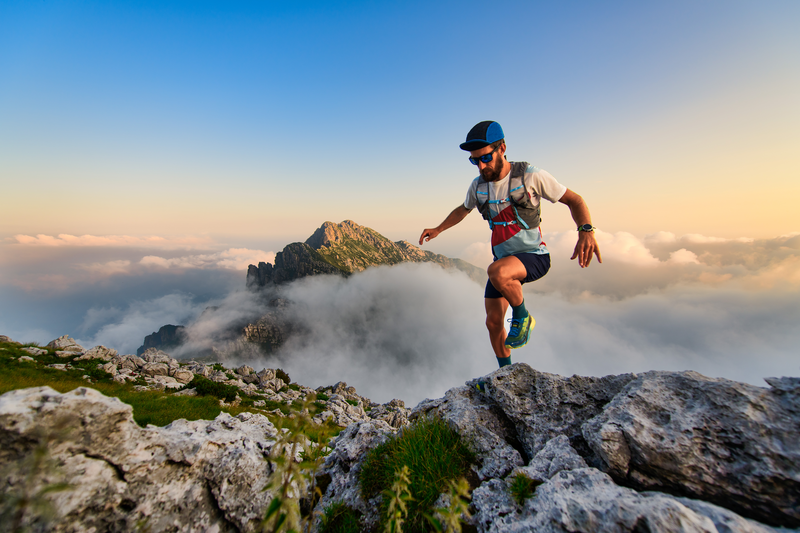
95% of researchers rate our articles as excellent or good
Learn more about the work of our research integrity team to safeguard the quality of each article we publish.
Find out more
ORIGINAL RESEARCH article
Front. Ecol. Evol. , 07 January 2022
Sec. Behavioral and Evolutionary Ecology
Volume 9 - 2021 | https://doi.org/10.3389/fevo.2021.799996
This article is part of the Research Topic The Development and Fitness Consequences of Sex Roles View all 11 articles
Parental care plays a central, reinforcing role in the evolution of sex roles and its development is often reported to be driven by genetic, rather than environmental effects. Based on these studies, however, genetic inheritance does not account fully for the often-significant phenotypic variability observed within species, a variation that we hypothesized may be explained by social effects from parents. Following a full cross-fostering design, here we aimed at disentangling genetic and social parental effects in the ontogeny of parental behaviours. Clutches of eggs were swapped, and we monitored parental behaviours in two consecutive generations of a captive population of the socially monogamous, biparental zebra finch (Taeniopygia guttata). Using nest box cameras, parental behaviour was recorded for 3 h in two reproductive stages: on day 8 of incubation and day 10 post-hatching. These fostered birds, after becoming fully matured, received a pair randomly and we observed parental care of this second generation too, following the same protocol. We then compared various parental behaviours (such as time spent incubating, or number of nest attendances during offspring provisioning) in the second generation to those of their genetic and social parents. Based on the results of our experiment, both genetic and social effects can contribute to intergenerational transmission of specific parental behaviours, with various weights. However, the strongest and most consistent effect that we found is that of the current mate; a social effect that can manifest both in negative and positive directions, depending on the behavioural trait. Our study suggests context-specific and sexually different genetic, social and non-social environmental effects in the ontogeny of parental sex roles and outline the importance of parental negotiation in explaining individual variation of parental behaviour in biparental species.
Males and females often differ in various aspects of their reproductive behaviours, for instance, in their competitiveness and choosiness during mating and their parental behaviour, so that they exhibit distinctive sex roles (Kokko et al., 2006; Fairbairn, 2013). Although the traditional definition of sex roles focuses on the competition aspect only, the frequent association with biased parental care and the reinforcing, positive interaction between them justifies a wider definition including parental sex roles (Kokko and Jennions, 2008). Parental sex role differences in terms of workload may manifest in one parent providing full care and the other providing no care at all (such as in uniparental systems), or it may manifest in unequal relative amount of care provided by the two sexes in biparental systems. Explaining the origin and consequences of parental sex role differences are central questions in evolutionary biology and behavioural ecology, due to their direct and significant impact on individual fitness and ultimately, their contribution to breeding system evolution (Clutton-Brock, 1991; Kokko and Jennions, 2008; Royle et al., 2012; Kahn et al., 2013; McNamara and Wolf, 2015; Fromhage et al., 2016; Fromhage and Jennions, 2016; Henshaw et al., 2019).
Sex-roles involve social behaviours, and as such, understanding how they change, develop, and evolve in non-human animals by genetic evolution, social learning and the interaction between them is challenging. Accumulating evidence suggests that social learning [defined as ‘learning that is influenced by observation of, or interaction with, another animal (typically a conspecific) or its products’; (Heyes, 1994; Hoppitt and Laland, 2013)] may play a more important role across a broad range of animal taxa than previously anticipated (Galef, 2012; Kendal, 2015; Whiten, 2017). In general, large adaptive value, and consequently, the prevalence of social learning are expected in situations when genetically determined behavioural variability is unproductive, non-social learning is costly or individuals are faced with uncertain, frequently changing environment (Laland, 2004; Rendell et al., 2011; Heyes and Pearce, 2015; Kendal et al., 2018); see also Rieucau and Giraldeau (2011) for a detailed review on the costs and benefits of social learning). Theory suggests that besides influencing whether social learning of a given trait is adaptive and thus expected, the speed of environmental change also influences the mode of social transmission (Laland and Kendal, 2003). Specifically, vertical (from parents to offspring) or oblique transmission (from non-parent adults to offspring) is expected if environmental changes are not significant between subsequent generations, and horizontal transmission (between peers, either immatures or adults) is expected when environment changes rapidly, e.g., from generation to generation (Cavalli-Sforza and Feldman, 1981; Laland and Kendal, 2003).
Parental care, especially when intense or extended such as in great apes and in most bird species, provides ample opportunity for vertical transmission to take place (Whiten, 2017). The predominant avian breeding system is social monogamy with biparental care that has been described in 90% of bird species (Cockburn, 2006). The high prevalence, great diversity and often substantial within-species variation of parental behaviours make biparental passerines an ideal group for investigating the origin of phenotypic variation in terms of genetic and social environmental effects. Since heritability estimates are often difficult to acquire in avian species due to dispersal and low survival, repeatability (the proportion of phenotypic variation in parental effort that is explained by genetic or consistent environmental variation among individuals) was first used as a proxy and upper limit of heritability (Falconer, 1981; Lessells and Boag, 1987). Although the validity of such interpretation of repeatability has later been challenged [repeatability does not necessarily set an upper limit to heritability in various scenarios, e.g., when genotype-environment interactions, or maternal effects are strong; (Dohm, 2002)], numerous studies reported individually consistent parental efforts in different bird species and for various parental behaviours. Remarkably, most of these studies reported sex differences in the repeatability estimates. In the house sparrow (Passer domesticus), a study reported highly repeatable male but unrepeatable female provisioning rates (Schwagmeyer and Mock, 2003), and another study corroborated this finding in addition to showing similar sex differences in repeatabilities for incubation time in this species (Nakagawa et al., 2007). Consistency of care decisions (care or desert the clutch and mate) at subsequent nests in the variable breeding system of the sequentially polygamous Eurasian penduline tit (Remiz pendulinus) showed a contrasting pattern with consistent female, but individually variable male care decisions (Pogány et al., 2008). Similarly, parental work load (measured as daily energy expenditure) was repeatable in female, but not in male pied flycatchers (Ficedula hypoleuca) (Potti et al., 1999). In Manx shearwaters (Puffinus puffinus), however, neither male nor female provisioning rates were repeatable (Gray et al., 2005).
The empirical studies that aimed at directly estimating heritability reported high and moderate heritabilities for parental efforts in birds. The study of Dor and Lotem (2010) stands out by applying an experimental approach. After cross-fostering nestlings between broods in four generations of house sparrows, their analysis revealed that approximately 50% of variation in feeding rates of sons can be explained by the previous generation, whereas genetic heritability was not significant in daughters. The majority of heritability studies, however, applied a quantitative genetic approach, and these early studies investigated heritability as a joint effect of genetic and social environmental effects, hindering discrimination between them. In line with the results of repeatability studies, these investigations also revealed sex differences in heritabilities. For instance, while more than 80% of the phenotypic variation in male feeding rates could be attributed to that of the previous generation in Savannah sparrows (Passerculus sandwichiensis), female feeding rates were not heritable (Freeman-Gallant and Rothstein, 1999). A long-term field study in long-tailed tits (Aegithalos caudatus), besides linking offspring survival to feeding rates, also found significant heritability for parental effort with ca. 43% of variation in male feeding rates explained by the parental generation (MacColl and Hatchwell, 2003).
With the advances of quantitative genetics in the last decades, environmental effects could be further partitioned, considering the indirect genetic effects by the social partners that may have significant influences on the interacting phenotypes (Moore et al., 1997; Wolf et al., 1998). With regards to parental care, a handful of investigations applied such a more-in-depth quantitative genetic framework by further partitioning environmental effects in variation of parental care into indirect genetic effects (IGEs) and indirect environmental effects (IEEs). Brommer and Rattiste (2008), for instance, reported 14.5% of variance in laying date of female common gulls (Larus canus) being explained by direct genetic effects, and an additional 4.8% of variance explained by indirect genetic effects via the males. Furthermore, their analysis revealed that the direct (female) and indirect (male) genetic effects were negatively correlated. Adams et al. (2015) also focused on social environmental effects in explaining variation in the feeding rates of parents and helpers in long-tailed tits, although their analysis did not reveal significant IGEs. Schroeder et al. (2019), however, found large social effects via IGEs in nest visit frequencies of both sexes in house sparrows. In addition to the conventionally calculated heritability estimates (13% in both sexes), IGEs accounted for an additional 11–12% variation, resulting in a total heritable variation of 24 and 25% in females and males, respectively.
The above studies demonstrate that parental care can often have large heritable variation. However, in monogamous species with no or insignificant extra-pair copulations, the transmission of genetic and social effects from a given parent toward the young are inseparable non-experimentally. Here we aim at filling a gap by experimentally investigating the potentially determining social effects of parenting. Previous studies revealed social interactions to have prominent influence on sex role-related behaviours in the socially monogamous, biparental zebra finch (Taeniopygia guttata), such as on mating preferences, nest building and parental care (Immelmann, 1972; Jones et al., 1996; Swaddle et al., 2005; Guillette et al., 2016; Katsis et al., 2018; Pogany et al., 2019). Based on these studies, we chose this small passerine as our model species, and applied a full cross-fostering design to disentangle experimentally genetic and social effects deriving from the parents. We hypothesized that vertical social learning is a crucial process in the ontogeny of parental behaviour. If so, we expected that a larger proportion of the phenotypic variation in nest attendance, incubation, brooding and nestling provisioning will be explained in the cross-fostered second generation by variation of the same traits of their same-sex social than that of their genetic parent.
This experiment was carried out using a population of captive zebra finches in the Animal House of Eötvös Loránd University, Hungary, between October 2015 and June 2019. All subjects were born in the Animal House and were approximately the fourth-generation descendants of our stock population [our stock population was established in 2013 from a domesticated population of Bielefeld University (Germany) (Forstmeier et al., 2007)]. Adult birds were kept in same-sex indoor aviaries until the beginning of the experiment. We established 35 breeding pairs (first generation, henceforth; mean ± SD brood size on day 10, post-hatching = 2.61 ± 1.34) avoiding genetic relatedness, but otherwise randomly assigning the pair members. None of the birds had any successful breeding attempts before the experiment. The breeding of the first-generation pairs allowed us to establish 43 pairs when birds from the offspring generation became sexually mature (second generation, henceforth). In the statistical analysis of the incubation period, we excluded four pairs (one pair each due to failed video recording, the female being clearly afraid of the camera, because the male was aggressive and hurt its mate, and because the pair had missing parental incubation data), while in the analysis of the post-hatching period we excluded five pairs (in one pair, the female, in two other pairs, the offspring died before the video recording, whereas two more pairs had to be excluded due to the male and the female hurting their mates, respectively, during feeding). Pairs were kept in separate cages (100 × 30 × 35 cm) with a wooden nest box attached to each (12 × 12 × 12 cm), and they were provided with fresh nest material (coconut fibres) every second day. Numbered aluminium rings (one ring per bird; Principle Kft., Újlengyel, Hungary) were used for individual identification of the subjects. Optimized light conditions were maintained in the experimental room; a 14:10 h light:dark cycle was adjusted (using full-spectrum tube lights, NASLI, Prague, Czechia). The air conditioning system of the animal house were set to provide moderate conditioning so that temperature and humidity varied somewhat over the 4-year course of the experiment (mean ± SD temperature: 23.7 ± 2.0°C, humidity: 47.7 ± 12.0), which we considered in our analyses. Food and water were provided ad libitum to the birds. Nourishment consisted of a seed mixture, supplemental egg-food (Egg food tropical finches, Orlux, Versele-Laga, Belgium) and home-made germinated seeds [for more details on the diet, see Morvai et al. (2016)].
Nest boxes of the pairs (first generation) were monitored every second day to determine the start of incubation. On day 6 of incubation, we swapped clutches with fertile eggs (presence of embryos were checked by lamping the eggs) between nests in similar stages, thus all pairs took care of an unrelated clutch from that point onward (full cross-fostered design). On day 8 of incubation, between 10:00 and 13:00 [time window for observations were chosen based on Morvai et al. (2016)], parental behaviour was recorded through a hole on the top of the nest boxes. Small digital cameras (Mobius Action Cam, JooVuu Store, United Kingdom) were used to monitor the birds, equipped with wide-angle lenses (116° field of view). The cameras were attached onto the nest boxes a day before timed recording started, and dummy cameras (black wooden blocks) covered the hole on the nest boxes every other day when there was no video recording. After the recording, clutch mass was measured (Radwag WTB 2000, Poland). Nest checks were continued every second day after the recording to determine hatching date (day 13–14 of incubation). On day 10 post-hatching (counted from hatching of the first nestling), between 10:00 and 13:00, parental behaviour was again recorded, and brood mass was measured. Nestlings fledged at around day 18–20 and were removed from their parents well after becoming independent, on day 35 post-hatching. They were kept together with other birds of similar age until their sex could be determined unambiguously. Male tutors were provided in a neighbouring cage to ensure normal development of acoustic communication. Juveniles were then placed into same-sex aviaries until they became sexually mature [approximately on day 90 post-hatching (Zann, 1996)]. Sexually mature second-generation birds were randomly assigned as pairs, and we repeated the same protocol that we applied for the first generation (including full cross-fostering of similar-stage clutches) so that data on their parental behaviour was collected.
Behavioural coding from the video recordings was carried out using Solomon Coder software (Péter, 2015). From the recordings, male and female parental behaviour was coded, simultaneously. We coded the frequency of, and time spent on the following parental behaviours: nest attendance (defined as whenever the bird is seen on the recording of the nestbox camera, i.e., irrespective of what he/she is doing), incubation (sitting on eggs) and brooding (sitting on nestlings). We also coded actual feedings (provisioning begging offspring with regurgitated seeds). For each of the above frequency variables, we calculated the hourly rates, whereas for time variables, the proportion of observation time spent with the given behaviour was calculated.
R statistical environment [v. 4.0.3; (R Core Team, 2020)] was used to analyse genetic and social inheritance in parental behaviour. Since we had multiple variables that were likely highly correlated (e.g., rate and time of any given behaviour, or incubation and nest attendance), to avoid redundancy in our analysis, first we filtered our response variables based on a Spearman’s rank correlation matrix (r > 0.8 between two variables were considered as conveying the same information, so that only one of the variables were included in further analysis). This resulted in the following rate response variables in our analyses (incubation and post-hatching period taken together): hourly incubation or brooding rate, feeding nestlings, and nest attendance. For time (duration) variables, we selected nest attendance time (i.e., the proportion of time the bird was seen on recordings; this variable highly correlated with incubation time (Spearman r = 0.88, df = 76, p < 0.001) and brooding time (r = 0.94, df = 74, p < 0.001), respectively, in the two reproductive stages). Our explanatory variables in focus were the same behaviours as the response variable from the same- and opposite-sex genetic and social parents (e.g., the analysis of nest attendance time (response variable) included nest attendance time by the genetic and social fathers and mothers as explanatory variables). In addition to these variables, in each model we tested for other, potentially confounding effects, such as clutch or brood size, temperature, humidity and the behaviour of the mate.
Response variables were analysed in separate Linear Mixed-effects Models [LMM; R package ‘nlme’ (Pinheiro et al., 2019)]. In addition to the above independent variables, all models included nest ID (the number of the nest where the subject grew up) as a random term, to account for non-independent sampling due to genetic and social relatedness between siblings. We carried out stepwise model selection based on likelihood ratio tests (LRT); we provide χ2 and the corresponding p-values of LRTs of models with and without the explanatory variables in question. We also report parameter estimates (β ± SE) and odds ratios [exp(β), with 95% confidence intervals for log-transformed responses in LMMs] for significant explanatory variables.
We applied two different approaches in our analyses. In our first approach, we analysed the same reproductive stages of the two generations (e.g., a given parental behaviour during incubation (or post-hatching) period of the second generation explained by the same behaviour during incubation (or post-hatching) of the first, parental generation). In our second approach, we analysed whether a given behaviour during incubation period in the second generation is explained by a similar behaviour during post-hatching period in the first generation. In the latter analysis, our aim was to compare the first reproductive stage of the second generation (incubation) with the reproductive stage of their parents which allowed them to socially interact and learn from them (i.e., provisioning during the post-hatching period). Hourly feeding rate and time spent feeding was excluded from the latter analysis, as these could not be matched with any behaviour in the incubation period.
The study was carried out following the Hungarian Laws for the experimentation with animals and with permission of the ethical board of our university (ELTE MÁB #02/2014). All experimental birds and their offspring remained for their entire life at the Animal House, Eötvös Loránd University, Budapest. Birds were visually monitored for health status daily.
Our analysis revealed significant variation in all investigated behavioural variables, allowing us to detect any potential genetic or social effects (see Supplementary Table 1). For a summary of statistical models with significant effects, see Supplementary Table 2).
Neither male, nor female incubation rate was explained by the same behaviour of their genetic (LMM, LRT: all p > 0.074) or social parents (all p > 0.738). However, incubation rate was strongly correlated between pair members (to avoid redundancy, for pair effects we report results with the behaviour of one sex as response and that of the other sex as explanatory variable only henceforth – the reverse analyses provided very similar results in each case; LMM of (log) hourly male incubation rate, effect of pair’s incubation rate: LRT: χ21 = 30.78, p < 0.001; exp(β) = 1.15 [1.10; 1.19], t15 = 6.67, p < 0.001; Figure 1.
Similar to incubation, male and female nest attendance frequencies were not explained by the genetic (all p > 0.218) or social parents’ behaviour (all p > 0.065), although we found positive correlation between the behaviour of pair members [LMM of (log) hourly male nest attendance rate, effect of pair’s attendance rate: LRT: χ21 = 6.48, p = 0.011; exp(β) = 1.13 [1.03; 1.25], t15 = 2.59, p = 0.021; Figure 2A]. Time spent attending the nest in males was not influenced by the same behaviour of their genetic or social parents (all p > 0.350), whereas humidity had a negative effect (LMM of proportion of time spent attending the nest by males, effect of humidity: LRT: χ21 = 3.74, p = 0.053; β ± SE = −0.004 ± 0.002, t12 = −1.90, p = 0.081). In contrast, female nest attendance time was explained by the behaviour of their genetic mother [LMM of (quadratic) proportion of time spent attending the nest by females, effect of genetic mother’s nest attendance time: LRT: χ21 = 4.45, p = 0.035; β ± SE = 0.58 ± 0.27, t24 = 2.13, p = 0.043], but not those of their social parents (p > 0.285). Furthermore, nest attendance time was influenced negatively by the pair’s behaviour (LRT: χ21 = 4.12, p = 0.042; β ± SE = −0.37 ± 0.18, t12 = −2.02, p = 0.066; Figure 2B).
Figure 2. Hourly nest attendance rate (A) and proportion of 3 h observation time spent attending the nest (B) in incubating zebra finch pairs.
Male brooding rate (sqrt-transformed) was influenced by the behaviour of the genetic father (LMM of hourly male brooding rate, effect of genetic father’s brooding rate, LRT: χ21 = 4.06, p = 0.044; β ± SE = 0.06 ± 0.03, t19 = 1.95, p = 0.066; Figure 3A), but not that of the social parents (p > 0.150). Besides brood size (LRT: χ21 = 4.12, p = 0.042; β ± SE = −0.17 ± 0.08, t10 = −1.96, p = 0.078), temperature also had a negative effect on male brooding rate (LMM, LRT: χ21 = 5.68, p = 0.017; β ± SE = −0.14 ± 0.06, t10 = −2.33, p = 0.042). In contrast, female brooding rate was not explained by the genetic (all p > 0.093) or social parents’ behaviour [all p > 0.570), whereas brood size had a negative effect (LRT: χ21 = 5.98, p = 0.015; exp(β) = 0.79 [0.66; 0.95], t11 = −2.48, p = 0.031].
Figure 3. Hourly brooding rate (A) and incubation rate (B) of male zebra finches based on hourly brooding rate of their genetic and social father, respectively.
In contrast to brooding, neither male, nor female nest attendance frequencies were explained by the genetic (all p > 0.191) or social parents’ behaviour (all p > 0.100). However, nest attendance rate was positively influenced by the pair’s behaviour [LMM of (log) hourly male nest attendance rate, effect of pair’s nest attendance rate: LRT: χ21 = 12.21, p < 0.001; exp(β) = 1.11 [1.05; 1.17], t14 = 3.69, p = 0.002]. Male and female nest attendance times were also not explained by either their genetic (all p > 0.100), or their social parents’ behaviour (all p > 0.503). Brood size, however, had negative effect on nest attendance time in both sexes [LMM of (sqrt-transformed) nest attendance time in males: LRT: χ21 = 5.94, p = 0.015, β ± SE = −0.05 ± 0.02, t14 = −2.47, p = 0.027; LMM of (logit) nest attendance time in females: LRT: χ21 = 9.65, p = 0.002, exp(β) = 0.64 [0.50; 0.83], t11 = −3.25, p = 0.008].
Offspring feeding rate was not influenced by the feeding rate of either the genetic (all p > 0.116) or social parents (all p > 0.157), or by any other investigated variables.
Incubation rate in males was influenced by their social father’s brooding rate (LMM of (log) hourly male incubation rate, effect of brooding rate of social father; LRT: χ21 = 8.59, p = 0.003; exp(β) = 1.06 [1.02; 1.10], t14 = 2.98, p = 0.010; Figure 3B), besides the effect of their pairs’ behaviour (see also above (Results, Incubation period); LRT: χ21 = 37.50, p < 0.001; exp(β) = 1.15 [1.11; 1.20], t14 = 7.63, p < 0.001). On the other hand, female incubation rate was not influenced by brooding rate of their social (p > 0.780) or genetic parents (p > 0.071).
Neither male, nor female nest attendance rate were explained by the corresponding behaviour of their genetic (all p > 0.146) or social parents (all p > 0.442) during the post-hatching period.
For the effects of the pair’s behaviour and humidity on parental care during incubation of the second generation, see the analysis of both generations’ incubation period above.
Applying a full cross-fostering experimental design, our study disentangled genetic and social parental effects in transmission of parental sex roles to the next generation. We revealed further evidence for sex-specific genetic and social inheritance, as well as non-social environmental effects, but in contrast with our expectations, social effects from parents overall proved to explain very little variation in different parental behaviours. Another component of the within-family social environment, nevertheless, turned out to be determining; we found strong interdependence between the behaviour of the focal parent and that of its current mate in various parental behaviours. Hence, our results are in line with current behavioural studies underlying the importance of negotiation between parents.
We found significant genetic transmission in two of the investigated parental behaviours: female nest attendance time and male brooding rate. In addition, social effects were revealed in incubation rate, whereby males, but not females, adjusted their incubation rate based on the brooding rate they had previously experienced from their social father. This similarity could be the result of social learning from parents (i.e., vertical social learning), as well as other mechanisms, including physiological and asocial environmental effects (such as the direct effects of low quality food from parents, instead of observing and learning parental behaviour per se). However, while previous studies reported heritable variation in various parental traits (Freeman-Gallant and Rothstein, 1999; Potti et al., 1999; MacColl and Hatchwell, 2003; Schwagmeyer and Mock, 2003; Nakagawa et al., 2007; Brommer and Rattiste, 2008; Pogány et al., 2008; Dor and Lotem, 2010; Adams et al., 2015; Schroeder et al., 2019), to our knowledge, our study stands out by presenting a detailed analysis of multiple parental behaviours while experimentally separating heritability via genes and social experiences from parents. Our findings on genetic and social heritability, in combination, suggest sex- and context-specific transgenerational transmission of parental behaviours. Corresponding with previous studies reporting sex differences in repeatability and heritability of parenting (Freeman-Gallant and Rothstein, 1999; Potti et al., 1999; Schwagmeyer and Mock, 2003; Pogány et al., 2008; Dor and Lotem, 2010), males and females were different in the relative strength of genetic and social effects that shape any particular parental behaviour. It is also apparent from our results that neither genetic, nor social inheritance should be considered as a universal process behind variation in parental behaviours, in general. In contrast to our separately caged breeding pairs, individuals in natural populations experience further social and asocial environmental influences, including competition over resources, interference by conspecifics, potential extra-pair activities. The lack of an overall strong social effect from parents under controlled laboratory conditions (where all the above mentioned potentially influential effects are excluded) suggest that social effects from parents cannot be significant in natural conditions, either.
Focusing on the sex differences in the three behavioural traits revealing heritability, female nest attendance time appears to be under stronger genetic influence. In contrast, male nest attendance time was not related to the behaviour of the genetic father, however, it was influenced negatively by humidity, suggesting a more flexible response and compensation to changes in the non-social environment by males than by females during incubation. Our results are in line with previous studies reporting male and female parents to react differently to environmental changes in various bird species (Magrath et al., 2005; Charmantier et al., 2008; Kosztolányi et al., 2009; Vincze et al., 2017; Rohwer and Purcell, 2019; Sharpe et al., 2021; Ton et al., 2021). In zebra finches, a recent study also corroborates our finding suggesting sexually different flexibility and reactions to changing environmental conditions, however, with a sexually contrasting effect of ambient temperature (Ton et al., 2021). In this study females, rather than males, decreased their nest attendance time in response to high ambient temperatures. Time spent attending the nest during incubation is one of the most expressed parental sex role differences in zebra finches, with a bias toward females in captive populations [ca. 39% vs 72% male vs female nest attendance time during incubation in our population, see also Gorman and Nager (2003), Morvai et al. (2016), and Ton et al. (2021)]. This bias, taken together with our results on male response to humidity changes and those of Ton et al. (2021) on female response to temperature changes suggest sexually different capacities to respond to specific environmental conditions, involving a possible ceiling-effect in females. Males might respond more to environmental changes (such as lower humidity) at lower temperatures because females perhaps already spend as much time with nest attendance as their metabolism and energy demands and overall condition allow under these conditions (reflected in a decreased effort when higher temperatures allow this). In addition, only female zebra finches have a brood patch, so that their more effective heat transfer may contribute to sexually different optimization strategies of incubation effort (Zann and Rossetto, 1991; Hill et al., 2014). Similar to female nest attendance, the analysis of male brooding rate also revealed genetic transmission, in addition to a negative effect of brood size (a similar effect was also found in female brooding rate analysis) and temperature.
Our cross-reproductive stage analysis (comparing post-hatching behaviour of the parental generation with parental behaviour of the second generation during incubation) provided the only evidence for parental social effects in our experiment. Variation of male incubation rate was explained by the brooding rate experienced from social fathers. The behavioural pattern experienced from parents, however, appeared to have only a temporal effect, because our analysis of the next reproductive stage, post-hatching period, revealed brooding rate to be influenced by the genetic, rather than the social father’s behaviour. Our previous experiment, in which we applied a split-family experimental design to create uniparentally raised young, resulted in very similar transitional social effects (Pogany et al., 2019). Both female-only and male-only cared zebra finches showed different parental sex role division when incubating during their first breeding based on their social experiences with each of their parents. This effect, however, could no longer be observed during the post-hatching period of their first breeding, or their second breeding attempt, suggesting that their own breeding experiences with their mate overrode any behavioural patterns they might have taken over from their parents socially. The results of our present study corroborate this scenario; with two strikingly different experimental approaches, both studies point toward the importance of current (due to the pair), rather than past (parental), social environmental effects in shaping parental behaviours, while suggesting a temporal social effect form the parents.
The most important and consistent social effect we found in our study is that of the current mate. This reflected in the number of parental behaviours and that we found significant pair effects in both reproductive stages (incubation rate, nest attendance rate during incubation and post-hatching period), but also in the effect sizes in relation to the above genetic and social effects due to parents. Pair members provided parental care in both reproductive stages in close coordination with each other. Considering the flexibility of parental coordination and the substantial differences between laboratory and natural conditions including the presence-absence of environmental constraints, our results are likely underestimating the importance and intensity of parental coordination in nature. Even so, during incubation, time spent with nest attendance correlated negatively, and visit rate correlated positively between pair members, suggesting turn-taking (cf. Johnstone and Savage, 2019), whereby males and females invest only after each contribution by their partner. During post-hatching, nest attendance rate was also positively correlated, suggesting coordinated, synchronized feeding of young (cf. Mariette and Griffith, 2012). Synchronized, rather than independent nest visits may reduce sibling competition through more equal food distribution between offspring or decrease the chance of depredation as a result of lower number of overall nest visits (Shen et al., 2010; Mariette and Griffith, 2012; Leniowski and Wegrzyn, 2018; Wojczulanis-Jakubas et al., 2018; Ihle et al., 2019). In addition, synchronized provisioning behaviour might be the result of other, non-parental activities, for instance foraging patterns (Masello et al., 2006). Incubation is a prolonged and continuous behaviour compared to offspring provisioning bouts. Therefore, the two behaviours are inherently different in the opportunity for pair members to replace each other. Turn-takings during incubation and synchronized offspring provisioning are in line with theoretical models of biparental care suggesting pair members should respond to each other’s parental effort by real-time negotiation (McNamara et al., 1999, 2003; Johnstone and Hinde, 2006; Lessells and McNamara, 2012; Johnstone and Savage, 2019; Barbasch et al., 2020). Accumulating empirical evidence support this view (Hinde, 2006; Hinde and Kilner, 2007; Kosztolányi et al., 2009; Meade et al., 2011; Lendvai et al., 2018); a recent study, besides reporting coordinated turn-taking during incubation, identified acoustic communication between zebra finch pair members as a key factor facilitating coordination (Boucaud et al., 2016, 2017). Since variability in female nest attendance time had a significant genetic component in our analysis, it is possible that female nest attendance behaviour is less flexible, whereas males more likely adjust their parental investment to maintain the level of total care needed for successfully raising the offspring (cf. Adams et al., 2015).
Our results further corroborate that parental behaviour of zebra finch pairs are in strong interdependence, as partners respond to each other’s behaviour in both reproductive stages, providing ample opportunities for IGEs to shape parental behaviour in this species. Although our study did not aim at investigating IGEs, our results suggest they likely play a prominent role in the evolution of parental care in this species, in line with recent theoretical and empirical studies (Brommer and Rattiste, 2008; Royle et al., 2014; Bailey et al., 2018; Schroeder et al., 2019).
Our findings also contribute to the growing body of empirical research reporting parental response to offspring needs (Gilby et al., 2011; Rehling et al., 2012; Royle et al., 2014; Trillmich et al., 2016; Lendvai et al., 2018; Westneat and Mutzel, 2019). Besides more food demands of a larger brood, thermoregulatory needs of non-feathered nestlings can differ depending on the number of nestlings in the nest, as more nestlings can keep each other warmer, allowing parents to leave a bigger brood alone for longer periods. The degree of recognizing offspring needs can be an important factor that can also influence parental negotiation. Lendvai et al. (2018), for instance, experimentally increased begging calls of tree swallow nestlings in the presence of their mother only. In response, females fed their offspring more, which elicited similar behaviour from their partner, providing an example for real-time response in a situation where males presumably relied on the female’s information about the offspring needs.
Our results provided further evidence for sex- and context-specific genetic and social heritability of parental behaviours. Instead of a universal genetic inheritance or vertical social learning, the relative importance of parental effects and the mode of transmission appears to vary between the sexes and various aspects of parenting. Changes in the non-social environment elicited sexually different parental care responses. In addition, we found strong interdependence between parental behaviour of the pair members, suggesting that current social effects due to the mate are more important than past social experiences or genetic transmission from parents in explaining variation in parental behaviours.
The original contributions presented in the study are included in the article/Supplementary Material, further inquiries can be directed to the corresponding author.
The animal study was reviewed and approved by the Animal Welfare Committee, Eötvös Loránd University, Budapest, Hungary (ELTE-MÁB).
ÁP conceived the study with input from ÁM. ÁP, BM, and EF contributed to experimental design and managing the experiments. BM and EF carried out the experiments and collected data. BM coded video recordings and managed the database. ÁP and BM analysed the data. BM and ÁP wrote the manuscript with input from ÁM and EF. All authors contributed to the revision and final manuscript.
This study was supported by the Hungarian Scientific Research Fund (OTKA K109337 and FK131966) and was completed as part of the ELTE Thematic Excellence Programme 2020 supported by the National Research, Development and Innovation Office (TKP2020-IKA-05). ÁP was supported by the János Bolyai Research Scholarship of the Hungarian Academy of Sciences, the ÚNKP-20-5 New National Excellence Program of the Ministry for Innovation and Technology from the source of the National Research, Development and Innovation Fund and the Hungarian Ethology Foundation.
The authors declare that the research was conducted in the absence of any commercial or financial relationships that could be construed as a potential conflict of interest.
All claims expressed in this article are solely those of the authors and do not necessarily represent those of their affiliated organizations, or those of the publisher, the editors and the reviewers. Any product that may be evaluated in this article, or claim that may be made by its manufacturer, is not guaranteed or endorsed by the publisher.
We thank László Zsolt Garamszegi for statistical advice. We also thank Buddhamas Kriengwatana and Roi Dor for their valuable comments on previous versions of the manuscript.
The Supplementary Material for this article can be found online at: https://www.frontiersin.org/articles/10.3389/fevo.2021.799996/full#supplementary-material
Adams, M. J., Robinson, M. R., Mannarelli, M. E., and Hatchwell, B. J. (2015). Social genetic and social environment effects on parental and helper care in a cooperatively breeding bird. Proc. R. Soc. B Biol. Sci. 282:1810. doi: 10.1098/rspb.2015.0689
Bailey, N. W., Marie-Orleach, L., and Moore, A. J. (2018). Indirect genetic effects in behavioral ecology: does behavior play a special role in evolution? Behav. Ecol. 29, 1–11. doi: 10.1093/beheco/arx127
Barbasch, T. A., Alonzo, S. H., and Buston, P. M. (2020). Power and punishment influence negotiations over parental care. Behav. Ecol. 31, 911–921. doi: 10.1093/beheco/araa034
Boucaud, I. C. A., Mariette, M. M., Villain, A. S., and Vignal, C. (2016). Vocal negotiation over parental care? Acoustic communication at the nest predicts partners’ incubation share. Biol. J. Linn. Soc. 117, 322–336. doi: 10.1111/bij.12705
Boucaud, I. C. A., Perez, E. C., Ramos, L. S., Griffith, S. C., and Vignal, C. (2017). Acoustic communication in zebra finches signals when mates will take turns with parental duties. Behav. Ecol. 28, 645–656. doi: 10.1093/beheco/arw189
Brommer, J. E., and Rattiste, K. (2008). “Hidden” reproductive conflict between mates in a wild bird population. Evolution 62, 2326–2333. doi: 10.1111/j.1558-5646.2008.00451.x
Cavalli-Sforza, L. L., and Feldman, M. W. (1981). Cultural Transmission and Evolution: A Quantitative Approach. Princeton, NJ: Princeton University Press.
Charmantier, A., McCleery, R. H., Cole, L. R., Perrins, C., Kruuk, L. E. B., and Sheldon, B. C. (2008). Adaptive phenotypic plasticity in response to climate change in a wild bird population. Science 320, 800–803. doi: 10.1126/science.1157174
Clutton-Brock, T. H. (1991). The Evolution of Parental Care. Princeton, NJ: Princeton University Press.
Cockburn, A. (2006). Prevalence of different modes of parental care in birds. Proc. Biol. Sci. 273, 1375–1383. doi: 10.1098/rspb.2005.3458
Dohm, M. R. (2002). Repeatability estimates do not always set an upper limit to heritability. Funct. Ecol. 16, 273–280.
Dor, R., and Lotem, A. (2010). Parental effort and response to nestling begging in the house sparrow: repeatability, heritability and parent-offspring co-evolution. J. Evol. Biol. 23, 1605–1612. doi: 10.1111/j.1420-9101.2010.02023.x
Fairbairn, D. J. (2013). Odd Couples: Extraordinary Differences Between the Sexes in the Animal Kingdom. Princeton, NJ: Princeton University Press.
Forstmeier, W., Segelbacher, G., Mueller, J. C., and Kempenaers, B. (2007). Genetic variation and differentiation in captive and wild zebra finches (Taeniopygia guttata). Mol. Ecol. 16, 4039–4050. doi: 10.1111/j.1365-294X.2007.03444.x
Freeman-Gallant, C. R., and Rothstein, M. D. (1999). Apparent heritability of parental care in Savannah Sparrows. Auk 116, 1132–1136.
Fromhage, L., Jennions, M., and Kokko, H. (2016). The evolution of sex roles in mate searching. Evolution 70, 617–624. doi: 10.1111/evo.12874
Fromhage, L., and Jennions, M. D. (2016). Coevolution of parental investment and sexually selected traits drives sex-role divergence. Nat. Commun. 7:12517. doi: 10.1038/ncomms12517
Galef, B. G. (2012). Social learning and traditions in animals: evidence, definitions, and relationship to human culture. Wiley Interdiscip. Rev. Cogn. Sci. 3, 581–592. doi: 10.1002/wcs.1196
Gilby, A. J., Mainwaring, M. C., and Griffith, S. C. (2011). The adaptive benefit of hatching asynchrony in wild zebra finches. Anim. Behav. 82, 479–484. doi: 10.1016/j.anbehav.2011.05.022
Gorman, H. E., and Nager, R. G. (2003). State-dependent incubation behaviour in the zebra finch. Anim. Behav. 65, 745–754. doi: 10.1006/anbe.2003.2120
Gray, C. M., Brooke, M. D. L., and Hamer, K. C. (2005). Repeatability of chick growth and food provisioning in Manx shearwaters Puffinus puffinus. J. Avian Biol. 36, 374–379. doi: 10.1111/j.0908-8857.2005.03597.x
Guillette, L. M., Scott, A. C. Y., and Healy, S. D. (2016). Social learning in nest-building birds: a role for familiarity. Proc. R. Soc. B Biol. Sci. 283:20152685. doi: 10.1098/rspb.2015.2685
Henshaw, J. M., Fromhage, L., and Jones, A. G. (2019). Sex roles and the evolution of parental care specialization. Proc. R. Soc. B Biol. Sci. 286:20191312. doi: 10.1098/rspb.2019.1312
Heyes, C., and Pearce, J. M. (2015). Not-so-social learning strategies. Proc. R. Soc. B Biol. Sci. 282:20141709. doi: 10.1098/rspb.2014.1709
Heyes, C. M. (1994). Social-learning in animals - categories and mechanisms. Biol. Rev. Camb. Philos. Soc. 69, 207–231. doi: 10.1111/j.1469-185X.1994.tb01506.x
Hill, D. L., Lindstrom, J., McCafferty, D. J., and Nager, R. G. (2014). Female but not male zebra finches adjust heat output in response to increased incubation demand. J Exp Biol 217(Pt 8), 1326–1332. doi: 10.1242/jeb.095323
Hinde, C. A. (2006). Negotiation over offspring care?—a positive response to partner-provisioning rate in great tits. Behav. Ecol. 17, 6–12. doi: 10.1093/beheco/ari092
Hinde, C. A., and Kilner, R. M. (2007). Negotiations within the family over the supply of parental care. Proc. Biol. Sci. 274, 53–60. doi: 10.1098/rspb.2006.3692
Hoppitt, W., and Laland, K. N. (2013). Social Learning: An Introduction to Mechanisms, Methods, and Models. Princeton, NJ: Princeton University Press.
Ihle, M., Pick, J. L., Winney, I. S., Nakagawa, S., Schroeder, J., and Burke, T. (2019). Rearing success does not improve with apparent pair coordination in offspring provisioning. Front. Ecol. Evol. 7:405. doi: 10.3389/fevo.2019.00405
Immelmann, K. (1972). Sexual and other long-term aspects of imprinting in birds and other species. Adv. Study Behav. 4, 147–174.
Johnstone, R. A., and Hinde, C. A. (2006). Negotiation over offspring care—how should parents respond to each other’s efforts? Behav. Ecol. 17, 818–827. doi: 10.1093/beheco/arl009
Johnstone, R. A., and Savage, J. L. (2019). Conditional cooperation and turn-taking in parental care. Front. Ecol. Evol. 7:335. doi: 10.3389/fevo.2019.00335
Jones, A. E., TenCate, C., and Slater, P. J. B. (1996). Early experience and plasticity of song in adult male zebra finches (Taeniopygia guttata). J. Compar. Psychol. 110, 354–369.
Kahn, A. T., Schwanz, L. E., and Kokko, H. (2013). Paternity protection can provide a kick-start for the evolution of male-only parental care. Evolution 67, 2207–2217. doi: 10.1111/evo.12103
Katsis, A. C., Davies, M. H., Buchanan, K. L., Kleindorfer, S., Hauber, M. E., and Mariette, M. M. (2018). Prenatal exposure to incubation calls affects song learning in the zebra finch. Sci. Rep. 8:15232. doi: 10.1038/s41598-018-33301-5
Kendal, R. L. (2015). “Social learning and culture in non-human organisms,” in International Encyclopedia of the Social & Behavioral Sciences, ed. J. D. Wright (Oxford: Elsevier), 401–408.
Kendal, R. L., Boogert, N. J., Rendell, L., Laland, K. N., Webster, M., and Jones, P. L. (2018). Social learning strategies: bridge-building between fields. Trends Cogn. Sci. 22, 651–665. doi: 10.1016/j.tics.2018.04.003
Kokko, H., and Jennions, M. D. (2008). Parental investment, sexual selection and sex ratios. J. Evol. Biol. 21, 919–948. doi: 10.1111/j.1420-9101.2008.01540.x
Kokko, H., Jennions, M. D., and Brooks, R. (2006). Unifying and testing models of sexual selection. Annu. Rev. Ecol. Evol. Syst. 37, 43–66. doi: 10.1146/annurev.ecolsys.37.091305.110259
Kosztolányi, A., Cuthill, I. C., and Székely, T. (2009). Negotiation between parents over care: reversible compensation during incubation. Behav. Ecol. 20, 446–452. doi: 10.1093/beheco/arn140
Laland, K. N., and Kendal, J. R. (2003). “What the models say about social learning,” in The Biology of Traditions: Models and Evidence, eds D. M. Fragaszy and S. Perry (Cambridge: Cambridge University Press), 33–55.
Lendvai, Á. Z., Akçay, Ç, Stanback, M., Haussmann, M. F., Moore, I. T., and Bonier, F. (2018). Male parental investment reflects the level of partner contributions and brood value in tree swallows. Behav. Ecol. Sociobiol. 72:185. doi: 10.1007/s00265-018-2594-3
Leniowski, K., and Wegrzyn, E. (2018). Synchronisation of parental behaviours reduces the risk of nest predation in a socially monogamous passerine bird. Sci. Rep. 8:7385. doi: 10.1038/s41598-018-25746-5
Lessells, C. M., and Boag, P. T. (1987). Unrepeatable repeatabilities - a common mistake. Auk 104, 116–121. doi: 10.2307/4087240
Lessells, C. M., and McNamara, J. M. (2012). Sexual conflict over parental investment in repeated bouts: negotiation reduces overall care. Proc. Biol. Sci. 279, 1506–1514. doi: 10.1098/rspb.2011.1690
MacColl, A. D. C., and Hatchwell, B. J. (2003). Heritability of parental effort in a passerine bird. Evolution 57, 2191–2195.
Magrath, M. J. L., van Overveld, T., and Komdeur, J. (2005). Contrasting effects of reduced incubation cost on clutch attendance by male and female European starlings. Behaviour 142, 1479–1493. doi: 10.1163/156853905774831837
Mariette, M. M., and Griffith, S. C. (2012). Nest visit synchrony is high and correlates with reproductive success in the wild Zebra finch Taeniopygia guttata. J. Avian Biol. 43, 131–140. doi: 10.1111/j.1600-048X.2012.05555.x
Masello, J. F., Pagnossin, M. L., Sommer, C., and Quillfeldt, P. (2006). Population size, provisioning frequency, flock size and foraging range at the largest known colony of Psittaciformes: the Burrowing Parrots of the north-eastern Patagonian coastal cliffs. Emu 106, 69–79. doi: 10.1071/Mu04047
McNamara, J. M., Gasson, C. E., and Houston, A. I. (1999). Incorporating rules for responding into evolutionary games. Nature 401, 368–371. doi: 10.1038/43872
McNamara, J. M., Houston, A. I., Barta, Z., and Osorno, J. L. (2003). Should young ever be better off with one parent than with two? Behav. Ecol. 14, 301–310. doi: 10.1093/beheco/14.3.301
McNamara, J. M., and Wolf, M. (2015). Sexual conflict over parental care promotes the evolution of sex differences in care and the ability to care. Proc. R. Soc. B Biol. Sci. 282:20142752. doi: 10.1098/rspb.2014.2752
Meade, J., Nam, K. B., Lee, J. W., and Hatchwell, B. J. (2011). An experimental test of the information model for negotiation of biparental care. PLoS One 6:e19684. doi: 10.1371/journal.pone.0019684
Moore, A. J., Brodie, E. D., and Wolf, J. B. (1997). Interacting phenotypes and the evolutionary process .1. direct and indirect genetic effects of social interactions. Evolution 51, 1352–1362. doi: 10.1111/j.1558-5646.1997.tb01458.x
Morvai, B., Nanuru, S., Mul, D., Kusche, N., Milne, G., Székely, T., et al. (2016). Diurnal and reproductive stage-dependent variation of parental behaviour in captive Zebra Finches. PloS one 11:e0167368. doi: 10.1371/journal.pone.0167368
Nakagawa, S., Gillespie, D. O. S., Hatchwell, B. J., and Burke, T. (2007). Predictable males and unpredictable females: sex difference in repeatability of parental care in a wild bird population. J. Evol. Biol. 20, 1674–1681. doi: 10.1111/j.1420-9101.2007.01403.x
Pinheiro, J., Bates, D., DebRoy, S., and Sarkar, D. R Core Team (2019). nlme: Linear and Non-Linear Mixed Effects Models”. R Package Version 3.1-139 ed. Vienna: R Foundation for Statistical Computing.
Pogany, A., Morvai, B., Krause, E. T., Kitsios, E., Bohm, T., Ruploh, T., et al. (2019). Short- and long-term social effects of parental sex roles in zebra finches. Front. Ecol. Evol. 7:294. doi: 10.3389/fevo.2019.00294
Pogány, A., Szentirmai, I., Komdeur, J., and Székely, T. (2008). Sexual conflict and consistency of offspring desertion in Eurasian penduline tit Remiz pendulinus. BMC Evol. Biol. 8:242. doi: 10.1186/1471-2148-8-242
Potti, J., Moreno, J., and Merino, S. (1999). Repeatability of parental effort in male and female Pied Flycatchers as measured with doubly labeled water. Can. J. Zool. Revue Can. Zool. 77, 174–179. doi: 10.1139/cjz-77-1-174
R Core Team (2020). R: A Language and Environment for Statistical Computing. Vienna: R Foundation for Statistical Computing.
Rehling, A., Spiller, I., Krause, E. T., Nager, R. G., Monaghan, P., and Trillmich, F. (2012). Flexibility in the duration of parental care: zebra finch parents respond to offspring needs. Anim. Behav. 83, 35–39. doi: 10.1016/j.anbehav.2011.10.003
Rendell, L., Fogarty, L., Hoppitt, W. J., Morgan, T. J., Webster, M. M., and Laland, K. N. (2011). Cognitive culture: theoretical and empirical insights into social learning strategies. Trends Cogn. Sci. 15, 68–76. doi: 10.1016/j.tics.2010.12.002
Rieucau, G., and Giraldeau, L. A. (2011). Exploring the costs and benefits of social information use: an appraisal of current experimental evidence. Philos. Trans. R. Soc. B Biol. Sci. 366, 949–957. doi: 10.1098/rstb.2010.0325
Rohwer, V. G., and Purcell, J. R. (2019). Geographic variation in incubation behavior of a widely distributed passerine bird. PLoS One 14:e0219907. doi: 10.1371/journal.pone.0219907
Royle, N. J., Russell, A. F., and Wilson, A. J. (2014). The evolution of flexible parenting. Science 345, 776–781. doi: 10.1126/science.1253294
Royle, N. J., Smiseth, P. T., and Kölliker, M. (2012). The Evolution of Parental Care. Oxford: Oxford University Press.
Schroeder, J., Dugdale, H., Nakagawa, S., Sparks, A., and Burke, T. (2019). Social genetic effects (IGE) and genetic intra- and intersexual genetic correlation contribute to the total heritable variance in parental care. EcoEvoRxiv [Preprint] doi: 10.32942/osf.io/nh8m2
Schwagmeyer, P. L., and Mock, D. W. (2003). How consistently are good parents good parents? Repeatability of parental care in the house sparrow, Passer domesticus. Ethology 109, 303–313. doi: 10.1046/j.1439-0310.2003.00868.x
Sharpe, L. L., Bayter, C., and Gardner, J. L. (2021). Too hot to handle? Behavioural plasticity during incubation in a small, Australian passerine. J. Therm. Biol. 98:102921. doi: 10.1016/j.jtherbio.2021.102921
Shen, S. F., Chen, H. C., Vehrencamp, S. L., and Yuan, H. W. (2010). Group provisioning limits sharing conflict among nestlings in joint-nesting Taiwan yuhinas. Biol. Lett. 6, 318–321. doi: 10.1098/rsbl.2009.0909
Swaddle, J. P., Cathey, M. G., Correll, M., and Hodkinson, B. P. (2005). Socially transmitted mate preferences in a monogamous bird: a non-genetic mechanism of sexual selection. Proc. R. Soc. B Biol. Sci. 272, 1053–1058. doi: 10.1098/rspb.2005.3054
Ton, R., Hurley, L. L., and Griffith, S. C. (2021). Higher experimental ambient temperature decreases female incubation attentiveness in Zebra Finches (Taeniopygia guttata) and lower effort yields negligible energy savings. IBIS 163, 1045–1055. doi: 10.1111/ibi.12922
Trillmich, F., Spiller, I., Naguib, M., and Krause, E. T. (2016). Patient parents: do offspring decide on the timing of fledging in zebra finches? Ethology 122, 411–418. doi: 10.1111/eth.12490
Vincze, O., Kosztolányi, A., Barta, Z., Küpper, C., Alrashidi, M., Amat, J. A., et al. (2017). Parental cooperation in a changing climate: fluctuating environments predict shifts in care division. Glob. Ecol. Biogeogr. 26, 347–358. doi: 10.1111/geb.12540
Westneat, D. F., and Mutzel, A. (2019). Variable parental responses to changes in offspring demand have implications for life history theory. Behav. Ecol. Sociobiol. 73:130. doi: 10.1007/s00265-019-2747-z
Whiten, A. (2017). A second inheritance system: the extension of biology through culture. Interf. Focus 7:20160142. doi: 10.1098/rsfs.2016.0142
Wojczulanis-Jakubas, K., Araya-Salas, M., and Jakubas, D. (2018). Seabird parents provision their chick in a coordinated manner. PLoS One 13:e0189969. doi: 10.1371/journal.pone.0189969
Wolf, J. B., Brodie, E. D., Cheverud, J. M., Moore, A. J., and Wade, M. J. (1998). Evolutionary consequences of indirect genetic effects. Trends Ecol. Evol. 13, 64–69. doi: 10.1016/S0169-5347(97)01233-0
Zann, R., and Rossetto, M. (1991). Zebra finch incubation - brood patch, egg temperature and thermal-properties of the nest. EMU 91, 107–120.
Keywords: sex differences, parental care, heritability, social environment, parental coordination, negotiation, Taeniopygia guttata
Citation: Morvai B, Fazekas EA, Miklósi Á and Pogány Á (2022) Genetic and Social Transmission of Parental Sex Roles in Zebra Finch Families. Front. Ecol. Evol. 9:799996. doi: 10.3389/fevo.2021.799996
Received: 22 October 2021; Accepted: 13 December 2021;
Published: 07 January 2022.
Edited by:
Hope Klug, University of Tennessee at Chattanooga, United StatesReviewed by:
Buddhamas Kriengwatana, University of St Andrews, United KingdomCopyright © 2022 Morvai, Fazekas, Miklósi and Pogány. This is an open-access article distributed under the terms of the Creative Commons Attribution License (CC BY). The use, distribution or reproduction in other forums is permitted, provided the original author(s) and the copyright owner(s) are credited and that the original publication in this journal is cited, in accordance with accepted academic practice. No use, distribution or reproduction is permitted which does not comply with these terms.
*Correspondence: Boglárka Morvai, Ym9nbGFya2EubW9ydmFpQHR0ay5lbHRlLmh1
Disclaimer: All claims expressed in this article are solely those of the authors and do not necessarily represent those of their affiliated organizations, or those of the publisher, the editors and the reviewers. Any product that may be evaluated in this article or claim that may be made by its manufacturer is not guaranteed or endorsed by the publisher.
Research integrity at Frontiers
Learn more about the work of our research integrity team to safeguard the quality of each article we publish.