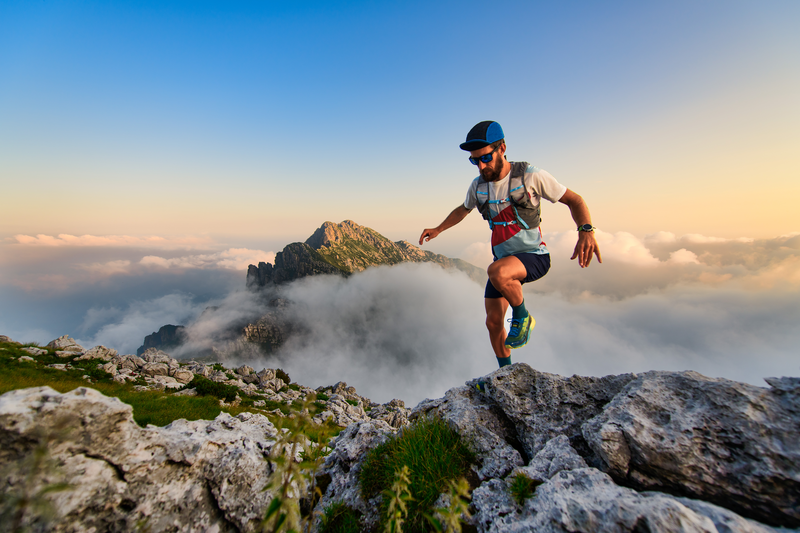
95% of researchers rate our articles as excellent or good
Learn more about the work of our research integrity team to safeguard the quality of each article we publish.
Find out more
ORIGINAL RESEARCH article
Front. Ecol. Evol. , 20 December 2021
Sec. Conservation and Restoration Ecology
Volume 9 - 2021 | https://doi.org/10.3389/fevo.2021.791364
This article is part of the Research Topic Conserving Cultural Ecosystems: The Biodiversity and Ecosystem Service Provision of Semi-Natural Anthropogenic Ecosystems versus Natural Ecosystems View all 7 articles
The ecosystem services framework can be used as a way of balancing economic, ecological and societal drivers in land management decision-making processes. As heathland management is typically linked directly to services, the aim of this study was to quantify trade-offs related to the effects of five common heathland management measures (grazing, mowing, burning, choppering, and sod-cutting) using quantitative data from empirical studies within a northwestern heathland in Germany. Besides important services (groundwater recharge and quality, carbon stocks and appreciation by the general public) we included ecosystem functions (balances of nitrogen, phosphorus and major cations) and the net cost of management implementation as trade-off components. We found that all management practices have advantages and disadvantages leading to unavoidable trade-offs. The effect of a management practice on the trade-off components was often closely related to the amount of biomass and/or soil removed during a management cycle (Rannual). Choppering and sod-cutting (large Rannual by involving soil removal) were very good at maintaining a low N system whilst concurrently increasing groundwater recharge, albeit at the cost of all other components considered. If the aim is to preserve heathlands and their associated ecosystem services in the long-term this trade-off is inevitable, as currently only these high-intensity measures are capable of removing enough nitrogen from the system to prevent the transition to non-heather dominated habitat types. Our study, therefore, shows that in order to maintain structural integrity and thereby the service potential a habitat provides, management decision frameworks may need to prioritize ecosystem functioning over ecosystem services. Burning and mowing (low Rannual) were best at retaining phosphorus, cations and carbon and had the lowest costs. Grazing (intermediate Rannual) provided the highest relative benefit in terms of groundwater quality and appreciation. Together these results can help identify management combinations in both space and time, which will be more beneficial for functions and services than management practices considered in isolation. Furthermore, our study assists in recognizing key areas of action for the development of novel management practices and can help raise awareness of the diversity of rare species and potential benefits to people that protected cultural landscapes provide.
European heathlands are some of the oldest cultural landscapes in Europe. The extent of heathland vegetation in northwestern Europe, which was originally restricted to small-scale, sparsely wooded areas, increased through human intervention from the late Neolithic onwards until the eighteenth century. With the onset of modern agriculture, the provisioning services of such areas became unprofitable and most heathlands were subject to neglect and natural succession or afforestation (Fagúndez, 2013; Doorenbosch and van Mourik, 2016). Today European dry heaths are valuable cultural landscapes which support a wide array of rare and characteristic species (Usher and Thompson, 1993; Webb et al., 2010) whilst also providing important ecosystem services (Wessel et al., 2004; van der Wal et al., 2011).
Despite an increased awareness of the importance of preserving such valuable habitats their conservation status is unfavorable and deteriorating in most of the habitat range (Olmeda et al., 2020). Due to their large distribution range and site-specific characteristics (affecting both biotic and abiotic factors) drivers of this situation are diverse and include habitat destruction and fragmentation, climate change and invasive exotic species (Fagúndez, 2013). The main threats to European dry heaths, however, are currently associated with inappropriate management, or indeed none at all, as well as atmospheric nitrogen (N) deposition and eutrophication and, in certain countries, afforestation (Olmeda et al., 2020).
A lack of management and the afforestation of heathland areas are primarily the result of political decision-making. As with other systems, a prerequisite for decision-making is a specification of objectives. In the case of dry heathlands, which are listed in Annex I of the EU Habitats directive (HT 4030), EU law states that the long-term preservation of the habitat is the main objective. It is, therefore, apparent that if heathlands and their associated biodiversity, ecosystem functions and services are to be maintained in the long-term they must be appropriately managed.
It is well established that increased atmospheric N input into heathlands can have multiple negative impacts on their functioning and biodiversity (Heil and Diemont, 1983; Brunsting and Heil, 1985; Berendse et al., 1994; Gordon et al., 1999; Roem et al., 2002; Jones and Power, 2012; Fagúndez, 2013; Taboada et al., 2016; Olmeda et al., 2020). In the absence of adequate reductions of atmospheric N deposition, counteracting the negative impact of N enrichment in these systems can, therefore, be considered to be one of the most important challenges for heathland managers, even at relatively low deposition rates (Power et al., 2006; Bobbink et al., 2010; Phoenix et al., 2012).
An array of management practices have been implemented in the past, some aiming to counteract the (ecological) effects of N loads, others addressing issues such as plant population degradation (e.g., demographics of Calluna vulgaris populations in relation to lack of grazing). The most common interventions are based on traditional practices such as grazing, mowing, burning and sod-cutting (Webb, 1998). Which of these management practices or which combination of them are applied will depend on a number of factors including the current (successional) state of the heathland, local factors such as N deposition rates, heathland area and fragmentation, edaphic and historical conditions but also operational issues such as regional by-laws, resource availability, local objectives and cost-efficiency. Whilst different management practices vary in their N removal efficiency, they concurrently affect other ecosystem properties as well as soil N, the outcomes of which can be both desirable and undesirable. It is, therefore, important to provide stakeholders with a decision-making framework that enables them to judge the effects of management practices on different components of ecosystem functioning and services and subsequently assess to what extent their conservation efforts are effective, whilst at the same time accounting for the socio-economic impacts a given management practice may have.
The ecosystem services framework has been put forward as a way of balancing economic, ecological and societal drivers in (adaptive) management decision-making processes (Brown et al., 2011; Martinez-Harms et al., 2015; Moore et al., 2017). In fact, a recent review has suggested that one of the most important outcomes of ecosystem service assessments has been to improve decision-making in landscape-planning (Valencia Torres et al., 2021). While many studies have addressed the concept of trade-offs in land-use management from a theoretical perspective (e.g., Raudsepp-Hearne et al., 2010; Cavender-Bares et al., 2015; Turkelboom et al., 2015; Deng et al., 2016; Cord et al., 2017; Vallet et al., 2018), its application on the ground remains challenging as it requires the assessment and subsequent valuation of all components under consideration.
The ability of ecosystems to simultaneously provide a range of different ecosystem functions and services, aka multifunctionality, is gaining traction in evidence-based policy (Manning et al., 2018). In the current study, we define and address multifunctionality as including both the explicit provisioning of ecosystem functions as well as services. This combined approach allows us to relate directly the effects of management actions on functions to a suite of different ecosystem services. In this study, we assess the extent to which grazing, mowing, prescribed burning, choppering and sod-cutting can preserve the multifunctionality of one of the largest remnants of ancient dry heathland in northwestern Germany. Based on the quantitative results of empirical studies within the case study area we aim to provide meaningful decision support to stakeholders and policy makers by quantifying trade-offs between selected ecosystem services and functions. At the same time, we highlight the limitations such an approach may have. Due to their above-mentioned importance in maintaining heathland integrity and vitality, we focus on ecosystem functions related to the nutrient status of the system (elemental balances of nitrogen, phosphorus and major cations) and furthermore include the net cost of management as a trade-off component.
In the present study, we reassessed data collected from various field experiments aimed at investigating the impact of specific management practices on heathland nutrient balances. All studies were carried out between February 2001 and January 2003 within the “Lüneburger Heide Nature Reserve” (NW Germany; 53°15′N, 9°58′E, 105 m a.s.l.). This 234 km2 area contains approximately 43 km2 of (sub)Atlantic lowland heath (EUNIS habitat type F4.2, EU Habitats directive Annex I: 4030). Pleistocene sandy deposits and nutrient-poor podzols or podzolic soils characterize the area with topsoil pH(H2O) values ranging from 3.2 to 3.6. The climate (1989-2019) can be classified as humid suboceanic with a mean annual precipitation of 788 mm and a mean annual temperature of 9.38°C [Deutscher Wetterdienst (DWD), 2020].
The management practices considered in this study are representative of those performed by the Verein Naturschutzpark Lüneburger Heide (VNP), a non-profit conservation organization which owns most of the heathland area within the nature reserve, on a regular basis. An overview of current heathland management practices performed within our case study area including information on the equipment involved can be found on the VNP website1.
In the following, we briefly outline the heathland management practices considered in this study and the design of the corresponding field experiments on which our data are based. For a detailed description of each experiment and the analyses involved, we refer the reader to the original publications. Details on the measurement of nutrient fluxes are provided in section “Ecosystem Functions.” An overview of the average management frequency, the criteria for the implementation as well as the general aim of these management practices and the reference for the data used in this study is given in Table 1.
All field experiments were based on a split plot design with a control plot and an adjacent plot that received the management treatment. With the exception of grazing there were four 20 × 20 m replicate plots per treatment (management vs. control). All experimental sites had been unmanaged for at least 10 years prior to management.
Grazing within the study area is based on the traditional management system characteristic of the Lüneburger Heath in which shepherd assisted grazing takes place throughout the year (management frequency = 1 year; Table 1) with the traditional breed of sheep (German gray heath sheep, German: “Heidschnucke”) receiving no additional fodder, except during lambing time. In this system, the flock generally remains on the heath for roughly 8 h per day with the remainder of the time spent in a sheepfold or moving between the sheepfold and the grazing areas. The effects of grazing were studied in a 1-year experiment based on grazing with a 541 strong flock of German heath sheep at a stocking rate of 1.1 ewes ha–1 (Fottner et al., 2007). Within the 486 ha area to be grazed by the flock of sheep, 40 sample plots (2 × 1 m) were randomly selected and divided into two equally sized subplots, one of which was fenced-off to prevent grazing.
Mowing was performed with a mechanical mower which cut the aboveground biomass at a height of 0.1 m and can thus be considered as low-intensity mowing (Webb, 1998) and did not affect the organic soil layer (Härdtle et al., 2006). Compared to grasslands, however, such mowing can be considered to be intensive as Calluna vulgaris is reduced to its rootstock-near stem bases. Mowing frequency in heathlands strongly depends on the time dwarf shrubs need for regeneration. Depending on site conditions, regrowth of Calluna vulgaris often takes 8–10 years and between 5 and 15 years to return to the same life cycle stage (Schellenberg and Bergmeier, 2021). Therefore, mowing frequencies in dwarf shrub-dominated communities cannot be compared with (e.g.) grasslands, where mowing is applied as an annual management measure. The management frequency of mowing within our case study area is, on average, 10 years (Table 1).
The effects of prescribed burning were studied in a 2-year experiment (Niemeyer et al., 2005). Controlled burning was performed in the winter and thus burning treatment represents “low-temperature” fires that did not directly affect the organic horizon (Niemeyer et al., 2004). For the same reasons as mowing, the management frequency of burning within our case study area is, on average, 10 years.
The effects of choppering and sod-cutting were studied by Niemeyer et al. (2007). The aim of these intensive management practices is the complete removal of the aboveground vegetation together with the organic layer O-horizon (choppering) and sections of the A-horizon (sod-cutting). In many respects, such intensive interventions can be considered to be counter-intuitive to conservation management. However, such topsoil removal techniques are generally recognized as necessary (and subsequently subsidized) where restoring degraded heathlands is the main objective (Table 1). Accordingly, the management frequency of choppering and sod-cutting within our case study area is, on average, 15 and 30 years, respectively. It should also be noted that some controlled soil disturbance (or top soil removal) provides a niche for some characteristic heathland species (e.g., solitary sand nesting bees and wasps. In the study of Niemeyer et al. (2007) choppering and sod-cutting was performed using a tractor equipped with a sled; the material was then transferred to a trailer and transported to near-by arable fields where it was incorporated into the soil as an organic amendment.
The trade-off analysis in this study is based on socio-ecological components of heathlands that can be grouped into three categories: ecosystem functions, ecosystem services and associated costs. Heathlands provide an important habitat for many endangered species, in particular arthropods and birds (Webb et al., 2010) but due to a lack of detailed empirical data from our study sites, we were not able to include any measures of biodiversity as trade-off components. However, we argue that many of the species concerned are predominantly found in structurally rich heathland habitats and that, therefore, maintaining the structure and trophic status of heathlands is a pre-requisite for the provision of heathland biodiversity.
Due to their relevance in maintaining heathland integrity and vitality, and thereby providing suitable habitats for associated biodiversity, we included ecosystem functions related to the nutrient status of the system (elemental balances of nitrogen, phosphorus and major cations, i.e., potassium, calcium and magnesium) in our analysis. We provide below a detailed description of how we measured the elemental fluxes and how we calculated net annual elemental balances whilst a simple overview of the relevant fluxes and parameters used in the calculation of the net annual elemental balances is shown in Figure 1. The data used to calculate the nutrient balances is provided in the supporting information (Supplementary Table 1).
Figure 1. Nutrient fluxes included in the calculation of nutrient balances. Drawings of management practices used with permission from Antje Kayser.
Fluxes of nitrogen, phosphorus, potassium, calcium and magnesium due to deposition, removal of biomass and soil as well as losses to the groundwater (leaching) were previously assessed for all management practices. Annual deposition rates were taken from Härdtle et al. (2007) as these represent the deposition at the location of the respective experimental sites. Atmospheric deposition was determined using bulk deposition samples installed in close proximity to the treatment plots at a height of 1 m. Samples were collected every 2 weeks for a period of 1 year starting immediately after the management practices had taken place and corrected for underestimation of total deposition (Härdtle et al., 2006). Furthermore, where appropriate, the phosphorus content of the original samples was reanalyzed with a more sensitive machine allowing for a precise evaluation, rather than the previously presented maximum estimate (Härdtle et al., 2009).
With the exception of the sheep grazing experiment, the removal of nutrients in plant biomass and soil was achieved by comparing stocks prior to and directly after management had taken place. Biomass output via sheep grazing was analyzed separately for graminoids and Calluna vulgaris (hereafter, Calluna) as the foraging behavior of sheep changes over the course of a year. For Calluna, the above-mentioned split plot grazing exclosure design was applied and differences analyzed after a 1-year period. For the graminoids (e.g., Deschampsia flexuosa) grazing was quantified as the difference in biomass weight of randomly selected grazed and ungrazed subplots between June and September on a monthly basis. In the burning experiment, ash deposition was determined by analyzing the nutrient stocks of the O-horizon prior to and directly after management. As a result, nutrient removal from this layer was negative which indicates a net deposition of nutrients in ash.
Leaching data are based on lysimeter measurements, consisting of intact soil cores 1 m in length (soil depth) and 0.5 m in diameter (replicated four times). Nutrient losses due to leaching were determined by multiplying drainage volumes obtained from lysimeter measurements with concentration of seepage water collected in tension controlled porous soil solution samplers. With the exception of the sheep grazing experiment, where it was assumed that the effect of sheep grazing on leaching losses was negligible, leaching losses were also assessed in control plots. As our measurements took place in lowland heathlands (i.e., no slope inclination) as well as the coarse sandy texture of the soil, horizontal flow, which was not considered, can be assumed to be negligible.
It is important to note that, as leaching was based on data from the first year after management, the measured difference in leaching between the control and the treatment plots does not account for the recovery of the vegetation to a state similar to that prior to the management of the plots [“status quo ante” (sqa)]. As in the original studies, we, therefore, accounted for this reduction in additional leaching losses over time by assuming a linear decrease in leaching rates during vegetation regeneration as shown in Equation 1
where (kg ha–1 yr–1) is the amount of nutrient j leached from the managed plots 1 year after management took place, and sqa is the time period (years) to reach status quo ante which was 1 year for sheep grazing, 5 years for mowing and burning, 10 years for choppering and 15 years for sod-cutting.
The annual balance of nutrient j for a given management practice i was calculated using the following equation:
where is the annual atmospheric deposition of nutrient j (kg ha–1 yr–1), is the annual amount of nutrient j leached from the control plots (kg ha–1 yr–1), Rj is the loss of nutrient j due to the removal of biomass and soil (kg ha–1), (kg ha–1) is the deposition of nutrient j in year i coming from ash production (burning only), (kg ha–1) is the input of nutrient j in year i via sheep excrement (grazing only), MF is the management frequency in years (Supplementary Table 1) and is the additional amount of nutrient j leached from the plots exposed to a management practice compared to the control plots (kg ha–1) which is calculated according to the following equation (Equation 3):
In our case study area, the only provisioning services directly linked to management which generate an income are sheep products (wool and meat) and high quality mown heather material. We, therefore, included the net cost of management as a trade-off component. Whilst the sale of mown material greatly reduces the net costs, the income from the sale of meat and wool does not compensate for the high costs associated with shepherd-assisted year round sheep grazing (personal communication Heike Brenken, VNP).
The net costs include administration and capital costs but do not include subsidies such as CAP payments for grazing. One could argue that without such subsidies the products would be more expensive and would generate more income. However, the management practices performed are primarily aimed at achieving conservation goals and none of these activities actually generates a profit. Subsequently, the associated costs reflect the costs of habitat conservation, which as outlined above, is the main objective and, therefore, most relevant for the trade-off analysis in this study. Net annual management costs (Euro ha–1 yr–1) were calculated by dividing the net costs for individual management practices given in Müller, 2004 by the management frequency in years.
One of the most comprehensive analysis to-date of the natural environment in terms of the benefits it provides to society and its contribution to prosperity has been the UK’s National Ecosystem Assessment, which included a chapter on “Mountains, Moorlands and heaths” (van der Wal et al., 2011). Of the 32 ecosystem services that were considered only 20 are of direct relevance to heathlands. From the data available, we were able to quantitatively assess the effect of the management practices on the following four ecosystem services.
Groundwater recharge (GR) was calculated as the difference in leachate volumes between managed and control plots up until the vegetation returns to its original state (status quo ante) as described in Niemeyer et al. (2005). Annual groundwater recharge (GR) was calculated as the difference between groundwater recharge from the managed plots (GRtreatment) and the control plots (GRcontrol) until status quo ante divided by the management frequency (MF, provided in Table 1) of management practice in years (Equation 4) in order to obtain an annual rate.
As a proxy for groundwater quality (GQ), we used the increase in N leaching due to management as described for nutrient leaching in general (Equation 3) which was observed in all management practices with the exception of sheep grazing, where it was assumed that management had no effect on groundwater quality. As with groundwater recharge, annual groundwater quality was calculated by dividing by the management frequency of the respective management practice.
Data on the concentrations of carbon (C) in atmospheric deposition and leachate was not available. However, the magnitude of C within these in- and output sources can be considered to be negligible compared to the removal of C with biomass and soil horizons. Subsequently, the annual change in the C storage within the C stocks was calculated as the sum of C removed in the form of biomass and/or soil during the management practice divided by the management frequency in years. Carbon stocks were calculated based on N removal data and the C:N ratios of the respective compartment over all experimental plots which were derived from combined CN analyzes and were 43.4, 23.4, and 29.1 for aboveground biomass, O- and A-horizons, respectively (unpublished).
It should be noted that the trade-off component “carbon stocks” is not equivalent to C storage or C balance, as it does not include any post management changes in fluxes (or C:N ratios), e.g., ecosystem respiration or gross primary production; it purely reflects the immediate effect of a management practice on biomass and soil C stocks. Consequently, we discourage the interpretation of this parameter as an absolute measure of the effect of management practices on C storage. Furthermore, the values provided here should be treated with care due to the uncertainties related to estimation methods (e.g., the use of C:N ratios averaged over all experimental plots) and the resulting low reliabilities.
Heathlands are socially valued landscapes that provide an array of cultural ecosystem services ranging from tourism and recreation to spiritual, religious, aesthetic and educational opportunities (van der Wal et al., 2011). Unfortunately, data on the effect of management practices on such services is very limited. We were, however, able to use data from a survey conducted between September 2001 and April 2003 in which visitors to the Lüneburger Heath Nature Reserve rated the effect of individual management practices on their visual appreciation of the heath (Müller, 2004).
A detailed description of how net annual appreciation was calculated is given in the supporting information. Briefly, the data is based on responses of interviewees to two pictures depicting the respective management practice directly after its implementation and 2 years thereafter. Using these responses, which were given on an ordinal scale (1 = “very attractive” to 5 = “very unattractive”), we calculated the yearly appreciation until year 5 when it was assumed that a “baseline” appreciation had been reached, i.e., the situation when the effect of management practice on area surfaces is no longer distinguishable for the interviewees. Net annual appreciation incorporated the sum of appreciation until baseline appreciation as well as the management frequency (Supplementary Equation 1).
To be able to compare components of the trade-off analysis (functions, services and costs) we standardized the data prior to further processing (visualization and quantification of trade-offs) following Bradford and D’Amato (2012) in which the standardized component (i.e., the “relative benefit”) is calculated as:
where TCstd is the standardized trade-off component value, TCobs is the observed value based on the mean over all replications per management practice and TCmax and TCmin are the maximum and minimum observed values of the given trade-off component across all management practices, respectively. Table 2 gives an overview of the trade-off components prior to standardization whilst absolute values of trade-off components prior to standardization are provided in the supporting information (Supplementary Table 3).
In this process the values of TCstd can range from 0 (lowest observed value) to 1 (highest observed value). If the desirable outcome is associated with a greater observed value, then a TCstd of 1 reflects the most desirable outcome. However, where the desirable outcome is associated with a lower observed value, TCstd needs to be subtracted from 1 to make the highest TCobs values the least desirable so that all TCstd values reflect the “relative benefit” as described above.
As can be seen from Table 2 a greater value (within a certain limit) is desirable for both the cation and phosphorus balance but not the nitrogen balance. This is because a loss of cations and phosphorus is detrimental to the stability of the system, due to both losses of nutrients and a reduction in cation exchange sites whilst the main aim of the management measures addressed in this study is to remove excess N from this naturally N-limited system. A greater value is also desirable for groundwater recharge, as the groundwater below the study area is a crucial source of fresh water for the nearby city of Hamburg.
Following the approach of Bradford and D’Amato (2012) we used the root mean square deviation (RMSD) to quantify trade-offs between TCstd (Equation 6). In Equation 6, RMSDi is the value calculated for management i, n is the total number of trade-off components considered for the calculation (n = 2 for pairwise combinations, n = 8 for RMSDtotal), TCstd,i is the standardized trade-off component value for management i, and TCstd,i is the average standardized trade-off component value calculated for management i using the n trade-off components under consideration. Besides calculating RMSD values for each of the possible 28 trade-off component combinations, we calculated the total trade-off (RMSDtotal) by using all of the TCstd in Equation 6.
In a two-dimensional space (i.e., pairwise comparison of two standardized trade-off components), the situation where there is no trade-off can be visualized by the 1:1 line whilst a move away from this line represents a trade-off. We refer the reader to Bradford and D’Amato (2012) for a graphical illustration. Accordingly, the RMSD values can range from zero (no trade-off, i.e., TCstd of both components are identical) to 0.71 (maximum trade-off where TCstd of one component is 0 and the other is 1).
Trade-offs between and within management practices were visualized by showing the relative benefit of all trade-off components for each management practice in radial bar plots and the RMSD values for each management practice in violin plots using the R package “ggplot2” (Wickham, 2016). Furthermore, we quantified relationships between TCstd using the Pearson correlation coefficient for each pairwise comparison. Based on the resulting correlation matrix we identified trade-off components that shared common drivers.
Our findings clearly indicate that all management practices have advantages but also disadvantages (Figure 2). Each management practice showed the most desirable outcome (relative benefit of 1) for at least one trade-off component, with the exception of sod-cutting where the highest relative benefit was 0.98 for groundwater recharge. In a similar manner, all management practices showed the least desirable outcome (relative benefit of 0) for at least one trade-off component, with the exception of mowing, where the lowest relative benefit was 0.09 for the N balance.
Figure 2. Relative benefits and trade-offs associated with heathland management practices. (A) Segment diagrams showing the relative benefit of trade-off components within and between management practices. (B) Violin plots depicting the trade-offs for all pairwise component comparisons (circles) calculated as the residual mean square deviation (RMSD, section “Trade-Off Analysis”) and the corresponding density distribution (gray shading) for individual management practices. The total trade-off (RMSDtotal), i.e., when all components are considered together, is shown as a dashed line. Pairwise comparisons that do not include the N balance (Nbal) are shown as open circles whilst those that are beneficial for the N balance and those where the benefit is not in favor of the N balance are shown as green and dark blue circles, respectively. Abbreviations as in Table 2.
The area filled by segments in Figure 2A gives an indication of how well a management practice performs over all components assessed. We found that choppering and sod-cutting had the lowest average relative benefits (0.30 and 0.37, respectively) with the individual trade-off components showing a very uneven distribution pattern. Choppering and sod-cutting were by far the most efficient practices at removing N and increasing groundwater recharge. However, the relative benefits for all other components were the lowest, with the exception of burning which was even less appreciated. Consequently, the distribution pattern of pairwise trade-off comparisons, as shown in the violin plots in Figure 2B, was dumbbell shaped indicating many very small and very large trade-offs, the latter being in favor of the N balance and groundwater recharge. The extent of this distribution, however, was less pronounced for sod-cutting as the relative benefits for the N balance and groundwater recharge were slightly lower and those for all other components slightly larger than for choppering. As a result choppering was the management practice with the largest total trade-off (RMSDtotal = 0.45). In contrast, sod-cutting was the management practice with the second lowest total trade-off (RMSDtotal = 0.35).
Apart from being the most appreciated management practice by visitors, sheep grazing, as opposed to all other practices, did not affect groundwater quality, i.e., no increase in N leaching (Supplementary Table 3). At the same time, it showed the lowest relative benefit for groundwater recharge and the cation balance. All other relative benefits ranged from 0.06 (Costs) to 0.47 (Cstocks). As a result, the average relative benefit (0.39) was only slightly higher than that of sod-cutting but the pairwise RMSD values were fairly evenly distributed although slightly shifted toward lower values (highest distribution density at RMSD ∼0.18, Figure 2B) leading to an intermediate total trade-off value (RMSDtotal = 0.41).
The pairwise trade-off comparisons (RMSD values) for burning were relatively evenly distributed although, in this case, slightly shifted toward intermediate values (highest distribution density at RMSD ∼0.38) leading to a total trade-off which was only slightly lower than that found for choppering (RMSDtotal = 0.44). In comparison to sheep grazing, however, burning provided more relative benefit for five out of the eight trade-off components assessed and the average relative benefit for burning (0.58) was, therefore, considerably higher than that of grazing. Interestingly, apart from removing the least carbon stocks, burning was the only management practice capable of retaining cations in the system and had an almost balanced P budget (Supplementary Table 3). Burning was, therefore, the most beneficial management practice for these trade-off components. In addition, it was less expensive than all other management practices with the exception of mowing. At the same time, it was the least appreciated management type and, together with mowing, the only practice which was not able to compensate N inputs thus leading to an accumulation of N in the system (N balance of 13.3 and 7.5 kg N ha–1 yr–1 for burning and mowing, respectively). It should be noted, however, that slightly negative N balance for grazing (Supplementary Table 3) is based on a stocking density of 1.1 ewes ha–1. Due to the observed effects of overgrazing this value has decreased over the years and is now at ∼ 0.8 ewes ha–1 (personal communication Dirk Mertens, VNP) which would reflect a positive N balance.
Apart from showing the highest relative benefit for costs, the second highest relative benefit for carbon stocks (TCstd = 0.97) and a very low relative benefit for the N balance (TCstd = 0.09) the relative benefit for all other trade-off components for mowing was between 0.5 and 0.77. Accordingly, mowing displayed the highest average relative benefit of all management practices (0.65). In addition, there were only few very low and very high trade-offs for mowing with the majority of RMSD values (64%) lying between 0.14 and 0.36. Consequently, mowing showed the lowest total trade-off of all management practices (RMSDtotal = 0.30).
The effect of a management practice on trade-off components can be affected by various drivers. In our study the effect of a management practice on trade-off components often followed a pattern which was related to the amount of biomass/soil removed during a management cycle (Rannual: choppering > sod-cutting > > sheep grazing > > burning ≈ mowing). This is the amount removed during the application of a management practice divided by the management frequency. Rannual was the main factor determining the relative benefits of trade-off components related to elemental balances (Nbal, Pbal, Cationbal, and Cstocks). Consequently, these components were highly correlated with each other (Figure 3). As one of the main goals of current management practices is to remove N, whilst simultaneously reducing losses of phosphorus, carbon and cations, a large relative benefit for the N balance will, therefore, automatically result in a low benefit for the other trade-off components related to elemental balances.
Figure 3. Pairwise comparisons between TCstd. The upper panel shows the relative benefits (TCstd) between all possible pairwise trade-off component comparisons. The 1:1 line represents the situation where there is no trade-off between the two TCstd being compared, whilst a move away from this line represents a trade-off. The diagonal from top left to bottom right (slope of −1, intercept zero) represents the line of average benefit (not shown). All values below this line represent a situation where the management practice provides less benefit with regard to the two TC compared, whilst any management practice above this line provides more benefit. In the lower panel, the Pearson correlation coefficients are shown scaled according to their absolute value. Abbreviations as in Table 2.
Unsurprisingly, groundwater quality was highly negatively correlated with groundwater recharge (Figure 3). As a result, management practices with large relative benefits for groundwater recharge had low relative benefits for groundwater quality and vice versa. Furthermore, with the exception of sheep grazing, which was assumed to have no impact and, therefore, had the highest relative benefit for groundwater quality and the lowest relative benefit for groundwater recharge, the effect of a management practice on leaching components (GR, GQ) considered in this study was also closely related to Rannual.
As removing biomass/soil from site is a major expenditure, net costs were also strongly correlated with the trade-off components related to Rannual and consequently the elemental balances (Figure 3).
Based on our experience and evidence from the literature we expected there to be inherent trade-offs between management practices (Calvo et al., 2005, 2015; Alonso and Härdtle, 2015; Cordingley et al., 2015, 2016). Accordingly, our analysis shows that there is no “best” management practice. This in itself is, however, an important finding as land managers need to be aware that, in trying to solve one problem, they may be creating another.
Our analysis clearly shows that most of the trade-off components considered here are highly correlated due to shared drivers. Whilst this was mainly the amount of biomass/soil removed during a management cycle (Rannual), the relative proportion of nutrients within inputs and outputs to the system had a pronounced effect on the phosphorus and cation balance for grazing and burning. For sheep grazing this is due to the disproportionally high animal uptake of phosphorus and cations compared to their return to the system in the form of excrements whilst for burning 77% of phosphorus and 79% of cations combusted were returned via ash deposition (Supplementary Table 1). In addition, nutrient leaching to the groundwater was elevated after burning due to a transient increase in nutrient availability in the organic horizon in combination with reduced plant uptake (Mohamed et al., 2007).
By knowing the negative impacts and the drivers we can try to develop novel management practices or adopt implementation strategies that can lead to more benefits and less disadvantages. Subsequently, in absence of adequate reductions in atmospheric N deposition, novel or adapted management measures should strive to find practical and cost effective ways of removing N from the system whilst simultaneously removing less of the other elements. This is only possible if system compartments are targeted that have a high N content in comparison to other elements. It has recently been shown that the moss layer is the main system compartment for N entering the system via deposition, at least in the short to medium term (Bähring et al., 2017a). Furthermore, in a heathland N fertilization experiment the C:N ratio of broom forkmoss (Dicranum scoparium) decreased continuously above an input of 10 kg N ha–1 yr–1 (Bähring et al., 2017b). Therefore, the removal of thick moss layers (‘‘scarification’’) consisting mainly of common mosses such as Hypnum cupressiforme and Dicranum scoparium has been identified as an adaptive management measure. Scarification has been adopted by the site managers within our study area as a routinely performed management practice and research is currently underway to assess its impact on biodiversity, nutrient balances, selected ecosystem services and gaseous emissions2.
One limitation of our study is that it does not include spatial and temporal aspects of management (Rodriguez et al., 2006; Holland et al., 2011; Obiang Ndong et al., 2020), i.e., multiple management cycles and management combinations. This is especially true when modeling the nutrient availability in heathlands, which should ideally be examined from a dynamic and functional perspective rather than a static or budget-based approach (Chapman et al., 1989). However, detailed data on biogeochemical cycles would be required, which remain limited for European shrublands (Beier et al., 2009). In addition, the underlying complexity and the resulting large uncertainties associated with such a task is probably the reason why such studies are scarce (Britton et al., 2001; Terry et al., 2004). Trade-off analyses such as the one presented here, which are based on quantitative, empirical data and include both functions and services can, however, form the basis for future scenario modeling. This, in turn, may help in identifying optimal management combinations over space and time, which will inevitably be more beneficial and reduce trade-offs for both functions and services than management practices considered in isolation. At the same time, management combinations, if performed on a small scale, are beneficial for biodiversity as they lead to greater structural variation of the vegetation within a given area (Usher and Thompson, 1993; Vandvik et al., 2005; Schirmel, 2010; Diaz et al., 2013). Based on our results, we suggest that after restoring degraded heathlands by means of choppering or sod-cutting, a combination of grazing and burning would have the most desirable effects on relative benefits of the trade-off components considered here and would also decrease trade-offs compared to individual management practices.
Our analysis helps identify common drivers and, therefore, underlying processes that need to be addressed when considering novel management practices or management combinations. But an important question remains unanswered: how can management decision frameworks that include evaluation and trade-off analyses help site managers decide on which management practice to apply? If the average relative benefit is considered as an indicator of overall performance, then one would conclude that mowing (0.65) is the management of choice, followed by burning (0.58) whereas sheep grazing and sod-cutting and particularly choppering (0.39, 0.37, and 0.30, respectively) would be far less desirable. This approach, however, does not account for trade-offs between functions, services and costs that, as shown above, are inevitable.
As there was no clear relationship between RMSDtotal and the average relative benefit of management practices (R2 of 0.23) a large average relative benefit does not necessarily lead to low trade-offs. In fact, the RMSDtotal for burning was almost identical to that of choppering and that of sod-cutting close to mowing. In our case study, however, mowing, which had the lowest total trade-off (42% of the maximum possible value), also had the highest average relative benefit. Furthermore, sod-cutting which had the highest total trade-off (63% of the maximum possible value) also had the lowest average relative benefit. Therefore, one could conclude that for our case study area, mowing is the “best” and choppering is the “worst” management option. There are several reasons why this is clearly not the case.
First, our analysis is restricted to a subset of ecosystem functions and services and does not include direct or indirect effects on biodiversity such as direct losses due to management or indirect effects via changes in vegetation structure, nutrient availability or species re-establishment (Usher and Thompson, 1993; Streitberger et al., 2021; Vogels et al., 2021). Secondly, there may be limitations to which management practices can be employed that are unrelated to ecosystem functioning, e.g., the protection of cultural heritage as well as local concerns or traditions. Thirdly, management needs to be considered in the context of resource availability (e.g., costs, time, equipment, personnel etc.).
In the context of this study, however, the most important issue is related to the approach itself. The min/max standardization procedure used here has been put forward as a way of comparing trade-off components that differ in units and scales (Bradford and D’Amato, 2012). However, without any weighting or other adjustments (see e.g., Manning et al., 2018) the quantification of RMSDtotal assumes that all trade-offs components are equally important. In addition, absolute differences within a given component are not considered which is of relevance when biophysical or socio-economic thresholds or critical values apply (Cavender-Bares et al., 2015; King et al., 2015). As an example, the removal of N from the system should ideally lead to a balanced budget (inputs are equal to outputs) but at the very least these should be under a “critical load.” For dry heaths such an effect threshold for N deposition has been estimated to lie between 10 and 20 kg N ha–1 yr–1 above which a transition from heather to grass dominated systems is likely to occur (Bobbink et al., 2010). Critical values may also be related to nutrient stoichiometry which can have an effect on both flora and fauna (Härdtle et al., 2009; von Oheimb et al., 2010; Vogels et al., 2017, 2020; Siepel et al., 2018). The same holds true for ecosystem services (Cavender-Bares et al., 2015; Deng et al., 2016). An example is the maximum acceptable groundwater nitrate concentration as outlined in the water frameworks directive of the European Parliament and of the Council (Directive 2000/60/EC).
In addition to threshold values, the balance between supply and demand, both in time and space, needs to be considered when valuing ecosystem services (Cimon-Morin et al., 2014; Mouchet et al., 2014; Martinez-Harms et al., 2015; Ament et al., 2017). For example, it can be assumed that the demand for the provision of high quality groundwater will increase as the population of Hamburg continues to grow. Under the scenario that per capita consumption remains unaltered, the demand will continue to increase if the climate becomes drier as has been predicted (Wagner et al., 2013; May et al., 2016).
The carbon sequestration potential of our case study area, in contrast has not received much attention to date, as the contribution of heathlands to the overall land-based carbon stock in Germany is small. In the UK, however, where the area covered by heathlands is large, the climate mitigation potential of (mainly upland) heaths is a key ecosystem service and has subsequently received much attention (Quin et al., 2015). Recent reviews (Alonso et al., 2021; Gregg et al., 2021) concluded that most of the carbon in heathland systems is in the soil (98%), with a small proportion in the vegetation (2%). We would like to reiterate, that the trade-off component C stocks is an estimate of the amount of carbon removed during management and is, therefore, not a precise indicator of the C balance (or C storage potential) over a full management cycle. This is because not all in- and outputs are included and more importantly it does not incorporate management driven changes in C cycling post management. However, we believe that such post management effects are small compared to the amounts of carbon exported due to biomass and/or soil removal. Nonetheless, to prevent carbon emissions, the best management options are those that result in the lowest soil disturbance and ensure that the habitat is restored or in good condition (Gregg et al., 2021).
Whilst it is apparent that the interpretation of within component differences needs to be based on absolute values and set in a local context, the main objective of our study was to identify management-related trade-offs amongst a set of functions and services, which are, as shown, unavoidable. The question at hand is, which trade-offs are we willing to accept, or put differently, where do our priorities lie? The answer depends on the objective and this will differ between sites, individuals and stakeholder groups. These are important decisions that need to be discussed in a wider societal context. Whilst this debate is beyond the scope of the study, the results presented here can help this discourse by making stakeholders and the public aware of the facts and, thereby, providing the basis for informative inclusion (Gray et al., 2020). This may contribute to “relational thinking,” which has been put forward as a novel approach in sustainability science, moving the focus more toward continually unfolding processes and relations and away from interactions between entities (West et al., 2020) and thus bridging gaps between actors (Fischer et al., 2020). It is possible that the normative value given to an ecosystem or landscape by an individual is not primarily based on the perceived benefit but the fear of losing a valuable relationship. This is supported by a recent finding that suggests that the willingness to donate to conservation is highest when protection of nonhuman nature for its own sake is conferred, at the least as part of the message (Batavia et al., 2018).
We clearly show that despite the large trade-off between N removal and all other components with the exception of groundwater recharge, high-intensity measures such as choppering are currently required to ensure that the Lüneburger Heath is preserved in the long-term. Without such measures, the competitive advantage of Calluna and other species that are adapted to these highly N-limited habitats would be lost and the vegetation would gradually change to a grass-dominated system. Other studies from different heathlands have come to similar conclusions and, despite numerous negative effects of these intensive measures (as shown here), have highlighted the need for their implementation (Power et al., 1998; Barker et al., 2004; van der Bij et al., 2017).
Interestingly, it has been suggested that intensive measures may not be required in heathlands where N deposition rates are lower than in our study region, such as toward the more eastern and southern margins of the European heathland distribution range (Sedláková and Milan, 1999; Seifert et al., 2015; Probo et al., 2017) as well as the Baltic Sea coast (Blindow et al., 2017). This highlights the need for trans-national reductions in N deposition rates, which will benefit N-limited semi- and natural habitats alike.
Despite the availability of management options that are able to counteract this airborne N enrichment, urgency is required as it has been shown that the interaction of increased N availability and climate change can have negative and non-additive effects on heathland vitality (Fagúndez, 2013; Meyer-Grünefeldt et al., 2013). Furthermore, this interaction may depend on Calluna life-stage and provenance (Meyer-Grünefeldt et al., 2016; Ibe et al., 2020). Future heathland management assessments should, therefore, include the generative rejuvenation capacity as a trade-off component which requires the presence of mineral soil horizons (Henning et al., 2017; Ibe et al., 2020; Schellenberg and Bergmeier, 2020; Walmsley and Härdtle, 2021).
We conclude that the trade-off analysis in the context of this study is capable of highlighting advantages and disadvantages of management practices but average relative benefits or RMSD values should not be used as sole metrics upon which decision should be made. Instead, in cases where the non-prioritization of certain functions will ultimately result in the loss of a protected habitat and the service potential they provide, a prioritization of ecosystem functioning over ecosystem services in decision-making frameworks is required.
The formulation of objectives is, therefore, a prerequisite for decision-making processes. However, evidence-based insights are necessary in order to successfully integrate multifunctionality into land-management, which has been identified as a key step in providing improved insights into ecosystem functioning which can then guide ecosystem management (Manning et al., 2018). We are aware that the availability of data will, in most cases, limit the extent to which site managers can perform trade-off analyses like the one described here. However, the method involved is rather straight forward and can be applied to both continuous as well as ordinal data. Despite the complexity in interpreting the results and the large efforts required in gathering data on ecosystem functioning (such as nutrient balances), we encourage all stakeholders to consider its merits but also to be aware of possible limitations that could lead to incorrect conclusions.
The original contributions presented in the study are included in the article/Supplementary Material, further inquiries can be directed to the corresponding author/s.
WH, IA, and DW conceived the study. DW and WH collected the data. BD and DW performed the analyses. DW, WH, VT, and BD decided on which analyses to include. DW led the writing of the manuscript. All authors contributed critically to the drafts and gave final approval for publication.
DW was funded by the cooperative project with the title “Sicherung der Ökosystemdienstleistungen und Biodiversität von extensiv bewirtschafteten Kulturlandschaften (ÖkoKult)” which was co-funded by the Federal Agency for Nature Conservation (BfN) with resources from the Federal Ministry for the Environment, Nature Conservation and Nuclear Safety (BMU) (grant FKZ 3514685A21) and the Federal Ministry of Education and Research (BMBF) (grant FKZ 16LC1321) within the joint framework “Research on the Implementation of the National Strategy on Biodiversity”(F&U-NBS).
The authors declare that the research was conducted in the absence of any commercial or financial relationships that could be construed as a potential conflict of interest.
All claims expressed in this article are solely those of the authors and do not necessarily represent those of their affiliated organizations, or those of the publisher, the editors and the reviewers. Any product that may be evaluated in this article, or claim that may be made by its manufacturer, is not guaranteed or endorsed by the publisher.
We would like to thank the VNP for their involvement in the original experiments and in particular Heike Brenken and Dirk Mertens for their valuable discussions on operational management within the study area. Finally, we would like to thank Antje Kayser for granting us with the permission to use her management practices drawings in Figure 1.
The Supplementary Material for this article can be found online at: https://www.frontiersin.org/articles/10.3389/fevo.2021.791364/full#supplementary-material
Alonso, I., Chamberlain, B., Fagundez, J., Glaves, D., and Smedley, M. (2021). “Heathlands,” in Nature-Based Solutions for Climate Change in the UK: A Report by the British Ecological Society, eds R. Stafford, B. Chamberlain, L. Clavey, P. K. Gillingham, S. McKain, M. D. Morecroft, et al. (London), 38–48.
Alonso, I., and Härdtle, W. (2015). Resolving potential conflicts between different heathland ecosystem services through adaptive management. Ecol. Quest. 21, 101–103. doi: 10.12775/eq.2015.017
Ament, J. M., Moore, C. A., Herbst, M., and Cumming, G. S. (2017). Cultural ecosystem services in protected areas: understanding bundles, trade-offs, and synergies. Conserv. Lett. 10, 439–449. doi: 10.1111/conl.12283
Bähring, A., Fichtner, A., Friedrich, U., von Oheimb, G., and Härdtle, W. (2017a). Bryophytes and organic layers control uptake of airborne nitrogen in low-N environments. Front. Plant Sci. 8:2080. doi: 10.3389/fpls.2017.02080
Bähring, A., Fichtner, A., Ibe, K., Schütze, G., Temperton, V. M., von Oheimb, G., et al. (2017b). Ecosystem functions as indicators for heathland responses to nitrogen fertilisation. Ecol. Indic. 72, 185–193. doi: 10.1016/j.ecolind.2016.08.013
Barker, C. G., Power, S. A., Bell, J. N. B., and Orme, C. D. L. (2004). Effects of habitat management on heathland response to atmospheric nitrogen deposition. Biol. Conserv. 120, 41–52. doi: 10.1016/j.biocon.2004.01.024
Batavia, C., Bruskotter, J. T., Jones, J. A., Vucetich, J. A., Gosnell, H., and Nelson, M. P. (2018). Nature for whom? How type of beneficiary influences the effectiveness of conservation outreach messages. Biol. Conserv. 228, 158–166. doi: 10.1016/j.biocon.2018.10.029
Beier, C., Emmett, B. A., Tietema, A., Schmidt, I. K., Peñuelas, J., Láng, E. K., et al. (2009). Carbon and nitrogen balances for six shrublands across Europe. Global Biogeochem. 23:381. doi: 10.1029/2008GB003381
Berendse, F., Schmitz, M., and de Visser, W. (1994). Experimental manipulation of succession in heathland ecosystems. Oecologia 100, 38–44. doi: 10.1007/BF00317128
Blindow, I., Gauger, D., and Ahlhaus, M. (2017). Management regimes in a coastal heathland-effects on vegetation, nutrient balance, biodiversity and gain of bioenergy. J. Coast. Conserv. 21, 273–288. doi: 10.1007/s11852-017-0499-3
Bobbink, R., Hicks, K., Galloway, J., Spranger, T., Alkemade, R., Ashmore, M., et al. (2010). Global assessment of nitrogen deposition effects on terrestrial plant diversity: a synthesis. Ecol. Appl. 20, 30–59. doi: 10.1890/08-1140.1
Bradford, J. B., and D’Amato, A. W. (2012). Recognizing trade-offs in multi-objective land management. Front. Ecol. Environ. 10:210–216. doi: 10.1890/110031
Britton, A. J., Pakeman, R. J., Carey, P. D., and Marrs, R. H. (2001). Impacts of climate, management and nitrogen deposition on the dynamics of lowland heathland. J. Veg. Sci. 12, 797–806. doi: 10.2307/3236867
Brown, C., Walpole, M., Simpson, L., and Tierney, M. (2011). “Introduction to the UK national ecosystem assessment,” in The UK National Ecosystem Assessment Technical Report, (Cambridge: UK National Ecosystem Assessment, UNEP-WCMC). Available online at: http://uknea.unep-wcmc.org/LinkClick.aspx?fileticket=BNpVOJWKNxA%3d&tabid=82.
Brunsting, A. M. H., and Heil, G. W. (1985). The role of nutrients in the interactions between a herbivorous beetle and some competing plant species in heathlands. Oikos 44, 23–26. doi: 10.2307/3544038
Calvo, L., Alonso, I., Fernàndez, A. J., and De Luis, E. (2005). Short-term study of effects of fertilisation and cutting treatments on the vegetation dynamics of mountain heathlands in Spain. Plant Ecol. 179, 181–191. doi: 10.1007/s11258-004-7511-3
Calvo, L., Huerta, S., Marcos, E., Calvo-Fernández, J., and Taboada, A. (2015). The role of prescribed fire in the provision of regulating ecosystem services of Spanish heathlands. Ecol. Quest. 21, 71–73. doi: 10.12775/EQ.2015.012
Cavender-Bares, J., Polasky, S., King, E., and Balvanera, P. (2015). A sustainability framework for assessing trade-offs in ecosystem services. Ecol. Soc. 20:117. doi: 10.5751/ES-06917-200117
Chapman, S. B., Rose, R. J., and Clarke, R. T. (1989). A model of the phosphorus dynamics of calluna heathland. J. Ecol. 77, 35–48. doi: 10.2307/2260914
Cimon-Morin, J., Darveau, M., and Poulin, M. (2014). Towards systematic conservation planning adapted to the local flow of ecosystem services. Glob. Ecol. Conserv. 2, 11–23. doi: 10.1016/j.gecco.2014.07.005
Cord, A. F., Bartkowski, B., Beckmann, M., Dittrich, A., Hermans-Neumann, K., Kaim, A., et al. (2017). Towards systematic analyses of ecosystem service trade-offs and synergies: main concepts, methods and the road ahead. Ecosyst. Serv. 28, 264–272. doi: 10.1016/j.ecoser.2017.07.012
Cordingley, J. E., Newton, A. C., Rose, R. J., Clarke, R. T., and Bullock, J. M. (2015). Habitat fragmentation intensifies trade-offs between biodiversity and ecosystem services in a heathland ecosystem in Southern England. PLoS One 10:e0130004. doi: 10.1371/journal.pone.0130004
Cordingley, J. E., Newton, A. C., Rose, R. J., Clarke, R. T., and Bullock, J. M. (2016). Can landscape-scale approaches to conservation management resolve biodiversity–ecosystem service trade-offs? J. Appl. Ecol. 53, 96–105. doi: 10.1111/1365-2664.12545
Deng, X., Li, Z., and Gibson, J. (2016). A review on trade-off analysis of ecosystem services for sustainable land-use management. J. Geogr. Sci. 26, 953–968. doi: 10.1007/s11442-016-1309-9
Diaz, A., Keith, S. A., Bullock, J. M., Hooftman, D. A. P., and Newton, A. C. (2013). Conservation implications of long-term changes detected in a lowland heath plant metacommunity. Biol. Conserv. 167, 325–333. doi: 10.1016/j.biocon.2013.08.018
Doorenbosch, M., and van Mourik, J. M. (2016). The impact of ancestral heath management on soils and landscapes: a reconstruction based on paleoecological analyses of soil records in the central and southeastern Netherlands. Soil 2, 311–324. doi: 10.5194/soil-2-311-2016
Fagúndez, J. (2013). Heathlands confronting global change: drivers of biodiversity loss from past to future scenarios. Ann. Bot. 111, 151–172. doi: 10.1093/aob/mcs257
Fischer, J., Riechers, M., Loos, J., Martin-Lopez, B., and Temperton, V. M. (2020). Making the UN decade on ecosystem restoration a social-ecological endeavour. Trends Ecol. Evol. 36, 20–28. doi: 10.1016/j.tree.2020.08.018
Fottner, S., Hardtle, W., Niemeyer, M., Niemeyer, T., von Oheimb, G., Meyer, H., et al. (2007). Impact of sheep grazing on nutrient budgets of dry heathlands. Appl. Veg. Sci. 10, 391–398. doi: 10.1111/j.1654-109X.2007.tb00438.x
Gordon, C., Woodin, S. J., Mullins, C. E., and Alexander, I. J. (1999). Effects of environmental change, including drought, on water use by competing Calluna vulgaris (heather) and Pteridium aquilinum (bracken). Funct. Ecol. 13, 96–106. doi: 10.1046/j.1365-2435.1999.00012.x
Gray, S., Aminpour, P., Reza, C., Scyphers, S., Grabowski, J., Murphy, R. Jr., et al. (2020). Harnessing the collective intelligence of stakeholders for conservation. Front. Ecol. Environ. 18:465–472. doi: 10.1002/fee.2232
Gregg, R., Elias, J. L., Alonso, I., Crosher, I. E., Muto, P., and Morecroft, M. D. (2021). Carbon Storage and Sequestration by Habitat: A Review of the Evidence Natural England Research Report NERR094.
Härdtle, W., Niemeyer, M., Niemeyer, T., Assmann, T., and Fottner, S. (2006). Can management compensate for atmospheric nutrient deposition in heathland ecosystems? J. Appl. Ecol. 43, 759–769. doi: 10.1111/j.1365-2664.2006.01195.x
Härdtle, W., Von Oheimb, G., Gerke, A. K., Niemeyer, M., Niemeyer, T., Assmann, T., et al. (2009). Shifts in N and P budgets of heathland ecosystems: effects of management and atmospheric inputs. Ecosystems 12, 298–310. doi: 10.1007/s10021-008-9223-3
Härdtle, W., Von Oheimb, G., Niemeyer, M., Niemeyer, T., Assmann, T., and Meyer, H. (2007). Nutrient leaching in dry heathland ecosystems: effects of atmospheric deposition and management. Biogeochemistry 86, 201–215. doi: 10.1007/s10533-007-9156-5
Heil, G. W., and Diemont, W. H. (1983). Raised nutrient levels change heathland into grassland. Vegetatio 53, 113–120. doi: 10.1007/BF00043031
Henning, K., von Oheimb, G., Härdtle, W., Fichtner, A., and Tischew, S. (2017). The reproductive potential and importance of key management aspects for successful Calluna vulgaris rejuvenation on abandoned continental heaths. Ecol. Evol. 7, 2091–2100. doi: 10.1002/ece3.2816
Holland, R. A., Eigenbrod, F., Armsworth, P. R., Anderson, B. J., Thomas, C. D., and Gaston, K. J. (2011). The influence of temporal variation on relationships between ecosystem services. Biodivers. Conserv. 20, 3285–3294. doi: 10.1007/s10531-011-0113-1
Ibe, K., Walmsley, D., Fichtner, A., Coners, H., Leuschner, C., and Härdtle, W. (2020). Provenance- and life-history stage-specific responses of the dwarf shrub Calluna vulgaris to elevated vapour pressure deficit. Plant Ecol. 221, 1219–1232. doi: 10.1007/s11258-020-01076-3
Jones, A. G., and Power, S. A. (2012). Field-scale evaluation of effects of nitrogen deposition on the functioning of heathland ecosystems. J. Ecol. 100, 331–342. doi: 10.1111/j.1365-2745.2011.01911.x
King, E., Cavender-Bares, J., Balvanera, P., Mwampamba, T. H., and Polasky, S. (2015). Trade-offs in ecosystem services and varying stakeholder preferences: evaluating conflicts, obstacles, and opportunities. Ecol. Soc. 20:325. doi: 10.5751/ES-07822-200325
Manning, P., van der Plas, F., Soliveres, S., Allan, E., Maestre, F. T., Mace, G., et al. (2018). Redefining ecosystem multifunctionality. Nat. Ecol. Evol. 2, 427–436. doi: 10.1038/s41559-017-0461-7
Martinez-Harms, M. J., Bryan, B. A., Balvanera, P., Law, E. A., Rhodes, J. R., Possingham, H. P., et al. (2015). Making decisions for managing ecosystem services. Biol. Conserv. 184, 229–238. doi: 10.1016/j.biocon.2015.01.024
May, W., Ganske, A., Leckebusch, G. C., Rockel, B., Tinz, B., and Ulbrich, U. (2016). “Projected change—atmosphere,” in North Sea Region Climate Change Assessment, eds M. Quante and F. Colijn (Cham: Springer International Publishing), 149–173. doi: 10.1007/978-3-319-39745-0_5
Meyer-Grünefeldt, M., Belz, K., Calvo, L., Marcos, E., Oheimb, G., and Härdtle, W. (2016). Marginal Calluna populations are more resistant to climate change, but not under high-nitrogen loads. Plant Ecol. 217, 111–122. doi: 10.1007/s11258-015-0563-8
Meyer-Grünefeldt, M., Friedrich, U., Klotz, M., Von Oheimb, G., and Härdtle, W. (2013). Nitrogen deposition and drought events have non-additive effects on plant growthevidence from greenhouse experiments. Plant Biosyst. 3504, 1–9. doi: 10.1080/11263504.2013.853699
Mohamed, A., Härdtle, W., Jirjahn, B., Niemeyer, T., and Von Oheimb, G. (2007). Effects of prescribed burning on plant available nutrients in dry heathland ecosystems. Plant Ecol. 189, 279–289. doi: 10.1007/s11258-006-9183-7
Moore, D. W., Booth, P., Alix, A., Apitz, S. E., Forrow, D., Huber-Sannwald, E., et al. (2017). Application of ecosystem services in natural resource management decision making. Integr. Environ. Assess. Manag. 13, 74–84. doi: 10.1002/ieam.1838
Mouchet, M. A., Lamarque, P., Martín-López, B., Crouzat, E., Gos, P., Byczek, C., et al. (2014). An interdisciplinary methodological guide for quantifying associations between ecosystem services. Glob. Environ. Chang. 28, 298–308. doi: 10.1016/j.gloenvcha.2014.07.012
Müller, J. (2004). Cost-benefit ratio and empirical examination of the acceptance of heathland maintenance in the Lueneburg heath nature reserve. J. Environ. Plan. Manag. 47, 757–771. doi: 10.1080/0964056042000274461
Niemeyer, M., Niemeyer, T., Fottner, S., Härdtle, W., and Mohamed, A. (2007). Impact of sod-cutting and choppering on nutrient budgets of dry heathlands. Biol. Conserv. 134, 344–353. doi: 10.1016/j.biocon.2006.07.013
Niemeyer, T., Fottner, S., Mohamed, A., Sieber, M., and Härdtle, W. (2004). Auswirkungen kontrollierten brennens auf die nährstoffdynamik von sand und moorheiden. NNA Berichte 17, 65–79.
Niemeyer, T., Niemeyer, M., Mohamed, A., Fottner, S., and Hardtle, W. (2005). Impact of prescribed burning on the nutrient balance of heathlands with particular reference to nitrogen and phosphorus. Appl. Veg. Sci. 8, 183–192. doi: 10.1111/j.1654-109X.2005.tb00644.x
Obiang Ndong, G., Therond, O., and Cousin, I. (2020). Analysis of relationships between ecosystem services: a generic classification and review of the literature. Ecosyst. Serv. 43:101120. doi: 10.1016/j.ecoser.2020.101120
Olmeda, C., Šefferová, V., Underwood, E., Millan, L., Gil, T., and Naumann, S. (2020). EU Action Plan to Maintain and Restore to Favourable Conservation Status the Habitat Type 4030 European Dry Heaths. Available online at: https://ec.europa.eu/environment/nature/natura2000/management/pdf/EU%20HABITAT%20ACTION%20PLAN%204030.pdf (accessed December 7, 2021).
Phoenix, G. K., Emmett, B. A., Britton, A. J., Caporn, S. J. M., Dise, N. B., Helliwell, R., et al. (2012). Impacts of atmospheric nitrogen deposition: responses of multiple plant and soil parameters across contrasting ecosystems in long-term field experiments. Glob. Chang. Biol. 18, 1197–1215. doi: 10.1111/j.1365-2486.2011.02590.x
Power, S. A., Ashmore, M. R., and Cousins, D. A. (1998). Impacts and fate of experimentally enhanced nitrogen deposition on a British lowland heath. Environ. Pollut. 102, 27–34. doi: 10.1016/S0269-7491(98)80011-0
Power, S. A., Green, E. R., Barker, C. G., Bell, J. N. B., and Ashmore, M. R. (2006). Ecosystem recovery: heathland response to a reduction in nitrogen deposition. Glob. Chang. Biol. 12, 1241–1252. doi: 10.1111/j.1365-2486.2006.01161.x
Probo, M., Ascoli, D., Lonati, M., Marzano, R., and Lombardi, G. (2017). Restoration treatments to control Molinia arundinacea and woody and alien species encroachment in Calluna vulgaris heathlands at the southern edge of their distribution. Biol. Conserv. 211, 102–109. doi: 10.1016/j.biocon.2017.05.013
Quin, S. L. O., Artz, R. R. E., Coupar, A. M., and Woodin, S. J. (2015). Calluna vulgaris-dominated upland heathland sequesters more CO2 annually than grass-dominated upland heathland. Sci. Total Environ. 505, 740–747. doi: 10.1016/j.scitotenv.2014.10.037
Raudsepp-Hearne, C., Peterson, G. D., and Bennett, E. M. (2010). Ecosystem service bundles for analyzing tradeoffs in diverse landscapes. Proc. Natl. Acad. Sci. U.S.A. 107, 5242–5247. doi: 10.1073/pnas.0907284107
Rodriguez, J. P., Bennett, T. D. Jr., Bennett, E. M., Cumming, G. S., Cork, S. J., Agard, J., et al. (2006). Trade-offs across space, time, and ecosystem services. Ecol. Soc. 11:14. doi: 10.5751/ES-01667-110128
Roem, W. J., Klees, H., and Berendse, F. (2002). Effects of nutrient addition and acidification on plant species diversity and seed germination in heathland. J. Appl. Ecol. 39, 937–948. doi: 10.1046/j.1365-2664.2002.00768.x
Schellenberg, J., and Bergmeier, E. (2020). Heathland plant species composition and vegetation structures reflect soil-related paths of development and site history. Appl. Veg. Sci. 23, 386–405. doi: 10.1111/avsc.12489
Schellenberg, J., and Bergmeier, E. (2021). The calluna life cycle concept revisited: implications for heathland management. Biodivers. Conserv. doi: 10.1007/s10531-021-02325-1
Schirmel, J. (2010). Short-term effects of modern heathland management measures on carabid beetles (coleoptera: carabidae). Appl. Ecol. Environ. Res. 8, 165–175.
Sedláková, I., and Milan, C. (1999). Regeneration patterns in a central European dry heathland: effects of burning, sod-cutting and cutting. Plant Ecol. 143, 77–87. doi: 10.1023/A:1009807411654
Seifert, R., Lorenz, A., Osterloh, S., Henning, K., and Tischew, S. (2015). Free-range grazing by large herbivores in degraded large-scale dry sandy grassland-heathland ecosystems. Ecol. Quest. 21, 87–89. doi: 10.12775/EQ.2015.015
Siepel, H., Vogels, J., Bobbink, R., Bijlsma, R. J., Jongejans, E., de Waal, R., et al. (2018). Continuous and cumulative acidification and N deposition induce P limitation of the micro-arthropod soil fauna of mineral-poor dry heathlands. Soil Biol. Biochem. 119, 128–134. doi: 10.1016/j.soilbio.2018.01.025
Streitberger, M., Holtmann, L., Bültmann, H., Schmidt, C., and Fartmann, T. (2021). Effects of montane heathland rejuvenation on phytodiversity depend on management intensity and succession. Ecol. Eng. 168:106292. doi: 10.1016/j.ecoleng.2021.106292
Taboada, A., Marcos, E., and Calvo, L. (2016). Disruption of trophic interactions involving the heather beetle by atmospheric nitrogen deposition. Environ. Pollut. 218, 436–445. doi: 10.1016/j.envpol.2016.07.023
Terry, A. C., Ashmore, M. R., Power, S. A., Allchin, E. A., and Heil, G. W. (2004). Modelling the impacts of atmospheric nitrogen deposition on calluna-dominated ecosystems in the UK. J. Appl. Ecol. 41, 897–909. doi: 10.1111/j.0021-8901.2004.00955.x
Turkelboom, F., Thoonen, M., Jacobs, S., and Berry, P. (2015). Ecosystem service trade-offs and synergies. Ecol. Soc. 21:43. doi: 10.13140/RG.2.1.4882.9529
Usher, M. B., and Thompson, D. B. (1993). Variation in the upland heathlands of great Britain: conservation importance. Biol. Conserv. 66, 69–81. doi: 10.1016/0006-3207(93)90136-O
Valencia Torres, A., Tiwari, C., and Atkinson, S. F. (2021). Progress in ecosystem services research: a guide for scholars and practitioners. Ecosyst. Serv. 49:101267. doi: 10.1016/j.ecoser.2021.101267
Vallet, A., Locatelli, B., Levrel, H., Wunder, S., Seppelt, R., Scholes, R. J., et al. (2018). Relationships between ecosystem services: comparing methods for assessing tradeoffs and synergies. Ecol. Econ. 150, 96–106. doi: 10.1016/j.ecolecon.2018.04.002
van der Bij, A. U., Pawlett, M., Harris, J. A., Ritz, K., and van Diggelen, R. (2017). Soil microbial community assembly precedes vegetation development after drastic techniques to mitigate effects of nitrogen deposition. Biol. Conserv. 212, 476–483. doi: 10.1016/j.biocon.2016.09.008
van der Wal, R., Bonn, A., Monteith, D., Reed, M., Blackstock, K., Hanley, N., et al. (2011). “Mountains, moorlands and heaths [chapter 5],” in UK National Ecosystem Assessment. Understanding Nature’s Value to Society, 105–160. Available online at: http://uknea.unep-wcmc.org/Resources/tabid/82/Default.aspx (accessed December 12, 2021).
Vandvik, V., Heegaard, E., Måren, I. E., and Aarrestad, P. A. (2005). Managing heterogeneity: the importance of grazing and environmental variation on post-fire succession in heathlands. J. Appl. Ecol. 42, 139–149. doi: 10.1111/j.1365-2664.2005.00982.x
Vogels, J. J., Verberk, W. C. E. P., Kuper, J. T., Weijters, M. J., Bobbink, R., and Siepel, H. (2021). How to restore invertebrate diversity of degraded heathlands? A case study on the reproductive performance of the field cricket Gryllus campestris (L.). Front. Ecol. Evol. 9:300. doi: 10.3389/fevo.2021.659363
Vogels, J. J., Verberk, W. C. E. P., Lamers, L. P. M., and Siepel, H. (2017). Can changes in soil biochemistry and plant stoichiometry explain loss of animal diversity of heathlands? Biol. Conserv. 212, 432–447. doi: 10.1016/j.biocon.2016.08.039
Vogels, J. J., Weijters, M. J., Bobbink, R., Bijlsma, R.-J., Lamers, L. P. M., Verberk, W. C. E. P., et al. (2020). Barriers to restoration: soil acidity and phosphorus limitation constrain recovery of heathland plant communities after sod cutting. Appl. Veg. Sci. 23, 94–106. doi: 10.1111/avsc.12471
von Oheimb, G., Power, S., Falk, K., Friedrich, U., Mohamed, A., Krug, A., et al. (2010). N:p ratio and the nature of nutrient limitation in calluna-dominated heathlands. Ecosystems 13, 317–327. doi: 10.1007/s10021-010-9320-y
Wagner, S., Berg, P., Schädler, G., and Kunstmann, H. (2013). High resolution regional climate model simulations for Germany: part II—projected climate changes. Clim. Dyn. 40, 415–427. doi: 10.1007/s00382-012-1510-1
Walmsley, D., and Härdtle, W. (2021). “Erfolgskontrolle beim management von heideökosystemen in zeiten globalen wandels - neue forschungsergebnisse für eine alte kulturlandschaft,” in Erfolgskontrollen im Naturschutz, Naturschutz und Biologische Vielfalt, ed. V. Scherfose (Bonn - Bad Godesberg: Bundesamt für Naturschutz), 257–272. doi: 10.19213/973171
Webb, J. R., Drewitt, A. L., and Measures, G. H. (2010). “Managing for species: integrating the needs of England’s priority species into habitat management. part 1 report,” Natural England Research Report No. 024. Natural England (Sheffield).
Webb, N. R. (1998). The traditional management of European heathlands. J. Appl. Ecol. 35, 987–990. doi: 10.1111/j.1365-2664.1998.tb00020.x
Wessel, W. W., Tietema, A., Beier, C., Emmett, B. A., Peñuelas, J., and Riis-Nielsen, T. (2004). A qualitative ecosystem assessment for different shrublands in western Europe under impact of climate change. Ecosystems 7, 662–671.
West, S., Haider, L. J., Stålhammar, S., and Woroniecki, S. (2020). A relational turn for sustainability science? Relational thinking, leverage points and transformations. Ecosyst. People 16, 304–325. doi: 10.1080/26395916.2020.1814417
Keywords: heaths, heathland, trade-offs, ecosystem functioning, ecosystem services, nutrient balances, multifunctionality, conservation management
Citation: Walmsley DC, Delory BM, Alonso I, Temperton VM and Härdtle W (2021) Ensuring the Long-Term Provision of Heathland Ecosystem Services—The Importance of a Functional Perspective in Management Decision Frameworks. Front. Ecol. Evol. 9:791364. doi: 10.3389/fevo.2021.791364
Received: 08 October 2021; Accepted: 02 December 2021;
Published: 20 December 2021.
Edited by:
Anita Diaz, Bournemouth University, United KingdomReviewed by:
Csaba Tölgyesi, University of Szeged, HungaryCopyright © 2021 Walmsley, Delory, Alonso, Temperton and Härdtle. This is an open-access article distributed under the terms of the Creative Commons Attribution License (CC BY). The use, distribution or reproduction in other forums is permitted, provided the original author(s) and the copyright owner(s) are credited and that the original publication in this journal is cited, in accordance with accepted academic practice. No use, distribution or reproduction is permitted which does not comply with these terms.
*Correspondence: David C. Walmsley, d2FsbXNsZXlAbGV1cGhhbmEuZGU=
Disclaimer: All claims expressed in this article are solely those of the authors and do not necessarily represent those of their affiliated organizations, or those of the publisher, the editors and the reviewers. Any product that may be evaluated in this article or claim that may be made by its manufacturer is not guaranteed or endorsed by the publisher.
Research integrity at Frontiers
Learn more about the work of our research integrity team to safeguard the quality of each article we publish.