- 1Department of Crop Production Ecology, Swedish University of Agricultural Sciences, Uppsala, Sweden
- 2Department of Biology, Lund University, Lund, Sweden
- 3Department of Ecology, Swedish University of Agricultural Sciences, Uppsala, Sweden
Ability to efficiently localize productive foraging habitat is crucial for nesting success of insectivorous birds. Some bird species can use olfaction to identify caterpillar-infested trees by detection of herbivore induced plant volatiles (HIPVs), but these cues probably need to be learned. So far, we know very little about the process of olfactory learning in birds, whether insectivorous species have a predisposition for detecting and learning HIPVs, due to the high ecological significance of these odors, and how olfaction is integrated with vision in making foraging decisions. In a standardized setup, we tested whether 35 wild-caught great tits (Parus major) show any preference for widely abundant HIPVs compared to neutral (non-induced) plant odors, how fast they learn to associate olfactory, visual and multimodal foraging cues with food, and whether the olfactory preferences and learning speed were influenced by bird sex or habitat (urban or rural). We also tested how fast birds switch to a new cue of the same modality. Great tits showed no initial preference for HIPVs compared to neutral odors, and they learned all olfactory cues at a similar pace, except for methyl salicylate (MeSA), which they learned more slowly. We also found no differences in learning speeds between visual, olfactory and multimodal foraging cues, but birds learned the second cue they were offered faster than the first one. Bird sex or habitat had no effect on learning speed or olfactory preference, but urban birds tended to learn visual cues more slowly. We conclude that insectivorous birds utilize olfactory and visual cues with similar efficiency in foraging, and that they probably don‘t have any special predisposition toward the tested HIPVs. These results confirm that great tits are flexible foragers with good learning abilities.
Introduction
Sensory systems of birds are usually adapted to optimize foraging behavior, which involves detecting and identifying relevant habitat cues that indicate presence of food (Martin, 2012). For insectivorous birds, abundance and distribution of prey varies across seasons; thus, birds must be able to efficiently localize and track productive foraging habitats in space and time (Murakami, 2002; Visser et al., 2006). Particularly during the nestling period, optimal timing and high foraging efficiency is crucial for the reproductive success of breeding birds (Tremblay et al., 2003; Shiao et al., 2019). Yet, we still know relatively little about how different sensory cues contribute to guiding bird foraging behavior.
Birds generally have more advanced visual abilities than mammals, for example with regards to color discrimination (e.g., Kelber, 2019) and movement detection (Boström et al., 2016; Donner, 2021). Thus, vision is regarded as the predominant sense in birds and visual discrimination tasks are commonly used to study bird behavior. Great tits (Parus major), for example, can learn to associate food reward with specific colors (Amy et al., 2012; Morand-Ferron et al., 2015; Outomuro et al., 2020). They also possess a highly accurate observational spatial memory, which possibly requires advanced processing of visual information (Brodin and Urhan, 2014, 2015).
Even though olfaction previously has been dismissed as being unimportant for most bird species, it has turned out to play an important role in bird foraging behavior (Mäntylä et al., 2004; Hiltpold and Shriver, 2018; Potier, 2020; Danel et al., 2021). Some bird species can use their sense of smell to identify caterpillar-infested trees by detection of herbivore induced plant volatiles (HIPVs) (Mäntylä et al., 2004, 2020; Amo et al., 2013). HIPVs are chemical compounds, which are released by plants under attack, for example by herbivorous insect larvae. These compounds play an important role in mediating tri-trophic interactions between plants, herbivores and their arthropod predators (Dicke et al., 2003; Dicke, 2015). Herbivorous larvae may also be the most important part of the diet for many bird species, especially while provisioning their young, which suggests that HIPVs could be important for foraging efficiency. Indeed, several studies have demonstrated bird attraction to or preference for HIPVs both in the laboratory (Amo et al., 2013; Mäntylä et al., 2017) and in the field (Mrazova and Sam, 2017; Hiltpold and Shriver, 2018; Rubene et al., 2019).
In order to develop a foraging preference for HIPVs, based on current evidence from great tits, these cues need to be learned through feeding experience. For instance, naïve great tits show no preference for trees releasing HIPVs (Amo et al., 2016; Sam et al., 2021), but they can develop a preference after repeated exposure, for both native and exotic tree species (Sam et al., 2021). Sam et al. (2021) found no evidence that birds were able to generalize between familiar and novel plant species based on shared HIPVs. Still, the authors suggested that generalization in olfactory learning might be important in habitats with high plant diversity, like tropical forests, where the ability to respond to specific HIPVs shared by many species might be more adaptive than learning one plant odor at a time (Sam et al., 2021). It is still unclear, whether insectivorous birds have a predisposition for detecting and learning HIPVs (e.g., because these odors have high ecological significance during nestling provision) and whether birds respond to specific compounds or complex blends. There is also a lack of understanding of the process of olfactory learning in birds, and how olfaction is integrated with vision in making foraging decisions. Comparisons of visual and olfactory learning under standardized conditions are therefore needed to gain insight in the relative importance of different sensory cues for bird foraging behavior.
In this study, we used wild-caught great tits in a standardized laboratory setup to assess olfactory preferences for widely abundant HIPVs shared by many plant species and non-induced plant odors, and compared learning of olfactory, visual and multimodal (combination of olfactory and visual) foraging cues. Great tits are generalist foragers that can exploit different types of resources, including many anthropogenic ones. They are well-known for their innovative foraging skills (Estók et al., 2010; Cole et al., 2011) and possess advanced cognitive abilities (Brodin and Urhan, 2014; Isaksson et al., 2018). Great tits have also been used in several studies investigating detection of HIPVs (Amo et al., 2013; Mäntylä et al., 2017; Sam et al., 2021), which makes them a suitable species for our study.
Our aim was to (i) determine whether the birds had any pre-existing preference toward single-HIPV odors. We also (ii) tested whether birds learn HIPV foraging cues faster than neutral (non-induced) plant odors. Further, we (iii) compared how fast birds could learn olfactory, visual and multimodal (olfactory and visual combined) cues. Finally, we tested (iv) whether learning of one cue would facilitate faster learning of a subsequent cue of the same type, i.e., a second color, odor or multimodal cue. Differences in the abilities of birds to learn different types of sensory cues might indicate a predisposition of the relative importance of these cues during foraging, and may additionally reflect the birds’ prior feeding preferences due to individual experience. Ability to switch preference to new cues might indicate how fast birds respond to, or generalize among, different foraging cues in nature.
We hypothesized that great tits show a preference for, or faster learning of, HIPV cues, either because they are familiar with them from previous foraging experience in their natural environment, or because they have a genetic predisposition for such cues, if their detection has been favored during natural selection. Based on the common assumption that vision is the most important sense in birds, we might expect that visual cues would be learned faster than olfactory cues. In nature, many foraging cues associated with resources might be multimodal, i.e., contain two or more sensory components. For example, HIPVs, leaf damage or other traces of insects might be present on the same plant. Such multimodal cues can substantially increase foraging efficiency in insects, due to faster processing in the brain via multisensory integration (Leonard and Masek, 2014; Balamurali et al., 2020). It is possible that, also in birds, a combination of visual and olfactory cues facilitates faster learning.
Materials and Methods
Birds
We captured 35 adult great tits outside of the breeding season, from November 2019 to February 2020. Using mist-nets, we captured birds at two urban localities in Lund, Sweden (55°42′52′′N, 13°12′26′′E; 55°41′53′′E, 13°14′60′′N) and three rural localities near Höör, about 30 km from Lund (55°55′18′′N, 13°27′11′′E; 55°53′27′′N, 13°36′57′′E; 55°52′34′′N, 13°35′47′′E). The rural localities were characterized by deciduous or mixed forest, interspersed with agricultural fields.
After capture, we transported the birds in cotton bird banding bags to the experimental facility as quickly as possible. The longest such transports lasted approximately 30 min. We housed the birds singly in wire mesh cages (60 × 60 × 40 cm) in an indoor bird facility at the Biology Department at Lund University. We placed the cages in pairs on three shelves in the experimental room. In this way, each bird could have close visual contact with another bird. The shelves with bird cages were separated from the rest of the room by a wall of plywood with doors in front of each cage, which could be either shut or open allowing the birds to view the rest of the room. The lights in the room had an outdoor light spectrum and intensity of 166 Lux (Schneider Electric) and we approximately followed the outdoor day length. In addition, the lights had a 30-min dimming function allowing us to simulate dusk and dawn. We provided the birds with a mixed commercial bird feed (Prestige Parrots, Versele-Laga, Belgium; containing several types of seeds, grains, dried fruits and nuts) ad libitum, dried mealworms, and water with added commercial bird vitamin mixture. They also received living mealworms (Telebrio molitor) daily as part of the experimental training. We allowed the birds to habituate to the housing conditions for 24 h prior to start of the experimental training. We kept no more than six birds at any time in the laboratory, each individual for up to 14 days, which was sufficient to conduct training and experimental sessions. After completion of experiments, we released each bird at the very same locations where it had been captured.
We performed the experiments according to a permit from the Swedish regional ethical permit board for animal experiments (permit number 5.2.18-04716/2018).
Experimental Setup
We conducted all experiments in an experimental room (5 × 3 × 2.6 m) with a foraging arena equipped with four vertically positioned wooden boards (2.5 m high, 4.5 cm deep and 9 cm wide; Supplementary Figure 1). These were positioned vertically on the wall opposite the wall through which the birds could enter the foraging arena through remotely controlled slide shutters (previously described in Brodin and Urhan, 2014). We positioned the boards with a 70 cm distance between them. Along each board, we had drilled five holes with a diameter of 5 mm spaced 30 cm vertically apart from each other. Under each hole, we had mounted a perch from which the birds could access the hole. Thus, the arena had 20 foraging holes in total, in which a food reward could be placed (Supplementary Figure 1). For some of the procedures, we covered the holes with small (1 × 2 cm) laminated pieces of white paper in order to make the contents of the hole invisible for the birds. These covers hang flexibly in front of the hole and could be easily moved aside by the birds. A small slit in the covers made it possible for us to move these up or down so that the holes were either covered or open with the contents visible.
We positioned two additional boards, one on each side of the arena in the corner of the room, to be used for resting/perching, as previous experiments have indicated that birds prefer to perch in the trees on the side of the arena.
A human observer could observe the arena from a small observation room separated from the main laboratory by one way glass. We also positioned a video camera (Sony Action Cam HDR-AS200VT) in the corner of the room to record all experimental sessions.
Visual and Olfactory Cues
As visual cues, we painted an area (1 × 1 cm) of the hole covers using dark blue or bright yellow marker pens (Supplementary Figures 1B,C). We positioned the colored covers in front of the holes that had been baited with mealworm pieces, whereas the covers of non-baited holes remained white. We used two colors in order to avoid potential effects of one specific color on the obtained a learning speed, considering that some colors could be learned faster than others, either because birds differ in their color preference (e.g., Roper and Marples, 1997a; Schmidt and Schaefer, 2004), or perceive some colors as more salient (Shettleworth, 2010). For a single bird, we also used green in addition to blue and yellow, as this individual learned a higher number of cues than other birds (see “switching cue” below). We measured the reflectance spectra of visual cues using a deuterium halogen light source together with a calibrated spectrophotometer (AvaSpec-2048, Avantes, Netherlands) and computer software (AvaSoft 7.0); the reflectance spectra are presented in Supplementary Figure 2.
As olfactory cues, we used two HIPVs, methyl salicylate (MeSA) and methyl jasmonate (MeJA). These are well-known substances produced by a wide range of plant species in response to herbivory or other stressors; MeJA is linked to damage by chewing herbivores like caterpillars and MeSA to sap-sucking herbivores like aphids (Thaler, 1999; Moreira et al., 2018; Kivimäenpää et al., 2020). They are known to attract predatory insects (e.g., James and Price, 2004; Simpson et al., 2011), and appear also to be detectable by insectivorous birds in the field (Mrazova and Sam, 2017; Hiltpold and Shriver, 2018; Rubene et al., 2019). As neutral plant odors, we used lavender (Lavandula angustifolia) and vanilla (Vanilla plantifolia), which have been previously used in olfactory experiments on birds, and seem to be detectable and not aversive (Roper and Marples, 1997b; Mennerat et al., 2005).
We incorporated the odor substances in small white vax pellets (approx. diam 2–3 mm and weight 0.001 g). The odor pellets contained 10% weight of the odor substance—chemical grade 95% MeSA (TCI Europe, Belgium) and MeJA (Sigma-Aldrich, Sweden), pure lavender essential oil (Interlam AB, Sweden) and natural vanilla essence (Interlam AB, Sweden). We prepared the pellets by mixing the odor substances with melted wax, and letting the mixture seep through a specially designed container to form small droplets (procedure details previously described in Ninkovic et al., 2003; Rubene et al., 2019). We packed the pellets inside small bags made of tea filter and attached the bags directly under the baited holes, above the perches (Supplementary Figures 1B,C). We used the non-baited holes as control holes and under each of these we taped visually identical bags with pellets without odor substance. The control pellets were prepared using the same procedure, only without adding the odor substance. Initially, we used five pellets per bag, but after observing a potentially avoiding response in the first group of birds toward MeSA and lavender, we reduced the amount of these two odors to a single pellet per bag. We set up the bags immediately before each experimental session and removed them directly after to minimize odor contamination and the experimental room was allowed to air via ventilation outlets (airflow 15 ls–1, Supplementary Figure 1) for at least 15 min between sessions.
Experimental Procedures
Habituation
First, each bird underwent a habituation phase in order to become comfortable with collecting food (small pieces of mealworm) in the test arena (Supplementary Figure 3A). This phase consisted of 2–6 sessions per bird depending on how quickly the bird learnt this task. During the first training sessions, the holes were uncovered and the mealworm pieces fully visible. We released the birds one at a time from their home cages into the arena by opening the slide shutter. We allowed each bird to explore the arena for up to 20 min and recorded each time it perched or removed a mealworm. Some birds appeared to have low motivation to search for food in the arena, therefore we food-deprived these individuals for 1 h prior to the ensuing experimental sessions. When a bird had visited over 50% of the holes and collected at least five mealworm pieces during one session, we allowed it to continue with the next phase of the training. In these sessions, we placed the covers in front of the holes. In this step, the birds had to learn to retrieve the mealworm from behind the cover. When a bird had visited over 50% of the holes and removed at least five mealworms in this “hole-covered” session, we considered it to have passed the first part of the training.
Initial Preference
In order to assess whether the birds had pre-existing olfactory preferences, we conducted a single-session test where 50% of the holes in test arena were baited with one of the olfactory cues: MeSA, MeJA or lavender (Supplementary Figure 3B). We did not bait any holes with mealworms during this session, and we recorded which holes the birds visited during 10 min. We used the first 10 choices to estimate possible preferences.
Associative Learning
After the initial preference test, we assigned the birds to one of the three test groups: visual, olfactory or multimodal (visual + olfactory). We made sure that all three test groups were represented in each batch of birds that had been captured simultaneously. We then trained the birds to associate food with their respective type of stimulus (colors: dark blue or bright yellow, odors: MeSA, MeJA, lavender, and combinations of colors and odors; see Supplementary Table 1), until they passed a learning criterion—five consecutive correct cue choices in the arena.
In the associative learning phase, we followed a similar procedure as in the first part of the training. In this stage, we released the birds and allowed them to forage in the arena with half of the holes baited and fitted with a foraging cue. We recorded each visit to the perches/holes and successful retrievals of mealworms. Each individual feeding event was counted as a learning trial, i.e., when the bird received a reward by approaching a cue. We terminated a session when a bird had removed more than 50% of the mealworms, or after 20 min. This means that we did not count visits to non-baited holes as learning events. We expressed the learning speed as the number of learning trials (summed over all training sessions) needed to pass the learning criterion.
During the first session in this phase of the training, we baited all holes in two of the trees with mealworms and a learning cue, in order to make the spatial separation of cue/non-cue easier (Supplementary Figure 3C). This was done, because, to our knowledge, the spatial scale of olfactory cue discrimination has so far not been studied in birds and we wanted to make sure that the birds were able to distinguish between odor and non-odor before proceeding. If a bird did not show progress during three subsequent sessions in this phase, it was removed from further study and released. Birds that did not progress would either display stress responses, stay in their home cage or perch in an almost immobile position for most of the time. If a bird was foraging in what appeared to be a relaxed manner and feeding on the mealworms, we proceeded with a semi-randomized placement of the cues in the third phase of the training (Supplementary Figure 3D). Due to the uncertainty about the birds’ ability of spatial odor discrimination, we baited randomized larger areas of 2–3 consecutive holes per tree, rather than just the individual hole (Supplementary Figure 2D). We selected these areas in a stratified semi-random manner, so that 50% of the top two rows (8 holes) and 50% of the bottom three rows (12 holes) were baited with cues. We used a stratified design, to obtain a more spatially balanced distribution with regard to bird preferences, because during the first phase of the training, we had observed that most birds preferred to perch on the top two rows of holes in the arena.
Switching Cue
In nature, different types of cues can be associated with prey at different times of season or in different habitats, e.g., plant species differ in their volatile emission (Effah et al., 2020; Sam et al., 2021). Thus, birds need to be able to switch to new cues frequently to be able to track seasonal changes in prey abundance. We wanted to test whether there were any differences in the ability of the birds to switch to new visual, olfactory and multimodal cues after the birds had associated food with the first cue. In order to test this, we replaced the training cue with a new cue of the same modality (colors: blue and yellow, odors: MeSA, MeJA, vanilla, and combinations of these) after the birds had passed the first associative learning task (Supplementary Figure 3E). Again, the birds were trained in up to 20-min sessions until they made five consecutive correct cue choices.
Analyses
We performed all statistical analyses in R version 3.4 (R Core Team, 2021). We examined the data graphically for meeting the assumptions of parametric statistics, according to Zuur et al. (2010). For all analyses, we used generalized linear mixed models (GLMM) with a Gamma distribution and log link in package lme4 version 1.1-27.1 (Bates et al., 2015).
First, we tested whether the initial odor preference differed between HIPV (MeSA and MeJA) and neutral (lavender) plant odors. As response variable, we used the number of odor choices out of the first 10 choices for each bird. As fixed explanatory factors we used odor (MeSA, MeJA, lavender), sex (female, male), and origin (urban, rural). We included group (birds captured and housed at the same time) as random factor, to control for different birds being tested at different times of the season, but it was later excluded, as it explained zero variation and to solve issues with singular model fit.
As the next step, we tested whether the birds learned olfactory HIPV cues faster than neutral plant cues. Here we included only the data from birds, which were trained with olfactory cues. As response variable, we used the number of trials that a bird required to pass the associative learning task, including the five final correct choices. As explanatory factors, we used odor (MeSA, MeJA, lavender, vanilla) and cue sequence (if the odor was learnt as the first or second cue). As random factors, we included individual, since each bird was tested with two cues, and group (birds captured and housed at the same time). Group was again subsequently removed from the model.
Finally, we tested differences in learning speed between olfactory, visual and multimodal cues. As response variable, we then used number of trials to pass for each bird. As fixed explanatory factors, we used cue type (olfactory, visual and multimodal), sex, origin and cue sequence. Individual and group were used as random factors. To this main-effects model, we added two-way interactions between sex, cue type, origin and cue sequence, tested one at a time. Interaction terms with p ≥ 0.1 were removed from the model, and group was also removed, as above.
We simplified the models by removing terms with p ≥ 0.1, and validated model fit by examining residual plots. Removing variables did not change the significance of the remaining variables. We assessed pairwise differences between explanatory variable categories by altering the reference category and rerunning the model. We also calculated “goodness of fit” as marginal (fixed effects only) and conditional (fixed and random effects) pseudo-R2 for GLMM, using MuMIn package version 1.43.17 (Kamil, 2020). We present the final model output and R2GLMM values in Table 1.
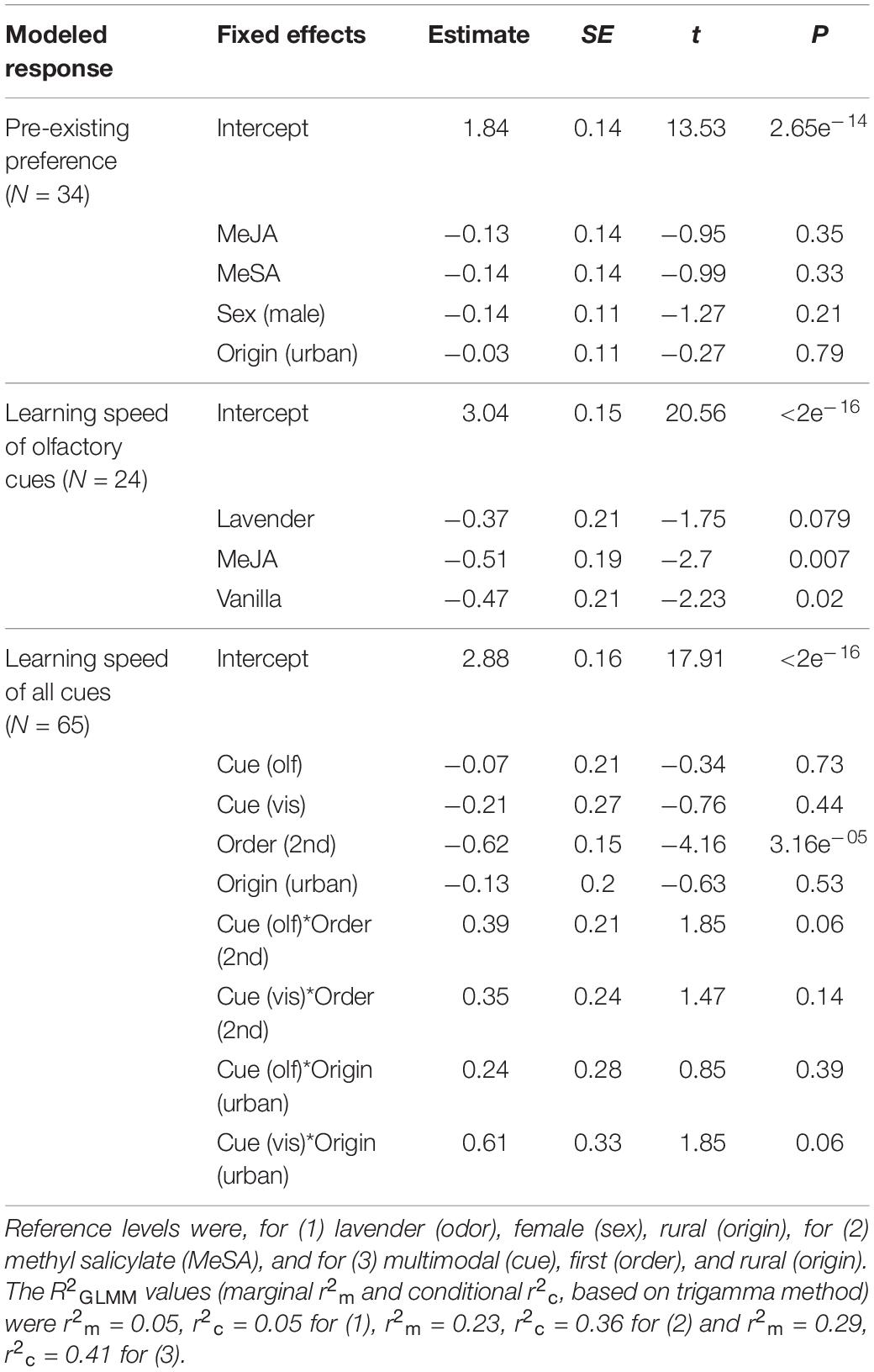
Table 1. Results of generalized linear mixed models (GLMM) analyses showing fixed effects of models for (1) pre-existing odor preference, (2) learning speed of olfactory herbivore-induced plant volatile (HIPV) and neutral cues, and (3) learning speed of olfactory, visual, and multimodal cues.
Results
Out of the 35 birds captured, 31 successfully completed all learning tasks. Four birds failed and we released these at the start of associative learning stage (1 bird trained with blue, 1 with MeSA and 2 with lavender). The birds, which failed to learn did not show any increased stress responses during the first stage of the training, and the average habituation time (number of sessions) for “failed” birds (mean ± SD: 3 ± 2) was similar to that of “successful birds”(mean ± SD: 3.26 ± 1.4).
The birds required between 5 and 37 foraging/learning trials in order to pass the associative learning task, including the five consecutive correct choices. One male individual demonstrated unusually high learning ability, as it passed the learning criterion in the first training session for both the first and the second cue. Therefore, we tested this bird with additional cues; in total two visual, two olfactory and one multimodal cue, and obtained learning speeds of 8 (blue = first cue), 5 (yellow), 11 (lavender), 13 (MeSA), and 11 (MeJA + green) trials.
We found no evidence that great tits had any pre-existing preference for any of the odor cues tested (Table 1; mean preference ± SD for MeSA = 0.52 ± 0.19, MeJA = 0.5 ± 0.15 and lavender = 0.57 ± 0.15). The associative learning test showed that, among olfactory cues, the birds learned MeSA significantly slower compared to MeJA and vanilla odors, and possibly also lavender (Figure 1 and Table 1).
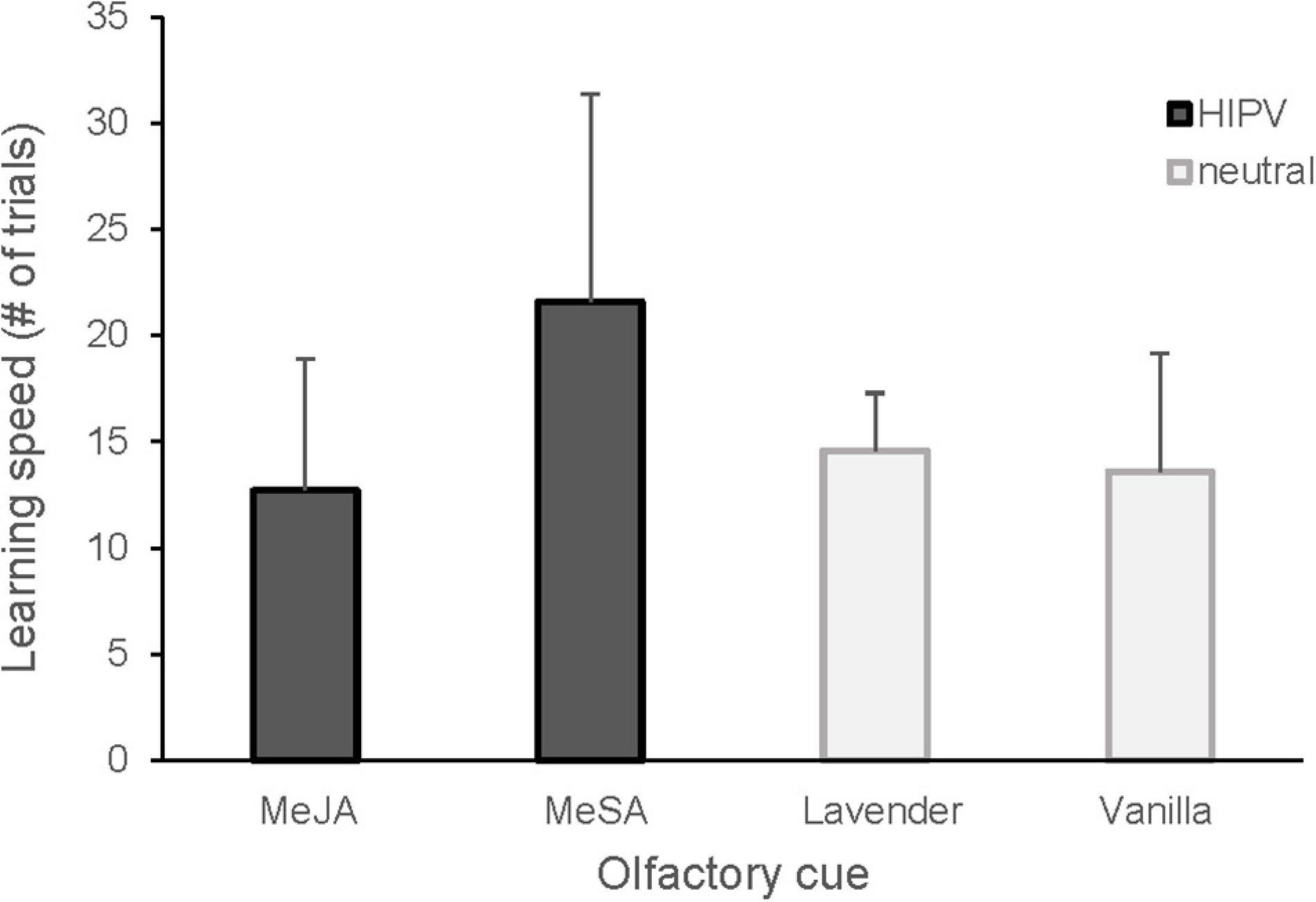
Figure 1. Learning speed in great tits presented with HIPV (MeSA, MeJA) and neutral (lavender, vanilla) olfactory cues. Mean number of trials and standard deviation are shown. Twelve great tits each (4 females and 8 males) learned two cues. The learning speed was significantly higher for MeSA compared to MeJA (GLMM, p = 0.007) and vanilla (GLMM, p = 0.02), with a similar tendency for lavender (p = 0.079).
When comparing all cue types, there were no differences in learning speed between olfactory, visual and multimodal foraging cues, but the second cue was learnt significantly faster than the first cue (Figure 2 and Table 1). A tendency for an interaction between cue type and order suggested that the birds learned the second multimodal cue faster than the second olfactory cue (Table 1). There was also a tendency for an interaction between cue type and bird origin, indicating that urban birds may learn visual cues slower than rural birds (Figure 3 and Table 1).
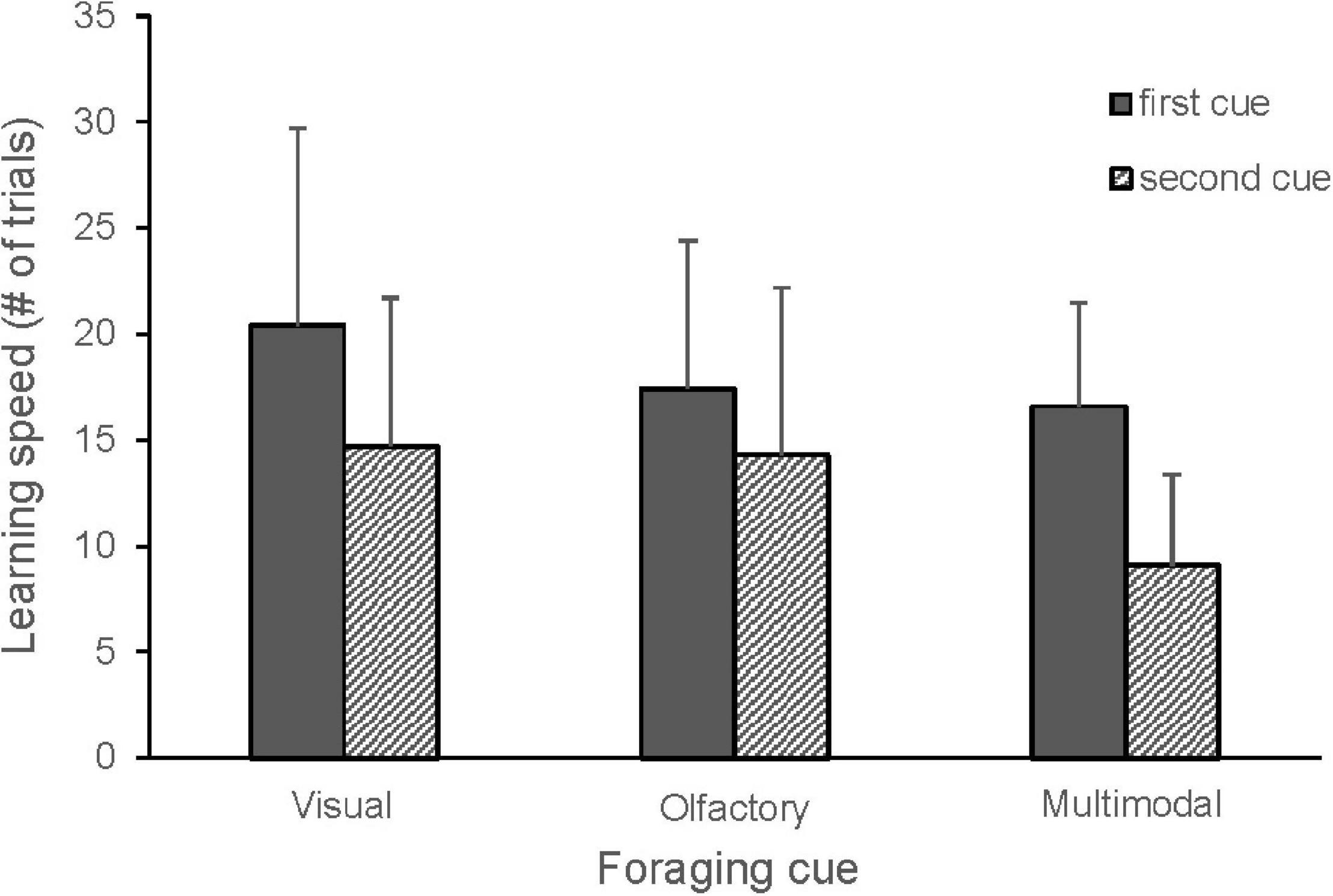
Figure 2. Learning speed in great tits for the first and the second visual (n = 16), olfactory (n = 24) and multimodal (n = 24) foraging cues. Mean number of trials and standard deviation are shown. The cues tested: visual (blue, yellow), olfactory (MeSA, MeJA, vanilla), multimodal (blue + MeSA, blue + MeJA, blue + vanilla, yellow + MeSA, yellow + MeJA, yellow + vanilla, green + MeJA). Thirty birds each learned two cues and a single bird learned five cues. Learning speed for the second cue was significantly lower (GLMM, p < 0.0001), with an additional tendency for lower learning speed of second multimodal compared to olfactory cues (GLMM, p = 0.06).
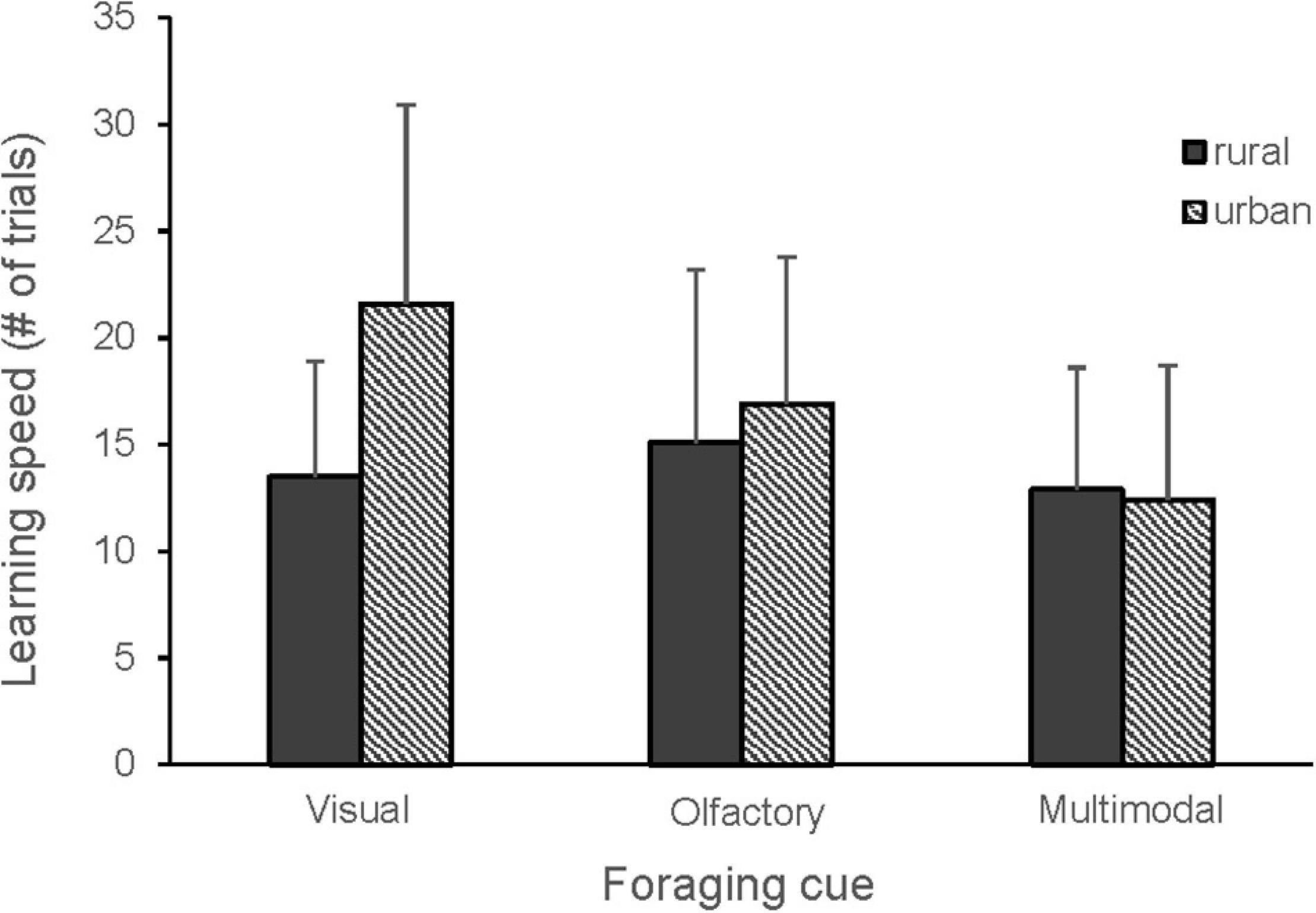
Figure 3. Learning speed in urban (n = 15) and rural (n = 17) great tits for visual, olfactory and multimodal foraging cues. Mean number of trials and standard deviation are shown. The cues tested: visual (blue, yellow), olfactory (MeSA, MeJA, vanilla), multimodal (blue + MeSA, blue + MeJA, blue + vanilla, yellow + MeSA, yellow + MeJA, yellow + vanilla, green + MeJA). Thirty birds learned two cues each and a single bird learned five cues. There was a tendency for higher learning speed of visual cues in urban birds (GLMM, p = 0.06).
Discussion
We found that great tits did not exhibit any initial preference for HIPV odors, and they did not learn HIPVs faster than neutral plant odors. The neutral plant odors used in this study are typically not associated with herbivorous insect prey. We also found no differences in learning speed between cue types, suggesting that the birds learned to associate odors, colors, and multimodal foraging cues with food equally well. Additionally, after learning one cue, they required a significantly shorter learning time to switch to a new cue, with a tendency for particularly faster switching for multimodal cues. Our results also suggest that visual learning might be slower in birds of urban origin.
Since birds are considered primarily to be visual foragers, it is perhaps surprising that great tits have similar learning abilities for olfactory and visual cues. Mäntylä et al. (2020) showed that great tits and blue tits (Cyanistes caeruleus), a close relative to the great tit, could identify pine branches releasing HIPVs by either visual or olfactory cues, whereas great tits in the study by Amo et al. (2013) located trees exposed to herbivory successfully by olfaction alone, but not by vision alone. For insectivorous birds specifically, ability to detect plant semiochemical cues might be highly adaptive, especially if they allow birds to localize prey more efficiently during the energetically demanding nestling provisioning periods. Such ability could even make it possible for the birds to predict the future level of prey abundance during the nesting period. Plant volatile emissions have been reported to change in response to eggs laid by herbivores (Hilker and Meiners, 2006), and these changes are detectable by birds (Mäntylä et al., 2018). Potentially, such changes in plant odor emission might inform birds about herbivore presence before any visual signs of larvae are detectable. A recent study on blue tits supports the possibility that plant chemical cues might contribute to bird timing decisions during breeding (Graham et al., 2021). How these processes play out in wild bird populations should be addressed by future research.
Great tits were able to learn a new cue considerably faster after learning the first cue, and almost half of the individuals passed the learning criterion already in the first training session after we introduced the new cue. This suggests that they could generalize the learning task to some extent, meaning that the birds learned the contingency between olfaction and reward and applied the same rule in consecutive tests, regardless of the type of the olfactory stimulus present. Sam et al. (2021) trained great tits to discriminate between induced and non-induced odors of one tree species and then tested bird preference with a second tree species that they had not previously encountered. The authors found that the birds were not able to generalize odors in this task. It is possible that generalizing an association to two new odors is more difficult than generalizing the rule of “presence of an odor signals presence of a reward.” In addition, the measure of preference, which was used in that study (Sam et al., 2021), i.e., time spent on a plant in a single test session, might have some limitation in that some important aspects of the learning/switching process are not captured. It is possible, for example, that the learning time was not sufficient for all individuals. Birds show considerable individual variation in time needed to learn a task with a subsequent acquisition of the correct preference, which may be related to both learning speed (Bolhuis et al., 2000) and how fast they tend to explore the available options (Amy et al., 2012). It is hence possible that birds that appear to be poor learners would be better characterized as “slow learners” (Bolhuis et al., 2000) and some birds may need longer time to generalize the sensory information. As we used a more standardized measure of learning (number of foraging events), all birds had a chance to achieve the learning criterion, irrespective of how many quick or slow learners there were.
Our results suggest that great tits have no genetic predisposition for any of the HIPVs used in this study. We expected that there could have been an initial preference or a more rapid learning of these cues, even if the birds have no innate preference (Amo et al., 2016; Sam et al., 2021). The reason is that adult birds most likely would have bred in the wild at least once before participating in the study. As great tits primarily feed their young with herbivorous insect larvae from deciduous trees, they should have encountered HIPVs before. Other studies have observed a preference for plants emitting HIPVs in wild-caught birds (e.g., Mäntylä et al., 2020). The differences in bird response between our study and the study by Mäntylä et al. (2020) suggest that birds might respond to complex volatile blends rather than single compounds. Birds studied by Mäntylä et al. (2020) were tested using live pine tree branches, which were exposed to larval herbivory prior to the test, and were therefore emitting a volatile blend similar to what birds may have encountered in nature.
Preferences for single HIPVs, e.g., MeJA (Mrazova and Sam, 2017) and MeSA (Hiltpold and Shriver, 2018; Rubene et al., 2019) have, however, been reported in some field studies. Even in these cases, birds might have responded to more complex volatile blends produced by the surrounding vegetation rather than the specific compounds of the experimental treatments. This is because when HIPVs are applied to (or near) plants, the plants respond by altering their own volatile emissions, via so-called priming, which helps undamaged plants to prepare for a herbivore attack (Kessler and Baldwin, 2001; Heil and Silva Bueno, 2007). Additionally, some insects respond only to HIPVs when these are present in specific proportions together with other compounds, or respond only to individual compounds if these are part of a more complex blend (e.g., Bruce and Pickett, 2011; Webster, 2012; Clavijo Mccormick et al., 2014). This may also be true for insectivorous birds, and lack of preference for HIPVs in our experiment could be because the birds did not recognize MeSA and MeJA in isolation from the rest of the natural odor blend. Alternatively, the odors in our experiment may no longer have contributed to the “search image” of birds outside of the breeding season, when temperatures are low, trees lose their foliage and herbivorous insects are difficult to find. Seasonal variation in olfactory function and preference for aromatic nesting material has been reported in male European starlings (Sturnus vulgaris) (de Groof et al., 2010), and a correlation between a reproductive trait (testosterone level) and olfactory response has also been observed in male great tits (Graham et al., 2021). In summary, it seems plausible that insectivorous birds have no predisposition (e.g., higher sensitivity) for widely abundant single HIPVs, or that the birds retain memory of (and preference for) ecologically relevant odors only for as long as they are directly beneficial.
Birds learned MeSA more slowly than other odors, possibly because it is produced in response to aphid feeding and aphids—due to their small size—are not profitable prey for breeding birds (e.g., Smith et al., 2009). Yet, if the learning speed was related to potential ecological relevance of the odors, we would expect significantly faster learning of MeJA, as it can be linked to larval herbivory. This was not the case, as the neutral odors were learnt at a similar pace. Both lavender and vanilla odors consist of many chemical compounds, so they represent complex volatile blends. The fact that we did not observe any general patterns in learning of HIPV/neutral or single/complex odors, suggests that there might not be an ecological explanation for slower learning of MeSA. Rather, this effect is probably attributable to an aversive response, as MeSA odor can be perceived as very intense by humans, and this might also be true for birds (we reduced the dose of MeSA early in the study). Even attractive odors can become aversive at high doses (Semmelhack and Wang, 2009), whereas doses that are too low are at risk of not being detected. Data are lacking on detection thresholds and relative perceived strength of biologically relevant odors in birds; therefore, we cannot be certain that all our tested odors had optimal doses. Nevertheless, great tits were able to learn all four odors, which indicates that their dosage was within a detectable range in relation to the spatial scale used in the study. Yet, in order to compare quantitatively bird responses to different odors, a range of doses for each odor should ideally be tested.
We found that the birds did not learn a combination of visual and olfactory cue (multimodal cue) faster, compared to either visual or olfactory cues on their own. In insects, multimodal cues (particularly color and odor combinations) facilitate more rapid learning and location of food sources (Leonard and Masek, 2014; Balamurali et al., 2020). Our results suggest that such a connection between visual and olfactory foraging cues is lacking in birds, perhaps because birds are more cognitively advanced and rely on learning, while insect behavior is to a larger extent driven by genetic predispositions and innate responses (Webster, 2012). Multimodal cues might be challenging to learn, because the great tits first need to learn the relationship between these cues (two cues together have one function), and then they have to learn reward contingency. Nevertheless, the birds in our experiment showed some tendency to switch faster to a second multimodal cue, which indicates that they were able to generalize the information, potentially through multi-sensory integration. The possibility that birds are able to use multisensory integration deserves a further study on mechanisms and functions of such ability.
We also found that urban birds learned visual cues somewhat slower compared to rural birds. These findings are not consistent with those of previous studies, where urban and rural birds performed similarly in visual associative learning tasks. Our findings are also surprising, because visual information has great importance for both rural and urban birds. Although we did not test for personality traits, most studies agree that rural birds and urban birds differ in their personality, where urban great tits are faster explorers, bolder and more alert compared to rural great tits (e.g., Charmantier et al., 2017; Riyahi et al., 2017). Therefore, urban great tits may tend to explore more options rather than solve tasks, which can give misleading results in standardized learning experiments such as ours, where increasing number of learning trials lowers the performance score. Why personality differences might not affect bird performance in the olfactory task is uncertain, as research on the use of olfaction and personality is lacking. Further studies should explore in more detail the potential differences in sensory abilities or preferences in relation to personality between birds of urban and rural origin.
Overall, our results show that great tits are able to quickly detect and learn olfactory and visual sensory cues to obtain food. Our findings support prior reports that great tits are innovative and apt learners in foraging contexts (Estók et al., 2010; Cole et al., 2011; Aplin et al., 2014; Brodin and Urhan, 2014). In addition, our findings suggest that the cognitive abilities of great tits pertain to visual, olfactory and multimodal foraging cues. Future research should aim at clarifying the roles that HIPVs as single compounds or in complex blends play in guiding foraging behavior of great tits and other insectivorous birds. Finally, a better understanding of when and how a preference for HIPV releasing plants is developed by breeding birds in nature, could contribute to unveiling the ecological and evolutionary significance of this preference.
Data Availability Statement
The original contributions presented in the study are included in the article/Supplementary Material, further inquiries can be directed to the corresponding author/s.
Ethics Statement
The animal study was reviewed and approved by the Malmö-Lunds Ethical Committee (permit number 5.2.18-04716/2018).
Author Contributions
DR conceived the idea and developed the framework of the study together with AB and VN. DR and UU designed the experimental setup and procedures. DR carried out behavioral observations and analyzed the data. DR wrote the manuscript with contributions from AB, UU, and VN. All authors approved the submitted version.
Funding
This research was funded by the Swedish Research council FORMAS to DR (grant no. 2017-01551) and the Carl Tryggers foundation to AB (grant no. 18-71).
Conflict of Interest
The authors declare that the research was conducted in the absence of any commercial or financial relationships that could be construed as a potential conflict of interest.
Publisher’s Note
All claims expressed in this article are solely those of the authors and do not necessarily represent those of their affiliated organizations, or those of the publisher, the editors and the reviewers. Any product that may be evaluated in this article, or claim that may be made by its manufacturer, is not guaranteed or endorsed by the publisher.
Acknowledgments
We would like to thank Dimitrije Markovic for assistance with production of odor pellets, Camilla Björklöv for lab supervision and Olle Håstad for help with spectrophotometer measurements.
Supplementary Material
The Supplementary Material for this article can be found online at: https://www.frontiersin.org/articles/10.3389/fevo.2021.800057/full#supplementary-material
References
Amo, L., Dicke, M., and Visser, M. E. (2016). Are naïve birds attracted to herbivore-induced plant defences? Behaviour 153, 353–366. doi: 10.1163/1568539X-00003345
Amo, L., Jansen, J. J., van Dam, N. M., Dicke, M., and Visser, M. E. (2013). Birds exploit herbivore-induced plant volatiles to locate herbivorous prey. Ecol. Lett. 16, 1348–1355. doi: 10.1111/ele.12177
Amy, M., van Oers, K., and Naguib, M. (2012). Worms under cover: relationships between performance in learning tasks and personality in great tits (Parus major). Anim. Cogn. 15, 763–770. doi: 10.1007/s10071-012-0500-3
Aplin, L. M., Farine, D. R., Mann, R. P., and Sheldon, B. C. (2014). Individual-level personality influences social foraging and collective behaviour in wild birds. Proc. R. Soc. B Biol. Sci. 281:20141016 doi: 10.1098/rspb.2014.1016
Balamurali, G. S., Rose, S., Somanathan, H., and Kodandaramaiah, U. (2020). Complex multi-modal sensory integration and context specificity in colour preferences of a pierid butterfly. J. Exp. Biol. 223:jeb223271. doi: 10.1242/jeb.223271
Bates, D., Mächler, M., Bolker, B. M., and Walker, S. C. (2015). Fitting linear mixed-effects models using lme4. J. Stat. Softw. 67, 1–48. doi: 10.18637/jss.v067.i01
Bolhuis, J. J., Cook, S., and Horn, G. (2000). Getting better all the time: improving preference scores reflect increases in the strength of filial imprinting. Anim. Behav. 59, 1153–1159. doi: 10.1006/anbe.2000.1413
Boström, J. E., Dimitrova, M., Canton, C., Håstad, O., Qvarnström, A., and Ödeen, A. (2016). Ultra-rapid vision in birds. PLoS One 11:e0151099. doi: 10.1371/journal.pone.0151099
Brodin, A., and Urhan, A. U. (2014). Interspecific observational memory in a non-caching Parus species, the great tit Parus major. Behav. Ecol. Sociobiol. 68, 649–656. doi: 10.1007/s00265-013-1679-2
Brodin, A., and Urhan, A. U. (2015). Sex differences in learning ability in a common songbird, the great tit—females are better observational learners than males. Behav. Ecol. Sociobiol. 69, 237–241. doi: 10.1007/s00265-014-1836-2
Bruce, T. J. A., and Pickett, J. A. (2011). Perception of plant volatile blends by herbivorous insects–finding the right mix. Phytochemistry 72, 1605–1611. doi: 10.1016/j.phytochem.2011.04.011
Charmantier, A., Demeyrier, V., Lambrechts, M., Perret, S., and Grégoire, A. (2017). Urbanization is associated with divergence in pace-of-life in great tits. Front. Ecol. Evol. 5:53. doi: 10.3389/fevo.2017.00053
Clavijo Mccormick, A., Gershenzon, J., and Unsicker, S. B. (2014). Little peaks with big effects: establishing the role of minor plant volatiles in plant-insect interactions. Plant Cell Environ. 37, 1836–1844. doi: 10.1111/pce.12357
Cole, E. F., Cram, D. L., and Quinn, J. L. (2011). Individual variation in spontaneous problem-solving performance among wild great tits. Anim. Behav. 81, 491–498. doi: 10.1016/j.anbehav.2010.11.025
Danel, S., Chiffard-Carricaburu, J., Bonadonna, F., and Nesterova, A. P. (2021). Exclusion in the field: wild brown skuas find hidden food in the absence of visual information. Anim. Cogn. 24, 867–876. doi: 10.1007/s10071-021-01486-4
de Groof, G., Gwinner, H., Steiger, S., Kempenaers, B., and van der Linden, A. (2010). Neural correlates of behavioural olfactory sensitivity changes seasonally in European starlings. PLoS One 5:e14337. doi: 10.1371/journal.pone.0014337
Dicke, M. (2015). Herbivore-induced plant volatiles as a rich source of information for arthropod predators: fundamental and applied aspects. J. Indian Inst. Sci. 95, 35–42.
Dicke, M., Van Poecke, R. M. P., and De Boer, J. G. (2003). Inducible indirect defence of plants: from mechanisms to ecological functions. Basic Appl. Ecol. 4, 27–42. doi: 10.1078/1439-1791-00131
Donner, K. (2021). Temporal vision: measures, mechanisms and meaning. J. Exp. Biol 224:jeb222679. doi: 10.1242/jeb.222679
Effah, E., Barrett, D. P., Peterson, P. G., Potter, M. A., Holopainen, J. K., and Clavijo McCormick, A. (2020). Seasonal and environmental variation in volatile emissions of the New Zealand native plant Leptospermum scoparium in weed-invaded and non-invaded sites. Sci. Rep. 10:11736. doi: 10.1038/s41598-020-68386-4
Estók, P., Zsebok, S., and Siemers, B. M. (2010). Great tits search for, capture, kill and eat hibernating bats. Biol. Lett. 6, 59–62. doi: 10.1098/rsbl.2009.0611
Graham, J. L., Charlier, T. D., Bonadonna, F., and Caro, S. P. (2021). Olfactory detection of trace amounts of plant volatiles is correlated with testosterone in a passerine bird. Horm. Behav. 136:105045. doi: 10.1016/j.yhbeh.2021.105045
Heil, M., and Silva Bueno, J. C. (2007). Within-plant signaling by volatiles leads to induction and priming of an indirect plant defense in nature. Proc. Natl. Acad. Sci. U.S.A. 104, 5467–5472. doi: 10.1073/pnas.0610266104
Hilker, M., and Meiners, T. (2006). Early herbivore alert: insect eggs induce plant defense. J. Chem. Ecol. 32, 1379–1397. doi: 10.1007/s10886-006-9057-4
Hiltpold, I., and Shriver, W. G. (2018). Birds bug on indirect plant defenses to locate insect prey. J. Chem. Ecol. 44, 576–579. doi: 10.1007/s10886-018-0962-0
Isaksson, E., Utku Urhan, A., and Brodin, A. (2018). High level of self-control ability in a small Passerine bird. Behav. Ecol. Sociobiol. 72:118. doi: 10.1007/s00265-018-2529-z
James, D. G., and Price, T. S. (2004). Field-testing of methyl salicylate for recruitment and retention of beneficial insects in grapes and hops. J. Chem. Ecol. 30, 1613–1628. doi: 10.1023/B:JOEC.0000042072.18151.6f
Kamil, B. (2020). MuMIn: Multi-Model Inference. Available online at: https://cran.r-project.org/web/packages/MuMIn/. (accessed August 6, 2021).
Kelber, A. (2019). Bird colour vision–from cones to perception. Curr. Opin. Behav. Sci. 30, 34–40. doi: 10.1016/j.cobeha.2019.05.003
Kessler, A., and Baldwin, I. T. (2001). Defensive function of herbivore-induced plant volatile emissions in nature. Science 291, 2141–2144. doi: 10.1126/science.291.5511.2141
Kivimäenpää, M., Babalola, A. B., Joutsensaari, J., and Holopainen, J. K. (2020). Methyl salicylate and sesquiterpene emissions are indicative for aphid infestation on Scots pine. Forests 11, 1–15. doi: 10.3390/F11050573
Leonard, A. S., and Masek, P. (2014). Multisensory integration of colors and scents: insights from bees and flowers. J. Comp. Physiol. A Neuroethol. Sens. Neural Behav. Physiol. 200, 463–474. doi: 10.1007/s00359-014-0904-4
Mäntylä, E., Kipper, S., and Hilker, M. (2020). Insectivorous birds can see and smell systemically herbivore-induced pines. Ecol. Evol. 10, 9358–9370. doi: 10.1002/ece3.6622
Mäntylä, E., Kleier, S., Kipper, S., and Hilker, M. (2017). The attraction of insectivorous tit species to herbivore-damaged Scots pines. J. Ornithol. 158, 479–491. doi: 10.1007/s10336-016-1412-9
Mäntylä, E., Kleier, S., Lindstedt, C., Kipper, S., and Hilker, M. (2018). Insectivorous birds are attracted by plant traits induced by insect egg deposition. J. Chem. Ecol. 44, 1127–1138. doi: 10.1007/s10886-018-1034-1
Mäntylä, E., Klemola, T., and Haukioja, E. (2004). Attraction of willow warblers to sawfly-damaged mountain birches: novel function of inducible plant defences? Ecol. Lett. 7, 915–918. doi: 10.1111/j.1461-0248.2004.00653.x
Martin, G. R. (2012). Through birds’ eyes: insights into avian sensory ecology. J. Ornithol. 153, 23–48. doi: 10.1007/s10336-011-0771-5
Mennerat, A., Bonadonna, F., Perret, P., and Lambrechts, M. M. (2005). Olfactory conditioning experiments in a food-searching passerine bird in semi-natural conditions. Behav. Process. 70, 264–270. doi: 10.1016/j.beproc.2005.07.005
Morand-Ferron, J., Hamblin, S., Cole, E. F., Aplin, L. M., and Quinn, J. L. (2015). Taking the operant paradigm into the field: associative learning in wild great tits. PLoS One 10:e0133821. doi: 10.1371/journal.pone.0133821
Moreira, X., Nell, C. S., Katsanis, A., Rasmann, S., and Mooney, K. A. (2018). Herbivore specificity and the chemical basis of plant–plant communication in Baccharis salicifolia (Asteraceae). New Phytol. 220, 703–713. doi: 10.1111/nph.14164
Mrazova, A., and Sam, K. (2017). Application of methyl jasmonate to grey willow (Salix cinerea) attracts insectivorous birds in nature. Arthropod. Plant. Interact. 12, 1–8. doi: 10.1007/s11829-017-9558-9
Murakami, M. (2002). Foraging mode shifts of four insectivorous bird species under temporally varying resource distribution in a Japanese deciduous forest. Ornithol. Sci. 1, 63–69. doi: 10.2326/osj.1.63
Ninkovic, V., Ahmed, E., Glinwood, R., and Pettersson, J. (2003). Effects of two types of semiochemical on population development of the bird cherry oat aphid Rhopalosiphum padi in a barley crop. Agric. For. Entomol. 5, 27–33. doi: 10.1046/j.1461-9563.2003.00159.x
Outomuro, D., Urhan, A. U., Brodin, A., and Johansson, F. (2020). Preference for supernormal stimuli tends to override initially learned associations for conspicuous prey traits: implications from a laboratory study. J. Ethol. 38, 365–371. doi: 10.1007/s10164-020-00661-3
Potier, S. (2020). Olfaction in raptors. Zool. J. Linn. Soc. 189, 713–721. doi: 10.1093/zoolinnean/zlz121
R Core Team (2021). R: A Language and Environment for Statistical Computing. Available online at: https://www.r-project.org/ (accessed July 28, 2021).
Riyahi, S., Björklund, M., Mateos-Gonzalez, F., and Senar, J. C. (2017). Personality and urbanization: behavioural traits and DRD4 SNP830 polymorphisms in great tits in Barcelona city. J. Ethol. 35, 101–108. doi: 10.1007/s10164-016-0496-2
Roper, T. J., and Marples, N. M. (1997a). Colour preferences of domestic chicks in relation to food and water presentation. Appl. Anim. Behav. Sci. 54, 207–213. doi: 10.1016/S0168-1591(96)01178-1
Roper, T.J., and Marples, N. M. (1997b). Odour and colour as cues for taste-avoidance learning in domestic chicks. Anim. Behav. 53, 1241–1250. doi: 10.1006/anbe.1996.0384
Rubene, D., Leidefors, M., Ninkovic, V., Eggers, S., and Low, M. (2019). Disentangling olfactory and visual information used by field foraging birds. Ecol. Evol. 9, 545–552. doi: 10.1002/ece3.4773
Sam, K., Kovarova, E., Freiberga, I., Uthe, H., Weinhold, A., Jorge, L. R., et al. (2021). Great tits (Parus major) flexibly learn that herbivore-induced plant volatiles indicate prey location: an experimental evidence with two tree species. Ecol. Evol. 11, 10917–10925. doi: 10.1002/ece3.7869
Schmidt, V., and Schaefer, H. M. (2004). Unlearned preference for red may facilitate recognition of palatable food in young omnivorous birds. Evol. Ecol. Res. 6, 919–925.
Semmelhack, J. L., and Wang, J. W. (2009). Select Drosophila glomeruli mediate innate olfactory attraction and aversion. Nature 459, 218–223. doi: 10.1038/nature07983
Shettleworth, S. J. (2010). Cognition, Evolution, and Behavior, 2nd Edn. New York, NY: Oxford Unversity Press.
Shiao, M. T., Chuang, M. C., Wu, S., Yuan, H. W., and Wang, Y. (2019). Differential larval phenology affects nestling condition of Green-backed Tit (Parus monticolus) in broadleaf and coniferous habitats, subtropical Taiwan. J. Ornithol. 160, 1003–1014. doi: 10.1007/s10336-019-01676-5
Simpson, M., Gurr, G. M., Simmons, A. T., Wratten, S. D., James, D. G., Leeson, G., et al. (2011). Insect attraction to synthetic herbivore-induced plant volatile-treated field crops. Agric. For. Entomol. 13, 45–57. doi: 10.1111/j.1461-9563.2010.00496.x
Smith, B., Holland, J., Jones, N., Moreby, S., Morris, A. J., and Southway, S. (2009). Enhancing invertebrate food resources for skylarks in cereal ecosystems: how useful are in-crop agri-environment scheme management options? J. Appl. Ecol. 46, 692–702. doi: 10.1111/j.1365-2664.2009.01638.x
Thaler, J. S. (1999). Jasmonate-inducible plant defences cause increased parasitism of herbivores. Nature 399, 686–688. doi: 10.1038/21420
Tremblay, I., Thomas, D. W., Lambrechts, M. M., Blondel, J., and Perret, P. (2003). Variation in Blue Tit breeding performance across gradients in habitat richness. Ecology 84, 3033–3043. doi: 10.1890/02-0663
Visser, M. E., Holleman, L. J. M., and Gienapp, P. (2006). Shifts in caterpillar biomass phenology due to climate change and its impact on the breeding biology of an insectivorous bird. Oecologia 147, 164–172. doi: 10.1007/s00442-005-0299-6
Webster, B. (2012). The role of olfaction in aphid host location. Physiol. Entomol. 37, 10–18. doi: 10.1111/j.1365-3032.2011.00791.x
Keywords: bird senses, olfactory learning, visual learning, HIPV, bird olfaction, great tit, foraging cues
Citation: Rubene D, Urhan U, Ninkovic V and Brodin A (2022) Great Tits Learn Odors and Colors Equally Well, and Show No Predisposition for Herbivore-Induced Plant Volatiles. Front. Ecol. Evol. 9:800057. doi: 10.3389/fevo.2021.800057
Received: 22 October 2021; Accepted: 23 December 2021;
Published: 13 January 2022.
Edited by:
Magdalena Ruiz Rodriguez, University of Granada, SpainReviewed by:
Gerhard Johannes Gries, Simon Fraser University, CanadaJessica Graham, North Dakota State University, United States
Copyright © 2022 Rubene, Urhan, Ninkovic and Brodin. This is an open-access article distributed under the terms of the Creative Commons Attribution License (CC BY). The use, distribution or reproduction in other forums is permitted, provided the original author(s) and the copyright owner(s) are credited and that the original publication in this journal is cited, in accordance with accepted academic practice. No use, distribution or reproduction is permitted which does not comply with these terms.
*Correspondence: Diana Rubene, diana.rubene@slu.se