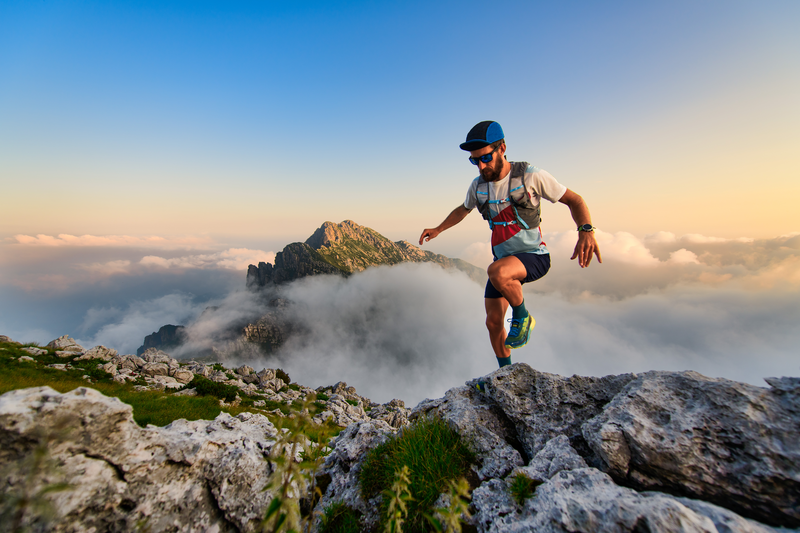
95% of researchers rate our articles as excellent or good
Learn more about the work of our research integrity team to safeguard the quality of each article we publish.
Find out more
ORIGINAL RESEARCH article
Front. Ecol. Evol. , 22 December 2021
Sec. Conservation and Restoration Ecology
Volume 9 - 2021 | https://doi.org/10.3389/fevo.2021.793947
This article is part of the Research Topic Drivers of Small-Mammal Community Structure in Tropical Savannas View all 14 articles
Seed dispersal and predation are critical processes for plant recruitment which can be affected by fire events. We investigated community composition of small mammals in gallery forests with distinct burning histories (burned or not burned ∼3 years before) in the Cerrado (neotropical savanna). We evaluated the role of these animals as seed removers of six native tree species, potentially mediated by the occurrence of fire. We sampled four previously burned sites and four unburned ones. Seed removal was assessed using two exclusion treatments: exclusive access of small rodents and access of all seed-removing vertebrates. The previous burning changed the structural characteristics of the forests, increasing the density of the understory vegetation and herbaceous cover, which determined differences in species composition, richness, and abundance of small rodents (abundance in the burned forests was 1/6 of the abundance in the unburnt ones). Seed removal rates across the six species were reduced in burnt forests in both treatments and were higher for the “all vertebrates” treatment. Other vertebrates, larger than small rodents, played a significant role as seed removers for five of the six species. The effects of fire were consistent across species, but for the two species with the largest seeds (Hymenaea courbaril and Mauritia flexuosa) removal rates for both treatments were extremely low in the burned forests (≦5%). The observed decline in small rodent seed predation in the burned forests may have medium to long-term consequences on plant communities in gallery forests, potentially affecting community composition and species coexistence in these forests. Moreover, fire caused a sharp decline in seed removal by large mammals, indicating that the maintenance of dispersal services provided by these mammals (mainly the agouti Dasyprota azarae) for the large-seeded species may be jeopardized by the burning of gallery forests. This burning would also affect several small mammal species that occur in the surrounding typical savanna habitats but also use these forests. Fire events have been increasing in frequency and intensity because of human activities and climate changing. This current scenario poses a serious threat considering that these forests are fire-sensitive ecosystems within the Cerrado.
Seed dispersal and predation are among the major factors limiting the recruitment of new individuals into plant communities (Schupp, 1988; Wang and Smith, 2002). After leaving the parent tree, seeds are often vulnerable to predation (Mittelbach and Gross, 1984; Hulme, 1993, 1998; Cummings and Alexander, 2002) and high rates of predation can suppress the recruitment, establishment and even population growth of some species (Maron and Kauffman, 2006; Bricker et al., 2010; Zwolak et al., 2010; Pearson et al., 2011) with potential to change the composition of the plant community (Brown and Heske, 1990; Curtin et al., 2000; Harms et al., 2000; Howe and Brown, 2000; Salazar et al., 2012). These changes are affected by the identity of the seed predator, which in some cases may also act as seed disperser (Li and Zhang, 2007; Mittelman et al., 2021). Both dispersal and predation of seeds may also be affected by disturbance events, such as wildfires. Fire events may affect not only the number of available seeds for recruitment but also the abundance of specific faunistic groups that may provide distinct services as seed predators or seed dispersers (Auld and Denham, 2001; Reed et al., 2004; Rusch et al., 2014). Fire-induced changes in the relative services (i.e., seed predation or dispersal) provided by distinct groups of seed-eating animals may alter the probability of establishment of new plant individuals in natural areas. The evaluation of such changes is highly relevant, mainly in fire-prone ecosystems, such as tropical savannas. The understanding of these and other aspects of the ecology of fire is essential for comprehending past or future changes in these ecosystems (Bond and Keeley, 2005).
Patterns of seed dispersal and seed predation produced by mammals can be affected by large-scale disturbances such as wildfires (Reed et al., 2004; Tasker et al., 2011). Fire events rapidly destroy the plant biomass of a region, including the propagules, but also markedly affect the fauna capable of interacting with them (Mutch, 1970; Bendell, 1974; Crowner and Barret, 1979; Catling et al., 1982; Happold, 1983; Simons, 1991). Fires of high severity and extension in forests results in drastic changes such as reduction of vegetation cover and biomass, formation of gaps consequent increasing in edge area, and reduced availability of shelter and food for the animals (Hoffmann and Moreira, 2002; Barlow and Peres, 2006; Legge et al., 2008; Vasconcelos et al., 2009; Diniz et al., 2011). In addition, forest fires also lead to habitat fragmentation by altering the size of the remaining areas and the spacing between them, which may increase rates of local extinction of animal species by reducing fecundity, population size and colonization of species from similar environments (Banks et al., 2011; Lindenmayer et al., 2013). An increasing number of studies point to the susceptibility of mammals from forest environments to the effects of fire (e.g., Converse et al., 2006; Banks et al., 2011; Mendonça et al., 2015; Chia et al., 2016), and this sensitivity is apparently related to the severity of the fire (Legge et al., 2008; Fontaine and Kennedy, 2012; Lindenmayer et al., 2013).
Besides the direct fire influence on involved organisms, there are also indirect effects, in the medium or long term, that burning events can cause on animal-plant interactions. These effects, which may be subtle and often neglected (Dafni et al., 2012), are related to medium- and long-term post-fire changes in the abundance and composition of the faunal assemblage, in the quantity and quality of resources available to the animals, and also in the structural characteristics of the vegetation (Barlow and Peres, 2006; Andersen, 2021; González et al., 2021). Post-fire vegetative cover and the availability of unburnt patches are affected by fire attributes, such as severity and extent of the burned area (Leonard et al., 2014; Tran et al., 2020). These factors, in their turn, alter the abundance and behavior of rodents, ants, and birds, which are the main predators of post-dispersed seeds (e.g., Manson and Stiles, 1998; Monamy and Fox, 2000; Fox et al., 2003; Lassau et al., 2004; Camargo et al., 2018). Some studies have already shown changes in seed predation rates due to the occurrence of fire events (e.g., Reed et al., 2005; Broncano et al., 2008; Tasker et al., 2011; Stuhler and Orrock, 2016).
Fire events might not only change predation rates but also alter the relative role of distinct groups within a guild of seed eaters. In Neotropical environments, small rodents, play a major role in the removal of post-dispersed seeds (Ostfeld et al., 1997; Díaz et al., 1999; Forget et al., 1999; Kelt et al., 2004; Iob and Vieira, 2008; Terborgh, 2012), being generally recognized as primarily seed predators (Vieira et al., 2003, 2006; Galetti et al., 2015b). In contrast, large rodents, such as agoutis, also predate seeds but provide plant dispersal services on a regular basis (Hallwachs, 1986). These distinct roles might be affected by disturbance events. Small seed predators, for instance, might replace larger animals as main seed removers after fire (a similar process is suggested to occur in Atlantic Forest sites defaunated by human disturbance; Galetti et al., 2015a). In case the large animals were the main group to provide real dispersal services, dispersal rates for some plant species could be lower in comparison to pre-fire patterns. Considering that seed establishment and recruitment are critical phases for the plant growth cycle (Hadas, 2005), the indirect effects of wildfires as mediators of the relative role of distinct seed-consumer groups are potentially highly relevant for tree species in fire-prone tropical systems.
Fire episodes occur frequently in areas of Cerrado (Miranda et al., 2002), the largest and biologically richest savanna in the world, with an original extension of about 2,000,000 km2 mainly in central Brazil (Oliveira-Filho and Ratter, 2002). In the Cerrado, fires are historically common, being a relevant ecological and evolutionary factor since at least 4 million years ago (Simon et al., 2009). Recent human action, however, has amplified the frequency of fire and its negative effects on flora, fauna, and water resources (Miranda et al., 2009). Fire events usually extinguishes at the edge of gallery forests (corridors of evergreen forests that typically occur along permanent water courses within the Cerrado; Felfili, 1995; Hoffmann et al., 2012). In extreme drought situations, however, very intense fires can overcome the edges of these forests, reaching their interior (Felfili, 1997; Hoffmann et al., 2009). In these situations, the passage of fire is usually devastating, since forest plant species generally do not possess characteristics that confer greater resistance to fire as those plants present in savanna and grassland phytophysiognomies (Walter and Ribeiro, 2010). This lack of protection causes structural and functional changes in this forest type (Hoffmann et al., 2009; González et al., 2021) and also on its associated fauna (González et al., 2021).
Fire effects on vertebrate fauna are related to animal body size. Medium and large animals generally have a significant post-fire reduction in abundance, which can be attributed to fire-induced changes in vegetation structure (Barlow and Peres, 2006). For small mammals, in areas where fire occurrence is not common, there may also be a reduction in species richness (Ojeda, 1989) or in abundance of some species (Lunney et al., 1987; Simons, 1991; Camargo et al., 2018; González et al., 2021). The small mammals, however, generally are less affected by fire than larger animals, because of their high densities and small body sizes (which allow them to find shelter from fire in small burrows and termite mounds; Vieira, 1999). The effects of fire on small mammals have been studied in the dominant savanna environments (Vieira and Marinho-Filho, 1998; Vieira, 1999; Vieira and Briani, 2013) and in woodland forests in the Cerrado (Mendonça et al., 2015; Camargo et al., 2018). In gallery forests, however, there are very few studies evaluating the effects of burning on small mammals (but see González et al., 2021). Moreover, to the best of our knowledge, the indirect effects of fire on keystone ecological processes occurring in gallery forests, such as seed predation by small mammals, have not been investigated at all.
We investigated small-mammal seed removal of native plant species in gallery forest areas with distinct burning histories (burned or not burned ∼3 years before). We aimed to evaluate the role of these animals as seed removers of six tree species, potentially mediated by the occurrence of fire. We hypothesized that because of the profound effects of fire burned forests have lower overall abundance and distinct species composition of small mammals compared to non-burned forests even after almost 3 years after the last fire event. In relation to seed removal, we expected overall seed removal to be lower in burned forests due to the reduction in abundance of seed-removing mammals. In relative terms, however, we expected that small rodents (<400 g) play a more relevant role as seed removers than large species (e.g., large rodents—agoutis and pacas) in burned forests in comparison to unburned ones, since those larger species would be more adversely affected by fire (Barlow and Peres, 2006).
We conducted our study in the core area of the Cerrado, which is characterized by two well-marked seasons, a cool-dry season and a warm-wet season, which occurs between October and April, when 90% of the annual precipitation of 1,100–1,600 mm occurs (Miranda et al., 1993). Fieldwork was conducted in the Ecological Reserve of the Instituto Brasileiro de Geografia e Estatística (RECOR/IBGE, hereafter IBGE) and in the Fazenda Agua Limpa (FAL), the research station of the Universidade de Brasilia. These two locations and the Ecological Station of the Botanic Garden of Brasília (EEJBB, in Portuguese) are part of the Area of Environmental Protection (APA) Gama e Cabeça-de-Veado, which covers about 15,000 ha of continuous native Cerrado vegetation. Its vegetation includes mainly typical savanna habitats (open woodlands) and grasslands, with gallery forests tracing the courses of streams that cross the landscape. This study was part of the Brazilian Long-term Ecological Research Program of the Brazilian National Research Council (Programa de Pesquisas Ecológicas de Longa Duracão—PELD/CNPq, in Portuguese)—Cerrados from the Central Plateau—Structure, dynamics and ecological processes—Phase 2.
In September 2011, an extensive wildfire occurred in the area, burning about 6,240 ha of native Cerrado vegetation (Mendonça et al., 2015), including 100% of IBGE, about 50% of FAL and 60% of EEJBB (Figure 1). The fire lasted 3 days and severely damaged most of the gallery forests that occurred within the burned area. For evaluating the effects of burning on seed removal patterns in these forests we selected for sampling eight gallery forest sites in the two adjacent conservation areas. Four of which were in gallery forests that burned in the fire in 2011 and four gallery forest areas that did not burn that year (three forests burned in RECOR and one in FAL and four non-burned forests in FAL). The selection of forest sites in relation to fire occurrence was based on observation of satellite images and information of FAL and RECOR employees. The maintenance of an apparent forest structure, even for the forests that had burned, was also a criterion for selecting the sites to be sampled. We sampled all the sites in 2014, 2.8 years after burning. We maintained a straight-line distance between the sites of at least 500 m for assuming independence among them (Figure 1).
Figure 1. Location of the eight sampled sites in gallery forests of the Cerrado (neotropical savanna) in Brazil and extent of the area burned in 2011 (shaded area on the largest map). The green area on the inset map (top left) indicates the area covered by Cerrado in Brazil. Letters ‘a’ to ‘d’ indicate the sites located in the unburned forests (a = Entrada, b = Ponte, c = Capetinga, and d = Represa) and letters ‘e’ to ‘h’ indicate the sites in the previously burned forests (e = Taquara, f = Monjolo, g = Pitoco, and h = Coruja). EEJBB refers to the Ecological Station of the Botanical Garden of Brasília, FAL refers to the Experimental Farm of the University of Brasília, and IBGE refers to the Ecological Reserve of the Brazilian Institute of Geography and Statistics (IBGE, in Portuguese). Adapted from Mendonça et al. (2015).
We experimentally tested removal rates of seeds from six native tree species that occur associated to Cerrado gallery forests of Central Brazil (Felfili et al., 2001). The species names are as follows [average length of the major axis ± SD (n = 30 for each species) and individual seed mass, obtained in this study, are also indicated]: Cariniana estrellensis (Raddi) Kuntze (Lecythidaceae; 1.25 ± 0.15 cm, 0.08 g), Copaifera langsdorffii Desf. (Fabaceae; 1.3 cm ± 0.16, 0.8 g), Dipteryx alata Vogel (Fabaceae; 2.4 ± 0.23 cm, 1.2 g), Platypodium elegans Vogel. (Fabaceae; 2.3 ± 0.18 cm, 1.3 g), Hymenaea courbaril L. var. stilbocarpa (Hayne) Lee and Lang. (Fabaceae; 2.53 ± 0.31 cm, 4.5 g), and Mauritia flexuosa L. f. (Arecaceae; 2.2 ± 0.11 cm, 7.4 g). We selected these species according to their range in size, possible occurrence in gallery forests of the studied region (Felfili et al., 2001; Marimon et al., 2010) and local availability for acquisition.
Fieldwork consisted of the structural characterization of the studied forests (from November to December 2014), capture of small mammals (from August to October 2014), and seed removal experiments (from July to August 2014). Additionally, we evaluated the consumption of seeds by the most abundant rodent species captured in the study sites. This evaluation was based on cafeteria experiments, in which we offered seeds of the six studied plant species to captive individuals.
We established linear transects in each forest site in order to carry out the structural characterization of the studied forests, small mammal trapping, and seed removal experiments. Each of these sampling transects was placed parallel to the forest stream, with 34 experimental stations in each forest site. The stations within the same transect were 15 m apart and the total length of each transect depended on the length of the forest. Whenever necessary, we placed a second transect parallel to the first one and separated at least 20 m from it, maintaining the 15-m distance between stations. The established transects had a minimum distance of ten meters from the border into the forest interior.
For the structural characterization of the studied forests, in each forest site we established a plot of 400 m2 (100 m × 4 m) along the sampling transect. Within this plot, we recorded the diameter at the breast height (DBH = 1.3 m) of all live trees with minimum height of 1.5 m, the number of trees with fire marks and the total number of trees. We also estimated the canopy cover of the forests through photographs taken with a hemispherical 180° (fisheye) lens (FC-E8, Nikon, Japan) coupled in a digital camera (Coolpix 950, Nikon, Japan) placed 1.3 m above ground supported by a tripod. We took one photograph every 15 m (seven photos per site) along the center of the major axis of each transect. We also evaluated understory plant density at each of the seven sampling points by taking four digital photographs (using a Sony® model DSC-HX1 digital camera) 1.2 m above the soil aiming to a white sheet (1.5 by 1.5 m) at a 3-m distance as a background. Each photograph was taken from the sampling point aiming at one of the four cardinal points. We also evaluated ground cover of the herbaceous vegetation using the same camera (mounted on a tripod at a height of 1.3 m) and taking, at each sampling point, four perpendicular photographs aiming to the soil. Each photograph was taken 2 m from the sampling point, in the four cardinal directions. We analyzed the photographs using the ImageJ program (Abramoff et al., 2004) calculating the average percentage of canopy cover and the average percentage of the green area of the photographs (from both understory and forest ground) of each transect.
We evaluated the abundance and composition of local small mammal assemblages by live trapping animals in each of the eight forest sites. In each sampling transect, we placed Sherman® traps (H. B. Sherman Co., Tallahassee, FL, United States) of two sizes alternately on 30 experimental stations [15 “large” (12 cm × 10 cm × 38 cm) and 15 “small” (9 cm × 8 cm × 24 cm) traps] and one wire mesh cage trap (45 cm × 16 cm × 16 cm) in the remaining four stations. We also placed 20 additional wire mesh cage traps at 20 randomly selected intermediate points (at mid-distance between transect stations) along the transect, for a total of 54 traps per site. We set up the traps only on the ground because we intended to capture individuals who used this stratum to search for food. In each site trapping campaign lasted nine consecutive nights.
We baited traps with a mixture of peanut butter, vanilla essence, corn flour, cod liver oil, and mashed banana. Every early morning, we inspected the traps and rebaited them. We identified captured animals by species and then weighed, sexed, ear-tagged (National Band and Tag; model 1005-1), and released them at the point of capture. Some individuals of the most common species were taken to the laboratory for the cafeteria experiments in captivity and released in the same point of capture after that. All field methods were consistent with the animal care guidelines of the American Society of Mammalogists (Sikes et al., 2011).
All seed removal experiments were conducted between July and August of 2014. We evaluated rates of seed removal by distinct fauna groups (small rodents and vertebrate fauna in general) in burned and unburned gallery forests. For that evaluation, we placed seeds on the ground at the experimental stations of the sampled forest sites. Seed trial in each experimental station was submitted to one of the two following treatments. (1) Access of small rodents (<400 g): seeds were surrounded with a semi-permeable exclosure using a wire-mesh cage staked to the ground with one opening at each side along the bottom edge, thus permitting access to small rodents only [SMRO = small rodent access; as in Iob and Vieira (2008); see Supplementary Figure 1 and Supplementary Video 1 for details]. (2) No exclusion: an open control where seeds were placed on the ground without any protection (ALAG = access to all animal groups). For the SMRO treatment we used cages with two different sizes (size #1: 24 cm × 24 cm wide and 10 cm high, mesh = 1.5 cm, side opening with 12 cm wide and 5 cm high; size #2: 40 cm × 40 cm wide and 13 cm high, mesh = 2.5 cm, side opening 12 cm wide and 8 cm high) available at the Laboratory of Vertebrate Ecology of the University of Brasília. The largest size still would not permit access of mammals with more than 700 g (hereafter large mammals), but we maintained the same proportion of both cage sizes for all sampled sites (10 size #1 and 7 size #2 in each site).
Each experimental station received one of the two treatments (SMRO or ALAG) randomly chosen. Thus, of the total of 34 experimental stations per area, 17 stations contained seeds under the treatment SMRO (exclusion cages) and the other 17 contained seeds without cover (ALAG). In all treatments, we placed the seeds in plastic Petri dishes (diameter = 90 mm and height = 15 mm) on the soil. We covered the outer border of the petri dishes with insect-trapping barrier (Tanglefoot®) to limit the access of non-flying insects to the seeds. We checked for the efficiency of the cages by always inspecting the petri dishes for any sign of invertebrates that might be removing the seeds. Only a very few times we found very small ants inside the petri dishes, which were not able to carry the seeds out. At all experimental stations, we removed the litter within a circular area (radius = 0.5 m) around the petri dishes, leaving the soil exposed to facilitate the visualization of fallen seeds outside the dishes.
The available space within the petri dishes did not allow the placement of seeds of the six studied species simultaneously in each experimental station. Because of that, we placed seeds into randomized groups of four species per experimental station. With this procedure, in each site there was a total of 22–24 stations with seeds of each plant species, keeping the same number of stations for each treatment (SMRO or ALAG) per site for each of the six studied plant species. The number of seeds of each species deposited in each experimental station was (from the lowest to the largest seed mass): C. estrellensis—10 seeds, Co. langsdorffii—6 seeds, P. elegans—6 seeds, D. alata—6 seeds, H. courbaril—5 seeds, and M. flexuosa—3 seeds.
We conducted the seed removal experiments by sampling alternately forest sites of the two fire treatments (burned in 2011 or unburned). In each site the experiment was conducted for 10 consecutive days and on the fifth and tenth days we verified the quantity of seeds of each species that had been removed or showed signs of damage. On the 5th day we replaced any removed or damaged seeds with new intact seeds. We considered seeds that were partially or totally eaten by vertebrates and seeds not found in the vicinity of the plates (up to 0.5 m) as “removed.” Thus, we included in our estimates of removal rates also seeds that might have been removed but not predated by the removal agent.
We evaluated how the studied seeds were handled by rodents by conducting experiments on seed offering in captivity (i.e., “cafeteria” type). We performed these experiments between August and October 2014 and used 19 rodent individuals (captured during fieldwork) of the following species (mean body mass ± standard deviation, number of individuals): Oligoryzomys nigripes (22.9 ± 8.3 g, N = 4), Rhipidomys macrurus (42.7 ± 15.5, N = 3), Hylaemys megacephalus (57.0 ± 9.0 g, N = 3), Nectomys squamipes (312.5 ± 47.7 g, N = 6), and Proechimys roberti (307.8 ± 172.7 g, N = 3). We selected these species because they were the most commonly rodents captured in this study and they are all reported as seed or fruit eaters (Paglia et al., 2012; Ribeiro et al., 2019). We avoided using seeds of unripe, old, or infested fruits.
During the period of experimental seed offering in captivity we kept live animals in small individual cages (25 cm × 15 cm × 15 cm or 45 cm × 16 cm × 16 cm). After the seed-offering period (3 days), we released the animals in the same place of capture. During this experiment period, all cages contained water ad libitum and small amounts of alternative food sources (dog chow and banana, in addition to the seeds offered). In this way, we avoided rodent starvation and hypothermia and the animals would not have to obligatorily feed on the offered fruits. The seeds were offered to the animals 24 h after they were placed in the cages and remained for 48 h, so that the animals had time to get used to the captivity and felt enough hunger to search for food.
We provided for the rodents, simultaneously, all seed species used in the field experiments, arranged in plastic petri dishes (90 mm × 15 mm) placed inside the cages, in the following amounts: C. estrellensis—6 seeds; Co. langsdorffii—3 seeds; P. elegans—3 seeds; D. alata—3 seeds; H. courbaril—2 seeds and M. flexuosa—1 seed. At the end of the experiment, we inspected the cages and recorded the quantity and state of the remaining seeds (for signs of predation). We considered as consumed the seeds that were totally or partially (at least 30%) eaten.
We tested the hypothesis that species richness and abundance differ between burning treatments using Mood’s median test (Mood, 1954) mainly because of the limited sample size. This conservative this test has low power compared with alternatives such as ANOVA or Kruskal–Wallis test, but it is robust to heteroscedasticity, non-normality and outliers (Siegel and Castellan, 1988). For this test, we used the number of captured individuals as a proxy for rodent abundance per forest site. The limited number of recaptures per site precluded the use of capture-recapture models for assessing population sizes.
We evaluated potential differences in small-mammal community composition according to previous fire occurrence by performing non-metric multidimensional scaling (NMDS) using Bray–Curtis dissimilarities on the site-by-species matrix. We included the habitat-structure variables as passive variables in the ordination. This approach enabled comparison of previously burned and unburned sites (with similar small-mammal communities appearing close to each other) and the evaluation of their association with the structural habitat variables. We tested for differences in species composition between the two forest groups (unburned and burned) using a Permutational Multivariate Analysis of Variance (PERMANOVA) with 10,000 iterations (Anderson, 2001). These analyses were performed with the Past software (Hammer et al., 2001).
We assessed the occurrence of spatial autocorrelation within our data sets using spatial correlograms of the Moran’s I coefficient with their relative p-values, calculated using Spatial Analysis in Macroecology (SAM; Rangel et al., 2010) and found no significant correlation for any of the six plant species studied (p > 0.28 for all species). We used a generalized linear mixed model (GLMM) with binomial errors to analyze variation in the proportion of seeds removed in response to previous occurrence of fire and exclosure treatment. Although size #2 cages could potentially allow access to rodents of a size slightly larger than size #1, preliminary tests indicated that there was no significant difference in removal rates between cage types (t-test, p ≥ 0.12 for all seed species in comparisons made within each forest site). Thus, for the analysis we pooled the data obtained in these two exclosure sizes, considering them as a single treatment. We considered as predictor variables fire history (fixed effect with two levels, previously burned vs. unburned) and exclosure treatment (fixed effects with two levels, small rodent access—SMRO vs. access to all animal groups—ALAG). Forest sites (nested within fire history) and experimental stations (nested within sites) were considered random effects. We also included species as a random effect (six levels, one for each species included in the analysis), with random intercepts and random slopes. In doing that, we were able to assess whether the effects of treatment were consistent across species. To evaluate species-specific responses to treatments, we also ran separate models for each of the six species (as in Williams et al., 2021). For these analyses, we used the lme4 package (Bates et al., 2014). We tested for overdispersion using the function dispersion_glmer from library blmeco (Korner-Nievergelt et al., 2015). Residuals of all GLMMs were inspected with the “DHARMa” package (Hartig, 2017). No deviations from the specified error distributions were detected for any species. All these analyzes were performed using the software RStudio (RStudio Team, 2020; RStudio: Integrated Development for R. RStudio, PBC, Boston, MA, United States, URL1).
We obtained 153 captures of 104 small mammal individuals distributed in eight species, seven rodents and one marsupial, with a total trapping effort of 3,780 trap nights (not considering malfunctioning traps). The marsupial Gracilinanus agilis was the most abundant species in this study (33.3% of the total number of individuals), followed by the rodent Oecomys sp. (21.6%). Five rodent species were captured only in the unburned forest patches, and none was captured exclusively in the burned patches (Supplementary Table 1). The overall trapping success was 4.05%, with trapping success being 84% lower for the burned forests (1.20%; 23 captures of 15 individuals from three species) in comparison to unburned forests (7.75%; 130 captures of 89 individuals from eight species). The overall abundance of small mammals also was significantly lower in the burned forests than in the unburned ones (Mood’s test for equal medians; χ2 = 4.8, p = 0.028), with the median value for burned sites being 79% lower than the median value for the unburned sites. A similar and significant pattern was observed for species richness (Mood’s test for equal medians; χ2 = 8.0, p = 0.005), with the median values in the burned sites being about 55% lower than in the unburned ones (Figure 2).
Figure 2. Mean small mammal abundance and richness in the sampled gallery forest sites in relation to previous fire history (burned or unburned ∼3 years before).
Our evaluation of the species composition of small mammals in the study sites indicated a clear separation according to the fire history, as shown by the NMDS ordination (Figure 3). This separation was corroborated by the PERMANOVA test, which indicated that species composition differed significantly between burned and unburned forests (total SS = 2.096, within-group SS = 1.389, F = 3.057, p = 0.029). The evaluation of the relative position of environmental variables (passive variables in the analysis) indicated that both groups of forest sites differed in structural characteristics related to the previous occurrence of fire (i.e., understory density, herbaceous cover and number of trees with fire scars; Figure 3).
Figure 3. Non-metric multidimensional scaling (NMDS; Bray-Curtis dissimilarity) for small mammal abundance in the eight sampled sites in the Brazilian Cerrado (stress = 0.16). We included habitat-structure variables as passive variables in the ordination. Habitat variables codes: Firescr—mean number of trees with fire scars; Treedia, tree diameter at breast height; %Under, understory density (%); %Herba, herbaceous cover (%); %Canop, canopy cover (%). Sites that burned ∼3 years before (circles) differed from those that did not burn (triangles; PERMANOVA test, see text for details).
The evaluation of the effects of fire and exclosure treatment on seed removal across all tree species indicated that the previous occurrence of fire caused a decline in seed removal (23.9% of seeds removed) in comparison with unburned sites (52.2% removed). Moreover, vertebrate seed removal significantly declined inside cages with access only to rodents (29.7% of seeds removed) as compared to uncaged seeds (46.5% removed), indicating that other vertebrates than small rodents were significant seed removers (Figure 4 and see Table 1 for statistical results).
Figure 4. Estimates of percentage of seeds removed differed in relation to fire history (burned vs. not burned ∼3 years before; mixed-effects logistic regression: P = 0.045) and to access of medium and small vertebrates (squares) vs. small rodents only (circles; P < 0.001) across the six studied plant species. Error bars show confidence intervals of the estimates (see Table 1 for complete statistical results).
Table 1. Results of a generalized linear mixed model testing the effects of previous fire occurrence (burned or not burned ∼3 years before) and exclosure treatment (caged = access only to small rodents vs. uncaged = access to all vertebrate groups) on seed-removal proportion of six plant species in gallery forests in the Cerrado (neotropical savanna).
In models with each species analyzed separately, for all species seeds were less removed in previously burned gallery forests than in the unburned forests and in uncaged stations than in caged ones (Figure 5). The decline in previously burned sites, however, was significant only for C. estrellensis (Table 2). But the interaction between fire and exclosure treatment was significant for two of the six species (P. elegans and D. alata), indicating that for these species the previous occurrence of fire was more detrimental to seed removal by all vertebrate groups in comparison to seed removal by rodents only. For other two species (C. langsdorffii and H. courbaril), seed removal was significantly lower in caged than in uncaged seeds, suggesting that other vertebrates than small rodents were significant seed removers of these species (Figure 5).
Figure 5. Estimates of percentage of seeds removed (error bars = 95% confidence intervals) in relation to fire history (burned vs. not burned ∼3 years before) and exclosure treatment (access to all vertebrates vs. access of small rodents only) for each of the six studied plant species (mixed-effects logistic regression). Species codes: CA, Cariniana estrellensis; CO, Copaifera langsdorffii; PE, Platypodium elegans; DA, Dipteryx alata; HC, Hymenaea courbaril; MF, Mauritia flexuosa. Significant effects of the factors (and their interaction) are indicated for each species (*p < 0.05, ***P < 0.001; see Table 2 for complete statistical results).
Table 2. Species-specific responses of six plant species to the effects of previous fire occurrence (burned or not burned ∼3 years before) and exclosure treatment (caged = access only to small rodents vs. uncaged = access to all vertebrate groups) on seed-removal proportion.
All species of seeds offered were consumed by at least three of the five rodent species in captivity. Mean seed consumption rates of seed species ranged from 30 to 100% (Supplementary Figure 2). The preferred plant species were D. alata and C. estrellensis, with > 90% of mean seed consumption rates considering the five rodent species. On the other hand, P. elegans, H. courbaril, and M. flexuosa had average consumption rates between 30 and 40% and were not consumed by at least one of the rodent species tested (Supplementary Figure 2).
We investigated the effects of fire on seed removal by distinct vertebrate groups and observed that the burning regime in gallery forests strongly influenced seed removal patterns by vertebrates about 3 years after the fire occurrence. This previous burning changed the structural characteristics of the forests, mainly increasing the density of the understory vegetation and the herbaceous cover. Differences in these environmental characteristics between burned and unburned forests likely determined differences in species composition, richness, and abundance of small rodents, which influenced the rates of seed removal. Other vertebrates, larger than small rodents, played a significant role as seed removers for five of the six species. Seed removal by both groups (small rodents and other vertebrates), however, was reduced by the large-scale wildfire. The effects of fire were consistent across species, but for the two species with the largest seeds (H. courbaril and M. flexuosa) removal rates (for both groups) were extremely low in the burned forests (up to 5%).
We relied on a large-scale natural fire evaluating the responses of seed removers (small rodents and other vertebrates) to mid-term fire-induced changes in forested habitats. This kind of experiment is subject to criticism to the lack of replication in the sense that there was only one fire event. Large-scale wildfires, however, are a kind of unpredictable natural experiment that provides opportunities for the investigation of relevant ecological processes that could not otherwise be evaluated (Legge et al., 2008; Mendonça et al., 2015). Natural experiments like wildfires are relevant components of ecological research and should not be discarded based merely on a questionable dogmatic use of the pseudoreplication concept (Schank and Koehnle, 2009; Davies and Gray, 2015) which could represent the loss of opportunities to learn from large-scale disturbance events where strictly adequate replication is not feasible (Davies and Gray, 2015). In our study, we relied on a hierarchical “experimental” design using a mixed-effect modeling approach to deal with this issue. The faunal responses to large-scale fires can only be evaluated in natural “experiments” designed after the occurrence of unplanned wildfires, since small, experimental fires could not mimic a large wildfire. We acknowledge, however, that our approach has statistical limitations and the generalization of the conclusions that are drawn from our results must be done with care.
Our results indicated that the areas that had been burned before presented a distinct species composition and a lower abundance of small mammals compared to areas that did not burn, as we expected. These differences in small mammal communities were probably associated to differences in vegetation structure, as suggested for other forested systems (Chia et al., 2016; González et al., 2021). There was a clear separation of the burnt from the unburnt forests in relation to the habitat variables measured in the field (tree diameter, canopy cover, understory plant density, herbaceous cover, and number of trees with fire scars). The previously burned forests showed an increase in understory density, herbaceous ground cover and number of trees with fire scars, as expected. This reinforces our choice and classification of forests as burned and unburned and indicates that structural differences are still evident even nearly 3 years after the fire event, reinforcing the reduced resilience of Cerrado forests in comparison to savanna formations (Coutinho, 1990; Reis et al., 2017). The increase in understory density and herbaceous cover was probably related to tree top-kill, which allows increased light to the forest interior but also lower air humidity causing dryness in the forest environment (Hoffmann et al., 2009; Prestes et al., 2020).
This increase in understory and herbaceous vegetation apparently did not result in more adequate resources which could potentially increase (or at least maintain) small mammal abundance. The average abundance of rodents in the burned forests was reduced to approximately one-sixth compared to unburned forests. This sharp reduction was probably related to the relatively small area covered by gallery forests in the study landscape (Figure 1), which results in low overall abundance of several forest species and limits the capacity of post-fire colonization by new individuals of forest-dweller small mammals. The burned forest sites were not occupied by non-forest specialists either. In a much larger riverine forest in the Colombian llanos, small mammal richness in burned sites 3 years after a large-scale wildfire (burning 350 ha of the forest) was lower than in unburned sites but responses varied across species. The occurrence of fire increased the abundance of the rodent Zygodontomys brevicauda and did not seem to impact the populations of Oecomys sp. whereas restricting the occurrence of the marsupial Didelphis marsupialis (González et al., 2021). These discrepancies with our results are probably related to the much larger forest area sampled by González et al. (2021) and consequent much higher recolonization opportunities for the small mammals (from the unburned neighboring areas) in comparison to our studied landscape.
The differences in small-mammal composition that we found were mainly due to the absence (or low number of records) of arboreal mammals in the burned areas. Some of these arboreal species were captured only in the unburned forests (i.e., Oecomys sp. and R. macrurus) or else were much less abundant in the burned forests (i.e., G. agilis). These arboreal species were rare or absent in the burned forests despite the high density of understory vegetation. This suggests that indirect factors, such as fire-induced reduction in canopy resources or increased predation rates (Leahy et al., 2016) could be affecting these animals. Arboreal mammals also were negatively affected by high-severity fire (like the wildfire that occurred in our study area) in Australian forests (Chia et al., 2016). In our studied gallery forests, in addition to fire-induced death of trees (Hoffmann et al., 2009) and consequent structural changes (González et al., 2021), the decline of arboreal small mammals was probably related to the potential post-fire reduction in the availability of fruits, their preferred resources (Paglia et al., 2012; Ribeiro et al., 2019). Other arboreal frugivorous rodents in the neotropics suffered a post-fire decline in abundance (or even local extinction), as reported for Oecomys concolor in Atlantic Forest fragments (Figueiredo and Fernandez, 2004) and Irenomys tarsalis in Araucaria-Nothofagus Chilean forests (Zúñiga et al., 2021). In neotropical savanna-gallery forests in Colombian llanos, however, the occupancy probability of Oecomys sp. did not differ between sites burned 3 years before and unburned sites, but the occurrence of this rodent in burned areas was related to the maintenance of an arboreal strata (González et al., 2021). Fire also negatively affected two arboreal small mammals (G. agilis and R. macrurus) in “cerradão” forests (closed woodland with arboreal cover of 50–90% and tree height mostly between 8–12; Oliveira-Filho and Ratter, 2002) burned by the same large-scale wildfire investigated in the present study. Both these species showed apparent fire-induced local extinction and were still absent or occurring in very low densities about 1.7 years after fire; Mendonça et al., 2015).
Terrestrial forest species were affected distinctly by fire. The large (∼220 g) semi-aquatic rodent N. squamipes occurred in both unburned and previously burned forests. This rodent is closely associated to water streams (Ernest and Mares, 1986), whose surrounding vegetation is generally less affected by fire because of its high humidity. This vegetation may confer protection and food resources for this species, which may feed on aquatic invertebrates and also on fruits and seeds (Crespo, 1982; Lessa et al., 2019). In fact, in our study all N. squamipes individuals were captured at stations near (≤10 m) watercourses. This species may have been responsible for most of rodent seed removal in the burned areas, which was corroborated by or results on seed consumption by N. squamipes in captivity. The other large terrestrial rodent captured, the frugivorous-granivorous echimyid P. roberti, was not captured in the burned forests, suggesting a low tolerance to this kind of disturbance. The role of P. roberti and other forest frugivorous rodents in burned forests could potentially be replaced by a more generalist fauna of “outsiders” small rodents, such as N. lasiurus, Oligoryzomys spp and Calomys spp., considering that these open-area species are able to occupy recently burned dry forests (i.e., cerradão) in the same study region (Camargo et al., 2018). This kind of Cerrado species replacement could maintain similar post-fire rates of seed removal in comparison to pre-fire patterns, especially for small seeds. In gallery forests, however, we did not detect post-fire colonization of burned forests by any open-area species.
We evaluated rates of seed removal for six native tree species in relation to the previous (∼3 years before) occurrence of fire and kind of seed remover (small rodents vs. all vertebrates) and detected that overall seed removal was lower in the recently burned areas and higher for the seeds available to all vertebrates. The observed lower seed removal rates in burned forests in comparison to the unburned ones mirrored the decrease in small mammal abundance that we observed in the burned forests, corroborating the second hypothesis that we raised. Similarly, seeds accessible to all animals were removed at lower rates in sites burned 4 months earlier than in unburned sites in Australia (Tasker et al., 2011). This fire-induced decrease in seed removal also occurred in our study, albeit over a longer post-fire time frame. This decrease indicates an indirect effect of the fire factor, even almost 3 years later, on the interaction between seeds and their removal agents inside gallery forests. A direct relationship between rodent abundance and seed removal, as observed in our study, was indicated by other authors (Ostfeld et al., 1997; Schnurr et al., 2002; Demattia et al., 2004; Pearson et al., 2014). Neotropical small rodents are considered to be essentially predators of seeds in the evaluated size range (Díaz et al., 1999; Forget et al., 1999; Vieira et al., 2003; Kelt et al., 2004), which reinforces the assumption that the removal rates obtained in the treatment of partial exclusion (SMRO) in fact reflected seed predation rates.
The reduction in vertebrate seed predation in previously burned areas might be considered beneficial for post-fire recruitment of tree species in gallery forests. This is not necessarily true, however, when considering the entire plant communities associated to these forests. Vertebrates generally are non-selective seed predators and, in their absence, the relative role of other groups of relevant seed predators in the neotropics, such as insects and fungi, probably increases (Williams et al., 2021). Therefore, fire occurrence might cause a switch from generalist seed predators that do not cause strong conspecific density-dependent mortality (following the classic Janzen-Connell model; Janzen, 1970; Connell, 1971) to specific predators that attack seeds in a density-dependence manner, like fungi and insects (Terborgh, 2012). This switch might have profound medium to long-term consequences in gallery forest plant communities (Williams et al., 2021). Considering that neotropical small rodents do not prey upon all seed species in the same way (Vieira et al., 2003; Galetti et al., 2015b; also as indicated by our seed-offering experiments), a fire-induced rodent decrease and consequent seed predator release per se could affect plant community patterns of the forests. A reduction in predation of some plant species could cause a high dominance of these species, reducing plant diversity (Dirzo and Miranda, 1990; Putz et al., 1990). Moreover, the rodent-poor burned forests could also be more prone to be occupied by exotic plant invaders, since field experiments demonstrated that seed predation by neotropical small rodents is a source of biotic resistance to plant invasions (Pearson et al., 2014).
The results on seed-offering to captive rodents were consonant with those obtained in field. The two most-consumed seed species in captivity were also those with the highest removal rates in the partial exclosure treatment (access to small rodents only), with estimated seed removal values of 89% (C. estrellensis) and 49% (D. alata). This indicates that these species are subject to high natural predation rates and rodents potentially play a relevant role for regulating natural populations of both species. Seed removal of C. estrellensis was relatively high in the burned forests, being much higher (35%) than for all the other species (range 2–11%). Considering that C. estrellensis has the smallest seed size among the studied species (seed mass = 0.08 g), we suggest that its seeds are potentially removed by more vertebrates than the species with larger seeds. Most forest birds that feed on the ground probably are able to consume C. estrellensis seeds but not the seeds of other studied species (Christianini and Galetti, 2007). The guild of C. estrellensis seed predators include even the howler monkey Alouatta caraya (Oliveira-Filho and Galetti, 1996), which occurs in the study area (Schneider et al., 2011). This large spectrum of seed removal agents possible ameliorate the fire effects on C. estrellensis seed removal by vertebrates. These fire effects seem to be higher for the plant species with large seeds, whose spectrum of seed removal agents is more restrict (Dirzo et al., 2007; Galetti et al., 2015b; Dylewski et al., 2020).
The effects of fire and exclusion treatment on seed removal were consistent across the six plant species studied. For five of the six species, seeds were significantly more removed from the open control stations. The exception was M. flexuosa, but even for this species in the unburned sites removal rates in the caged stations (i.e., access to small rodents only) were about 40% of the values found for the open control stations. This overall pattern indicates a relevant role of large vertebrates as seed removers in the studied forests. High removal rates in plots accessible to all vertebrates was also reported for H. courbaril in Peruvian Amazon Forest (Terborgh et al., 1993) and for other forest species (see Demattia et al., 2004) in Mexican lowland forests. In our study area, the potential “large” seed-removal agents include collared peccaries, tapirs, agoutis, pacas, primates (Fonseca and Redford, 1984; Schneider et al., 2011), and frugivorous-granivorous birds (e.g., the gray-necked wood-rail Aramides cajaneus, the pale-vented pigeon Patagioenas cayennensis, and the undulated tinamou Crypturellus undulatus; Tubelis, 2011).
Seed removal by both vertebrate groups decreased in the previously burned forests, as indicated by the overall analysis across all species. This decrease was more accentuated for the open control stations (i.e., access to all vertebrates), with overall seed removal estimates changing from 85 to 13% across all plant species. This indicates that previous occurrence of fire reduces severely seed removal by all group of vertebrates. This reduction was still more extreme for the species with the largest seeds—P. elegans, H. courbaril, and M. flexuosa (2–5% of seed removal in the burned forests). Large-sized seeds are removed and consumed mainly by medium to large mammals, such as agoutis, pacas, tapirs and wild pigs (Dirzo et al., 2007; Mendieta-Aguilar et al., 2015; van der Hoek et al., 2019; Portela and Dirzo, 2020). Tapirs (Tapirus terrestris) and collared peccaries (Pecari tajacu) are probably locally extinct (Schneider et al., 2011) in the study region, with agoutis (Dasyprocta azarae) being by far the most common “large” mammal, as indicated by camera trapping data (EMV, unpublished).
The probable low density (or local extinction) of large frugivorous vertebrates in the burned forests are probably related to structural changes of these forests and a reduction in fruit resources. In south-eastern Amazon Forest, the occurrence of fire every 3 years caused a 50% decrease in the abundance of forest-specialist frugivorous butterflies (de Andrade et al., 2017). Moreover, available data for central Brazilian Amazon forests indicate that fruiting tree abundance is severely reduced in forest sites burned 3 years before than in unburnt forest sites and several vertebrate species (including large frugivores) declined in response to fires. One of these species with significant decline in abundance in the burned sites was an agouti species (Dasyprocta agouti; Barlow and Peres, 2006).
The fire-inducted decrease in seed removal by large mammals that we detected in our study may have marked effect on post-fire tree recruitment. The low removal of seeds (mainly those with the largest seed sizes) indicate that these species were probably not being dispersed adequately in such areas. Agoutis (Dasyprocta spp.) are the main seed dispersers of both H. courbaril (Hallwachs, 1986; Gorchov et al., 2004) and M. flexuosa (Mendieta-Aguilar et al., 2015; Acevedo-Quintero and Zamora, 2016) through scatter-hoarding. In general, it is recognized that the reduction of medium and large frugivorous seed dispersing mammals may result in less recruitment of large seed species and may eventually have negative demographic effects for species particularly dispersed by this guild (Stoner et al., 2007). A study carried out in Venezuela suggested that the regeneration of H. courbaril in the absence of agouti, the main disperser of this species (Hallwachs, 1986) was quite limited (Asquith et al., 1999). Other studies in neotropical forests indicated that documented changes in mammal community composition, including the loss of agouti, play a relevant role in the reduction of forest diversity (Leigh et al., 1993; Asquith et al., 1997). A decrease in local densities of H. courbaril, M. flexuosa and other large-seeded agouti-dispersed species would also affect several animal species that depend on them. The palm M. flexuosa, for instance, was suggested to be a “hyperkeystone” species, considering that at least 74 vertebrate species directly use this palm as a food or nest resource (van der Hoek et al., 2019).
We hypothesized that the role all vertebrates and small rodents on seed removal would be affected by the previous occurrence of fire, with a higher relative role of small rodents in comparison with the latter in burned forests. For most species, however, our results did not support this expectation, with fire reducing seed removal rates for both vertebrate groups. Only for P. elegans, the previous occurrence of fire declined seed removal rates relatively more in open control stations than in the caged stations (exclusive access to small rodents). This indicate that, for this species, fire not only decreases total seed removal, but also causes a shift from mixed interactions of seed predation and seed dispersal (through scatter-hoarding) to a higher relative frequency of an exclusive and primarily negative interaction (seed predation from the small rodents). In this way, for P. elegans fire simulates other sources of disturbances in neotropical forests, such as fragmentation (Dirzo et al., 2007; Galetti et al., 2015a).
Historically, gallery forests have not suffered frequently from fire events and, for this reason, studies on the influence of fire in their associated communities are rare. The present study was an important opportunity to assess the effects of fire on the composition of non-volant small mammal communities as well as their role as seed removal agents. We recommend that future studies should evaluate the fate of seeds removed in gallery forest environments disturbed by fire, so that it would be possible to discern between predation or dispersal processes. Furthermore, the fire effects on community composition of large mammals in this forest type should also be investigated.
We investigated the mid-term effects (∼3 years) of a large wildfire on gallery forests of the Cerrado (neotropical savanna) and showed that these effects include a reduction in small mammal richness and abundance (affecting mainly the arboreal frugivorous species). We conclude that the observed decline in small rodent seed predation in the burned forests may have marked medium- and long-term effects on plant communities in gallery forests. These potential effects include changes in community composition, species coexistence, and diversity.
Fire also caused a decline in seed removal by large mammals. These animals (mainly the agouti D. azarae) provide dispersal services that generally are not performed by small vertebrates, mainly for the large-seeded species. The maintenance of such services and consequently of the trees that depend on them may be jeopardized by the burning of gallery forests. We believe that the occurrence of rare and not very extensive wildfires in such forest type probably do not affect the occurrence of small and large mammals (nor their roles as seed predators and seed dispersers) at a landscape scale, if there is enough time for forest regeneration and unburned sources for mammal recolonization. Fire events, however, have been increasing in frequency and intensity in the Cerrado because of human activities and climate changing (Pivello et al., 2021), being capable of destroying otherwise resistant Gallery Forests (Redford and Fonseca, 1986). This is a serious threat considering that these forests are fire-sensitive ecosystems (Pivello et al., 2021) and keystone habitats that play a crucial role in maintaining mammalian diversity in the Cerrado (Redford and Fonseca, 1986). This role is not restricted to exclusive forest mammals. Even though covering a small total area within the landscape (about 5% of the entire Cerrado area; Cássia-Silva et al., 2020), gallery forests are used to some extent (obligately or opportunistically) by 84% of 31 small mammal genera (marsupials and rodents) reported to occur in the Cerrado, including several genera that occur primarily in typical savanna environments (Redford and Fonseca, 1986).
The raw data supporting the conclusions of this article will be made available by the authors, without undue reservation.
The animal study was reviewed and approved by Committee on Animal Research and Ethics (CEUA, Universidade de Brasilia—UnB), license number 44372/2012.
TC collected data in the field. EV and TC performed statistical analysis. Both authors contributed to the conception and design of study, wrote the manuscript, and contributed equally to manuscript revision, read, and approved the submitted version.
This study was financed in part by the Coordenação de Aperfeiçoamento de Pessoal de Nível Superior, Brasil—CAPES (Coordination and Improvement of Higher Level of Education Personnel—Finance Code 001), which provided a graduate scholarship to TC. The Brazilian Conselho Nacional de Desenvolvimento Científico e Tecnológico—CNPq (National Council for Scientific and Technological Development) provided personal research grants to EV (no. 311988/2017-2). This study conducted as part of the Long-term Ecological Research Program (PELD/CNPq; grant no. 403845/2012-2).
The authors declare that the research was conducted in the absence of any commercial or financial relationships that could be construed as a potential conflict of interest.
All claims expressed in this article are solely those of the authors and do not necessarily represent those of their affiliated organizations, or those of the publisher, the editors and the reviewers. Any product that may be evaluated in this article, or claim that may be made by its manufacturer, is not guaranteed or endorsed by the publisher.
The Ecological and Agricultural Field Station of the University of Brasília (Fazenda Água Limpa—FAL/UnB) and the Ecological Reserve of the IBGE (Instituto Brasileiro de Geografia e Estatística - RECOR) kindly authorized the fieldwork and their staff provided invaluable logistical support.
The Supplementary Material for this article can be found online at: https://www.frontiersin.org/articles/10.3389/fevo.2021.793947/full#supplementary-material
Supplementary Video 1 | This video shows a small rodent (probably Hylaeamys megacephalus) entering a semipermeable exclosure with seeds in its interior during the field experiment. Inside the cage there was another rodent of the same species.
Abramoff, M. D., Magalhaes, P. J., and Ram, S. J. (2004). Image processing with ImageJ. Biophotonics Int. 11, 36–42.
Acevedo-Quintero, J., and Zamora, G. (2016). Role of mammals on seed dispersal and predation processes of Mauritia flexuosa (Arecaceae) in the Colombian Amazon. Rev. Biol. Trop. 64, 5–15. doi: 10.15517/rbt.v64i1.18157
Andersen, A. N. (2021). Faunal responses to fire in Australian tropical savannas: insights from field experiments and their lessons for conservation management. Divers. Distrib. 27, 828–843. doi: 10.1111/ddi.13198
Anderson, M. J. (2001). A new method for non-parametric multivariate analysis of variance. Aust. Ecol. 26, 32–46. doi: 10.1111/j.1442-9993.2001.01070.pp.x
Asquith, N. M., Wright, S. J., and Clauss, M. J. (1997). Does mammal community composition control recruitment in neotropical forests? Evidence from Panama. Ecology 78, 941–946. doi: 10.1890/0012-9658(1997)078[0941:dmcccr]2.0.co;2
Asquith, N., Terborgh, J., Arnold, A., and Riveros, C. (1999). The fruits the agouti ate: Hymenaea courbaril seed fate when its disperser is absent. J. Trop. Ecol. 15, 229–235. doi: 10.1017/S0266467499000772
Auld, T. D., and Denham, A. J. (2001). The impact of seed predation by mammals on post-fire seed accumulation in the endangered shrub Grevillea caleyi (Proteaceae). Biol. Conserv. 97, 377–385. doi: 10.1016/S0006-3207(00)00136-1
Banks, S. C., Knight, E. J., Mcburney, L., Blair, D., and Lindenmayer, D. B. (2011). The effects of wildfire on mortality and resources for an arboreal marsupial: resilience to fire events but susceptibility to fire regime change. PLoS One 6:e22952. doi: 10.1371/journal.pone.0022952
Barlow, J., and Peres, C. A. (2006). Effects of single and recurrent wildfires on fruit production and large vertebrate abundance in a central Amazonian forest. Biodivers. Conserv. 15, 985–1012. doi: 10.1007/s10531-004-3952-1
Bates, D., Mächler, M., Bolker, B., and Walker, S. (2014). Fitting linear mixed-effects models using lme4. arXiv [preprint] arXiv:1406.5823
Bendell, J. F. (1974). “Effects of fire on birds and mammals,” in Fire and Ecosystems, ed. T. T. Koslowsky (New York, NY: Academic press), 73–138. doi: 10.1016/b978-0-12-424255-5.50009-2
Bond, W. J., and Keeley, J. E. (2005). Fire as a global ‘herbivore’: the ecology and evolution of flammable ecosystems. Trends Ecol. Evol. 20, 387–394. doi: 10.1016/j.tree.2005.04.025
Bricker, M., Pearson, D., and Maron, J. (2010). Small-mammal seed predation limits the recruitment and abundance of two perennial grassland forbs. Ecology 91, 85–92. doi: 10.1890/08-1773.1
Broncano, M. J., Rodrigo, A., and Retana, J. (2008). Post-dispersal seed predation in Pinus halepensis and consequences on seedling establishment after fire. Int. J. Wildland Fire 17, 407–414. doi: 10.1071/WF07095
Brown, J. H., and Heske, E. J. (1990). Temporal changes in a Chihuahuan Desert rodent community. Oikos 59, 290–302.
Camargo, A. C. L., Barrio, R. O. L., De Camargo, N. F., Mendonça, A. F., Ribeiro, J. F., Rodrigues, C. M. F., et al. (2018). Fire affects the occurrence of small mammals at distinct spatial scales in a neotropical savanna. Eur. J. Wildl. Res. 64, 63–74. doi: 10.1007/s10344-018-1224-8
Cássia-Silva, C., Cianciaruso, M. V., Dias, P. A., Freitas, C. G., Souza-Neto, A. C., and Collevatti, R. G. (2020). Among cradles and museums: seasonally dry forest promotes lineage exchanges between rain forest and savanna. Plant Ecol. Divers. 13, 1–13. doi: 10.1080/17550874.2019.1709103
Catling, P. C., Newsome, A. E., and Dudzinski, G. (1982). Small Mammals, Habitat Components, and Fire in Southeastern Australia. United States Department of Agriculture, Forest Service, General Technical Report, PSW 58. Berkeley, CA: U.S. Department of Agriculture, 199–206.
Chia, E. K., Bassett, M., Leonard, S. W. J., Holland, G. J., Ritchie, E. G., Clarke, M. F., et al. (2016). Effects of the fire regime on mammal occurrence after wildfire: site effects vs landscape context in fire-prone forests. For. Ecol. Manag. 363, 130–139. doi: 10.1016/j.foreco.2015.12.008
Christianini, A. V., and Galetti, M. (2007). Spatial variation in post-dispersal seed removal in an Atlantic forest: effects of habitat, location and guilds of seed predators. Acta Oecol. 32, 328–336. doi: 10.1016/j.actao.2007.06.004
Connell, J. H. (1971). “On the role of the natural enemies in preventing competitive exclusion in some marine animals and in rain forest trees,” in Dynamics of Populations, eds P. J. Den Boer and G. Gradwell (Wageningen: PUDOC), 298–312.
Converse, S. J., Block, W. M., and White, G. C. (2006). Small mammal population and habitat responses to forest thinning and prescribed fire. For. Ecol. Manag. 228, 263–273. doi: 10.1016/j.foreco.2006.03.006
Coutinho, L. M. (1990). “Fire in the ecology of the Brazilian Cerrado,” in Fire in the Tropical Biota: Ecosystem Processes and Global Challenges, ed. J. G. Goldammer (Berlin: Springer Berlin Heidelberg), 82–105. doi: 10.1007/978-3-642-75395-4_6
Crespo, J. A. (1982). Ecologia de la comunidad de mamiferos del Parque Nacional Iguazu, Misiones. Rev. Museo Argent. Cien. Nat. Bernardino Rivadavia. III, 51–162.
Crowner, A. W., and Barret, G. W. (1979). Effects of fire on the small mammal component of an experimental grassland community. J. Mammal. 60, 803–813. doi: 10.1002/ecy.2986
Cummings, C. L., and Alexander, H. M. (2002). Population ecology of wild sunflowers: effects of seed density and post-dispersal vertebrate seed predators. Oecologia 130, 274–280. doi: 10.1007/s004420100806
Curtin, C. G., Kelt, D. A., Frey, T. C., and Brown, J. H. (2000). On the role of small mammals in mediating climatically driven vegetation change. Ecol. Lett. 3, 309–317. doi: 10.1046/j.1461-0248.2000.00166.x
Dafni, A., Izhaki, I., and Ne’eman, G. (2012). The effect of fire on biotic interactions in Mediterranean basin ecosystems: pollination and seed dispersal. Isr. J. Ecol. Evol. 58, 235–250. doi: 10.1560/IJEE.58.2-3.235
Davies, G., and Gray, A. (2015). Don’t let spurious accusations of pseudoreplication limit our ability to learn from natural experiments (and other messy kinds of ecological monitoring). Ecol. Evol. 5, 5295–5304. doi: 10.1002/ece3.1782
de Andrade, R. B., Balch, J. K., Carreira, J. Y. O., Brando, P. M., and Freitas, A. V. L. (2017). The impacts of recurrent fires on diversity of fruit-feeding butterflies in a south-eastern Amazon forest. J. Trop. Ecol. 33, 22–32. doi: 10.1017/S0266467416000559
Demattia, E., Curran, L., and Rathcke, B. (2004). Effects of small rodents and large mammals on neotropical seeds. Ecology 85, 2161–2170. doi: 10.1890/03-0254
Díaz, I. A., Papic, C., and Armesto, J. (1999). An assessment of post-dispersal seed predation in temperate rain forest fragments in Chiloé Island, Chile. Oikos 87, 228–238. doi: 10.2307/3546738
Diniz, I. R., Higgins, B., and Morais, H. C. (2011). How do frequent fires in the Cerrado alter the lepidopteran community? Biodivers. Conserv. 20, 1415–1426. doi: 10.1007/s10531-011-0034-z
Dirzo, R., and Miranda, A. (1990). Contemporary neotropical defaunation and forest structure, function, and diversity – a sequel to John Terborgh. Conserv. Biol. 4, 444–447. doi: 10.1111/j.1523-1739.1990.tb00320.x
Dirzo, R., Mendoza, E., and Ortíz, P. (2007). Size-Related differential seed predation in a heavily defaunated neotropical rain forest. Biotropica 39, 355–362. doi: 10.1111/j.1744-7429.2007.00274.x
Dylewski, A., Ortega, Y. K., Bogdziewicz, M., and Pearson, D. E. (2020). Seed size predicts global effects of small mammal seed predation on plant recruitment. Ecol. Lett. 23, 1024–1033. doi: 10.1111/ele.13499
Ernest, K. A., and Mares, M. A. (1986). Ecology of Nectomys squamipes, the Neotropical water rat, in central Brazil: home range, habitat selection, reproduction and behaviour. J. Zool. 210, 599–612. doi: 10.1111/j.1469-7998.1986.tb03658.x
Felfili, J. M. (1995). Growth, recruitment and mortality in the Gama gallery forest in central Brazil over a six-year period (1985–1991). J. Trop. Ecol. 11, 67–83. doi: 10.1017/S0266467400008415
Felfili, J. M. (1997). Dynamics of the natural regeneration in the Gama gallery forest in central Brazil. For. Ecol. Manag. 91, 235–245. doi: 10.1016/S0378-1127(96)03862-5
Felfili, J. M., Mendonça, R., Walter, B., Silva Júnior, M., Nóbrega, M., Fagg, C. W., et al. (2001). “Flora fanerogâmica das matas de galeria e ciliares do Brasil Central,” in Cerrado: Caracterização e Recuperação de Matas de Galeria, eds J. Ribeiro, C. Fonseca, and J. Souza-Silva (Planaltina: Embrapa Cerrados), 195–263.
Figueiredo, M. D. L., and Fernandez, F. A. D. (2004). Contrasting effects of fire on populations of two small rodent species in fragments of Atlantic Forest in Brazil. J. Trop. Ecol. 20, 225–228. doi: 10.1017/s0266467403001093
Fonseca, G. A. B., and Redford, K. H. (1984). The mammals of IBGE’s ecological reserve, Brasilia, and an analysis of the role of gallery forests in increasing diversity. Rev. Bras. Biol. 44, 517–523.
Fontaine, J. B., and Kennedy, P. L. (2012). Meta-analysis of avian and small-mammal response to fire severity and fire surrogate treatments in U.S. fire-prone forests. Ecol. Appl. 22, 1547–1561. doi: 10.1890/12-0009.1
Forget, P. M., Kitajima, K., and Foster, R. B. (1999). Pre- and post-dispersal seed predation in Tachigali versicolor (Caesalpiniaceae): effects of timing of fruiting and variation among trees. J. Trop. Ecol. 15, 61–81. doi: 10.1017/s026646749900067x
Fox, B. J., Taylor, J. E., and Thompson, P. T. (2003). Experimental manipulation of habitat structure: a retrogression of the small mammal succession. J. Anim. Ecol. 72, 927–940. doi: 10.1046/j.1365-2656.2003.00765.x
Galetti, M., Guevara, R., Galbiati, L. A., Neves, C. L., Rodarte, R. R., and Mendes, C. P. (2015b). Seed predation by rodents and implications for plant recruitment in defaunated Atlantic forests. Biotropica 47, 521–525. doi: 10.1111/btp.12246
Galetti, M., Bovendorp, R. S., and Guevara, R. (2015a). Defaunation of large mammals leads to an increase in seed predation in the Atlantic forests. Glob. Ecol. Conserv. 3, 824–830. doi: 10.1016/j.gecco.2015.04.008
González, T. M., González-Trujillo, J. D., Muñoz, A., and Armenteras, D. (2021). Differential effects of fire on the occupancy of small mammals in neotropical savanna-gallery forests. Perspect. Ecol. Conserv. 19, 179–188. doi: 10.1016/j.pecon.2021.03.005
Gorchov, D. L., Palmeirim, J. M., Jaramillo, M., and Ascorra, C. (2004). Dispersal of seeds of Hymenaea courbaril (Fabaceae) in a logged rain forest in the Peruvian Amazonian. Acta Amazon. 34, 265–273.
Hadas, A. (2005). “Germination and seedling establishment,” in Encyclopedia of Soils in the Environment, ed. D. Hillel (Oxford: Elsevier), 130–137.
Hallwachs, W. (1986). “Agoutis (Dasyprocta punctata): the inheritors of Guapinol (Hymenaea courbaril: leguminosae),” in Frugivores and Seed Dispersal. Tasks for Vegetation Science, Vol. 15, eds A. Estrada and T. H. Fleming (Dordrecht: Springer). doi: 10.1007/978-94-009-4812-9_25
Hammer, Ø., Harper, D. A. T., and Ryan, P. D. (2001). PAST: paleontological statistics software package for education and data analysis. Palaeontol. Electronica 4:9.
Happold, D. C. D. (1983). “Rodents and lagomorphs,” in Tropical Savannas, ed. F. Bourliere (Amsterdam: Elsevier), 363–400.
Harms, K. E., Wright, S. J. Calderón, O. Hernández, A., and Herre, E. A. (2000). Pervasive density-dependent recruitment enhances seedling diversity in a tropical forest. Nature 404, 493–495. doi: 10.1038/35006630
Hartig, F. (2017). DHARMa: Residual diagnostics for Hierarchical (Multi-Level/Mixed) Regression Models. R Package Version 0.1.5.
Hoffmann, W. A., Adasme, R., Haridasan, M., De Carvalho, M. T., Geiger, E. L., Pereira, M. A. B., et al. (2009). Tree topkill, not mortality, governs the dynamics of savanna–forest boundaries under frequent fire in central Brazil. Ecology 90, 1326–1337. doi: 10.1890/08-0741.1
Hoffmann, W. A., and Moreira, A. G. (2002). “The cerrados of Brazil: ecology and natural history of a Neotropical savanna,” in The Role of Fire in Population Dynamics of Woody Plants, eds S. O. Paulo and J. M. Robert (New York, NY: Columbia University Press), 159–177.
Hoffmann, W. A., Geiger, E. L., Gotsch, S. G., Rossatto, D. R., Silva, L. C. R., Lau, O. L., et al. (2012). Ecological thresholds at the savanna-forest boundary: how plant traits, resources and fire govern the distribution of tropical biomes. Ecol. Lett. 15, 759–768. doi: 10.1111/j.1461-0248.2012.01789.x
Howe, H. F., and Brown, J. S. (2000). Early effects of rodent granivory on experimental forb communities. Ecol. Appl. 10, 917–924. doi: 10.1890/1051-0761(2000)010[0917:EEORGO]2.0.CO;2
Hulme, P. E. (1993). Post-dispersal seed predation by small mammals. Symp. Zool. Soc. Lond. 65, 269–287.
Hulme, P. E. (1998). Post-dispersal seed predation: consequences for plant demography and evolution. Perspect. Plant Ecol. Evol. Syst. 1, 32–46. doi: 10.1078/1433-8319-00050
Iob, G., and Vieira, E. M. (2008). Seed predation of Araucaria angustifolia (Araucariaceae) in the Brazilian Araucaria Forest: influence of deposition site and comparative role of small and ‘large’ mammals. Plant Ecol. 198, 185–196. doi: 10.1007/s11258-007-9394-6
Janzen, D. (1970). Herbivores and number of tree species in tropical forests. Am. Nat. 104, 501–528. doi: 10.1086/282687
Kelt, D. A., Meserve, P. L., Forister, M. L., Nabors, L. K., and Gutiérrez, J. R. (2004). Seed predation by birds and small mammals in semiarid Chile. Oikos 104, 133–141. doi: 10.1111/j.0030-1299.2004.12714.x
Korner-Nievergelt, F., Roth, T., Felten, S. V., Guelat, J., Almasi, B., and Korner-Nievergelt, P. (2015). blmeco: Data files and Functions Accompanying the Book “Bayesian Data Analysis in Ecology Using R, BUGS and Stan. New York, NY: Elsevier.
Lassau, S. A., Hochuli, D. F., and Fuchs, T. (2004). Effects of habitat complexity on ant assemblages. Ecography 27, 157–164. doi: 10.1111/j.0906-7590.2004.03675.x
Leahy, L., Legge, S. M., Tuft, K., Mcgregor, H. W., Barmuta, L. A., Jones, M. E., et al. (2016). Amplified predation after fire suppresses rodent populations in Australia’s tropical savannas. Wildl. Res. 42, 705–716. doi: 10.1071/WR15011
Legge, S., Murphy, S., Heathcote, J., Flaxman, E., Augusteyn, J., and Crossman, M. (2008). The short-term effects of an extensive and high-intensity fire on vertebrates in the tropical savannas of the central Kimberley, northern Australia. Wildl. Res. 35, 33–43.
Leigh, E., Wright, S. J., Herre, E., and Putz, F. E. (1993). The decline of tree diversity on newly isolated tropical islands: a test of a null hypothesis and some implications. Evol. Ecol. 7, 76–102. doi: 10.1007/BF01237735
Leonard, S., Bennett, A., and Clarke, M. (2014). Determinants of the occurrence of unburnt forest patches: potential biotic refuges within a large, intense wildfire in south-eastern Australia. For. Ecol. Manag. 314, 85–93. doi: 10.1016/j.foreco.2013.11.036
Lessa, L. G., Paula, C. S., and Pessoa, R. S. (2019). Food habits and Endozoochorous seed dispersal by small rodents (Cricetidae and Echimyidae) in a riparian forest in southeastern Brazil. Neotrop. Biol. Conserv. 14, 349–359. doi: 10.3897/neotropical.14.e47403
Li, H., and Zhang, Z. (2007). Effects of mast seeding and rodent abundance on seed predation and dispersal by rodents in Prunus armeniaca (Rosaceae). For. Ecol. Manag. 242, 511–517. doi: 10.1016/j.foreco.2007.01.063
Lindenmayer, D. B., Blanchard, W., Mcburney, L., Blair, D., Banks, S. C., Driscoll, D., et al. (2013). Fire severity and landscape context effects on arboreal marsupials. Biol. Conserv. 167, 137–148. doi: 10.1016/j.biocon.2013.07.028
Lunney, D., Cullis, B., and Eby, P. (1987). Effects of logging and fire on small mammals in Mumbulla state forest, near Bega, New South Wales. Aust. Wildl. Resid. 14, 163–181. doi: 10.1071/wr9870163
Manson, R. H., and Stiles, E. W. (1998). Links between microhabitat preferences and seed predation by small mammals in old fields. Oikos 82, 37–50. doi: 10.2307/3546915
Marimon, B., Felfili, J., Lima, E., Duarte, W., and Marimon-Junior, B. H. (2010). Environmental determinants for natural regeneration of gallery forest at the Cerrado/Amazonia boundaries in Brazil. Acta Amazon. 40, 107–118. doi: 10.1590/S0044-59672010000100014
Maron, J. L., and Kauffman, M. J. (2006). Habitat-specific impacts of multiple consumers on plant population dynamics. Ecology 87, 113–124. doi: 10.1890/05-0434
Mendieta-Aguilar, G., Pacheco, L., and Roldán, A. (2015). Dispersión de semillas de Mauritia flexuosa (Arecaceae) por frugívoros terrestres en Laguna Azul, Beni, Bolivia. Acta Amazon. 45, 45–56. doi: 10.1590/1809-4392201400283
Mendonça, A. F., Armond, T., Camargo, A. C. L., Camargo, N. F., Ribeiro, J. F., Zangrandi, P. L., et al. (2015). Effects of an extensive fire on arboreal small mammal populations in a neotropical savanna woodland. J. Mammal. 96, 368–379. doi: 10.1093/jmammal/gyv038
Miranda, A. C., Miranda, H. S., Dias, I. D. F. O., and Dias, B. F. D. S. (1993). Soil and air temperatures during prescribed Cerrado fires in central Brazil. J. Trop. Ecol. 9, 313–320. doi: 10.1017/s0266467400007367
Miranda, H. S., Bustamante, M. M. C., and Miranda, A. C. (2002). “The fire factor,” in The Cerrados of Brazil, eds P. S. Oliveira and R. J. Marquis (New York, NY: Columbia University Press), 51–68. doi: 10.1525/9780520324626-008
Miranda, H. S., Sato, M. N., Neto, W. N., and Aires, F. S. (2009). “Fires in the cerrado, the Brazilian savanna,” in Tropical Fire Ecology, ed. M. A. Cochrane (Berlin: Springer), 427–450. doi: 10.1007/978-3-540-77381-8_15
Mittelbach, G. G., and Gross, K. L. (1984). Experimental studies of seed predation in old-fields. Oecologia 65, 7–13. doi: 10.1007/BF00384455
Mittelman, P., Dracxler, C. M., Santos-Coutinho, P. R. O., and Pires, A. S. (2021). Sowing forests: a synthesis of seed dispersal and predation by agoutis and their influence on plant communities. Biol. Rev. 96, 2425–2445. doi: 10.1111/brv.12761
Monamy, V., and Fox, B. J. (2000). Small mammal succession is determined by vegetation density rather than time elapsed since disturbance. Aust. Ecol. 25, 580–587. doi: 10.1111/j.1442-9993.2000.tb00063.x
Mood, A. M. (1954). On the asymptotic efficiency of certain nonparametric two-sample tests. Ann. Math. Stat. 25, 514–522. doi: 10.1002/bimj.200900131
Mutch, R. W. (1970). Wildland fires and ecosystems – a hypothesis. Ecology 51, 1046–1051. doi: 10.2307/1933631
Ojeda, R. A. (1989). Small mammals responses to fire in the monte desert, Argentina. J. Mammal. 70, 416–420. doi: 10.2307/1381531
Oliveira-Filho, A. T., and Ratter, J. A. (2002). “Vegetation Physiognomies and Woody Flora of the Cerrado Biome,” in The Cerrados of Brazil: Ecology and Natural History of a Neotropical Savanna, eds P. S. Oliveira and R. J. Marquis (New York, NY: Columbia University Press), 91–120. doi: 10.1590/1519-6984.02016
Oliveira-Filho, A., and Galetti, M. (1996). Seed predation of Cariniana estrellensis (Lecythidaceae) by black howler monkeys, Alouatta caraya. Primates 37, 87–90. doi: 10.1007/BF02382924
Ostfeld, R. S., Manson, R. H., and Canham, C. D. (1997). Effects of rodents on survival of tree seeds and seedlings invading old fields. Ecology 78, 1531–1542. doi: 10.1890/0012-9658(1997)078[1531:eoroso]2.0.co;2
Paglia, A. P., Fonseca, G. A. B. D., Rylands, A. B., Herrmann, G., Aguiar, L. M. S., Chiarello, A. G., et al. (2012). Lista Anotada dos Mamíferos do Brasil / Annotated Checklist of Brazilian Mammals, 2nd Edn. Arlington, TX: Conservation International, 1–82.
Pearson, D. E., Callaway, R. M., and Maron, J. L. (2011). Biotic resistance via granivory: establishment by invasive, naturalized, and native asters reflects generalist preference. Ecology 92, 1748–1757. doi: 10.1890/11-0164.1
Pearson, D. E., Hierro, J. L., Chiuffo, M., and Villarreal, D. (2014). Rodent seed predation as a biotic filter influencing exotic plant abundance and distribution. Biol. Invasions 16, 1185–1196. doi: 10.1007/s10530-013-0573-1
Pivello, V., Guimarães Vieira, I., Christianini, A., Ribeiro, D., Menezes, L., Berlinck, C., et al. (2021). Understanding Brazil’s catastrophic fires: causes, consequences and policy needed to prevent future tragedies. Perspect. Ecol. Conserv. 19, 233–255. doi: 10.1016/j.pecon.2021.06.005
Portela, R. D. C. Q., and Dirzo, R. (2020). Forest fragmentation and defaunation drive an unusual ecological cascade: predation release, monkey population outburst and plant demographic collapse. Biol. Conserv. 252:108852. doi: 10.1016/j.biocon.2020.108852
Prestes, N. C. C. D. S., Massi, K. G., Silva, E. A., Nogueira, D. S., De Oliveira, E. A., Freitag, R., et al. (2020). Fire effects on understory forest regeneration in Southern Amazonia. Front. For. Glob. Change 3:10. doi: 10.3389/ffgc.2020.00010
Putz, F. E., Leigh, E. G., and Wright, S. J. (1990). Solitary confinement in Panama. Garden 14, 18–23.
Rangel, T., Diniz-Filho, J. A., and Bini, L. (2010). SAM: a comprehensive application for spatial analysis in macroecology. Ecography 33, 46–50. doi: 10.1111/j.1600-0587.2009.06299.x
Redford, K. H., and Fonseca, G. A. B. (1986). The role of gallery forests in the zoogeography of the cerrado’s non-volant mammalian fauna. Biotropica 18, 126–135. doi: 10.2307/2388755
Reed, A. W., Kaufman, G. A., and Kaufman, D. W. (2004). Influence of fire, topography, and consumer abundance on seed predation in tallgrass prairie. Can. J. Zool. 82, 1459–1467. doi: 10.1139/z04-126
Reed, A. W., Kaufman, G. A., and Kaufman, D. W. (2005). Rodent seed predation and GUDs: effect of burning and topography. Can. J. Zool. 83, 1279–1285. doi: 10.1139/z05-124
Reis, S. M., De Oliveira, E. A., Elias, F., Gomes, L., Morandi, P. S., Marimon, B. S., et al. (2017). Resistance to fire and the resilience of the woody vegetation of the “Cerradão” in the “Cerrado”–Amazon transition zone. Braz. J. Bot. 40, 193–201. doi: 10.1007/s40415-016-0336-1
Ribeiro, J. F., Guaraldo, A., Nardoto, G. B., Santoro, G., and Vieira, E. M. (2019). Habitat type and seasonality influence the isotopic trophic niche of small mammals in a neotropical savanna. Hystrix Ital. J. Mammal. 30, 30–38. doi: 10.4404/hystrix-00150-2018
Rusch, U. D., Midgley, J., and Anderson, B. (2014). Seasonal fluctuations in rodent seed caching and consumption behaviour in fynbos shrublands: implications for fire management. South Afr. J. Bot. 93, 217–221. doi: 10.1016/j.sajb.2014.04.011
Salazar, A., Goldstein, G., and Franco, A. C. Miralles-Wilhelm, F. (2012). Seed limitation of woody plants in Neotropical savannas. Plant Ecol. 213, 273–287. doi: 10.1007/s11258-011-9973-4
Schank, J. C., and Koehnle, T. J. (2009). Pseudoreplication is a pseudoproblem. J. Comp. Psychol. 123, 421–433. doi: 10.1037/a0013579
Schneider, M., Alho, C. J. R., and Aguiar, L. M. D. S. (2011). “Contribuição da reserva ecológica do IBGE para o conhecimento da mastozoologia do bioma Cerrado,” in Reserva Ecológica do IBGE. Biodiversidade terrestre, ed. M. L. Ribeiro (Rio de Janeiro: Instituto Brasileiro de Geografia e Estatística – IBGE), 157–172.
Schnurr, J. L., Ostfeld, R. S., and Canham, C. D. (2002). Direct and indirect effects of masting on rodent populations and tree seed survival. Oikos 96, 402–410. doi: 10.1034/j.1600-0706.2002.960302.x
Schupp, E. W. (1988). Seed and early seedling predation in the forest understory and in treefall gaps. Oikos 51, 71–78. doi: 10.2307/3565808
Siegel, S., and Castellan, N. J. (1988). Nonparametric Statistics for the Behavioral Sciences. New York, NY: McGraw-Hill.
Sikes, R. S., Gannon, W. L., and The Animal Care and Use Committee of the American Society of Mammalogists (2011). Guidelines of the American society of mammalogists for the use of wild mammals in research. J. Mammal. 92, 235–253. doi: 10.1644/10-mamm-f-355.1
Simon, M. F., Grether, R., De Queiroz, L. P., Skema, C., Pennington, R. T., and Hughes, C. E. (2009). Recent assembly of the Cerrado, a neotropical plant diversity hotspot, by in situ evolution of adaptations to fire. Proc. Natl. Acad. Sci. U.S.A. 106, 20359–20364. doi: 10.1073/pnas.0903410106
Simons, L. H. (1991). Rodent dynamics in relation to fire in the Sonoran desert. J. Mammal. 72, 518–524. doi: 10.2307/1382135
Stoner, K., Riba-Hernandez, P., Vulinec, K., and Lambert, J. (2007). The role of mammals in creating and modifying seedshadows in tropical forests and some possible consequences of their elimination. Biotropica 39, 316–327. doi: 10.1111/j.1744-7429.2007.00292.x
Stuhler, J. D., and Orrock, J. L. (2016). Past agricultural land use and present-day fire regimes can interact to determine the nature of seed predation. Oecologia 181, 463–473. doi: 10.1007/s00442-016-3585-6
Tasker, E. M., Denham, A. J., Taylor, J. E., and Strevens, T. C. (2011). Post-fire seed predation: does distance to unburnt vegetation matter? Aust. Ecol. 36, 755–766. doi: 10.1111/J.1442-9993.2010.02214.X
Terborgh, J. (2012). Enemies maintain hyperdiverse tropical forests. Am. Nat. 179, 303–314. doi: 10.1086/664183
Terborgh, J., Losos, E., Riley, M. P., and Riley, M. B. (1993). Predation by vertebrates and invertebrates on the seeds of five canopy tree species of an Amazonian forest. Vegetatio 107/108, 375–386. doi: 10.1007/978-94-011-1749-4_26
Tran, B. N., Tanase, M. A., Bennett, L. T., and Aponte, C. (2020). High-severity wildfires in temperate Australian forests have increased in extent and aggregation in recent decades. PLoS One 15:e0242484. doi: 10.1371/journal.pone.0242484
Tubelis, D. P. (2011). “A Reserva Ecológica do IBGE e sua contribuição ao conhecimento e à conservação da avifauna do Cerrado,” in Reserva Ecológica do IBGE. Biodiversidade Terrestre, ed. M. L. Ribeiro (Rio de Janeiro: Instituto Brasileiro de Geografia e Estatística - IBGE), 145–156.
van der Hoek, Y., Álvarez Solas, S., and Peñuela, M. C. (2019). The palm Mauritia flexuosa, a keystone plant resource on multiple fronts. Biodivers. Conserv. 28, 539–551. doi: 10.1007/s10531-018-01686-4
Vasconcelos, H. L., Pacheco, R., Silva, R. C., Vasconcelos, P. B., Lopes, C. T., Costa, A. N., et al. (2009). Dynamics of the leaf-litter arthropod fauna following fire in a neotropical woodland Savanna. PLoS One 4:e7762. doi: 10.1371/journal.pone.0007762
Vieira, E. M. (1999). Small mammal communities and fire in the Brazilian Cerrado. J. Zool. 249, 75–81. doi: 10.1111/j.1469-7998.1999.tb01061.x
Vieira, E. M., and Briani, D. C. (2013). Short-term effects of fire on small rodents in the Brazilian Cerrado and their relation with feeding habits. Int. J. Wildl. Fire 22, 1–9. doi: 10.1071/WF12153
Vieira, E. M., and Marinho-Filho, J. (1998). Pre- and post-fire habitat utilization by rodents of Cerrado from Central Brazil. Biotropica 30, 491–496. doi: 10.1111/j.1744-7429.1998.tb00086.x
Vieira, E. M., Paise, G., and Machado, P. H. D. (2006). Feeding of small rodents on seeds and fruits: a comparative analysis of three species of rodents of the Araucaria forest, southern Brazil. Acta Theriol. 51, 311–318. doi: 10.1007/bf03192683
Vieira, E. M., Pizo, M. A., and Izar, P. (2003). Fruit and seed exploitation by small rodents of the Brazilian Atlantic forest. Mammalia 67, 533–539.
Walter, B., and Ribeiro, J. (2010). “Diversidade fitofisionômica e o papel do fogo no bioma Cerrado,” in Efeitos do Regime do Fogo Sobre a Estrutura de Comunidades de Cerrado: Resultados do Projeto Fogo, ed. H. S. Miranda (Brasília: IBAMA), 59–76.
Wang, B. C., and Smith, T. B. (2002). Closing the seed dispersal loop. Trends Ecol. Evol. 17, 379–386. doi: 10.1016/S0169-5347(02)02541-7
Williams, P. J., Ong, R. C., Brodie, J. F., and Luskin, M. S. (2021). Fungi and insects compensate for lost vertebrate seed predation in an experimentally defaunated tropical forest. Nat. Commun. 12:1650. doi: 10.1038/s41467-021-21978-8
Zúñiga, A. H., Rau, J. R., Jaksic, F. M., Vergara, P. M., Encina-Montoya, F., and Fuentes-Ramírez, A. (2021). Rodent assemblage composition as indicator of fire severity in a protected area of south-central Chile. Aust. Ecol. 46, 249–260. doi: 10.1111/aec.12975
Keywords: Cerrado (Brazilian savanna), gallery forest, rodents, seed predation, seed dispersal, fire
Citation: Cazetta TC and Vieira EM (2021) Fire Occurrence Mediates Small-Mammal Seed Removal of Native Tree Species in a Neotropical Savanna. Front. Ecol. Evol. 9:793947. doi: 10.3389/fevo.2021.793947
Received: 12 October 2021; Accepted: 30 November 2021;
Published: 22 December 2021.
Edited by:
Alvaro Soutullo, Universidad de la República, UruguayReviewed by:
Ariel A. Farias, Universidad de la República, UruguayCopyright © 2021 Cazetta and Vieira. This is an open-access article distributed under the terms of the Creative Commons Attribution License (CC BY). The use, distribution or reproduction in other forums is permitted, provided the original author(s) and the copyright owner(s) are credited and that the original publication in this journal is cited, in accordance with accepted academic practice. No use, distribution or reproduction is permitted which does not comply with these terms.
*Correspondence: Emerson M. Vieira, ZW12aWVpcmFAdW5iLmJy
Disclaimer: All claims expressed in this article are solely those of the authors and do not necessarily represent those of their affiliated organizations, or those of the publisher, the editors and the reviewers. Any product that may be evaluated in this article or claim that may be made by its manufacturer is not guaranteed or endorsed by the publisher.
Research integrity at Frontiers
Learn more about the work of our research integrity team to safeguard the quality of each article we publish.