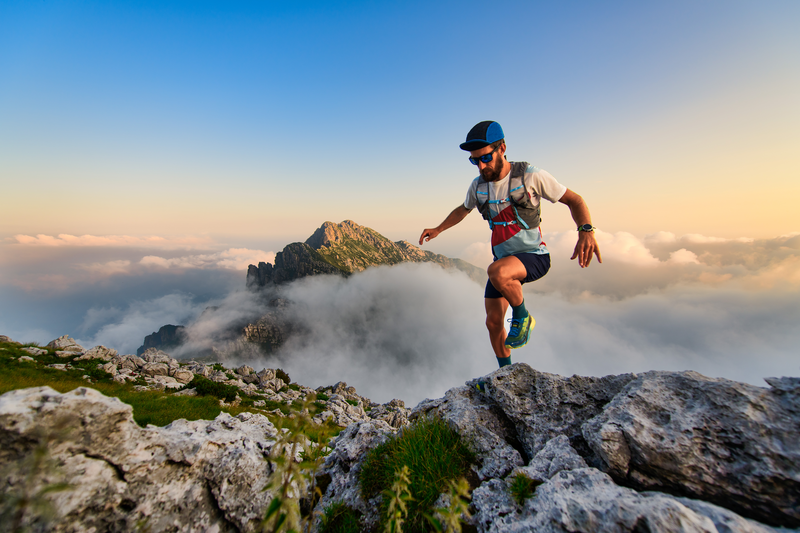
94% of researchers rate our articles as excellent or good
Learn more about the work of our research integrity team to safeguard the quality of each article we publish.
Find out more
ORIGINAL RESEARCH article
Front. Ecol. Evol. , 18 January 2022
Sec. Behavioral and Evolutionary Ecology
Volume 9 - 2021 | https://doi.org/10.3389/fevo.2021.802078
This article is part of the Research Topic What Sensory Ecology Might Learn From Landscape Ecology? View all 13 articles
Anthropogenic activities are changing the sensory landscape, interfering with transmission and reception of sexual signals. These changes are leading to alterations in mating behaviour with consequences to fitness. In systems where mate-finding involves long-distance signalling by one sex and approach by the other sex, the spatial distribution of signallers can have implications for male and female fitness. Spatial distribution of signallers is typically determined by an interplay of multiple factors, both ecological and evolutionary, including male competition, female choice and resources, such as calling and oviposition sites. We investigated the possible influence of resource distribution (signalling sites) on the strength and direction of sexual selection acting on false-leaf katydid Onomarchus uninotatus males, signalling in a human-modified landscape in the Western Ghats, India, a biodiversity hotspot. The landscape has changed from evergreen forests to plantations owing to human settlements. We first determined the spatial distribution of calling males and of available calling sites, which are trees of the genus Artocarpus, in the landscape. Using the information on male spacing, call transmission and hearing thresholds, the perceptual spaces of male signals were computed to understand the acoustic environment of calling males and females. It was found that both calling males and females could hear calls of males from neighbouring trees with a probability of 0.76 and 0.59, respectively. Although calling males were found to be spaced apart more than predicted by chance, significant overlap was seen in their acoustic ranges. Clustering of males enables females to easily sample multiple males, facilitating mate choice, but is detrimental to males as it increases competition for females. Using simulations, we determined the optimal spatial distributions of O. uninotatus males for female choice, and for reduction of male competition, given the signalling site distribution. The observed distribution of signallers was then compared with the hypothetical optimal distributions to examine the drivers of signaller spacing. Spacing of calling males in the field was found to be not optimal for either males or females. Resource distribution was found to limit the effectiveness of sexual selection drivers in pushing male spacing toward fitness optima of males or females.
In many animal species, mate-finding involves signalling by one sex and approach by the other (Bradbury and Vehrencamp, 1998), wherein the environment can have a direct bearing on the success of individuals in finding a potential mate (Römer, 1998). Anthropogenic disturbances to the environment can interfere with different sensory and physiological processes in organisms, affecting the expression, transmission, and reception of signals (Kern and Radford, 2016; Gurule-Small and Tinghitella, 2018). As a result, this can affect the perception of signals and mate-choice decisions of the receiver, leading to changes in the intensity of intra- and inter-sexual competition (Bent et al., 2021; Pilakouta and Ålund, 2021). For example, Candolin et al. (2007) showed the effect of reduced visibility in the breeding habitats of the three-spined stickleback Gasterosteus aculeatus owing to eutrophication. There was an increase in the courtship effort of males but without a proportional increase in mate attraction. In the red mason bee, Osmia bicornis, temperature has been shown to affect odour plumes, influencing female choice (Conrad et al., 2017). This, in turn, can affect the overall strength and direction of sexual selection.
The two components of sexual selection, male competition and female mate choice (Darwin, 1871), can act differently on traits in terms of the direction and strength of selection (Qvarnström and Forsgren, 1998; Moore and Moore, 1999; Candolin, 2004; Wong and Candolin, 2005; Yang and Richards-Zawacki, 2021). Female mate choice is expected to select for “superior males” with competitive advantage over other males, conferring direct or indirect benefits to the female (Qvarnström and Forsgren, 1998; Wong and Candolin, 2005). Under this assumption, male competition and female mate choice can favour the same traits, leading to reinforcing selection on these traits (Andersson, 1994; Berglund et al., 1996; Wiley and Poston, 1996). However, in cases where selection favouring male traits introduces costs on females, the interplay between the two forces can be counteracting (Qvarnström and Forsgren, 1998; Moore and Moore, 1999; Bussiere, 2002; Pitnick and García-González, 2002; Bonduriansky and Rowe, 2003).Therefore, studying these mechanisms singly or in isolation can lead to incomplete information about sexual selection on a trait (Hunt et al., 2009).
Understanding the possible effects of anthropogenic disturbances on the overall strength of sexual selection on a trait requires looking into effects of environmental change on the different mechanisms of sexual selection. There is a paucity of studies that have looked into the effect of human disturbances on these mechanisms separately to understand the cumulative effect of these changes on sexual selection.
Male orthopteran insects use long-range acoustic signals (calling songs) with species-specific temporal and spectral features for mate attraction (Robinson and Hall, 2002). These signals are used by females to locate conspecific males (Alexander, 1967). Intraspecific variation in these call features allow females to discriminate among signalling males and possibly exercise choice (Brown et al., 1996; Greenfield, 1997; Wagner, 1998; Brown, 1999; Gerhardt and Huber, 2002). The relative spacing of signalling males and females and the acoustic range of signals (distance at which the average sound pressure level of male calling song attenuates to the level of male/female hearing threshold) determines whether males and females are able to hear neighbouring callers. The detection ranges of their signals often extend beyond average inter-male distances, leading to overlaps in the acoustic ranges of callers (Mhatre and Balakrishnan, 2006; Ritz and Köhler, 2007). Such overlaps in acoustic ranges can have negative fitness consequences for the advertising sex, a fact that is well substantiated by both theoretical analysis (Forrest and Green, 1991; Forrest and Raspet, 1994) and empirical evidence (Arak et al., 1990; Greenfield, 1994; Farris et al., 1997).
Spacing apart is one of the strategies that male callers can employ to increase their success in attracting females by reducing masking of their signals: calling males in many systems space apart to avoid overlaps in their acoustic ranges (Whitney and Krebs, 1975; Campbell and Shipp, 1979; Bailey and Morris, 1986; Deb and Balakrishnan, 2014). This also reduces competition, enabling signallers to broadcast within a range free of competitors (Arak and Eiriksson, 1992; Deb and Balakrishnan, 2014). However, in many species, calling males also form clustered aggregations (Gerhardt and Huber, 2002; Mhatre and Balakrishnan, 2006; Ritz and Köhler, 2007).
Female mate choice is invoked as an explanation for the evolution of spatial clustering of advertising males. Females use different features of male acoustic signals to exercise choice (Latimer and Schatral, 1986; Forrest and Green, 1991; Tuckerman et al., 1993; Brown et al., 1996) and typically prefer call features with greater acoustical energy, such as longer call bout lengths and higher call rates (Ryan and Keddy-Hector, 1992; Brown, 1999). These features could be indicators of male quality due to the higher costs involved in their production (Loher and Dambach, 1989; Scheuber et al., 2003a,b). Exercising mate choice involves searching and sampling of potential mates, which has associated time and energetic costs, and risk of predation (Gwynne, 1987; Heller and Arlettaz, 1994; Bradbury and Vehrencamp, 1998; Raghuram et al., 2015; Rosenthal, 2017). Simultaneous sampling of signallers can help minimise search costs, and female mate choice can therefore be expected to drive spatial clustering of signallers, facilitating quick and easy assessment of potential mates (Morales et al., 2001; Murphy and Gerhardt, 2002; Murphy, 2012). This premise has been used to develop many theoretical models to explain the evolution of lekking systems (Bradbury, 1981; Gibson et al., 1990; Gibson, 1992).
An important ecological factor that can dictate spacing behaviour is the spatial distribution of resources such as food, potential mates, oviposition, and signalling sites (Emlen and Oring, 1977; Greenfield and Shelly, 1985; Shelly et al., 1987; Arak and Eiriksson, 1992; Hews, 1993). Male grasshoppers, Ligurotettix coquilletti distribute spatially according to the food plants most attractive to females (Greenfield and Shelly, 1985; Shelly et al., 1987). Spatial distribution of katydid Tettigonia viridissima and Amblycorypha parvipennis males was shown to be affected by availability of singing sites higher than the surroundings, which increases the acoustic range of their signals (Shaw et al., 1981; Arak and Eiriksson, 1992). Changes to spatial distribution of resources owing to human-induced changes in the landscape can affect communication in species, leading to changes in space use by organisms to facilitate communication.
Spacing behaviour of advertising males in acoustically communicating species has been well studied in anurans and orthopterans (Cade, 1981; Telford, 1985; Campbell, 1990; Dyson and Passmore, 1992; Bourne, 1993; Gerhardt and Huber, 2002; Mhatre and Balakrishnan, 2006; Ritz and Köhler, 2007; Deb and Balakrishnan, 2014). Most of these studies have examined male competition, female mate choice and resources as the key factors, albeit in isolation. There are only a few studies that have looked at a combination of these factors dictating male spacing (Shaw et al., 1982; Weidemann et al., 1990; Arak and Eiriksson, 1992; Allen, 1995) and none that have incorporated all three.
In this study, we examined the role of all three factors in spacing of signallers in an acoustically signalling, paleotropical false-leaf katydid species, Onomarchus uninotatus (Rajaraman et al., 2015), in a human-modified landscape. Since spacing behaviour can be an outcome of multiple factors, the role of each can be dissected by simulating different hypothetical spatial distributions of signallers, given the actual distribution of resources in the natural habitat, and then examining the implications of the different possible spatial distributions of calling males for male competition and female mate choice. This allows us to examine the optimal spatial distribution(s) that maximise male and female fitness, respectively and whether the actual distribution of signallers observed in the field benefits males and/or females.
We first examined the spatial distribution of O. uninotatus signallers in their natural habitat and compared it with the distribution of available calling sites. If calling males are not spaced randomly with respect to the distribution of calling sites, other factors such as male competition and/or female mate choice can be postulated to play a role in the spacing behaviour observed in the field. We then simulated different spatial distributions of calling males with varying minimum nearest neighbour distances using the actual distribution of available calling sites. For all simulated distributions, the number of females within the acoustic range of individual calling males and proportion of females that could hear multiple males were calculated, to quantify the implications of male spacing for male competition and female choice respectively. The theoretical optimal spatial distributions for both were determined and compared with the observed spatial distribution of males in the field to understand the effect of the two drivers (male competition and/or female choice) on male spacing behaviour, as well as the effect of resource distribution on the strength of sexual selection drivers in this species.
Onomarchus uninotatus (Serville 1838) is a canopy inhabiting, paleotropical false-leaf katydid species (Subfamily Pseudophyllinae: Family Tettigoniidae: Order Orthoptera). It has been described in Northeast India (Srinivasan and Prabakar, 2012), Southwestern India (Diwakar and Balakrishnan, 2007), China (de Jong, 1946), Malaysia (Heller, 1995), Indonesia (de Jong, 1946), and Australia (de Jong, 1946). Males have often been observed to call singly from Artocarpus trees (Nair, 2020, Ph.D. thesis). Both males and females feed on Artocarpus leaves, with females using the trees for oviposition as well. O. uninotatus has one breeding season per year, from November to April. The study was conducted in Kadari village in Karnataka, India (13°21′N–75°08′E) during the peak breeding season from January to May 2015 and 2016.
Random acoustic sampling of calling males was conducted in the study area during the peak calling hours (19:00–00:30 h). Calling O. uninotatus males were located by listening and the species of the tree on which the call was heard was recorded. The use of tree species by calling males was compared with the abundance of different tree species in the study area by calculating the selection ratio (proportion used/proportion available) (Manly et al., 2002) to examine preference for particular tree species as calling sites.
For abundance count of tree species in the study area, Girth at Breast Height (GBH) of tree species on which callers were found calling based on observations during random acoustic sampling was used as a criterion to define relevant calling sites. A distribution of GBH of the trees on which the callers were found was obtained by random sampling of around 50 trees of each plant species on which males were found calling. However, in the case of one of the tree species, Vateria indica, only 17 trees where available in the study area. From this distribution, a GBH cut-off of 20 cm was taken for the abundance count of trees, as only around 1% of the sampled trees had GBH < 20 cm (Supplementary Figure 1).
The area under study (1.35 km2) was gridded using Mapsource, QGIS, and Google Earth into 112 grids (each 111 m × 109 m). About 58 grids out of 112 were selected randomly for the abundance count of trees. A 300 m transect walk was carried out in each grid and the number of trees of Artocarpus spp. and non-Artocarpus spp. (with GBH ≥ 20 cm) within 5 m on each side of the transect was recorded. At every obstacle encountered, a random angle was chosen using a magnetic compass to continue the transect walk. Walking at the borders of the grid was avoided. The relative abundance of Artocarpus spp. and non-Artocarpus spp. was compared with the relative use of Artocarpus versus non-Artocarpus tree species as calling sites to examine preference of O. uninotatus for Artocarpus species.
GPS locations of all the Artocarpus trees in the study area (1.35 km2) were obtained using a GPS receiver (Garmin eTrex Vista HCx, United States) and the trees were also physically marked with individual codes using paint. A spatial map of the GPS locations of all the Artocarpus trees in the area was then obtained through QGIS 2.8.2. The spatial distribution of Artocarpus trees was analysed using a Clark and Evans test (Clark and Evans, 1954), which uses the nearest neighbour distances in a spatial point pattern as a measure of spatial relationships. The test compares the observed nearest neighbour distances with the expected nearest neighbour distances if the points were distributed randomly. The expected nearest neighbour distribution is generated by constructing the smallest possible rectangle over all points. If the mean observed nearest neighbour distribution is significantly lower than the expected mean for a random distribution, the test indicates a clustered distribution (Clark and Evans, 1954; Campbell, 1990).
Fifteen calling males were located in the canopy of Artocarpus trees, captured and marked on the pronotum with unique three-colour codes using non-toxic paint markers (Edding 780, Germany). The marked callers were released back at the site of capture. The tree codes and caller identification codes and GPS locations of trees were recorded. Each tree on which a marked caller was released was acoustically sampled for seven consecutive nights and, if a call was heard from the tree, an attempt was made at identifying the caller in the canopy.
The positions and identities of trees on which males were heard calling were recorded in the entire area under study. Over 16 nights, a total of 773 (83.5% of total trees) trees were sampled in the area under study for positions of callers during the peak calling hours (19:00–00:30 h). A cut-off of 25 min (obtained from calling effort data: refer to Supplementary Methods and Supplementary Figure 2) was used as the sampling time for each Artocarpus tree to determine whether a calling male was present on the tree. On an average around 47 trees were sampled each night and calling males on the sampled trees were located.
The spatial map of the callers in the study area was obtained using observations of the positions of the callers recorded on all 16 nights, which was then overlaid on the map of the Artocarpus trees using QGIS 2.8.2. It should be noted here that the exact GPS location of a caller within the canopy of the tree was not possible to attain. Therefore, the GPS location of the tree was taken as caller location, under the assumption that the caller was located at the centre of the tree. The distribution of the nearest neighbour distances of the trees was then compared with the nearest neighbour distribution of callers, and a randomisation test was performed using R (R Core Team, 2018) to investigate whether the callers were distributed randomly with respect to the trees or were clumped or dispersed. The observed difference between the mean nearest neighbour distance of trees and callers was compared to the generated expected difference between the mean nearest neighbour distance of trees and callers if the callers were to space randomly on trees. The distribution of the expected difference was generated using a randomisation test in R (R Core Team, 2018). Randomisation test was also performed on nearest neighbour distances of calling males and trees sampled on each night separately, as the Artocarpus tree distribution in the entire study area is a heterogeneous distribution, with high clustering in some pockets and more dispersed in others. Therefore, the nearest neighbour calculations were also performed separately for each night, as pooling of the nearest neighbour distances for the entire area can obscure any localised pattern in the spatial distribution of calling males. The test was performed using R (R Core Team, 2018).
Using the spatial map of calling males in the study area, acoustic ranges of calling males with respect to females were constructed. Acoustic range is represented as a circle with the caller as the center and radius defined by the distance at which the average sound pressure level of the male’s call at the center, around 71.9 ± 0.5 dB (Rajaraman et al., 2018), attenuates to the threshold of female hearing (35 dB SPL: Rajaraman et al., 2018). The average transmission distance for O. uninotatus calling song was computed using average sound pressure level at source, the SPL attenuation curve for the O. uninotatus call in the habitat (Jain, 2010) and average hearing threshold of females. To examine the acoustic environment of calling males, median overlap in the acoustic ranges along with the proportion of callers in the overlap areas was determined using R (R Core Team, 2018), by calculating the area of intersection in the acoustic ranges constructed around each point using gBuffer() and over() function from the rgeos package and sp package, respectively. To examine if the calling males could hear each other, the number of caller locations that lie within the areas of intersection of constructed acoustic ranges were then calculated in R (R Core Team, 2018).
In case of females, it was not possible to obtain their actual locations in the study area as they are acoustically silent. Therefore, two assumptions were made with respect to female locations: (1) one female to every available Artocarpus tree and (2) females located on randomly picked Artocarpus trees with an adult sex ratio of 1:1 (172 males and females). For the second assumption, multiple runs with random distributions of females were carried out in R (R Core Team, 2018). Proportion of females that might hear a male in an adjacent tree (in addition to the male in their tree) was then calculated under the two assumptions (R Core Team, 2018) by getting the female locations that are within the acoustic range of more than one male.
Using the measured Artocarpus tree locations, different caller distributions were simulated in R (R Core Team, 2018) with different minimum nearest neighbour distances, ranging from 5 to 50 m. This was done by sampling tree positions which were at a minimum specified distance from each other. In this way, 10 spatial distributions of calling males were obtained with minimum nearest neighbour distances of 5, 10, 15, 20 25, 30, 40, 45, and 50 m.
For each simulated distribution of calling males, two outcomes were computed: (1) average number of females that were within only one male’s acoustic range (as a measure of male competition) and (2) proportion of females that could hear more than one male caller (as a measure of female mate choice). Signalling in a zone free from neighbouring signallers reduces the number of females that are within acoustic ranges of multiple callers, thereby increasing a male’s probability of getting a mate. Therefore, measuring the average number of females that are within only one male’s acoustic range, would serve as a measure of fitness for males in the system. An aggregated distribution of males would increase the probability of occurrence of females in the overlap areas of signals of multiple callers, facilitating simultaneous sampling. Measuring the proportion of females in the population that are in the acoustic overlap areas enables us to study the type of spatial structure of signallers that benefits females in the system.
For female locations, two sets of simulations were carried out, based on the following assumptions: (1) one female to each tree and (2) females randomly located on the Artocarpus trees with an adult sex ratio of 1:1. A point to be noted here is that since the spatial distribution of available calling sites (Artocarpus trees) is constant for all simulations, the number of calling males changes in inverse relation with minimum nearest neighbour distance.
The two measured outcomes, (1) average number of females per male within only one male’s acoustic range (for male competition) and (2) proportion of females that could hear more than one caller (for female mate choice), were plotted against the minimum nearest neighbour distances of calling males, for each simulated distribution of calling males, to understand the implications of spatial distribution of signallers on male competition and female choice.
About 93.3% of the O. uninotatus calling males (28 out of 30) were found on trees of the genus Artocarpus. The use of Artocarpus species as calling sites was not however mirrored in their proportional availability. Artocarpus made up only around 2% of the total number of trees within the study area (121 out of 5148). The selection ratio (proportion used/proportion available) for Artocarpus spp. was found to be 39.7 and for non-Artocarpus spp. was found to be 0.06. This implies that the O. uninotatus males strongly prefer Artocarpus trees as calling sites.
Out of the 15 marked males, data could be obtained only for five males either because no call was heard during the sampling period from the tree across all days of sampling or because the caller was not visually accessible. These callers were found to maintain their calling sites (trees) across nights for an average of 78% ± 14.7% (mean ± SD) of the nights when a call was heard from the tree.
The distribution of nearest neighbour distances of Artocarpus trees was right skewed, with smaller distances dominating the distribution (Figure 1A). The Clark and Evans test showed that the distribution of Artocarpus trees in the study area was highly clustered (R = 0.51, z = -13.07, P < 0.005).
Figure 1. Comparison of nearest neighbour distances of calling males and trees pooled across 16 nights. (A) Distribution of nearest neighbour distances of Artocarpus trees across all plots (n = 773 trees). (B) Distributions of trees and callers superimposed. (C) Results of randomisation test. The observed difference between the mean nearest neighbour distance of trees and the mean nearest neighbour distance of callers is marked on the plot by the vertical dotted line.
The spatial locations of calling males in relation to the Artocarpus tree distribution (n = 773 trees) in the study area is shown in Figure 2. A total of 172 calling males were located in the study area. A visual comparison of the distribution of nearest neighbour (NN) distances of trees and that of callers revealed that the NN distances of callers were on average greater than of trees (Figure 1B). This suggests that calling males are dispersing and spacing out more than would be expected if they were to occupy trees randomly. To statistically test for overdispersion of calling males, data were pooled across nights and the spatial distributions of trees and callers were analysed using a randomisation test (Figure 1C). Callers were found to be spacing themselves apart since the observed difference between the mean NN distances of callers and of trees was found to be significantly higher than the expected difference if the callers were to distribute themselves randomly with respect to trees (Figure 1C).
The mean nearest neighbour distance of callers per night was 31.6 ± 3.8 m (mean ± SE, N = 16) and the mean nearest neighbour distance of trees on each night was 15.7 ± 3.6 m (mean ± SE, N = 16). Randomisation tests were also performed for each of the 16 nights separately, where observed difference between the nearest neighbour distances of callers and trees in the area sampled that night (ΔNNobs) was compared with the expected difference between nearest neighbour distances of callers and trees in the area sampled that night if callers were randomly distributed (ΔNNrandom). The males were seen to be distributing themselves non-randomly and spacing apart (Table 1). On fourteen out of sixteen nights, the observed difference in the nearest neighbour distances of callers and trees was found to be much higher than the expected difference. Two out of 16 nights (highlighted in red) showed a random pattern of distribution of callers (Table 1). This could be because the nearest neighbour tree distances in these two areas were much larger than other areas and were either almost equal to or more than the average nearest neighbour distance (32 m) that the callers were seen to maintain (Table 1).
The proportion of females that could hear more than one male when one female was assumed to be found on every Artocarpus tree was 0.59 (Figures 3A,B). Interestingly, this value did not change if the females were assumed to be randomly distributed, with a 1:1 sex ratio, where the median value was found to be 0.6 (Figure 3B). Therefore, there is a high chance that, given this spatial structure of callers and habitat, females can potentially hear multiple males irrespective of their relative distribution. There was considerable overlap in the acoustic ranges of the calling males. The median overlap in the acoustic ranges of males was 24.4% (IQR = 7.57–51.6%) with maximum overlap of 96% and minimum overlap of 0.024% (Figure 3C).
Figure 3. Acoustic environment of calling males and females. (A) Figure depicting the acoustic ranges of a small sample of callers (taken from the spatial map of callers), with the callers (circles) as the centres of circles, the radius of which is defined by the distance at which the average SPL of the male’s call drops to the female hearing threshold. Triangles indicate assumed locations of individual females, where females are assumed to be one per each tree. (B) Proportion of females that could potentially hear more than one male (apart from the male on their tree) under the two assumptions: (1) One female to each tree (nfemales = 773, nmales = 172), (2) Females randomly distributed on trees (nfemales = 172, nmales = 172); (C) Percentage overlap in acoustic ranges of males (n = 172).
Under both assumptions for female locations, implications of male spacing for males and females showed opposite trends with increasing minimum nearest neighbour distances between calling males (Figures 4A,B). When females were one to each tree (Figure 4A), the proportion of females who could hear multiple males, a situation conducive for mate choice, starts showing a decline once the minimum nearest neighbour distance between males exceeds 25 m. On the other hand, for males, average number of females per male that are confined to acoustic range of only one male, increases with increasing distance between males. The number of females exceeds 1 when the minimum nearest neighbour distance between males is 35 m or more (Figure 4A). Therefore, by increasing their inter-individual distances, callers can increase their probability of getting a mate, reducing male–male competition. As expected, male competition is pushing the spatial structure of callers to a more dispersed distribution. On the other hand, female choice seems to be pushing the signaller distribution to a more clustered distribution. With decreasing nearest neighbour distances of callers, proportion of females in the population that can hear multiple males approaches 1, indicating that at highly clustered distribution of calling males, nearly every female in the population can hear multiple males. The observed range of nearest neighbour distances maintained by maximum number of callers in the field (15–30 m), lies in between the two optima. This indicates a counteracting effect of male competition and female choice on male spacing behaviour in this species.
Figure 4. Effect of male spacing on fitness of calling males and females under the assumptions of (A) one female to each Artocarpus tree and (B) females randomly distributed on Artocarpus trees with 1:1 sex ratio. Dotted lines represent females in non-overlap areas (male fitness) and solid lines represent proportion of females that can hear multiple males (female fitness/mate choice). The shaded region represents the observed range of distribution of nearest neighbour (NN) distances maintained by calling males in the field.
When females are randomly distributed with 1:1 sex ratio, the trend does not change much for females. However, for males, the average number of females per male that are within only one male’s acoustic range does not even reach one, even when the males are at least 50 m apart (Figure 4B). This means that when females are distributed randomly on Artocarpus trees with 1:1 sex ratio, probability of securing a mate is low for calling males even when males are spacing apart.
Onomarchus uninotatus calling males displayed non-random spacing and were found to be overdispersed. However, unlike other systems where males space apart to avoid or reduce overlaps in their signal ranges (Whitney and Krebs, 1975; Campbell and Shipp, 1979; Deb and Balakrishnan, 2014), there was considerable overlap observed in the acoustic ranges of O. uninotatus calling males. This points to a possible role of other factors such as female sampling or resource clustering in the observed spacing behaviour of calling males.
Manipulating the spacing between callers through simulations indicates that the two mechanisms of sexual selection have conflicting effects on male spacing behaviour, with different selective optima for nearest neighbour distances between callers. Calling males benefit more in terms of reduced competition (measured here as number of females confined to acoustic ranges of only one male) when the males maintain a minimum distance of 35 m or more: in the case of one female to each tree, males have at least one female in their acoustic ranges that is not in the overlap area of multiple callers. For females, on the other hand, a clustered assemblage of males with less than 10 m nearest neighbour distances, is conducive for simultaneous sampling of multiple calling males. In the natural population, the nearest neighbour distance maintained by maximum number of callers lies in the range of 15–30 m which is in between the two optima. This range, however, does not seem to be equally advantageous for both males and females. Whereas a high proportion of females (∼0.9) are privy to multiple calling males at a time, males are unable to avoid competition. On average, males do not have even one female that is confined to the acoustic range of only one caller.
The landscape under study has been transformed from evergreen forests to plantations owing to human settlement, leading to a clustered distribution of Artocarpus trees, which are planted by humans. This clustering of Artocarpus trees in the landscape seems to have differential effects on female choice and male competition. From the field data, it can be seen that females are able to hear multiple males and the probability of being able to hear a calling male from an adjacent tree is not affected by the spatial distribution of the females. This is due to the aggregated distribution of Artocarpus trees to which the females are limited. This, combined with large acoustic ranges of the calling males (∼45 m), results in most of the Artocarpus trees falling within the acoustic range of one or more callers. Therefore, irrespective of the female distribution, the possibility of a given female hearing a caller on another tree is high.
Females have been shown to use calling songs for discriminating among males and show preferential attraction for certain call features (Hedrick, 1986; Tuckerman et al., 1993; Gerhardt and Huber, 2002). Best-of-n and threshold strategy, two popular models for mate-sampling, show reduced fitness with increasing search costs (Real, 1990). In O. uninotatus, which is a canopy-inhabiting katydid, movement across trees in response to male calls could put females under high predation risk. Raghuram et al. (2015) observed, in flight cage experiments, that the predatory bat, Megaderma spasma, a known predator of O. uninotatus, responded 100% of the time to tethered females in flight as opposed to about 30% of the time to male calls. Therefore, females would benefit from an aggregated distribution of males as it increases their access to multiple males without having to sequentially sample the callers, which would require them to fly between multiple trees.
Males, on the other hand, can increase the probability of attracting a mate by choosing calling positions which would result in multiple Artocarpus trees falling within their acoustic ranges, as females are also confined to Artocarpus trees for the purposes of foraging and oviposition (Nair and Rajaraman, personal observations). However, given that the calls have a large acoustic range of 45 m and are in an area with clumped distribution of calling sites, a caller simultaneously runs the risk of overlap of his acoustic range with other callers in the vicinity. Males bear a fitness cost when they have overlapping acoustic ranges with other callers (Arak et al., 1990; Farris et al., 1997; Mhatre and Balakrishnan, 2006). This suggests that males should avoid overlap altogether to reduce competition. However, it can be seen from the data that males are unable to avoid acoustic overlaps.
From the simulations, it can be seen that, with a minimum nearest neighbour distance of 40 m or more, the average number of females per male in the non-overlap areas increases to ∼3, when there is one female to each tree. However, at a minimum nearest neighbour distance of 50 m, given the clustered distribution of Artocarpus trees, the number of calling males reduces to 141, whereas in the wild the number of calling males recorded were 172. Therefore, the distribution of calling sites seems to be placing a constraint on the extent to which males can space apart and avoid overlap.
These results show that anthropogenic changes can affect sexual selection on traits, with the effect varying between the two components of sexual selection. Human disturbances can influence the sexes differently and this highlights the importance of partitioning the effects of these disturbances on the different mechanisms of selection. It also points to the importance of interaction between evolutionary and ecological factors in shaping phenotypes.
The raw data supporting the conclusions of this article will be made available by the authors, without undue reservation.
Ethical clearance for carrying out this project was obtained from the Institutional Animal Ethics Committee (IAEC), Indian Institute of Science (Project No. CAF/Ethics/519/2016).
RB and AN designed the study, interpreted the results, and wrote the manuscript. AN performed the field observations and simulations and carried out the data analyses. Both authors gave final approval for publication.
This study was financially supported by the DST-SERB, Govt. of India (Grant number EMR/2016/002293 to RB) and the DBT-IISc Partnership Program (Phase II, Grant number BT/PR27952/INF/22/212/2018, Govt. of India).
The authors declare that the research was conducted in the absence of any commercial or financial relationships that could be construed as a potential conflict of interest.
All claims expressed in this article are solely those of the authors and do not necessarily represent those of their affiliated organizations, or those of the publisher, the editors and the reviewers. Any product that may be evaluated in this article, or claim that may be made by its manufacturer, is not guaranteed or endorsed by the publisher.
We thank Sudhakar Malekudiya Gowda and Balakrishna for their help in carrying out observations and experiments in the field. We thank Navendu Page for his help with the methodology for abundance count of Artocarpus trees. We also thank Aakanksha Rathore for helpful discussions regarding the simulations. We thank the Ministry of Human Resource development, Govt. of India for the student fellowship to AN.
The Supplementary Material for this article can be found online at: https://www.frontiersin.org/articles/10.3389/fevo.2021.802078/full#supplementary-material
Alexander, R. D. (1967). Acoustical communication in arthropods. Annu. Rev. Entomol. 12, 495–526. doi: 10.1146/annurev.en.12.010167.002431
Allen, G. R. (1995). The calling behaviour and spatial distribution of male bushcrickets (Sciarasaga quadrata) and their relationship to parasitism by acoustically orienting tachinid flies. Ecol. Entomol. 20, 303–310. doi: 10.1111/j.1365-2311.1995.tb00461.x
Andersson, M. (1994). Sexual Selection. Princeton, NJ: Princeton Univ. Press. doi: 10.1016/0024-4066(95)90031-4
Arak, A., and Eiriksson, T. (1992). Choice of singing sites by male bushcrickets (Tettigonia viridissima) in relation to signal propagation. Behav. Ecol. Sociobiol. 30, 365–372. doi: 10.1007/bf00176170
Arak, A., Eiriksson, T., and Radesäterthirteen, T. (1990). The adaptive significance of acoustic spacing in male bushcrickets Tettigonia viridissima: a perturbation experiment. Behav. Ecol. Sociobiol. 26, 1–7. doi: 10.1007/bf00174019
Bailey, W. J., and Morris, G. K. (1986). Confusion of phonotaxis by masking sounds in the bushcricket Conocephalus brevipennis (Tettigoniidae: Conocephalinae). Ethology 73, 19–28. doi: 10.1111/j.1439-0310.1986.tb00996.x
Bent, A. M., Ings, T. C., and Mowles, S. L. (2021). Anthropogenic noise disrupts mate choice behaviors in female Gryllus bimaculatus. Behav. Ecol. 32, 201–210. doi: 10.1093/beheco/araa124
Berglund, A., Bisazza, A., and Pilastro, A. (1996). Armaments and ornaments: an evolutionary explanation of traits of dual utility. Biol. J. Linn. Soc. 58, 385–399. doi: 10.1111/j.1095-8312.1996.tb01442.x
Bonduriansky, R., and Rowe, L. (2003). Interactions among mechanisms of sexual selection on male body size and head shape in a sexually dimorphic fly. Evolution 57, 2046–2053. doi: 10.1111/j.0014-3820.2003.tb00384.x
Bourne, G. R. (1993). Proximate costs and benefits of mate acquisition at leks of the frog Ololygon rubra. Anim. Behav. 45, 1051–1059. doi: 10.1006/anbe.1993.1131
Bradbury, J. W. (1981). “The evolution of leks,” in Natural Selection and Social Behavior, eds R. D. Alexander and D. W. Tinkle (New York: Chiron), 138–169.
Bradbury, J. W., and Vehrencamp, S. L. (1998). Principles of Animal Communication. Sunderland, MA: Sinauer Associates.
Brown, W. D. (1999). Mate choice in tree crickets and their kin. Annu. Rev. Entomol. 44, 371–396. doi: 10.1146/annurev.ento.44.1.371
Brown, W. D., Wideman, J., Andrade, M. C., Mason, A. C., and Gwynne, D. T. (1996). Female choice for an indicator of male size in the song of the black-horned tree cricket, Oecanthus nigricornis (Orthoptera: Gryllidae: Oecanthinae). Evolution 50, 2400–2411. doi: 10.1111/j.1558-5646.1996.tb03627.x
Bussiere, L. F. (2002). A model of the interaction between ‘good genes’ and direct benefits in courtship-feeding animals: when do males of high genetic quality invest less? Philos. Trans. R. Soc. Lond. B. 357, 309–317. doi: 10.1098/rstb.2001.0922
Cade, W. H. (1981). Field cricket spacing, and the phonotaxis of crickets and parasitoid flies to clumped and isolated cricket songs. Z. Tierpsychol. 55, 365–375. doi: 10.1111/j.1439-0310.1981.tb01278.x
Campbell, D. J. (1990). Resolution of spatial complexity in a field sample of singing crickets Teleogryllus commodus (Walker)(Gryllidae): a nearest-neighbour analysis. Anim. Behav. 39, 1051–1057. doi: 10.1016/s0003-3472(05)80777-8
Campbell, D. J., and Shipp, E. (1979). Regulation of spatial pattern in populations of the field cricket Teleogryllus commodus (Walker). Z. Tierpsychol. 51, 260–268. doi: 10.1111/j.1439-0310.1979.tb00688.x
Candolin, U. (2004). Opposing selection on a sexually dimorphic trait through female choice and male competition in a water boatman. Evolution 58, 1861–1864. doi: 10.1111/j.0014-3820.2004.tb00470.x
Candolin, U., Salesto, T., and Evers, M. (2007). Changed environmental conditions weaken sexual selection in sticklebacks. J. Evol. Biol. 20, 233–239. doi: 10.1111/j.1420-9101.2006.01207.x
Clark, P. J., and Evans, F. C. (1954). Distance to nearest neighbor as a measure of spatial relationships in populations. Ecology 35, 445–453. doi: 10.2307/1931034
Conrad, T., Stöcker, C., and Ayasse, M. (2017). The effect of temperature on male mating signals and female choice in the red mason bee Osmia bicornis (L.). Ecol. Evol. 7, 8966–8975. doi: 10.1002/ece3.3331
Darwin, C. (1871). The Descent of Man, and Selection in Relation to Sex. London: John Murray, doi: 10.5962/bhl.title.24784
de Jong, C. (1946). A new variety of an Onomarchus from British India (Orthoptera, Tettigoniidae). Zool. Meded. 26, 268–270.
Deb, R., and Balakrishnan, R. (2014). The opportunity for sampling: the ecological context of female mate choice. Behav. Ecol. 25, 967–974. doi: 10.1093/beheco/aru072
Diwakar, S., and Balakrishnan, R. (2007). The assemblage of acoustically communicating crickets of a tropical evergreen forest in southern India: call diversity and diel calling patterns. Bioacoustics 16, 113–135. doi: 10.1080/09524622.2007.9753571
Dyson, M. L., and Passmore, N. I. (1992). Inter-male spacing and aggression in African painted reed frogs, Hyperolius marmoratus. Ethology 91, 237–247. doi: 10.1111/j.1439-0310.1992.tb00865.x
Emlen, S. T., and Oring, L. W. (1977). Ecology, sexual selection, and the evolution of mating systems. Science 197, 215–223. doi: 10.1126/science.327542
Farris, H. E., Forrest, T. G., and Hoy, R. R. (1997). The effects of calling song spacing and intensity on the attraction of flying crickets (Orthoptera: Goryllidae: Nemobiinae). J. Insect. Behav. 10, 639–653. doi: 10.1007/BF02765384
Forrest, T. G., and Green, D. M. (1991). Sexual selection and female choice in mole crickets (Scapteriscus: Gryllotalpidae): modelling the effects of intensity and male spacing. Bioacoustics 3, 93–109. doi: 10.1080/09524622.1991.9753166
Forrest, T. G., and Raspet, R. (1994). Models of female choice in acoustic communication. Behav. Ecol. 5, 293–303. doi: 10.1093/beheco/5.3.293
Gerhardt, H. C., and Huber, F. (2002). Acoustic Communication in Insects and Anurans: Common Problems and Diverse Solutions. Chicago, IL: University of Chicago Press.
Gibson, R. M. (1992). Lek formation in sage grouse: the effect of female choice on male territory settlement. Anim. Behav. 43, 443–450. doi: 10.1016/S0003-3472(05)80103-4
Gibson, R. M., Taylor, C. E., and Jefferson, D. R. (1990). Lek formation by female choice: a simulation study. Behav. Ecol. 1, 36–42. doi: 10.1093/beheco/1.1.36
Greenfield, M. D. (1994). Synchronous and alternating choruses in insects and anurans: common mechanisms and diverse functions. Am. Zool. 34, 605–615. doi: 10.1093/icb/34.6.605
Greenfield, M. D. (1997). “Acoustic communication in Orthoptera,” in The Bionomics of Grasshoppers, Katydids and their Kin, eds S. K. Gangwere, M. C. Muralirangan, and M. Muralirangan (Wallingford: CABI), 197–230. doi: 10.5860/choice.35-3861
Greenfield, M. D., and Shelly, T. E. (1985). Alternative mating strategies in a desert grasshopper: evidence of density-dependence. Anim. Behav. 33, 1192–1210. doi: 10.1016/S0003-3472(85)80180-9
Gurule-Small, G. A., and Tinghitella, R. M. (2018). Developmental experience with anthropogenic noise hinders adult mate location in an acoustically signalling invertebrate. Biol. Lett. 14:20170714. doi: 10.1098/rsbl.2017.0714
Gwynne, D. T. (1987). Sex-biased predation and the risky mate-locating behaviour of male tick-tock cicadas (Homoptera: Cicadidae). Anim. Behav. 35, 571–576. doi: 10.1016/s0003-3472(87)80283-x
Hedrick, A. V. (1986). Female preferences for male calling bout duration in a field cricket. Behav. Ecol. Sociobiol. 19, 73–77. doi: 10.1007/BF00303845
Heller, K. G. (1995). Acoustic signalling in palaeotropical bushcrickets (Orthoptera: Tettigonioidea: Pseudophyllidae): does predation pressure by eavesdropping enemies differ in the Palaeo-and Neotropics? J. Zool. 237, 469–485. doi: 10.1111/j.1469-7998.1995.tb02775.x
Heller, K. G., and Arlettaz, R. (1994). Is there a sex ratio bias in the bushcricket prey of the scops owl due to predation on calling males? J. Orthoptera Res. 2, 41–42. doi: 10.2307/3503607
Hews, D. K. (1993). Food resources affect female distribution and male mating opportunities in the iguanian lizard Uta palmeri. Anim.Behav. 46, 279–291. doi: 10.1006/anbe.1993.1189
Hunt, J., Breuker, C. J., Sadowski, J. A., and Moore, A. J. (2009). Male–male competition, female mate choice and their interaction: determining total sexual selection. J. Evol. Biol. 22, 13–26. doi: 10.1111/j.1420-9101.2008.01633.x
Jain, M. (2010). Habitat Acoustics and Microhabitat Selection in an Ensiferan Assemblage of a Tropical Evergreen Forest. Ph.D. thesis. Bengaluru: Indian Institute of Science.
Kern, J. M., and Radford, A. N. (2016). Anthropogenic noise disrupts use of vocal information about predation risk. Environ. Pollut. 218, 988–995. doi: 10.1016/j.envpol.2016.08.049
Latimer, W., and Schatral, A. (1986). Information cues used in male competition by Tettigonia cantans (Orthoptera: Tettigoniidae). Anim. Behav. 34, 162–168. doi: 10.1016/0003-3472(86)90019-9
Loher, W., and Dambach, M. (1989). “Reproductive behavior,” in Cricket Behavior and Neurobiology, eds F. Huber, T. E. Moore, and W. Loher (Ithaca, NY: Cornell University Press), 43–82. doi: 10.7591/9781501745904-004
Manly, B. F. J., McDonald, L. L., Thomas, D. L., McDonald, T. L., and Erickson, W. P. (2002). Resource Selection by Animals: Statistical Design and Analysis for Field Studies, 2nd Edn. Dordrecht: Kluwer Academic.
Mhatre, N., and Balakrishnan, R. (2006). Male spacing behaviour and acoustic interactions in a field cricket: implications for female mate choice. Anim. Behav. 72, 1045–1058. doi: 10.1016/j.anbehav.2006.02.022
Moore, A. J., and Moore, P. J. (1999). Balancing sexual selection through opposing mate choice and male competition. Proc. R. Soc. Lond. B 266, 711–716. doi: 10.1098/rspb.1999.0694
Morales, M. B., Jiguet, F., and Arroyo, B. (2001). Exploded leks: what bustards can teach us. Ardeola 48, 85–98. doi: 10.13157/arla.60.1.2012.85
Murphy, C. G. (2012). Simultaneous mate-sampling by female barking treefrogs (Hyla gratiosa). Behav. Ecol. 23, 1162–1169. doi: 10.1093/beheco/ars093
Murphy, C. G., and Gerhardt, H. C. (2002). Mate sampling by female barking treefrogs (Hyla gratiosa). Behav. Ecol. 13, 472–480. doi: 10.1093/beheco/13.4.472
Nair, A. (2020). ). Multimodal Duetting and Pair Formation in a Paleotropical False Leaf Katydid, Onomarchus uninotatus. Ph.D. thesis. Bengaluru: Indian Institute of Science.
Pilakouta, N., and Ålund, M. (2021). Sexual selection and environmental change: what do we know and what comes next? Curr. Zool. 67, 293–298. doi: 10.1093/cz/zoab021
Pitnick, S., and García-González, F. (2002). Harm to females increases with male body size in Drosophila melanogaster. Proc. R. Soc. Lond. B 269, 1821–1828. doi: 10.1098/rspb.2002.2090
Qvarnström, A., and Forsgren, E. (1998). Should females prefer dominant males? Trends Ecol. Evol. 13, 498–501. doi: 10.1016/S0169-5347(98)01513-4
R Core Team. (2018). R: A Language and Environment for Statistical Computing. Vienna: R Foundation for Statistical Computing.
Raghuram, H., Deb, R., Nandi, D., and Balakrishnan, R. (2015). Silent katydid females are at higher risk of bat predation than acoustically signalling katydid males. Proc. R. Soc. Lond. B Biol. Sci. 282, 20142319. doi: 10.1098/rspb.2014.2319
Rajaraman, K., Godthi, V., Pratap, R., and Balakrishnan, R. (2015). A novel acoustic-vibratory multimodal duet. J. Exp. Biol. 218, 3042–3050.
Rajaraman, K., Nair, A., Dey, A., and Balakrishnan, R. (2018). Response mode choice in a multimodally duetting paleotropical pseudophylline bushcricket. Front. Ecol. Evol. 6:172. doi: 10.3389/fevo.2018.00172
Real, L. (1990). Search theory and mate choice. I. Models of single-sex discrimination. Am. Nat. 136, 376–405. doi: 10.1086/285103
Ritz, M. S., and Köhler, G. (2007). Male behaviour over the season in a wild population of the field cricket Gryllus campestris L. Ecol. Entomol. 32, 384–392. doi: 10.1111/j.1365-2311.2007.00887.x
Robinson, D. J., and Hall, M. J. (2002). “Sound signalling in Orthoptera,” in Advances in Insect Physiology, Vol. 29, ed. P. Evans (New York, NY: Elsevier Ltd), 151–278. doi: 10.1016/S0065-2806(02)29003-7
Römer, H. (1998). “The sensory ecology of acoustic communication in insects,” in Comparative Hearing: Insects, eds R. R. Hoy, A. N. Popper, and R. R. Fay (New York, NY: Springer), 63–96. doi: 10.1007/978-1-4612-0585-2_3
Rosenthal, G. G. (2017). Mate Choice: The Evolution of Sexual Decision Making from Microbes to Humans. Princeton NJ: Princeton University Press. doi: 10.1515/9781400885466
Ryan, M. J., and Keddy-Hector, A. (1992). Directional patterns of female mate choice and the role of sensory biases. Am. Nat. 139, S4–S35. doi: 10.1086/285303
Scheuber, H., Jacot, A., and Brinkhof, M. W. (2003a). Condition dependence of a multicomponent sexual signal in the field cricket Gryllus campestris. Anim. Behav. 65, 721–727. doi: 10.1006/anbe.2003.2083
Scheuber, H., Jacot, A., and Brinkhof, M. W. (2003b). The effect of past condition on a multicomponent sexual signal. Proc. R. Soc. Lond. B 270, 1779–1784. doi: 10.1098/rspb.2003.2449
Shaw, K. C., Bitzer, R. J., and North, R. C. (1982). Spacing and movement of Neoconocephalus ensiger males (Conocephalinae: Tettigoniidae). J. Kans. Entomol. Soc. 55, 581–592.
Shaw, K. C., North, R. C., and Meixner, A. J. (1981). Movement and spacing of singing Amblycorypha parvipennis males. Ann. Entomol. Soc. Am. 74, 436–444. doi: 10.1093/aesa/74.5.436
Shelly, T. E., Greenfield, M. D., and Downum, K. R. (1987). Variation in host plant quality: influences on the mating system of desert grasshopper. Anim. Behav. 35, 1200–1209. doi: 10.1016/S0003-3472(87)80177-X
Srinivasan, G., and Prabakar, D. (2012). Additional records of Tettigoniidae from Arunachal Pradesh, India. J Threat. Taxa 4, 3255–3268. doi: 10.11609/JoTT.o3065.3255-68
Telford, S. R. (1985). Mechanisms and evolution of inter-male spacing in the painted reedfrog (Hyperolius marmoratus). Anim. Behav. 33, 1353–1361. doi: 10.1016/S0003-3472(85)80198-6
Tuckerman, J. F., Gwynne, D. T., and Morris, G. K. (1993). Reliable acoustic cues for female mate preference in a katydid (Scudderia curvicauda, Orthoptera: Tettigoniidae). Behav. Ecol. 4, 106–113. doi: 10.1093/beheco/4.2.106
Wagner, W. E. (1998). Measuring female mating preferences. Anim. Behav. 55, 1029–1042. doi: 10.1006/anbe.1997.0635
Weidemann, S., Stiedl, O., and Kalmring, K. (1990). Distribution and population density of the bushcricket Decticus verrucivorus in a damp-meadow biotope. Oecologia 82, 369–373. doi: 10.1007/BF00317485
Whitney, C. L., and Krebs, J. R. (1975). Spacing and calling in Pacific tree frogs, Hyla regilla. Can. J. Zool. 53, 1519–1527. doi: 10.1139/z75-187
Wiley, R. H., and Poston, J. (1996). Perspective: indirect mate choice, competition for mates, and coevolution of the sexes. Evolution 50, 1371–1381. doi: 10.1111/j.1558-5646.1996.tb03911.x
Wong, B. B., and Candolin, U. (2005). How is female mate choice affected by male competition? Biol. Rev. 80, 559–571. doi: 10.1017/s1464793105006809
Keywords: acoustic signals, acoustic range, female mate choice, male competition, Orthoptera, spatial distribution, anthropogenic disturbances
Citation: Nair A and Balakrishnan R (2022) Ecological Constraints on Sexual Selection in a Human-Modified Landscape. Front. Ecol. Evol. 9:802078. doi: 10.3389/fevo.2021.802078
Received: 26 October 2021; Accepted: 14 December 2021;
Published: 18 January 2022.
Edited by:
Daniel Marques Almeida Pessoa, Federal University of Rio Grande do Norte, BrazilReviewed by:
Michael Greenfield, UMR 7261 Institut de Recherche sur la Biologie de l’insecte (IRBI), FranceCopyright © 2022 Nair and Balakrishnan. This is an open-access article distributed under the terms of the Creative Commons Attribution License (CC BY). The use, distribution or reproduction in other forums is permitted, provided the original author(s) and the copyright owner(s) are credited and that the original publication in this journal is cited, in accordance with accepted academic practice. No use, distribution or reproduction is permitted which does not comply with these terms.
*Correspondence: Aswathy Nair, YXN3YXRoeW5haXJAYWx1bS5paXNjLmFjLmlu; Rohini Balakrishnan, YnJvaGluaUBpaXNjLmFjLmlu
Disclaimer: All claims expressed in this article are solely those of the authors and do not necessarily represent those of their affiliated organizations, or those of the publisher, the editors and the reviewers. Any product that may be evaluated in this article or claim that may be made by its manufacturer is not guaranteed or endorsed by the publisher.
Research integrity at Frontiers
Learn more about the work of our research integrity team to safeguard the quality of each article we publish.