- 1State Key Laboratory of Urban and Regional Ecology, Research Center for Eco-Environmental Sciences, Chinese Academy of Sciences, Beijing, China
- 2College of Resources and Environment, University of Chinese Academy of Sciences, Beijing, China
- 3Hulunbuir Eco-Environmental Monitoring Center of Inner Mongolia, Hulunbuir, China
- 4State Key Laboratory of Earth Surface Processes and Resource Ecology, Faculty of Geographical Science, Beijing Normal University, Beijing, China
Both livestock grazing and soil freeze-thaw cycles (FTCs) can affect the soil-atmosphere exchange of greenhouse gases (GHGs) in grasslands. However, the combined effects of grazing and FTCs on GHG fluxes in meadow steppe soils remain unclear. In this study, we collected soils from paired grazing and enclosed sites and conducted an incubation experiment to investigate the effect of grazing on soil GHG fluxes in the meadow steppes of Inner Mongolia during three FTCs. Our results showed that FTCs substantially stimulated the emissions of soil N2O and CO2 and the uptake of CH4 in the meadow steppes. However, compared with enclosure treatments, grazing significantly reduced the cumulative N2O, CO2 and CH4 fluxes by 13.3, 14.6, and 26.8%, respectively, during the entire FTCs experiment. The soil dissolved organic carbon (DOC) and nitrogen (DON), NH4+-N and NO3–-N, significantly increased after three FTCs and showed close correlations with N2O and CO2 emissions. Structural equation modeling (SEM) revealed that the increase in NO3–-N induced by FTCs dominated the variance in N2O emissions and that DOC strongly affected CO2 emissions during thawing periods. However, long-term grazing reduced soil substrate availability and microbial activity and increased soil bulk density, which in turn decreased the cumulative GHG fluxes during FTCs. In addition, the interaction between grazing and FTCs significantly affected CO2 and CH4 fluxes but not N2O fluxes. Our results indicated that livestock grazing had an important effect on soil GHG fluxes during FTCs. The combined effect of grazing and FTCs should be taken into account in future estimations of GHG budgets in both modeling and experimental studies.
Introduction
Greenhouse gases (GHGs), including carbon dioxide (CO2), methane (CH4) and nitrous oxide (N2O), can significantly promote climate warming on Earth. Upland soils normally serve as a major source of N2O and CO2 but a sink for CH4 to atmosphere (Holst et al., 2008). Recent studies have reported that soil GHG fluxes vary substantially both spatially and temporally (Wagner-Riddle et al., 2017; Congreves et al., 2018), which is attributed to the crucial biophysical processes in soil and their responses to external environmental factors (Smith et al., 2018; Jalaludin et al., 2020). For example, short-term N2O pulse emissions triggered by fertilization, soil freeze-thaw events or soil rewetting can dominate the annual GHG budget in a variety of terrestrial ecosystems (Wolf et al., 2010; Kostyanovsky et al., 2019; Gu et al., 2021).
Freeze-thaw cycles (FTCs), which occur predominantly in the soils of some temperate and most high-latitude and high-altitude regions, can greatly affect soil-atmosphere exchange of GHGs in the soil (Smith et al., 2018; Li et al., 2021). Previous studies have observed increased emissions of N2O and CO2 and uptake of CH4 during soil FTCs both in field investigations and laboratory incubations (Goldberg et al., 2010; Wu et al., 2020). For instance, researchers have reported that more than 50–70% of the annual cumulative N2O emissions may originate from FTCs (Goldberg et al., 2010; Wu et al., 2014a). Several mechanisms can potentially explain the release of soil GHG fluxes during FTCs: the release of previously generated gases from the unfrozen parts of the soil during soil thawing (Congreves et al., 2018), the increased available carbon and nitrogen substrates (de Bruijn et al., 2009) and the changes in the microbial community during FTCs (Hu et al., 2015). However, the contribution of the mechanisms to the soil GHG exchanges is not well quantified (Congreves et al., 2018).
Grasslands cover nearly 26% of the global land area and account for a large proportion of the global terrestrial carbon and nitrogen pools (Wu et al., 2014a). Grazing is the main anthropogenic management practice applied to grasslands. It has important impacts on various grassland ecological processes, mainly through animal feed intake, excreta deposition and grass trampling (Liu et al., 2021). Field investigations have found that grazing could reduce annual N2O and CO2 emissions and CH4 uptake from grassland soils, which might attribute to the decrease in gas diffusion and the reduction in the microbial biomass, organic carbon and nitrogen contents of the soil, especially for long-term and heavy grazing (Wolf et al., 2010; Chen et al., 2011; Abdalla et al., 2018). Although a large proportion of grasslands worldwide suffered from freeze-thaw events (Holst et al., 2008; Chen et al., 2021), the effects of grazing on the soil-atmosphere exchange of GHGs during FTCs are not well understood.
The semi-arid grasslands of Inner Mongolia cover more than 20% of the total grassland area in China and are representative of the Eurasian grassland belt (Wang et al., 2005). Meadow steppe is one of the local major grassland types, which covers approximately 11% of the Inner Mongolia grassland (Han et al., 2008), and is highly sensitive to climate change and anthropogenic activities (Wu et al., 2020). For example, anthropogenic disturbances, such as over-grazing resulting from the rapid growth in population and food demand in recent decades, may have significantly affected soil carbon and nitrogen turnover, and GHG fluxes. Moreover, previous field studies have shown that soil FTCs are frequently observed in this region from late October to April and have a strong influence on soil–atmosphere GHG exchanges (Holst et al., 2008; Wolf et al., 2010). Although the separate effects of livestock grazing and FTCs on soil GHG fluxes have been well studied, the combined effect of grazing and FTCs on soil GHG fluxes remains uncertain, especially for the meadow steppes of Inner Mongolia.
Therefore, to better understand the effect of livestock grazing on soil GHG fluxes during FTCs, we collected intact soil cores from three paired grazing and enclosed sites in meadow steppes in Inner Mongolia and then conducted three consecutive FTCs. The main objectives of this study were to investigate the effect of livestock grazing on soil GHG fluxes and to reveal the key soil properties that determine GHG fluxes during FTCs. We proposed three hypotheses: (1) soil FTCs will stimulate the emissions of N2O and CO2 and the uptake of CH4 by meadow steppe soils; (2) livestock grazing might reduce the soil-atmosphere exchange of GHGs during FTCs; and (3) grazing and FTCs might have an interactive effect on soil GHG fluxes.
Materials and Methods
Study Area and Soil Sampling
Hulunbuir grassland (47°05′–53°20′ N, 115°31′–126°04′ E) is a typical semi-arid grassland in Inner Mongolia, which is selected as the research area. It is located in the western part of the Greater Khingan Mountains. The topography is relatively flat, with an altitude of 650–700 m above sea level. Chernozem and Kastanozem are the main soil types in this region (Wu et al., 2014b). The study area is dominated by Aneurolepidium chinense, Stipa baicalensis, and Carex korshinskyi. The area experiences a temperate continental monsoon climate, and the mean annual precipitation is 339 mm, of which approximately 60% falls in summer (June to September). The average annual air temperature was –2.2°C from 1980 to 2010, and the intra-annual range was from –25.8°C in January to 19.3°C in July. Due to the low temperature in winter, the topsoil normally begins to freeze in mid-October and thaws in the following April.
In late September 2015, three sites with similar soil type, land use history and plant species were chosen to account for spatial heterogeneity in this region. At each site, plots were established in pairs, that was a long-term free grazing plot and a nearby enclosed plot (ungrazed since 2008). Before fencing in 2008, all sites had been continuously grazed with approximate 1–3 sheep unit hm–1 yr–1 over last decades according to local farmers. The free grazing plots were continually grazed when the soil was sampled. The distance between plots in each site ranged approximately 100–200 m. All the paired plots have the same soil type and similar physiographic conditions, including slope degree, altitude and topography. The average ground covers were about 40 and 85% for long-term grazing and enclosed plots, respectively. The location and the main characteristics of each site are shown in Supplementary Table 1. The dominant vegetation in three enclosed plots was Aneurolepidium chinense. The dominant species in free grazing plots were Carex pediformis and Stipa baicalensis. The average bulk density of 0–10 cm soil depth in enclosed plots and grazing plots was 1.13 g cm–3 and 1.19 g cm–3, respectively. The size of each plot was about 100 m × 100 m, and the internal plant community was investigated by the line transect method. In each plot, we selected three sampling subplots (1 m × 1 m) at a distance of 15 m along a random transect. Within each subplot, the surface litter was carefully collected and then the aboveground biomass was harvested by scissors. Subsequently, the root biomass was collected from 3 sampling points to a depth of 30 cm in the main rooting zone with a 5 cm diameter soil auger. Moreover, three replicate intact soil cores (15 cm inner diameter and 20 cm height) were collected from each sampling plot by PVC tubes (15 cm inner diameter of and 30 cm height, 3 sites × 2 treatments × 3 replicates = 18 in total) after removing surface vegetation and litter. The top 10 cm of the PVC tubes was left empty for the measurements of trace gas fluxes. The tubes were carefully driven into the soil in order to alleviate inside soil compaction. In addition, we also collected 21 intact soil samples (7 measurements with three replicates, Figure 1) in each plot with a soil sampler (5 cm diameter and 10 cm height, 3 sites × 2 treatments × 21 samples = 126 in total) for the continuous analyses of soil chemical and microbial characteristics during FTCs. The 18 intact soil cores and 126 soil samples were stored at a constant temperature of + 4.0°C in laboratory before the freeze-thaw experiments to allow the adaption of soil microbiome to cold temperatures.
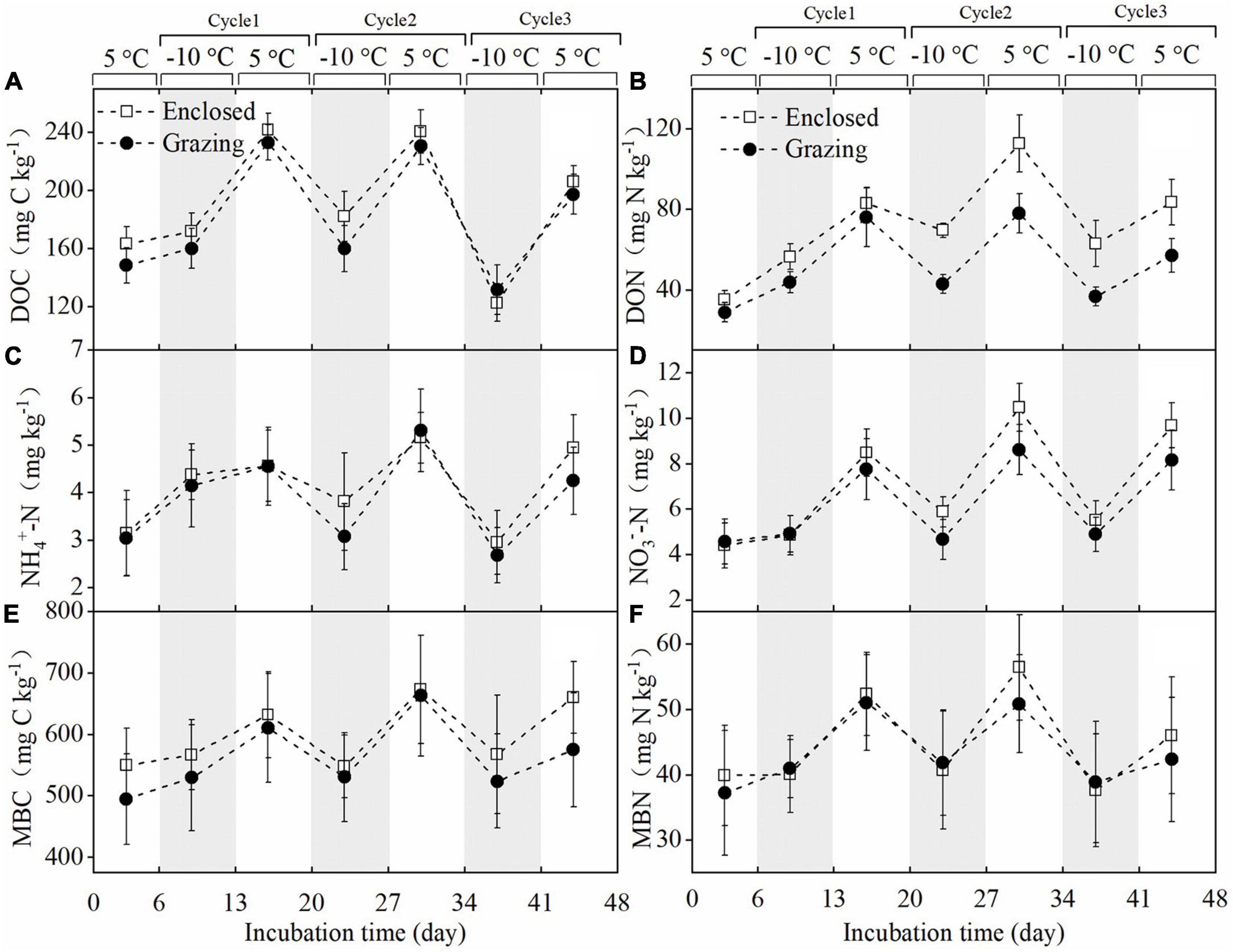
Figure 1. The dynamics of soil DOC (A), DON (B), NH4+-N (C), and NO3–-N (D) contents and microbial biomass C (E) and microbial biomass N (F) during FTCs (mean ± SE, N = 9). The gray areas indicate the freezing periods.
Freeze-Thaw Incubation Experiments
All incubation experiments were conducted in incubators (JYH-412F, Jiayu Instrument Co., Ltd., China), which can periodically adjust the temperature to simulate FTCs. In order to prevent the inside gas accumulation, a pump was used to mix together the inside air and the outside air. The external sidewall and bottom of each PVC tube were covered with 2.0 cm thick insulative material (Armaflex, Armacell GmbH, Münster, Germany) to mitigate the temperature fluctuations from sidewalls and bottoms. We set the beginning temperature at 5.0°C for 5 days to activate the microbes and to ensure stable GHG fluxes. Subsequently, three FTCs were stimulated to investigate the effect of livestock grazing on soil GHG fluxes during sequential FTCs. According to the field observations of the study area, the temperature in each cycle was set to –10°C for 7 days followed by + 5°C for the next 7 days. Using the sensors inserted through the 5 cm depth holes in the soil cores, the soil temperatures were recorded hourly with a data logger. The soil water content for all soil cores and samples was maintained at the same level as in the field. The average water-filled pore space (WFPS) of the grazing and enclosed plots was 41.6 and 47.8%, respectively. Considering that the soil water content would decrease due to evaporation during the experimental period, we weighed the soil cores and samples daily and compensated the lost weight by spraying deionized water on the soil surface.
The parallel incubation was conducted in the same time for the 126 soil samples that were used for the analysis of chemical and microbial characteristics. The concentrations of soil dissolved organic carbon (DOC) and nitrogen (DON), NH4+-N and NO3–-N, microbial biomass carbon (MBC) and nitrogen (MBN) were determined by the destructive harvesting of triplicate soil samples at day 3, 9, 16, 23, 30, 37, and 44 for GHG fluxes measurements throughout the entire incubation period.
Gas and Soil Analysis
Static chamber method was used to determine the soil N2O, CO2 and CH4 fluxes at daily resolution. The PVC tubes were sealed with stainless steel lids to enclose a headspace of about 1.8 L. Each lid was equipped with a circulating fan to completely mix the gas in the headspace and a rubber stopper was used for sampling gases. At 0, 10, 20, 30, and 40 min after the lid was closed, five 10 mL gas samples were collected from the headspace of chamber with gas-tight syringes. The gas samples were analyzed within 8 h using a gas chromatograph (Agilent 7890 A, California, United States). N2O was detected by electron capture detector, and CO2 and CH4 were detected by flame ionization detector. The DN-Ascarite and DN-CO2 methods described by Yao et al. (2010) were utilized for N2O analysis. The fluxes were calculated according to the change rate of the gas concentration in the headspace of enclosed chamber with time. When the valid measurement value of gas concentration was fewer than five times, or significant non-linearity was not detected, linear regression was used to calculate the fluxes. Otherwise, the non-linear model (Ct = k1/k2 + (C0 - k1/k2) × exp(-k2t), where C0 is the N2O concentration at the beginning of the enclosure, and k1 and k2 are the fitting parameters) proposed by Kroon et al. (2008) was used. The cumulative gas fluxes during each FTC and all three FTC periods were then calculated as the sum of daily fluxes.
The soil water content was determined by weight loss method and dried the soil samples at 105°C for 24 h. The soil pH was measured using a pH meter (PHS-25, Shanghai, China) with soil:water ratio at 1:5. The soil TN and SOC contents were determined using an automated C and N analyzer (Elementar, Hanau, Germany). Soil ammonium (NH4+-N) and nitrate (NO3–-N) were extracted from 20 g of fresh soil with 1 M KCl (soil: water = 1:5 w/v) and quantified colorimetrically using a flow injection analyzer (Seal AA3, Norderstedt, Germany). The MBC and MBN contents in the soil samples were measured using the chloroform fumigation-extraction method (Wu et al., 2014a).
Statistical Analyses
All data are presented as the mean and the standard error of the mean unless otherwise stated. The data were tested for normality (Kolmogorov-Smirnov test) and homogeneity (Levene’s test) before analysis. Pearson correlations and analysis of variance (ANOVA) of soil properties and GHG fluxes following the Student-Newman-Keuls (SNK) test were performed in SPSS 21 (IBM, Armonk, NY, United States). One-way ANOVA was performed to examine the effect of FTCs on the basis of the soil properties before and after the complete FTC experiment. Two-way ANOVA was conducted to test the effect of grazing, FTCs and their interaction on the fluxes of the three gases. In addition, one-way ANOVA was used to test the effect of grazing on the total cumulative fluxes of the three gases during the FTC experiment. Differences were considered statistically significant at P < 0.05. Scatter diagrams and bar plots were generated in OriginPro 2018 (Origin Lab Corporation, United States). The joint statistical analysis of the GHG fluxes and soil properties in the form of scatterplots and structural equation modeling was matched according to the same sampling time. The scatterplot matrix was mapped with scatterplotMatrix function in the “car” package using R3.4.3. Structural equation modeling (SEM) was used to examine the direct and indirect effects of grazing, FTCs and soil properties on the daily GHG fluxes. The structural equation models were constructed and analyzed using R3.4.3 with the sem.fit and sem.lavaan functions in the “lavaan” and “piecewiseSEM” packages.
Results
Effect of Freeze-Thaw Cycles and Grazing on Plant and Soil Characteristics
The aboveground, litter and belowground biomass were significantly lower in the grazing treatments than in the enclosed treatments, but the soil bulk density in the grazing treatments was significantly higher (Supplementary Table 1). There was no significant difference in pH between the grazing and enclosed treatments. Soil DOC and DON were significantly lower (P < 0.05) in grazing treatments than those in enclosed treatments before the FTCs (Supplementary Table 2). However, the NH4+-N, NO3–-N, MBC, and MBN contents showed no significant differences between grazing and enclosed treatments before the FTCs. As shown in Figure 1, in the first FTC, the contents of DOC, DON, NH4+-N, NO3–-N, MBC, and MBN increased in both the freezing and subsequent thawing periods compared to those in the pre-incubation period, whereas in the second and third FTCs, the contents of the above soil chemicals declined during soil freezing but increased in the following thawing period. In general, after three consecutive FTCs, the contents of DOC, DON, NH4+-N, and NO3–-N were significantly increased in both the grazing and enclosed treatments (Table 1). The contents of MBC and MBN increased only slightly after three FTCs compared with those at the beginning of the experiment, and the changes were not significant.
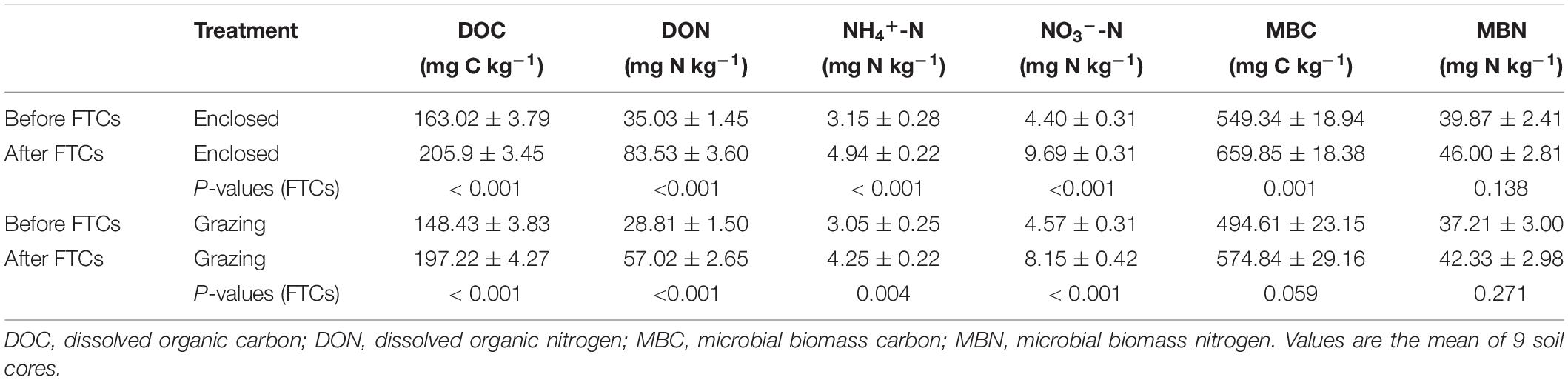
Table 1. One-way ANOVA of the effect of FTCs on soil characteristics and microbial biomass carbon and nitrogen.
Dynamics of N2O Flux During Freeze-Thaw Cycles and the Impact of Grazing
The daily N2O flux ranged from 0.10 to 114.87 μg N m–2 h–1 in the grazing treatments and from 1.04 to 127.80 μg N m–2 h–1 in the enclosed treatments during the whole incubation period (Figure 2). In the freezing periods, the N2O emissions were reduced compared to those in the pre-incubation period, and N2O emissions were substantially promoted in the three thawing periods. By comparison, the peak N2O emission value was relatively low in the first thawing period, was highest in the second thawing period and was lower in the third thawing period. In addition, during the three thawing periods, the daily N2O flux was lower in the grazing treatments than in the enclosed treatments. After three FTCs, grazing significantly reduced the total cumulative N2O flux by 13.3% (average 23.60 mg N m–2 in the grazing treatments and average 27.22 mg N m–2 in the enclosed treatments) (P = 0.020, Figure 3). Two-way ANOVA (Table 2) showed that FTCs had a significant effect on the daily N2O flux (P < 0.001); however, the effect of grazing was not significant (P = 0.284), nor was the interaction between grazing and FTCs (P = 0.512).
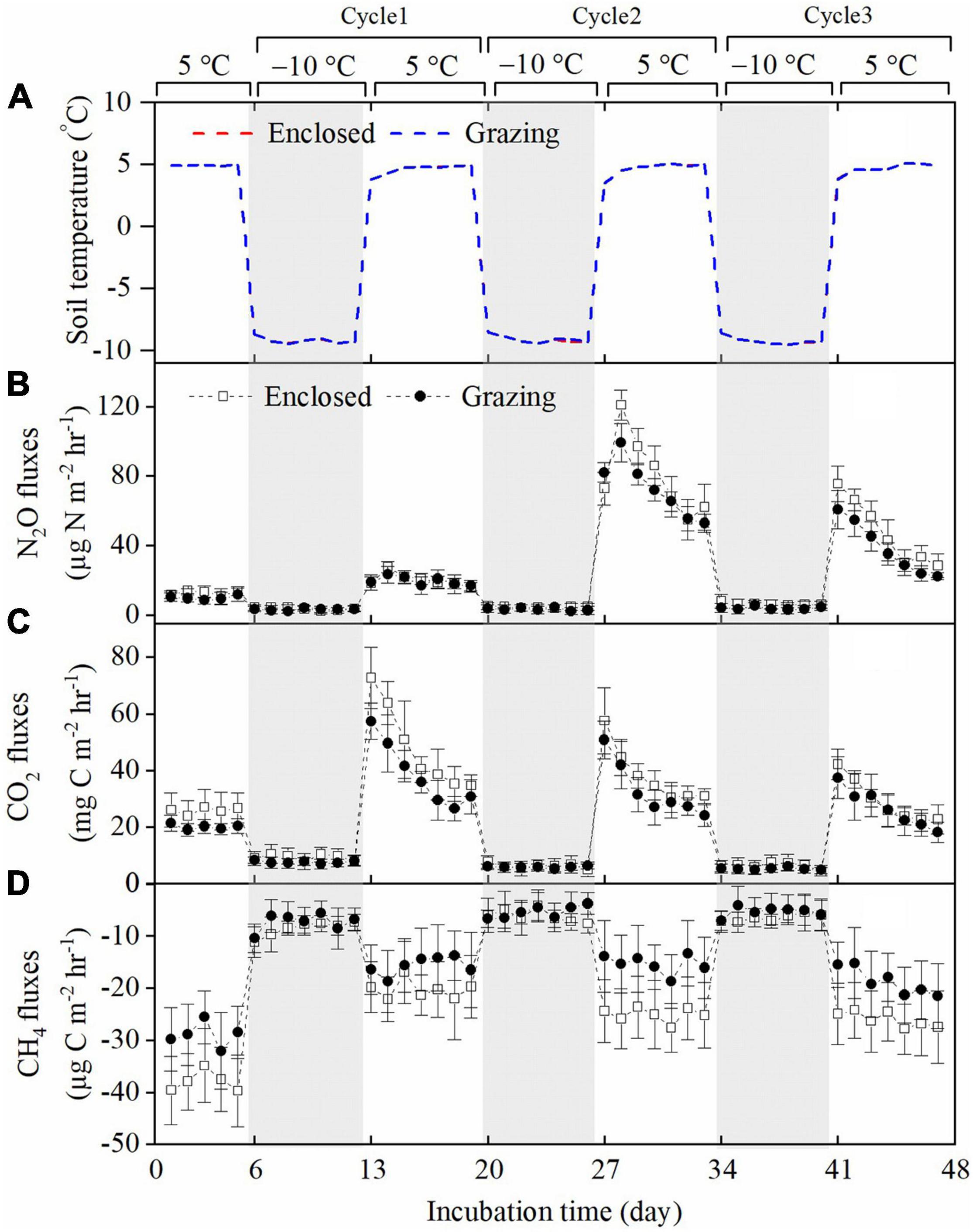
Figure 2. The dynamics of soil temperature (A), N2O flux (B), CO2 flux (C) and CH4 flux (D) during three FTCs (mean ± SE, N = 9). The gray areas indicate the freezing periods.
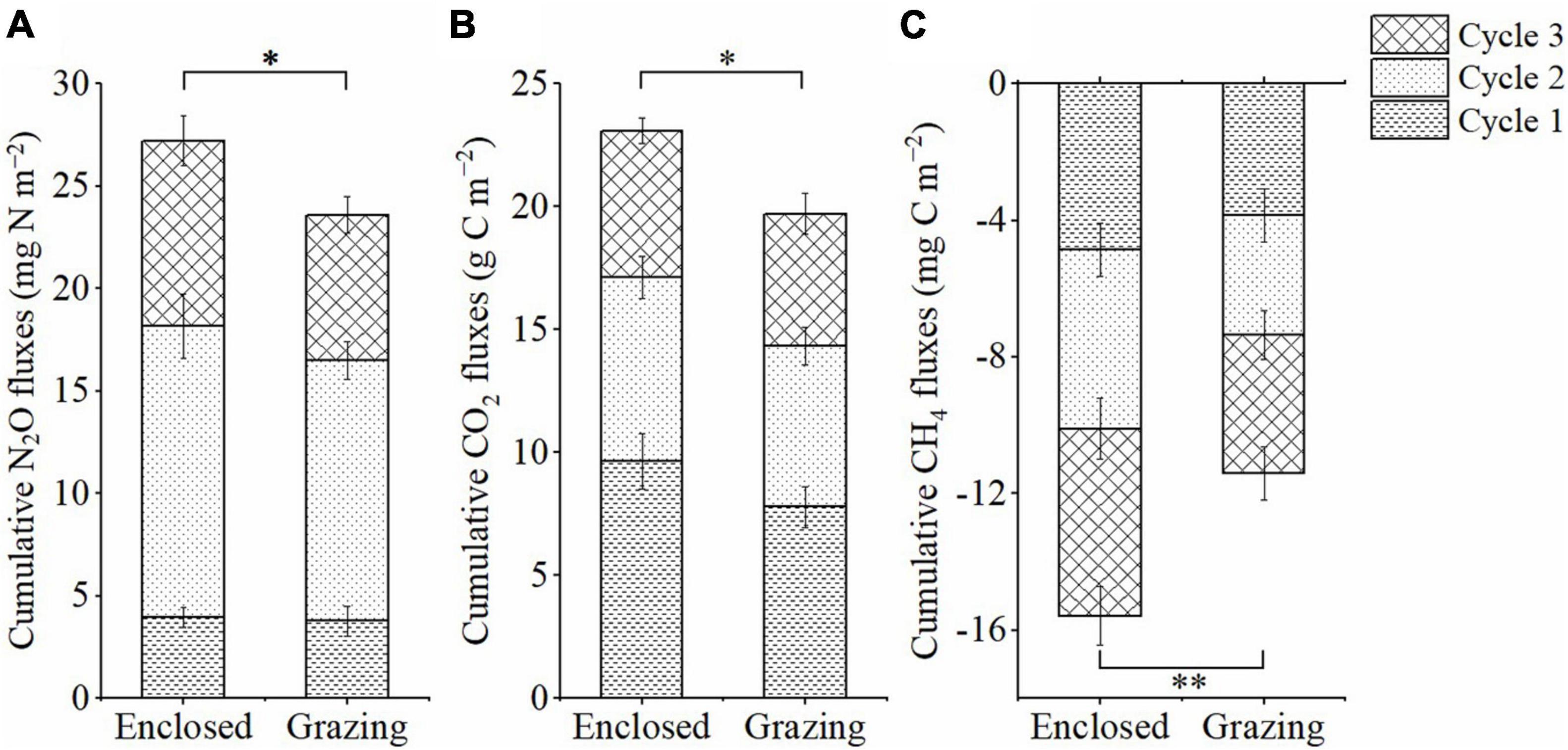
Figure 3. Total cumulative fluxes of N2O (A), CO2 (B) and CH4 (C) during three FTCs (mean ± SE, N = 9). Symbol “*” indicate significant differences (P < 0.05) and “**” indicate notable significant differences (P < 0.01) between grazing and enclosed treatments.
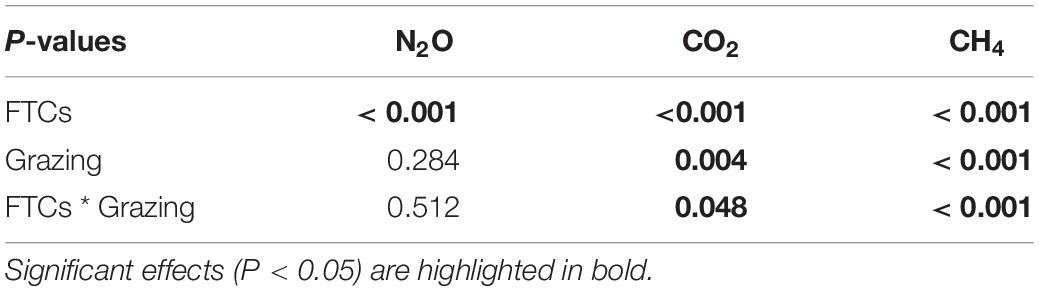
Table 2. Two-way ANOVA of the effects of FTCs, grazing and their interaction on soil N2O, CO2 and CH4 fluxes during FTCs.
Dynamics of CO2 Flux During Freeze-Thaw Cycles and the Impact of Grazing
The range of the daily CO2 flux was 3.03∼68.72 mg C m–2 h–1 in the grazing treatments throughout the incubation period; among the enclosed treatments, the range was 2.07∼91.48 mg C m–2 h–1 (Figure 2). Similarly, the daily CO2 flux was quite low in frozen soils (< 15 mg C m–2 h–1) but significantly increased during thawing periods. The peak value of CO2 emissions occurred in the first thawing period, and CO2 emissions gradually declined with the sequence of FTCs. The daily CO2 flux was continuously lower in the grazing treatments than in the enclosed treatments in the three thawing periods. Grazing significantly reduced the total cumulative CO2 flux (by 14.6%), which was 19.7 g C m–2 in the grazing treatments and 23.09 g C m–2 in the enclosed treatments (P = 0.01, Figure 3). According to the two-way ANOVA (Table 2), FTCs had a significant impact on the daily CO2 exchange (P < 0.001); grazing and the interaction between FTCs and grazing also had significant impacts (P = 0.004, P = 0.048).
Dynamics of CH4 Flux During Freeze-Thaw Cycles and the Impact of Grazing
The flux of CH4 was consistently negative, meaning that CH4 was absorbed by the meadow steppe soil. In the grazing treatments and enclosed treatments, the CH4 flux ranged from –39.90 to –0.04 and from –49.39 to –0.13 μg C m–2 h–1, respectively, during the whole incubation period (Figure 2). The CH4 flux also decreased in freezing periods and increased when the soil thawed, while the peaks of the CH4 uptake rate gradually increased along the sequence of FTCs. The daily CH4 uptake in thawing periods was consistently lower in the grazing treatments than in the exclusion treatments. Consequently, compared to that in the exclusion treatments, the total cumulative CH4 uptake in the gazing treatments was significantly reduced, by 26.8% (11.40 mg C m–2 compared to 15.58 mg C m–2, P < 0.001, Figure 3). Two-way ANOVA indicated that FTCs, livestock grazing and their interaction effect all had significant impacts on CH4 uptake (Table 2, P < 0.001).
Effects of Grazing and Freeze-Thaw Cycles on Soil Properties and Greenhouse Gases Fluxes
In the scatterplot matrix (Figure 4), the correlation coefficients between soil chemical and microbial properties and the daily GHG flux are provided in the upper right corner, while the subfigures in the lower left show the smooth fitting curves between the two variables on the diagonal line. The correlations between soil properties and the daily N2O and CO2 fluxes were significant, but for CH4 uptake, the edaphic effects were not significant.
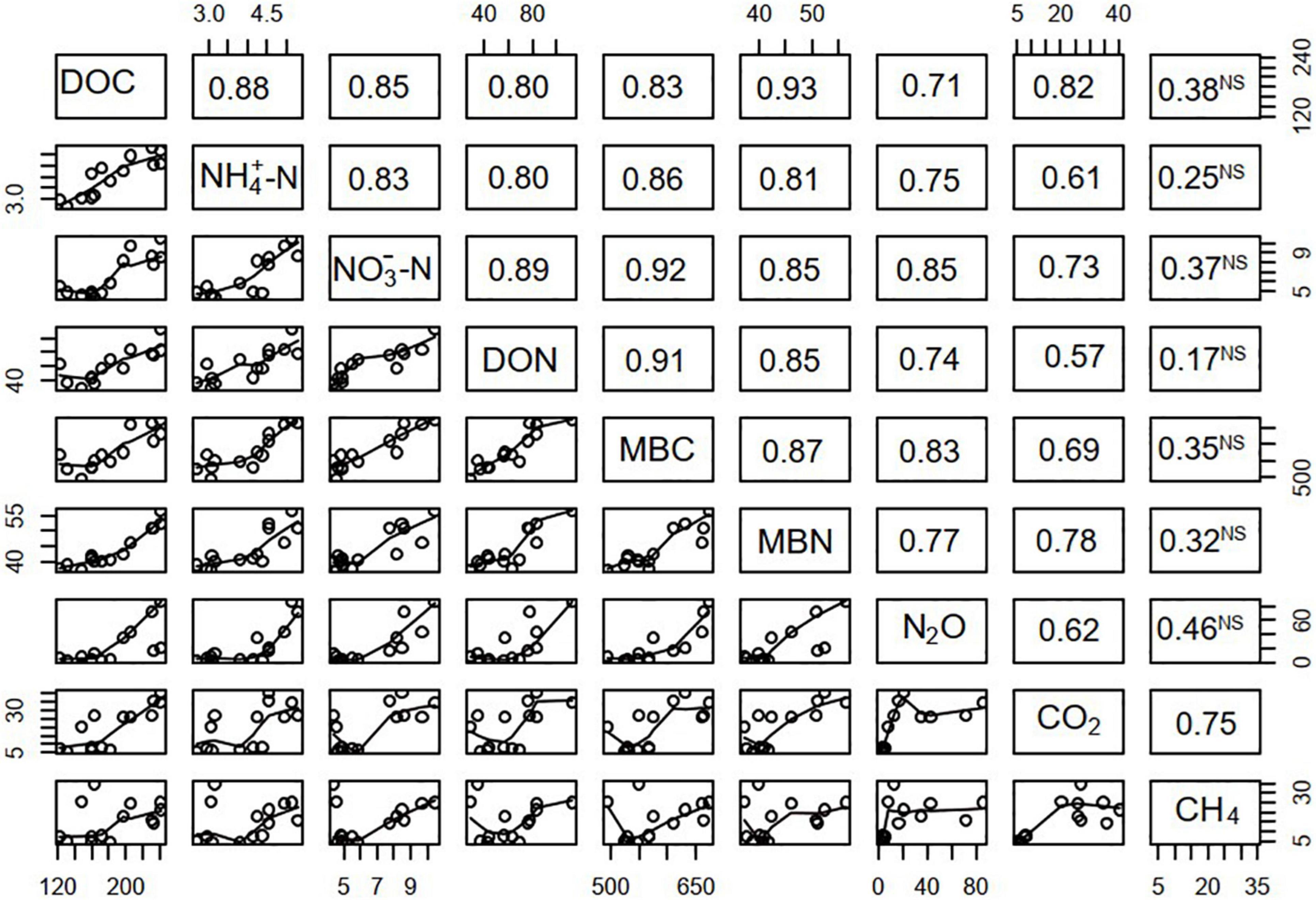
Figure 4. Scatterplot matrix showing the Pearson correlation coefficients between the fluxes of N2O, CO2, and CH4 and soil characteristics during FTCs. The upper right half of the matrix shows the correlation coefficients. The superscript “NS” next to the correlation coefficient indicates non-significance (P ≥ 0.05); unmarked coefficients are significant (P < 0.05). The lower left half shows the scatterplots and smooth curves.
SEM showed that the FTCs had a significant positive effect on soil DOC and NO3–-N (r = 0.64, P < 0.001; r = 0.58, P < 0.001), thus stimulating the N2O flux (Figure 5A), but that grazing had a negative effect on soil DOC, NO3–-N and N2O fluxes. The standardized total effect of NO3–-N was the highest (Figure 5B), which suggested that soil NO3–-N dominated the variance in N2O exchange during the FTCs (r = 0.71, P < 0.001). FTe in grazing plots compCs significantly promoted CO2 emissions by increasing the soil DOC and MBC contents (r = 0.66, P < 0.001; r = 0.35, P < 0.001), while grazing had negative effects on CO2 emissions (Figure 5C). DOC and MBC were positive influencing factors on CO2 emissions (r = 0.28, P < 0.001; r = 0.25, P < 0.001), with DOC playing a more important role (Figure 5D). However, for CH4 uptake, the fitness index (RMSEA = 0.192) failed to meet the requirements of SEM (RMSEA < 0.08), indicating that the proposed soil chemical factors could not explain the variation in CH4 fluxes during FTCs (Figures 5E,F).
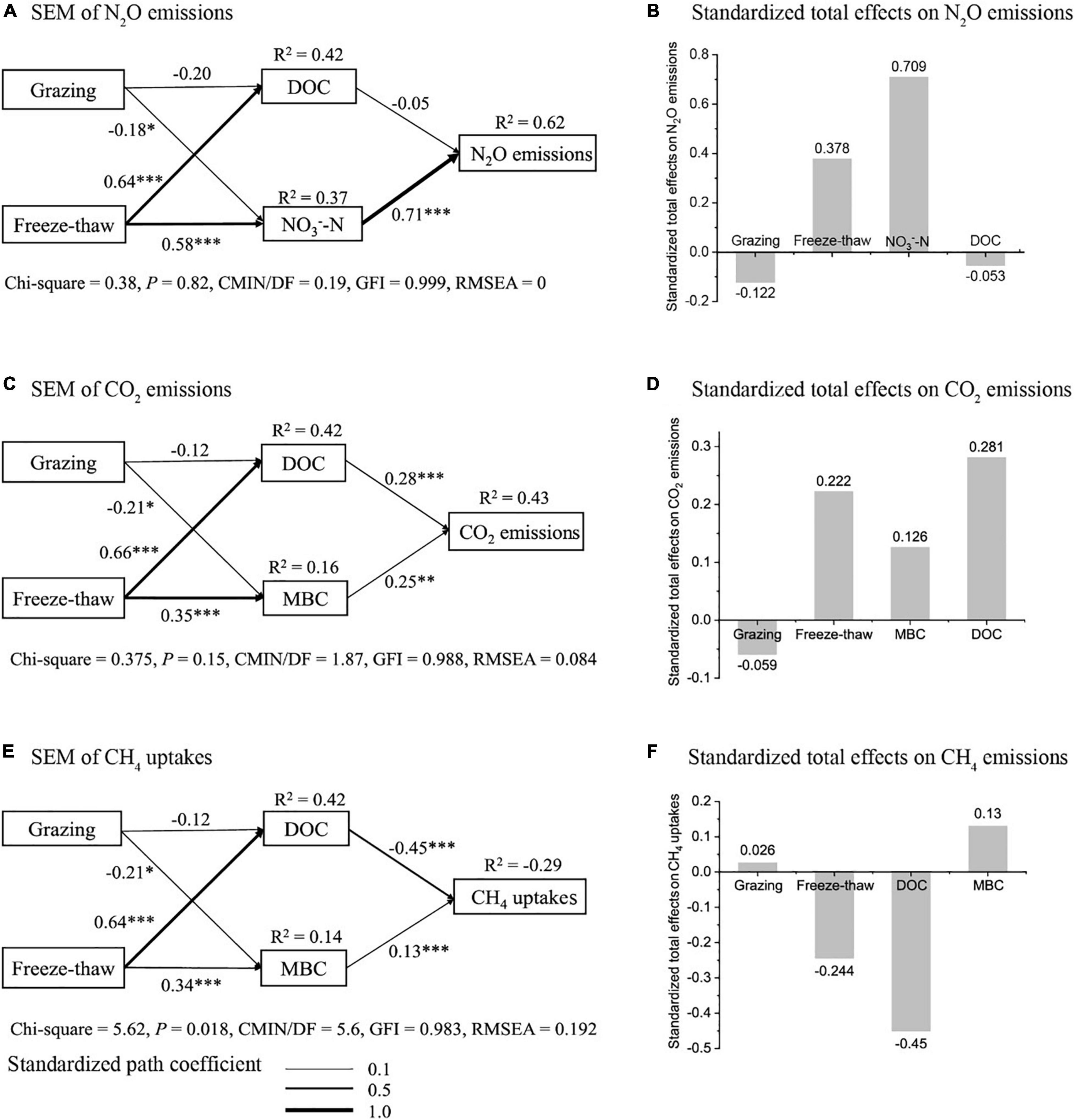
Figure 5. Structural equation modeling (SEM) showing the effects of the combination of grazing and FTCs on soil chemical properties and GHG fluxes (sample size = 126). (A) SEM of N2O emissions, (B) standardized total effects on N2O emissions, (C) SEM of CO2 emissions, (D) standardized total effects on CO2 emissions, (E) SEM of CH4 uptake, (F) standardized total effects on CH4 uptake. The numbers on the arrows are the standardized path coefficients; a positive value represents a positive influence, and a negative value represents a negative influence. The “*” next to the path coefficient indicates the significant level of the path coefficient, *P < 0.05; **P < 0.01, ***P < 0.001. R2 indicates the explanation degree of response variables.
Discussion
Effect of Grazing and Freeze-Thaw Cycles on N2O Fluxes
Our study detected the stimulation of soil N2O emissions during FTCs, which is consistent with the results of previous studies in various ecosystems, including grasslands (Wang et al., 2005; Yao et al., 2010), wetlands (Wang et al., 2013) and forests (Goldberg et al., 2010). The substantial enhancement of N2O emissions following soil thawing in the meadow steppe can be basically explained by several physical factors. First, the expansion of the WFPS leads to increased anaerobic volume, thus favoring N2O production through denitrification (Bollmann and Conrad, 1998; Yin et al., 2020). Second, it has been well documented that the N2O emission rate rises exponentially with soil temperature (Yao et al., 2010; Smith et al., 2018). In addition, when soil thaws, the substrate accumulated during the previous freezing period may activate N2O-producing soil microbes (Chen et al., 2021), which can be justified by the increased DOC and NO3–-N content induced by FTCs and the strong positive correlation between NO3–-N content and N2O emissions during FTCs. However, SEM showed that DOC had a weak negative effect on N2O emissions. Denitrification normally starts to occur at 60% WFPS and higher (Wu et al., 2020), thus leading to N2O emissions. DOC, as the carbon source for heterotrophic denitrifying bacteria, is widely considered to have a positive effect on N2O emissions when the denitrification process is dominant (Khalil et al., 2004). In this study, under WFPS levels from 41.6 to 47.8%, nitrification and denitrification may occur simultaneously and lead to N2O release; therefore, DOC did not show a significant effect on N2O emissions. Taken together, physical mechanisms and the variations in substrate supply are predominantly responsible for the increased N2O emissions during soil FTCs (Kim et al., 2012).
The total cumulative N2O emissions were significantly lower in the grazing treatments than in the enclosed treatments during FTCs. As long-term grazing will reduce the aboveground vegetation biomass, the resulting lower litter input into the soil will reduce soil nitrogen availability (Liu et al., 2015). Moreover, it has been revealed that long-term grazing will lead to NO3–-N leaching from soil more readily (Steffens et al., 2008). The lack of substrate may limit soil microbial nitrification and denitrification, thus reducing N2O exchange. This inference can be justified by the SEM result showing that grazing had a negative effect on N2O emissions through the mediating effect of DOC and NO3–-N. In addition, livestock grazing can increase the soil bulk density (Steffens et al., 2008), as confirmed in this study. Consequently, the relatively limited soil aeration may result in the reduction of N2O emissions under grazing conditions (Smith et al., 2018).
Effect of Grazing and Freeze-Thaw Cycles on CO2 Fluxes
In this study, the soil respiration rates were also markedly enhanced during FTCs, which agreed with the findings of previous studies on grasslands (Chen et al., 2019; Wu et al., 2020). The soil CO2 emissions were low in the freezing periods and high in the subsequent thawing periods. A strong positive correlation (r = 0.81) was observed because the soil microbial respiration rate is driven mainly by soil temperature (Wu et al., 2014a). In addition, during freezing periods, soil aggregates are covered by a thin film of ice that reduces gas diffusivity (Kim et al., 2012; Congreves et al., 2018) and thereby inhibits soil respiration. Upon soil thawing, the release of accumulated substrates can substantially stimulate microbial respiration (Wu et al., 2020). Correlation analysis and SEM revealed the significant impacts of soil DOC and MBC contents on CO2 fluxes during FTCs. Therefore, the physical conditions and increased DOC and MBC contents may explain the rising CO2 emissions during FTCs in meadow steppe soils (Kim et al., 2012; Wu et al., 2014a).
Similar to previous studies on meadow steppe soils (Wu et al., 2020), we found reduced total cumulative CO2 emissions in grazing treatments compared with those in enclosed plots during soil FTCs. One potential reason for this finding is that grazing had significantly reduced the aboveground and underground biomass (Supplementary Table 1), which in turn would cause a decline in soil labile carbon content as revealed by our results (Supplementary Table 2). These will reduce substrate availability for soil heterotrophic respiration (Yu et al., 2019). According to a global meta-analysis, heavy grazing leads to a 10.3% decline in soil organic carbon (Tang et al., 2019). SEM also confirmed that grazing can reduce CO2 emissions by negatively affecting soil DOC and MBC. Another possible reason could be the lower soil moisture in grazing plots compared to those in enclosed plots. It is well documented that the grazing-induced decrease in soil moisture could inhibit microbial activities, leading to reduced soil CO2 emissions (Savadogo et al., 2007; Tian et al., 2016).
Effect of Grazing and Freeze-Thaw Cycles on CH4 Fluxes
The soil CH4 flux was consistently negative during FTCs, confirming a previous finding that meadow steppes function predominantly as a sink for atmospheric CH4 (Chen et al., 2011). Similar to the CO2 and N2O fluxes, the soil CH4 flux was low during soil freezing and high during soil thawing, which was also observed in previous studies (Wu et al., 2014a). Soil-atmosphere CH4 exchange is controlled by two opposite biological processes: methane oxidation induced by methanotrophs and methanogenesis induced by methanogens (Holst et al., 2008). However, because methanogens live mainly under anaerobic conditions, methanogenesis was considered negligible in this study. The CH4 exchange in frozen soil was restricted mainly by low gas diffusivity, substrate availability and soil microbial enzyme activity (Chen et al., 2011; Smith et al., 2018). During soil thawing, the rising temperature and increased soil water availability have a strong stimulatory effect on CH4-oxidizing microbes, therefore increasing CH4 uptake in meadow steppes (Wu et al., 2010). The correlation analysis did not find a significant correlation between the contents of DOC, DON, MBC, or MBN and CH4 fluxes during FTCs in our study area. Therefore, we recommend further research to identify additional potential factors that may drive microbial processes related to soil CH4 oxidation during FTCs.
Compared to that in the enclosed treatments, the total cumulative CH4 uptake in the grazing treatments was significantly lower during the three FTCs. This may be partly attributed to the lower soil gas diffusivity caused by animal trampling, since the availability of substrate gases (O2 and CH4) is essential for the soil microbial CH4 oxidation process (Liu et al., 2007). Moreover, the biomass of CH4-oxidizing bacteria may be lower in long-term livestock grazing sites due to the reduced carbon inputs from plants (Tracy and Frank, 1998). Considering that many soil characteristics can be markedly changed by grazing through herbivore species selection, plant rhizosphere exudation and animal excreta deposition (Clegg, 2006) but that only a few soil characteristics were measured in this study, the potential causes of the difference in CH4 uptake between grazed and enclosed grasslands during FTCs have not yet been fully explained.
Conclusion
The results of our incubation experiments demonstrated that livestock grazing and soil FTCs had significant effects on soil-atmosphere exchange of GHGs in meadow steppes in Inner Mongolia. Although soil FTCs could substantially stimulate soil N2O and CO2 emissions and CH4 uptake, long-term grazing significantly reduced the cumulative N2O, CO2 and CH4 fluxes by 13.3, 14.6, and 26.8%, respectively, during the entire experiment. SEM revealed that the increase in NO3–-N induced by FTCs dominated the variance in N2O emissions and that DOC strongly affected CO2 emissions during thawing periods. These results suggest that the increase in substrate availability induced by FTCs can largely explain the increase in GHG fluxes. Moreover, long-term grazing reduced soil substrate availability and microbial activity and increased soil bulk density, which in turn decreased the cumulative GHG fluxes during FTCs. Therefore, our results suggested that the combined effect of grazing and FTCs should be taken into account for accurately estimating regional GHG budgets in meadow steppes.
Data Availability Statement
The original contributions presented in the study are included in the article/Supplementary Material, further inquiries can be directed to the corresponding author/s.
Author Contributions
FW, YC, and XW conceptualized this study and led the writing. TL, CW, and DW collected and analyzed the data. BF, YL, and XW interpreted the results and revised the text. All authors contributed to this work and approved the final manuscript before submission.
Funding
This study was supported by the National Natural Science Foundation of China (41773070 and 42041005), the Second Tibetan Plateau Scientific Expedition and Research Program (2019QZKK0308), and the Youth Innovation Promotion Association of the Chinese Academy of Sciences (2018056).
Conflict of Interest
The authors declare that the research was conducted in the absence of any commercial or financial relationships that could be construed as a potential conflict of interest.
Publisher’s Note
All claims expressed in this article are solely those of the authors and do not necessarily represent those of their affiliated organizations, or those of the publisher, the editors and the reviewers. Any product that may be evaluated in this article, or claim that may be made by its manufacturer, is not guaranteed or endorsed by the publisher.
Acknowledgments
We would like to thank the editor and the reviewers for valuable suggestions and comments to improve the previous version of the manuscript.
Supplementary Material
The Supplementary Material for this article can be found online at: https://www.frontiersin.org/articles/10.3389/fevo.2021.795203/full#supplementary-material
References
Abdalla, M., Hastings, A., Chadwick, D. R., Jones, D. L., Evans, C. D., Jones, M. B., et al. (2018). Critical review of the impacts of grazing intensity on soil organic carbon storage and other soil quality indicators in extensively managed grasslands. Agric. Ecosyst. Environ. 253, 62–81. doi: 10.1016/j.agee.2017.10.023
Bollmann, A., and Conrad, R. (1998). Influence of O2 availability on NO and N2O release by nitrification and denitrification in soils. Glob. Change Biol. 4, 387–396. doi: 10.1046/j.1365-2486.1998.00161.x
Chen, W., Wolf, B., Zheng, X., Yao, Z., Butterbach-Bahl, K., Brueggemann, N., et al. (2011). Annual methane uptake by temperate semiarid steppes as regulated by stocking rates, aboveground plant biomass and topsoil air permeability. Glob. Change Biol. 17, 2803–2816. doi: 10.1111/j.1365-2486.2011.02444.x
Chen, W., Zheng, X., Wolf, B., Yao, Z., Liu, C., Butterbach-Bahl, K., et al. (2019). Long-term grazing effects on soil-atmosphere exchanges of CO2, CH4 and N2O at different grasslands in Inner Mongolia: a soil core study. Ecol. Indic. 105, 316–328. doi: 10.1016/j.ecolind.2017.09.035
Chen, Z., Ge, S., Zhang, Z., Du, Y., Yao, B., Xie, H., et al. (2021). Soil moisture but not warming dominates nitrous oxide emissions during freeze–thaw cycles in a Qinghai–Tibetan Plateau alpine meadow with discontinuous permafrost. Front. Ecol. Evol. 9:676027. doi: 10.3389/fevo.2021.676027
Clegg, C. D. (2006). Impact of cattle grazing and inorganic fertiliser additions to managed grasslands on the microbial community composition of soils. Appl. Soil Ecol. 31, 73–82. doi: 10.1016/j.apsoil.2005.04.003
Congreves, K. A., Wagner-Riddle, C., Si, B. C., and Clough, T. J. (2018). Nitrous oxide emissions and biogeochemical responses to soil freezing-thawing and drying-wetting. Soil Biol. Biochem. 117, 5–15. doi: 10.1016/j.soilbio.2017.10.040
de Bruijn, A. M. G., Butterbach-Bahl, K., Blagodatsky, S., and Grote, R. (2009). Model evaluation of different mechanisms driving freeze-thaw N2O emissions. Agric. Ecosyst. Environ. 133, 196–207. doi: 10.1016/j.agee.2009.04.023
Goldberg, S. D., Borken, W., and Gebauer, G. (2010). N2O emission in a norway spruce forest due to soil frost: concentration and isotope profiles shed a new light on an old story. Biogeochemistry 97, 21–30. doi: 10.1007/s10533-009-9294-z
Gu, B., van Grinsven, H. J. M., Lam, S. K., Oenema, O., Sutton, M. A., Mosier, A., et al. (2021). A credit system to solve agricultural nitrogen pollution. Innovation 2:100079. doi: 10.1016/j.xinn.2021.100079
Han, G., Hao, X., Zhao, M., Wang, M., Ellert, B. H., Willms, W., et al. (2008). Effect of grazing intensity on carbon and nitrogen in soil and vegetation in a meadow steppe in Inner Mongolia. Agric. Ecosyst. Environ. 125, 21–32. doi: 10.1016/j.agee.2007.11.009
Holst, J., Liu, C., Yao, Z., Brueggemann, N., Zheng, X., Giese, M., et al. (2008). Fluxes of nitrous oxide, methane and carbon dioxide during freezing-thawing cycles in an Inner Mongolian steppe. Plant Soil. 308, 105–117. doi: 10.1007/s11104-008-9610-8
Hu, H. W., Chen, D. L., and He, J. Z. (2015). Microbial regulation of terrestrial nitrous oxide formation: understanding the biological pathways for prediction of emission rates. Fems Microbiol. Rev. 39, 729–749. doi: 10.1093/femsre/fuv021
Jalaludin, B., Johnston, F., Vardoulakis, S., and Morgan, G. (2020). Reflections on the catastrophic 2019–2020 Australian bushfires. Innovation 1:100010. doi: 10.1016/j.xinn.2020.04.010
Khalil, K., Mary, B., and Renault, P. (2004). Nitrous oxide production by nitrification and denitrification in soil aggregates as affected by O2 concentration. Soil Biol. Biochem. 36, 687–699. doi: 10.1016/j.soilbio.2004.01.004
Kim, D. G., Vargas, R., Bond-Lamberty, B., and Turetsky, M. R. (2012). Effects of soil rewetting and thawing on soil gas fluxes: a review of current literature and suggestions for future research. Biogeosciences 9, 2459–2483. doi: 10.5194/bg-9-2459-2012
Kostyanovsky, K. I., Huggins, D. R., Stockle, C. O., Morrow, J. G., and Madsen, I. J. (2019). Emissions of N2O and CO2 following short-term water and N fertilization events in wheat-based cropping systems. Front. Ecol. Evol. 7:63. doi: 10.3389/fevo.2019.00063
Kroon, P. S., Hensen, A., van den Bulk, W. C. M., Jongejan, P. A. C., and Vermeulen, A. T. (2008). The importance of reducing the systematic error due to non-linearity in N2O flux measurements by static chambers. Nutr. Cycl. Agroecosyst. 82, 175–186. doi: 10.1007/s10705-008-9179-x
Li, T., Chen, Y., Han, L., Cheng, L., Lv, Y., Fu, B., et al. (2021). Shortened duration and reduced area of frozen soil in the Northern Hemisphere. Innovation 2:100146. doi: 10.1016/j.xinn.2021.100146
Liu, C., Holst, J., Brueggemann, N., Butterbach-Bahl, K., Yao, Z., Yue, J., et al. (2007). Winter-grazing reduces methane uptake by soils of a typical semi-arid steppe in Inner Mongolia, China. Atmos. Environ. 41, 5948–5958. doi: 10.1016/j.atmosenv.2007.03.017
Liu, N., Kan, H. M., Yang, G. W., and Zhang, Y. J. (2015). Changes in plant, soil, and microbes in a typical steppe from simulated grazing: explaining potential change in soil C. Ecol. Monogr. 85, 269–286. doi: 10.1890/14-1368.1
Liu, Y., Liu, S., Wang, F., Sun, Y., Li, M., Wang, Q., et al. (2021). Responses of habitat quality and animal biodiversity to grazing activities on the Qinghai-Tibet Plateau. Front. Ecol. Evol. 9:681775. doi: 10.3389/fevo.2021.681775
Savadogo, P., Sawadogo, L., and Tiveau, D. (2007). Effects of grazing intensity and prescribed fire on soil physical and hydrological properties and pasture yield in the savanna woodlands of Burkina Faso. Agric. Ecosyst Environ. 118, 80–92. doi: 10.1016/j.agee.2006.05.002
Smith, K. A., Ball, T., Conen, F., Dobbie, K. E., Massheder, J., and Rey, A. (2018). Exchange of greenhouse gases between soil and atmosphere: interactions of soil physical factors and biological processes. Eur. J. Soil Sci. 69, 10–20. doi: 10.1111/ejss.12539
Steffens, M., Kolbl, A., Totsche, K. U., and Kogel-Knabner, I. (2008). Grazing effects on soil chemical and physical properties in a semiarid steppe of Inner Mongolia (P.R. China). Geoderma 143, 63–72. doi: 10.1016/j.geoderma.2007.09.004
Tang, S. M., Wang, K., Xiang, Y. Z., Tian, D. S., Wang, J. S., Liu, Y. S., et al. (2019). Heavy grazing reduces grassland soil greenhouse gas fluxes: a global meta-analysis. Sci. Total Environ. 654, 1218–1224. doi: 10.1016/j.scitotenv.2018.11.082
Tian, D. S., Niu, S. L., Pan, Q. M., Ren, T. T., Chen, S. P., Bai, Y. F., et al. (2016). Nonlinear responses of ecosystem carbon fluxes and water-use efficiency to nitrogen addition in Inner Mongolia grassland. Funct. Ecol. 30, 490–499. doi: 10.1111/1365-2435.12513
Tracy, B. F., and Frank, D. A. (1998). Herbivore influence on soil microbial biomass and nitrogen mineralization in a northern grassland ecosystem: Yellowstone National Park. Oecologia 114, 556–562. doi: 10.1007/s004420050480
Wagner-Riddle, C., Congreves, K. A., Abalos, D., Berg, A. A., Brown, S. E., Ambadan, J. T., et al. (2017). Globally important nitrous oxide emissions from croplands induced by freeze-thaw cycles. Nat. Geosci. 10, 279–283. doi: 10.1038/ngeo2907
Wang, J. Y., Song, C. C., Miao, Y. Q., and Meng, H. N. (2013). Greenhouse gas emissions from southward transplanted wetlands during freezing-thawing periods in northeast China. Wetlands 33, 1075–1081. doi: 10.1007/s13157-013-0463-4
Wang, Y. S., Xue, M., Zheng, X. H., Ji, B. M., Du, R., and Wang, Y. F. (2005). Effects of environmental factors on N2O emission from and CH4 uptake by the typical grasslands in the Inner Mongolia. Chemosphere 58, 205–215. doi: 10.1016/j.chemosphere.2004.04.043
Wolf, B., Zheng, X. H., Brueggemann, N., Chen, W. W., Dannenmann, M., Han, X. G., et al. (2010). Grazing-induced reduction of natural nitrous oxide release from continental steppe. Nature 464, 881–884. doi: 10.1038/nature08931
Wu, X., Brueggemann, N., Butterbach-Bahl, K., Fu, B., and Liu, G. (2014a). Snow cover and soil moisture controls of freeze-thaw-related soil gas fluxes from a typical semi-arid grassland soil: a laboratory experiment. Biol. Fertil. Soils 50, 295–306. doi: 10.1007/s00374-013-0853-z
Wu, X., Li, Z., Fu, B., Zhou, W., Liu, H., and Liu, G. (2014b). Restoration of ecosystem carbon and nitrogen storage and microbial biomass after grazing exclusion in semi-arid grasslands of Inner Mongolia. Ecol. Eng. 73, 395–403. doi: 10.1016/j.ecoleng.2014.09.077
Wu, X., Li, T., Wang, D., Wang, F., Fu, B., Liu, G., et al. (2020). Soil properties mediate the freeze-thaw-related soil N2O and CO2 emissions from temperate grasslands. Catena 195:104797. doi: 10.1016/j.catena.2020.104797
Wu, X., Yao, Z., Brueggemann, N., Shen, Z. Y., Wolf, B., Dannenmann, M., et al. (2010). Effects of soil moisture and temperature on CO2 and CH4 soil atmosphere exchange of various land use/cover types in a semi-arid grassland in Inner Mongolia, China. Soil Biol. Biochem. 42, 773–787. doi: 10.1016/j.soilbio.2010.01.013
Yao, Z. S., Wu, X., Wolf, B., Dannenmann, M., Butterbach-Bahl, K., Brueggemann, N., et al. (2010). Soil-atmosphere exchange potential of NO and N2O in different land use types of inner mongolia as affected by soil temperature, soil moisture, freeze-thaw, and drying-wetting events. J. Geophys. Res. Atmos. 115:D17116. doi: 10.1029/2009JD013528
Yin, M. Y., Gao, X. P., Tenuta, M., Li, L., Gui, D. W., Li, X. Y., et al. (2020). Enhancement of N2O emissions by grazing is related to soil physicochemical characteristics rather than nitrifier and denitrifier abundances in alpine grassland. Geoderma 375:114511. doi: 10.1016/j.geoderma.2020.114511
Keywords: grazing, freeze-thaw cycles, nitrous oxide, carbon dioxide, methane, meadow steppe
Citation: Wang F, Chen Y, Li T, Wang C, Wang D, Fu B, Lv Y and Wu X (2021) Grazing Reduces the Soil-Atmosphere Exchange of Greenhouse Gases During Freeze-Thaw Cycles in Meadow Steppes in Inner Mongolia. Front. Ecol. Evol. 9:795203. doi: 10.3389/fevo.2021.795203
Received: 14 October 2021; Accepted: 22 November 2021;
Published: 10 December 2021.
Edited by:
Jianshuang Wu, Institute of Environment and Sustainable Development in Agriculture, Chinese Academy of Agricultural Sciences (CAAS), ChinaReviewed by:
Mousong Wu, Nanjing University, ChinaOlga Gavrichkova, Research Institute on Terrestrial Ecosystems, National Research Council (CNR), Italy
Copyright © 2021 Wang, Chen, Li, Wang, Wang, Fu, Lv and Wu. This is an open-access article distributed under the terms of the Creative Commons Attribution License (CC BY). The use, distribution or reproduction in other forums is permitted, provided the original author(s) and the copyright owner(s) are credited and that the original publication in this journal is cited, in accordance with accepted academic practice. No use, distribution or reproduction is permitted which does not comply with these terms.
*Correspondence: Xing Wu, eGluZ3d1QHJjZWVzLmFjLmNu