- 1Museum für Naturkunde Berlin, Leibnitz Institute for Research on Evolution and Biodiversity, Berlin, Germany
- 2Department of Biological Sciences, Louisiana State University, Baton Rouge, LA, United States
- 3Department of Biology, Humboldt University, Berlin, Germany
Regeneration, the replacement of body parts in a living animal, has excited scientists for centuries and our knowledge of vertebrate appendage regeneration has increased significantly over the past decades. While the ability of amniotes to regenerate body parts is very limited, members of other vertebrate clades have been shown to have rather high regenerative capacities. Among tetrapods (four-limbed vertebrates), only salamanders show unparalleled capacities of epimorphic tissue regeneration including replacement of organ and body parts in an apparently perfect fashion. The closest living relatives of Tetrapoda, the lungfish, show regenerative abilities that are comparable to those of salamanders and recent studies suggest that these high regenerative capacities may indeed be ancestral for bony fish (osteichthyans) including tetrapods. While great progress has been made in recent years in understanding the cellular and molecular mechanisms deployed during appendage regeneration, comparatively few studies have investigated gross morphological and histological features of regenerated fins and limbs. Likewise, rather little is known about how fin regeneration compares morphologically to salamander limb regeneration. In this study, we investigated the morphology and histology of regenerated fins in all three modern lungfish families. Data from histological serial sections, 3D reconstructions, and x-ray microtomography scans were analyzed to assess morphological features, quality and pathologies in lungfish fin regenerates. We found several anomalies resulting from imperfect regeneration in regenerated fins in all investigated lungfish species, including fusion of skeletal elements, additional or fewer elements, and distal branching. The similarity of patterns in regeneration abnormalities compared to salamander limb regeneration lends further support to the hypothesis that high regenerative capacities are plesiomorphic for sarcopterygians.
Introduction
The capability to replace lost organ and body parts, better known as regeneration, has fascinated scientists for several centuries (Reaumur, 1712). While this ability varies widely among Metazoa (e.g., Morgan, 1898; Lenhoff and Lenhoff, 1991; Bely and Nyberg, 2010), epimorphic regeneration was considered exceptional among extant vertebrates (e.g., Alvarado, 2000; Tsonis, 2000). This form of regeneration, considered to be true regeneration, is characterized by the formation of two crucial structures, the blastema and the apical epithelial cap (or AEC) (Londono et al., 2018). Full regeneration of limbs after loss by injury has been reported only in salamanders and frogs, albeit appendage regeneration in frogs is limited to tadpole stages before metamorphic climax and is lost in adults (Dent, 1962; Girvan et al., 2002). Hence, urodeles are the only living tetrapods capable of fully regenerating limbs throughout their whole lifespan, even though larval salamanders show regenerates with less abnormalities than adults (Bothe et al., 2021). Through intensive research, especially on the model organism axolotl (Ambystoma mexicanum) the underlying processes of epimorphic regeneration in salamanders are quite well understood (amongst others: Kragl et al., 2009; McCusker et al., 2015; Bryant et al., 2017a). More recently, salamander lineage-specific genes (LSGs) were identified and shown to play a role in limb development as well as regeneration in salamanders, which led to the proposal that certain features in salamander limb development and their capacities to fully regenerate limbs may indeed be lineage specific for urodeles (Garza-Garcia et al., 2010; Brockes and Gates, 2014). However, data from fossil shows that limb regeneration already occurred in ancient amphibians long before the emergence of salamanders (Fröbisch et al., 2014, 2015), suggesting that the high regenerative capacities of body appendages are an ancient feature of tetrapods that was lost in the amniote lineage. This was later supported by molecular data that demonstrated that the genetic toolkit playing a role in lungfish tail regeneration is very similar to that seen in axolotl (Verissimo et al., 2020). Moreover, high regenerative capacities of the endochondral appendage skeleton were also demonstrated for several clades of osteichthyians, including paddle fish, gar, Polypterus, and several members of teleost fish (Cuervo et al., 2012; Amaral and Schneider, 2018; Darnet et al., 2019). Darnet et al. (2019) therein also showed that osteichthyians deploy a similar genetic toolkit for appendage regeneration, lending support to an ancient origin of epimorphic regeneration in vertebrates. However, many aspects of the evolution of vertebrate regeneration remain poorly understood and will require more detailed molecular, morphological, evolutionary, and ecological investigations of non-model as well as model organisms to gain a better understanding of the drivers of epimorphic regeneration in vertebrates.
Lungfish (Dipnoi) play a central role in this context, as they display a high degree of tissue regeneration in body appendages comparable to modern salamanders. Dipnoi are an ancient lineage of osteichthyan fish (bony fish) and, next to coelacanths, the only extant sarcopterygian (lobe-finned) fish. They first appeared in the Early Devonian period, about 419.2–393.3 million years ago (Chang and Yu, 1984) and were widespread and common in both marine and freshwater habitats. Several phylogenomic analyses and genome sequencing over the past few years have revealed that lungfish, rather than coelacanths, are the closest extant relatives of tetrapods (Amemiya et al., 2013; Biscotti et al., 2016; Irisarri and Meyer, 2016).
Although Dipnoi were notably abundant during the Devonian, most lungfish went extinct after the end Permian mass extinction (Nelson et al., 2016). Only three freshwater genera survived until now, represented by six species: South American lungfish (Lepidosiren, one species), African lungfish (Protopterus, four species), and the Australian lungfish (Neoceratodus, one species).
Lepidosiren was the first lungfish to be discovered in the 1830s (Bischoff, 1840) and have been the focus of many studies on sarcopterygian and vertebrate evolution, the transition from fishes to land vertebrates, genome size studies and the evolution of tetrapod feeding systems (Reilly and Lauder, 1990; Ericsson et al., 2010; Boisvert et al., 2013; Ziermann et al., 2018). Moreover, their high regenerative abilities, which rival those of salamanders, make them a highly relevant subject for regeneration research (Conant, 1970; Darnet et al., 2019; Verissimo et al., 2020).
Anatomically, paired lungfish fins rest on a single cartilaginous girdle element and are constructed according to the archipterygial fin type, in which the metapterygial stem consists of a projecting series of endoskeletal basal fin elements running along the middle of the fin. From this central axis, preaxial (anterior) and postaxial (posterior) radials proceed outward to sides of the fin for further support (Kardong, 1997). Among the modern taxa, the Australian lungfish resembles most closely the ancestral fin anatomy of ancient lobe-finned fishes (Kardong, 1997). Viewed from the outside, the fin appears leaf-shaped and narrow at its base. Cartilaginous, serially arranged elements comprising the fin main axis, called mesomeres, follow the pectoral or pelvic girdle, respectively, and become increasingly smaller toward the fin tip. The first mesomere is considered homologous to the femur/humerus of tetrapods, the second to radius and ulna or tibia and fibula, respectively (Romer et al., 1959; Kardong, 1997). Numerous fin radials are articulated dorsally (preaxially) and ventrally (postaxially) from the second mesomere onward, whereas the amount and arrangement of the fin radials is variable (Braus, 1900). The second mesomere usually has one pre-axial side radial on the pectoral and two or more on the pelvic fin, as well as about 4–5 postaxial lateral radials on the pectoral and about three on the pelvic fin. Subsequent mesomeres each carry one or two side radials postaxially and preaxially.
By contrast, the fins of South American and African lungfish species are significantly reduced. Preaxial and postaxial radials are missing altogether in Lepidosiren, whereas vestigial post-axial radials are present in Protopterus, which is why the fins look thin and thread-like, a conformation considered to be derived (Johanson et al., 2007). A striking difference between the two genera is that the pelvic fins of the South American male lungfish are covered with a unique array of filaments. These structures are not found in fins of other lungfish species. The function of these fin attachments has not yet been fully clarified. Suggestions that they serve as “limb gills” for the release or uptake of oxygen could not be directly confirmed (Lima et al., 2017).
Despite its great potential as a model organism for regenerative research very few studies of tail or fin regeneration in lungfish were published in the following decades after initial observations by Traquair (1871) and Conant (1970).
One cause for this lies in the difficulties of lungfish housing and breeding for research and the unavailability of embryos. Despite this, lungfish have attracted great scientific interest in the field of regeneration in only recently, yielding important insights into the regeneration process and its evolution, via comparisons of lungfish and salamanders regeneration. While it has been known that lungfish develop a blastema at the wound site during the regeneration process that is comparable to those in salamander appendage regeneration (Conant, 1970), recent studies detected further similarities in the formation of a proliferative blastema, development of an Apical Epithelial Cap (AEC), and self-replacement of original structures including muscles, skeleton, and spinal cord (Verissimo et al., 2020). Transcriptome and differential gene expression analyses identified strong parallels in gene regulation and transcriptional profiles applied in lungfish and salamander appendage blastema formation (Nogueira et al., 2016). These commonalities highlight the importance of lungfish as model for regenerative research of body appendages and lend further support for the hypothesis of a deep evolutionary origin of regenerative capacities.
Apart from studies on regeneration in controlled laboratory experiments, investigations of naturally occurring regeneration provide crucial insights into the ecological and evolutionary parameters that may have influenced and directed regeneration in sarcopterygians. Since lungfish are very territorial animals (Curry-Lindahl, 1956), conspecific biting of fins and tails occur frequently both in the wild but also especially in captivity. In the Australian lungfish, primarily the juvenile individuals behave aggressive toward conspecifics, especially in view of food consumption and shelter areas. However, dominance hierarchies are also know among adults (Kind, 2002; Department of the Environment, Water, Heritage and the Arts, 2009; Jorgensen and Joss, 2016). In these settings, whole appendages or only parts may be bitten off and repeated biting may occur. As a result, fin pathologies and anomalies can often be observed in natural regenerates.
The main aim of our study was to compare the natural regenerative abilities of pectoral and pelvic fins of the three modern lungfish genera, the South American lungfish Lepidosiren paradoxa, the African lungfish Protopterus spp., as well as the Australian lungfish, Neoceratodus forsteri.
We present morphological and histological data of natural fin regenerates in all three modern taxa based to understand overall regeneration abilities as well as occurring anomalies and pathologies after failed regeneration. For this purpose, we have analyzed data from gross morphology, via histological serial sections, cleared, and double stained specimens, as well as x-ray computed tomography (μCT-scanning) and 3D reconstructions. The results are discussed in comparison to natural limb regenerates in the salamander model Ambystoma mexicanum, the Mexican axolotl.
Materials and Methods
Material
Two fins of Lepidosiren paradoxa (ZMB_Pisces_37121, ZMB_Pisces_37122) were provided by Igor Schneider. These specimens were adults collected as wild caught in the city of Breves, state of Para, Brazil. The six specimens of Protopterus were obtained from the Royal Belgian Institute of Natural Science (RBINS 148, RBINS 8112) and the Royal Museum for Central Africa (RMCA_Vert_1973.015.P.0001, RMCA_Vert_1991.024.P.0001-0002, RMCA_Vert_P.124855-124859, RMCA_Vert_P.165214-165235). Three living specimens of Neoceratodus forsteri used for external gross observation were imported by Jindalee International Pty Ltd from an Australian lungfish farm, where they were reared in groups and obtained bite wounds on their appendages by conspecifics. In the animal husbandry of the Museum für Naturkunde Berlin individuals were kept separate in order to investigate the regeneration process of the fins without continued biting. Neoceratodus Specimen (ZMB_Pisces_33693) used for x-ray microtomography and histology is housed at the Museum für Naturkunde Berlin.
Gross morphology of specimen was examined with a Leica (MZ12) binocular microscope using ordinary transmitted light in magnifications ranging from 8x to 50x. Images were taken with a Leica DFC 495 Digital Color Microscope Camera (Leica Application Suite V4.2. software) and a Nikon D3100 digital camera.
Methods
Before using material for any analytical method, samples were fixed in fresh 4% paraformaldehyde in PBS (Phosphate Buffered Saline) for about 48 h. No information on the original fixation method of the specimens was available, but all collection material has been stored in 70% ethanol for long periods of time (several years to decades).
Because availability of material for destructive investigation was limited, we applied as many methods as possible to any given sample to maximize informative outcome. As μCT (X-ray microtomography) scanning without prior tissue staining produced low inherent contrast of non-mineralized soft tissues, a new suitable staining protocol using PTA (phosphotungstic acid) and/or iodine was developed to produce images with better tissue-specific gray contrasts. Unfortunately, these staining agents exert negative effects on subsequently performed histological serial sections and cleared and double staining methods.
Contrast-Enhanced Micro-CT Imaging and Analysis
Due to restrictions for the use of invasive methods on some of the collection material, different staining protocols were applied to the individual specimens. All specimens were stained at room temperature on a plate stirrer. Therein, the two most important staining parameters are the concentration of the staining solution and the duration of time that the specimen remains in solution. The concentration of the staining solutions was gradually increased within the first days to protect the tissue while achieving the best possible staining results. To check the progress of the staining and to avoid overstaining, test scans were carried out in the concomitantly.
Both fins of Lepidosiren paradoxa (ZMB_Pisces_37121, ZMB_Pisces_37122) were stained in a 10% solution of Lugol’s iodine (I2KI) in distilled water for 7 days. Fins of the genus Protopterus (RMCA_Vert_P.165214-165235, RMCA_Vert_1991.024.P.0001-0002, RMCA_Vert_1973.015.P. 0001, RMCA_Vert_P.124855-124859) were stained with 1.25% phosphotungstic acid (PTA) in distilled water for 3 weeks with the PTA solution changed twice during this period. A double staining protocol with iodine and PTA was tested on the fins of Neoceratodus forsteri (ZMB_Pisces_33693) with the first 7 days of staining with a 10% solution of Lugol’s iodine (I2KI) in distilled water followed by 2 weeks with 1.5% PTA in distilled water. The fins were examined through micro-tomographic analysis by using a YXLON FF35 CT. Scan settings varied depending on the object and among other things, were dependent on the size of the objects. Lepidosiren paradoxa (ZMB_Pisces_37121, ZMB_Pisces_37122) were scanned at 100 kV and 120 μA, generating 1,440 projections with 1,250 ms per picture. Effective voxel size was 6 μm. Protopterus specimen (RBINS 8112, RMCA_Vert_P.165214-165235, RMCA_Vert_1991.024.P.0001-0002, RMCA_Vert_1973.015.P.0001, RMCA_Vert_P.124855-124859) were scanned at 90 kV and 150 μA, generating 1,440 projections with 750 ms per picture. Effective voxel size ranged between 14 and 15 μm. Fins of Neoceratodus forsteri (ZMB_Pisces_33693) were scanned at 90/120 kV and 100/150 μA, generating 1,440 projections with 750 ms per picture. Effective voxel size ranged between 16 and 20μm. The cone beam reconstruction was performed using the datos| x-reconstruction software (GE Sensing and Inspection Technologies GMBH phoenix| x-ray datos| x 2) and the three-dimensional reconstructions were visualized in VGStudio Max 3.1. (Volume Graphics Inc., Germany). Fin skeletons were segmented manually from the stained scans.
Clearing and Double Staining
After micro CT scanning the left pelvic fin of Neoceratodus forsteri (ZMB_Pisces_33693) was skinned and cleared and double stained (Alcian blue and Alizarin red) for visualizing cartilage and bone. The protocol was modified according to Ovchinnikov (2009). The cartilaginous skeletal elements of the fins were stained in a 0.015%-Alcian-blue-solution for approximately 12 h and washed afterward in an ethanol series. Maceration was performed in trypsin (0.1%, Sigma) for 2 weeks at 37°C. Bony skeletal elements were stained in 0.01%-Alizarin-red-solution for approximately 6 h and washed afterward in a 30%-glycerin solution. For final storage, the sample was transferred to a 100% solution of glycerin.
Histological Staining
Lungfish fins were decalcified in 20% EDTA solution for about 2 weeks. Afterward, tissue was dehydrated by means of an ascending alcohol series (80–96–100%) and cleared with Xylene. Finally, the preparations were soaked and embedded in paraffin. The samples were serial sectioned at a thickness of 7–10 μm with a microtome. First, slides were deparaffinized with xylenes and thereafter rehydrated in a graded series of decreasing ethanol concentrations and distilled water. The serial sections were each stained alternately with Alcian Blue/Nuclear Fast Red or Heidenhain’s Azan. Subsequently, the sections were dehydrated through an ascending series of ethanol and xylene and covered with Entellan (Merck KGaA) and a cover glass (detailed protocols for staining are attached in Supplementary Materials). Sections were viewed with a transmitted light microscope and photographed by using the Leica DFC495 Digital Color Microscope Camera mounted on the Axioskop and the Leica Application Suite V 4.2. Software.
Results
Notably, in the majority of cases it is difficult to identify a regenerated fin from the outer morphology once the regeneration process is finished. The most evident indication for regeneration are fin abnormalities such as stark morphological deformations, bifurcations, but also constrictions, foreshortened fins and paired fins of uneven lengths.
Lepidosiren paradoxa (South American Lungfish)
In their original state, South American lungfish fins consist of only one fin radial composed of numerous serially repeated cartilaginous fin radial elements. For this study we investigated two regenerated pectoral fins of this taxon in detail (ZMB_Pisces_37121, ZMB_Pisces_37122).
The pectoral fin of specimen number ZMB_Pisces_37121 does not show any obvious signs of regeneration in its outer morphology (Figure 1A), except for a noticeable narrowing in the very proximal region of the fin (Figure 1A, dashed box). CT scanning and the 3D reconstruction revealed constrictions of two proximal radial elements (Figures 1B–D, arrow) and an amalgamation with the adjacent distal elements (Figures 1B–D, arrow). Additionally, several fused elements were identified in the more distal region of the fin (Figures 1B, dashed box; 1E, arrow). Histological investigation revealed detailed information on the cellular level and showed that the constrictions of the skeletal fin radial elements affect all types of tissue at this position of the fin, including the perichondrium, the cartilaginous matrix, and musculature (Figures 1F,G). In addition, the epidermis is not fully regenerated (Figures 1D,F, asterisks). Contrary to the assumption that the epidermis regenerates pretty fast in order to protect the wound healing area from infection while internal parts regenerate slower, in this specimen it is unusually thin in some places. At the narrowest point of the constriction, it seems to be completely absent. This type of pathology is not known from salamander limb regeneration, but may be a sign of repeated biting. However, causes other than regeneration for this anomaly are also possible, as for example and infection or skin disease. Nevertheless, it appears likely that the restriction coincides with the location of the bite sites and sections also show jointed individual fin radial elements in several regions of the fin (Figure 1G). Despite the greater resolution on the cellular level, it remains unclear whether these are fused elements, for which complete separation failed during initial fin development, or whether they were also caused by constrictions of the periosteum and the cartilaginous matrix of a single fin radial element during the regeneration process.
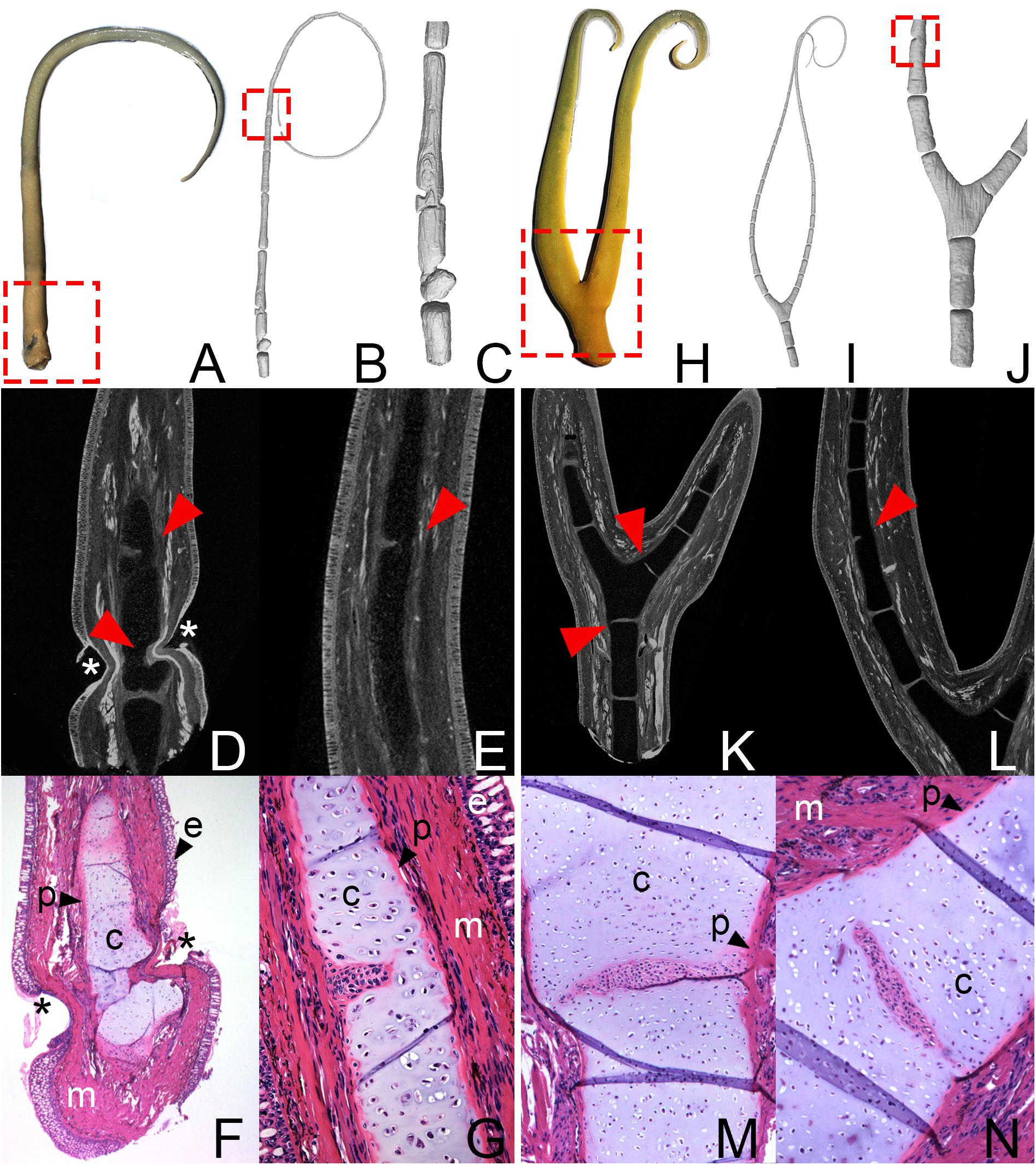
Figure 1. (A–G) Pectoral fin of Lepidosiren paradoxa (ZMB_Pisces_37121). 3D reconstruction: (A) Exterior view with the suspected bite site (dashed box). 3D reconstruction: (B) of the entire fin, dashed box shows area of fused elements, and (C) detailed view of the proximal fin area. μCT scans: (D) of the constricted fin area and (E) fusion of the skeletal elements. Arrows indicate fused and constricted skeletal elements. Asterisks indicate area with missing epidermis. Histological serial section with Azan staining (F) of the constricted fin area and (G) fusion of the skeletal elements. (H–N) Bifurcated pectoral fin of Lepidosiren paradoxa (ZMB_Pisces_37121). (H) Exterior view with the suspected bite site (dashed box). 3D reconstruction: (B) of the entire fin and (C) detailed view of the proximal fin area, dashed box shows area of fused elements. μCT scans: (D) of the bifurcated element and (E) fusion of the skeletal elements. Arrows indicate fused skeletal elements. Histological serial section with Azan staining: (F) of the most proximal part of the bifurcated element and (G) fusion of the bifurcated element with the adjacent skeletal element. c, cartilage; e, epidermis; m, muscles; p, perichondrium.
The second fin of Lepidosiren (ZMB_Pisces_37121) shows an obvious anomaly that is already visible in gross observation. The fin divides at the proximal end and branches into two fin axes (Figure 1H, dashed box). Histology and μCT-scanning reveal that this bifurcation is caused by branching of a single proximal skeletal element (Figures 1I–N). Additionally, the bifurcated element displays partial amalgamation with the adjacent elements (Figure 1K, arrows; Figures 1M,N). Partial fusion is also visible between more distal elements (Figure 1J, dashed box; Figure 1L, arrow).
Protopterus (African Lungfish)
Protopterus fins have an overall similar structure to those of Lepidosiren, except for the filamentous structures on the pelvic fins of males, which are completely absent in Protopterus.
Six lungfish individuals of three Protopterus species with regeneration malformations were identified in the collections of the Royal Belgian Institute of Natural Science and the Royal Museum for Central Africa (RBINS 148, RBINS 8112, RMCA_Vert_1973.015.P.0001, RMCA_Vert_1991.024.P.0001-0002, RMCA_Vert_P.124855-124859, RMCA_Vert_P.165214-165235). Anomalies were identified in both pectoral and pelvic fins. Evident characteristics are bifurcations, as well as constrictions and bilaterally asymmetrical, foreshortened fins (Figures 2, 3).
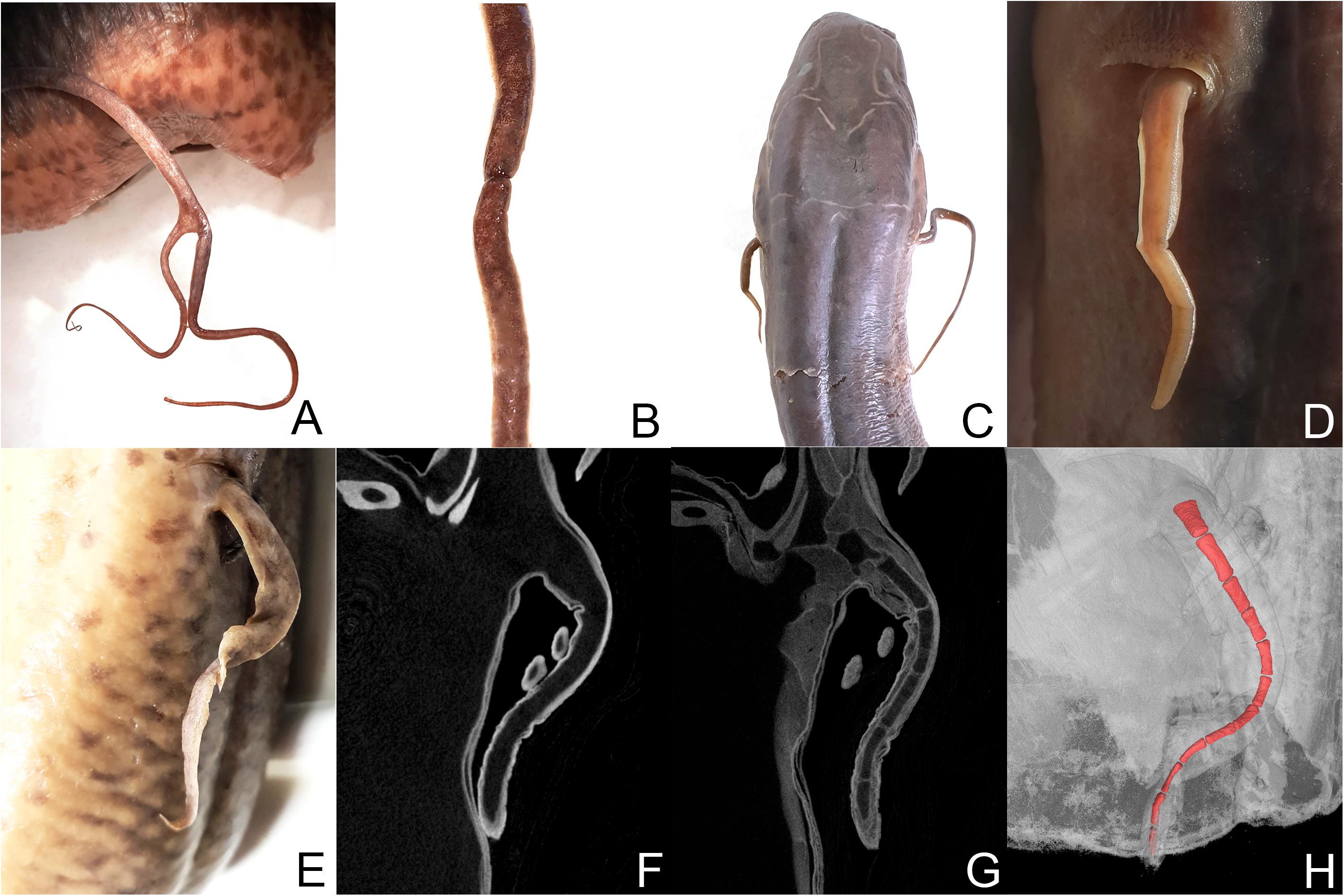
Figure 2. Protopterus aethiopicus (RBINS 148): (A) bifurcation of the left pectoral fin and (B) constriction of the right pectoral fin. Protopterus dolloi (RBINS 8112): (C) paired pectoral fins. (D) Close-up of the shorten fin. Protopterus annectens brieni (RMCA_Vert_P.165214-165235): (E) left pectoral fin. (F) μCT scan without tissue staining. (G) μCT scan after staining with iodine. (H) 3D modeling of the fin.
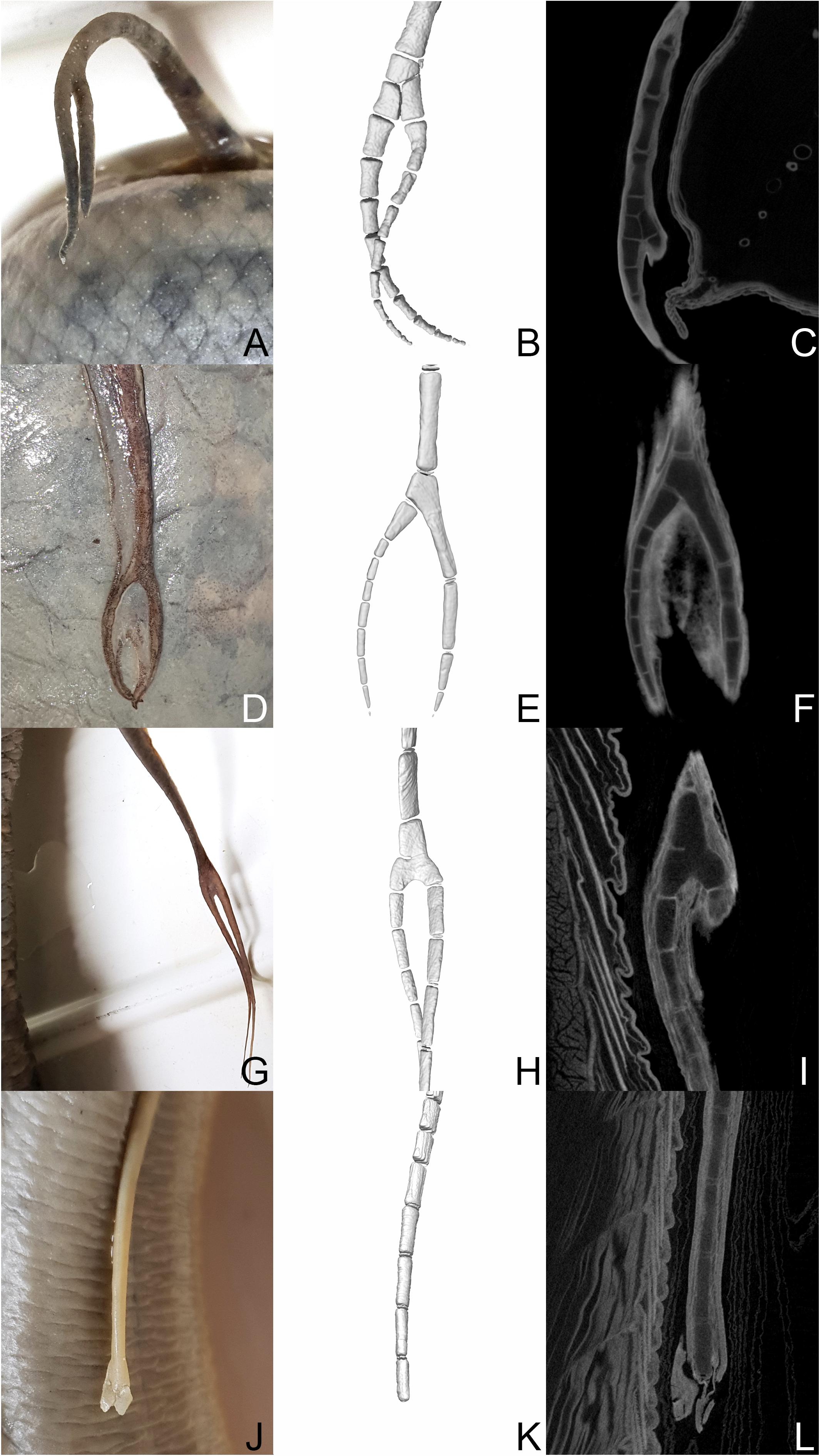
Figure 3. Protopterus annectens spec. (RMCA_Vert_1991.024.P.0001-0002): (A–C) left pelvic fin. Protopterus annectens annectens (RMCA_Vert_1973.015.P.0001): (D–F) left pelvic fin, (G–I) right pectoral fin. Protopterus annectens annectens (RMCA_Vert_P.124855-124859): (J–L) Right pectoral fin. (B,E,H,K) 3D reconstructions of the areas of bifurcation. (C,F,I,L) μCT scannings of the areas of bifurcation.
One specimen of the species Protopterus aethiopicus (RBINS 148) exhibits bifurcation of the left pectoral fin and a constriction of the right pectoral fin, but was only available for external observation (Figures 2A,B). One individual of the species Protopterus dolloi (RBINS 8112) with paired fins of unequal length was investigated by micro CT (Figures 2C,D). However, permission for tissue staining was not granted for this specimen and hence the resolution was insufficient to reconstruct the fin skeleton in 3D. The strong differences in the visibility of structures in unstained and stained material are shown in the comparison of the CT scans of pectoral fins of Protopterus annectens brieni (RMCA_Vert_P.165214-165235) before and after staining with I2KI and PTA (Figures 2E–G). The latter specimen shows a relatively short, strangely shaped fin morphology as well as incisions in the skin of the fin. The strange shape may be a preservational relic from being squeezed into a jar for an extended period. However, despite the unusual outer morphology, no skeletal abnormalities were identified in the 3D reconstruction (Figure 2H). Therefore, the regeneration process may have proceeded normally in this fin, but was not yet fully completed at the time the animal was collected.
Four fins of Protopterus annectens that showed bifurcations in external morphology, were investigated by μCT-scanning. The scans revealed that bifurcation do not all follow the same anatomical pattern but instead can be produced by different branching patterns involving the structures of the fin skeleton (Figure 3). In the left pelvic fin of RMCA_Vert_1991.024.P.0001-0002, one proximal element forms the base point for two more distal elements, which continue distally in building two separate fin radials (Figures 3A–C). In the left pelvic fin of Protopterus annectens annectens RMCA_Vert_1973.015.P.0001, the bifurcation is built by two elements, whereas an additional short fin element attaches laterally at the lower end of a significantly elongated element of the metapterygial axis and thus forms an additional lateral fin radial (Figures 3D–F). In this specimen the fins remain relatively short distal to the bifurcation. Whether this condition was caused by failed regeneration or rather in completed regeneration, cannot be resolved by the CT data.
A third branching pattern can be observed in the in the right pectoral fin of Protopterus annectens annectens RMCA_Vert_1973.015.P.0001. In this fin, a single fin element bifurcates distally and forms the attachment point for two further fin radial elements. The 3D reconstruction of the branching point suggests that this type of bifurcation was caused by incomplete segmentation of several elements (Figure 3G). Yet another fin regenerate morphology is visible in the right pectoral fin of Protopterus annectens annectens (RMCA_Vert_P.124855-124859) (Figures 3J–L). It shows a short fin, which externally shows signs of bifurcation in the soft tissue, but the internal anatomy of the cartilaginous fin skeleton still shows a single row of fin elements. The distal end of the fin, which is most likely the site of a bite injury, contains no skeletal elements. This fin morphology can most likely be explained with incomplete regeneration at the time of death of the animal or the regeneration process has failed for some reason. Fusions of several adjacent axis elements distal to the presumed amputation plane, as observed in Lepidosiren fins (Figure 1), were not observed in the investigated regenerates of Protopterus.
Neoceratodus forsteri (Australian Lungfish)
The third genus of extant lungfish is Neoceratodus with only a single species, the Australian lungfish Neoceratodus fosteri. In contrast to the other extant lungfish genera, the fin morphology of Neoceratodus resembles most closely that of its fossil relatives in having strong, fleshy fins, with a much more complex skeletal anatomy (Figures 4A,B). The fin consists of a large element, mesomere 1, which articulates with the shoulder girdle and does not carry any radials. A series of further mesomeres articulate distally to mesomere 1 and form the medial axis of the fin with preaxial and postaxial radials articulating to the mesomeres. Although regenerative capacities have been assumed for Neoceratodus, to our knowledge there has thus far not been a published report as to whether and how well Neoceratodus is able to regenerate their fins. However, the following results of detailed studies of the fin in μCT scans and histology indicate that regeneration processes are taking place.
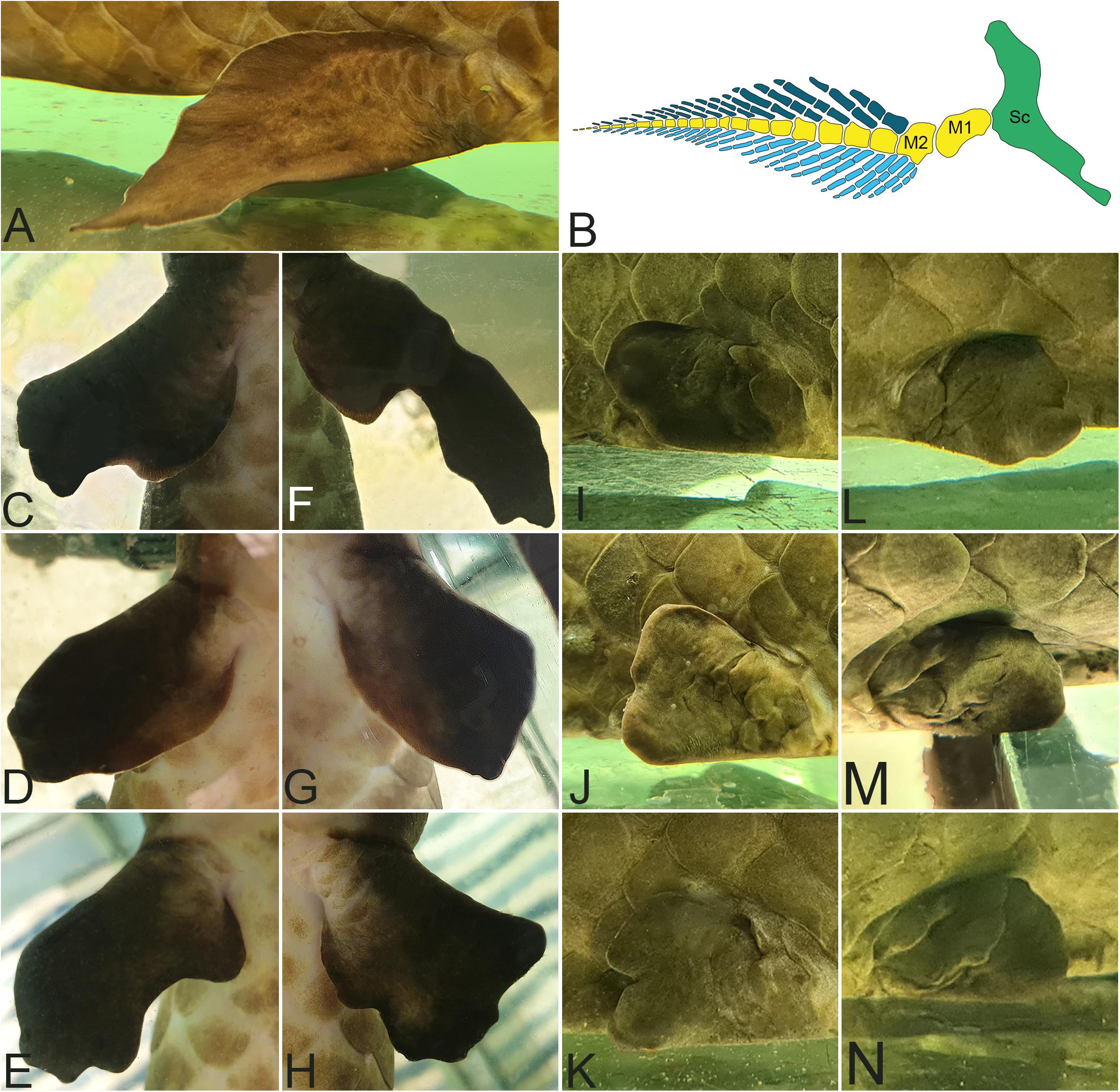
Figure 4. Neoceratodus fosteri: (A) leaf-like shape of a not regenerated pelvic fin (B) Model of a Skeleton anatomy of a not regenerated pectoral lungfish fin. Sc, scapula in green; M, Mesomere in yellow. Preaxial radials in dark blue. Postaxial radial in light blue. (C–H) Left and right pectoral fins with deformations in ventral view. (I–N) Left and right pelvic fins with deformations in lateral view.
Figures 4C–N show some examples of pectoral and pelvic fins of living individuals of Neoceratodus forsteri with obvious fin abnormalities after regeneration following repeated biting. Shortened, misshapen and stunted fins can clearly be recognized. Some of these anomalies look very severe. The reason for the severity of the abnormalities lies most likely in repeated and multiple biting of the fins. We cannot state with certainty whether the regeneration of these fins has been completed, or whether the fins are still in the process of regeneration. Notably, observation and documentation of these Neoceratodus individuals and their fins over a period of 2 years (provided in Supplementary Data) did not show any noticeable changes in the shape of these fins.
In addition to investigations of the living animals, Neoceratodus specimen ZMB_Pisces_33693 from the collection of the Museum für Naturkunde Berlin showing obvious fin anomalies was provided for destructive sampling (Figure 5). In particular, both pectoral fins displayed strongly deformed morphology upon external observation (Figures 5A,E). Both fins are shortened, malformed and do not show the original leaf-like fin shape. The pelvic fins did not display clear external signs for regeneration, yet the right fin is slightly shorter than the left, indicating a possible earlier regeneration event (Figures 5I,M).
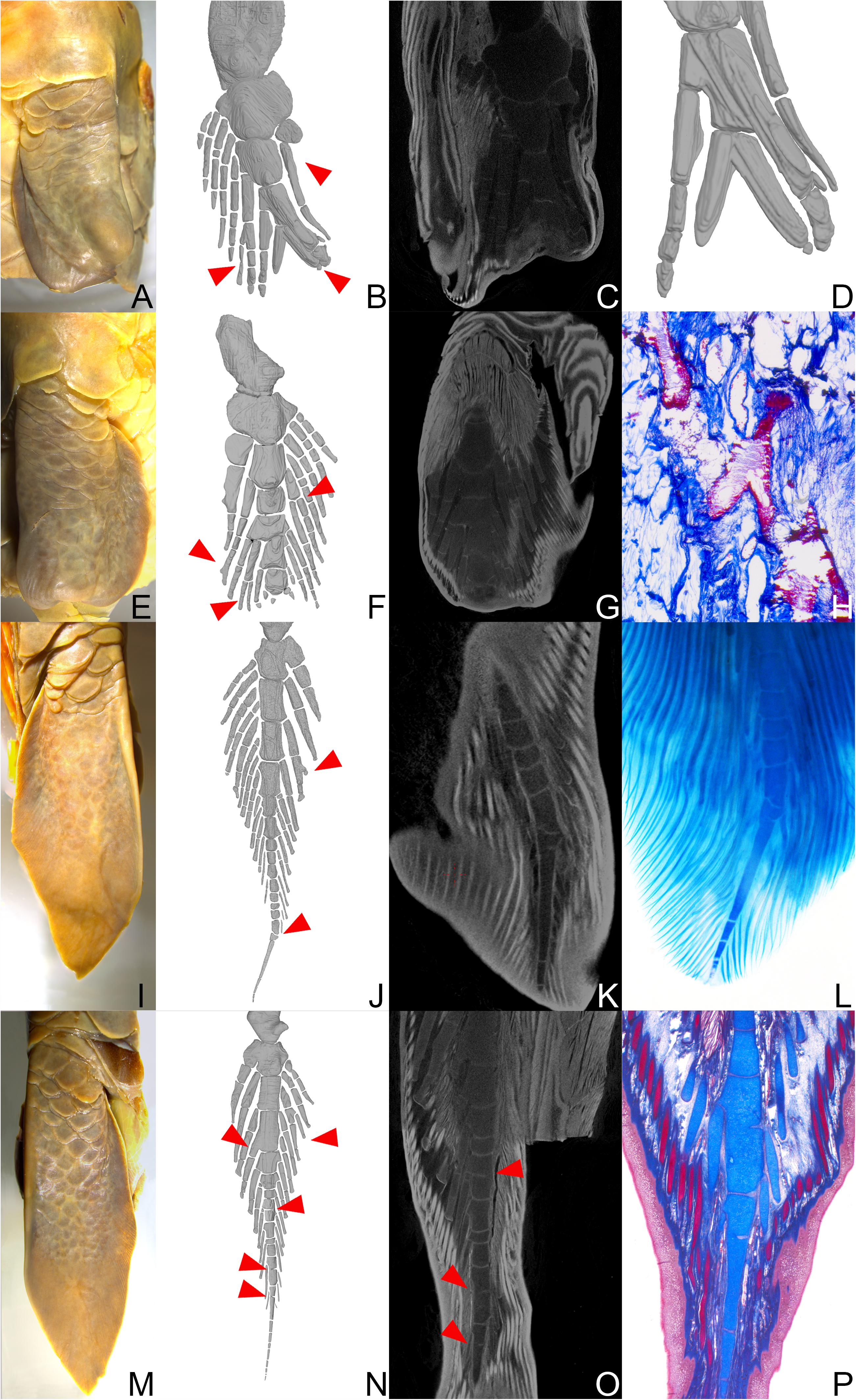
Figure 5. Neoceratodus forsteri (ZMB_Pisces_33693): (A) Left pectoral fin. (E) Right pectoral fin. (I) Left pelvic fin. (M) Right pelvic fin. All fins in ventral view. (B,F,J,N) 3D reconstructions of the entire fins. (C,G,K,O) μCT scannings. (D) Detailed 3D reconstruction of the distal fin tip. (H,P) Histological serial sections, stained with Alcian blue. (L) Clearing and double staining of the distal fin tip. Red arrows indicate striking malformations of the fin skeleton.
The results of the CT scans and 3D modeling clearly showed that in both the left and right pectoral fin the central axis is strongly foreshortened as compared to the normal fin anatomy (Figures 5B,C,F,G). Moreover, preaxial and postaxial fin radials are asymmetric, some radials show distal branching while other radials are missing altogether. In the left pectoral fin, the skeletal abnormalities begin at the level of the second mesomere (Figures 5B–D). The preaxial lateral radials attached to mesomere 2 is strongly shortened and consists of only one skeletal element instead of at least three as in the normal fin anatomy. Further distally, the skeletal anatomy of the fin is severely altered. The fourth and fifth mesomeres are completely deformed. The skeletal elements in this area are partially shifted in the transverse plane so that they overlap (Figure 5D). Furthermore, a postaxial radial shows clear branching (Figure 5B). Finally, the most distal end of the fin, which normally tapers peripherally to a thin thread, is missing entirely.
In the right pectoral fin, the pathologies start more distally than in the left fin at the height of the fifth mesomere, indicating a more distal bite wound. The anomaly in skeletal anatomy is overall less severe than in the left fin. The most noticeable anomalies are the missing, thread-like distal fin tip and bifurcating pre- and postaxial lateral radials (Figures 5F,H). One pre-axial lateral radial starting at the fifth mesomere even shows a double branch, which is extraordinarily wide.
μCT-scanning as well as clearing and double staining of the left pelvis fin revealed a bent distal tip of the main axis which is caused by multiple deformed axial elements at the distal end. At the level of the fifth mesomere, bifurcation of an element of the pre-axial lateral radial is visible (Figures 5J–L).
Branching in postaxial radial was also identified in the right pelvic fin, where also one preaxial lateral radial is fused with the fourth mesomere (Figure 5N). In addition, amalgamation of adjacent axial segments along the central axis is present in three positions (Figure 5O), as is particularly well visible in the histological serial sections (Figure 5P).
Discussion
Regenerative Abilities of Lungfish
Earlier work has shown that the various extant lungfish genera are able to regenerate both tails and fins. Some studies have carried out controlled amputation experiments in the laboratory (Conant, 1970; Nogueira et al., 2016; Verissimo et al., 2020), while others reported numerous regenerated fins in natural populations (Nogueira et al., 2016). Most studies, however, are based on the South American lungfish, Lepidosiren, and the African lungfish, Protopterus. These genera are characterized by their thread-like fin structure and a lack of pre- and post-axial radial elements. To our knowledge, in contrast, nothing is known on the regenerative abilities of fins in the Australian lungfish, Neoceratodus forsteri. However, Neoceratodus is of particular interest for regeneration research, because among the modern taxa its fin anatomy resembles most closely the ancestral condition of lungfish fin anatomy, which is quite a bit more complex than that of the other two genera. The phylogenetic position of lungfish as the closest living relatives of tetrapods also makes them crucial taxa for investigations on the evolution of the regenerative program allowing for fin and tail regeneration in sarcopterygians (Verissimo et al., 2020).
While not in every single case it can be excluded that fin anomalies were caused by developmental defects, our study demonstrates that all five studied lungfish species are very likely able to regenerate fins after natural bite injuries, including the Australian lungfish. Strong indicators for ongoing regenerative processes in Neoceratodus in contrast to malformations caused by severe bite injuries, are distally branching radials, abnormal numbers of radials attached to respective mesomeres and deformed mesomeres, which strongly deviate from the original anatomy.
In an evolutionary context, these findings suggest the ability to regenerate body appendages is plesiomorphic for modern lungfish genera, which is in line with molecular studies that indicate a deep evolutionary origin of appendage regeneration (Darnet et al., 2019; Verissimo et al., 2020).
Therein, the regeneration of the missing parts of the fin occurs to various degrees, from partial to near complete regrowth, with lighter and more severe pathologies. The factors are very likely depending on the living conditions and the occurrence of repeated biting, but differences between the overall regenerative capacities of different lungfish taxa can also not be ruled out based on our data.
Regeneration Investigations Under Controlled Laboratory Conditions vs. in the Wild
The frequency of regenerated fins found in specimens in natural history collections as well as in the living specimens in animal husbandry and in the wild (Nogueira et al., 2016) strongly suggest that fin regeneration and the occurrence of associated pathologies are widespread phenomena. Since lungfish are very territorial animals, damage to a fin or loss of a fin as a result of conspecifics biting happens frequently in both in captivity and the wild. Conant (1973) observed a rate of about 20% of bite injuries among captive African lungfish and Nogueira et al. (2016) report a similar rate of almost 19% of externally visible fin pathologies in wild-caught South American lungfish. Mlewa and Green (2004), on the other hand, reported a much lower rate of pathologies in the fins and tails of African lungfish Protopterus athiopicus (approx. 4%) during their investigations in the wild. In their study they also mention developmental abnormalities, but it remains unclear if these may indeed not represent cases of failed regeneration as well.
Moreover, all of the above-mentioned studies refer to the obvious, externally visible pathologies. Here we showed that fins with a seemingly normal external morphology indeed show anatomical abnormalities when investigated by histology and/or Ct scanning methods. Hence, the actual frequency of bite injuries and regenerated body appendages in lungfish populations is difficult to determine by gross observation alone and may indeed be much higher, especially when also taking into consideration the instances where regeneration proceeds normally and results in an anatomically normal fin. Moreover, environmental factors such as population density, food availability, age distribution, and season certainly have a strong influence on aggression and bite frequency and hence regeneration frequency in all lungfish taxa. Animals housed in natural history collections or caught in the wild for a different research purpose often lack the metadata that would allow for a better assessment of the impact of these parameters on regeneration frequency and the numerical distribution of pathologies. Therefore, there are several points that limit the interpretation of the results based on this material. In retrospect, it cannot be determined exactly whether limbs were completely severed or only partially. Especially in case of the very compact fins of the Australian lungfish, it seems likely that often only parts of the fin are lost to conspecific biting rather than a whole fin. It remains unknown whether regeneration in lungfish fins is hampered by, proceeds equally well or rather proceeds better if only a part of the fin is severed from a fin as compared to the loss of a complete fin. Observations have shown that some injuries are challenging for axolotls and not all types of wounds trigger a regeneration process. For example, lateral limb wounds and larger gabs of certain dimension in long bones do not heal properly or even show no evidence for regeneration at the injury site at all (Roy and Lévesque, 2006; Hutchison et al., 2007; Lee and Gardiner, 2012; Vieira et al., 2019). These findings indicate that the mechanisms underlying regeneration are different from those of regular bone healing. It is also possible that fins are injured repeatedly, and follow-up injuries can occur after regeneration is complete, during an ongoing regeneration process or during the important phase of blastema formation. In fact, a study by Bryant et al. (2017b) on regenerative abilities in axolotl limbs has revealed a state of persistent wound healing reaction generated by multiple repeated amputations at the same site, which in turn inhibits successful regeneration of the missing limb part. Therefore it can be assumed that the more often a fin is damaged, the higher the probability that it will develop anomalies during regeneration. Furthermore, recurring bite injuries cause renewed disruption of tissue structures and hence positional information needed for the proper replacement of missing body parts. This is also indicated by the fact that anomalies in skeletal limb and fin structure do not always only occur directly at the amputation level or location of bite injury, but sometimes also in more distal regions (Bothe et al., 2021), which can hamper identification of the exact plane of amputation.
Another aspect that remains unresolved by the data at hand is the age of the animal at the time when the fin was injured and the regeneration process started. It is unknown whether the regenerative abilities or qualities vary with age in lungfish, but this is well documented in frogs and salamanders. Frogs are only able to regenerate limbs in the tadpole stage, but once metamorphosis is completed, this ability is absent in postmetamorphic individuals (Dent, 1962). Salamanders, on the other hand, are able to regenerate appendages throughout their entire lifespan (Zeleny, 1909). However, the speed and quality of regeneration seem to decrease with increasing age (Vieira et al., 2020; Bothe et al., 2021). Coincidently with this, pathologies in the regenerated axolotl occur more frequently in older animals than in young larvae (Bothe et al., 2021). Furthermore, it is usually unknown whether the regeneration process was completed or stopped when the animal died. Shortened fins, for example, could either indicate a failed or halted regeneration in the living lungfish or be the result of incomplete regeneration, because the animal died before the process could be completed.
Finally, it cannot be completely ruled out that the identified fin anomalies have not already resulted from faulty fin development.
Quality of Regeneration and Types of Anomalies
Despite the limitation of the data outlined above, it is very important to examine regeneration after bite injuries in lungfish and to compare it to controlled amputation attempts in the laboratory. Studies on salamanders indicate that pathologies and anomalies occur predominantly after bite injuries caused either by conspecifics or predators (Bothe et al., 2021). Hence, these pathologies are not random oddities, but are a common feature of the regeneration process and understanding the forms and causes of pathological regenerates is essential for understanding the underlying processes and evolutionary context of regeneration. In bites, tissue damage is much more severe than in the clean cuts of targeted amputations. The fin is torn, ruptures and is squeezed leading to frayed and disrupted tissue at the wound site. This in turn seems to often have a negative impact on wound healing, blastema development, and subsequent replacement of body parts. Multiple studies of cell identity during regeneration in the axolotl have discovered that cells at the injury site hold positional information in relation to one another along the proximodistal and anteroposterior limb axes, which are responsible for a successful rebuilding of limb structures with respect to growth and pattern formation (French et al., 1976; Bryant et al., 1981; Gardiner et al., 1995; Torok et al., 1998; Echeverri and Tanaka, 2005; Mercader et al., 2005; Roensch et al., 2013). Therefore, it appears logical that, the more severe the damage to the tissue, the more chaotic the tissue arrangement in the wound area is and the more often regenerative pathologies occur as a result to faulty positional information and pattern formation. Anomalies resulting from imperfect fin regeneration occur frequently and were visible by exterior and interior observation in all specimens studied. Some pathologies were less severe, others significantly changed the original anatomical structure of the respective lungfish fin. They include constrictions of various types of tissues, fusion of skeletal elements, distal branching and additional or less skeletal elements relative to the normal fin anatomy. The most basic element of the axillary radial (mesomere 1) articulated with the endoskeletal shoulder girdle or pelvic girdle and is rarely affected by pathologies. This is likely due to its position close to the body wall, which makes it less likely to be affected by bite injuries than more distal parts of the fin. No significant differences were registered in the type of pathology between pelvic and pectoral fins.
The data also hints at the possibility that there may be a connection between anatomical complexity and frequency and severity of regenerative pathologies. The fins of Protopterus and Lepidosiren have a less complex skeletal anatomy than the fins of Neoceratodus or a salamander limb. In Protopterus and Lepidosiren fin regeneration entails replacement of a thread-like fin without lateral radials and an overall rather simple anatomical structure, which therefore may be less prone to regenerative pathologies than the more complex structures of a Neoceratodus fin or a tetrapod limb. In accordance with this hypothesis, pathologies are less pronounced and less obvious externally, in Protopterus and Lepidosiren fins than in Neoceratodus. Moreover, the available data for Neoceratodus indicates that the more distal the injury, the less complex is the anatomical structures that has to be replaced by regeneration and the fewer pathologies occur. However, a potential connection between structure complexity and frequency of pathologies in regenerates will have to be tested in a rigorous experimental framework in order to be conclusively demonstrated or dismissed.
In any case, overall all lungfish fin anomalies follow a very similar patterns and structure and includes, failed segmentation, merging of elements, reduced or increased number of segments, bifurcation of elements, and constrictions of the various tissues and are likewise very similar to pathologies observed in regenerated salamander limbs (Dearlove and Dresden, 1976; Stock and Bryant, 1981; Bryant and Gardiner, 2016; Soto-Rojas et al., 2017; Bothe et al., 2021).
Biological Importance of Perfect Regeneration
The relative frequency with which more or less severe pathologies occur during appendage regeneration raises the question of how important the quality or anatomical perfection of the regenerated appendages is in a biological context, i.e., for the fitness of the animal.
The original function of the structures plays an important role in this context, especially with respect to paired appendages such as fins and limbs. Ecology and habitat may influence how strong the impact of a severely malformed limb regenerate is for a given individual or taxon, as e.g., the limbs are less important for effective locomotion in an aquatic axolotl that can propel through the water effectively with its tail, than for a highly terrestrial plethodontid salamander inhabiting steep rock surfaces. Likewise, a lungfish fin may be less physically strained in deep water locomotion as compared to the limb of a terrestrial salamander, but nevertheless fulfills important functions, e.g., the Australian lungfish uses its strong, fleshy fins as support for the tail when swimming forward in ascending movements, to maneuver in shallow water and to support on the substrate when eating (Dean, 1906; Kemp, 1986). Lungfish fins can also be used in a tetrapod-like fashion in Neoceratodus (Dean, 1912) which was also demonstrated for the African lungfish Protopterus annectens, despite its reduced fin anatomy (King et al., 2011). King et al. (2011) showed that pectoral fins of Protopterus are used to lift the body of the substrate for terapod-like walking and bounding movements in aquatic environments. Therefore, it is not easy to assess how strongly severe pathologies in regenerated appendages may impact the fitness of individual lungfish in natural environments. Therein, some types of pathologies such as bifurcation and fusion of skeletal elements are unlikely to have a major negative impact on movements such as swimming or supporting the body, whereas truncated or mutilated fins could severely restrict the usability of the fin for maneuvering and pushing off the ground. With this disability, these individuals could become victims of new bite attacks and predation more easily or have a disadvantage in the competition for food.
Comparison to Pathologies Occurring in Salamander Limb Regeneration
While axolotls are well known for their outstanding regenerative abilities, axolotl limbs often show a wide variety of limb and digit anomalies after bite injuries (Thompson et al., 2014; Bothe et al., 2021) that are very similar to those seen in lungfish fin regeneration. Common abnormalities after imperfect regeneration are for example syndactyly (fusion of two or more digits), ectrodactyly (split limb), brachydactyly (short digits), and limbs with additional or missing digits (Dearlove and Dresden, 1976; Young, 1977; Stock and Bryant, 1981; Bryant and Gardiner, 2016; Soto-Rojas et al., 2017; Bothe et al., 2021). Thompson et al. (2014) investigated the probability of regenerating a proper limb after bite injuries by conspecifics among larvae and adult axolotl in a laboratory setting. The rate of bite injuries among the larvae was very high with a value of 80%. After regeneration, more than half of the larvae exhibited pathologies on at least one limb, including variant digit numbers, fused digits, and digits growing from atypical anatomical positions. However, not only after conspecific biting, but also after controlled amputations with clean surgical cuts, regenerated limbs often did not regenerate perfectly (Bothe et al., 2021). Malformations occurring after surgical amputations are usually less severe and not immediately noticeable in external observation. Frequently occurring anomalies are partial or full constriction of the perichondrium, intercellular space in the cartilage matrix, narrowing of radius and ulna, reduced numbers of mesopodial bones caused by fusions and shorter digits with a reduced number of phalangeal elements.
The ability to regenerate is extremely widespread in the salamander clade, and it is therefore not surprising that regeneration pathologies have been reported not only in the axolotl, but also in other salamander species, including the Eastern newt, Notophthalmus viridescens (Dearlove and Dresden, 1976), and the red−backed salamander, Plethodon cinereus (Dinsmore and Hanken, 1986). Overall, the frequency and patterns of anomalies following regeneration are very similar in the investigated salamander taxa and all species of modern lungfish and are suggestive of shared processes governing appendage regeneration in all these taxa.
Origin of Body Appendage Regeneration
The high regenerative capacities of some organisms, but the lack thereof in others has fascinated researchers for centuries and has led to discussions on the evolution of regeneration and the reasons for its emergence and loss in various animal lineages (e.g., Bely, 2010; Bely and Nyberg, 2010; Nogueira et al., 2016; Amaral and Schneider, 2018). Among extant tetrapods, only salamanders are capable of regenerating limbs (in addition to other body parts such as tails, lenses, and parts of inner organs) throughout their entire lifespan and with near perfection. Because of its uniqueness among extant tetrapods, it was frequently suggested that this ability arose independently in the evolutionary lineage of salamanders (Garza-Garcia et al., 2010; Brockes and Gates, 2014). This assumption was supported by the discovery of salamander lineage-specific genes (LSGs) which were shown to be involved in the limb regeneration process (Kumar et al., 2007; Looso et al., 2012, 2013; Brockes and Gates, 2014). However, the fossil record showed that high regenerative capacities as seen in modern salamanders are indeed not salamander specific, but salamander-like regeneration of limbs and tails was already present in the temnospondyl- and lepospondyl lineage of anamniote tetrapods some 300 million years ago (Fröbisch et al., 2014, 2015).
The fossil data was complemented by morphological and molecular studied, which further supported an ancient origin of epigenetic regeneration in vertebrates. Nogueira et al. (2016) compared the molecular program of appendage regeneration in axolotl and the South American lungfish, Lepidosiren paradoxa, and found extensive similarities in the molecular program deployed during appendage regeneration in both taxa. They also reported stark morphological similarities in the regeneration process, including in the formation of a wound epithelium, histolysis, dedifferentiation, subsequent blastema proliferation, and repatterning of missing structures. Similarly, great similarities mechanisms in the molecular program governing tail regeneration in salamanders and lungfish were found by Verissimo et al. (2020) lending further support for a shared molecular regeneration program in sarcopterygians.
Outside of Sarcopterygii, fin regeneration including the endoskeleton was demonstrated for the basal actinopterygian Polypterus by Cuervo et al. (2012) leading to the suggestion that appendage regeneration be plesiomorphic for Osteichthyes (bony vertebrates), which, however, seemed to stand in contrast to the notion that teleost fish are capable of regenerating dermal fin radials but not of regeneration of their fin endoskeleton. In a broad approach Darnet et al. (2019) combined fin regeneration assays and comparative RNA-sequencing analysis of Polypterus and axolotl blastemas revealing a shared regeneration-specific genetic program in the basal actinopterygian and salamanders. Moreover, Darnet et al. (2019) were able to show through fin endoskeleton amputation experiments, that further non-teleost actinopterygians, namely the American paddlefish (Polyodon) and the spottet gar (Lepisosteus), as well as three teleost species were capable of full fin regeneration after endochondral amputation (Darnet et al., 2019).
The similarity in frequency and patterns of fin regeneration pathologies between all three extant lungfish genera and salamander limbs demonstrated here lend further support for the similarity of the underlying processes in and limitations to appendage regeneration in these groups.
Taken together, the morphological, paleontological, and molecular data strongly suggests that the capacity for full appendage regeneration is a plesiomorphic feature for all sarcopterygians (lobe-finned fish including four limbed vertebrates), which was lost at least once, in the amniote lineage for reasons yet unknown.
Conclusion and Outlook
The findings of this study showed that modern lungfish are valuable and promising model organisms for body appendage regeneration and can provide important evolutionary and developmental insights into the mechanisms governing vertebrate regeneration. Currently, there is a lack of data with respect to initial lungfish fin development and the ecological and biological factors influencing regeneration in lungfish, such as age dependency of regenerative capacities and regeneration after partial vs. whole fin loss. These are in part based on the elaborate conditions for animal housing and breeding, restricting access to embryos and larvae as well as controlled conditions for adult animals. Despite its enormous size, the Australian lungfish genome has recently been published (Meyer et al., 2021) and in the future, developing the lungfish into a model organism holds great potential for studies on regeneration and evolution.
The results of this study have shown that it can be difficult to recognize regenerated body parts by external observation alone and that a more detailed analysis of the anatomy and severity of anomalies can only be achieved through histological observations or CT scanning. The advancement of new imagining and molecular technologies allow for an inclusion non-model organisms in studies on regeneration, which can contribute significant new data on patterns and processes in regeneration and ultimately to the development applications in human medicine.
Data Availability Statement
The original contributions presented in the study are included in the article/Supplementary Material, further inquiries can be directed to the corresponding author/s.
Ethics Statement
Ethical review and approval was not required for the animal study because collections material was used. No animals were killed for this study.
Author Contributions
VB, IS, and NF designed the study, discussed the data, and wrote the manuscript. VB compiled and analyzed the data. All authors contributed to the article and approved the submitted version.
Funding
The project was funded by the DFG Grant (FR 2647/8-1) to NF.
Conflict of Interest
The authors declare that the research was conducted in the absence of any commercial or financial relationships that could be construed as a potential conflict of interest.
Publisher’s Note
All claims expressed in this article are solely those of the authors and do not necessarily represent those of their affiliated organizations, or those of the publisher, the editors and the reviewers. Any product that may be evaluated in this article, or claim that may be made by its manufacturer, is not guaranteed or endorsed by the publisher.
Acknowledgments
We would like to thank Peter Bartsch, Edda Aßel, Olivier Pauwels, Emmanuel Vreven, and Miguël Parrent for access to the material; Kristin Mahlow for performing the CT scans and Dr. Christian Mitgutsch for his support in the laboratory.
Supplementary Material
The Supplementary Material for this article can be found online at: https://www.frontiersin.org/articles/10.3389/fevo.2021.784828/full#supplementary-material
Supplementary Figure 1 | Pectoral fins of three lungfish, Neoceratodus forsteri. (A–F) Fins with obvious signs of fin regeneration. (G–L) Same fins after two years with no significant changes in shape.
Supplementary Table 1 | Histological staining protocol for Heidenhain’s Azan.
Supplementary Table 2 | Histological staining protocol for Alcian blue/nuclear fast red.
References
Alvarado, A. S. (2000). Regeneration in the metazoans: why does it happen? Bioessays 22:578590. doi: 10.1002/(SICI)1521-1878(200006)22:6<578::AID-BIES11>3.0.CO;2-#
Amaral, D. B., and Schneider, I. (2018). Fins into limbs: recent insights from Sarcopterygian fish. Genesis 56:e23052. doi: 10.1002/dvg.23052
Amemiya, C. T., Alföldi, J., Lee, A. P., Fan, S., Philippe, H., MacCallum, I., et al. (2013). The African coelacanth genome provides insights into tetrapod evolution. Nature 496, 311–316.
Bely, A. E. (2010). Evolutionary loss of animal regeneration: pattern and process. Integr. Comp. Biol. 50, 515–527. doi: 10.1093/icb/icq118
Bely, A. E., and Nyberg, K. G. (2010). Evolution of animal regeneration: re-emergence of a field. Trends Ecol. Evol. 25, 161–170. doi: 10.1016/j.tree.2009.08.005
Bischoff, T. L. W. (1840). Lepidosiren Paradoxa: Anatomisch Untersucht und Beschrieben. Leipzig: Verlag von Leopold Voss.
Biscotti, M. A., Gerdol, M., Canapa, A., Forconi, M., Olmo, E., Pallavicini, A., et al. (2016). The lungfish transcriptome: a glimpse into molecular evolution events at the transition from water to land. Sci. Rep. 6:21571. doi: 10.1038/srep21571
Boisvert, C. A., Joss, J. M., and Ahlberg, P. E. (2013). Comparative pelvic development of the axolotl (Ambystoma mexicanum) and the Australian lungfish (Neoceratodus forsteri): conservation and innovation across the fish-tetrapod transition. EvoDevo 4:3.
Bothe, V., Mahlow, K., and Fröbisch, N. B. (2021). A histological study of normal and pathological limb regeneration in the Mexican axolotl Ambystoma mexicanum. J. Exp. Zool. Part B Mol. Dev. Evol. 336, 116–128.
Brockes, J. P., and Gates, P. B. (2014). Mechanisms underlying vertebrate limb regeneration: lessons from the salamander. Biochem. Soc. Trans. 42, 625–630.
Bryant, D. M., Johnson, K., DiTommaso, T., Tickle, T., Couger, M. B., Payzin-Dogru, D., et al. (2017a). A tissue-mapped axolotl de novo transcriptome enables identification of limb regeneration factors. Cell Rep. 18, 762–776.
Bryant, D. M., Sousounis, K., Payzin-Dogru, D., Bryant, S., Sandoval, A. G. W., Fernandez, J. M., et al. (2017b). Identification of regenerative roadblocks via repeat deployment of limb regeneration in axolotls. NPJ Regen. Med. 2:30.
Bryant, S. V., and Gardiner, D. M. (2016). The relationship between growth and pattern formation. Regeneration 3, 103–122.
Bryant, S. V., French, V., and Bryant, P. J. (1981). Distal regeneration and symmetry. Science 212, 993–1002. doi: 10.1126/science.212.4498.993
Chang, M. M., and Yu, X. (1984). Structure and phyogenetic significance of Diabolepis speratus gen. et sp. nov., a new dipnoan-like form from the Lower Devonian of eastern Yunnan, China. Proc. Linn. Soc. N. S. W. 107, 171–184.
Conant, E. B. (1970). Regeneration in the African lungfish, Protopterus. I. Gross aspects. J. Exp. Zool. 174, 15–31. doi: 10.1002/jez.1401740103
Conant, E. B. (1973). Regeneration in the African lungfish, Protopterus. III. Regeneration during fasting and estivation. Biol. Bull. 144, 248–261. doi: 10.2307/1540006
Cuervo, R., Hernández-Martínez, R., Chimal-Monroy, J., Merchant-Larios, H., and Covarrubias, L. (2012). Full regeneration of the tribasal Polypterus fin. Proc. Natl. Acad. Sci. U.S.A. 109, 3838–3843. doi: 10.1073/pnas.1006619109
Curry-Lindahl, K. (1956). On the ecology, feeding behaviour and territoriality of the African lungfish, Protopterus aethiopicus Heckel. Arkiv Zool. 9, 479–497.
Darnet, S., Dragalzew, A. C., Amaral, D. B., Sousa, J. F., Thompson, A. W., Cass, A. N., et al. (2019). Deep evolutionary origin of limb and fin regeneration. Proc. Natl. Acad. Sci. U.S.A. 116, 15106–15115. doi: 10.1073/pnas.1900475116
Dean, B. (1906). “Notes on the living specimens of the Australian lungfish, Ceratodus forsteri, in the Zoological Society’s collection,” in Proceedings of the Zoological Society of London, Vol. 76, (Oxford: Blackwell Publishing Ltd), 168–178. doi: 10.1111/j.1469-7998.1906.tb08428.x
Dean, B. (1912). Additional notes on the living specimens of the Australian Lung-fish (Ceratodus forsteri) in the collection of the Zoological Society of London. Proc. Zool. Soc. Lond. 82, 607–612. doi: 10.1111/j.1469-7998.1912.tb07541.x
Dearlove, G. E., and Dresden, M. H. (1976). Regenerative abnormalities in Notophthalmus viridescens induced by repeated amputations. J. Exp. Zool. 196, 251–261. doi: 10.1002/jez.1401960212
Dent, J. N. (1962). Limb regeneration in larvae and metamorphosing individuals of the South African clawed toad. J. Morphol. 110, 61–77. doi: 10.1002/jmor.1051100105
Department of the Environment, Water, Heritage and the Arts (2009). Neoceratodus forsteri – Australian lungfish” (Online). Canberra, ACT: Department of the Environment, Water, Heritage and the Arts.
Dinsmore, C. E., and Hanken, J. (1986). Native variant limb skeletal patterns in the red-backed salamander, Plethodon cinereus, are not regenerated. J. Morphol. 190, 191–200. doi: 10.1002/jmor.1051900204
Echeverri, K., and Tanaka, E. M. (2005). Proximodistal patterning during limb regeneration. Dev. Biol. 279, 391–401. doi: 10.1016/j.ydbio.2004.12.029
Ericsson, R., Joss, J., and Olsson, L. (2010). “Early head development in the Australian lungfish, Neoceratodus forsteri,” in The Biology of Lungfishes, eds J. M. Jorgensen and J. Joss (Boca Raton, FL: CRC Press), 165–184.
French, V., Bryant, P. J., and Bryant, S. V. (1976). Pattern regulation in epimorphic fields. Science 193, 969–981.
Fröbisch, N. B., Bickelmann, C., and Witzmann, F. (2014). Early evolution of limb regeneration in tetrapods: evidence from a 300-million-year-old amphibian. Proc. R. Soc. B Biol. Sci. 281:20141550. doi: 10.1098/rspb.2014.1550
Fröbisch, N. B., Bickelmann, C., Olori, J. C., and Witzmann, F. (2015). Deep-time evolution of regeneration and preaxial polarity in tetrapod limb development. Nature 527, 231–234. doi: 10.1038/nature15397
Gardiner, D. M., Blumberg, B., Komine, Y., and Bryant, S. V. (1995). Regulation of HoxA expression in developing and regenerating axolotl limbs. Development 121, 1731–1741. doi: 10.1242/dev.121.6.1731
Garza-Garcia, A. A., Driscoll, P. C., and Brockes, J. P. (2010). Evidence for the local evolution of mechanisms underlying limb regeneration in salamanders. Integr. Comp. Biol. 50, 528–535. doi: 10.1093/icb/icq022
Girvan, J. E., Olson, W. M., and Hall, B. K. (2002). Hind-limb regeneration in the dwarf African clawed frog, Hymenochirus boettgeri (Anura: pipidae). J. Herpetol. 36, 537–543.
Hutchison, C., Pilote, M., and Roy, S. (2007). The axolotl limb: a model for bone development, regeneration and fracture healing. Bone 40, 45–56. doi: 10.1016/j.bone.2006.07.005
Irisarri, I., and Meyer, A. (2016). The identification of the closest living relative (s) of tetrapods: phylogenomic lessons for resolving short ancient internodes. Syst. Biol. 65, 1057–1075. doi: 10.1093/sysbio/syw057
Johanson, Z., Joss, J., Boisvert, C. A., Ericsson, R., Sutija, M., and Ahlberg, P. E. (2007). Fish fingers: digit homologues in Sarcopterygian fish fins. J. Exp. Zool. Part B Mol. Dev. Evol. 308, 757–768. doi: 10.1002/jez.b.21197
Kardong, K. V. (1997). Vertebrates: Comparative Anatomy, Function, Evolution. Houston, TX: Heinle and Heinle Publishers.
Kemp, A. (1986). The biology of the Australian lungfish, Neoceratodus forsteri (Krefft 1870). J. Morphol. 190, 181–198.
Kind, P. K. (2002). Movement Patterns and Habitat Use in the Queensland Lungfish Neoceratodus forsteri (Krefft 1870). Ph.D. thesis. St Lucia, QLD: The University of Queensland.
King, H. M., Shubin, N. H., Coates, M. I., and Hale, M. E. (2011). Behavioral evidence for the evolution of walking and bounding before terrestriality in sarcopterygian fishes. Proc. Natl. Acad. Sci. U.S.A. 108, 21146–21151. doi: 10.1073/pnas.1118669109
Kragl, M., Knapp, D., Nacu, E., Khattak, S., Maden, M., Epperlein, H. H., et al. (2009). Cells keep a memory of their tissue origin during axolotl limb regeneration. Nature 460, 60–65. doi: 10.1038/nature08152
Kumar, A., Godwin, J. W., Gates, P. B., Garza-Garcia, A. A., and Brockes, J. P. (2007). Molecular basis for the nerve dependence of limb regeneration in an adult vertebrate. Science 318, 772–777. doi: 10.1126/science.1147710
Lee, J., and Gardiner, D. M. (2012). Regeneration of limb joints in the axolotl (Ambystoma mexicanum). PLoS One 7:e5061. doi: 10.1371/journal.pone.0050615
Lenhoff, H. M., and Lenhoff, S. G. (1991). “Abraham Trembley and the origins of research on regeneration in animals,” in A History of Regeneration Research. Milestones in the Evolution of a Science, ed. C. E. Dinsmore (Cambridge: Cambridge University Press), 47–66. doi: 10.3389/fgene.2021.670695
Lima, S. Q., Costa, C. M., Amemiya, C. T., and Schneider, I. (2017). Morphological and molecular analyses of an anatomical novelty: the pelvic fin filaments of the South American lungfish. J. Exp. Zool. Part B Mol. Dev. Evol. 328, 97–105. doi: 10.1002/jez.b.22711
Londono, R., Sun, A. X., Tuan, R. S., and Lozito, T. P. (2018). Tissue repair and epimorphic regeneration: an overview. Curr. Pathobiol. Rep. 6, 61–69.
Looso, M., Michel, C. S., Konzer, A., Bruckskotten, M., Borchardt, T., Krüger, M., et al. (2012). Spiked-in pulsed in vivo labeling identifies a new member of the CCN family in regenerating newt hearts. J. Proteome Res. 11, 4693–4704. doi: 10.1021/pr300521p
Looso, M., Preussner, J., Sousounis, K., Bruckskotten, M., Michel, C. S., Lignelli, E., et al. (2013). A de novo assembly of the newt transcriptome combined with proteomic validation identifies new protein families expressed during tissue regeneration. Genome Biol. 14:R16. doi: 10.1186/gb-2013-14-2-r16
McCusker, C., Bryant, S. V., and Gardiner, D. M. (2015). The axolotl limb blastema: cellular and molecular mechanisms driving blastema formation and limb regeneration in tetrapods. Regeneration 2, 54–71. doi: 10.1002/reg2.32
Mercader, N., Tanaka, E. M., and Torres, M. (2005). Proximodistal identity during vertebrate limb regeneration is regulated by Meis homeodomain proteins. Development 132, 4131–4142. doi: 10.1242/dev.01976
Meyer, A., Schloissnig, S., Franchini, P., Du, K., Woltering, J. M., Irisarri, I., et al. (2021). Giant lungfish genome elucidates the conquest of land by vertebrates. Nature 590, 284–289. doi: 10.1038/s41586-021-03198-8
Mlewa, C. M., and Green, J. M. (2004). Biology of the marbled lungfish, Protopterus aethiopicus Heckel, in Lake Baringo, Kenya. Afr. J. Ecol. 42, 338–345. doi: 10.1111/j.1365-2028.2004.00536.x
Morgan, T. H. (1898). Experimental studies of the regeneration of Planaria maculata. Dev. Genes Evol. 7, 364–397. doi: 10.1007/bf02161491
Nelson, J. S., Grande, T. C., and Wilson, M. V. (2016). Fishes of the World. Hoboken, NJ: John Wiley & Sons.
Nogueira, A. F., Costa, C. M., Lorena, J., Moreira, R. N., Frota-Lima, G. N., Furtado, C., et al. (2016). Tetrapod limb and sarcopterygian fin regeneration share a core genetic programme. Nat. Commun. 7:13364. doi: 10.1038/ncomms13364
Ovchinnikov, D. (2009). Alcian blue/alizarin red staining of cartilage and bone in mouse. Cold Spring Harb. Protoc. 2009:pdb-prot5170.
Reaumur, R.-A. F. D. (1712). Sur les diverses reproductions qui se fontdans les Ecrivisses, les Omars, les Crabes, etc. et entre Autressur celles de leurs jambes et de Leurs ecailles. Mem. R. Sci. 12, 226–245.
Reilly, S. M., and Lauder, G. V. (1990). The evolution of tetrapod feeding behavior: kinematic homologies in prey transport. Evolution 44, 1542–1557. doi: 10.1111/j.1558-5646.1990.tb03845.x
Roensch, K., Tazaki, A., Chara, O., and Tanaka, E. M. (2013). Progressive specification rather than intercalation of segments during limb regeneration. Science 342, 1375–1379. doi: 10.1126/science.1241796
Romer, A. S., Frick, H., and Starck, D. (1959). Vergleichende Anatomie der Wirbeltiere. Berlin: Parey.
Roy, S., and Lévesque, M. (2006). Limb regeneration in axolotl: is it superhealing? Sci. World J. 6, 12–25. doi: 10.1100/tsw.2006.324
Soto-Rojas, C., Suazo-Ortuño, I., Montoya Laos, J. A., and Alvarado-Díaz, J. (2017). Habitat quality affects the incidence of morphological abnormalities in the endangered salamander Ambystoma ordinarium. PLoS One 12:e0183573. doi: 10.1371/journal.pone.0183573
Stock, G. B., and Bryant, S. V. (1981). Studies of digit regeneration and their implications for theories of development and evolution of vertebrate limbs. J. Exp. Zool. 216, 423–433. doi: 10.1002/jez.1402160311
Thompson, S., Muzinic, L., Muzinic, C., Niemiller, M. L., and Voss, S. R. (2014). Probability of regenerating a normal limb after bite injury in the Mexican axolotl (Ambystoma mexicanum). Regeneration 1, 27–32. doi: 10.1002/reg2.17
Torok, M. A., Gardiner, D. M., Shubin, N. H., and Bryant, S. V. (1998). Expression of HoxD genes in developing and regenerating axolotl limbs. Dev. Biol. 200, 225–233. doi: 10.1006/dbio.1998.8956
Traquair, R. H. (1871). On the restoration of the tail in Protopterus annectens. Rep. Brit. Ass. Adv. Sci. 1871:143.
Verissimo, K. M., Perez, L. N., Dragalzew, A. C., Senevirathne, G., Darnet, S., Barroso Mendes, W. R., et al. (2020). Salamander-like tail regeneration in the West African lungfish. Proc. R. Soc. B 287:20192939. doi: 10.1098/rspb.2019.2939
Vieira, W. A., Wells, K. M., and McCusker, C. D. (2020). Advancements to the axolotl model for regeneration and aging. Gerontology 66, 212–222. doi: 10.1159/000504294
Vieira, W. A., Wells, K. M., Raymond, M. J., De Souza, L., Garcia, E., and McCusker, C. D. (2019). FGF, BMP, and RA signaling are sufficient for the induction of complete limb regeneration from non-regenerating wounds on Ambystoma mexicanum limbs. Dev. Biol. 451, 146–157. doi: 10.1016/j.ydbio.2019.04.008
Young, H. E. (1977). Anomalies of limb regeneration in the adult salamander, Ambystoma annulatum. J. Arkansas Acad. Sci. 31, 110–111.
Zeleny, C. (1909). The relation between degree of injury and rate of regeneration—additional observations and general discussion. J. Exp. Zool. 7, 513–561. doi: 10.1002/jez.1400070306
Keywords: regeneration, lungfish, pathologies, salamander, axolotl
Citation: Bothe V, Schneider I and Fröbisch NB (2021) A Morphological and Histological Investigation of Imperfect Lungfish Fin Regeneration. Front. Ecol. Evol. 9:784828. doi: 10.3389/fevo.2021.784828
Received: 28 September 2021; Accepted: 08 November 2021;
Published: 02 December 2021.
Edited by:
Alessandro Minelli, University of Padua, ItalyReviewed by:
Ryan Robert Kerney, Gettysburg College, United StatesRenata Freitas, Universidade do Porto, Portugal
Karen Echeverri, Marine Biological Laboratory (MBL), United States
Copyright © 2021 Bothe, Schneider and Fröbisch. This is an open-access article distributed under the terms of the Creative Commons Attribution License (CC BY). The use, distribution or reproduction in other forums is permitted, provided the original author(s) and the copyright owner(s) are credited and that the original publication in this journal is cited, in accordance with accepted academic practice. No use, distribution or reproduction is permitted which does not comply with these terms.
*Correspondence: Vivien Bothe, Vml2aWVuLkJvdGhlQG1mbi5iZXJsaW4=