- 1Heilongjiang River Fisheries Research Institute, Chinese Academy of Fishery Sciences, Harbin, China
- 2Heilongjiang River Basin Fishery Ecological Environment Monitoring Center, Ministry of Agriculture and Rural Affairs, Harbin, China
- 3Harbin Management Station of the Fourth Administration Bureau of Reserve Assets, The Joint Logistics Support Force of PLA, Harbin, China
- 4Jilin Chagan Lake National Nature Reserve Administration, Songyuan, China
Watershed land-use changes have been identified as major threats to lake fauna, subsequently affecting ecosystem functioning. In this study, the functional-based approach was used to examine the effects of land use and environmental changes on phytoplankton communities in four selected lakes in Northeast China. We also identified the sensitive functional traits as indicators of environmental stressors. The integration of RLQ analysis with the fourth-corner approach significantly identified five of 18 functional trait categories, including flagella, filamentous, unicellular, mixotrophic, and chlorophyll c, as potential indicators to changes in watershed land-use intensity and environmental gradients. Significant relationships between traits and land use and water quality highlighted the consequential indirect impact of extensive agricultural and urban development on phytoplankton via allochthonous nutrient inputs and various contaminants. In addition, the functional richness of phytoplankton assemblages generally increased along with surface area and forests, but decreased along with intensive agricultural and urban land use, implying that functional homogenization may cause a reduction in ecosystem productivity and reliability to land-use intensity. Given the superior performance of the functional-based approach, our findings also highlighted the importance of the application of both the biological traits and functional diversity index in monitoring programs for lake ecosystems.
Introduction
Agriculture and urbanization have drastically transformed complex natural ecosystems into simplified managed ecosystems in many locations (Flynn et al., 2009; Hooke and Martín-Duque, 2012). By changing contaminant, sediment, nutrient, and organic matter loading (Williamson et al., 2008), land-use changes have far-reaching consequences for aquatic ecosystems (Foster et al., 2003). Lakes can be sensitive to land-use changes because they often occupy low points in landscapes, effectively accumulating effects across watersheds (Arbuckle and Downing, 2001; Stachelek et al., 2020). For example, intensive and extensive agricultural practices, and specifically the application of fertilizer or manure within watersheds, are strongly responsible for lake eutrophication (Bennett et al., 2001; Carpenter, 2005), in conjunction with the proliferation of harmful algal blooms, decrease in dissolved oxygen (DO) levels, and loss of aquatic biodiversity (Carpenter et al., 1998), while watershed urban development is associated with several environmental consequences, including nutrient pollution, and sewage, pathogen and other contaminants (Bai et al., 2018). The strong linkage between lake ecosystems and their watershed (Williamson et al., 2008) highlights the effects of watershed land use exerted on lake habitats and biota and their consequential indirect impact on ecosystem functioning.
Several studies described the decline in species diversity as a consequence of habitat degradation due to watershed development (Lenat and Crawford, 1994; Dodson et al., 2005; Xu et al., 2019). Changes in the structure and composition of regional habitats may lead to local extinction through changes in nutrient loading and runoff of pollutants from surrounding areas (Ricciardi and Rasmussen, 1999; Meador and Goldstein, 2003). Moreover, as watershed land-use types are likely to influence greenhouse gas emissions that can affect the regional climate (Smith et al., 2008), community structure is expected to respond significantly to differences in thermal conditions (Dell et al., 2011). Species should be shaped by environments based on their niches, which are better conceptualized by their morphological, behavioral, or life-history traits that impact their fitness via growth, reproduction, and survival, rather than their taxonomical identity (McGill et al., 2006). Thus, functional trait-based approaches can be informative for understanding the biotic communities in response to environmental gradients (Mouillot et al., 2013).
Phytoplankton, the foundation of aquatic food webs, are a highly diverse group of eukaryotes and cyanobacteria. As phytoplankton in aquatic systems have fast growth rates and short-generation times (Sommer, 1989), they are highly influenced by a multitude of factors, including nutrients, temperature, light, carbon dioxide (CO2), and grazers (Sarnelle, 1992; Elliott et al., 2006; Burson et al., 2018), and they are known to be sensitive and predictive to environmental changes. Ecological studies investigating the effects of environmental gradients often assess phytoplankton community changes based on taxonomy-based approaches such as species composition, biomass, or diversity. Some evidence suggests that urban and agricultural land use within a watershed, indirectly representing increased nutrient inputs and various contaminants (Carpenter et al., 1998; Nielsen et al., 2012; Beaver et al., 2014), may influence the composition of phytoplankton assemblages by favoring the growth of cyanobacteria toward the agricultural and artificial land-use types (Sánchez et al., 2021). Phytoplankton biomass and production in the lakes surrounded by urban areas or agricultural land tend to be increased by anthropogenic nutrient enrichment (Peng et al., 2021). Despite substantial progress in the understanding of the changes in phytoplankton communities due to watershed land-use changes, studies using trait-based approaches and exploring the consequential functional outcomes following land-use change are still scarce.
In this study, we evaluate how environmental stress gradients, representing a range of land uses and trophic conditions, affect phytoplankton functional traits and the diversity of lakes in northeast China. We used RLQ and fourth-corner analyses to assess the bivariate relationships between environmental stress gradients and species traits. The aims of this study were to: (1) examine the patterns of species composition and trait distribution of phytoplankton in the selected lakes in relation to environmental changes and (2) identify the sensitive functional traits as indicators of environmental stressors. We hypothesized that the phytoplankton communities in lakes vary along a land use and environmental gradient. The assumed variation in communities could be promoted by environmental filtering for specific traits. In addition, we hypothesized with increasing agricultural and urban land-use pressure and nutrient enrichment, there would be a corresponding decrease in functional diversity of phytoplankton communities.
Materials and Methods
Study Area
The study was conducted in four natural lakes in northeast China: the Chagan, Jingpo, Longhupao, and Wudalianchi (Figure 1), all belonging to the Songhua River Basin. The region is characterized by a temperate continental monsoon climate, with a mean annual temperature of 2.8°C and average annual precipitation of 531.1 mm. During the last several decades, the region has undergone massive changes in land cover and a large amount of land has formed sparse grassland and reed land which has become a place for local farmers to graze (Liu et al., 2005). Lake Chagan and Longhupao belong to the lowland part of the Songhua River Basin with loamy soils as the dominant geological features, while Lake Wudalianchi and Jingpo are lava-dammed lakes with sandy and loamy soils. The selected lakes varied in both development in their catchments and represented different morphometric and water quality conditions (Table 1).
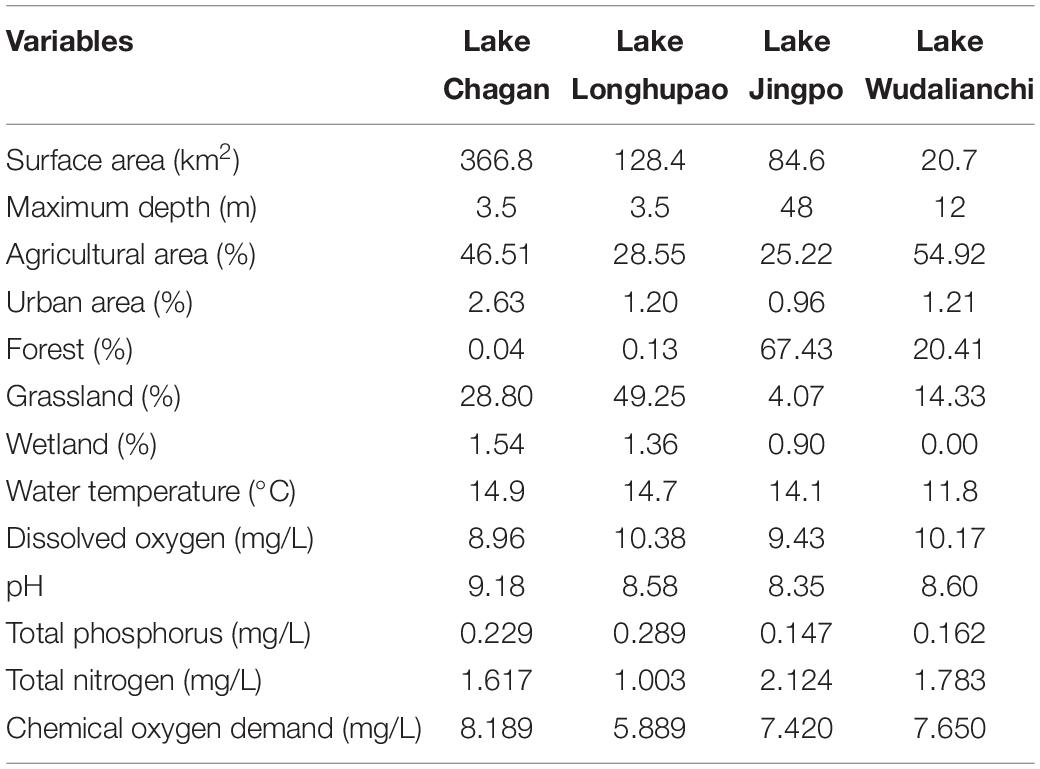
Table 1. Information on watershed land use and environmental gradient parameters in four selected lakes.
Land Use and Environmental Gradients
The land-use variables comprised proportions of the forest, grassland, wetland, and agricultural and urban areas within lake watersheds. These were determined at the whole topographic catchment scale. The Geographic Information System (GIS) program ArcGIS (version 10.7) was used to analyze the percentage of different land cover types. Environmental gradients were described in terms of lake morphology and water quality. Lake morphological variables comprised surface area and maximum depth. The surface area was drawn with ArcGIS software based on physical barriers and topographical features. Maximum water depth was obtained from Jing et al. (2014). Water quality variables comprised DO, water temperature, and pH that were measured in site at the subsurface (∼0.5 m) using the YSI Professional Plus Multi-meter (YSI Incorporation, Yellow Springs, OH, United States) and variables related to trophic status including total nitrogen (TN), total phosphorus (TP), and chemical oxygen demand (COD). TN and TP were analyzed using the persulfate digestion method and COD was determined with open reflux method (American Public Health Association [APHA], 1992).
Functional Trait Selection
Phytoplankton were collected from the four lakes seasonally in May (spring), August (summer), November (autumn) 2019, and February (winter) 2020. Composite water samples combining euphotic depth-integrated subsamples were collected for phytoplankton at 12 locations in each lake. Phytoplankton counts and cell volume estimates were performed using the inverted microscope method (Utermöhl, 1958). Phytoplankton was identified at the species or genus level according to Hu and Wei (2006). A total of 18 functional traits (Table 2) that are considered crucial for reproduction, resource acquisition, and predator avoidance (Weithoff, 2003; Litchman and Klausmeier, 2008) were assigned for each of the 279 phytoplankton species. Cell size and greatest axial linear dimension (GALD) (μm) were obtained by averaging measurements from at least 10 individuals for each taxa. Other traits were compiled based on scientific literature, web search, and expert knowledge (Jones, 2000; Weithoff, 2003; Hu and Wei, 2006; Litchman and Klausmeier, 2008; Klais et al., 2017; Loewen et al., 2021).
Statistical Analysis
To demonstrate the location of different algal groups in relation to their functional traits, principal component analysis (PCA) was conducted on trait matrix of species. Species in the PCA plot were phylogenetically grouped in major taxonomic groups (phyla): chlorophytes, chrysophytes, cryptophytes, cyanobacteria, diatoms, dinoflagellates, and euglenophytes.
Two complementary multivariate analyses, the RLQ analysis (Dolédec et al., 1996) and the fourth-corner approach (Dray and Legendre, 2008; Dray et al., 2014), were conducted to investigate the covariation between environmental characteristics and functional traits. RLQ (R, sites by environmental variables; L, sites by species abundance data; Q, species by traits) analysis performs a double inertia analysis between the Q and R through L ordination (Dolédec et al., 1996). Thus, it is possible to identify the effects of land use and environmental gradients on the traits based on the phytoplankton community structure. As suggested by Dray and Legendre (2008), we combined two permutation models for both the analyses tested the null hypotheses that “species assemblages are randomly attributed to sites, irrespective of the site characteristics” (model 2) and that “species are distributed according to their preferences for site conditions, but irrespective of their traits” (model 4). Finally, the fourth-corner approach was used to evaluate the specifically predictive role of functional traits in watershed land-use intensity and environmental gradients.
We calculated three multidimensional functional diversity indexes: functional richness (FRic), functional evenness (FEve), and functional divergence (FDiv). Increases in these three index values indicate that more niche space is occupied by species, a more evenly abundance distribution in niche space, and a larger divergence in the abundance distribution in niche space, respectively (Mason et al., 2005). The relationships between functional diversity index and land use and environmental gradients were examined using Pearson correlation.
All the analyses and graphics were performed in R version 4.0.3 (R Core Team, 2020) with the ade4 (Dray and Dufour, 2007), vegan (Oksanen et al., 2020), FD (Laliberté and Legendre, 2010), corrplot (Wei and Simko, 2021), and ggfortify (Tang et al., 2016) packages.
Results
The first two axes of the PCA performed on phytoplankton functional traits explained 26.11 and 20.07% of the variation, respectively (Figure 2). Of the four general trait groups, the first was dominated by species that are small to medium sized and chlorophyll b-producing and form colonies or coenobiums (i.e., chlorophytes); the second comprised filamentous and toxin-producing species with the ability of buoyancy regulation and nitrogen fixation (i.e., cyanobacteria); the third consist of large-sized species that are unicellular, chlorophyll c-producing, and require silica (i.e., diatoms); the fourth contained species that are mixotrophic and flagellate (i.e., dinoflagellates, crypto-, and euglenophytes).
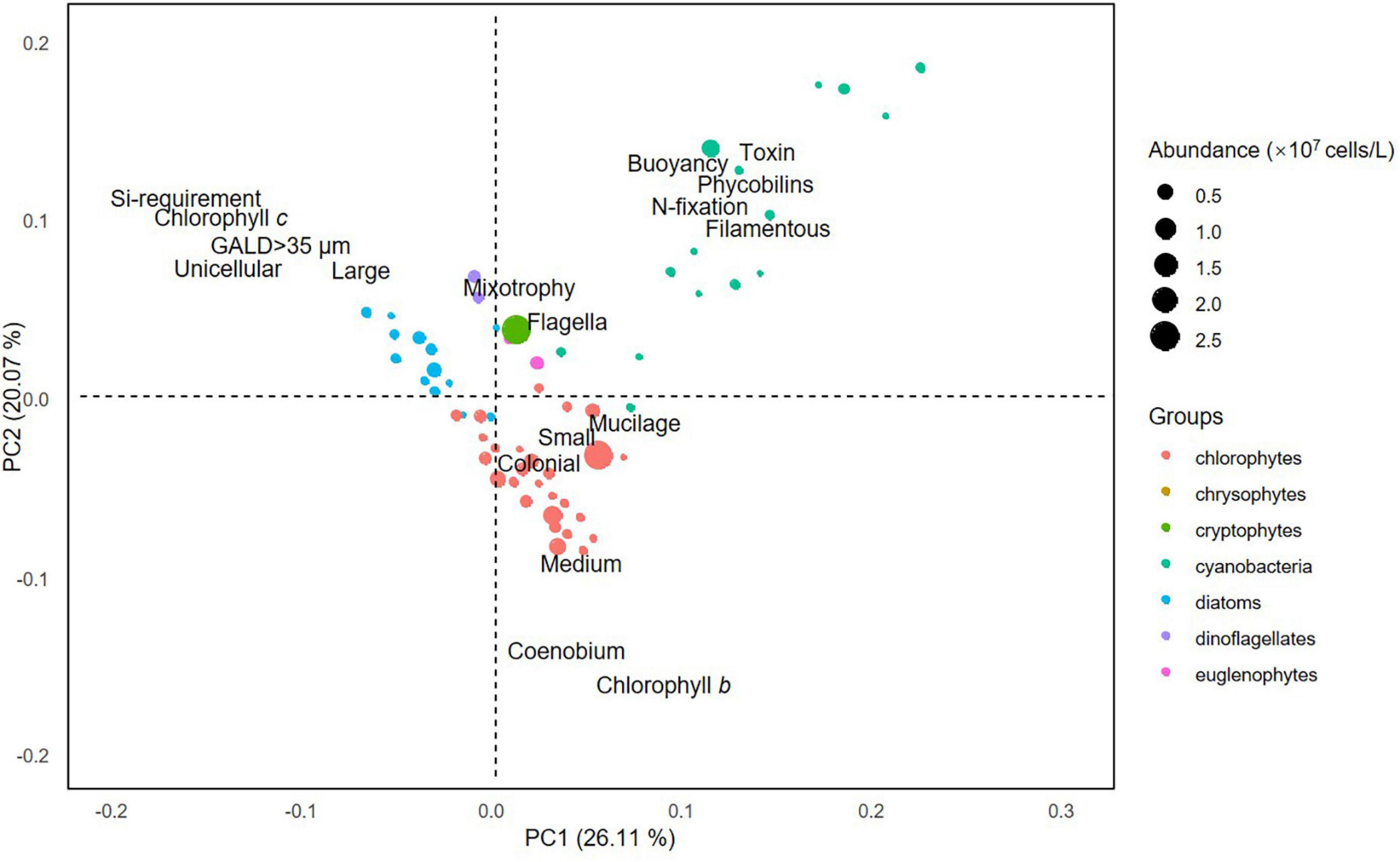
Figure 2. Results of principal component analysis performed on the functional traits of phytoplankton species.
The RLQ analysis revealed that land use and environmental gradients influence the distribution of phytoplankton species with specific traits (model 2, p < 0.001) and that the composition of phytoplankton assemblages is dependent on the land use and environmental gradients of studied lakes and influenced by species traits (model 4, p = 0.028). The first two RLQ axes accounted for 97.44% of the variation in relationships between land use and environmental gradients and functional traits. The first RLQ axis was positively correlated with urban and agricultural land use, surface area, pH, and TN. Grassland, wetland, DO, and TP loaded positively onto the second RLQ axis, while forest cover, maximum depth, and water temperature loaded negatively onto the second RLQ axis (Figure 3A). The traits filamentous, phycobilins, toxin-producing, and nitrogen fixation occurred in cyanobacteria and the traits chlorophyll b-producing, medium sized, and coenobium generally occurred in chlorophytes positively correlated with the first RLQ axis, whereas the traits unicellular, mixotrophic, and chlorophyll c-producing negatively correlated with the first RLQ axis. The traits flagella, large sized, and the silica requirement were negatively related to the second RLQ axis (Figure 3B).
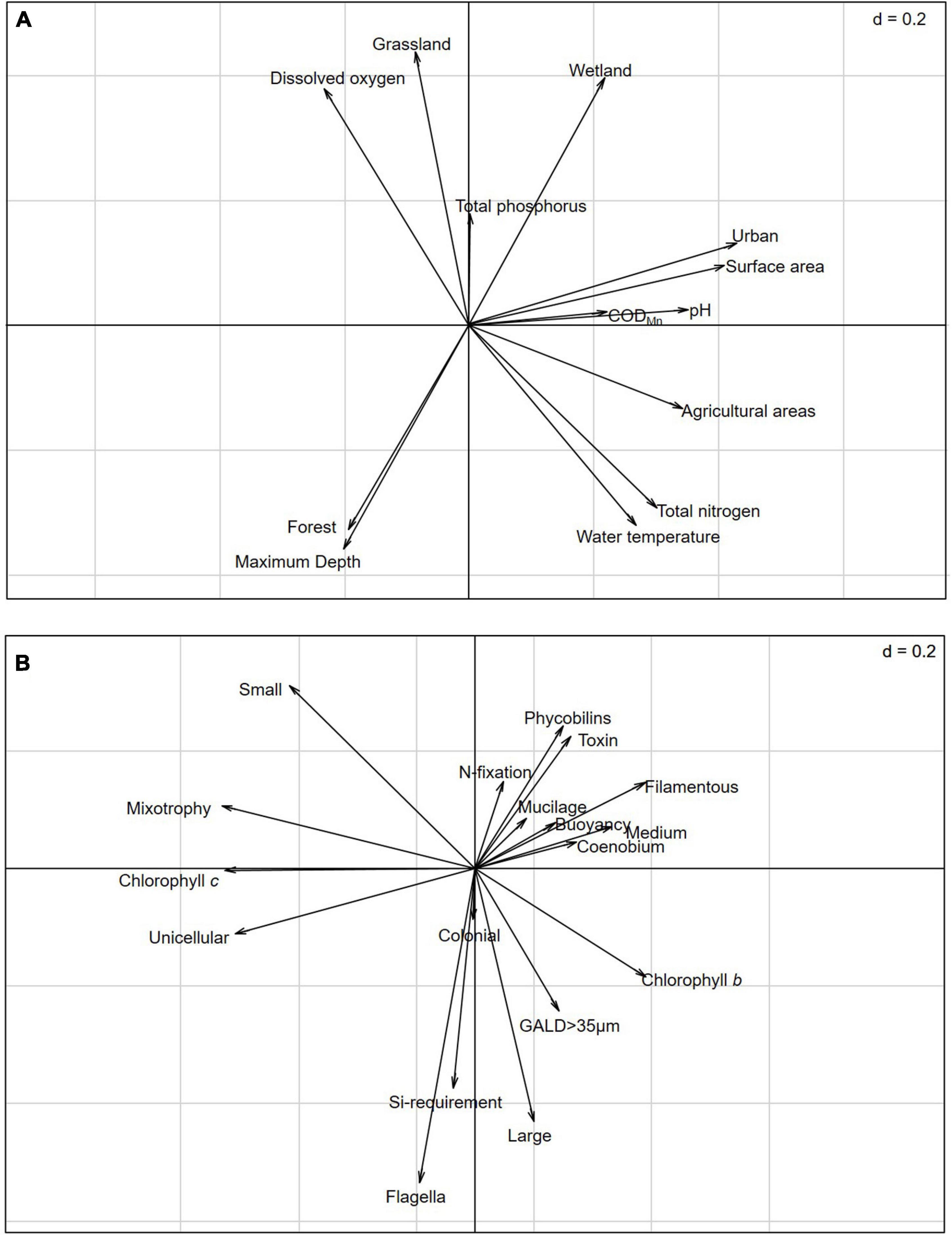
Figure 3. RLQ analysis revealing the correlations between land use and environmental gradient variables (R table 101 × 13) and functional traits (Q table 279 × 18), constrained by species abundance (L table 101 × 279): (A) Coefficients for the land use and environmental gradient variables and (B) coefficients for the functional traits.
The fourth-corner analysis provided the significant interactions between individual traits and land use and environmental gradients. Of the 18 traits examined in this study, five were deemed indicators of morphological, land use, and environmental variables (Figure 4). Filamentous taxa possessed flagella were significantly positively associated with surface area, maximum depth, and urban areas, whereas unicellular, mixotrophic, chlorophyll c-containing taxa were significantly negatively associated with surface area, agricultural, and urban land use.
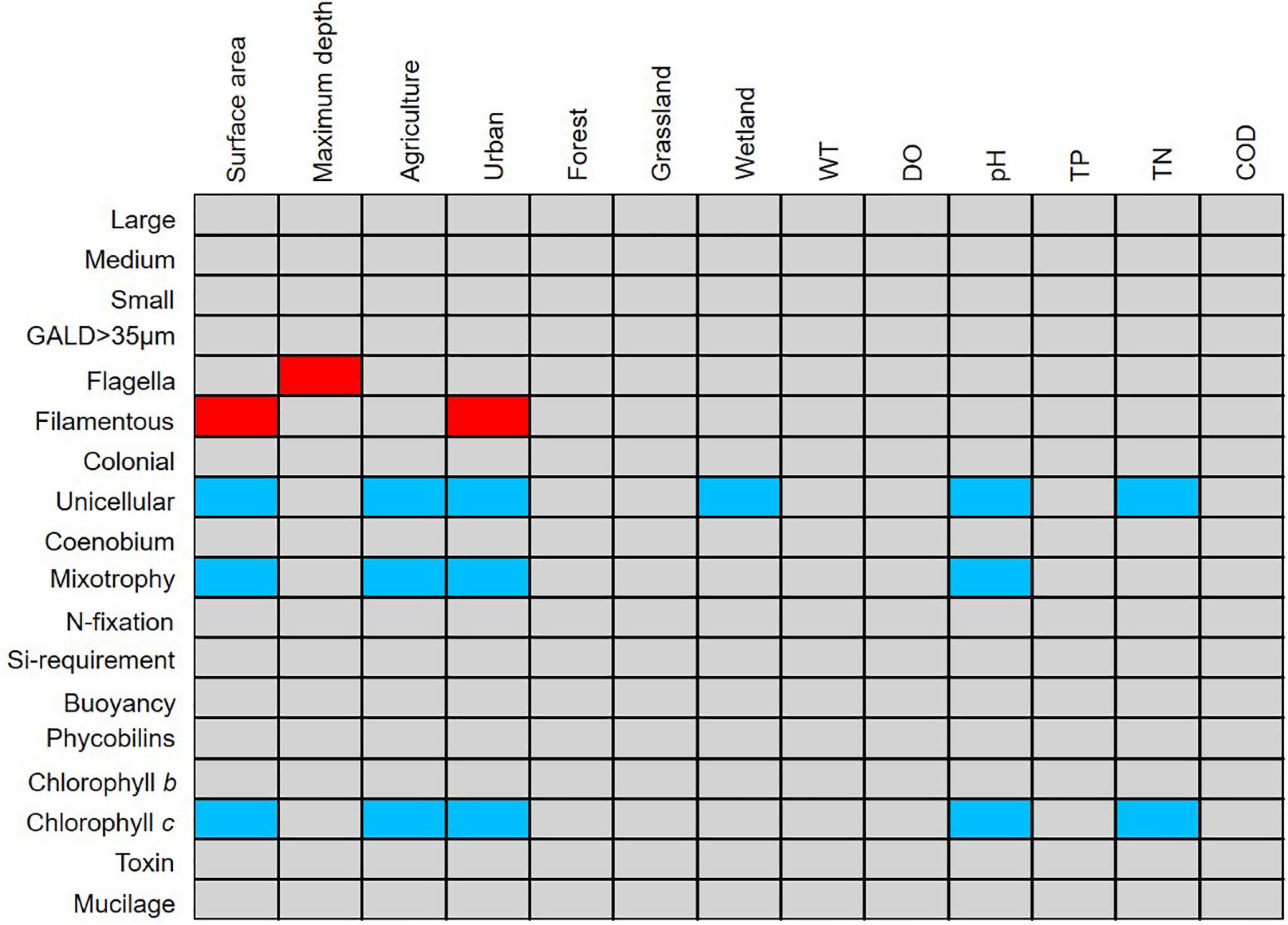
Figure 4. Results of the fourth-corner analysis revealing the correlation between phytoplankton traits and land use and environmental gradient variables in the selected lakes. Red cells indicate significant positive correlations (p < 0.05) and blue cells indicate significant negative correlations (p < 0.05).
Functional richness of phytoplankton assemblages generally increased along with surface area and forests, but decreased along with intensive agricultural and urban land use (Figure 5). There were no relationships between FEve and morphological and environmental variables. FDiv was positively correlated with wetland and higher TP, but negatively with agricultural land use (Figure 5).
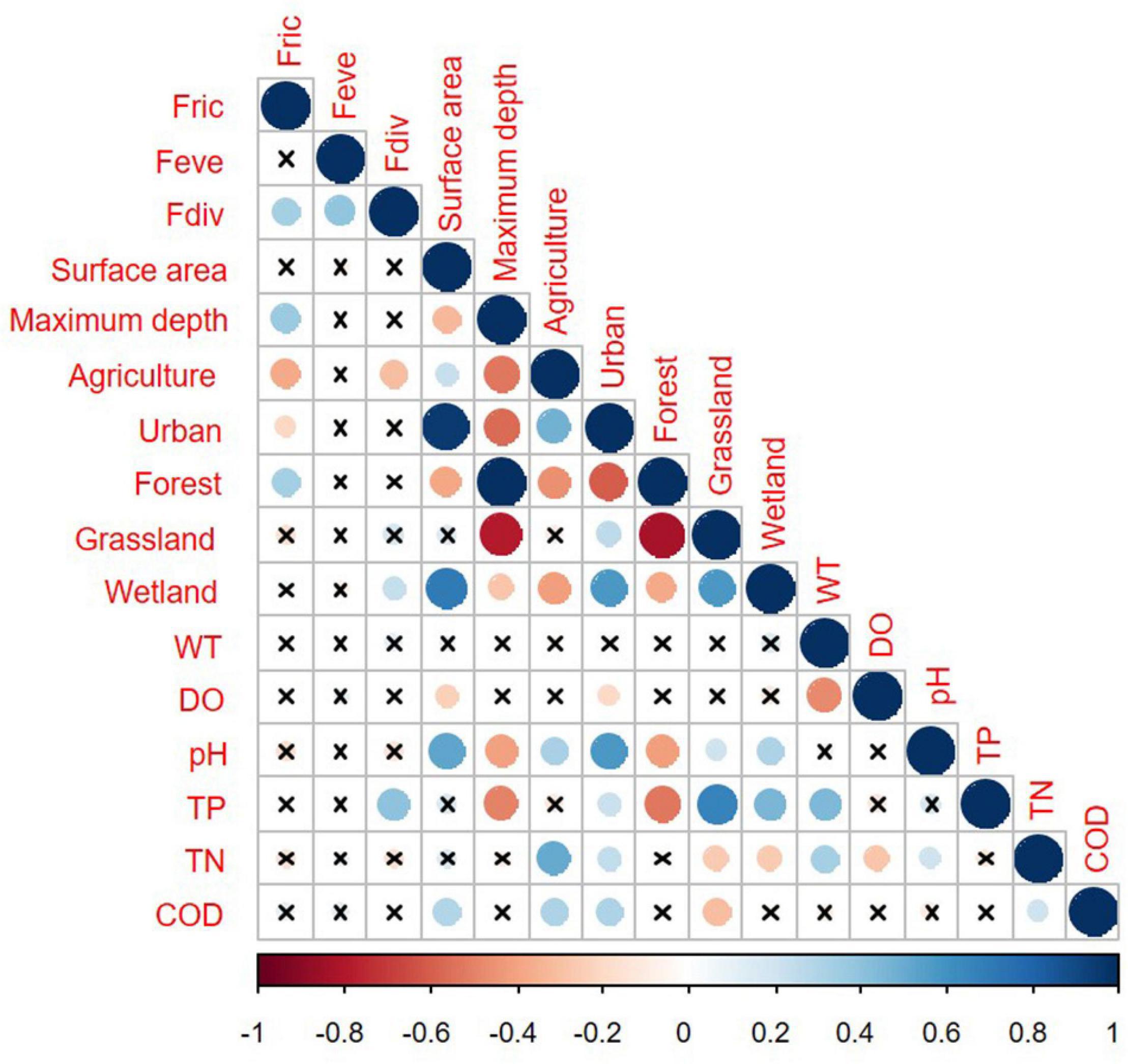
Figure 5. Pearson correlation matrix for the functional diversity indices and morphometric and environmental descriptors. Non-significant correlations (p > 0.05) are represented by the crossed-out points.
Discussion
Lakes provide for a wide range of valuable ecosystem services, including provisioning, regulation, and maintenance, as well as cultural services for instance, by improving human well-being (Reynaud and Lanzanova, 2017; Custódio et al., 2020). To this end, conventional approaches to lake restoration and preservation have typically focused on the mechanisms and factors regulating species assemblages. The observable functional differentiation of the traits of phytoplankton community revealed that of the stress gradients studied, human land-use activities had a consistent and strong influence upon phytoplankton functional structure via their consequences for nutrient loading. In addition, land-use transformations due to extensive agricultural and urban development cause a reduction of the FRic in phytoplankton assemblages.
Our results indicate significant relationships between traits and environmental stress variables, which provide a framework to link phytoplankton communities of lakes to environmental filtering. More specifically, increased agricultural and urban areas around lakes associated with changes in physicochemical properties, such as the increase in nitrogen, pH, or decrease of DO generally favored filamentous and toxin-producing algae as opposed to mixotrophic algae. These results are in agreement with other studies, which found that agricultural or urban land use could increase nutrient inputs from the watershed and in turn influence phytoplankton functional traits (Phillips et al., 1978; Carpenter and Waite, 2000; Bosch et al., 2009; Loewen et al., 2021). However, different anthropogenic land-use types have changing effects on lake N and P stoichiometry, with agricultural runoffs generally having a higher nitrogen-to-phosphorus ratio than those from urban areas or grasslands (Carpenter et al., 1998). In this study, agricultural lands were positively associated with TN, but negatively associated with trait nitrogen fixation. These results suggest a reduction of N:P ratio under generally eutrophic conditions that may favor the dominance by nitrogen-fixing cyanobacteria due to their competitive advantage under nitrogen-limited conditions (Smith, 1983; Sharpley and Withers, 1994). We also found that increased phosphorus inputs from grassland and wetland were positively associated with toxic cyanobacterial species, providing further support for the role of phosphorus load in fueling toxic cyanobacterial blooms (Bormans et al., 2016). As the water sources of Lake Chagan and Longhupao pass through manured grassland and a large number of wetlands were transferred to irrigation ditches for rice fields (Liu et al., 2021), a higher level of phosphorus loading to lakes and low N:P ratios can be expected.
Our results also showed that land use and environmental gradients could potentially influence resource acquisition traits of phytoplankton. Mixotrophy, which is an important trait related to nutrient acquisition (Litchman and Klausmeier, 2008), is advantageous under low nutrient conditions (Troost et al., 2005). Mixotrophic taxa increased in lakes with relatively low land-use intensity and nutrient conditions, suggesting a competitive advantage of these taxa under low nutrient supply. In addition, differences in pigment composition allow differences in spectral utilization patterns that can provide opportunities for species coexistence under light limitation (Stomp et al., 2004). Experimental studies with chlorophyll c-containing species Stephanodiscus hantzschii have shown negative effects of light intensity on chlorophyll c, especially under high nutrient conditions (Marzetz et al., 2020). We observed the opposite pattern with high chlorophyll c-containing species under nitrogen-limitation conditions. This might be due to decreasing light availability in deeper lakes with more forested dominated watershed, which favors the growth of chlorophyll c-containing species, as has been found in other north-temperate lakes (Loewen et al., 2021).
We revealed significant morphological factors (maximum depth and surface area) constraining the composition of phytoplankton communities. Our results indicate deeper water favoring flagellated algae. These results further demonstrate that the physical factors related to lake morphology influence functional strategies to survive in terms of functional traits related to motility (Wentzky et al., 2020). For instance, lake depth exerts a strong influence on the stability of the water column (e.g., mixing and thermal stratification) and thus on the light and oxygen condition and the internal nutrient loading from sediment into the hypolimnion (O’Brien et al., 2003; Yang et al., 2016). The flagellates possess active motility that enable them to alter position within the water column to alleviate any spatial limitation or segregation of resources and select optimum conditions (Salmaso and Padisák, 2007; Litchman and Klausmeier, 2008; Wentzky et al., 2020). Deep lakes where turbulence and internal nutrient loading were relatively low that may give a competitive advantage of flagellated algae to overcome nutrient deficiency and sedimentation losses by adjusting the position in the water column. In contrast, the negative association between maximum depth and traits nitrogen fixation and toxin production is possible because the increasing internal phosphorus loading with decreasing N:P ratios in shallow lakes is the main driver of the nitrogen-fixing cyanobacterial blooms (Smith, 1983; Sharpley and Withers, 1994). We also observed links between water temperature and algal traits in deep lakes. In this study, the higher water temperature may increase the prevalence of flagellated algae. Similar to water depth, the water temperature could influence the vertical distribution of oxygen and upward mixing of nutrients (Straile et al., 2003) and thus mediate physiological processes and behavioral responses of phytoplankton. The other morphological factor that was positively associated with the filamentous nitrogen-fixing algae is lake surface area. As larger lakes in this study are common in urban areas and have relatively shallow sloped basins and high phosphorus loadings, their phosphorus-rich conditions and conducive environment for germination of akinetes may promote the proliferation of nitrogen-fixing cyanobacteria (Kovács et al., 2012).
In aquatic systems, land-use changes are major driving forces of biodiversity loss (Allan, 2004). Increasing environmental stress gradient in our study, representing intensive urbanization or agricultural practices generally reduce FRic. Several studies have previously identified the significant effect of environmental disturbance upon freshwater biodiversity, and land-use changes have been identified as further significant factors influencing lake ecosystems (Arbuckle and Downing, 2001; Light and Marchetti, 2007; Nielsen et al., 2012; McGoff et al., 2013). Watershed land-use development has often been cited as having a negative effect upon the biodiversity of lakes (Dudgeon et al., 2006; van Soesbergen et al., 2019). Functional diversity has been shown with potential applications for predicting environmental disturbance. In this study, FRic of phytoplankton communities decreased with increasing land-use intensity. Similar patterns of functional changes of community in response to environmental disturbance have been reported, for instance, a reduction in functional diversity of aquatic insects in southeastern Brazil (Castro et al., 2018), and of fishes in Malaysia (Wilkinson et al., 2018), and of benthic diatoms in Italy (Falasco et al., 2021). Potentially higher functional diversity represented much higher levels of productivity and reliability (Dìaz and Cabido, 2001; Vallina et al., 2017). Our result of a significant decline in FRic with increasing land-use intensity suggests that homogenization may cause a reduction in ecosystem productivity and reliability to human disturbance. Given the importance of functional diversity to productivity and reliability (Mason et al., 2013; Gherardi and Sala, 2015), we suggest that management at the watershed and local scales such as mitigating grazing and cultivation practices can help to ensure a stronger determinant of ecosystem processes in face of anthropogenic disturbances.
Functional richness represents the amount of functional space occupied by a species assemblage (Mouchet et al., 2010). We suggest that the significant decrease in FRic along the agricultural and urban land-use gradients may imply a low degree of niche differentiation among species, reflecting the occupation by species with similar traits inhabiting the area, in response to extensive habitat degradation due to increased intensity of land use. Although increasing nutrient loadings from watershed are likely to favor cyanobacteria bloom (Doubek et al., 2015; Richardson et al., 2019), the results suggest that increasing land-use intensity is to the detriment of FRic, indicating that the functional community structure of phytoplankton is susceptible to agriculture and urbanization-driven disturbances. In this study, a possible explanation for a reduced functional diversity along the environmental stress gradient is eutrophication and anthropogenic contamination by agriculture and urbanization. For example, eutrophication of lakes is characterized by a potential deficit of oxygen, blooms of cyanobacteria, decrease water transparency, and deterioration of water quality (Carpenter et al., 1998). Other detrimental consequences of converting natural land covers include pesticides and other toxic chemicals from agricultural and urban areas. For instance, urban runoffs are widely recognized as transport vectors of salts, pharmaceuticals, and trace metals such as cadmium, copper, and lead (Müller et al., 2020), while agricultural runoffs are widely recognized as transport vectors of pesticides and phosphorus and nitrogen in forms of fertilizers and manure (Carpenter et al., 1998; Munz et al., 2017). These contaminants will lead to the loss of biodiversity directly through simple toxicity effects on physiological, developmental, and reproductive processes (Burant et al., 2018) or indirectly by altering the environmental conditions (Yang et al., 2022).
Conclusion
In this study, we considered the effect of land-use changes and environmental gradients upon the functional response of phytoplankton assemblages. The integration of RLQ analysis with the fourth-corner approach significantly identified functional traits as potential indicators to aquatic environments. Furthermore, anthropogenic disturbance such as agricultural and urban land use can modify phytoplankton traits which consequentially decreased functional diversity of phytoplankton assemblages. Moreover, declines in FRic representing functional homogenization may cause a reduction in ecosystem productivity and reliability. Given the superior performance of functional-based approach, our findings also highlighted the importance of the application of both the biological traits and functional diversity index in monitoring programs for lake ecosystems.
Data Availability Statement
The original contributions presented in the study are included in the article/Supplementary Material, further inquiries can be directed to the corresponding author.
Author Contributions
XD and DS designed and orchestrated the research and wrote up the findings. KM, JY, and XJ providing help for field sampling. HW, HL, LW, and CZ provided review and comments and edited the manuscript. TH revised the content of the manuscript and responsible for the overall submission. All authors contributed to the article and approved the submitted version.
Funding
This study was financially supported by the National Key Research and Development Program of China (Nos. 2019YFD0900605 and 2019YFD0900602), the National Natural Science Foundation of China (No. 31802298), and the Finance Special Fund of the Ministry of Agriculture and Rural Affairs of China (Fisheries Resources and Environment Survey in the Key Water Areas of Northeast China).
Conflict of Interest
The authors declare that the research was conducted in the absence of any commercial or financial relationships that could be construed as a potential conflict of interest.
Publisher’s Note
All claims expressed in this article are solely those of the authors and do not necessarily represent those of their affiliated organizations, or those of the publisher, the editors and the reviewers. Any product that may be evaluated in this article, or claim that may be made by its manufacturer, is not guaranteed or endorsed by the publisher.
Acknowledgments
We thank Feng Ji for providing excellent technical support.
Supplementary Material
The Supplementary Material for this article can be found online at: https://www.frontiersin.org/articles/10.3389/fevo.2021.819252/full#supplementary-material
References
Allan, J. D. (2004). Landscapes and riverscapes: The influence of land use on stream ecosystems. Annu. Rev. Ecol. Evol. 35, 257–284. doi: 10.1146/annurev.ecolsys.35.120202.110122
American Public Health Association [APHA] (1992). Standard Methods for the Examination of Water and Wastewater, 18th Edn. Washington DC: American Public Health Association.
Arbuckle, K. E., and Downing, J. A. (2001). The influence of watershed land use on lake N: P in a predominantly agricultural landscape. Limnol. Oceanogr. 46, 970–975. doi: 10.4319/lo.2001.46.4.0970
Bai, X., Lutz, A., Carroll, R., Keteles, K., Dahlin, K., Murphy, M., et al. (2018). Occurrence, distribution, and seasonality of emerging contaminants in urban watersheds. Chemosphere 200, 133–142. doi: 10.1016/j.chemosphere.2018.02.106
Beaver, J. R., Manis, E. E., Loftin, K. A., Graham, J. L., Pollard, A. I., and Mitchell, R. M. (2014). Land use patterns, ecoregion, and microcystin relationships in US lakes and reservoirs: A preliminary evaluation. Harmful Algae 36, 57–62. doi: 10.1016/j.hal.2014.03.005
Bennett, E. M., Carpenter, S. R., and Caraco, N. F. (2001). Human Impact on Erodable Phosphorus and Eutrophication: A Global Perspective: Increasing accumulation of phosphorus in soil threatens rivers, lakes, and coastal oceans with eutrophication. BioScience 51, 227–234.
Bormans, M., Marsálek, B., and Jancula, D. (2016). Controlling internal phosphorus loading in lakes by physical methods to reduce cyanobacterial blooms: a review. Aquat. Ecol. 50, 407–422. doi: 10.1007/s10452-015-9564-x
Bosch, I., Makarewicz, J. C., Lewis, T. W., Bonk, E. A., Finiguerra, M., and Groveman, B. (2009). Management of agricultural practices results in declines of filamentous algae in the lake littoral. J. Great Lakes Res. 35, 90–98. doi: 10.1016/j.jglr.2008.10.007
Burant, A., Selbig, W., Furlong, E. T., and Higgins, C. P. (2018). Trace organic contaminants in urban runoff: Associations with urban land-use. Environ. Pollut. 242, 2068–2077. doi: 10.1016/j.envpol.2018.06.066
Burson, A., Stomp, M., Greenwell, E., Grosse, J., and Huisman, J. (2018). Competition for nutrients and light: testing advances in resource competition with a natural phytoplankton community. Ecology 99, 1108–1118. doi: 10.1002/ecy.2187
Carpenter, K., and Waite, I. (2000). Relations of Habitat-Specific Algal Assemblages to Land Use and Water Chemistry in the Willamette Basin, Oregon. Environ. Monit. Assess. 64, 247–257. doi: 10.1023/A:1006460802772
Carpenter, S. R. (2005). Eutrophication of aquatic ecosystems: Bistability and soil phosphorus. Proc. Natl. Acad. Sci.U.S.A. 102, 10002–10005. doi: 10.1073/pnas.0503959102
Carpenter, S. R., Caraco, N. F., Correll, D. L., Howarth, R. W., Sharpley, A. N., and Smith, V. H. (1998). Nonpoint pollution of surface waters with phosphorus and nitrogen. Ecol. Appl. 8, 559–568.
Castro, D. M. P. D., Dolédec, S., and Callisto, M. (2018). Land cover disturbance homogenizes aquatic insect functional structure in neotropical savanna streams. Ecol. Indic. 84, 573–582. doi: 10.1016/j.ecolind.2017.09.030
Custódio, M., Villasante, S., Calado, R., and Lillebø, A. I. (2020). Valuation of Ecosystem Services to promote sustainable aquaculture practices. Rev. Aquac. 12, 392–405. doi: 10.1111/raq.12324
Dell, A. I., Pawar, S., and Savage, V. M. (2011). Systematic variation in the temperature dependence of physiological and ecological traits. Proc. Natl. Acad. Sci. U.S.A. 108, 10591–10596. doi: 10.1073/pnas.1015178108
Dìaz, S., and Cabido, M. (2001). Vive la différence: plant functional diversity matters to ecosystem processes. Trends Ecol. Evol. 16, 646–655. doi: 10.1016/s0169-5347(01)02283-2
Dodson, S. I., Lillie, R. A., and Will-Wolf, S. (2005). Land use, water chemistry, aquatic vegetation, and zooplankton community structure of shallow lakes. Ecol. Appl. 15, 1191–1198. doi: 10.1890/04-1494
Dolédec, S., Chessel, D., ter Braak, C. J. F., and Champely, S. (1996). Matching species traits to environmental variables: a new three-table ordination method. Environ. Ecol. Stat. 3, 143–166. doi: 10.1007/BF02427859
Doubek, J., Carey, C., and Cardinale, B. (2015). Anthropogenic land use is associated with N-fixing cyanobacterial dominance in lakes across the continental United States. Aquat. Sci. 77, 681–694. doi: 10.1007/s00027-015-0411-x
Dray, S., Choler, P., Dolédec, S., Peres-Neto, P. R., Thuiller, W., Pavoine, S., et al. (2014). Combining the fourth-corner and the RLQ methods for assessing trait responses to environmental variation. Ecology 95, 14–21. doi: 10.1890/13-0196.1
Dray, S., and Dufour, A. (2007). The ade4 Package: Implementing the Duality Diagram for Ecologists. J. Stat. Softw. 22, 1–20.
Dray, S., and Legendre, P. (2008). Testing the species traits–environment relationships: the fourth-corner problem revisited. Ecology 89, 3400–3412. doi: 10.1890/08-0349.1
Dudgeon, D., Arthington, A. H., Gessner, M. O., Kawabata, Z.-I., Knowler, D. J., Lévêque, C., et al. (2006). Freshwater biodiversity: importance, threats, status and conservation challenges. Biol. Rev. 81, 163–182. doi: 10.1017/S1464793105006950
Elliott, J. A., Jones, I. D., and Thackeray, S. J. (2006). Testing the sensitivity of phytoplankton communities to changes in water temperature and nutrient load, in a temperate lake. Hydrobiologia 559, 401–411. doi: 10.1007/s10750-005-1233-y
Falasco, E., Bona, F., Risso, A. M., and Piano, E. (2021). Hydrological intermittency drives diversity decline and functional homogenization in benthic diatom communities. Sci. Total Environ. 762:143090. doi: 10.1016/j.scitotenv.2020.143090
Flynn, D. F. B., Gogol-Prokurat, M., Nogeire, T., Molinari, N., Richers, B. T., Lin, B. B., et al. (2009). Loss of functional diversity under land use intensification across multiple taxa. Ecol. Lett. 12, 22–33. doi: 10.1111/j.1461-0248.2008.01255.x
Foster, D., Swanson, F., Aber, J., Burke, I., Brokaw, N., Tilman, D., et al. (2003). The Importance of Land-Use Legacies to Ecology and Conservation. BioScience 53, 77–88.
Gherardi, L. A., and Sala, O. E. (2015). Enhanced interannual precipitation variability increases plant functional diversity that in turn ameliorates negative impact on productivity. Ecol. Lett. 18, 1293–1300. doi: 10.1111/ele.12523
Hooke, R. L., and Martín-Duque, J. F. (2012). Land transformation by humans: a review. GSA Today. 12, 4–10. doi: 10.1130/GSAT151A.1
Hu, H., and Wei, Y. (2006). The Freshwater Algae of China-Systematics, Taxonomy and Ecology. Beijing: Science Press.
Jing, Z., Jiao, Y., Zhou, Y., and Chen, X. (2014). Encyclopedia of Rivers and Lakes in China Heilongjiang River and Liaohe River. Beijing: China Water Power Press.
Jones, R. I. (2000). Mixotrophy in planktonic protists: an overview. Freshw. Biol. 45, 219–226. doi: 10.1046/j.1365-2427.2000.00672.x
Klais, R., Norros, V., Lehtinen, S., Tamminen, T., and Olli, K. (2017). Community assembly and drivers of phytoplankton functional structure. Func. Ecol. 31, 760–767. doi: 10.1111/1365-2435.12784
Kovács, A. W., Tóth, V. R., and Vörös, L. (2012). Light-dependent germination and subsequent proliferation of N2-fixing cyanobacteria in a large shallow lake. Ann. Limnol-Int. J. Lim. 48, 177–185. doi: 10.1051/limn/2012010
Laliberté, E., and Legendre, P. (2010). A distance-based framework for measuring functional diversity from multiple traits. Ecology 91, 299–305. doi: 10.1890/08-2244.1
Lenat, D., and Crawford, J. K. (1994). Effects of Land Use on Water Quality and Aquatic Biota of Three North Carolina Piedmont Streams. Hydrobiologia 294, 185–199. doi: 10.1007/BF00021291
Light, T., and Marchetti, M. P. (2007). Distinguishing between Invasions and Habitat Changes as Drivers of Diversity Loss among California’s Freshwater Fishes. Conserv. Biol. 21, 434–446. doi: 10.1111/j.1523-1739.2006.00643.x
Litchman, E., and Klausmeier, C. A. (2008). Trait-Based Community Ecology of Phytoplankton. Annu. Rev. Ecol. Evol. Syst. 39, 615–639. doi: 10.1146/annurev.ecolsys.39.110707.173549
Liu, P., Zheng, C., Wen, M., Luo, X., Wu, Z., Liu, Y., et al. (2021). Ecological Risk Assessment and Contamination History of Heavy Metals in the Sediments of Chagan Lake. Northeast China. Water 13:894.
Liu, Y., Wang, D., Gao, J., and Deng, W. (2005). Land Use/Cover Changes, the Environment and Water Resources in Northeast China. Environ. Manage. 36, 691–701. doi: 10.1007/s00267-004-0285-5
Loewen, C. J. G., Vinebrooke, R. D., and Zurawell, R. W. (2021). Quantifying seasonal succession of phytoplankton trait-environment associations in human-altered landscapes. Limnol. Oceanogr. 66, 1409–1423. doi: 10.1002/lno.11694
Marzetz, V., Spijkerman, E., Striebel, M., and Wacker, A. (2020). Phytoplankton community responses to interactions between light intensity, light variations, and phosphorus supply. Front. Envir. Sci. 8:539733. doi: 10.3389/fenvs.2020.539733
Mason, N., Bello, F., Dray, S., Mouillot, D., and Pavoine, S. (2013). A guide for using functional diversity indices to reveal changes in assembly processes along ecological gradients. J. Veg. Sci. 24, 794–806. doi: 10.1111/jvs.12013
Mason, N., Mouillot, D., Lee, W., and Wilson, J. (2005). Functional richness, functional evenness and functional divergence: The primary components of functional diversity. Oikos 111, 112–118. doi: 10.1111/j.0030-1299.2005.13886.x
McGill, B. J., Enquist, B. J., Weiher, E., and Westoby, M. (2006). Rebuilding community ecology from functional traits. Trends Ecol. Evol. 21, 178–185. doi: 10.1016/j.tree.2006.02.002
McGoff, E., Solimini, A. G., Pusch, M. T., Jurca, T., and Sandin, L. (2013). Does lake habitat alteration and land-use pressure homogenize European littoral macroinvertebrate communities? J. Appl. Ecol. 50, 1010–1018. doi: 10.1111/1365-2664.12106
Meador, M. R., and Goldstein, R. M. (2003). Assessing water quality at large geographic scales: Relations among land use, water physicochemistry, riparian condition, and fish community structure. Environ. Manage. 31, 504–517. doi: 10.1007/s00267-002-2805-5
Mouchet, M. A., Villéger, S., Mason, N. W. H., and Mouillot, D. (2010). Functional diversity measures: an overview of their redundancy and their ability to discriminate community assembly rules. Funct. Ecol. 24, 867–876. doi: 10.1111/j.1365-2435.2010.01695.x
Mouillot, D., Graham, N. A. J., Villéger, S., Mason, N. W. H., and Bellwood, D. R. (2013). A functional approach reveals community responses to disturbances. Trends Ecol. Evol. 28, 167–177. doi: 10.1016/j.tree.2012.10.004
Müller, A., Österlund, H., Marsalek, J., and Viklander, M. (2020). The pollution conveyed by urban runoff: A review of sources. Sci. Total Environ. 709:136125. doi: 10.1016/j.scitotenv.2019.136125
Munz, N. A., Burdon, F. J., de Zwart, D., Junghans, M., Melo, L., Reyes, M., et al. (2017). Pesticides drive risk of micropollutants in wastewater-impacted streams during low flow conditions. Water Res. 110, 366–377. doi: 10.1016/j.watres.2016.11.001
Nielsen, A., Trolle, D., Søndergaard, M., Lauridsen, T. L., Bjerring, R., Olesen, J. E., et al. (2012). Watershed land use effects on lake water quality in Denmark. Ecol. Appl. 22, 1187–1200. doi: 10.1890/11-1831.1
O’Brien, K. R., Ivey, G. N., Hamilton, D. P., Waite, A. M., and Visser, P. M. (2003). Simple mixing criteria for the growth of negatively buoyant phytoplankton. Limnol. Oceanogr. 48, 1326–1337. doi: 10.4319/lo.2003.48.3.1326
Oksanen, J., Blanchet, F. G., Friendly, M., Kindt, R., Legendre, P., McGlinn, D., et al. (2020). Vegan: community ecology package. R package version 2.5–7. Available online at: https://cran.r-project.org/web/packages/vegan/index.html
Peng, X., Zhang, L., Li, Y., Lin, Q., He, C., Huang, S., et al. (2021). The changing characteristics of phytoplankton community and biomass in subtropical shallow lakes: Coupling effects of land use patterns and lake morphology. Water Res. 200:117235. doi: 10.1016/j.watres.2021.117235
Phillips, G. L., Eminson, D., and Moss, B. (1978). A mechanism to account for macrophyte decline in progressively eutrophicated freshwaters. Aquat. Bot. 4, 103–126. doi: 10.1016/0304-3770(78)90012-8
R Core Team (2020). R: A Language and Environment for Statistical Computing. Vienna: R Foundation for Statistical Computing.
Reynaud, A., and Lanzanova, D. (2017). A Global Meta-Analysis of the Value of Ecosystem Services Provided by Lakes. Ecol. Econ. 137, 184–194. doi: 10.1016/j.ecolecon.2017.03.001
Ricciardi, A., and Lanzanova, J. B. (1999). Extinction rates of North American freshwater fauna. Conserv. Biol. 13, 1220–1222. doi: 10.1046/j.1523-1739.1999.98380.x
Richardson, J., Feuchtmayr, H., Miller, C., Hunter, P. D., Maberly, S. C., and Carvalho, L. (2019). Response of cyanobacteria and phytoplankton abundance to warming, extreme rainfall events and nutrient enrichment. Glob. Chang. Biol. 25, 3365–3380. doi: 10.1111/gcb.14701
Salmaso, N., and Padisák, J. (2007). Morpho-Functional Groups and phytoplankton development in two deep lakes (Lake Garda. Italy and Lake Stechlin, Germany). Hydrobiologia 578, 97–112. doi: 10.1007/s10750-006-0437-0
Sánchez, M. L., Schiaffino, M. R., Graziano, M., Huber, P., Lagomarsino, L., Minotti, P., et al. (2021). Effect of land use on the phytoplankton community of Pampean shallow lakes of the Salado River basin (Buenos Aires Province. Argentina). Aquat. Ecol. 55, 417–435. doi: 10.1007/s10452-021-09835-8
Sarnelle, O. (1992). Nutrient Enrichment and Grazer Effects on Phytoplankton in Lakes. Ecology 73, 551–560. doi: 10.2307/1940761
Sharpley, A. N., and Withers, P. J. A. (1994). The environmentally-sound management of agricultural phosphorus. Fertil. Res. 39, 133–146. doi: 10.1007/BF00750912
Smith, P., Martino, D., Cai, Z., Gwary, D., Janzen, H., Kumar, P., et al. (2008). Greenhouse gas mitigation in agriculture. Philos. Trans. R. Soc. Lond. B Biol. Sci. 363, 789–813. doi: 10.1098/rstb.2007.2184
Smith, V. H. (1983). Low Nitrogen to Phosphorus Ratios Favor Dominance by Blue-Green Algae in Lake Phytoplankton. Science 221, 669–671. doi: 10.1126/science.221.4611.669
Sommer, U. (1989). Nutrient status and nutrient competition of phytoplankton in a shallow, hypertrophic lake. Limnol. Oceanogr. 34, 1162–1173. doi: 10.4319/lo.1989.34.7.1162
Stachelek, J., Weng, W., Carey, C. C., Kemanian, A. R., Cobourn, K. M., Wagner, T., et al. (2020). Granular measures of agricultural land use influence lake nitrogen and phosphorus differently at macroscales. Ecol. Appl. 30:e02187. doi: 10.1002/eap.2187
Stomp, M., Huisman, J., de Jongh, F., Veraart, A. J., Gerla, D., Rijkeboer, M., et al. (2004). Adaptive divergence in pigment composition promotes phytoplankton biodiversity. Nature 432, 104–107. doi: 10.1038/nature03044
Straile, D., Jöhnk, K., and Rossknecht, H. (2003). Complex effects of winter warming on the physicochemical characteristics of a deep lake. Limnol. Oceanogr. 48, 1432–1438. doi: 10.4319/lo.2003.48.4.1432
Tang, Y., Horikoshi, M., and Li, W. (2016). Ggfortify: Unified Interface to Visualize Statistical Result of Popular R Packages. R J. 2, 474–485.
Troost, T. A., Kooi, B. W., and Kooijman, S. (2005). Ecological specialization of mixotrophic plankton in a mixed water column. Am. Nat. 166, E45–E61. doi: 10.1086/432038
Utermöhl, H. (1958). Methods of collecting plankton for various purposes are discussed. SIL Commun. 9, 1–38. doi: 10.1080/05384680.1958.11904091
Vallina, S. M., Cermeno, P., Dutkiewicz, S., Loreau, M., and Montoya, J. M. (2017). Phytoplankton functional diversity increases ecosystem productivity and stability. Ecol. Modell. 361, 184–196. doi: 10.1016/j.ecolmodel.2017.06.020
van Soesbergen, A., Sassen, M., Kimsey, S., and Hill, S. (2019). Potential impacts of agricultural development on freshwater biodiversity in the Lake Victoria basin. Aquat. Conserv.: Mar. Freshw. Ecosyst. 29, 1052–1062. doi: 10.1002/aqc.3079
Wei, T., and Simko, V. (2021). R package ‘corrplot’: Visualization of a Correlation Matrix (Version 0.90). Available atonline at: https://github.com/taiyun/corrplot (accessed November 2021).
Weithoff, G. (2003). The concepts of ‘plant functional types’ and ‘functional diversity’ in lake phytoplankton - a new understanding of phytoplankton ecology? Freshw. Biol. 48, 1669–1675.
Wentzky, V. C., Tittel, J., Jäger, C. G., Bruggeman, J., and Rinke, K. (2020). Seasonal succession of functional traits in phytoplankton communities and their interaction with trophic state. J. Ecol. 108, 1649–1663. doi: 10.1111/1365-2745.13395
Wilkinson, C., Yeo, D., Tan, H., Fikri, A. H., and Ewers, R. (2018). Land-use change is associated with a significant loss of freshwater fish species and functional richness in Sabah. Malaysia. Biol. Conserv. 222, 164–171. doi: 10.1016/j.biocon.2018.04.004
Williamson, C. E., Dodds, W., Kratz, T. K., and Palmer, M. A. (2008). Lakes and streams as sentinels of environmental change in terrestrial and atmospheric processes. Front. Ecol. Environ. 6:247–254. doi: 10.1890/070140
Xu, L., Chen, S. S., Xu, Y., Li, G., and Su, W. (2019). Impacts of Land-Use Change on Habitat Quality during 1985–2015 in the Taihu Lake Basin. Sustainability 11:3513. doi: 10.3390/su11133513
Yang, Y., Chen, H., Abdullah Al, M., Ndayishimiye, J. C., Yang, J. R., Isabwe, A., et al. (2022). Urbanization reduces resource use efficiency of phytoplankton community by altering the environment and decreasing biodiversity. J. Environ. Sci. 112, 140–151. doi: 10.1016/j.jes.2021.05.001
Keywords: algae, community structure, eutrophication, functional-based approach, human disturbance
Citation: Du X, Song D, Ming K, Yang J, Jin X, Wang H, Liu H, Wang L, Zhao C and Huo T (2022) Functional Responses of Phytoplankton Assemblages to Watershed Land Use and Environmental Gradients. Front. Ecol. Evol. 9:819252. doi: 10.3389/fevo.2021.819252
Received: 21 November 2021; Accepted: 17 December 2021;
Published: 13 January 2022.
Edited by:
Tian Zhao, Key Laboratory of Mountain Ecological Rehabilitation and Biological Resource Utilization, Chengdu Institute of Biology, Chinese Academy of Sciences (CAS), ChinaReviewed by:
Xiangjiang Liu, Huazhong Agricultural University, ChinaDavid Almeida, CEU San Pablo University, Spain
Zhicong Wang, Key Laboratory of Aquatic Biodiversity and Conservation, Institute of Hydrobiology, Chinese Academy of Sciences (CAS), China
Copyright © 2022 Du, Song, Ming, Yang, Jin, Wang, Liu, Wang, Zhao and Huo. This is an open-access article distributed under the terms of the Creative Commons Attribution License (CC BY). The use, distribution or reproduction in other forums is permitted, provided the original author(s) and the copyright owner(s) are credited and that the original publication in this journal is cited, in accordance with accepted academic practice. No use, distribution or reproduction is permitted which does not comply with these terms.
*Correspondence: Tangbin Huo, tbhuo@163.com