutrients in soil at the different stages of secondary forest succession in Ziwulin, Northwest China. For. Ecol. Manage. 217, 117–125. doi: 10.1016/j.foreco.2005.05.055
Kerr, D. D., and Ochsner, T. E. (2020). Soil organic carbon more strongly related to soil moisture than soil temperature in temperate grasslands. Soil Sci. Soc. Am. J. 84, 587–596. doi: 10.1002/saj2.20018
Kramer, C., and Gleixner, G. (2008). Soil organic matter in soil depth profiles: Distinct carbon preferences of microbial groups during carbon transformation. Soil Biol. Biochem. 40, 425–433. doi: 10.1016/j.soilbio.2007.09.016
Li, F., McCormack, M. L., Liu, X., Hu, H., Feng, D., and Bao, W. (2020). Vertical fine-root distributions in five subalpine forest types shifts with soil properties across environmental gradients. Plant Soil 456, 129–143. doi: 10.1007/s11104-020-04706-x
Li, M., Liang, D., Xia, J., Song, J., Cheng, D., Wu, J., et al. (2021). Evaluation of water conservation function of Danjiang River Basin in Qinling Mountains, China based on InVEST model. J. Environ. Manage. 286:112212. doi: 10.1016/j.jenvman.2021.112212
Li, Q., Liu, G., Zhang, Z., Tuo, D., and Xu, M. (2015). Effect of root architecture on structural stability and erodibility of topsoils during concentrated flow in hilly Loess Plateau. Chin. Geogr. Sci. 25, 757–764. doi: 10.1007/s11769-014-0723-0
Liang, C., Schimel, J. P., and Jastrow, J. D. (2017). The importance of anabolism in microbial control over soil carbon storage. Nat. Microbiol. 2:17105. doi: 10.1038/nmicrobiol.2017.105
Liu, X., Lin, T., Vadeboncoeur, M. A., Yang, Z., Chen, S., Xiong, D., et al. (2019). Root litter inputs exert greater influence over soil C than does aboveground litter in a subtropical natural forest. Plant Soil 444, 489–499. doi: 10.1007/s11104-019-04294-5
Maioli, V., Monteiro, L. M., Tubenchlak, F., Pepe, I. S., de Carvalho, Y. B., Gomes, F. D., et al. (2021). Local perception in forest landscape restoration planning: a case study from the Brazilian Atlantic forest. Front. Ecol. Evol. 9:612789. doi: 10.3389/fevo.2021.612789
Marschner, P., Crowley, D., and Rengel, Z. (2011). Rhizosphere interactions between microorganisms and plants govern iron and phosphorus acquisition along the root axis-model and research methods. Soil Biol. Biochem. 43, 883–894. doi: 10.1016/j.soilbio.2011.01.005
Naveed, M., Moldrup, P., Vogel, H. J., Lamandé, M., Wildenschild, D., Tuller, M., et al. (2014). Impact of long-term fertilization practice on soil structure evolution. Geoderma 217–218, 181–189. doi: 10.1016/j.geoderma.2013.12.001
Nguyen, T. T., Pham, V. D., and Tenhunen, J. (2013). Linking regional land use and payments for forest hydrological services: a case study of Hoa Binh Reservoir in Vietnam. Land Use Pol. 33, 130–140. doi: 10.1016/j.landusepol.2012.12.015
Niu, X., Wang, B., Liu, S., Liu, C., Wei, W., and Kauppi, P. E. (2012). Economical assessment of forest ecosystem services in China: characteristics and implications. Ecol. Complex. 11, 1–11. doi: 10.1016/j.ecocom.2012.01.001
Olsson, B. A., Guedes, B. S., Dahlin, A. S., and Hyvönen, R. (2019). Predicted long-term effects of decomposition of leaf litter from Pinus taeda, Eucalyptus cloeziana and deciduous miombo trees on soil carbon stocks. Glob. Ecol. Conserv. 17:e00587. doi: 10.1016/j.gecco.2019.e00587
Panagea, I. S., Berti, A., Čermak, P., Diels, J., Elsen, A., Kusá, H., et al. (2021). Soil water retention as affected by management induced changes of soil organic carbon: analysis of long-term experiments in Europe. Land 10:1362. doi: 10.3390/land10121362
Pastore, G., Kaiser, K., Kernchen, S., and Spohn, M. (2020). Microbial release of apatite- and goethite-bound phosphate in acidic forest soils. Geoderma 370:114360. doi: 10.1016/j.geoderma.2020.114360
Pereira, L. C., Balbinot, L., Matus, G. N., Dias, H. C. T., and Tonello, K. C. (2021). Aspects of forest restoration and hydrology: linking passive restoration and soil-water recovery in Brazilian Cerrado. J. For. Res. 32, 2301–2311. doi: 10.1007/s11676-021-01301-3
Qiu, D., Xu, R., Wu, C., Mu, X., Zhao, G., and Gao, P. (2022). Vegetation restoration improves soil hydrological properties by regulating soil physicochemical properties in the Loess Plateau, China. J. Hydrol. 609:127730. doi: 10.1016/j.jhydrol.2022.127730
Seneviratne, S. I., Corti, T., Davin, E. L., Hirschi, M., Jaeger, E. B., Lehner, I., et al. (2010). Investigating soil moisture-climate interactions in a changing climate: a review. Earth Sci. Rev. 99, 125–161. doi: 10.1016/j.earscirev.2010.02.004
Setälä, H. M., Francini, G., Allen, J. A., Hui, N., Jumpponen, A., and Kotze, D. J. (2016). Vegetation type and age drive changes in soil properties, nitrogen, and carbon sequestration in urban parks under cold climate. Front. Ecol. Evol. 4:93. doi: 10.3389/fevo.2016.00093
Shao, P., Liang, C., Lynch, L., Xie, H., and Bao, X. (2019). Reforestation accelerates soil organic carbon accumulation: evidence from microbial biomarkers. Soil Biol. Biochem. 131, 182–190. doi: 10.1016/j.soilbio.2019.01.012
Shi, T., Cui, L., Wang, J., Fei, T., Chen, Y., and Wu, G. (2013). Comparison of multivariate methods for estimating soil total nitrogen with visible/near-infrared spectroscopy. Plant Soil 366, 363–375. doi: 10.1007/s11104-012-1436-8
Singh, S., Mayes, M. A., Shekoofa, A., Kivlin, S. N., Bansal, S., and Jagadamma, S. (2021). Soil organic carbon cycling in response to simulated soil moisture variation under field conditions. Sci. Rep. 11:10841. doi: 10.1038/s41598-021-90359-4
Srivastava, P., Singh, R., Bhadouria, R., Tripathi, S., and Raghubanshi, A. S. (2020). Temporal change in soil physicochemical, microbial, aggregate and available C characteristic in dry tropical ecosystem. Catena 190:104553. doi: 10.1016/j.catena.2020.104553
Sun, P., Wu, Y., Xiao, J., Hui, J., Hu, J., Zhao, F., et al. (2019). Remote sensing and modeling fusion for investigating the ecosystem water-carbon coupling processes. Sci. Total Environ. 697:134064. doi: 10.1016/j.scitotenv.2019.134064
Tian, L., Zhao, L., Wu, X., Fang, H., Zhao, Y., Hu, G., et al. (2018). Soil moisture and texture primarily control the soil nutrient stoichiometry across the Tibetan grassland. Sci. Total Environ. 622, 192–202. doi: 10.1016/j.scitotenv.2017.11.331
Wang, F., Zhang, X., Neal, A. L., Crawford, J. W., Mooney, S. J., and Bacq-Labreuil, A. (2022). Evolution of the transport properties of soil aggregates and their relationship with soil organic carbon following land use changes. Soil Tillage Res. 215:105226. doi: 10.1016/j.still.2021.105226
Wang, H., Shi, X., Yu, D., Weindorf, D. C., Huang, B., Sun, W., et al. (2009). Factors determining soil nutrient distribution in a small-scaled watershed in the purple soil region of Sichuan Province, China. Soil Tillage Res. 105, 300–306. doi: 10.1016/j.still.2008.08.010
Wang, H., Wu, J., Li, G., and Yan, L. (2020). Changes in soil carbon fractions and enzyme activities under different vegetation types of the northern Loess Plateau. Ecol. Evol. 10, 12211–12223. doi: 10.1002/ece3.6852
Wang, T., Xu, Q., Gao, D., Zhang, B., Zuo, H., and Jiang, J. (2021). Effects of thinning and understory removal on the soil water-holding capacity in Pinus massoniana plantations. Sci. Rep. 11:13029. doi: 10.1038/s41598-021-92423-5
Wang, Y., Shao, M., and Liu, Z. (2013). Vertical distribution and influencing factors of soil water content within 21-m profile on the Chinese Loess Plateau. Geoderma 193, 300–310. doi: 10.1016/j.geoderma.2012.10.011
Wu, G., Liu, Y., Yang, Z., Cui, Z., Deng, L., Chang, X., et al. (2017). Root channels to indicate the increase in soil matrix water infiltration capacity of arid reclaimed mine soils. J. Hydrol. 546, 133–139. doi: 10.1016/j.jhydrol.2016.12.047
Wu, G., Yang, Z., Cui, Z., Liu, Y., Fang, N., and Shi, Z. (2016). Mixed artificial grasslands with more roots improved mine soil infiltration capacity. J. Hydrol. 535, 54–60. doi: 10.1016/j.jhydrol.2016.01.059
Wu, X., Shi, W., and Tao, F. (2021). Estimations of forest water retention across China from an observation site-scale to a national-scale. Ecol. Indic. 132:108274. doi: 10.1016/j.ecolind.2021.108274
Xia, J., Zhao, Z., and Fang, Y. (2017). Soil hydro-physical characteristics and water retention function of typical shrubbery stands in the Yellow River Delta of China. Catena 156, 315–324. doi: 10.1016/j.catena.2017.04.022
Xu, H., Qu, Q., Lu, B., Zhang, Y., Liu, G., and Xue, S. (2020a). Variation in soil organic carbon stability and driving factors after vegetation restoration in different vegetation zones on the Loess Plateau, China. Soil Tillage Res. 204:104727. doi: 10.1016/j.still.2020.104727
Xu, H., Qu, Q., Wang, M., Li, P., Li, Y., Xue, S., et al. (2020b). Soil organic carbon sequestration and its stability after vegetation restoration in the Loess Hilly Region, China. Land Degrad. Dev. 31, 568–580. doi: 10.1002/ldr.3472
Yang, X., Shao, M., Li, T., Zhang, Q., Gan, M., Chen, M., et al. (2021). Distribution of soil nutrients under typical artificial vegetation in the desert-loess transition zone. Catena 200:105165. doi: 10.1016/j.catena.2021.105165
Yinglan, A., Wang, G., Liu, T., Xue, B., and Kuczera, G. (2019). Spatial variation of correlations between vertical soil water and evapotranspiration and their controlling factors in a semi-arid region. J. Hydrol. 574, 53–63. doi: 10.1016/j.jhydrol.2019.04.023
Zhang, P., Li, L., Pan, G., and Ren, J. (2006). Soil quality changes in land degradation as indicated by soil chemical, biochemical and microbiological properties in a karst area of southwest Guizhou, China. Environ. Geol. 51, 609–619. doi: 10.1007/s00254-006-0356-4
Zhang, Q., Shao, M., Jia, X., and Wei, X. (2019). Changes in soil physical and chemical properties after short drought stress in semi-humid forests. Geoderma 338, 170–177. doi: 10.1016/j.geoderma.2018.11.051
Zhang, S., Deng, Q., Wang, Y., Chen, J., Yu, M., Fang, X., et al. (2021). Linkage of microbial living communities and residues to soil organic carbon accumulation along a forest restoration gradient in southern China. For. Ecosyst. 8:57. doi: 10.1186/s40663-021-00334-8
Zhang, W., An, S., Xu, Z., Cui, J., and Xu, Q. (2011). The impact of vegetation and soil on runoff regulation in headwater streams on the east Qinghai–Tibet Plateau, China. Catena 87, 182–189. doi: 10.1016/j.catena.2011.05.020
Zhang, W., Gao, D., Chen, Z., Li, H., Deng, J., Qiao, W., et al. (2018a). Substrate quality and soil environmental conditions predict litter decomposition and drive soil nutrient dynamics following afforestation on the Loess Plateau of China. Geoderma 325, 152–161. doi: 10.1016/j.geoderma.2018.03.027
Zhang, W., Qiao, W., Gao, D., Dai, Y., Deng, J., Yang, G., et al. (2018b). Relationship between soil nutrient properties and biological activities along a restoration chronosequence of pinus tabulaeformis plantation forests in the Ziwuling Mountains, China. Catena 161, 85–95. doi: 10.1016/j.catena.2017.10.021
Zhang, Y., and Shangguan, Z. (2016). The coupling interaction of soil water and organic carbon storage in the long vegetation restoration on the Loess Plateau. Ecol. Eng. 91, 574–581. doi: 10.1016/j.ecoleng.2016.03.033
Zhang, Y., and Shangguan, Z. (2018). Interaction of soil water storage and stoichiometrical characteristics in the long-term natural vegetation restoration on the Loess Plateau. Ecol. Eng. 116, 7–13. doi: 10.1016/j.ecoleng.2018.02.026
Zhang, Y., Guo, S., Zhao, M., Du, L., Li, R., Jiang, J., et al. (2015). Soil moisture influence on the interannual variation in temperature sensitivity of soil organic carbon mineralization in the Loess Plateau. Biogeosciences 12, 3655–3664. doi: 10.5194/bg-12-3655-2015
Zhang, Z., Zha, T., Yu, Y., Zhang, X., Smith, P., and Rodrigo-Comino, J. (2022). Evaluating indices of soil organic carbon stability. A case study for forest restoration projects near Beijing, China. Ecol. Indic. 142:109222. doi: 10.1016/j.ecolind.2022.109222
Zhao, F., Wu, Y., Hui, J., Sivakumar, B., Meng, X., and Liu, S. (2021). Projected soil organic carbon loss in response to climate warming and soil water content in a loess watershed. Carbon Balanc. Manag. 16, 1–14. doi: 10.1186/s13021-021-00187-2
Zhao, W., Cui, Z., Zhang, J., and Jin, J. (2017). Temporal stability and variability of soil-water content in a gravel-mulched field in northwestern China. J. Hydrol. 552, 249–257. doi: 10.1016/j.jhydrol.2017.06.031
Zhao, X., Liu, P., Feng, Y., Zhang, W., Njoroge, B., Long, F., et al. (2022). Changes in soil physico-chemical and microbiological properties during natural succession: a case study in lower subtropical China. Front. Plant Sci. 13:878908. doi: 10.3389/fpls.2022.878908
Zheng, H., Nie, X., Liu, Z., Mo, M., and Song, Y. (2021). Identifying optimal ridge practices under different rainfall types on runoff and soil loss from sloping farmland in a humid subtropical region of Southern China. Agric. Water Manage. 255:107043. doi: 10.1016/j.agwat.2021.107043
Appendix
Keywords: vegetation restoration, soil properties, soil organic carbon, soil water content, carbon-water coupling, subtropical forest
Citation: Zhao X, Zhang W, Feng Y, Mo Q, Su Y, Njoroge B, Qu C, Gan X and Liu X (2022) Soil organic carbon primarily control the soil moisture characteristic during forest restoration in subtropical China. Front. Ecol. Evol. 10:1003532. doi: 10.3389/fevo.2022.1003532
Received: 26 July 2022; Accepted: 13 September 2022;
Published: 06 October 2022.
Edited by:
Sharif A. Mukul, University of the Sunshine Coast, AustraliaReviewed by:
Shixing Zhou, Sichuan Agricultural University, ChinaPurabi Saikia, Central University of Jharkhand, India
Copyright © 2022 Zhao, Zhang, Feng, Mo, Su, Njoroge, Qu, Gan and Liu. This is an open-access article distributed under the terms of the Creative Commons Attribution License (CC BY). The use, distribution or reproduction in other forums is permitted, provided the original author(s) and the copyright owner(s) are credited and that the original publication in this journal is cited, in accordance with accepted academic practice. No use, distribution or reproduction is permitted which does not comply with these terms.
*Correspondence: Xianhua Gan, gdfri@163.com; Xiaodong Liu, liuxd@scau.edu.cn
†These authors have contributed equally to this work and share first authorship