- 1Cawthron Institute, Nelson, New Zealand
- 2Biosecurity New Zealand, Ministry for Primary Industries, Wellington, New Zealand
- 3Centre for Sustainable Tropical Fisheries and Aquaculture, College of Science and Engineering, James Cook University, Townsville, QLD, Australia
The aquatic ornamental species (AOS) trade is a significant pathway for the introduction and establishment of non-indigenous species into aquatic environments. The likelihood of such occurrences is expected to increase worldwide as industry growth continues and warmer conditions emerge under future climate scenarios. This study used recent (2015 – 2019) New Zealand importation data to determine the composition, diversity, abundance, and arrival frequency of AOS. Our analysis revealed that ca. 300,000 aquatic ornamental individuals are imported annually to New Zealand, with freshwater fish comprising 98% of import quantities. Despite the relatively small market size, the estimated AOS diversity of 865 taxa (89 and 9.5% identified to species and genus level, respectively) is comparable to larger markets with ∼60% of taxa being of marine origin. Species (n = 20) for further investigation were prioritized based on quantity and frequency of import. These prioritized AOS were exclusively tropical and subtropical freshwater fish and align with the most frequently imported AOS globally, including the top three: neon tetra (Paracheirodon innesi), guppy (Poecilia reticulata), and tiger barb (Puntigrus tetrazona). Species distribution modeling of the 20 prioritized AOS predicted that 13 species are suitable for New Zealand’s current climate conditions, most notably sucker-belly loach (Pseudogastromyzon myersi), white cloud mountain minnow (Tanichthys albonubes), and golden otocinclus (Macrotocinclus affinis). Potential changes in habitat suitability were predicted under future climate scenarios, with largest increases (29%) for Po. reticulata. The described approach provides an adaptable framework to assess establishment likelihood of imported AOS to inform regulatory decision making.
Introduction
Maintaining aquatic animals and plants is a centuries-old hobby with an ever-increasing popularity and global reach (Bruckner, 2005; Brunner, 2012). The modern aquatic ornamental species (AOS) trade – including bony fish, crustaceans, mollusks, corals, and associated equipment – has grown to a US$15–30 billion per year industry (Penning et al., 2009; Raja et al., 2019). Growth of this sector has continued despite global fluctuations in trade practices and difficulties in characterizing the economic scale of the industry (Andrews, 1990; Rhyne et al., 2012; Biondo and Burki, 2020). However, the popularity of aquatic pets has also led to increased scrutiny of the industry’s impact, both positive and negative, on source and destination regions, and for industry’s handling, processing, and distribution of biota (Rhyne et al., 2017). Research outputs regarding the trade of AOS have also grown considerably over the past three decades (Figure 1), highlighting environmental and economic costs and benefits to communities – often comprised of developing countries or remote areas. Research topics include consideration of a range of habitat impacts, species conservation issues, industry practices for wild and cultured stocks, societal education and enjoyment, and the roles and responsibilities of industry and regulatory policy (Green, 2003; Penning et al., 2009; Prakash et al., 2017; Rhyne et al., 2017; Evers et al., 2019; Trujillo-González and Militz, 2019).
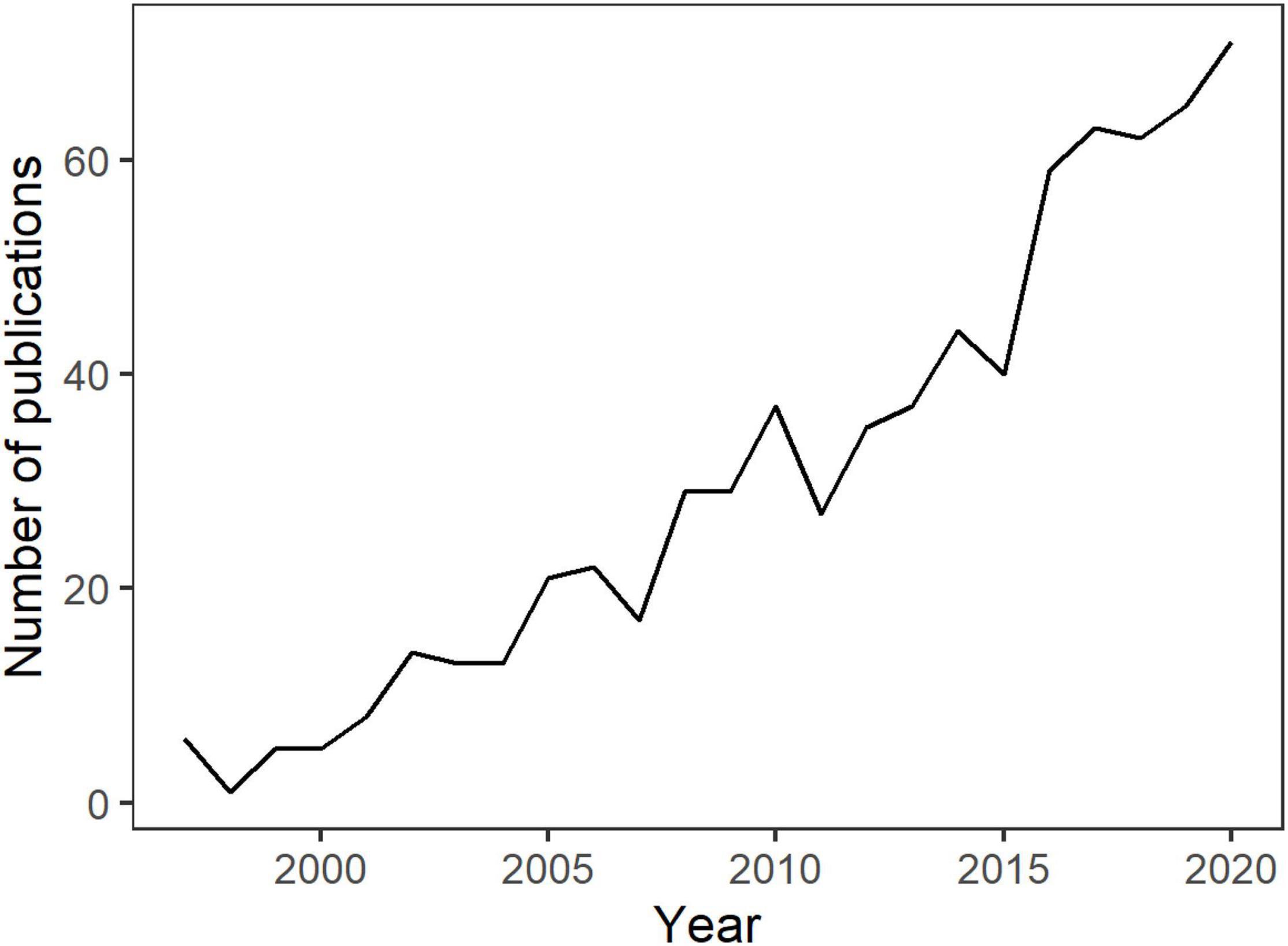
Figure 1. Number of publications per year recorded in the Web of Science databases between 1995 and 2020 using the search terms: “aquarium trade” and “aquatic ornamental trade”.
One major implication of a global industry in AOS is its role in global patterns of biotic exchange and the resulting impacts (Padilla and Williams, 2004). Release of AOS is a well acknowledged pathway of non-indigenous species introduction, establishment, and associated impacts for many regions, particularly for freshwater systems (Semmens et al., 2004; Copp et al., 2007; Nunes et al., 2015; García-Díaz et al., 2018). Several high-profile species “invasions” have resulted via this pathway, including: the wide ranging distributions of goldfish and ornamental carp (Andrews, 1990; Koehn and MacKenzie, 2004; Beatty et al., 2017); lionfish in coastal environments of the Western Atlantic (Morris and Whitfield, 2009); Caulerpa taxifolia in the Mediterranean (Thibaut and Meinesz, 2004), Australia (Glasby, 2013), and California (Williams and Grosholz, 2002); and a range of prominent freshwater plants (Champion and Clayton, 2000; Kay and Hoyle, 2001; Martin and Coetzee, 2011). In addition to widespread or high impact species, the cumulative and ongoing effect of established assemblages of AOS in the wild includes co-invasion of pathogens and parasites and changes to community distinctness among water bodies (Whittington and Chong, 2007; Magalhães et al., 2020). These may contribute to broader impacts on ecosystem processes in aquatic ecosystems (Capps and Flecker, 2013), as well as cascade effects on terrestrial ecosystems and livestock production (Carolus et al., 2019). Meanwhile altered environmental conditions due to climate change can facilitate or restrict AOS establishment and associated consequences (Stefan et al., 2001; Howeth et al., 2016).
The AOS trade is a challenging biosecurity risk pathway to manage due in part to the scale and dynamism of international trade (Reid et al., 2019) and wide-ranging diversity of species in flux. In addition, the end-user population is large and diffuse and has a range of motivations that can lead to environmental release (Duggan et al., 2006). Policy mechanisms to reduce the numbers of introductions from this pathway have long been advocated (Andrews, 1990) and are implemented to varying degrees around the world (Chan et al., 2019). Regulatory frameworks include risk assessment procedures, controls on importation of species using prohibited species lists (‘blacklists’), permitted species lists (‘whitelists’), and release bans on species already imported (Chan et al., 2019). In New Zealand, lists of approved aquatic species for importation have been maintained and refined since 1972 (Biosecurity New Zealand, 2009). An import health standard for aquatic ornamental species was first issued in 2002 under the 1993 Biosecurity Act, this was further refined based on the risk assessments of Hine and Diggles (2005) and Biosecurity New Zealand (2009), and a subsequent review in 2016 (Ministry for Primary Industries, 2016). This ‘whitelist’ approach to manage the range of AOS that can be imported is arguably the strongest and most centralized node of control for this pathway, providing an avenue for subsequent assessments regarding the likelihood of establishment and associated consequences.
The aim of this study was to use New Zealand’s recent import history to prioritize AOS for assessment of establishment likelihood based on temperature tolerance and current and future climate conditions. The approach taken was based on: (i) a combination of the analysis of import quantities and frequency, (ii) the identification of temperature tolerance for priority species through a literature review, and (iii) species distribution modeling to determine current and future habitat suitability. Such an approach provides an adaptable framework which can be used to inform regulatory decision making regarding the AOS pathway (Whittington and Chong, 2007).
Materials and Methods
Data Capture and Compilation
Available data for all aquatic ornamental species (AOS) imported to New Zealand from 2015 to 2019 (inclusive) were extracted from the invoices that accompanied each consignment for import verification purposes. Appropriate permission to access and use the data was obtained from New Zealand’s Ministry for Primary Industries (MPI). A total of 284 PDF consignment invoices containing AOS names and quantities was converted by MPI into a spreadsheet format with all sensitive information removed. These data were compiled and supplied by MPI to enable analyses of the total number of individuals for each imported species (i.e., import quantity) and the number of times that species had entered New Zealand (i.e., import frequency) in each of the 5 years.
All entries of the scientific name as deduced from consignment invoices were initially reviewed to identify typographic errors and inaccuracies and were manually modified to match the most likely correct scientific name reported in FishBase (Froese and Pauly, 2021). Following some initial grooming of entries, distinct putative taxa were compared to taxonomic databases using the taxize package (Chamberlain and Szöcs, 2013) in the software R (R Core Team, 2021). In the first step, taxonomic names were resolved, to obtain the correct spelling and most up to date names, by querying taxa against the Catalog of Life, World Register of Marine Species (WoRMS), and Global Biodiversity Information Facility (GBIF) backbone taxonomy databases using the taxize function gnr_resolve (Bisby et al., 2010; GBIF Secretariat, 2015; WoRMS Editorial Board, 2020). Following the grooming and name-matching process, taxonomic classification of all distinct putative taxa names was further interrogated using the GBIF taxonomic database (GBIF Secretariat, 2015) to identify accepted taxa, synonyms (which were corrected and merged), and any doubtful taxa. Separate investigations were conducted for unmatched/doubtful taxa by searching online taxonomic databases (e.g., WoRMS, Web of Science, and Google) with the most plausible valid taxon included in the groomed data set. The resulting dataset containing quantity and frequency data for each accepted species was evaluated to identify trends. Eighty-three taxa (9.6% of all taxa) that were not recorded to species level were excluded because higher level classification was not suited to subsequent species-specific analyses of temperature tolerance and habitat suitability. A prioritized list of species (n = 20) was developed using a basic, unweighted summation of quantity and frequency rankings over the 5-year study period. This is similar to a consideration of propagule pressure, a key factor for the understanding of introduction and establishment risk (Lockwood et al., 2005; Duggan et al., 2006; Leung and Mandrak, 2007).
Temperature Tolerance of Prioritized Aquatic Ornamental Species
Temperature tolerance of prioritized AOS (all freshwater species) was assessed using a structured literature review. Firstly, species were matched with their temperature ranges reported from FishBase (Froese and Pauly, 2021). Web of Science (all databases, 1980–2020) was then used to search for relevant data using the steps described in Supplementary Material 1. The Globtherm database (Bennett et al., 2018) was also used to search for relevant data, however, no additional records were found.
The structured literature search resulted in 360 papers for screening (using title and/or abstract). The inclusion criteria for screening are described in Supplementary Material 1. From these 360 papers, 115 papers were more thoroughly assessed (using text, figures, or tables). Overall, 71 studies yielded 84 rows of data on high and low temperatures for 15 of the 20 prioritized species. There were no data for five of the species. The available data were collated to provide a maximum and minimum temperature for each species, noting that in some cases only one datum was available. For each species with data, temperature ranges were plotted for comparison to water temperatures in New Zealand and the FishBase-reported temperature range.
Species Distribution Modeling of Prioritized Aquatic Ornamental Species
Species distribution modeling (SDM) was used to predict habitat suitability of prioritized AOS using ‘maximum entropy’ modeling (MaxEnt, Phillips et al., 2006). MaxEnt is a machine learning algorithm for estimating species distribution subject to constraints of incomplete information. MaxEnt models are fitted by combining occurrence records and climate predictor layers and can be fitted using presence-only data, such as species’ occurrence records. Model results were presented in logistic format showing areas with lower and higher probabilities of species occurrence (i.e., 0–1), allowing identification of establishment likelihood hotspots and quantification of total suitable habitat for each species.
In February 2021, global occurrence records for each species were obtained from GBIF.org using the R library ‘rgbif’ (Chamberlain and Boettiger, 2017). GBIF is the largest source of primary biodiversity data, providing access to occurrence records obtained from worldwide sources including museums, research projects, scientific literature, naturalists, and networks of observers. Although there are some challenges associated with the GBIF database (Anderson et al., 2016), including the varying quality of records, it provides an invaluable source of standardized occurrence data at a global scale that can be readily accessed, downloaded, and used in SDMs. Occurrence records were checked for consistency, with duplicate, implausible (e.g., incorrect habitat or extreme latitudes), old (before 1900), and non-georeferenced data removed. Sample sizes for occurrence records were generally well above the minimum of 13 required by MaxEnt (van Proosdij et al., 2016, Supplementary Material 1, mean = 487, max = 3,139 and min = 14).
Current environmental layers (1970–2000) included in the models were altitude, maximum temperature of the warmest month, minimum temperature of the coldest month, and annual precipitation. Layers had a spatial resolution of 30 arc s (∼1 km2). These were obtained from the WorldClim database (version 2.1) accessed through the library ‘sdmpredictors’ (Bosch et al., 2018) of the software R (R Core Team, 2021). Climate predictors (air temperature and annual precipitation) were used as proxies for instream hydrological conditions, as they are known to provide comparable modeling results for aquatic organisms (McGarvey et al., 2018). Predictor layers were considered non-collinear based on pairwise Pearson’s correlation coefficients < 0.8. A random sample (20%) of the species’ occurrence data was withheld and used to test the validity and accuracy of the training model. Response curves, jack-knife measures of variable importance, and area under curve (AUC) were used to interpret and evaluate the models. Final MaxEnt models were then used to predict habitat suitability for each prioritized species throughout New Zealand. The maximum test sensitivity plus specificity value was used as a threshold to cut-off the continuous logistic probability into a binary variable (Liu et al., 2011). This was used to calculate the proportion of suitable habitat for each species in relation to the total area of New Zealand for which habitat suitability estimates were predicted.
Cross-validation showed that the models were quite robust, with all training models having high AUC values (mean: 0.94, range: 0.82 – 0.99, Supplementary Material 3), indicative of good fit, which were similar to models fitted on the test data (mean AUC: 0.92, range: 0.81 – 0.99, Supplementary Material 3). Furthermore, predicted habitat suitability for all modeled species was highly correlated across replicated models using training datasets (r > 0.93) and similarly high between replicated training models and those fitted with all occurrence records (r > 0.95). Models were subsequently built using all the occurrence records to maximize data availability.
Changes in habitat suitability for prioritized AOS under future climate scenarios were predicted using SDM with bioclimatic layers projected according to the framework of the World Climate Research Programme’s Coupled Model Intercomparison Project Phase 5 (CMIP5, Taylor et al., 2012). Bioclimatic projections were obtained for 2050 and 2070 for the least and most extreme (‘best’ and ‘worst’) climate change scenarios, out of the four main predictions adopted by the Intergovernmental Panel on Climate Change (Moss et al., 2010; Van Vuuren et al., 2011). These scenarios include one that requires a reduction in atmospheric greenhouse gas concentrations [Representative Concentration Pathway (RCP) 2.6], and another that is ‘business as usual’ resulting in very high greenhouse concentrations (RCP8.5). Future climate predictions were based on three different General Circulation Models (GCMs) to incorporate variability and uncertainty associated to different GCMs and the potential impact on future species distributions predictions. These were the Hadley Centre Global Environmental Model 2-Earth System model (HadGEM2-ES, Collins et al., 2011), Model for Interdisciplinary Research On Climate – Earth System Model (MIROC-ESM, Watanabe et al., 2011) and the fourth version of the Community Climate System Model (CCSM4, Gent et al., 2011).
Results
Aquatic Ornamental Species Imports
The summary data obtained from the consignments included 1,694 putative taxa names associated with AOS imports from 2015 to 2019 inclusive. Initial grooming and matching resulted in 995 unique taxa. Further interrogation using the GBIF database revealed 820 accepted taxa, 169 synonyms, and eight doubtful taxa. Resolving the taxonomic classification, including merging synonymized taxa, doubtful classification taxa, correcting misclassified taxa (i.e., outside Animalia), and fuzzy matching, resulted in 865 accepted taxa. Of these, 782 were classified to species level, 82 were to genus level, and one to order level (Figure 2). Fish belonging to the genus Ancistrus comprised 53% of import quantity of taxa identified to genus level. The import dataset can be interactively visualized and explored at https://cawthron.shinyapps.io/aquatic_ornamental_species/.
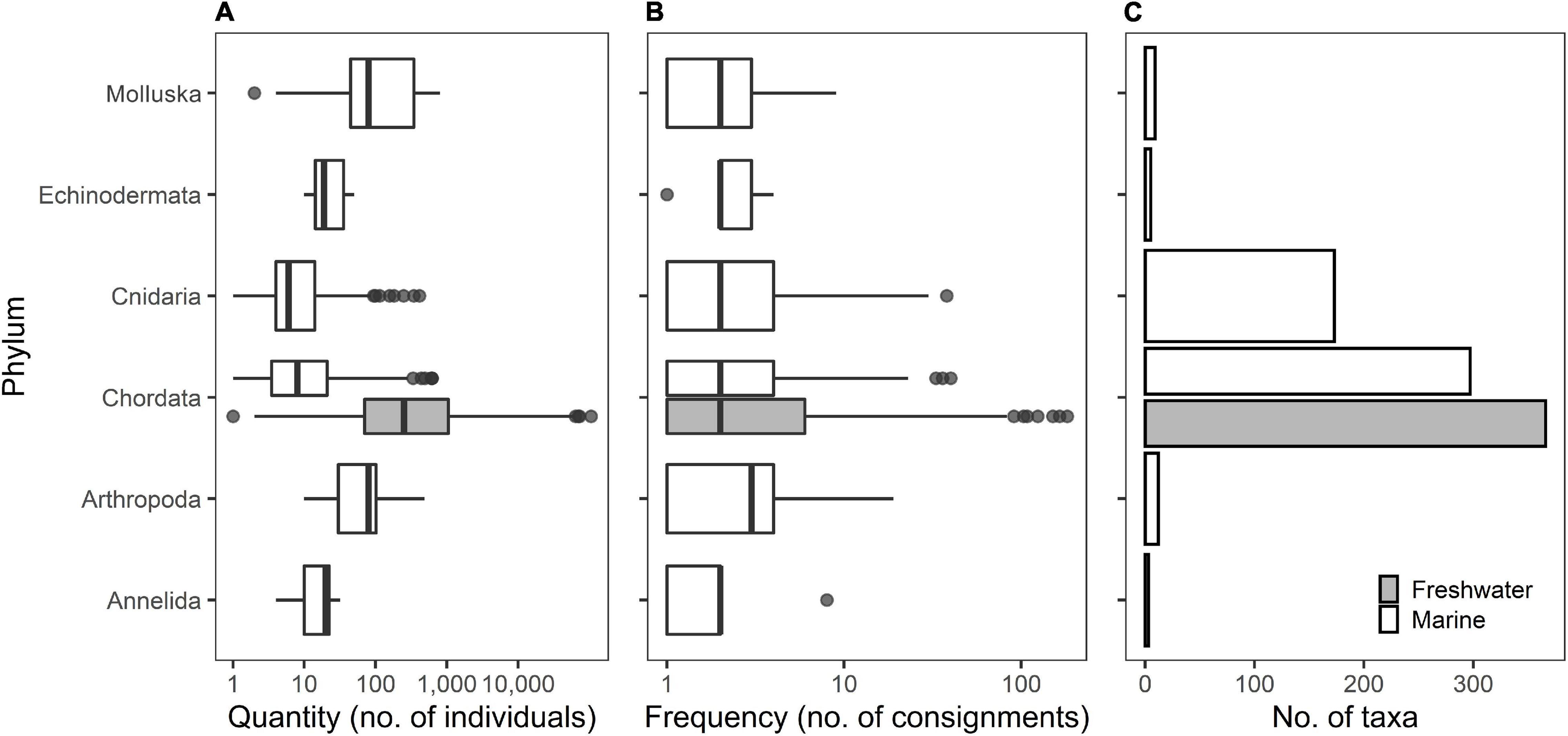
Figure 2. Box plots of quantity (A) and frequency (B), and bar plot of number of taxa (C) of imported aquatic ornamental species by phyla into New Zealand from 2015 to 2019 (inclusive). Lines in boxes are medians, box ends are lower and upper quartiles, and whiskers extend to the lowest or highest data point ≤1.5 the interquartile range. Black dots are outliers. Note that boxplots of quantity and frequency are in a log scale. Shading to indicate freshwater data applies to panels (A–C).
From the available data, a total of 1,479,952 AOS individuals were imported to New Zealand between 2015 and 2019 in 11,526 separate consignments. On average 295,990 (±35,163 S.E., range: 262,222 – 435,694) AOS individuals were imported each year, with an annual average import frequency of 2,305 consignments (±175, range: 1822 – 2880). Most consignments originated from Singapore (66.4%), followed by Indonesia (12.9%), Sri Lanka (4.6%) and 13 other countries with <4% of the total number of consignments. AOS imports included species from six different phyla and were dominated by phylum Chordata (hereafter referred to as fish) both in terms of number of taxa (77%), quantity (99% of imported individuals), and frequency (89% of records). The second most popular phylum was Cnidaria (mainly coral colonies) which represented 20% of the total number of taxa, 0.3% of the quantity, and 9.3% of the frequency of imports. Other imported phyla include Arthropoda, Mollusca, Echinodermata, and Annelida, which, respectively, comprised <1.5% of the number of taxa, quantity, and frequency of imports (Figure 2). No freshwater invertebrates were recorded which is not unexpected as they are excluded by New Zealand’s import health standard (Ministry for Primary Industries, 2021).
From the total of 865 taxa, 499 comprised marine (57.7%), 361 freshwater (41.7%), and five (0.6%) were either anadromous or catadromous taxa. Despite the larger diversity of marine taxa, the quantity of imports was heavily dominated by freshwater taxa (97.5%). Frequency of imports was more balanced between freshwater (62.7%) and marine taxa (36.9%). Fish were largely dominated by freshwater species, in terms of quantity (98.3%) and frequency (70.6%), but taxa richness was comparable between marine and freshwater fish, with 366 and 297 species, respectively (Figure 2C). The neon tetra, Paracheirodon innesi, was the species imported in largest quantities, with an annual mean of 74,210 (±8,462 S.E., Figure 3) imported individuals, nearly five-fold higher than the second most imported species, the tiger barb Puntigrus tetrazona (15,199 ± 1,386), and seven-fold higher that the third species, the guppy Poecilia reticulata (10,156 ± 3,125). The Siamese fighting fish Betta splendens was the most frequently imported species (140 ± 17 consignments per year, Figure 3), followed by dwarf gourami Trichogaster lalius (72 ± 11) and Pa. innesi (66 ± 11). The prioritized species list derived from the summary statistics for import quantity and frequency showed that 97 of the top 100 species were fish. The exceptions were the marine cleaner shrimp Lysmata amboinensis, the hermit crab Dardanus gemmatus, and the star snail Lithopoma tectum.
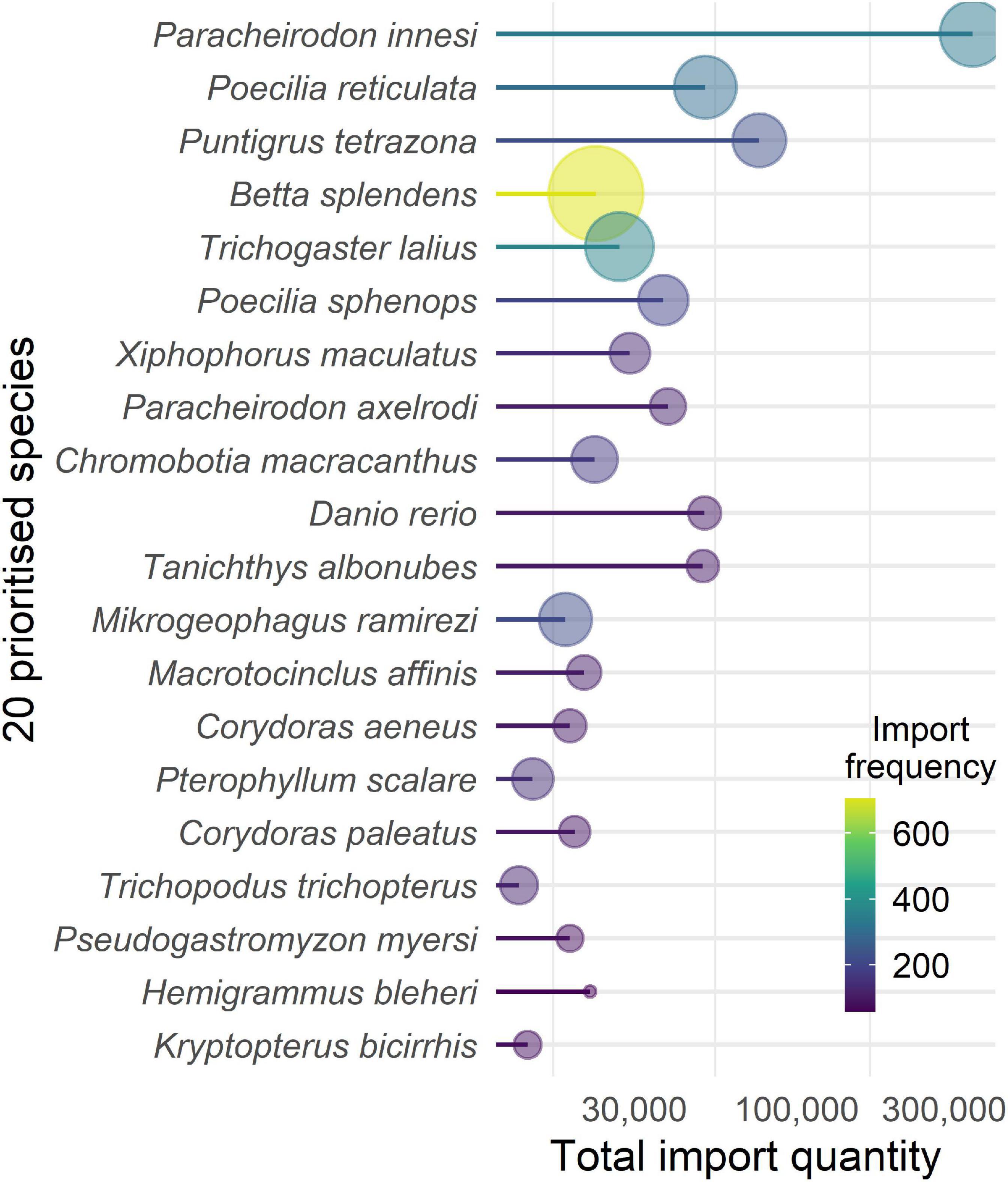
Figure 3. The 20 prioritized aquatic ornamental species imported to New Zealand from 2015 to 2019 (inclusive) derived from, and ordered by, summed ranks of quantity and frequency. Total quantity (x-axis) and frequency (color scale and bubble size) is shown for each species.
Temperature Tolerance of Prioritized Aquatic Ornamental Species
Temperature tolerance data from the literature provided temperature ranges for 14 species and a single temperature from a constant temperature experiment for one species (Hemigrammus bleheri). The reviewed literature reported more critical maximum temperatures (n = 31) than critical minima (19). This was mainly due to many studies of the zebra fish, Danio rerio, a model organism for physiological research. Temperature ranges collated from literature studies were broader than those reported in FishBase in all of 13 cases for which there were comparable data (Figure 4). FishBase temperature ranges are largely derived from aquarist textbooks and appear to provide optimal tank water temperatures for home hobbyists. There were two cases where the FishBase temperature range was not completely overlapped by the literature range, in these cases the literature data did not include a critical minimum temperature (for Pa. innesi) or a maximum temperature (for Pu. tetrazona). Ten of the species tolerance ranges overlapped with freshwater (riverine) water temperatures reported in New Zealand (Figure 4), including one that extended the full range of New Zealand temperatures (Corydoras paleatus). Several species had a FishBase low temperature tolerance of 18°C, including Po. reticulata, Poecilia sphenops, Xiphophorus maculatus, D. rerio, T. albonubes, and Co. paleatus, matching that of New Zealand’s highest water temperatures (18.2°C). There were no data for Ma. affinis. While eight species had low temperature tolerances that exceeded New Zealand’s highest water temperatures by 1.8–8.8°C, there was a paucity of temperature tolerance information for each of these species (Figure 4).
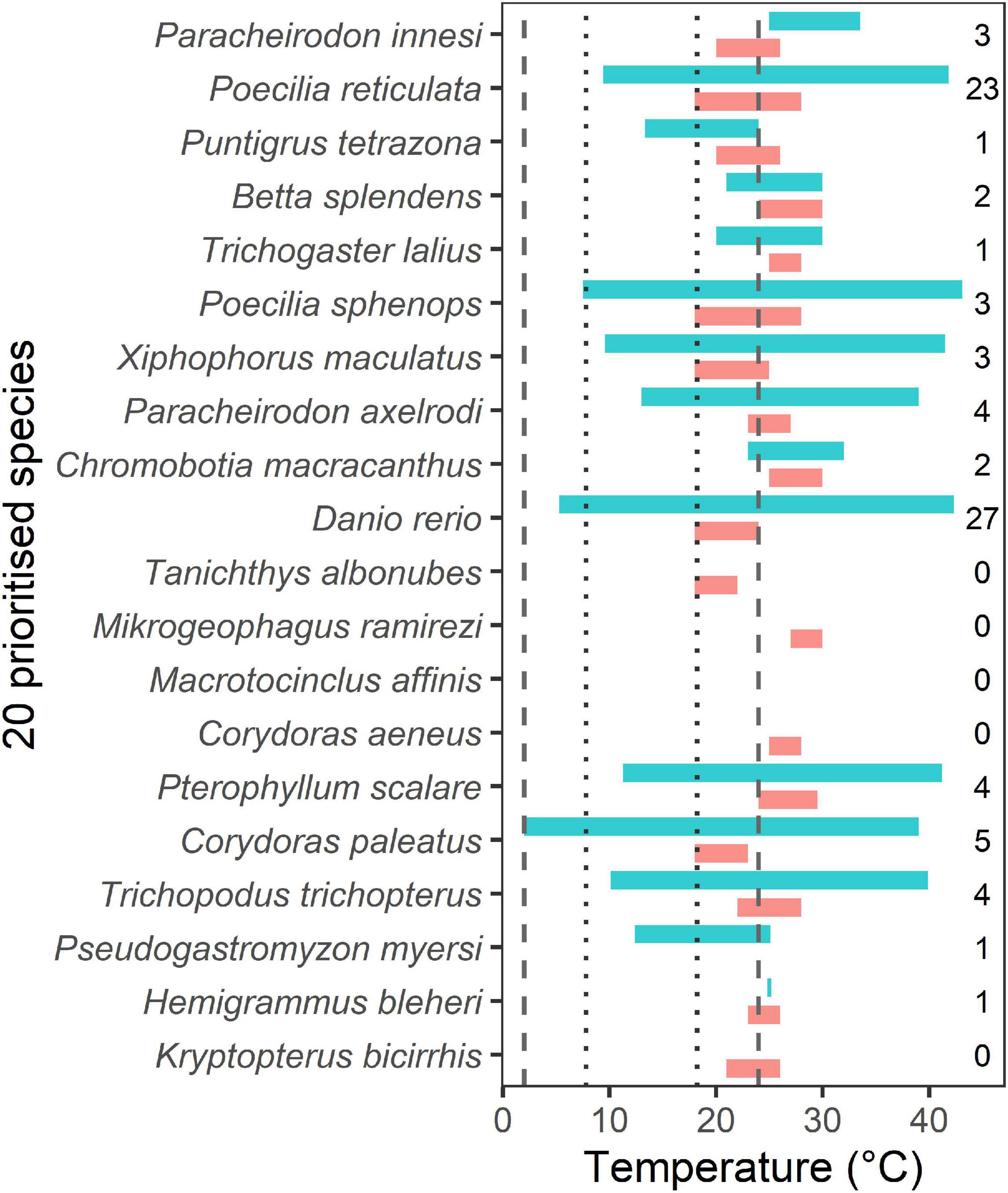
Figure 4. Temperature tolerance ranges for the 20 prioritized aquatic ornamental species imported to New Zealand. Data are from FishBase (red bars) and structured literature searches (blue bars). The numbers of relevant studies from which the data was derived are shown on the right. The ranges shown for literature-based data include the maximum and minimum temperatures from all studies assessed for each species, regardless of methods and locations (or fish populations). The dotted and dashed lines show the temperature range of New Zealand’s riverine waters (Scarsbrook, 2006) and lake waters (Hamilton et al., 2012; Jones and Hamilton, 2014), respectively.
Species Distribution Modeling of Prioritized Aquatic Ornamental Species
Occurrence records showed that all modeled AOS are distributed outside of New Zealand, with the exception of one record for Po. reticulata (Supplementary Material 2). Models predicted that 13 of the 20 prioritized species were suited to New Zealand habitats under current conditions (Figure 5). Highest habitat suitability was predicted for sucker-belly loach (Pseudogastromyzon myersi, 88.4% of suitable habitat in relation to New Zealand’s total surface, Figure 5), closely followed by the cloud mountain minnow (Ta. albonubes, 81.9%), the golden otocinclus (Ma. affinis, 78.1%, Figure 5), and the tiger barb (Pu. tetrazona, 53.0%, Figure 5). By contrast, New Zealand’s habitat was predicted as unsuitable for seven species: Pa. innesi, Po. sphenops, Mikrogeophagus ramirezi, Pterophyllum scalare, H. bleheri, Kryptopterus bicirrhis, and B. splendens (Figure 5). Three species – cardinal tetra Paracheirodon axelrodi, three spot gourami Trichopodus trichopterus, and D. rerio – were predicted to have <2% suitable habitat (Figure 5). The most important predictor variables across species were altitude (mean importance 28.9%), annual precipitation (27.6%), minimum temperature (23.9%), and maximum temperature (19.5%, Figure 6). Altitude was particularly important for clown loach Chromobotia macracanthus and Ma. affinis models, whereas maximum temperature was a driver of distribution predictions for Mi. ramirezi, Ta. albonubes, and Pu. tetrazona (Figure 6). Minimum temperature was important for Co. paleatus, Po. reticulata, and X. maculatus, and annual precipitation for Pa. axelrodi and D. rerio (Figure 6). Although the literature review revealed a large range of temperature tolerance for D. rerio that overlapped with water temperatures in New Zealand, SDMs did not predict a broad range of suitability for this species since annual precipitation was a main driver of its predicted occurrence (Figure 6).
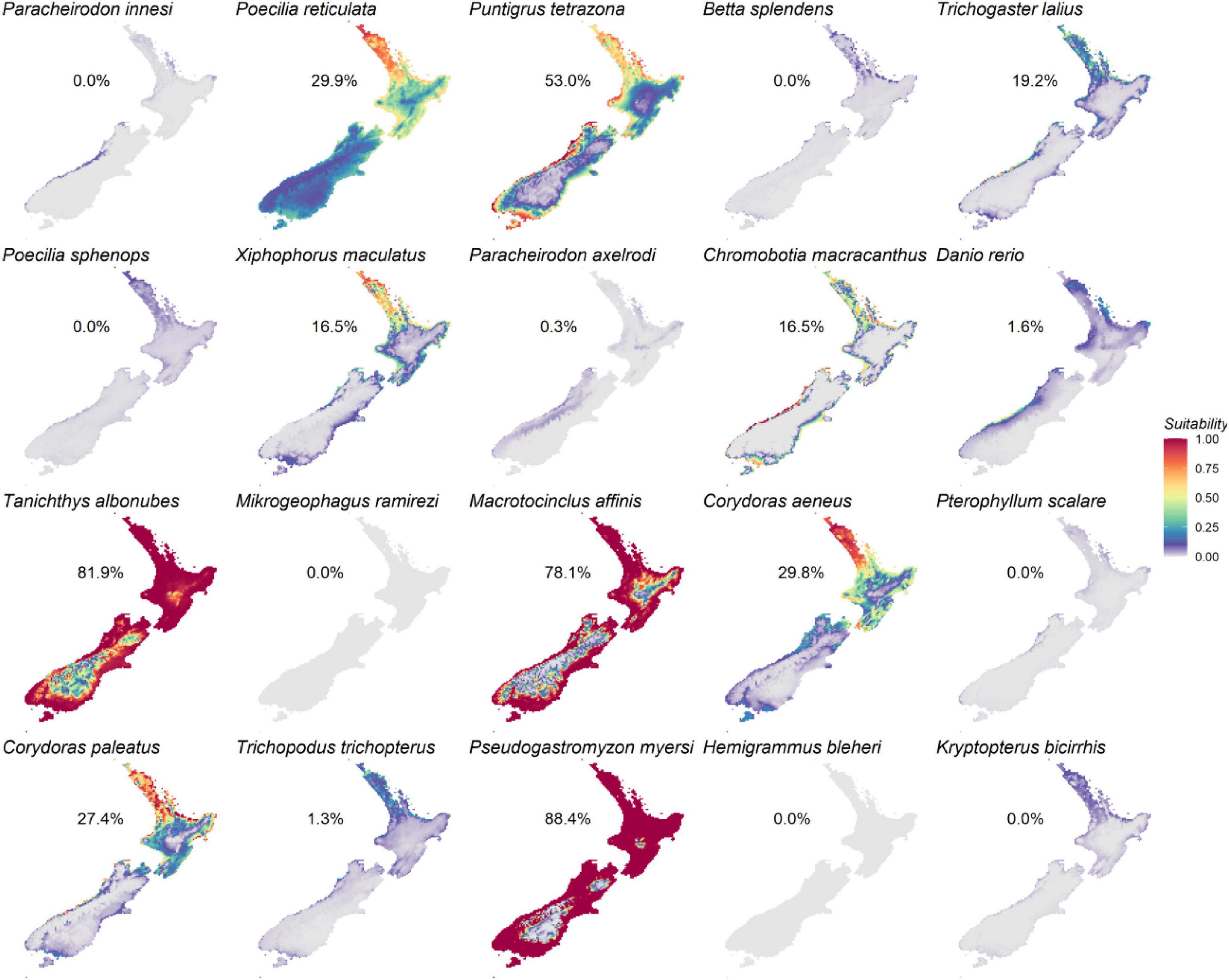
Figure 5. Heat maps of New Zealand’s habitat suitability for the 20 prioritized aquatic ornamental species imported to New Zealand as predicted by the species distribution models. Suitable habitat, based on the 10th percentile training presence threshold, is shown for each species as a percentage of the surface area of New Zealand.
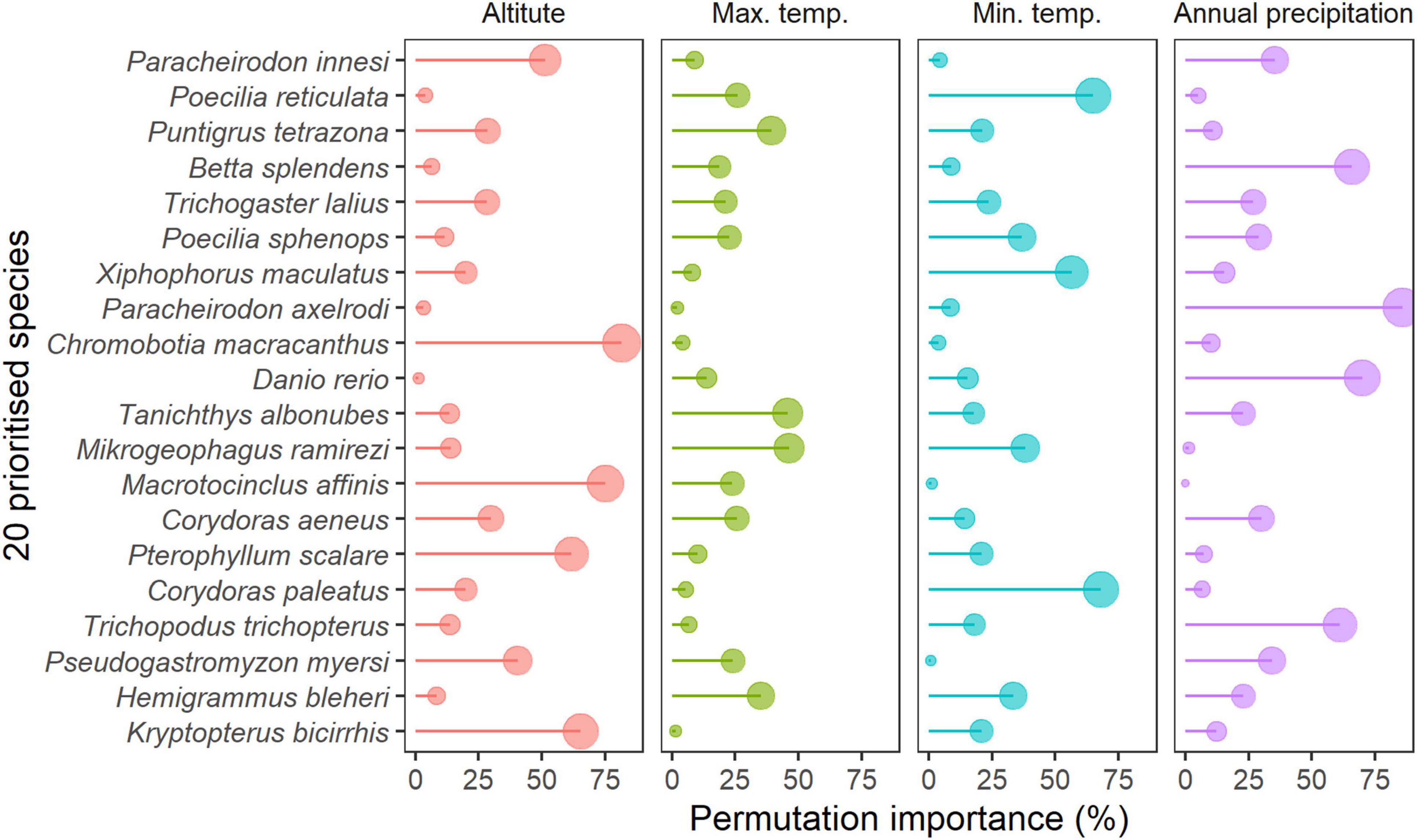
Figure 6. Importance of climatic variables included in the species distribution (MaxEnt) models for the 20 prioritized aquatic ornamental species imported to New Zealand.
Models predicted considerable changes in the proportion of habitat suitability under future climate scenarios in relation to current climate predictions (Figure 7). Predicted changes were consistent among the three tested GCMs; however, largest changes were predicted under HAdGEM2-ES. As expected, changes were less pronounced for the 2050 RCP2.6 scenarios compared to the 2070 RCP8.5 scenarios (Figure 7). Most of the predicted changes involved increases in the proportion of suitable habitat in relation to current climate suitability, however, substantial reductions (>1%) were predicted for four species, namely Ps. myersi (13% reduction for 2,070 under RCP8.5, Figure 7), Pu. tetrazona, Ma. affinis, and Ch. macracanthus (Figure 7). Largest predicted increases in habitat suitability were predicted for Po. reticulata (29% increase for 2070 under RCP8.5), Tr. lalius, X. maculatus, Co. paleatus, and Co. aeneus (Figure 7). Minimal changes (<1%), were predicted for several species, including B. splendens, Ch. macracanthus, K. bicirrhis, Pa. axelrodi, and Pa. innesi. Habitat remained unsuitable for H. bleheri, Mi. ramirezii, Po. sphenops, and Pt. scalare (Figure 7).
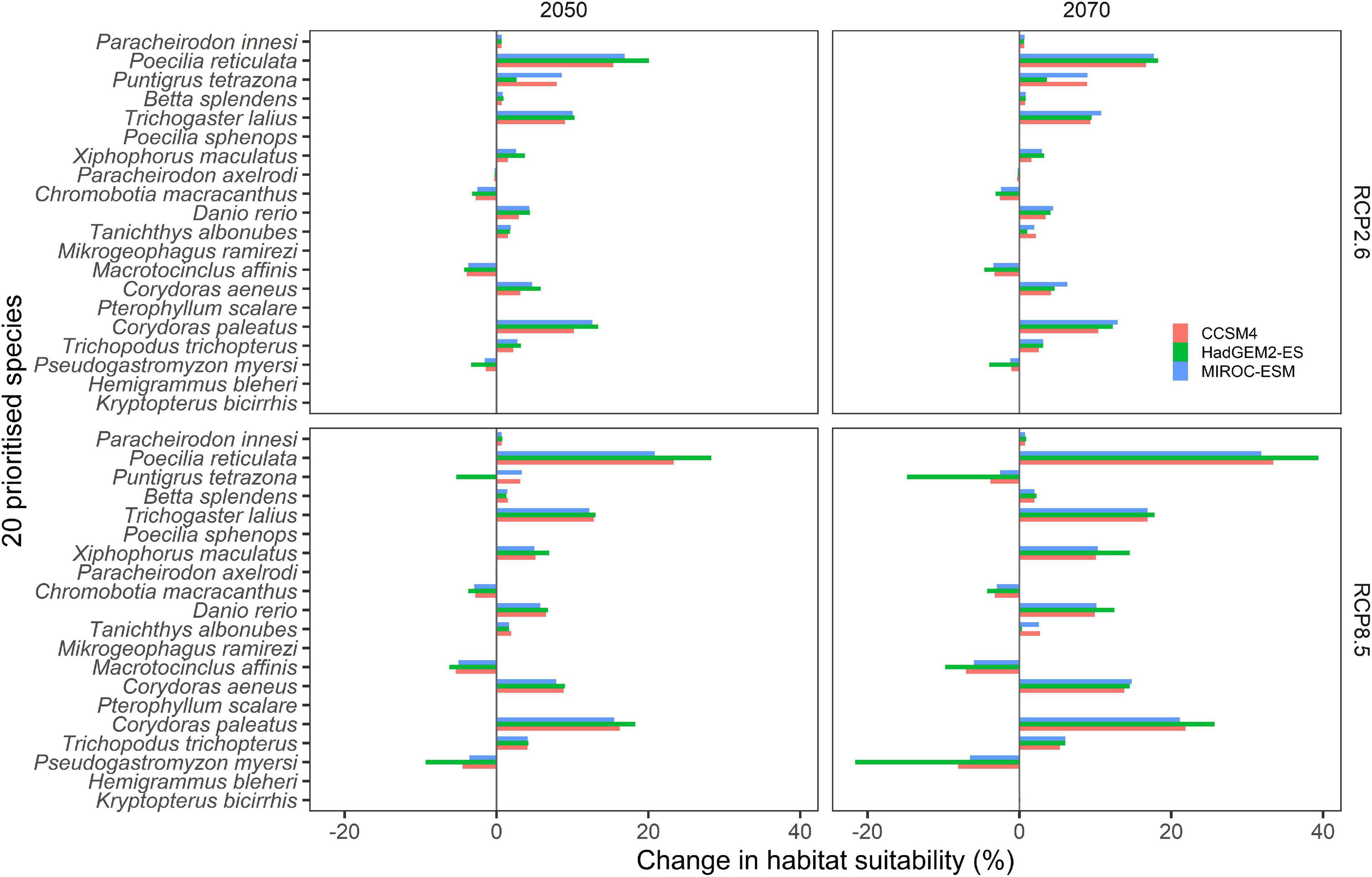
Figure 7. Predicted changes in habitat suitability for the 20 prioritized aquatic ornamental species imported to New Zealand under future (2050 and 2070) climate scenarios (RCP2.6 and RCP8.5) relative to current habitat suitability as predicted by the MaxEnt species distribution models (see Figure 3).
Discussion
Aquatic Ornamental Species Imports to New Zealand
Species variety is a key feature and driver of popularity for aquarium hobbyists worldwide, which results in a broad diversity of aquatic ornamental species traversing the globe. This is the case in New Zealand, where imports of 865 taxa over 5 years included 361 freshwater species to a geographically isolated country with an indigenous freshwater fish fauna of just 35 species (McDowall, 1998). Accurate quantitative information on the AOS trade is key for effective biosecurity and conservation management (Raghavan et al., 2013). In many cases AOS trade figures are not available, are inaccurate, or do not have sufficient taxonomic resolution to allow effective measures to be implemented (Williams et al., 2015; Rhyne et al., 2017). Based on the available data, the diversity of 865 taxa imported to New Zealand is quite considerable, although smaller than for top AOS importers such as the United States (1,802 marine species imported in 2005, Rhyne et al., 2012), Canada (∼1,500 species annually, Mandrak et al., 2014), and Australia (1,100 species annually, McNee, 2002). While the data-reporting framework for AOS imported to New Zealand includes logistical challenges (e.g., hard-copy import paperwork), and does not completely differentiate organisms to species, it provides relatively strong taxonomic rigor, with 89% of imported organisms identified to species and 9.5% genus level, respectively, with only one taxon identified to higher level (i.e., Order). The authors note that this conclusion is derived from analysis of the raw data only and that risk management for this pathway also includes the actions taken regarding the compliance and verification of said paperwork and with the imported species themselves.
The New Zealand data-reporting framework compares favorably to Australia where Trujillo-González and Militz (2019) found a framework with very limited capacity to identify imported organisms to species level for a regulatory system that permits the import of >4,600 species. In the case of Australia, although species-level data are recorded on import documents, the coarse categories of data processing according to transcription and data retention policies means only goldfish, Carassius auratus, are represented to species level. As a result, the capacity for downstream analyses of establishment likelihood as well as likelihood and consequences of pathogen or parasite spread via assessments of quantity and frequency of species imported is severely curtailed (McDowall, 2004; Collins et al., 2013). New Zealand’s collation of species-level data for AOS allows species-level risk analyses to occur, but further resolution of source location information (collection origins) would provide additional benefits for assessments, for example, including considerations of the prevalence of parasites and pathogens associated to AOS in the country of origin.
Our analysis of the available data revealed that ca. 1.5 million AOS individuals were imported into New Zealand from 2015 to 2019 inclusive, representing ca. 300,000 individuals per year. This is an extremely small proportion of the 1 billion AOS individuals traded globally each year (Evers et al., 2019) and is dwarfed by the quantities reported for some of the largest importers, such as the United States, the United Kingdom, several western European countries, Australia, and Japan (Monticini, 2010). For example, imports into the United States have been estimated at ca. 200 million individuals a year (Chapman et al., 1997) and ca. 11.2 million in Australia (Trujillo-González and Militz, 2019). The relatively modest size of the AOS trade is not surprising given the size of New Zealand’s aquarist market (current human population ca. 5 million compared to ca. 26 million in Australia and ca. 333 million in United States). In addition, the (probable) most popular AOS – C. auratus (goldfish) – is an approved species on the Import Health Standard (Ministry for Primary Industries, 2021) but has not been imported to New Zealand since 1972 (Hine and Diggles, 2005) and supply is from domestic captive breeding (Anonymous, 2020).
Almost 60% of the AOS arriving to New Zealand were from the marine environment, however, freshwater fish comprised 97.5% of total imports by quantity. Freshwater fish largely dominate the global AOS trade (90% of global trade value, Evers et al., 2019) and are by far the most popular species among hobbyists, as they are cheaper and easier to maintain than marine species (Scott, 1995). The AOS prioritized in this study were exclusively of tropical and subtropical freshwater fish, which generally overlap with those most commonly traded globally (Evers et al., 2019). In particular, fish in the family Poeciliidae – that includes live bearers such as the guppy, molly, platy, and swordtail – and in the family Characidae, including tetras, were imported at the highest quantities and frequencies. The top two ranked species, Po. reticulata and Pa. innesi, have been previously identified as the most imported species globally (Monticini, 2010), together accounting for more than 25% of the global market (Evers et al., 2019). On the other hand, only 16 marine species were within the top 100 prioritized species by quantity, with the highest prioritized marine species being the damselfish, Chromis viridis, at position 40.
Temperature Tolerance and Habitat Suitability of Prioritized Aquatic Ornamental Species
Prioritizing AOS by quantity and frequency of import to New Zealand represents a good starting point to evaluate the establishment likelihood of AOS in New Zealand waterways. The most numerous AOS imported – those with high propagule pressure for arrival at the border and to businesses and homes throughout the country – are more likely than rarer species to be intentionally or inadvertently introduced to aquatic habitats (Duggan et al., 2006; Strecker et al., 2011). Temperature matching between the physiological tolerance of AOS and the recipient environment represents a key determinant of habitat suitability (Sunday et al., 2012; Yanar et al., 2019). A literature review and collation of thermal range data for 20 prioritized imported AOS to New Zealand yielded relatively few data on temperature tolerances outside of two fish species used as model organisms in physiological research (i.e., D. rerio and Po. reticulata), and included no data for five species. Nonetheless, available information revealed 13 species have reported temperature tolerances that overlap with freshwater temperatures found in New Zealand. Aquarist data sources, including all of the temperature ranges reported for the prioritized AOS on FishBase suggest most imported tropical fish cannot tolerate temperatures below 18°C and would not survive in most New Zealand environments. New Zealand’s lakes average surface water temperatures range between 8 – 24°C and between 2 – 16°C, in the North and South Island, respectively (Hamilton et al., 2012; Jones and Hamilton, 2014), while river temperatures range between 7.8 and 18.2°C nationwide (Scarsbrook, 2006). One species, Co. paleatus, has reported tolerance to the full range of New Zealand’s freshwater temperatures, although the lower limit reported for this species is based on gray literature (cited in Baigún et al., 2002).
While 10 prioritized AOS thermal ranges extend into New Zealand riverine temperatures (below 18°C), the highest priority species, Pa. innesi, was not one of them. The broadest temperature tolerances collated from literature sources were typically based on experimental work that assessed critical maxima and minima for organisms based on short-term, acute exposure. FishBase estimates of thermal range appear quite narrow since they are likely based on aquarist concerns and broadscale species distributions and thus represent a probable pitfall to avoid when considering the likelihoods of species establishment (Bennett et al., 2018). Critical thermal methodologies (Cowles and Bogert, 1944; Yanar et al., 2019) provide a strong basis for future work to determine establishment likelihood by quantifying thermal niches of imported species for comparison with actual thermal conditions of New Zealand waters. Such an approach is independent of species’ realized ranges (Bennett et al., 2018), which provides a benefit for establishment assessments to focus on prominent components of a species’ fundamental niche.
The outputs of SDMs indicated that 13 of the 20 prioritized AOS were predicted to be tolerant of New Zealand’s current climate conditions, most notably Ps. myersi, Ta. albonubes, and Ma. affinis. There are no reports of Ps. myersi having an introduced range outside its native distribution in Hong Kong and southern China where it inhabits unpolluted stony streams with minimum water temperatures of 12.4°C (Yang and Dudgeon, 2009). Similarly, no introductions of the golden otocinclus Ma. affinis have been recorded outside its native habitats in the Amazon basin (U.S. Fish and Wildlife Service, 2014). The white cloud mountain minnow Ta. albonubes is a cold-water tolerant species often kept in aquaria. It is endemic to South China and Vietnam and was, until a few populations were found in the 2000s, thought to be extinct within its native range since 1980 (Chan and Chen, 2009). Ta. albonubes have established feral populations in other bioregions, including Colombia (Welcomme, 1988), Madagascar (Stiassny and Raminosoa, 1994), and Australia (Corfield et al., 2008). Based on its temperature tolerance, import frequency, and invasion history, Ta. albonubes can be considered to have a high likelihood of establishment in New Zealand, as it has in other temperate bioregions including Canada’s Great Lakes region (Rixon et al., 2005). Although the ecological impacts of this species are currently unknown, it has wide feeding preferences, including plankton, meiobenthos, and larvae of aquatic insects (Liang et al., 2008), suggesting potential for disrupting native foodwebs. The seven priority species that were unsuited to current climate conditions in New Zealand included some of the most popular species, such as the cardinal tetra (Pa. innesi), Siamese fighters (B. splendens), and the common molly (Po. sphenops).
One of the most prevalent effects of climate change on aquatic systems is a shift in species distributions in response to increasing temperatures (Melbourne-Thomas et al., 2021). Significant warming is predicted globally, up to 5.7°C by the end of the century under worst case scenarios (IPCC, 2021), with warming predicted for New Zealand at higher elevations and during summer and autumn (Mullan et al., 2016). Substantial changes in precipitation patterns are also expected, with wetter conditions predicted for the west and south and drier for the north and east of the country (Mullan et al., 2016). These predicted changes underpinned increased habitat suitability under future climate scenarios for AOS with distributions strongly associated with minimum temperature, such as Po. reticulata and Co. paleatus. By contrast, predicted habitat suitability was lower for a few taxa strongly related to precipitation patterns, or unaffected for tropical species when predicted New Zealand temperatures remained below those species’ minimum tolerance thresholds. Precipitation is directly linked to freshwater habitat parameters including water levels, flow, and sediment run-off (Milly et al., 2005), which are major drivers of fish distributional patterns (Logez et al., 2012). Predicted changes were consistent among the three explored GCMs, although they were more pronounced under HadGEM2-ES scenarios. Notably, future scenario predictions mostly involved habitat suitability expansions, rather than increasing the pool of species (among the 20 prioritized) that could potentially survive under future compared to current conditions. Predicted changes in habitat suitability are in line with previously observed and predicted climate-induced changes in freshwater fish distributions, with most cold-water species ranges being reduced or shifted to higher altitude or latitude and most warm-water species ranges expanded toward the poles (Comte et al., 2013).
The habitat suitability predictions in this study are based on species’ realized niches as one of the main assumptions of SDM methodology, whereas the fundamental thermal niche of many species may be substantially larger. For example, the white cloud mountain minnow’s (Ta. albonubes) nominal temperature tolerance range in the wild is between 18 and 22°C but it can survive water temperatures below 15°C (Chan and Chen, 2009) and potentially down to 5°C (Froese and Pauly, 2021). The differences in reported temperature tolerance ranges from an example like this have serious consequences for biosecurity assessment, planning, and implementation, and a more protective approach would consider fundamental thermal niches as a first step. Relying exclusively on fundamental thermal niches that do not consider biotic and abiotic interactions may lead to over-estimation of risk, but new introductions may not be constrained by factors that exist in native species ranges (Shea and Chesson, 2002). Although reproduction and the establishment of self-sustaining populations is less likely to occur outside a species realized niche, extended survival outside of realized niche predicted temperatures could increase the risks of spreading pathogens and parasites associated with AOS (Cable et al., 2017). As such, an iterative process using quantitative import metrics, thermal tolerance experiments, distribution modeling, and life-history considerations (in that order) offers a sound approach for resource prioritization and establishment evaluations regarding the AOS pathway.
Interestingly, the largest habitat suitability increases were predicted for the guppy, Po. reticulata, which is not only one of the most popular species in New Zealand and globally, but is also an invasive species in many parts of the world (Courtenay and Meffe, 1989). Guppies have established in at least 69 countries outside their native range in the Caribbean and north-eastern South America (Deacon et al., 2011). Guppies are viviparous and can store sperm, enabling them to establish viable populations at very small propagule size, with a single pregnant female being able to produce multiple broods of live offspring (Courtenay and Meffe, 1989). With a critical thermal minimum of 9.41°C (Yanar et al., 2019), the distribution of Po. reticulata in temperate regions is restricted to artificially warmed or geothermally influenced water bodies. An important caveat to consider is that the environmental layers used for the models in this study do not capture small-scale geothermally-influenced pools and river stretches (Houlihan, 2016). In fact, Po. reticulata and Poecilia latipinna are established in thermal waters at several sites on the North Island of New Zealand (Houlihan, 2016), Canada, and Russia (Deacon et al., 2011). While it is unlikely that under New Zealand’s current climate these species can overwinter outside of thermal habitats, the results of this study suggest that there is an increasing risk of range expansion under future climate scenarios. Although the likelihood of pest-associated ecological impacts associated with the introduction of Po. reticulata and other poecilids are considered low (Rowe and Wilding, 2012). From work undertaken as a separate part of the funded research, this species has the potential risk of introducing >40 parasites and pathogens into receiving environments.
Biosecurity Risk Management and the Aquatic Ornamental Species Trade
A range of strategies for managing the risks associated with AOS importations have been implemented by different countries, ranging from specific legislation to address the AOS pathway or under more generic import standards, policies, and regulations (see Chan et al., 2019 for a review). Import controls (lists of suitable, prohibited, and controlled species), risk screening, and release bans each play a role in managing invasion risk and all require investment in compliance verification and enforcement to succeed. New Zealand’s “Import Health Standard: Ornamental fish and marine invertebrates” (Ministry for Primary Industries, 2021) provides a list of species that can be imported, which imposes some control on arrivals at the border. Controls also include ensuring that imported AOS have either undergone quarantine offshore or on arrival at an approved transitional facility, official veterinary inspections, as well as treatment and testing of certain species during the quarantine period. The standard also provides a basis for subsequent risk analyses when further information becomes available. New Zealand has a storied history of intentional and inadvertent species introductions, including an oversized role of acclimatization societies on the country’s biogeography and invasion history (Pears, 1982). In aquatic systems, ships have been the dominant vector in the country’s marine invasion history (Cranfield et al., 1998; Georgiades et al., 2020), while significant freshwater incursions have occurred from intentional brown trout introductions in the 19th century (Townsend, 1996) and a notorious case of illicit intentional introductions of freshwater (sport) fish in more recent decades (Mitchell, 2020). Historical AOS introductions – via acclimatization societies – of goldfish and several submerged aquatic weeds have also had an enduring effect on New Zealand waterways (Champion and Clayton, 2000). To the authors’ knowledge, few AOS of the types covered by the Import Health Standard have established wild populations in New Zealand (Rowe and Wilding, 2012). Implementation of the standard and associated verification and compliance actions are considered the first line defense against such events.
Crucial to any pathway management strategy is the development of comprehensive species risk assessment tools based on likelihoods of introduction, establishment, and consequences to inform evidence-based policy and management actions. There is a wide range of risk screening tools that have been suggested for the AOS trade, including scoring systems, decision trees, and probabilistic models (see Chan et al., 2019 for a review), but risk screening in the AOS trade can be overwhelmed by the quantities, diversity, and lack of data regarding the organisms traded which can reduce the likelihood of acceptable outcomes (Whittington and Chong, 2007). Although our assessment did not formally include species traits and history of establishment, we capitalized on a wealth of AOS trade data to show that screening tools based on a combination of import quantities and frequency, temperature tolerance data, and habitat suitability modeling can assist establishment assessments to support biosecurity outcomes. Risk assessment and subsequent management strategies should carefully consider the consistent use of taxonomic nomenclature, paying particular attention to insufficient resolution that could compromise the screening process. Detailed digital import records, including consignment composition, origin, and species level quantities, that can be programmatically scrutinized against global taxonomic databases could be used for quality control of the screening process and add value to import compliance and verification processes. These approaches could be widely adopted where AOS importation data are accessible and robust.
In a dynamic industry with significant quantities of organisms in transit and a burgeoning online trade, it is important to periodically assess import trends and review associated risks in relation to changing environmental conditions, invasion histories, and animal health and disease issues (Trujillo-González et al., 2018). Biosecurity requirements such as import health standards facilitate this process, but other information sources to consider include assessing patterns of AOS release by the public to better understand release rates and the full extent of this invasion pathway (Strecker et al., 2011). Aquarium releases have been an issue in waterways globally, including New Zealand, with subsequent impacts requiring costly ongoing mitigation (Ranford, 2018). Policy makers should consider the findings of this study to inform future risk management decisions regarding inclusions of organisms within species-lists. Maintaining public and industry awareness of their responsibilities is a crucial step to prevent unwanted impacts in waterways and ultimately support the biosecurity outcomes associated with regulatory requirements for AOS importation (Morrisey et al., 2011). As such, educational materials regarding the proper care and disposal of AOS should be provided at their point of sale, including websites.
Data Availability Statement
The datasets presented in this study can be found in online repositories. The names of the repository/repositories and accession number(s) can be found below: Javier Atalah. (2021). jatalah/ornamental_fish: Frontiers submission (v1.3). Zenodo. https://doi.org/10.5281/zenodo.5610004.
Author Contributions
JA, ID, MT, EG, and KH conceived and designed the study and wrote the manuscript. JA analyzed the data. All authors contributed to the article and approved the submitted version.
Funding
This study was funded by the New Zealand Government (Ministry for Primary Industries) through contract 406087 - Ornamental fish – What are the risks?
Conflict of Interest
The authors declare that the research was conducted in the absence of any commercial or financial relationships that could be construed as a potential conflict of interest.
Publisher’s Note
All claims expressed in this article are solely those of the authors and do not necessarily represent those of their affiliated organizations, or those of the publisher, the editors and the reviewers. Any product that may be evaluated in this article, or claim that may be made by its manufacturer, is not guaranteed or endorsed by the publisher.
Acknowledgments
The MPI Operational Research Team made this research possible. The efforts of Tanya Hurley (MPI) who performed the data extraction and summarization from the original import consignment invoices are greatly appreciated. We thank Deborah Long for project support and Gretchen Rasch for reviewing the draft manuscript. Cara Brosnahan and Henry Lane assisted with project conception. Diana Jaramillo provided project support and manuscript reviews.
Supplementary Material
The Supplementary Material for this article can be found online at: https://www.frontiersin.org/articles/10.3389/fevo.2021.804160/full#supplementary-material
References
Anderson, R. P., Araújo, M., Guisan, A., Lobo, J. M., Martínez-Meyer, E., Peterson, A. T., et al. (2016). Are Species Occurrence Data in Global Online Repositories Fit for Modeling Species Distributions? The Case of the Global Biodiversity Information Facility (GBIF). Final Report of the Task Group on GBIF Data Fitness for Use in Distribution Modelling. Copenhagen: Global Biodiversity Information Facility.
Andrews, C. (1990). The ornamental fish trade and fish conservation. J. Fish Biol. 37, 53–59. doi: 10.1111/j.1095-8649.1990.tb05020.x
Anonymous (2020). Te Aroha Goldfish Head to all Parts of New Zealand. Available online at: https://www.nzherald.co.nz/north-island/news/article.cfm?c_id=1503932&objectid=12295471 (accessed February 2021)
Baigún, C., López, G., Dománico, A., Ferriz, R., Sverlij, S., and Schenke, R. D. (2002). Presencia de Corydoras paleatus (Jenyns, 1842), una nueva especie brasílica en el norte de la Patagonia (río Limay) y consideraciones ecológicas relacionadas con su distribución. Ecol. Aust. 12, 41–48.
Beatty, S. J., Allen, M. G., Whitty, J. M., Lymbery, A. J., Keleher, J. J., Tweedley, J. R., et al. (2017). First evidence of spawning migration by goldfish (Carassius auratus); implications for control of a globally invasive species. Ecol. Freshw. Fish 26, 444–455. doi: 10.1111/eff.12288
Bennett, J. M., Calosi, P., Clusella-Trullas, S., Martínez, B., Sunday, J., Algar, A. C., et al. (2018). GlobTherm, a global database on thermal tolerances for aquatic and terrestrial organisms. Sci. Data 5, 1–7. doi: 10.1038/sdata.2018.22
Biondo, M. V., and Burki, R. P. (2020). A systematic review of the ornamental fish trade with emphasis on coral reef fishes–an impossible task. Animals 10:2014. doi: 10.3390/ani10112014
Biosecurity New Zealand (2009). Import Risk Analysis: Tropical, Subtropical and Temperate Freshwater and Marine Ornamental Fish and Marine Molluscs and Crustaceans. Review of Submissions and Supplementary Risk Analisis. Wellington: Ministry of Agriculture and Forestry, 163.
Bisby, F. A., Roskov, Y., Orrell, T., Nicolson, D., Paglinawan, L., Bailly, N., et al. (2010). Species 2000 and ITIS Catalogue of Life. Annual Checklist. Available online at: www.catalogueoflife.org/ (accessed February 1, 2021).
Bosch, S., Tyberghein, L., and De Clerck, O. (2018). sdmpredictors: Species Distribution Modelling Predictor Datasets. R package version 0.2.9. Available online at: https://CRAN.R-project.org/package=sdmpredictors (accessed February 19, 2021).
Bruckner, A. (2005). The importance of the marine ornamental reef fish trade in the wider Caribbean. Rev. Biol. Trop. 53, 127–137.
Brunner, B. (2012). The Ocean at Home: An Illustrated History of the Aquarium, 2nd Edn. London: Reaktion Books, 160.
Cable, J., Barber, I., Boag, B., Ellison, A. R., Morgan, E. R., Murray, K., et al. (2017). Global change, parasite transmission and disease control: lessons from ecology. Philos. Trans. R. Soc. B Biol. Sci. 372:20160088. doi: 10.1098/rstb.2016.0088
Capps, K. A., and Flecker, A. S. (2013). Invasive aquarium fish transform ecosystem nutrient dynamics. Proc. R. Soc. B Biol. Sci. 280:20131520. doi: 10.1098/rspb.2013.1520
Carolus, H., Muzarabani, K. C., Hammoud, C., Schols, R., Volckaert, F. A., Barson, M., et al. (2019). A cascade of biological invasions and parasite spillback in man-made Lake Kariba. Sci. Total Environ. 659, 1283–1292. doi: 10.1016/j.scitotenv.2018.12.307
Chamberlain, S. A., and Boettiger, C. (2017). R Python, and Ruby clients for GBIF species occurrence data. PeerJ [Preprint]. 5:e3304v1. doi: 10.7287/peerj.preprints.3304v1
Chamberlain, S. A., and Szöcs, E. (2013). taxize: taxonomic search and retrieval in R. F1000Res. 2:119. doi: 10.12688/f1000research.2-191.v2
Champion, P. D., and Clayton, J. S. (2000). Border Control for Potential Aquatic Weeds. Border Control for Potential Aquatic Weeds. Stage 1. Weed Risk Model. Wellington: Department of Conservation Wellington, 141.
Chan, B. P. L., and Chen, X. L. (2009). Discovery of Tanichthys albonubes Lin 1932 (Cyprinidae) on Hainan Island, and notes on its ecology. Zool. Res. 30, 209–214. doi: 10.3724/sp.j.1141.2009.02209
Chan, F. T., Beatty, S. J., Gilles, A. S., Hill, J. E., Kozic, S., Luo, D., et al. (2019). Leaving the fish bowl: the ornamental trade as a global vector for freshwater fish invasions. Aquat. Ecosyst. Health Manag. 22, 417–439.
Chapman, F. A., Fitz-Coy, S. A., Thunberg, E. M., and Adams, C. M. (1997). United States of America trade in ornamental fish. J. World Aquacult. Soc. 28, 1–10.
Collins, R. A., Armstrong, K. F., Holyoake, A. J., and Keeling, S. (2013). Something in the water: biosecurity monitoring of ornamental fish imports using environmental DNA. Biol. Invasions 15, 1209–1215. doi: 10.1007/s10530-012-0376-9
Collins, W., Bellouin, N., Doutriaux-Boucher, M., Gedney, N., Halloran, P., Hinton, T., et al. (2011). Development and evaluation of an Earth-System model–HadGEM2. Geosci. Model Dev. 4, 1051–1075. doi: 10.5194/gmd-4-1051-2011
Comte, L., Buisson, L., Daufresne, M., and Grenouillet, G. (2013). Climate-induced changes in the distribution of freshwater fish: observed and predicted trends. Freshw. Biol. 58, 625–639.
Copp, G. H., Warrington, S., and Wesley, K. J. (2007). “Management of an ornamental pond as a conservation site for a threatened native fish species, crucian carp Carassius carassius,” in Pond Conservation in Europe. Developments in Hydrobiology 210, Vol 210. eds B. Oertli, R. Céréghino, J. Biggs, S. Declerck, A. Hull, and M. R. Miracle (Dordrecht: Springer). doi: 10.1007/978-90-481-9088-1_13
Corfield, J., Diggles, B., Jubb, C., McDowall, R. M., Moore, A., Richards, A., et al. (2008). Review of the Impacts of Introduced Ornamental Fish Species That Have Established Wild Populations in Australia. Canberra, ACT: Department of the Environment, Water, Heritage and the Arts, 277.
Courtenay, W. Jr., and Meffe, G. (1989). “Small fishes in strange places: a review of introduced poeciliids,” in Ecology and Evolution of Livebearing Fishes (Poeciliidae), eds G. K. Meffe and F. F. Snelson (Englewood Cliffs, NJ: Prentice-Hall, Inc), 319–331.
Cowles, R. B., and Bogert, C. M. (1944). A preliminary study of the thermal requirements of desert reptiles. Iguana 83:53.
Cranfield, H. J., Gordon, D., Willan, R., Marshall, B., Battershill, C., Francis, M., et al. (1998). Adventive Marine Species in New Zealand. Technical Report 34. Wellington: National Institute of Water and Atmospheric Research, 48.
Deacon, A. E. I, Ramnarine, W., and Magurran, A. E. (2011). How reproductive ecology contributes to the spread of a globally invasive fish. PLoS One 6:e24416. doi: 10.1371/journal.pone.0024416
Duggan, I. C., Rixon, C. A., and MacIsaac, H. J. (2006). Popularity and propagule pressure: determinants of introduction and establishment of aquarium fish. Biol. Invasions 8, 377–382.
Evers, H. G., Pinnegar, J. K., and Taylor, M. I. (2019). Where are they all from?–Sources and sustainability in the ornamental freshwater fish trade. J. Fish Biol. 94, 909–916. doi: 10.1111/jfb.13930
Froese, R., and Pauly, D. (eds) (2021). FishBase. World Wide Web Electronic Publication. Available online at: www.fishbase.org (accessed Nov 21, 2020).
García-Díaz, P. A., Kerezsy, P. J. Unmack, Lintermans, M., Beatty, S. J., Butler, G. L., Freeman, R., et al. (2018). Transport pathways shape the biogeography of alien freshwater fishes in Australia. Divers. Distrib. 24, 1405–1415. doi: 10.1111/ddi.12777
Gent, P. R., Danabasoglu, G., Donner, L. J., Holland, M. M., Hunke, E. C., Jayne, S. R., et al. (2011). The Community Climate System Model Version 4. J. Clim. 24, 4973–4991.
Georgiades, E., Kluza, D., Bates, T., Lubarsky, K., Brunton, J., Growcott, A., et al. (2020). Regulating vessel biofouling to support New Zealand’s marine biosecurity system – a blue print for evidence-based decision making. Front. Mar. Sci. 7:390. doi: 10.3389/fmars.2020.00390
Glasby, T. M. (2013). Caulerpa taxifolia in seagrass meadows: killer or opportunistic weed? Biol. Invasions 15, 1017–1035.
Green, E. (2003). “International trade in marine aquarium species: using the global aquarium database,” in Marine Ornamental Species: Collection, Culture and Conservation, eds J. Cato and C. Brown (Iowa, IA: Iowa State Press), 29–48. doi: 10.1016/j.dib.2020.106337
Hamilton, D. P., Jones, H. F. E., Özkundakci, D., McBride, C. G., Allan, M. G., Faber, J., et al. (2012). Waituna Lagoon Modelling: Developing Quantitative Assessments to Assist With Lagoon Management. ERI Report 26. Prepared for Environment Southland. Environmental Research Institute, Faculty of Science and Engineering. Waikato: The University of Waikato, 114.
Hine, P., and Diggles, B. (2005). Import Risk analysis: Ornamental Fish. Biosecurity New Zealand. Wellington: Ministry of Agriculture and Forestry, 132.
Houlihan, D. (2016). A Study of the Length Weight Relationships and Diets of Non-Indigenous Poecillids in New Zealand Geothermal Streams. Ph.D. thesis, Waikato: University of Waikato.
Howeth, J. G., Gantz, C. A., Angermeier, P. L., Frimpong, E. A., Hoff, M. H., Keller, R. P., et al. (2016). Predicting invasiveness of species in trade: climate match, trophic guild and fecundity influence establishment and impact of non-native freshwater fishes. Divers. Distrib. 22, 148–160. doi: 10.1111/ddi.12391
IPCC (2021). “Summary for policymakers,” in Climate Change 2021: The Physical Science Basis. Contribution of Working Group I to the Sixth Assessment Report of the Intergovernmental Panel on Climate Change, eds V. Masson-Delmotte, P. Zhai, A. Pirani, S. Connors, C. Péan, S. Berger, et al. (Cambridge: Cambridge University Press), 150.
Jones, H. F. E., and Hamilton, D. P. (2014). Assessment of the Waikato River Estuary Delta for Whitebait Habitat Management: Field Survey, GIS Modelling and Hydrodynamic Modelling. ERI Report 27. Prepared for Waikato Regional Council. Hamilton, CA: The University of Waikato.
Kay, S. H., and Hoyle, S. T. (2001). Mail order, the internet, and invasive aquatic weeds. J. Aquat. Plant Manag. 39, 88–91.
Koehn, J. D., and MacKenzie, R. F. (2004). Priority management actions for alien freshwater fish species in Australia, New Zealand. J. Mar. Freshw. Res. 38, 457–472.
Leung, B., and Mandrak, N. E. (2007). The risk of establishment of aquatic invasive species: joining invasibility and propagule pressure. Proc. R. Soc. B Biol. Sci. 274, 2603–2609. doi: 10.1098/rspb.2007.0841
Liang, X.-F., Chen, G.-Z., Chen, X.-L., and Yue, P.-Q. (2008). Threatened fishes of the world: Tanichthys albonubes Lin 1932 (Cyprinidae). Environ. Biol. Fishes 82, 177–178. doi: 10.1007/s10641-007-9286-6
Liu, C., White, M., and Newell, G. (2011). Measuring and comparing the accuracy of species distribution models with presence–absence data. Ecography 34, 232–243. doi: 10.1111/j.1600-0587.2010.06354.x
Lockwood, J. L., Cassey, P., and Blackburn, T. (2005). The role of propagule pressure in explaining species invasions. Trends Ecol. Evol. 20, 223–228. doi: 10.1016/j.tree.2005.02.004
Logez, M., Bady, P., and Pont, D. (2012). Modelling the habitat requirement of riverine fish species at the European scale: sensitivity to temperature and precipitation and associated uncertainty. Ecol. Freshw. Fish 21, 266–282.
Magalhães, A. L. B., Daga, V. S., Bezerra, L. A. V., Vitule, J. R. S., Jacobi, C. M., and Silva, L. G. M. (2020). All the colors of the world: biotic homogenization-differentiation dynamics of freshwater fish communities on demand of the Brazilian aquarium trade. Hydrobiologia 847, 3897–3915.
Mandrak, N. E., Gantz, C. A., Jones, L. A., Marson, D., and Cudmore, B. (2014). Evaluation of five Freshwater Fish Screening-Level Risk Assessment Protocols and Application To Non-Indigenous Organisms in Trade in Canada. Canadian Science Advisory Secretariat Research Document. Toronto, CA: Department of Fisheries and Oceans, 125.
Martin, G. D., and Coetzee, J. A. (2011). Pet stores, aquarists and the internet trade as modes of introduction and spread of invasive macrophytes in South Africa. Water SA 37, 371–380.
McDowall, R. M. (1998). Driven by diadromy: its role in the historical and ecological biogeography of the New Zealand freshwater fish fauna. Ital. J. Zool. 65, 73–85. doi: 10.1080/11250009809386799
McDowall, R. M. (2004). Shoot first, and then ask questions: A look at aquarium fish imports and invasiveness in New Zealand. N. Z. J. Mar. Freshw. Res. 38, 503–510.
McGarvey, D. J., Menon, M., Woods, T., Tassone, S., Reese, J., Vergamini, M., et al. (2018). On the use of climate covariates in aquatic species distribution models: are we at risk of throwing out the baby with the bath water? Ecography 41, 695–712.
McNee, A. (2002). A National Approach to the Management of Exotic Fish Species in the Aquarium Trade: an Inventory of Exotic Freshwater Species. Report to the Fisheries Resources Research Fund. Canberra, ACT: Bureau of Rural Sciences.
Melbourne-Thomas, J., Audzijonyte, A., Brasier, M. J., Cresswell, K. A., Fogarty, H. E., Haward, M., et al. (2021). Poleward bound: adapting to climate-driven species redistribution. Rev. Fish Biol. Fish. doi: 10.1007/s11160-021-09641-3
Milly, P. C. D., Dunne, K. A., and Vecchia, A. V. (2005). Global pattern of trends in streamflow and water availability in a changing climate. Nature 438, 347–350. doi: 10.1038/nature04312
Ministry for Primary Industries (2016). Review of Submissions on: Draft Import Health Standard for Ornamental Fish and Marine Invertebrates from all Countries. Wellington: Ministry for Primary Industries, 79.
Ministry for Primary Industries (2021). Import Health Standard: Ornamental Fish and Marine Invertebrates – Import Health Standard. Available online at: https://www.mpi.govt.nz/dmsdocument/17425-Ornamental-fish-and-marine-invertebrates-from-all-countries-Import-Health-Standard (accessed Oct 28, 2021).
Mitchell, C. (2020). The Liberator: How One Man’s 15,000 Pest Fish Changed New Zealand’s Waterways. Wellington: Stuff.
Monticini, P. (2010). The Ornamental Fish Trade: Production and Commerce of Ornamental fish: Technical-Managerial and Legislative Aspects. GLOBEFISH Research Programme. Rome: FAO.
Morris, J. A., and Whitfield, P. E. (2009). Biology, Ecology, Control and Management of the Invasive Indo-Pacific lionfish: An Updated Integrated Assessment. NOAA Technical Memorandum NOS NCCOS 99. Washington, DC: NOAA, 57.
Morrisey, D., Inglis, G., Neil, K., Bradley, A., and Fitridge, I. (2011). Characterization of the marine aquarium trade and management of associated marine pests in Australia, a country with stringent import biosecurity regulation. Environ. Conserv. 38, 89–100. doi: 10.1017/s0376892911000014
Moss, R. H., Edmonds, J. A., Hibbard, K. A., Manning, M. R., Rose, S. K., Van Vuuren, D. P., et al. (2010). The next generation of scenarios for climate change research and assessment. Nature 463, 747–756. doi: 10.1038/nature08823
Mullan, B., Sood, A., Stuart, S., and Carey-Smith, T. (2016). Climate Change Projections for New Zealand: Atmosphere Projections Based on Simulations From the IPCC fifth Assessment. Wellington: Ministry for the Environment.
Nunes, A. L., Tricarico, E., Panov, V. E., Cardoso, A. C., and Katsanevakis, S. (2015). Pathways and gateways of freshwater invasions in Europe. Aquat. Invasions 10, 359–370.
Padilla, D. K., and Williams, S. L. (2004). Beyond ballast water: aquarium and ornamental trades as sources of invasive species in aquatic ecosystems. Front. Ecol. Environ. 2:131–138. doi: 10.1890/1540-92952004002[0131:BBWAAO]2.0.CO;2
Pears, N. (1982). Familiar aliens: the acclimatisation societies’ role in New Zealand’s biogeography. Scott. Geogr. Mag. 98, 23–34. doi: 10.1080/00369228208736512
Penning, M., Reid, G., Koldewey, H., Dick, G., Andrews, B., Arai, K., et al. (2009). Turning the Tide: A Global Aquarium Strategy for Conservation and Sustainability. Bern: World Association of Zoos and Aquariums, 77.
Phillips, S. J., Anderson, R. P., and Schapire, R. E. (2006). Maximum entropy modeling of species geographic distributions. Ecol. Modell. 190, 231–259. doi: 10.1016/j.ecolmodel.2005.03.026
Prakash, S., Kumar, T. T. A., Raghavan, R., Rhyne, A., Tlusty, M. F., and Subramoniam, T. (2017). Marine aquarium trade in India: challenges and opportunities for conservation and policy. Mar. Policy 77, 120–129.
R Core Team (2021). R: A Language and Environment for Statistical Computing. Vienna: R Foundation for Statistical Computing.
Raghavan, R., Dahanukar, N., Tlusty, M. F., Rhyne, A. L., Kumar, K. K., Molur, S., et al. (2013). Uncovering an obscure trade: threatened freshwater fishes and the aquarium pet markets. Biol. Conserv. 164, 158–169.
Raja, K., Aanand, P., Padmavathy, S., and Sampathkumar, J. S. (2019). Present and future market trends of Indian ornamental fish sector. Int. J.Fish. Aquat. Stud. 7, 6–15.
Ranford, C. (2018). Goddammit: Dam-Dumped Goldfish a ‘Monumental’ Problem. Stuff. August 2nd 2018. Avaialble online at: https://www.stuff.co.nz/national/105755686/goddammit-damdumped-goldfish-a-monumental-problem (accessed May, 2021).
Reid, A. J., Carlson, A. K. I, Creed, F., Eliason, E. J., Gell, P. A., Johnson, P. T., et al. (2019). Emerging threats and persistent conservation challenges for freshwater biodiversity. Biol. Rev. 94, 849–873. doi: 10.1111/brv.12480
Rhyne, A. L., Tlusty, M. F., Schofield, P. J., Kaufman, L., Morris, J. A. Jr., and Bruckner, A. W. (2012). Revealing the appetite of the marine aquarium fish trade: the volume and biodiversity of fish imported into the United States. PLoS One 7:e35808. doi: 10.1371/journal.pone.0035808
Rhyne, A. L., Tlusty, M. F., Szczebak, J. T., and Holmberg, R. J. (2017). Expanding our understanding of the trade in marine aquarium animals. PeerJ 5:e2949. doi: 10.7717/peerj.2949
Rixon, C. A. I, Duggan, C., Bergeron, N. M., Ricciardi, A., and MacIsaac, H. J. (2005). Invasion risks posed by the aquarium trade and live fish markets on the Laurentian Great Lakes. Biodivers. Conserv. 14, 1365–1381. doi: 10.1007/s10531-004-9663-9
Rowe, D. K., and Wilding, T. (2012). Risk assessment model for the introduction of non-native freshwater fish into New Zealand. J. Appl. Ichthyol. 28, 582–589. doi: 10.1111/j.1439-0426.2012.01966.x
Scarsbrook, M. R. (2006). State and Trends in the National River Water Quality Network (1989-2005). Wellington: Ministry for the Environment.
Scott, P. W. (1995). The Complete Aquarium: A Practical Guide to Building, Stocking, and Maintaining Freshwater and Marine aquariums. New York, NY: DK Publishing, Inc, 192.
Semmens, B. X., Buhle, E. R., Salomon, A. K., and Pattengill-Semmens, C. V. (2004). A hotspot of non-native marine fishes: evidence for the aquarium trade as an invasion pathway. Mar. Ecol. Prog. Ser. 266, 239–244. doi: 10.3354/meps266239
Shea, K., and Chesson, P. (2002). Community ecology theory as a framework for biological invasions. Trends Ecol. Evol. 17, 170–176. doi: 10.1016/s0169-5347(02)02495-3
Stefan, H. G., Fang, X., and Eaton, J. G. (2001). Simulated fish habitat changes in North American lakes in response to projected climate warming. Trans. Am. Fish. Soc. 130, 459–477. doi: 10.1577/1548-8659(2001)130<0459:sfhcin>2.0.co;2
Stiassny, M., and Raminosoa, N. (1994). “The fishes of the inland waters of Madagascar,” in Biological Diversity of African Fresh- and Brackish Water Fishes, eds G. G. Teugels, J. F. Guegan, and J. J. Albaret (Paris: ORSTOM), 177. doi: 10.1021/jf048993y
Strecker, A. L., Campbell, P. M., and Olden, J. D. (2011). The aquarium trade as an invasion pathway in the Pacific Northwest. Fisheries 36, 74–85. doi: 10.1577/03632415.2011.10389070
Sunday, J. M., Bates, A. E., and Dulvy, N. K. (2012). Thermal tolerance and the global redistribution of animals. Nat. Clim. Change 2, 686–690. doi: 10.1038/nclimate1539
Taylor, K. E., Stouffer, R. J., and Meehl, G. A. (2012). An overview of CMIP5 and the experiment design. Bull. Am. Meteorol. Soc. 93, 485–498. doi: 10.1175/bams-d-11-00094.1
Thibaut, T., and Meinesz, A. (2004). “Caulerpa taxifolia: 18 years of infestation in the Mediterranean Sea,” in Aquatic Invasions in the Black, Caspian, and Mediterranean Seas, eds H. Dumont, T. A. Shiganova, and U. Niermann (Dordrecht: Springer), 287–298. doi: 10.1007/1-4020-2152-6_17
Townsend, C. R. (1996). Invasion biology and ecological impacts of brown trout Salmo trutta in New Zealand. Biol. Conserv. 78, 13–22. doi: 10.1016/0006-3207(96)00014-6
Trujillo-González, A., Becker, J. A., and Hutson, K. S. (2018). Parasite dispersal from the ornamental goldfish trade. Adv. Parasitol. 100, 239–281. doi: 10.1016/bs.apar.2018.03.001
Trujillo-González, A., and Militz, T. A. (2019). Taxonomically constrained reporting framework limits biodiversity data for aquarium fish imports to Australia. Wildl. Res. 46, 355–363. doi: 10.1071/wr18135
U.S. Fish and Wildlife Service (2014). Golden Otocinclus (Otocinclus affinis) Ecological Risk Screening Summary. Available online at: https://www.fws.gov/fisheries/ANS/erss/uncertainrisk/ERSS-Otocinclus-affinis-FINAL-Jun2020.pdf (acessed February, 2021).
van Proosdij, A. S. J., Sosef, M. S. M., Wieringa, J. J., and Raes, N. (2016). Minimum required number of specimen records to develop accurate species distribution models. Ecography 39, 542–552. doi: 10.1111/ecog.01509
Van Vuuren, D. P., Edmonds, J., Kainuma, M., Riahi, K., Thomson, A., Hibbard, K., et al. (2011). The representative concentration pathways: an overview. Clim. Change 109, 5–31. doi: 10.1007/s10584-011-0148-z
Watanabe, S., Hajima, T., Sudo, K., Nagashima, T., Takemura, T., Okajima, H., et al. (2011). MIROC-ESM 2010: model description and basic results of CMIP5-20c3m experiments. Geosci. Model Dev. 4, 845–872. doi: 10.5194/gmd-4-845-2011
Welcomme, R. L. (1988). International Introductions of Inland Aquatic Species. FAO Fisheries Technical Paper 294. Quebec, CA: FAO, 318.
Whittington, R., and Chong, R. (2007). Global trade in ornamental fish from an Australian perspective: the case for revised import risk analysis and management strategies. Prev. Vet. Med. 81, 92–116. doi: 10.1016/j.prevetmed.2007.04.007
Williams, S. L., Crafton, R. E., Fontana, R. E., Grosholz, E. D., Ha, G., Pasari, J. R., et al. (2015). A vector analysis of marine ornamental species in California. Manag. Biol. Invasions 6:13. doi: 10.3391/mbi.2015.6.1.02
Williams, S. L., and Grosholz, E. D. (2002). Preliminary reports from the Caulerpa taxifolia invasion in southern California. Mar. Ecol. Prog. Ser. 233, 307–310. doi: 10.3354/meps233307
WoRMS Editorial Board (2020). World Register of Marine Species. Available online at: http://www.marinespecies.org at VLIZ (accessed June 07, 2020).
Yanar, M., Erdoðan, E., and Kumlu, M. (2019). Thermal tolerance of thirteen popular ornamental fish species. Aquaculture 501, 382–386. doi: 10.1016/j.aquaculture.2018.11.041
Keywords: aquarium trade, species distribution modeling (SDM), New Zealand, biosecurity, Poecilia reticulata (guppy), non-indigenous species (NIS)
Citation: Atalah J, Davidson IC, Thoene M, Georgiades E and Hutson KS (2022) Evaluating Importation of Aquatic Ornamental Species for Biosecurity Purposes. Front. Ecol. Evol. 9:804160. doi: 10.3389/fevo.2021.804160
Received: 28 October 2021; Accepted: 06 December 2021;
Published: 06 January 2022.
Edited by:
Hang Sun, Kunming Institute of Botany, Chinese Academy of Sciences (CAS), ChinaReviewed by:
Nigel G. Taylor, Ecological Consultant, United KingdomAndrew Anthony David, Clarkson University, United States
Copyright © 2022 Atalah, Davidson, Thoene, Georgiades and Hutson. This is an open-access article distributed under the terms of the Creative Commons Attribution License (CC BY). The use, distribution or reproduction in other forums is permitted, provided the original author(s) and the copyright owner(s) are credited and that the original publication in this journal is cited, in accordance with accepted academic practice. No use, distribution or reproduction is permitted which does not comply with these terms.
*Correspondence: Javier Atalah, amF2aWVyLmF0YWxhaEBjYXd0aHJvbi5vcmcubno=