- Department of Ecology, Evolution, and Organismal Biology, Iowa State University, Ames, IA, United States
Oviparous animals, such as turtles, lay eggs whose success or demise depends on environmental conditions that influence offspring phenotype (morphology, physiology, and in many reptiles, also sex determination), growth, and survival, while in the nest and post-hatching. Consequently, because turtles display little parental care, maternal provisioning of the eggs and female nesting behavior are under strong selection. But the consequences of when and where nests are laid are affected by anthropogenic habitat disturbances that alter suitable nesting areas, expose eggs to contaminants in the wild, and modify the thermal and hydric environment experienced by developing embryos, thus impacting hatchling survival and the sexual fate of taxa with temperature-dependent sex determination (TSD) and genotypic sex determination (GSD). Indeed, global and local environmental change influences air, water, and soil temperature and moisture, which impact basking behavior, egg development, and conditions within the nest, potentially rendering current nesting strategies maladaptive as offspring mortality increases and TSD sex ratios become drastically skewed. Endocrine disruptors can sex reverse TSD and GSD embryos alike. Adapting to these challenges depends on genetic variation, and little to no heritability has been detected for nest-site behavior. However, modest heritability in threshold temperature (above and below which females or males develop in TSD taxa, respectively) exists in the wild, as well as interpopulation differences in the reaction norm of sex ratio to temperature, and potentially also in the expression of gene regulators of sexual development. If this variation reflects additive genetic components, some adaptation might be expected, provided that the pace of environmental change does not exceed the rate of evolution. Research remains urgently needed to fill current gaps in our understanding of the ecology and evolution of nest-site choice and its adaptive potential, integrating across multiple levels of organization.
Introduction
Reproduction is an essential component of individual fitness, and it must take place at the appropriate place and time for it to be successful. For oviparous animals such as turtles, nesting is a fundamental part of their reproductive cycle. Diverse strategies have evolved of nesting behavior combined with other maternal effects that can potentially maximize offspring and female fitness. The main elements that make up a female reproductive strategy are: when, where, and how nesting takes place and the allocation of resources to the eggs laid in those nests. These variables are important given the thermal requirements for egg formation and embryonic development of ectotherms, such as turtles, and how embryogenesis is also restricted by moisture conditions in the nesting substrate in many species (Kamel and Mrosovsky, 2004). Here we first briefly review turtle maternal effects and nesting behavior, then the challenges posed by climate change and other anthropogenic disturbances, mostly in turtles with environmental sex determination, but also in turtles with sex ratios insensitive to temperature whose biology is still vulnerable to environmental change. We concentrate, not exclusively, on North American freshwater turtles, particularly those for which more data are available. Figure 1 illustrates the various causes and consequences of nest-site choice.
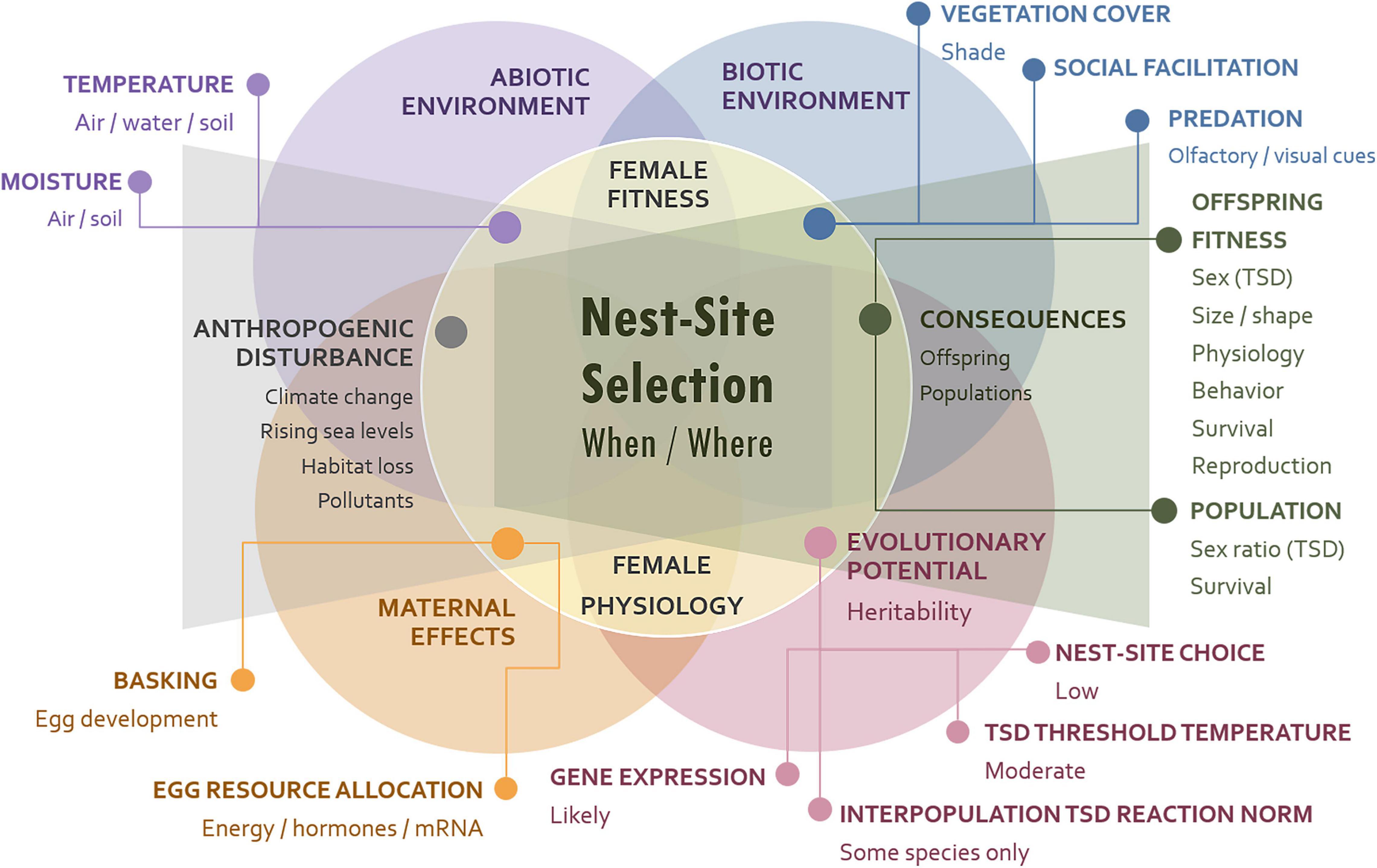
Figure 1. Causes and consequences of nest-site choice. Biotic factors, e.g., predation risk, social facilitation, and vegetation cover, impact nest-site selection directly, whereas abiotic factors may have indirect effects, via their influence of air and water temperature on basking behavior and egg resource allocation. Vegetation cover plus air temperature and humidity affect the thermal and moisture conditions of the soil and those experienced by the developing embryos, thus impacting offspring phenotype and fitness, and population dynamics. Anthropogenic disturbances are detrimental for habitat quality and incubation conditions, and adaptive responses are less likely for nesting behaviors than for TSD reaction norms or for developmental gene networks. In TSD species whose reaction norms vary among localities, habitat loss may preclude recolonization of populations extirpated by environmental change from other populations pre-adapted to altered conditions.
Maternal Effects Other Than Nest-Site Selection
Maternal effects in animals contribute significantly to their offspring’s phenotype and fitness (Bernardo, 1996; Moore et al., 2019). One potential maternal effect is parental care after oviposition, which researchers often describe as lacking in turtles, but examples of parental care exist in at least a few species. These include nest guarding in natural populations of the mud turtle, Kinosternon flavescens, potentially reducing predation and altering the nest moisture conditions in ways that improve offspring fitness (Iverson, 1990), as well as nest guarding in a captive Asian forest tortoise, Manouria emys (McKeown et al., 1982). Recently, vocalizations were recorded between hatchlings of the Amazonian giant river turtle (Podocnemis expansa) and females who waited nearby nesting beaches and communicated with newborns to guide them to feeding areas immediately after hatching (Ferrara et al., 2013). Thus, while few examples exist of parental care in turtles, growing evidence suggests that it plays a more prominent role in some species than previously thought. Nonetheless, because parental care is less extensive in turtles than in crocodilians and some python snakes (Shine, 1988; Balshine, 2012), nutrient and hormonal allocation to the eggs remain dominant maternal effects observed in this clade (Roosenburg and Dunham, 1997) other than nest-site choice.
Indeed, reproductive female turtles invest heavily into egg production, and different life-history traits have evolved to improve hatchling fitness. For instance, in several turtles, larger females, who possess more energy to allocate to reproduction, tend to produce more and/or larger eggs compared to smaller females, who may compensate by elongating egg shape (Rowe, 1994; Valenzuela, 2001a; Walde et al., 2007; Escalona et al., 2018). Yet, many turtles defy expectations from optimality models, such that egg size never reaches a plateau as female size increases. The positive correlation between female size and egg size can be vital because larger eggs produce larger hatchlings who are better at surviving (e.g., Miller, 1993; Valenzuela, 2001a; Rollinson and Brooks, 2008; Ceballos et al., 2014; reviewed for sea turtle hatchlings in Booth, 2017). Besides nutrients, females allocate hormones such as estrogen, progesterone, and testosterone into their eggs, affecting embryonic sexual development in turtles (Bowden and Paitz, 2021), of which only a brief overview is provided here.
Turtles exhibit two main types of sex-determining mechanisms by which embryos commit to their sexual fate: temperature-dependent sex determination (TSD) of various patterns and genotypic sex determination (GSD) with independently evolved female and male heterogametic sex chromosomes (Valenzuela and Lance, 2004; Tree of Sex Consortium, 2014; Bista and Valenzuela, 2020). No reported mixed system in turtles has withstood empirical scrutiny (Valenzuela et al., 2014; Mu et al., 2015), whereas sex reversals (Valenzuela et al., 2003) are documented in natural populations of other reptiles (reviewed in Whiteley et al., 2021). TSD is more prevalent in turtles than GSD, and its potential adaptive value has been studied extensively and is reviewed elsewhere (see Charnov and Bull, 1977; Valenzuela, 2004, 2021; Schwanz and Georges, 2021). TSD has received much attention in the last few decades, especially as TSD taxa must adapt to overcome environmental challenges such as rising global temperatures to avoid extinction. This is true for TSD turtles that produce males at low temperatures and females at high temperatures (TSD Ia) as well as for turtles that produce males at intermediate temperatures and females above and below (TSD II). Moreover, reptiles like tuatara, some lizards, and crocodilians that produce females at colder temperatures and males at warmer values (TSD Ib) (Valenzuela and Lance, 2004) might be even more vulnerable to global warming as population growth is severely reduced when females are scarce. Temperatures within turtle nests are influenced not only by global climate but by multiple environmental factors at micro-geographic and micro-temporal scales, such that the temperatures experienced by the developing embryos, including during the thermosensitive period for sex determination (Valenzuela, 2008; Mitchell et al., 2013; but see Gómez-Saldarriaga et al., 2016), are affected by the nest-site choices females make.
Maternal effects via the allocation of hormones also matter for sexual development because higher concentrations of yolk estrogen have a feminizing effect within clutches of TSD taxa, as in painted (Chrysemys picta) and red-eared slider (Trachemys scripta) turtles, particularly at intermediate temperatures around the pivotal value that produces 1:1 population sex ratios (Bowden et al., 2000, 2002; Bowden and Paitz, 2018). These yolk estrogen concentrations increase during the breeding season (Bowden et al., 2000, 2002), yielding more female-biased clutches later in the season (Bowden and Paitz, 2018). Thus, nesting phenology must be taken into account when analyzing sex ratio dynamics in populations. Turtles with GSD have been understudied in this respect, although no association between offspring sex and maternal yolk hormone levels was detected in two GSD softshell turtles, Apalone mutica and A. spinifera (Radder, 2007). Subtle effects of maternal age on sex ratios have also been documented in TSD turtles and leopard geckos (Roush and Rhen, 2018). Notably, females nesting in habitats polluted with endocrine-disrupting chemicals (EDCs) may pay fitness costs due to the increased mortality or suboptimal offspring phenotypes induced by these substances, including altered sexual development (Mizoguchi and Valenzuela, 2016). Pollutants may be transferred maternally to the offspring, or eggs could be contaminated directly in the nesting substrate, leading to developmental abnormalities, sex reversal, abnormal sex steroid production, and reduced hatching success (Barraza et al., 2021).
When to Nest: Basking and Timing of Oviposition
Egg formation concludes after fertilization but before females are ready to nest, and air temperature and basking behavior are crucial factors in that process as they influence body temperature. Basking elevates the internal body temperature of turtles above that of the water in which they live and toward their preferred temperature (Crawford et al., 1983). Some turtle species bask more than others, either on the shoreline, on floating logs, on debris in the water, and often using aquatic plants to support their weight (Schwarzkopf and Brooks, 1985). Basking in painted turtles differs by age (adults bask more than juveniles) and time of day (concentrating around 10–11 a.m.), but not by sex. In temperate turtles, basking occurs more frequently in the Spring and Fall when the difference between air and water temperature is greatest (Crawford et al., 1983). Still, as the water temperatures warm in the Spring and into the early Summer, basking events are less frequent and shorter in duration, as observed in painted turtles (Lefevre and Brooks, 1995).
Air temperatures differ seasonally in temperate regions, and most temperate turtle species lay eggs only during a restricted breeding season from Spring to Fall (Kennett, 1999), whereas some species and populations living in warmer climates, such as the gopher tortoise (Gopherus polyphemus), breed throughout the year (Allman et al., 2019). Environmental temperature affects the gonadal cycles of turtles, which determines the first date of nesting for a population. Not surprisingly, increased basking events raise body temperature and are associated with earlier nesting (these clutches hatch earlier), as shown in snapping turtles (Chelydra serpentina) (Bobyn and Brooks, 1994). Such earlier nesting in painted turtles increases the likelihood that a female produces a second clutch in the same season (Iverson and Smith, 1993; Krawchuk and Brooks, 1998). Likewise, warmer Springs elicit earlier onset of nesting in both painted and snapping turtles (Obbard and Brooks, 1987; Rowe et al., 2003; Grayson and Dorcas, 2004; Schwanz and Janzen, 2008). Thus, thermal pollution in aquatic systems from power plants (e.g., Crear et al., 2016) and global warming can potentially alter female physiology and nesting phenology (Hedrick et al., 2021). On the other hand, clutches laid too late in the Summer (typically or during protracted seasons due to global warming) may risk failure if temperatures drop below their thermal minimum before embryonic development is completed. Yet some species, such as the striped mud turtle, Kinosternon baurii that lay 1–3 clutches per year, avoid that risk as later-season clutches enter embryonic diapause and resume development in the Spring when temperatures warm (Wilson et al., 1999). However, embryonic diapause is rare among turtles [K. scorpioides cruentatum (Iverson, 2010), Chelodina rugosa (Kennett et al., 1993), perhaps others (Hernández-Montoya et al., 2017)], and thus, restricted to few multiple-clutch producing species as a potential response to climate change.
Where to Nest: Female Nest-Site Choice
Turtles do not nest at random. Instead, natural selection shapes nesting behavior, and adult females select nesting sites that differ in their characteristics (e.g., vegetation cover, soil moisture, distance from water) from random locations (reviewed in Refsnider and Janzen, 2010). Nest-site choice can influence female survival, offspring phenotype, and offspring survival (during embryonic development or post-hatching) (Refsnider and Janzen, 2010) compared to random locations (Hughes and Brooks, 2006). For instance, female striped mud turtles (Kinosternon baurii) choose sites closer to vegetation cover that are 2°C cooler than random sites (Wilson, 1998), while snapping turtles (Chelydra serpentina) select nesting areas with shorter vegetation, more sand, and lacking cacti compared to other available locations (Kolbe and Janzen, 2002). The choices by these two TSD species influenced nest temperatures, potentially sex ratios, and in the case of C. serpentina, the survival probability of hatchlings traveling from their nest to the wetlands (Kolbe and Janzen, 2002). Similarly, some leatherback turtles (Dermochelys coriacea) lay nests close to ocean currents that will guide their offspring to suitable post-hatching habitats (Lohmann et al., 2008). In contrast, in the Australian GSD Murray River Turtle (Emydura macquarii), females select sites based on predation levels, closer to the shore in areas with high predation and further from the beach in low predation areas, thus trading off survival gains from optimal temperatures for development against predation risk (Spencer, 2002; Spencer and Thompson, 2003). This factor is essential because clutches are unprotected after oviposition, and predators (e.g., foxes, raccoons) may raid nests to eat the eggs, sometimes causing 90% loss of nests in a given location (Feinberg and Burke, 2003). This risk is highest soon after oviposition, as observed in diamond-backed terrapins (Malaclemys terrapin), whose nests suffered 71% predation on the first night (Burke et al., 2005). While predator nest detection was attributed to visual cues earlier, recent research demonstrated that predators likely use olfactory cues from disturbed soil to locate the nests, which may decrease in intensity over time, as observed in several turtle species (e.g., Buzuleciu et al., 2016; Edmunds et al., 2018; and references therein). Perhaps the most remarkable nest-site choice is observed in Chelodina rugosa turtles, whose females nest underwater in the inundated billabongs of Australia, where embryos remain in diapause until the substrate dries out and development proceeds (Kennett et al., 1993).
Importantly, females choose oviposition sites that decrease predation risk to themselves during nesting (Rand and Dugan, 1983) while also maximizing the fitness of their clutches as mentioned above. Yet, disturbance experienced by females while nesting can negatively affect their oviposition site choices. For instance, female painted turtles exposed to simulated predation (handled after they started excavating a nest), who were then released and allowed complete nesting, chose new nest sites that suffered higher predation than the first nest site they had selected (Delaney and Janzen, 2020). Although the cause of increased mortality is unknown (distance to water was the same for first and second nest sites) (Delaney and Janzen, 2020), more frequent predator attacks or human disturbances induced by anthropogenic environmental perturbation could lead to maladaptive nesting behavior for offspring survival.
Additionally, the sites a female selects to oviposit may be influenced by the behavior of other females in the population. For instance, social facilitation is an important component in some species, such as the yellow-spotted Amazon river turtle (Podocnemis unifilis). Some females nest in large groups, following other females (perhaps more experienced nesters), particularly during full moon (Escalona et al., 2019), and laying their eggs at nearby sites (Escalona et al., 2009). Social facilitation is adaptive in this species because eggs of females that nest in larger groups suffer lower predation (Escalona et al., 2009). A consequence of this behavior is that clutches may experience comparable environmental conditions resulting in more similar sex ratios than otherwise. Communal nesting may also decrease the risk that females get predated (Doody et al., 2009). In painted turtles, females appeared attracted to olfactory cues left by previous nesting females (tested experimentally using female urine), which they follow by ground-nuzzling, such that clutches may cluster in areas of seemingly uniform characteristics (Iverson et al., 2016). Although nesting painted turtles do not use ground-nuzzling to select oviposition sites based on thermal properties (Morjan and Valenzuela, 2001), their chosen sites may be warmer than random sites (Schwarzkopf and Brooks, 1987), perhaps because females cued on slope or vegetation cover.
Importantly, nest-site choice may have fitness effects after the eggs hatch. A remarkable example comes from species in temperate regions whose hatchlings often overwinter in their nest, such as Chrysemys picta, Graptemys geographica, Trachemys scripta, and some Emys orbicularis (reviewed in Ultsch, 2006; Costanzo et al., 2008 and references therein), where they may be exposed to temperatures below freezing. For instance, vegetation not only cools painted turtle nests during the breeding season by impeding direct solar radiation to reach the nest surface, but also in the Winter, increasing the risk of mortality for these hatchlings when temperatures fall below –8°C (Weisrock and Janzen, 1999) (the lowest value they can survive via supercooling), and affecting their time of emergence in the Spring (Murphy et al., 2020). Hatchlings of pig-nosed turtles (Carettochelys insculpta) delay emergence from their nest via diapause, until the first floods of the wet season indicate improved conditions for survival (Doody et al., 2001). Further, females of several freshwater and sea turtle species return to the same or similar nesting sites yearly when available (Meylan et al., 1990; Lindeman, 1992; Allard et al., 1994; Valenzuela, 2001b; Valenzuela and Janzen, 2001; Morjan, 2003; Freedberg et al., 2005). While such choices may be adaptive and yield high-quality offspring today, they may become detrimental in the future if conditions change over time due to anthropogenic environmental change, and if females do not alter their nesting behavior accordingly.
Factors Influencing Environmental Conditions at the Chosen Nest-Site Locations
The outcomes of female nest-site choices are not only affected by biotic drivers such as predation pressure or vegetation cover, but also by multiple abiotic factors that impose selection due to their effect on nest temperatures and moisture, and thus, on whether developing offspring are exposed to optimal conditions for survival and growth or not. Indeed, the temperature experienced during embryogenesis impacts morphology, physiology, performance, behavior, and survival of turtles (Noble et al., 2018; While et al., 2018), which may be under stronger natural selection than sex ratios of TSD turtles as these effects are profound and long-lasting over the offspring lifetime (Noble et al., 2018). But perhaps embryos are not passive in their phenotypic response to the conditions at the selected nest sites, as documented for several turtles, some crocodilians and snakes, some birds, but not lizards (Li et al., 2014). For instance, embryos of Mauremys reevesii turtles are capable of thermoregulating within the egg in the laboratory in ways that impact their survival and sexual development (Ye et al., 2019). However, because this thermotaxis alters sex ratios only around the pivotal temperature, takes place over a week (during which thermal conditions are likely to change in the wild), and occurs only when a gradient is set between the egg poles, the ecological and evolutionary relevance of this intriguing behavior for sex ratio adjustment remains debatable (While and Wapstra, 2019). But it may be an important means to maintain embryonic development within their thermal tolerance (Zhao et al., 2013).
Air Temperature
The ambient temperature eggs experience depends on many external factors (Czaja et al., 2020), including air temperature, which is influenced by clouds, solar radiation, and time of day. Cloud cover reduces the shortwave solar radiation reaching the ground, leading to a lower maximum daily temperature and increasing the minimum daily temperature by enhancing the longwave radiation (Janzen and Morjan, 2001). Also, air temperature is warmer on average during the day and cooler at night (Pyrgou et al., 2019). Ambient air temperature is a crucial driver of nest temperature because it warms or cools the soil, thus affecting survival and sexual development during the thermosensitive period of TSD taxa such as painted turtles (Janzen, 1994b; Bowen et al., 2005; Warner and Shine, 2011), and it may be a prevalent factor for nests placed in unvegetated areas in many species, e.g., Podocnemis (Escalona and Fa, 1998; Valenzuela, 2001a). Not surprisingly, global warming poses a risk by altering air temperature.
Water Temperature
Water temperature also influences nest temperature, mainly in turtles that nest near the shore (Ackerman, 1997; Rasmussen et al., 2011). Because the nest position in relation to the water table influences the moisture levels in the nest chamber, higher moisture will insulate the soil against rapid air temperature fluctuations (Seybold et al., 2002). This insulation is due to the high specific heat of water which allows it to store more heat before registering temperature changes compared to other substances. In rivers and streams, water temperature correlates strongly to the ambient air temperature in the long term (months, years). In the short term (days, weeks), river and stream temperatures vary substantially due to solar radiation, ambient air temperature, the movement of water flowing over the streambed, and precipitation events (Isaak et al., 2012). Lake temperatures change throughout the year, with streams warming the fastest during the Spring (Woolway and Merchant, 2019). Lake water circulates, allowing for the mixing of water layers with different temperatures. Ice-covered lakes warm faster than the ambient air temperature in the Spring, yet about 10% of lakes, streams, and rivers cool due to increased glacial runoff in the Spring (O’Reilly et al., 2015). These dynamics (combined with air temperature) would affect the conditions experienced by basking females and developing embryos of freshwater turtles living in these habitats. Additionally, the oceans and large lakes act as a heat sink on Earth, absorbing, redistributing, and storing heat over long timescales. Due to the high specific heat of water, oceans lose heat energy slower than air or soil. Most of the heat absorption occurs in the Southern Hemisphere, at a rate four times faster than the Northern Hemisphere (Wijffels et al., 2016). Thus, basking females and developing embryos of sea turtle populations inhabiting Northern and Southern latitudes are expected to be differentially affected by changes in ocean thermal dynamics due to global warming.
Soil Temperature
Several factors affect soil temperature, including ambient air temperature, moisture, solar radiation, and precipitation (Al-Kayssi et al., 1990; Seybold et al., 2002; Parrott and Logan, 2010). Solar radiation occurs only during the daytime but is also very stochastic (Parrott and Logan, 2010). The radiation reaching the soil is affected by cloud cover, vegetation cover, and other abiotic elements within any given day, such that shaded nests are cooler (Weisrock and Janzen, 1999) and would tend to produce more males in TSD Ia taxa, such as painted turtles (Janzen, 1994b). Soil moisture is influenced by rising and falling water tables and increases with proximity to the shore and precipitation events, affecting the temperatures that eggs experience (Kraemer and Bell, 1980; Janzen, 1994a). In spotted turtles (Clemmys guttata), the higher the soil moisture, the more stable and cooler nests are (Al-Kayssi et al., 1990; Ernst and Zug, 1994) because moist soil has a higher specific heat than dry soil and more heat energy is required to warm the nest. Soil types also influence nest temperatures, with some soil types and granule sizes absorbing more moisture/thermal energy than others (Parrott and Logan, 2010; Mitchell and Janzen, 2019). Soil temperature and moisture can also be influenced by supra-annual natural climatic oscillations, such as El Niño events, which reduce average precipitation and increase mean temperature and irradiance, potentially affecting nest temperatures of South American turtles (Valenzuela, 2021). Nest depth is another important factor altering nest temperatures. Deeper nests are cooler than shallower nests and are less affected by extreme temperature fluctuations detrimental to hatchling viability (Valenzuela, 2001a; Marco et al., 2018). For instance, hatching success in diamondback terrapins (Malaclemys terrapin) improved with nest depth (which ranges from 13 to 17 cm) during an unusually hot and dry season, but not during a more favorable season (Czaja et al., 2020). Further, no evidence was detected that females altered their nesting location to improve offspring survival short-term. In contrast, nest depth of shallower nesting species such as painted turtles (7–11 cm) did not affect hatchling sex ratio, survival, or growth (Refsnider et al., 2013). Female limb length and body size constrain the maximum nest depth possibly reached for turtles, as observed in giant Amazonian river turtles, Podocnemis expansa (e.g., Valenzuela, 2001a).
Anthropogenic Challenges to Nesting Behavior
Humans have altered and destroyed natural habitats, leading to a drastic decrease in the land available for nesting (Mainwaring et al., 2017) and forcing some turtle species to tolerate human presence in their nesting habitat. Habitat disturbance includes urban development, which increases absorbed solar radiation and thus, raises the temperature of urban landscapes (Yang et al., 2016). Moreover, urban lights have a disorienting effect on nesting females and hatchling sea turtles (McFarlane, 1963; Perry et al., 2008). Human recreational activity may also alter nesting behavior, as in painted turtles nesting around R.V.’s and campsites (Bowen and Janzen, 2008), leading to higher mortality by vehicles, removal as pets, or exposure to pollutants. Additionally, anthropogenic disturbance may attract predators that raid turtle nests, making predator removal programs necessary in some locations (Wirsing et al., 2012). In some cases, removal of predators from turtle habitats can decrease predation rates by > 50%, significantly improving hatching success (Spencer, 2002).
Adding to the local dangers of human-altered ecosystems is global anthropogenic climate change, which poses another significant threat to turtles, particularly TSD turtles, but could also affect turtles in general. According to NASA’s Goddard Institute for Space Studies (GISS), the average temperature on Earth has increased by 1.0°C since 1880, with 67% of that warming occurring since 1975 at a pace of 0.20°C per decade, on average. The Earth is predicted to warm between 2.0 and 4.5°C by the end of the century (Rogelj et al., 2012). Land air temperatures will increase on average, but with much variability on different spatial and temporal scales across the globe (Ji et al., 2014). Egg and hatchling mortality are expected to increase with increasing global temperature (Santidrián Tomillo et al., 2015; Hays et al., 2017). Increasing temperatures will lead to rising sea levels at frequently used nesting sites of sea turtles due to rising temperatures melting ice caps in the poles, reducing the nesting area of specific beaches by 86% (Patrício et al., 2019). Rising oceans often flood green sea turtle nests, killing the developing embryos as time underwater increases (Fuentes et al., 2010; Mainwaring et al., 2017). This happens when storms increase the wave runup on the shore, and these events can cover a large portion of a nesting area. Rising oceans are predicted to inundate nesting areas of many sea turtle populations, as well as some species inhabiting brackish water, such as the diamondback terrapin (Malaclemys terrapin) (Woodland et al., 2017).
Additionally, fully masculinizing and fully feminizing temperatures in TSD turtles are separated by a few degrees Celsius, called the transitional range of temperatures (TRT), often 1–3°C (Mrosovsky, 1994; Valenzuela and Lance, 2004). For instance, 26°C produce only males and 30°C only females in painted turtles (Valenzuela, 2009). Leatherback sea turtles have a very narrow TRT of about 1°C (Binckley et al., 1998), whereas the TRT of red-eared sliders spans about 2.5°C (Godfrey et al., 2003). Not surprisingly, warming global temperatures raise the thermal profile of nests, often negatively affecting the offspring via sex ratio distortions, developmental abnormalities, and reducing embryonic survival (Janzen, 1994b; Seybold et al., 2002; Telemeco et al., 2013; Hays et al., 2017). Indeed, global warming can potentially cause dangerous feminization of TSD turtle populations (Blechschmidt et al., 2020), as reported in some Australian populations of green sea turtles (Jensen et al., 2018) whose sex ratio reached 99.1% female (99.8% in juveniles and 86.8% in adults). This population has been female-biased for over 20 years and will potentially feminize entirely in the near future. If temperatures continue to warm as predicted, populations of other TSD turtles could eventually become fully feminized, but species with a broader TRT are less vulnerable to such risks because they produce mixed sex ratios over a wider range of temperatures than taxa with a narrow TRT (Hulin et al., 2009). Sex ratio distortion by global warming is not a risk unique to turtles, but one with taxonomically broad impact in other TSD groups and in GSD taxa susceptible to thermal sex reversal (Valenzuela et al., 2003; Edmands, 2021).
And to make things worse, climate change predictions suggest temperature variability within nests will increase in addition to rising averages (Deschênes and Greenstone, 2007; Stouffer and Wetherald, 2007; Neuwald and Valenzuela, 2011; Valenzuela et al., 2019). Temperature variability within nests can accelerate the feminization of TSD turtle populations and cause increased mortality of developing embryos, such as in painted turtles (Valenzuela et al., 2019) and potentially in other shallow nesting taxa, with long-lasting consequences (Noble et al., 2018). In contrast, the observation in Malaclemys terrapin that greater nest depth can protect against extreme and fluctuating temperatures (Czaja et al., 2020), even though nests in this turtle are only 13–17 cm below the surface, suggests that deeper-nesting taxa may be somewhat buffered against climate change. But shallower-nesting taxa, such as painted turtles, whose nests are 7–11 cm below the surface, remain vulnerable to accentuated thermal fluctuations. For green sea turtles and painted turtles, warmer nests also decrease hatchling success, further reducing the species survival (Janzen, 1994a; Cavallo et al., 2015; Laloë et al., 2017; Valenzuela et al., 2019).
TSD and GSD turtles in a warming world may bask and nest earlier in the year (Hedrick et al., 2021), as temperature is a primary factor driving the onset of nesting (Rowe et al., 2003; Bowen et al., 2005). Earlier nesting due to climate change is observed in some turtles but not others (Hedrick et al., 2021), and it might appear as an adaptive response that might mitigate some of the detrimental impacts of climate change on offspring survival and sex ratio for TSD species. Yet, the opposite may be true because earlier nesting and a protracted reproductive season due to global warming can induce females to produce an extra clutch too late in the year for eggs to complete development. The failure of these late-season clutches to develop fully represents a large waste of energy that would be better invested in overwintering and producing the first clutch the following season (Schwanz and Janzen, 2008). Warmer air and water can also influence food availability for reproductive females, and consequently, alter their resource allocation to the eggs in both TSD and GSD turtles (Hedrick et al., 2021).
Climate change will also impact the behavior of marine turtles. Many sea turtles nest in the tropical and subtropical regions, and very rarely in more temperate areas. Over the last 30 years, Kemp’s Ridley sea turtles have shifted their nesting range from these tropical regions to further North away from the equator (Pike, 2013), resulting in cooler nest temperatures than experienced toward the equator, and perhaps more sea turtles will begin nesting further away from the equator. If global warming continues to alter the environment at the pace of these predictions, turtle biodiversity will significantly decrease over the next century, with over 50% threatened with extinction (Stanford et al., 2020). Much has been elucidated about the factors that influence nest temperature and offspring survival, but more questions remain unanswered, as described below.
Can Female Nesting Behavior Respond Adaptively to Anthropogenic Challenges?
Our ability to predict the effects of contemporary climate change on sex ratios of TSD turtles depends on how reliable our models are to explain sexual development under natural nesting conditions (pristine conditions or under climate change scenarios). Many incubation studies have shifted from constant temperature experiments, to simple thermal fluctuating regimes, to replicating natural thermal profiles from nests in ecologically relevant studies (Neuwald and Valenzuela, 2011; Valenzuela et al., 2019; Bowden and Paitz, 2021). Simple fluctuations around a mean temperature that produces only males or only females, if constant, can induce sex reversal in painted turtles from that expected by the mean temperature alone (Neuwald and Valenzuela, 2011). But when the amplitude of fluctuations is accentuated experimentally around naturally fluctuating profiles, only the male-to-female sex reversal is observed around an otherwise male-producing profile, whereas no sex reversal and high mortality are induced by wider fluctuations around an otherwise female-producing profile (Valenzuela et al., 2019). These simplistic and natural fluctuation experiments have demonstrated that high temperatures have greater feminizing potency than the masculinizing potency of low temperatures due to their effect on developmental rate (Georges, 1989; Valenzuela, 2001c; Georges et al., 2005; Valenzuela et al., 2019). Similarly, short periods (days) of increased incubation temperatures (heat waves) have a higher likelihood of feminizing red-eared slider nests compared to nests experiencing more constant conditions (Carter et al., 2018). Findings like these helped develop new models to predict sex ratio responses to naturally fluctuating thermal regimes (Fuentes et al., 2017; Carter et al., 2018, 2020; Valenzuela et al., 2019) and temperature profiles altered by anthropogenic environmental disturbance. Future thermal profiles of nests in the wild will depend on the oviposition site choices made by females in disturbed habitats, such that the following are some outstanding questions that warrant further research.
Although it is clear that female nesting behavior impacts the phenotype (including sex in TSD taxa) and survival of her offspring, the genetic basis of these behaviors remains unclear. Existing data from an Illinois population of painted turtles indicate that the heritability of nest-site choice is low or nil (Morjan, 2003; McGaugh et al., 2010; Janzen et al., 2019; Delaney et al., 2020), and without heritability, adaptive behavioral responses to environmental change are precluded. Furthermore, the evolutionary potential of these nesting behaviors is hampered because nest predation, hatching success, and sex ratio are not repeatable at micro-geographic nesting sites or at vegetation cover types in this painted turtle population (Valenzuela and Janzen, 2001), such that female nest-site choices are overwhelmed by interannual environmental variation in biotic and abiotic conditions. Additionally, these potential responses must offset the lethal incubation temperatures predicted to become more frequent in the wild with climate change (Telemeco et al., 2013; Refsnider and Janzen, 2016; Valenzuela et al., 2019), and which will impact TSD and GSD turtles alike.
An alternative route for adaptation by TSD taxa facing global warming is via the evolution of the reaction norm of sex ratios to temperature (Bull et al., 1982; Valenzuela, 2004). Likewise, evolution of the embryonic thermal tolerance could also help both TSD and GSD turtles respond to climate change for traits other than sexual development that are affected by global warming (Du and Shine, 2015). A caveat is that heritability estimates of the threshold temperature (the inflection value between male and female development) in turtle populations are scarce, limited to painted and snapping turtles, inflated when calculated under constant temperature (Bull et al., 1982), and inflated by confounding maternal effects (Bull et al., 1982; Janzen, 1992; Rhen and Lang, 1998; McGaugh and Janzen, 2011), and potentially by embryonic behavioral thermoregulation (Ye et al., 2019), such that they represent broad sense heritability and not additive genetic variance alone. Nonetheless, narrow sense heritability for threshold temperature, estimated for natural nests of painted turtles, ranges from h2 = 0 to h2 = 0.35 (when including clutches with many or with only fewer fathers, respectively) (McGaugh et al., 2011). Further, broad sense heritability (clutch effects) was detected for the expression of gene regulators of gonadal development in painted turtles (Valenzuela et al., 2013). If these clutch effects reflect additive genetic variation, then natural selection could act on it and drive transcriptional evolution underlying sex determination in the face of climate change. This type of variation may also exist among populations and underpin the differences observed in the reaction norm of TSD within turtle species (Ewert et al., 2004). This interpopulation variation may also counter extinction risk because individuals from populations that produce males at warmer temperatures could colonize areas left open by extirpated populations. However, isolated populations (either due to habitat fragmentation or freshwater turtles and tortoises living on islands) likely cannot alter their home range in response to a warming climate. These species must embrace a decrease in island habitat from rising sea levels and warmer nesting areas (Gibbons et al., 2000). Research is needed to test whether extinction risk differs among turtle species across different geographical locations.
Conclusion
Turtles play essential roles in the ecosystem, occupying many different and unique niches around the world. As these species head toward extinction, their communities will be negatively impacted (Lovich et al., 2018). Further research is urgently needed to fill the gaps in our understanding of the ecology and evolution of nest-site choice, and its evolutionary potential at multiple levels of organization. First, a taxonomic bias exists because relatively few turtle species are the focus of extensive research while a substantial proportion, including some highly endangered taxa, are poorly studied. A bias also exists in how we address the questions of nest-site choice. For instance, here we show that variables not typically considered in the literature on nesting behavior can have profound effects, such as (1) how water temperature impacts basking behavior, an abiotic factor that influences female physiology, which in turn may alter the timing of nesting and resource allocation to the eggs; or (2) how biotic factors such as social facilitation influences nest-site choice; or (3) how water and not just air and soil temperature may affect the conditions experienced by developing embryos in the nest. Filling these gaps is important to assess what are the rules and what are the exceptions in turtle nesting biology, and to predict and evaluate the impact of environmental change. Indeed, anthropogenic climate change is detrimental to animals that have few options to overcome the effects of rising temperatures. TSD turtles have few options to survive highly biased sex ratios and decreased offspring viability in a warming world. And while the general long lifespan of turtles offers some buffering for these biased sex ratios (Sabath et al., 2016) and some heritability exists for adaptation via the threshold temperature for sexual development of TSD embryos, the rate of warming likely exceeds the evolutionary potential of many turtle taxa (Morjan, 2003). But rising temperatures also imperil the survival of GSD turtles because extreme temperatures induce high embryonic mortality, and endocrine disruptors affect sexual development across species irrespective of their sex-determining mechanism, yet little attention has been paid to GSD taxa in these respects. Likewise, predation risks accentuated by anthropogenic disturbance interact negatively with female nesting behavior and clutch survival, both for TSD and GSD turtles. Breeding programs that control the sex of eggs will probably need to be implemented to delay the demise of the most endangered TSD species, but highly imperiled GSD taxa may also need such measures. Ultimately, humans must curb carbon emissions, sequester carbon excess in the atmosphere, mitigate pollution, and ameliorate other environmental disturbances to protect these species and their native habitats, as evolutionary responses alone may not be enough.
Author Contributions
Both authors listed have made a substantial, direct, and intellectual contribution to the work, and approved it for publication.
Funding
This work was funded in part by NSF grant IOS1555999 to NV. Open Access Publication Fees were covered by the Iowa State University Library.
Conflict of Interest
The authors declare that the research was conducted in the absence of any commercial or financial relationships that could be construed as a potential conflict of interest.
Publisher’s Note
All claims expressed in this article are solely those of the authors and do not necessarily represent those of their affiliated organizations, or those of the publisher, the editors and the reviewers. Any product that may be evaluated in this article, or claim that may be made by its manufacturer, is not guaranteed or endorsed by the publisher.
Acknowledgments
We thank LingSze Lee and Thea Gessler for their comments on the manuscript.
References
Ackerman, R. A. (1997). “The nest environment and the embryonic development of sea turtles,” in The Biology of Sea Turtles, eds P. L. Lutz and J. A. Musick (Boca Raton, FL: CRC Press), 83–106.
Al-Kayssi, A., Al-Karaghouli, A., Hasson, A., and Beker, S. (1990). Influence of soil moisture content on soil temperature and heat storage under greenhouse conditions. J. Agric. Eng. Res. 45, 241–252. doi: 10.1016/S0021-8634(05)80152-0
Allard, M. W., Miyamoto, M. M., Bjorndal, K. A., Bolten, A. B., and Bowen, B. W. (1994). Support for natal homing in green turtles from mitochondrial DNA sequences. Copeia 1994, 34–41. doi: 10.2307/1446668
Allman, P., Bowden, R. M., Donini, J., and Serra, I. (2019). Year-round plasma steroid hormone profiles and the reproductive ecology of gopher tortoises (Gopherus polyphemus) at the southernmost edge of their range. Gen. Comp. Endocrinol. 282:113213. doi: 10.1016/j.ygcen.2019.113213
Balshine, S. (2012). Patterns of parental care in vertebrates. Evol. Parent. Care 62:80. doi: 10.1093/acprof:oso/9780199692576.003.0004
Barraza, A. D., Finlayson, K. A., Leusch, F. D., and van de Merwe, J. P. (2021). Systemic review of reptile reproductive toxicology to inform future research directions on endangered or threatened species, such as sea turtles. Environ. Pollut. 286:117470. doi: 10.1016/j.envpol.2021.117470
Bernardo, J. (1996). Maternal effects in animal ecology. Am. Zool. 36, 83–105. doi: 10.1093/icb/36.2.83
Binckley, C. A., Spotila, J. R., WiLsoN, K. S., and Paladino, F. V. (1998). Sex determination and sex ratios of Pacific leatherback turtles, Dermochelys coriacea. Copeia 1998, 291–300. doi: 10.2307/1447425
Bista, B., and Valenzuela, N. (2020). Turtle insights into the evolution of the reptilian karyotype and the genomic architecture of sex determination. Genes 11:416. doi: 10.3390/genes11040416
Blechschmidt, J., Wittmann, M. J., and Blüml, C. (2020). Climate change and green sea turtle sex ratio—preventing possible extinction. Genes 11:588. doi: 10.3390/genes11050588
Bobyn, M. L., and Brooks, R. J. (1994). Interclutch and interpopulation variation in the effects of incubation conditions on sex, survival and growth of hatchling turtles (Chelydra serpentina). J. Zool. 233, 233–257. doi: 10.1111/j.1469-7998.1994.tb08586.x
Booth, D. T. (2017). Influence of incubation temperature on sea turtle hatchling quality. Integr. Zool. 12, 352–360. doi: 10.1111/1749-4877.12255
Bowden, R., Ewert, M., and Nelson, C. (2000). Environmental sex determination in a reptile varies seasonally and with yolk hormones. Proc. R. Soc. Lond. Ser. B Biol. Sci. 267, 1745–1749. doi: 10.1098/rspb.2000.1205
Bowden, R. M., Ewert, M. A., Freedberg, S., and Nelson, C. E. (2002). Maternally derived yolk hormones vary in follicles of the painted turtle, Chrysemys picta. J. Exp. Zool. 293, 67–72. doi: 10.1002/jez.10094
Bowden, R. M., and Paitz, R. T. (2018). Temperature fluctuations and maternal estrogens as critical factors for understanding temperature-dependent sex determination in nature. J. Exp. Zool. Part A Ecol. Integr. Physiol. 329, 177–184. doi: 10.1002/jez.2183
Bowden, R. M., and Paitz, R. T. (2021). Is thermal responsiveness affected by maternal estrogens in species with temperature-dependent sex determination? Sex. Dev. 15, 69–79. doi: 10.1159/000515187
Bowen, K. D., and Janzen, F. J. (2008). Human recreation and the nesting ecology of a freshwater turtle (Chrysemys picta). Chelonian Conserv. Biol. 7, 95–100. doi: 10.2744/CCB-0650.1
Bowen, K. D., Spencer, R.-J., and Janzen, F. J. (2005). A comparative study of environmental factors that affect nesting in Australian and North American freshwater turtles. J. Zool. 267, 397–404. doi: 10.1017/S0952836905007533
Bull, J., Vogt, R. C., and Bulmer, M. (1982). Heritability of sex ratio in turtles with environmental sex determination. Evolution 36, 333–341. doi: 10.1111/j.1558-5646.1982.tb05049.x
Burke, R. L., Schneider, C. M., and Dolinger, M. T. (2005). Cues used by raccoons to find turtle nests: effects of flags, human scent, and diamond-backed terrapin sign. J. Herpetol. 39, 312–315. doi: 10.1670/199-03N
Buzuleciu, S. A., Crane, D. P., and Parker, S. L. (2016). Scent of disinterred soil as an olfactory cue used by raccoons to locate nests of diamond-backed terrapins (Malaclemys terrapin). Herpetol. Conserv. Biol. 11, 539–551.
Carter, A., Sadd, B., Tuberville, T., Paitz, R., and Bowden, R. (2018). Short heatwaves during fluctuating incubation regimes produce females under temperature-dependent sex determination with implications for sex ratios in nature. Sci. Rep. 8, 1–13. doi: 10.1038/s41598-017-17708-0
Carter, A. W., Paitz, R. T., and Bowden, R. M. (2020). Reply to Monsinjon, Girondot, and Guillon. Integr. Comp. Biol. 60, 1351–1354. doi: 10.1093/icb/icaa141
Cavallo, C., Dempster, T., Kearney, M. R., Kelly, E., Booth, D., Hadden, K. M., et al. (2015). Predicting climate warming effects on green turtle hatchling viability and dispersal performance. Funct. Ecol. 29, 768–778. doi: 10.1111/1365-2435.12389
Ceballos, C. P., Hernández, O. E., and Valenzuela, N. (2014). Divergent sex-specific plasticity in long-lived vertebrates with contrasting sexual dimorphism. Evol. Biol. 41, 81–98. doi: 10.1007/s11692-013-9249-0
Charnov, E. L., and Bull, J. (1977). When is sex environmentally determined? Nature 266, 828–830. doi: 10.1038/266828a0
Costanzo, J. P., Lee, R. E. Jr., and Ultsch, G. R. (2008). Physiological ecology of overwintering in hatchling turtles. J. Exp. Zool. Part A Ecol. Genet. Physiol. 309, 297–379. doi: 10.1002/jez.460
Crawford, K. M., Spotila, J. R., and Standora, E. A. (1983). Operative environmental temperatures and basking behavior of the turtle Pseudemys scripta. Ecology 64, 989–999. doi: 10.2307/1937806
Crear, D. P., Lawson, D. D., Seminoff, J. A., Eguchi, T., LeRoux, R. A., and Lowe, C. G. (2016). Seasonal shifts in the movement and distribution of green sea turtles Chelonia mydas in response to anthropogenically altered water temperatures. Mar. Ecol. Prog. Ser. 548, 219–232. doi: 10.3354/meps11696
Czaja, R. A., Scholz, A. L., Figueras, M. P., and Burke, R. L. (2020). The role of nest depth and site choice in mitigating the effects of climate change on an oviparous Reptile. Diversity 12:151. doi: 10.3390/d12040151
Delaney, D. M., Hoekstra, L. A., and Janzen, F. J. (2020). Becoming creatures of habit: among-and within-individual variation in nesting behaviour shift with age. J. Evol. Biol. 33, 1614–1624. doi: 10.1111/jeb.13701
Delaney, D. M., and Janzen, F. J. (2020). Risk-sensitive maternal investment: an evaluation of parent–offspring conflict over nest site choice in the wild. Anim. Behav. 163, 105–113. doi: 10.1016/j.anbehav.2020.03.004
Deschênes, O., and Greenstone, M. (2007). The economic impacts of climate change: evidence from agricultural output and random fluctuations in weather. Am. Econ. Rev. 97, 354–385. doi: 10.1257/000282807780323604
Doody, J. S., Freedberg, S., and Keogh, J. S. (2009). Communal egg-laying in reptiles and amphibians: evolutionary patterns and hypotheses. Q. Rev.Biol. 84, 229–252. doi: 10.1086/605078
Doody, J. S., Georges, A., Young, J. E., Pauza, M. D., Pepper, A. L., Alderman, R. L., et al. (2001). Embryonic aestivation and emergence behaviour in the pig-nosed turtle, Carettochelys insculpta. Can. J. Zool. 79, 1062–1072. doi: 10.1139/z01-061
Du, W. G., and Shine, R. (2015). The behavioural and physiological strategies of bird and reptile embryos in response to unpredictable variation in nest temperature. Biol. Rev. 90, 19–30. doi: 10.1111/brv.12089
Edmands, S. (2021). Sex ratios in a warming world: thermal effects on sex-biased survival, sex determination, and sex reversal. J. Hered. 112, 155–164. doi: 10.1093/jhered/esab006
Edmunds, S. E., Kasparov, C. N., Yoon, J. B., Kanonik, A. K., and Burke, R. L. (2018). Twelve years later: reassessing visual and olfactory cues raccoons use to find diamondback terrapin nests. J. Herpetol. 52, 307–312. doi: 10.1670/17-029
Ernst, C. H., and Zug, G. R. (1994). Observations on the reproductive biology of the spotted turtle, Clemmys guttata, in southeastern Pennsylvania. J. Herpetol. 28, 99–102. doi: 10.2307/1564688
Escalona, T., Adams, D. C., and Valenzuela, N. (2018). A lengthy solution to the optimal propagule size problem in the large-bodied South American freshwater turtle, Podocnemis unifilis. Evol. Ecol. 32, 29–41. doi: 10.1007/s10682-017-9922-3
Escalona, T., and Fa, J. E. (1998). Survival of nests of the terecay turtle (Podocnemis unifilis) in the Nichare-Tawadu Rivers, Venezuela. J. Zool. 244, 303–312. doi: 10.1111/j.1469-7998.1998.tb00034.x
Escalona, T., Valenzuela, N., and Adams, D. C. (2009). Nesting ecology in the freshwater turtle Podocnemis unifilis: spatiotemporal patterns and inferred explanations. Funct. Ecol. 23, 826–835. doi: 10.1111/j.1365-2435.2009.01562.x
Escalona, T., Valenzuela, N., and Adams, D. C. (2019). Do local environmental factors and lunar cycle influence timing and synchrony of oviposition of a turtle with strict nocturnal nesting? Diversity 11:78. doi: 10.3390/d11050078
Ewert, M., Etchberger, C., Nelson, C., Valenzuela, N., and Lance, V. (2004). “Temperature-dependent sex determination in vertebrates,” in Temperature-Dependent Sex Determination in Fishes, eds N. Valenzuela and V. Lance (Washington, DC: Smithsonian Books), 11–20.
Feinberg, J. A., and Burke, R. L. (2003). Nesting ecology and predation of diamondback terrapins, Malaclemys terrapin, at Gateway National recreation Area, New York. J. Herpetol. 37, 517–526. doi: 10.1670/207-02A
Ferrara, C. R., Vogt, R. C., and Sousa-Lima, R. S. (2013). Turtle vocalizations as the first evidence of posthatching parental care in chelonians. J. Comp. Psychol. 127:24. doi: 10.1037/a0029656
Freedberg, S., Ewert, M. A., Ridenhour, B. J., Neiman, M., and Nelson, C. E. (2005). Nesting fidelity and molecular evidence for natal homing in the freshwater turtle, Graptemys kohnii. Proc. R. Soc. B Biol. Sci. 272, 1345–1350. doi: 10.1098/rspb.2005.3080
Fuentes, M., Limpus, C. J., Hamann, M., and Dawson, J. (2010). Potential impacts of projected sea-level rise on sea turtle rookeries. Aquat. Conserv. Mar. Freshw. Ecosyst. 20, 132–139. doi: 10.1002/aqc.1088
Fuentes, M. M., Monsinjon, J., Lopez, M., Lara, P., Santos, A., dei Marcovaldi, M. A., et al. (2017). Sex ratio estimates for species with temperature-dependent sex determination differ according to the proxy used. Ecol. Model. 365, 55–67. doi: 10.1016/j.ecolmodel.2017.09.022
Georges, A. (1989). Female turtles from hot nests: is it duration of incubation or proportion of development at high temperatures that matters? Oecologia 81, 323–328. doi: 10.1007/BF00377078
Georges, A., Beggs, K., Young, J. E., and Doody, J. S. (2005). Modelling development of reptile embryos under fluctuating temperature regimes. Physiol. Biochem. Zool. 78, 18–30. doi: 10.1086/425200
Gibbons, J. W., Scott, D. E., Ryan, T. J., Buhlmann, K. A., Tuberville, T. D., Metts, B. S., et al. (2000). The global decline of Reptiles, Déjà Vu Amphibians: Reptile species are declining on a global scale. Six significant threats to reptile populations are habitat loss and degradation, introduced invasive species, environmental pollution, disease, unsustainable use, and global climate change. Bioscience 50, 653–666. doi: 10.1641/0006-3568(2000)050[0653:TGDORD]2.0.CO;2
Godfrey, M. H., Delmas, V., and Girondot, M. (2003). Assessment of patterns of temperature-dependent sex determination using maximum likelihood model selection. Ecoscience 10, 265–272. doi: 10.1080/11956860.2003.11682773
Gómez-Saldarriaga, C., Valenzuela, N., and Ceballos, C. P. (2016). Effects of incubation temperature on sex determination in the endangered Magdalena river turtle, Podocnemis lewyana. Chelonian Conserv. Biol. 15, 43–53. doi: 10.2744/CCB-1170.1
Grayson, K. L., and Dorcas, M. E. (2004). Seasonal temperature variation in the painted turtle (Chrysemys picta). Herpetologica 60, 325–336. doi: 10.1655/03-43
Hays, G. C., Mazaris, A. D., Schofield, G., and Laloë, J.-O. (2017). Population viability at extreme sex-ratio skews produced by temperature-dependent sex determination. Proc. R. Soc. B Biol. Sci. 284:20162576. doi: 10.1098/rspb.2016.2576
Hedrick, A. R., Greene, D. U., Lewis, E. L., Hood, A. S., and Iverson, J. B. (2021). Climate effects on nesting phenology in Nebraska turtles. Ecol. Evol. 11, 1225–1239. doi: 10.1002/ece3.7105
Hernández-Montoya, V., Páez, V. P., and Ceballos, C. P. (2017). Effects of temperature on sex determination and embryonic development in the red-footed tortoise, Chelonoidis carbonarius. Chelonian Conserv. Biol. 16, 164–171. doi: 10.2744/CCB-1267.1
Hughes, E., and Brooks, R. (2006). The good mother: does nest-site selection constitute parental investment in turtles? Can. J. Zool. 84, 1545–1554. doi: 10.1139/z06-148
Hulin, V., Delmas, V., Girondot, M., Godfrey, M. H., and Guillon, J.-M. (2009). Temperature-dependent sex determination and global change: are some species at greater risk? Oecologia 160, 493–506. doi: 10.1007/s00442-009-1313-1
Isaak, D., Wollrab, S., Horan, D., and Chandler, G. (2012). Climate change effects on stream and river temperatures across the northwest US from 1980–2009 and implications for salmonid fishes. Clim. Change 113, 499–524. doi: 10.1007/s10584-011-0326-z
Iverson, J. B. (1990). Nesting and parental care in the mud turtle, Kinosternon flavescens. Can. J. Zool. 68, 230–233. doi: 10.1139/z90-034
Iverson, J. B. (2010). Reproduction in the red-cheeked mud turtle (Kinosternon scorpioides cruentatum) in southeastern Mexico and Belize, with comparisons across the species range. Chelonian Conserv. Biol. 9, 250–261. doi: 10.2744/CCB-0827.1
Iverson, J. B., Klondaris, H., Angell, C. S., and Tori, W. P. (2016). Olfaction as a cue for nest-site choice in turtles. Chelonian Conserv. Biol. 15, 206–213. doi: 10.2744/CCB-1199.1
Iverson, J. B., and Smith, G. R. (1993). Reproductive ecology of the painted turtle (Chrysemys picta) in the Nebraska Sandhills and across its range. Copeia 1993, 1–21. doi: 10.2307/1446291
Janzen, F. J. (1992). Heritable variation for sex ratio under environmental sex determination in the common snapping turtle (Chelydra serpentina). Genetics 131, 155–161. doi: 10.1093/genetics/131.1.155
Janzen, F. J. (1994b). Vegetational cover predicts the sex ratio of hatchling turtles in natural nests. Ecology 75, 1593–1599. doi: 10.2307/1939620
Janzen, F. J. (1994a). Climate change and temperature-dependent sex determination in reptiles. Proc. Natl. Acad. Sci. U.S.A. 91, 7487–7490. doi: 10.1073/pnas.91.16.7487
Janzen, F. J., Delaney, D. M., Mitchell, T. S., and Warner, D. A. (2019). Do covariances between maternal behavior and embryonic physiology drive sex-ratio evolution under environmental sex determination? J. Hered. 110, 411–421.
Janzen, F. J., and Morjan, C. L. (2001). Repeatability of microenvironment-specific nesting behaviour in a turtle with environmental sex determination. Anim. Behav. 62, 73–82. doi: 10.1093/jhered/esz021
Jensen, M. P., Allen, C. D., Eguchi, T., Bell, I. P., LaCasella, E. L., Hilton, W. A., et al. (2018). Environmental warming and feminization of one of the largest sea turtle populations in the world. Curr. Biol. 28, 154–159.e4. doi: 10.1006/anbe.2000.1732
Ji, F., Wu, Z., Huang, J., and Chassignet, E. P. (2014). Evolution of land surface air temperature trend. Nat. Clim. Change 4, 462–466. doi: 10.1016/j.cub.2017.11.057
Kamel, S. J., and Mrosovsky, N. (2004). Nest site selection in leatherbacks, Dermochelys coriacea: individual patterns and their consequences. Anim. Behav. 68, 357–366. doi: 10.1038/nclimate2223
Kennett, R. (1999). Reproduction of two species of freshwater turtle, Chelodina rugosa and Elseya dentata, from the wet–dry tropics of northern Australia. J. Zool. 247, 457–473. doi: 10.1016/j.anbehav.2003.07.021
Kennett, R., Christian, K., and Pritchard, D. (1993). Underwater nesting by the tropical fresh-water turtle, Chelodina-Rugosa (Testudinata, Chelidae). Austr. J. Zool. 41, 47–52. doi: 10.1017/S0952836999004057
Kolbe, J. J., and Janzen, F. J. (2002). Impact of nest-site selection on nest success and nest temperature in natural and disturbed habitats. Ecology 83, 269–281. doi: 10.1071/ZO9930047
Kraemer, J. E., and Bell, R. (1980). Rain-induced mortality of eggs and hatchlings of loggerhead sea turtles (Caretta caretta) on the Georgia coast. Herpetologica 36, 72–77. doi: 10.1890/0012-9658(2002)083[0269:IONSSO]2.0.CO;2
Krawchuk, M. A., and Brooks, R. J. (1998). Basking behavior as a measure of reproductive cost and energy allocation in the painted turtle, Chrysemys picta. Herpetologica 54, 112–121.
Laloë, J. O., Cozens, J., Renom, B., Taxonera, A., and Hays, G. C. (2017). Climate change and temperature-linked hatchling mortality at a globally important sea turtle nesting site. Glob. Change Biol. 23, 4922–4931.
Lefevre, K., and Brooks, R. J. (1995). Effects of sex and body size on basking behavior in a northern population of the painted turtle, Chrysemys picta. Herpetologica 51, 217–224. doi: 10.1111/gcb.13765
Li, T., Zhao, B., Zhou, Y.-K., Hu, R., and Du, W.-G. (2014). Thermoregulatory behavior is widespread in the embryos of reptiles and birds. Am. Nat. 183, 445–451.
Lindeman, P. V. (1992). Nest-site fixity among painted turtles (Chrysemys picta) in northern Idaho. Northwest. Nat. 73, 27–30. doi: 10.1086/675065
Lohmann, K. J., Putman, N. F., and Lohmann, C. M. (2008). Geomagnetic imprinting: a unifying hypothesis of long-distance natal homing in salmon and sea turtles. Proc. Natl. Acad. Sci. U.S.A. 105, 19096–19101. doi: 10.2307/3536569
Lovich, J. E., Ennen, J. R., Agha, M., and Gibbons, J. W. (2018). Where have all the turtles gone, and why does it matter? Bioscience 68, 771–781. doi: 10.1073/pnas.0801859105
Mainwaring, M. C., Barber, I., Deeming, D. C., Pike, D. A., Roznik, E. A., and Hartley, I. R. (2017). Climate change and nesting behaviour in vertebrates: a review of the ecological threats and potential for adaptive responses. Biol. Rev. 92, 1991–2002. doi: 10.1093/biosci/biy095
Marco, A., Abella, E., Martins, S., López, O., and Patino-Martinez, J. (2018). Female nesting behaviour affects hatchling survival and sex ratio in the loggerhead sea turtle: implications for conservation programmes. Ethol. Ecol. Evol. 30, 141–155. doi: 10.1111/brv.12317
McFarlane, R. W. (1963). Disorientation of loggerhead hatchlings by artificial road lighting. Copeia 1963:153. doi: 10.1080/03949370.2017.1330291
McGaugh, S., and Janzen, F. (2011). Effective heritability of targets of sex-ratio selection under environmental sex determination. J. Evol. Biol. 24, 784–794. doi: 10.1111/j.1420-9101.2010.02211.x
McGaugh, S. E., Bowden, R. M., Kuo, C.-H., and Janzen, F. J. (2011). Field-measured heritability of the threshold for sex determination in a turtle with temperature-dependent sex determination. Evol. Ecol. Res. 13:75. doi: 10.2307/1441283
McGaugh, S. E., Schwanz, L. E., Bowden, R. M., Gonzalez, J. E., and Janzen, F. J. (2010). Inheritance of nesting behaviour across natural environmental variation in a turtle with temperature-dependent sex determination. Proc. R. Soc. B Biol. Sci. 277, 1219–1226.
McKeown, S., Juvik, J., and Meier, D. (1982). Observations on the reproductive biology of the land tortoises Geochelone emys and Geochelone yniphora in the Honolulu Zoo. Zoo Biol. 1, 223–235. doi: 10.1098/rspb.2009.1883
Meylan, A. B., Bowen, B. W., and Avise, J. C. (1990). A genetic test of the natal homing versus social facilitation models for green turtle migration. Science 248, 724–727. doi: 10.1002/zoo.1430010306
Miller, K. (1993). The improved performance of snapping turtles (Chelydra serpentina) hatched from eggs incubated on a wet substrate persists through the neonatal period. J. Herpetol. 27, 228–233. doi: 10.1126/science.2333522
Mitchell, T. S., and Janzen, F. J. (2019). Substrate influences turtle nest temperature, incubation period, and offspring sex ratio in the field. Herpetologica 75, 57–62. doi: 10.2307/1564943
Mitchell, T. S., Maciel, J. A., and Janzen, F. J. (2013). Does sex-ratio selection influence nest-site choice in a reptile with temperature-dependent sex determination? Proc. Biol. Sci. 280:20132460. doi: 10.1655/D-18-00001
Mizoguchi, B. A., and Valenzuela, N. (2016). Ecotoxicological perspectives of sex determination. Sex. Dev. 10, 45–57. doi: 10.1098/rspb.2013.2460
Moore, M. P., Whiteman, H. H., and Martin, R. A. (2019). A mother’smother’s legacy: the strength of maternal effects in animal populations. Ecol. Lett. 22, 1620–1628. doi: 10.1159/000444770
Morjan, C., and Valenzuela, N. (2001). Is ground-nuzzling by female turtles associated with soil surface temperatures? J. Herpetol. 35, 668–672. doi: 10.1111/ele.13351
Morjan, C. L. (2003). How rapidly can maternal behavior affecting primary sex ratio evolve in a reptile with environmental sex determination? Am.Nat. 162, 205–219. doi: 10.2307/1565908
Mu, Y., Zhao, B., Tang, W.-Q., Sun, B.-J., Zeng, Z.-G., Valenzuela, N., et al. (2015). Temperature-dependent sex determination ruled out in the Chinese soft-shelled turtle (Pelodiscus sinensis) via molecular cytogenetics and incubation experiments across populations. Sex. Dev. 9, 111–117. doi: 10.1002/jez.1402700104
Murphy, K. M., Bodensteiner, B. L., Delaney, D. M., Strickland, J. T., and Janzen, F. J. (2020). Nest temperatures predict nest emergence of painted turtle (Chrysemys picta) offspring. Chelonian Conserv. Biol. 19, 72–77. doi: 10.1159/000373903
Neuwald, J. L., and Valenzuela, N. (2011). The lesser known challenge of climate change: thermal variance and sex-reversal in vertebrates with temperature-dependent sex determination. PLoS One 6:e18117. doi: 10.2744/CCB-1391.1
Noble, D. W., Stenhouse, V., and Schwanz, L. E. (2018). Developmental temperatures and phenotypic plasticity in reptiles: a systematic review and meta-analysis. Biol. Rev. 93, 72–97. doi: 10.1371/journal.pone.0018117
Obbard, M. E., and Brooks, R. J. (1987). Prediction of the onset of the annual nesting season of the common snapping turtle, Chelydra serpentina. Herpetologica 43, 324–328. doi: 10.1111/brv.12333
O’Reilly, C. M., Sharma, S., Gray, D. K., Hampton, S. E., Read, J. S., Rowley, R. J., et al. (2015). Rapid and highly variable warming of lake surface waters around the globe. Geophys. Res. Lett. 42, 10,773–10,781.
Parrott, A., and Logan, J. D. (2010). Effects of temperature variation on TSD in turtle (C. picta) populations. Ecol. Model. 221, 1378–1393. doi: 10.1002/2015GL066235
Patrício, A. R., Varela, M. R., Barbosa, C., Broderick, A. C., Catry, P., Hawkes, L. A., et al. (2019). Climate change resilience of a globally important sea turtle nesting population. Glob. Change Biol. 25, 522–535. doi: 10.1016/j.ecolmodel.2010.02.004
Perry, G., Buchanan, B. W., Fisher, R. N., Salmon, M., and Wise, S. E. (2008). Effects of artificial night lighting on amphibians and reptiles in urban environments. Urban Herpetol. 3, 239–256. doi: 10.1111/gcb.14520
Pike, D. A. (2013). Climate influences the global distribution of sea turtle nesting. Glob. Ecol. Biogeogr. 22, 555–566.
Pyrgou, A., Santamouris, M., and Livada, I. (2019). Spatiotemporal analysis of diurnal temperature range: effect of urbanization, cloud cover, solar radiation, and precipitation. Climate 7:89. doi: 10.1111/geb.12025
Radder, R. S. (2007). Maternally derived egg yolk steroid hormones and sex determination: review of a paradox in reptiles. J. Biosci. 32, 1213–1220. doi: 10.3390/cli7070089
Rand, A. S., and Dugan, B. (1983). Structure of complex iguana nests. Copeia 1983, 705–711. doi: 10.1007/s12038-007-0123-z
Rasmussen, A. R., Murphy, J. C., Ompi, M., Gibbons, J. W., and Uetz, P. (2011). Marine reptiles. PLoS One 6:e27373. doi: 10.2307/1444336
Refsnider, J., Bodensteiner, B., Reneker, J., and Janzen, F. (2013). Nest depth may not compensate for sex ratio skews caused by climate change in turtles. Anim. Conserv. 16, 481–490. doi: 10.1371/journal.pone.0027373
Refsnider, J. M., and Janzen, F. J. (2010). Putting eggs in one basket: ecological and evolutionary hypotheses for variation in oviposition-site choice. Ann. Rev. Ecol. Evol. Syst. 41, 39–57. doi: 10.1111/acv.12034
Refsnider, J. M., and Janzen, F. J. (2016). Temperature-dependent sex determination under rapid anthropogenic environmental change: evolution at a turtle’sturtle’s pace? J. Hered. 107, 61–70. doi: 10.1146/annurev-ecolsys-102209-144712
Rhen, T., and Lang, J. W. (1998). Among-family variation for environmental sex determination in reptiles. Evolution 52, 1514–1520. doi: 10.1093/jhered/esv053
Rogelj, J., Meinshausen, M., and Knutti, R. (2012). Global warming under old and new scenarios using IPCC climate sensitivity range estimates. Nat. Clim. Change 2, 248–253. doi: 10.1111/j.1558-5646.1998.tb02034.x
Rollinson, N., and Brooks, R. J. (2008). Optimal offspring provisioning when egg size is “constrained:”: a case study with the painted turtle Chrysemys picta. Oikos 117, 144–151. doi: 10.1038/nclimate1385
Roosenburg, W. M., and Dunham, A. E. (1997). Allocation of reproductive output: egg-and clutch-size variation in the diamondback terrapin. Copeia 1997, 290–297. doi: 10.1111/j.2007.0030-1299.16088.x
Roush, D., and Rhen, T. (2018). Developmental plasticity in reptiles: Critical evaluation of the evidence for genetic and maternal effects on temperature-dependent sex determination. J. Exp. Zool. Part A Ecol. Integr. Physiol. 329, 287–297. doi: 10.2307/1447749
Rowe, J. W. (1994). Egg size and shape variation within and among Nebraskan painted turtle (Chrysemys picta bellii) populations: relationships to clutch and maternal body size. Copeia 1994, 1034–1040. doi: 10.1002/jez.2194
Rowe, J. W., Coval, K. A., and Campbell, K. C. (2003). Reproductive characteristics of female midland painted turtles (Chrysemys picta marginata) from a population on Beaver Island, Michigan. Copeia 2003, 326–336. doi: 10.2307/1446729
Sabath, N., Itescu, Y., Feldman, A., Meiri, S., Mayrose, I., and Valenzuela, N. (2016). Sex determination, longevity, and the birth and death of reptilian species. Ecol. Evol. 6, 5207–5220. doi: 10.1643/0045-8511(2003)003[0326:RCOFMP]2.0.CO;2
Santidrián Tomillo, P., Roberts, S. A., Hernández, R., Spotila, J. R., and Paladino, F. V. (2015). Nesting ecology of East Pacific green turtles at Playa C abuyal, Gulf of Papagayo, Costa Rica. Mar. Ecol. 36, 506–516. doi: 10.1002/ece3.2277
Schwanz, L. E., and Georges, A. (2021). Sexual development and the environment: conclusions from 40 years of theory. Sex. Dev. 15, 7–22. doi: 10.1111/maec.12159
Schwanz, L. E., and Janzen, F. J. (2008). Climate change and temperature-dependent sex determination: can individual plasticity in nesting phenology prevent extreme sex ratios? Physiol. Biochem. Zool. 81, 826–834. doi: 10.1159/000515221
Schwarzkopf, L., and Brooks, R. J. (1985). Application of operative environmental temperatures to analysis of basking behavior in Chrysemys picta. Herpetologica 41, 206–212. doi: 10.1086/590220
Schwarzkopf, L., and Brooks, R. J. (1987). Nest-site selection and offspring sex ratio in painted turtles, Chrysemys picta. Copeia 1987, 53–61.
Seybold, C. A., Mersie, W., Huang, J., and McNamee, C. (2002). Soil redox, pH, temperature, and water-table patterns of a freshwater tidal wetland. Wetlands 22, 149–158. doi: 10.2307/1446037
Shine, R. (1988). Parental care in reptiles. Biol. Reptilia Ecol. B Defense Life History 16, 275–329. doi: 10.1672/0277-5212(2002)022[0149:SRPTAW]2.0.CO;2
Spencer, R.-J. (2002). Experimentally testing nest site selection: fitness trade-offs and predation risk in turtles. Ecology 83, 2136–2144. doi: 10.2307/1445750
Spencer, R. J., and Thompson, M. B. (2003). The significance of predation in nest site selection of turtles: an experimental consideration of macro-and microhabitat preferences. Oikos 102, 592–600. doi: 10.1890/0012-9658(2002)083[2136:ETNSSF]2.0.CO;2
Stanford, C. B., Iverson, J. B., Rhodin, A. G., van Dijk, P. P., Mittermeier, R. A., Kuchling, G., et al. (2020). Turtles and tortoises are in trouble. Curr. Biol. 30, R721–R735. doi: 10.1034/j.1600-0706.2003.12436.x
Stouffer, R., and Wetherald, R. (2007). Changes of variability in response to increasing greenhouse gases. Part I: temperature. J. Clim. 20, 5455–5467. doi: 10.1016/j.cub.2020.04.088
Telemeco, R. S., Warner, D. A., Reida, M. K., and Janzen, F. J. (2013). Extreme developmental temperatures result in morphological abnormalities in painted turtles (Chrysemys picta): a climate change perspective. Integr. Zool. 8, 197–208. doi: 10.1175/2007JCLI1384.1
Tree of Sex Consortium. (2014). Tree of sex: a database of sexual systems. Sci. Data 1:140015. doi: 10.1111/1749-4877.12019
Ultsch, G. R. (2006). The ecology of overwintering among turtles: where turtles overwinter and its consequences. Biol. Rev. 81, 339–367. doi: 10.1038/sdata.2014.15
Valenzuela, N. (2001a). Constant, shift, and natural temperature effects on sex determination in Podocnemis expansa turtles. Ecology 82, 3010–3024. doi: 10.1017/S1464793106007032
Valenzuela, N. (2001b). Genetic differentiation among nesting beaches in the highly migratory giant river turtle (Podocnemis expansa) from Colombia. Herpetologica 57, 48–57. doi: 10.1890/0012-9658(2001)082[3010:CSANTE]2.0.CO;2
Valenzuela, N. (2001c). Maternal effects on life-history traits in the Amazonian giant river turtle Podocnemis expansa. J. Herpetol. 35, 368–378.
Valenzuela, N. (2004). “Evolution and maintenance of temperature-dependent sex determination,” in Temperature Dependent Sex Determination in Vertebrates, eds N. Valenzuela and V. A. Lance (Washington, DC: Smithsonian Institute), 131–147.
Valenzuela, N. (2008). Sexual development and the evolution of sex determination. Sex. Dev. 2, 64–72. doi: 10.2307/1565954
Valenzuela, N. (2009). Egg incubation and collection of painted turtle embryos. Cold Spring Harb. Protoc. 2009:pdb. prot5238. doi: 10.1159/000129691
Valenzuela, N. (2021). Podocnemis expansa turtles hint to a unifying explanation for the evolution of temperature-dependent sex determination in long-lived and short-lived vertebrates. Sex. Dev. 15, 23–37. doi: 10.1101/pdb.prot5238
Valenzuela, N., Adams, D. C., and Janzen, F. J. (2003). Pattern does not equal process: exactly when is sex environmentally determined? Am. Nat. 161, 676–683. doi: 10.1159/000515208
Valenzuela, N., Badenhorst, D., Montiel, E. E., and Literman, R. (2014). Molecular cytogenetic search for cryptic sex chromosomes in painted turtles Chrysemys picta. Cytogenet. Genome Res. 144, 39–46. doi: 10.1086/368292
Valenzuela, N., and Lance, V. (2004). Temperature-Dependent Sex Determination in Vertebrates. Washington, DC: Smithsonian Books. doi: 10.1159/000366076
Valenzuela, N., Literman, R., Neuwald, J. L., Mizoguchi, B., Iverson, J. B., Riley, J. L., et al. (2019). Extreme thermal fluctuations from climate change unexpectedly accelerate demographic collapse of vertebrates with temperature-dependent sex determination. Sci. Rep. 9, 1–11.
Valenzuela, N., Neuwald, J. L., and Literman, R. (2013). Transcriptional evolution underlying vertebrate sexual development. Dev. Dyn. 242, 307–319. doi: 10.1038/s41598-019-40597-4
Valenzuela, N. M., and Janzen, F. J. (2001). Nest-site philopatry and the evolution of temperature-dependent sex determination. Evol. Ecol. Res. 3:779. doi: 10.1002/dvdy.23897
Walde, A. D., Bider, J. R., Masse, D., Saumure, R. A., and Titman, R. D. (2007). Nesting ecology and hatching success of the wood turtle, Glyptemys insculpta, in Quebec. Herpetol. Conserv. Biol. 2, 49–60.
Warner, D. A., and Shine, R. (2011). Interactions among thermal parameters determine offspring sex under temperature-dependent sex determination. Proc. R. Soc. B Biol. Sci. 278, 256–265.
Weisrock, D., and Janzen, F. (1999). Thermal and fitness-related consequences of nest location in painted turtles (Chrysemys picta). Funct. Ecol. 13, 94–101. doi: 10.1098/rspb.2010.1040
While, G. M., Noble, D. W., Uller, T., Warner, D. A., Riley, J. L., Du, W. G., et al. (2018). Patterns of developmental plasticity in response to incubation temperature in reptiles. J. Exp. Zool. Part A Ecol. Integr. Physiol. 329, 162–176. doi: 10.1046/j.1365-2435.1999.00288.x
While, G. M., and Wapstra, E. (2019). Developmental biology: embryonic movement influences sex determination in a turtle. Curr. Biol. 29, R883–R886. doi: 10.1016/j.cub.2019.07.080
Whiteley, S. L., Castelli, M. A., Dissanayake, D. S., Holleley, C. E., and Georges, A. (2021). Temperature-induced sex reversal in reptiles: prevalence, discovery, and evolutionary implications. Sex. Dev. 15, 148–156. doi: 10.1002/jez.2181
Wijffels, S., Roemmich, D., Monselesan, D., Church, J., and Gilson, J. (2016). Ocean temperatures chronicle the ongoing warming of Earth. Nat. Clim. Change 6, 116–118. doi: 10.1159/000515687
Wilson, D. S. (1998). Nest-site selection: microhabitat variation and its effects on the survival of turtle embryos. Ecology 79, 1884–1892. doi: 10.1038/nclimate2924
Wilson, D. S., Mushinsky, H. R., and McCoy, E. D. (1999). Nesting behavior of the striped mud turtle, Kinosternon baurii (Testudines: Kinosternidae). Copeia 1999, 958–968. doi: 10.1890/0012-9658(1998)079[1884:NSSMVA]2.0.CO;2
Wirsing, A. J., Phillips, J. R., Obbard, M. E., and Murray, D. L. (2012). Incidental nest predation in freshwater turtles: inter-and intraspecific differences in vulnerability are explained by relative crypsis. Oecologia 168, 977–988. doi: 10.2307/1447971
Woodland, R. J., Rowe, C. L., and Henry, P. F. (2017). Changes in habitat availability for multiple life stages of diamondback terrapins (Malaclemys terrapin) in Chesapeake Bay in response to sea level rise. Estuar. Coast. 40, 1502–1515. doi: 10.1007/s00442-011-2158-y
Woolway, R. I., and Merchant, C. J. (2019). Worldwide alteration of lake mixing regimes in response to climate change. Nat. Geosci. 12, 271–276. doi: 10.1007/s12237-017-0209-2
Yang, L., Qian, F., Song, D.-X., and Zheng, K.-J. (2016). Research on urban heat-island effect. Proc. Eng. 169, 11–18. doi: 10.1038/s41561-019-0322-x
Ye, Y.-Z., Ma, L., Sun, B.-J., Li, T., Wang, Y., Shine, R., et al. (2019). The embryos of turtles can influence their own sexual destinies. Curr. Biol. 29, 2597–2603.e4. doi: 10.1016/j.proeng.2016.10.002
Keywords: oviposition-site selection, freshwater and marine reptile vertebrate, temperature-dependent sex determination, natural selection and heritability, genotypic sex determination, maternal effects and egg allocation, hatchling success and female fitness, adaptation to climate change
Citation: Topping NE and Valenzuela N (2021) Turtle Nest-Site Choice, Anthropogenic Challenges, and Evolutionary Potential for Adaptation. Front. Ecol. Evol. 9:808621. doi: 10.3389/fevo.2021.808621
Received: 03 November 2021; Accepted: 24 November 2021;
Published: 14 December 2021.
Edited by:
Jeanine M. Refsnider, University of Toledo, United StatesReviewed by:
Lisa Schwanz, University of New South Wales, AustraliaYang Wang, Hebei Normal University, China
Copyright © 2021 Topping and Valenzuela. This is an open-access article distributed under the terms of the Creative Commons Attribution License (CC BY). The use, distribution or reproduction in other forums is permitted, provided the original author(s) and the copyright owner(s) are credited and that the original publication in this journal is cited, in accordance with accepted academic practice. No use, distribution or reproduction is permitted which does not comply with these terms.
*Correspondence: Nicholas E. Topping, dG9wcGluZ0BpYXN0YXRlLmVkdQ==; Nicole Valenzuela, bnZhbGVuenVAaWFzdGF0ZS5lZHU=
†These authors have contributed equally to this work