- 1Animal Ecology, University of Potsdam, Potsdam, Germany
- 2Leibniz Institute of Freshwater Ecology and Inland Fisheries (IGB), Berlin, Germany
Differences in natural light conditions caused by changes in moonlight are known to affect perceived predation risk in many nocturnal prey species. As artificial light at night (ALAN) is steadily increasing in space and intensity, it has the potential to change movement and foraging behavior of many species as it might increase perceived predation risk and mask natural light cycles. We investigated if partial nighttime illumination leads to changes in foraging behavior during the night and the subsequent day in a small mammal and whether these changes are related to animal personalities. We subjected bank voles to partial nighttime illumination in a foraging landscape under laboratory conditions and in large grassland enclosures under near natural conditions. We measured giving-up density of food in illuminated and dark artificial seed patches and video recorded the movement of animals. While animals reduced number of visits to illuminated seed patches at night, they increased visits to these patches at the following day compared to dark seed patches. Overall, bold individuals had lower giving-up densities than shy individuals but this difference increased at day in formerly illuminated seed patches. Small mammals thus showed carry-over effects on daytime foraging behavior due to ALAN, i.e., nocturnal illumination has the potential to affect intra- and interspecific interactions during both night and day with possible changes in personality structure within populations and altered predator-prey dynamics.
Introduction
When animals show foraging behavior, they constantly have to face the trade-off between acquiring food and avoiding predation (Sih, 1980; Lima and Dill, 1990). As predation risk varies across space and time, prey reduce foraging at certain times and/or in certain microhabitats to reduce the risk of being preyed upon (Lima and Dill, 1990; Jacob and Brown, 2000). A well-known environmental factor that influences foraging activity in many nocturnally active species is moonlight (Kronfeld-Schor et al., 2013). Under bright moonlight, animals often reduce foraging activity itself (Daly et al., 1992; Kotler et al., 2010) or shift their activity toward safer habitats with a higher amount of cover (Bowers, 1988; Perea et al., 2011). This shows that higher levels of nighttime illumination lead to an increase in the perceived predation risk in many species, especially in small mammals.
However, the use of artificial light at night (ALAN) has increased dramatically over the last decades, eliminating very dark conditions in large areas in and surrounding urban centers (Hölker et al., 2010a; Falchi et al., 2016). The spatial spread and intensity of nighttime illumination is predicted to steadily increase (Kyba et al., 2017) especially through the development and widespread adoption of new energy-efficient lighting systems such as the light-emitting diode (LED; Kyba et al., 2017; Donatello et al., 2019). Several studies show that ALAN influences several aspects of an animals’ behavior such as timing of activity (de Jong et al., 2017; Eccard et al., 2018), movement (Hoffmann et al., 2018; Laforge et al., 2019), reproduction (Baker and Richardson, 2006; Russ et al., 2017) and foraging (Bird et al., 2004), which in turn can alter social interactions and group dynamics (Kurvers and Hölker, 2015). Under natural conditions, many animals experience dark nights as periods where the perceived predation risk is low and foraging can be extended or expanded to open habitats. In areas that are constantly polluted by ALAN, these foraging opportunities disappear. LED lamps are predicted to have particularly strong negative effects on the environment as they typically have a broad emission spectrum that can affect a large range of species (Gaston et al., 2013; Davies et al., 2017) and are used at higher light intensities due to their higher energy efficiency (rebound effect; Herring and Roy, 2007; Kyba et al., 2014, 2017). Light pollution often affects large areas and illuminates them homogenously through sky glow. However, LED street lamps and other strong light sources may create illumination of high intensity on a local scale. The attraction (e.g., insects, insect predators) or avoidance (e.g., prey animals) of these illuminated areas in an area of heterogenous light pollution is not well understood.
Most research focusses on the influence of ALAN on nocturnal species as they are expected to suffer severely from the loss of the nocturnal niche (Hölker et al., 2010b). Nevertheless, there are several mammal species such as shrews, voles and lemurs that show a polyphasic activity pattern or cathemerality with activity bouts during both day and night (Curtis and Rasmussen, 2006; Halle, 2006) that might be strongly affected by ALAN as well. However, there are few studies, which focus on this group of species and/or chronotypes in the context of changing nighttime conditions (Hoffmann et al., 2018, 2019; van Grunsven et al., 2018). These species might not only show changes in nocturnal behavior when subjected to ALAN but there might be carry-over effects to their diurnal behavior. For example, when animals face increased perceived predation risk during the night through elevated illumination levels and reduce their foraging accordingly, they might compensate for this by increasing foraging during the day exposing them to additional stressors or predation risks. Similarly, under heterogenous nighttime illumination animals might avoid brighter areas at night and seek them out during the day to compensate for lost foraging opportunities. Studies on the influence of moonlight on activity and foraging behavior of rodents show a reduced activity at night under full moon while activity at twilight and day, respectively, was increased (Daly et al., 1992; Gutman et al., 2011). However, studies on the influence of ALAN on behavior during the day using realistic and/or heterogenous light pollution scenarios are scarce (but see Kurvers et al., 2018).
Individuals within a species might respond differently to human-induced changes driven by personality traits (Gosling, 2001). This includes their risk-taking behavior (Wilson et al., 1994). While relatively shy individuals favor reducing predation risk over the opportunity to gain access to food resources or mates, bold individuals maximize their fitness through gaining access to more or higher-quality resources while as a consequence having a higher predation risk (Réale et al., 2000; Smith and Blumstein, 2008; Ballew et al., 2017; Kashon and Carlson, 2018). As ALAN is potentially altering the perceived predation risk of animals, individuals that show inter-individual differences in their risk-taking behavior might respond differently to this anthropogenic change (Merrick and Koprowski, 2017). Shy individuals might be at a disadvantage compared to bold individuals as they miss out on foraging opportunities by avoiding illuminated areas. Bold insectivorous animals might actively seek out the areas surrounding street lamps to take advantage of the higher abundance of insects that were attracted by light. On the other hand, bold individuals might be exposed to an increased predation risk if the respective predators are not deterred by nighttime illumination and are able to use the better visual conditions to increase predation success themselves. While some predatory animal species such as spiders (Willmott et al., 2019), bats (Stone et al., 2015) and owls (Canário et al., 2012) experience increased foraging opportunities around light sources and can themselves be increasingly preyed upon (Negro et al., 2000), it remains unclear how this is influenced by inter-individual differences.
We conducted a laboratory experiment and an experiment using large grassland enclosures to investigate the effects of partial illumination created by common LED lamps on the movement and foraging behavior of the bank vole (Myodes glareolus). The bank vole is a common polyphasic rodent species (Halle, 2006) that is widely distributed throughout Eurasia. It feeds on plant and animal matter (Hansson and Larsson, 1978) and is important prey for a wide range of avian and mammalian predators (Norrdahl and Korpimäki, 1995). As the species shows consistent inter-individual differences in behavior (Korpela et al., 2011; Šíchová et al., 2014; Mazza et al., 2018; Schirmer et al., 2019), it is a suitable model organism to investigate the impact of partial nighttime illumination on the foraging behavior of individuals differing in their personality. Under laboratory conditions we expect voles to reduce activity and foraging in illuminated areas due to a perceived increase in predation risk. As a consequence, higher nighttime giving-up densities (GUDs) should be measurable in illuminated compared to dark foraging patches. We further predict that voles compensate for the restricted foraging opportunities at night by foraging more in the formerly illuminated patches at day compared to the patches that are dark at night. Shy individuals should avoid illuminated areas while bold individuals may increase foraging due to higher risk-taking behavior.
In naturally vegetated outdoor enclosures we expect similar behavioral responses albeit potentially reduced compared to the laboratory experiment due to lower light intensity and dense vegetation cover. In a previous study we found that bank voles reduced their home ranges in outdoor enclosures under relatively homogeneous nighttime illumination (Hoffmann et al., 2018) but could not determine if this was due to voles opportunistically exploiting food resources from insect attraction to lighting or if it was due to reduced activity through perceived predation risk. This study measures foraging and movement behavior in both illuminated and dark patches to better address predation risk behavior under ALAN.
Materials and Methods
Laboratory Experiment
Study Subjects and Housing
The experiment was conducted under laboratory conditions from April to June 2018 using 21 adult male bank voles. Voles were wild-captured from July to September 2017 at four sites in and surrounding Potsdam, Germany with low levels of light pollution (for GPS coordinates see Supplementary Material 1). Animals were housed individually in standard makrolon cages (Ehret GmbH Germany, type III; 42 cm × 27 cm × 16 cm) and were provided with ad libitum water, hay and food pellets (ssniff® NM, ssniff® R/M-H Ered II). The room was shielded from natural light while ceiling light tubes (Osram Lumilux T8 L 58W/830 3,000 K and L 58W/840 4,000 K, Osram, Germany; for spectral distributions see links in Supplementary Material 2) simulated daylight from 8 am to 8 pm with an average light intensity of 254 ± 44 lx at cage level. Millet was offered 1 week prior to testing so the animals could get accustomed to its taste and scent. Additionally, a wooden nest box (20 cm × 14 cm × 10 cm) was introduced to the cages so that animals could be moved with the nest box to the foraging arena without handling by humans. Animals were weighed directly before entering the arena.
Experimental Design
Four foraging arenas (240 cm × 175 cm × 75 cm) were set up in a room secluded from outdoor light. Each of the arenas was divided by a wall into two compartments of equal size. The separating wall stopped 10 cm above the ground so that the animals were able to move freely around the whole arena (Figure 1).
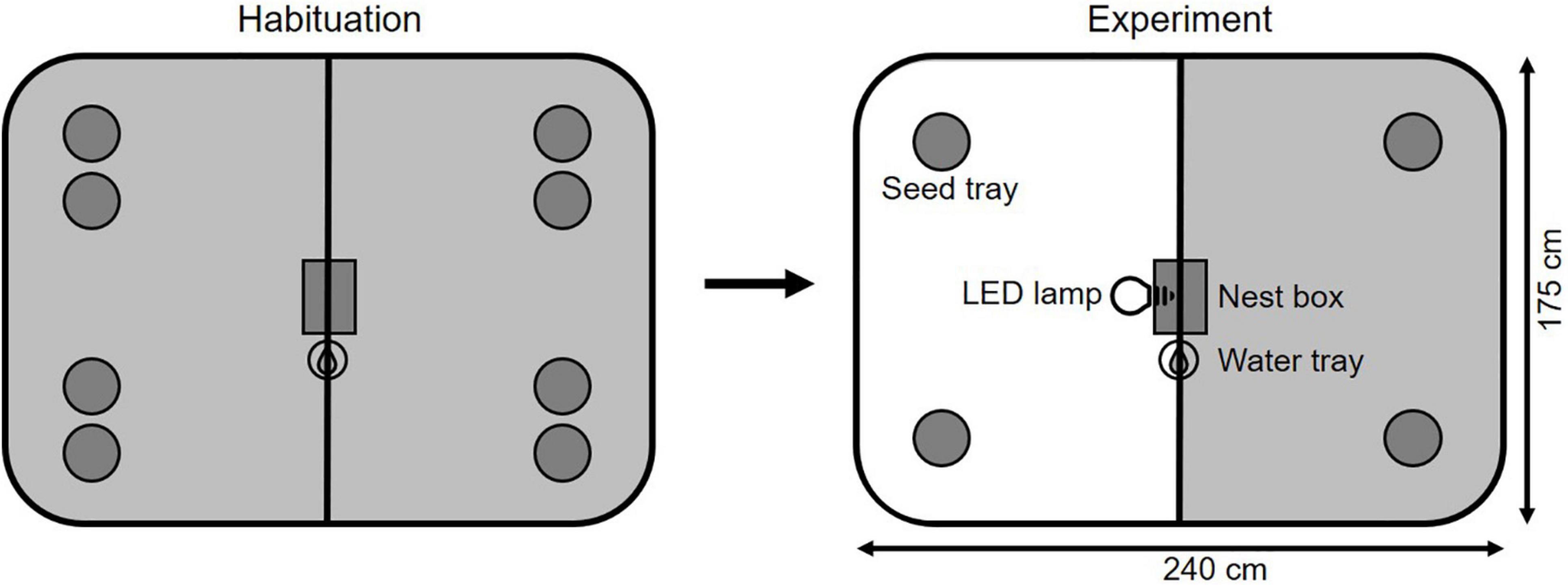
Figure 1. Experimental arena with seed trays containing 450 ml sand and 0.6 g millet. Each of the arenas was divided by a wall into two compartments of equal size. The separating wall stopped 10 cm above the ground so that the animals were able to move freely around the whole arena. Number of seed trays was halved from the habituation phase to the experimental phase as seed trays were exchanged once a day in the habituation phase and twice in the experimental phase. The habituation phase was characterized by dark nights, in the experimental phase one side of the arena was artificially illuminated at night.
During daytime (8 am – 8 pm) the room was illuminated by the same lamps as the housing room with an average light intensity of 246 ± 22 lx at ground level. During nighttime (8 pm – 8 am), the room was either completely dark or one side of each arena was illuminated by a LED lamp (Paulmann Licht GmbH LED AGL: 6.5 W, 470 lm, 2,700 K, Germany) with an average light intensity of 8.5 ± 0.9 lx at ground level while the other side was shielded from that light by the separating wall. To create a light intensity similar to nighttime street light, the lamps were covered with two filter foils [Rosco e-color No. 209 (51% transmission) and No. 210 (24% transmission), United Kingdom]. Light intensities were measured using a lux meter (Extech HD450, United States, measuring range: 0.1 – 400,000 lx).
At the start of the experiment, each animal was placed individually with the nest box in the center of an arena. As the nest box had two exits on opposite sides, voles were able to enter either side of the arena directly. Water was provided ad libitum next to the nest box. Above each arena a camera (ABUS analog HD 720p outdoor dome camera, Germany) was mounted that video-recorded the movement of the animals outside of the nest box during the experiment.
Animals were placed in the arenas for four nights. They were introduced at 7 pm on the first day and left to habituate to the arena for the first two nights. During this period the animals were provided with eight seed trays (13 cm × 13 cm × 4.5 cm) containing 450 ml of sand and 0.6 g of millet each, which were placed pairwise in the corners of the arena 40 cm from the wall. These seed trays were replaced with new trays every 24 h and no nighttime illumination was present. In the following night, one side of the arena was illuminated. During the treatment night and the following day, seed trays were reduced to four per arena and were replaced at 8:30 am and 7:30 pm, respectively. The millet remaining in each seed tray was weighted to determine the giving-up density (GUD) for each tray and the variation among GUDs between the two seed trays within one side. This was done for the treatment night and the following day. Using video recordings, we analyzed the movement and foraging behavior of voles. To quantify time spent on either side of the arena (dark or illuminated) at night and day the position of the animal was recorded every 5 s. Additionally, we measured the cumulative time animals spent in the seed trays and the number of visits to the seed trays per side of the arena at night and day. As time spent on either side of the arena and time spent in seed trays are highly correlated (Spearman correlation; ρ = 0.94, P < 0.001, N = 84), we will only present and discuss the results of the behavioral variable time spent in trays.
To quantify the personality type of individuals along a shyness-boldness axis, we measured latency to emerge from the familiar nest box with the full body in the very first night (latency body). Emergence tests (Dark-Light tests) are a common measure of boldness in other studies of wild, small rodents and are related to other measures of risk taking (Herde and Eccard, 2013; Schirmer et al., 2019). A short latency body is regarded to characterize bolder individuals and a longer to characterize shyer individuals. We confirmed that first emergence from a nest box in the arena was repeatable in a pilot study (R = 0.429, P = 0.014, NIndividuals = 26; Hoffmann et al., 2020).
Data Analysis and Statistics
We analyzed the effects of light treatment, latency body, daytime and initial body mass on the response variables time in trays, number of tray visits, GUD and variation in GUD by using linear mixed models (LMMs). Full models included a three-way interaction of light treatment, latency body and daytime and the single factor initial body mass as fixed effects. The models further contained a random factor consisting of the individual nested in the experimental group to account for repeated measures. We log transformed the variables time in trays, number of tray visits and variation in GUD to be able to use LMMs. Latency body was scaled by dividing the centered data points by their standard deviations.
We used the Akaike Information Criterion (AIC) to reduce the models via stepwise backwards selection to find the most parsimonious model (Table 1). Non-significant interactions were excluded from the model to allow for interpretation of lower order effects. The random factor experimental group was excluded from the LMM analyzing GUD since it explained no variance. The proportion of explained variance in the most parsimonious models by the fixed factors alone (marginal R2) and fixed and random factors combined (conditional R2) was assessed according to Nakagawa and Schielzeth (2013). The Wald test (χ2) and calculated confidence intervals were used to subsequently determine the significance of fixed factors from the minimal models. If the confidence intervals of fixed factors excluded zero post hoc tests were conducted. The most parsimonious model of variation in GUD included a three-way interaction of treatment, latency body and daytime while initial body mass had no effect (Tables 1, 2). Since this interaction had a confidence interval including zero, we were not able to conduct a post hoc analysis.
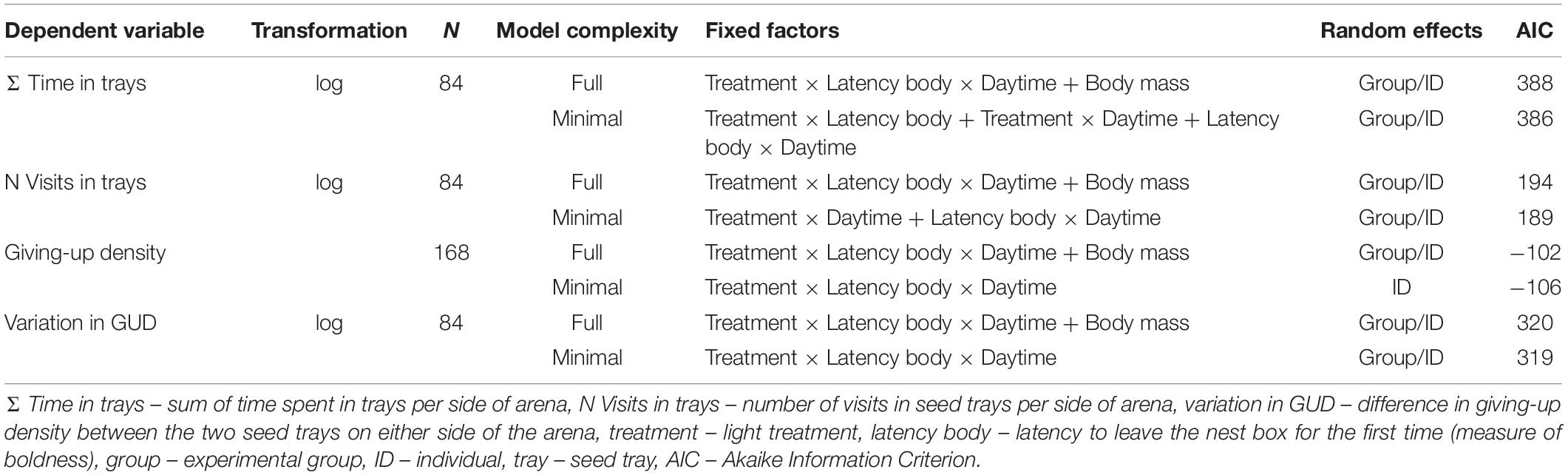
Table 1. Full and minimal linear mixed models testing the effects of independent variables and their interactions on response variables measured during the arena experiment in bank voles (NIndividuals = 21).
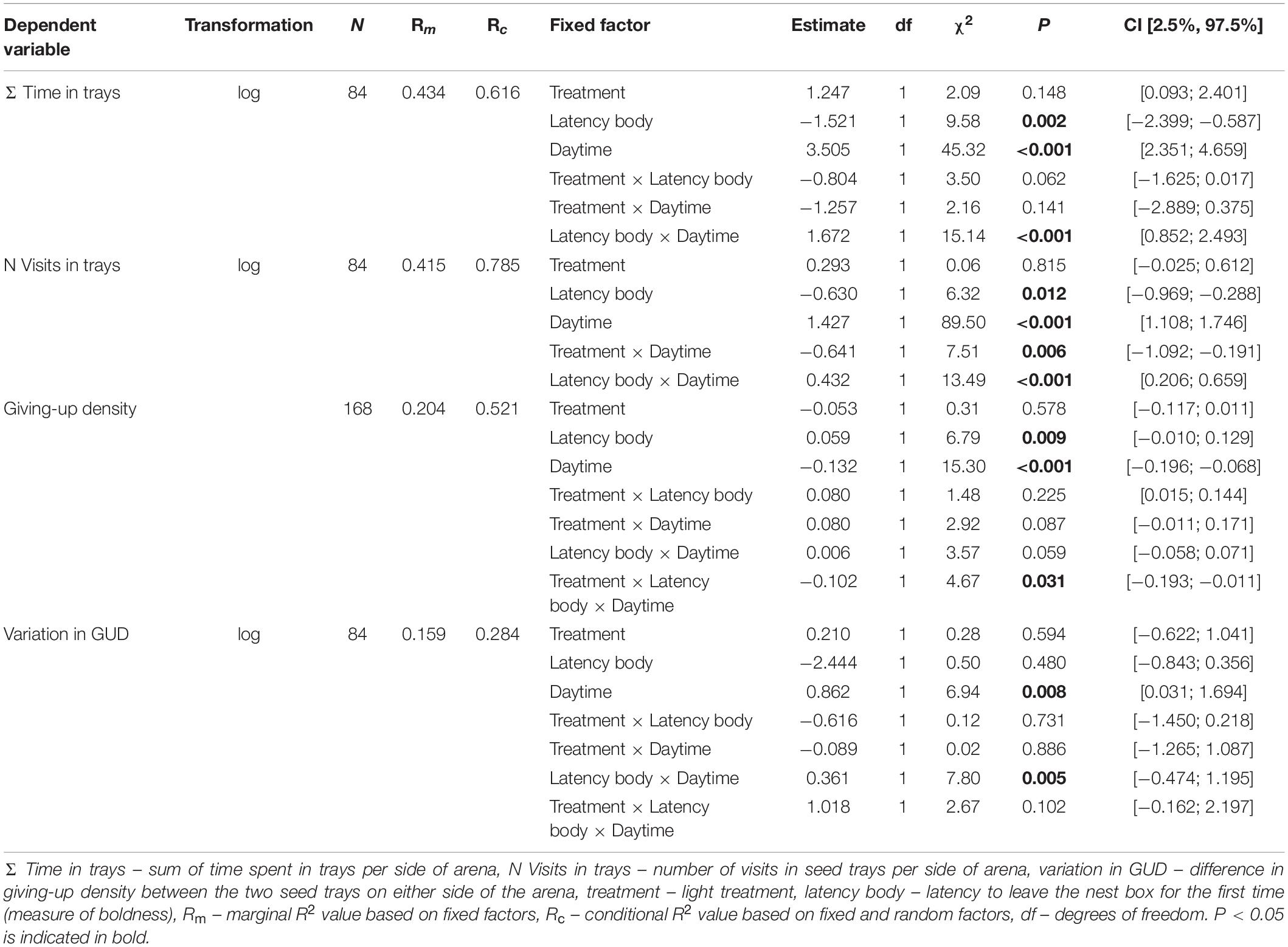
Table 2. Results of Wald chi-square (χ2) tests for linear mixed models showing the effects of fixed factors on behavioral response variables of bank voles (NIndividuals = 21).
Interactions of two categorical fixed factors were analyzed via a pairwise interaction comparison of factor levels of one variable while the other was held constant and vice versa. Interaction of a categorical and a continuous factor were analyzed via pairwise comparison of factor levels as a function of the continuous covariate. Furthermore, steepness of the slope was analyzed within all factor levels.
We calculated a Pearson correlation to test for an association between boldness (latency body) and change in body mass during the experiment.
For data analyses we used the software R Version 3.5.1 (R Core Team, 2018). Models were built with the function lmer from the R package “lme4” (Version 1.1 – 17, Bates, 2010) and post hoc tests were conducted using the R package “phia” (Version 0.2 – 1; Martínez, 2015). The mean and standard deviation for each response variable analyzed are presented.
Ethical Note
Experiments were conducted under the permission of the Landesamt für Arbeitsschutz, Verbraucherschutz und Gesundheit, Brandenburg (LAVG 2347-27-2017) and the Stadtverwaltung der Landeshauptstadt Potsdam, Bereich Veterinärwesen und Lebensmittelüberwachung (AZ 386-1-). All applicable institutional and national guidelines for the care and use of animals were followed.
Grassland Enclosure Experiment
Study Subjects and Experimental Design
Bank voles were equipped with a passive integrated transponder tag (PIT, Trovan ID-100, 2.12 mm × 11.5 mm, 0.1 g) for individual identification and kept in standard makrolon cages on a standard rodent diet until the start of the experiment. The study was conducted from November 2013 to April 2014 in six large naturally vegetated grassland enclosures near Potsdam, Germany. Each enclosure was 0.25 ha (50 × 50 m) in size and was surrounded by a galvanized metal wall extending 1 m below and 0.5 m above ground. Voles were protected against terrestrial predators through an electrical veterinary fence surrounding the facility. Enclosures were open to avian predation.
Artificial light at night was created by using small solar powered garden lamps with single LEDs (Conrad Electronics, Hirschau, Germany, Model 572129, for spectral distribution see Eccard et al., 2018). Four enclosures were partially illuminated (half of each enclosure, Figure 2), while two enclosures served as controls where the whole enclosure was uniformly dark and illuminated, respectively. Lamps were 60 cm high and were above the grass layer in winter, but immersed in the grass layer later in spring. Lamps emitted a cold white light with a high proportion of blue light (color temperature = 7,250 K) through one diode and contained a diffuser to scatter the light. Diode and diffuser created a brighter zone surrounding the lamp (radius r = 25 cm, illuminance i = 0.8 lx) and a dimmer outer zone (r = 2.5 m, i < 0.1 lx; for details see Eccard et al., 2018).
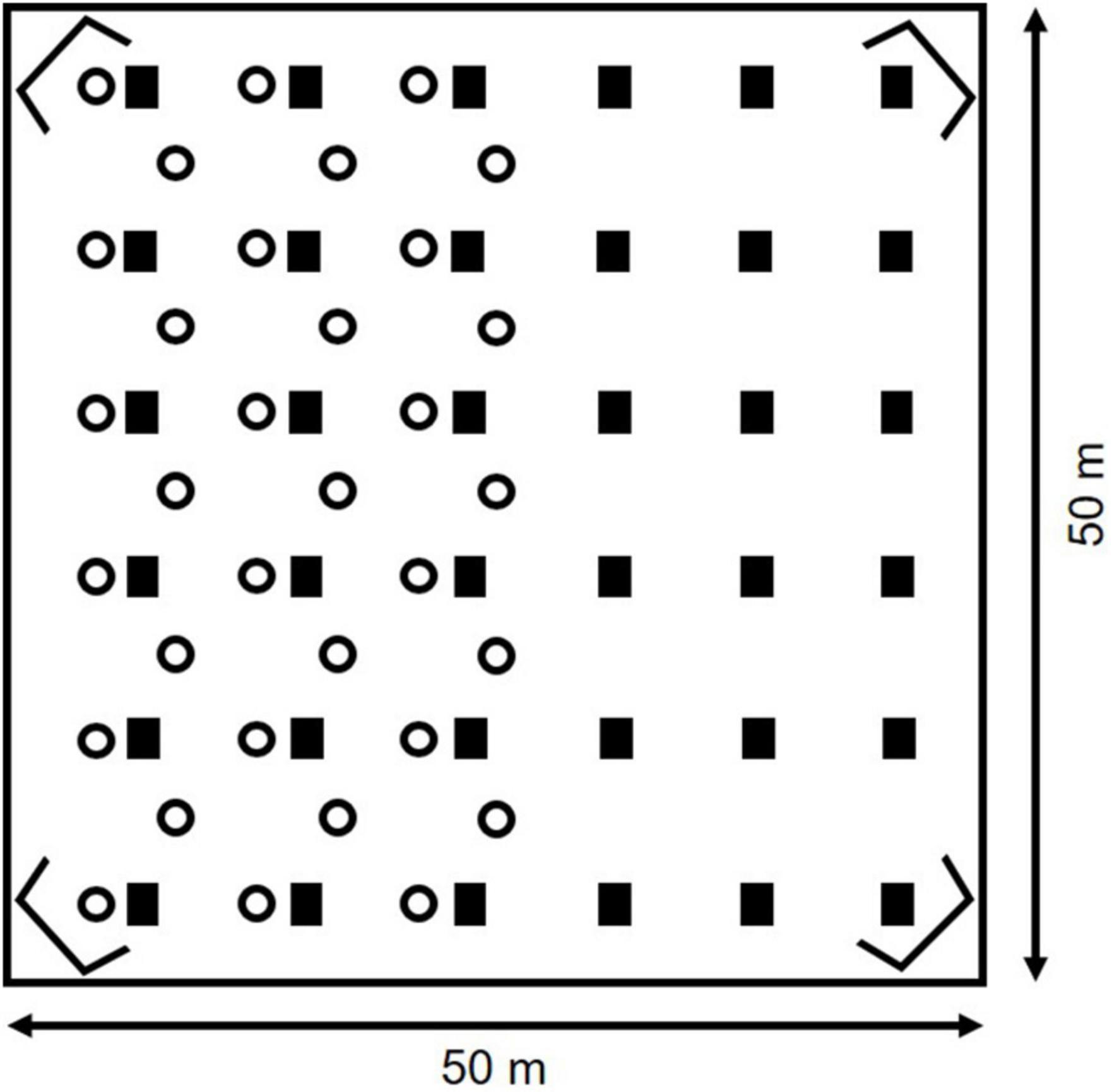
Figure 2. Naturally vegetated outdoor enclosure with partial nighttime illumination. Artificial light at night was created using small LED garden lamps (circles). Multicapture live traps were evenly distributed across each enclosure (black boxes). A 24 h radio telemetry was conducted during half moon and new moon using Yagi antennae in the corners of the enclosure connected to an automatic receiving unit.
Thirty-six bank voles were released into enclosures from November until December with three females and three males in each enclosure. Multicapture live traps (Ugglan special No 2, Grahnab, Sweden) were evenly distributed across each enclosure (N = 36, 6 × 6 grid). Traps were sheltered against wind and sun by metal boxes (30 × 20 × 20 cm) and a tile as cover. Animals were captured in January and February to obtain survival estimates.
Radio Telemetry
We conducted 24 h radio telemetry at the beginning of April during half moon and at the end of April during new moon. To conduct the telemetry with sufficient sample size, we transferred additional animals (N = 24, two females and two males per enclosure) into the enclosure in mid-March. For detailed information on the methods see Hoffmann et al. (2018). In short, each enclosure was equipped with an automated radio telemetry system, consisting of eight Yagi antennae (Winkler-Spezialantennen, Germany) connected to an automatic receiving unit (Sparrow System, United States). After a calibration using stationary transmitters, locations of each transmitter could be calculated via trigonometry.
Voles were live-trapped and fitted with radio telemetry transmitters (Holohil BD-2C, ∼1 g). In the half moon telemetry session 13 individuals were tracked (4 individuals in uniform enclosures, 9 individuals in partially illuminated enclosures) and in the new moon session 19 individuals were tracked (6 individuals in uniform enclosures, 13 individuals in partially illuminated enclosures). A location was calculated every 10 min resulting in 144 locations per animal in 24 h.
Statistical Analysis
All statistical analyses were performed with R version 4.0.3 (R Core Team, 2020) and for each analyzed variable we present the mean together with the standard deviation.
Side distribution of individuals within enclosures at day and night was analyzed using a Wilcoxon rank sum test. Separate tests were conducted for individuals tracked during half moon and new moon. Number of locations on the illuminated side divided by the total number of locations was compared to a theoretical value of 0.5 were no side preference or avoidance would be present. Additionally, side distribution of individuals within partially illuminated enclosures was compared to a random side distribution of individuals living in uniformly dark or illuminated enclosures at day and night using a Wilcoxon rank sum test. This was limited to new moon as under half moon only three control animals could be tracked.
Ethical Note
The experiment was conducted under the permission of the “Landesamt für Umwelt, Gesundheit und Verbraucherschutz” (LUGV; reference number V3-2347-44-2011) investigating effects of animal personality on risk taking (here: ALAN). Animals were housed under the permission and control of the LUGV (reference number 3854-1-132). All applicable institutional and national guidelines for the care and use of animals were followed.
Results
Laboratory Experiment
Time Spent in Seed Trays
On average, voles spent 84.71 ± 125.74 min (NIndividuals = 21) in all four seed trays combined during the treatment night and the following day. The interaction of latency body and daytime was significant (χ2 = 15.14, P < 0.001, N = 84, Table 2). During the day, shy animals (longer latencies to emerge) spent less time in trays than bold animals, but during the night we detected no difference (Figure 3 and Table 3). Initial body mass had no effect on time spent in trays (Table 1).
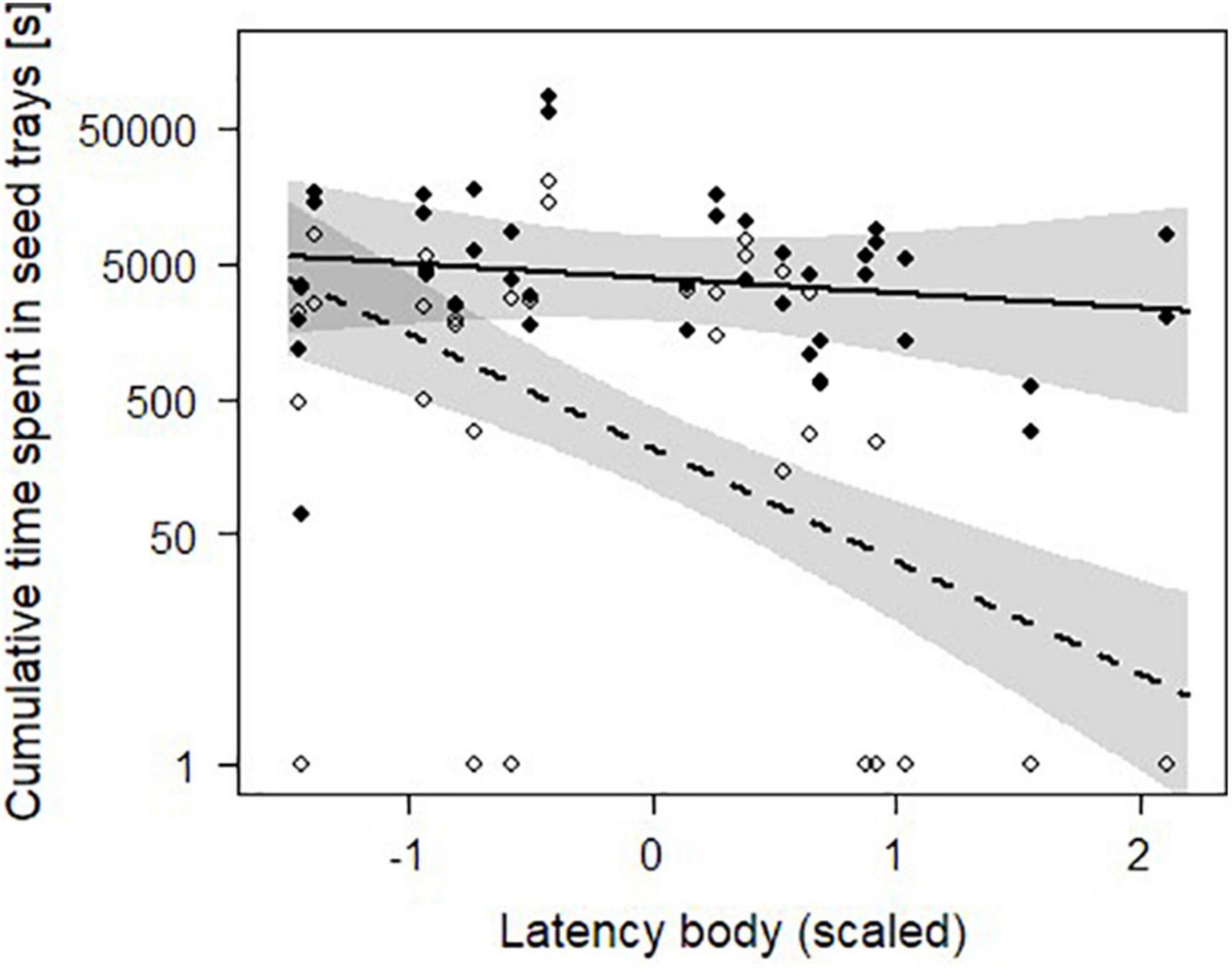
Figure 3. Influence of daytime and latency body (scaled) on the cumulative time animals spent in seed trays on each side of the experimental arena of 21 bank voles. Black dots and the solid line show the raw nighttime data and the prediction line from the linear mixed effects model, respectively. White dots and the dashed line show the raw daytime data and the prediction line from the model. Gray areas represent 95%-confidence intervals. The y-axis is log scaled. The higher the latency body, the shyer is the animal.
Number of Visits to Seed Trays
Animals conducted 50.3 ± 56.3 visits to all seed trays combined (NIndividuals = 21). Animals visited seed trays more often during night than during day at the dark (night: 21.5 ± 25.0, N = 21; day: 6.5 ± 9.3, N = 21) and the illuminated side (night: 14.5 ± 16.0, N = 21; day: 7.9 ± 10.5, N = 21; Tables 2, 3 and Figure 4A). At night, voles tended to visit the seed trays at the dark side of the arena more often (21.5 ± 25.0, N = 21) than those on the illuminated side (14.5 ± 16.0, N = 21) while during the day voles tended to visit those trays more often on the side of the arena that had been illuminated at night (7.9 ± 10.5, N = 21) compared to those on the formerly dark side (6.5 ± 9.3, N = 21; Table 3 and Figure 4A). While latency body did not explain the number of visits to the seed trays at night, bold animals (short latency) visited trays more often than shy animals (long latency) during the following day (χ2 = 13.49, P < 0.001, N = 84; Table 3 and Figure 4B). Initial body mass had no effect on the number of visits to seed trays (Table 1).
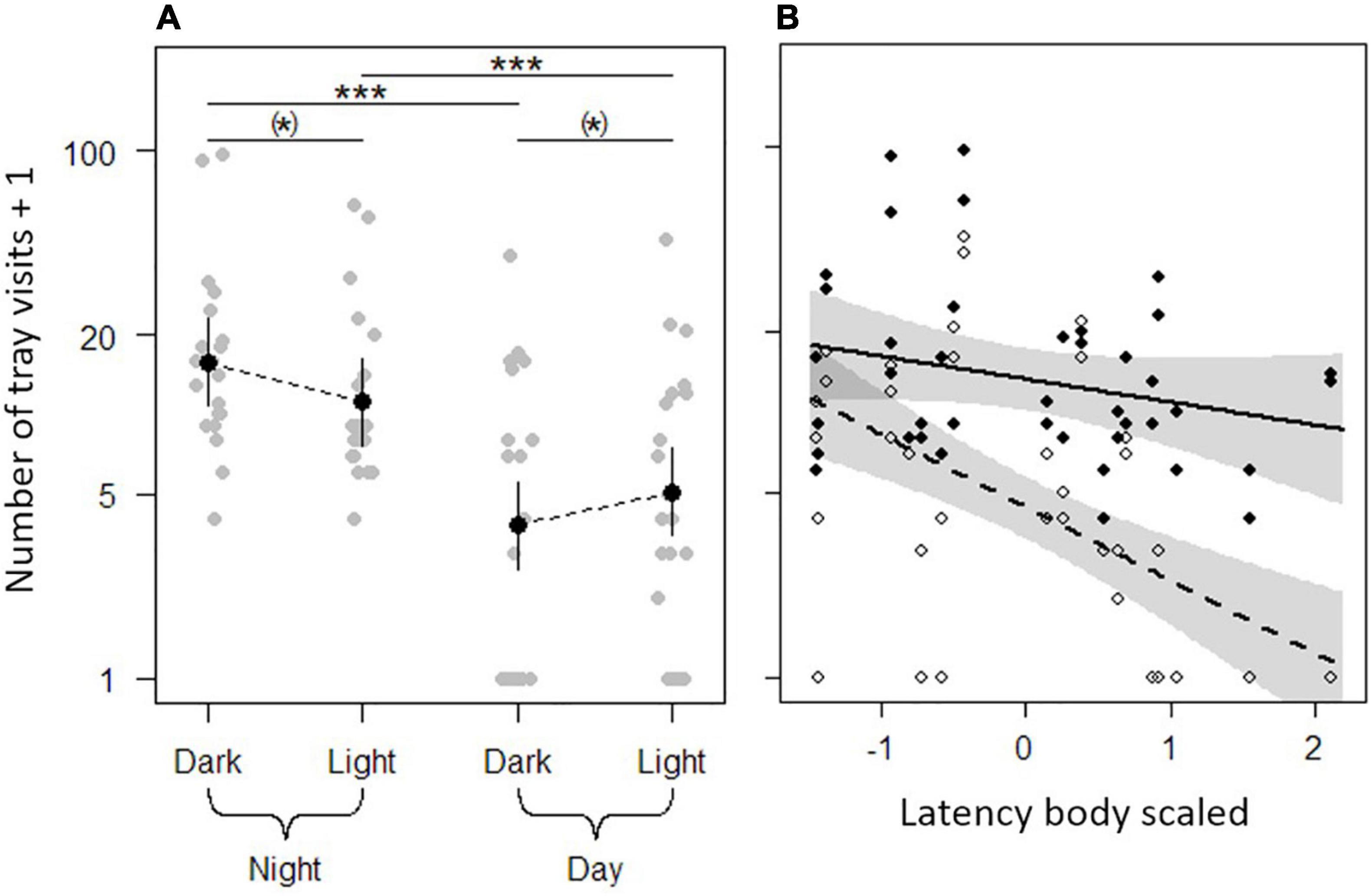
Figure 4. Influence of (A) light treatment and daytime and (B) daytime and latency body (scaled) on the number of visits to seed trays on each side of the experimental arena of 21 bank voles. (A) Gray dots show the underlying raw data and black dots show the predicted means of the linear mixed effects model. Solid lines represent confidence intervals. *P < 0.1, ***P < 0.001. (B) Black dots and the solid line show the raw nighttime data and the prediction line from the linear mixed effects model, respectively. White dots and the dashed line show the raw daytime data and the prediction line from the model. Gray areas represent 95%-confidence intervals. The higher the latency body, the shyer is the animal. The y-axes are log scaled.
Giving-Up Density
Averaged over all seed trays, giving-up density (GUD) was 0.33 ± 0.21 g of millet per 450 ml of sand (N = 168). GUD was influenced by an interaction of treatment, latency body and daytime but not by the initial body mass of the animal (Tables 1, 2). The influence of latency body on GUD did not differ between day and night at the dark side of the arena while at the illuminated side the effect of latency body was stronger at day than at night (Table 3 and Figure 5). Bold individuals (short latency) exploited trays to lower GUDs than shy individuals (long latency) but this difference was greater during the day at the formerly illuminated side of the arena compared to the formerly dark side (Table 3 and Figure 5).
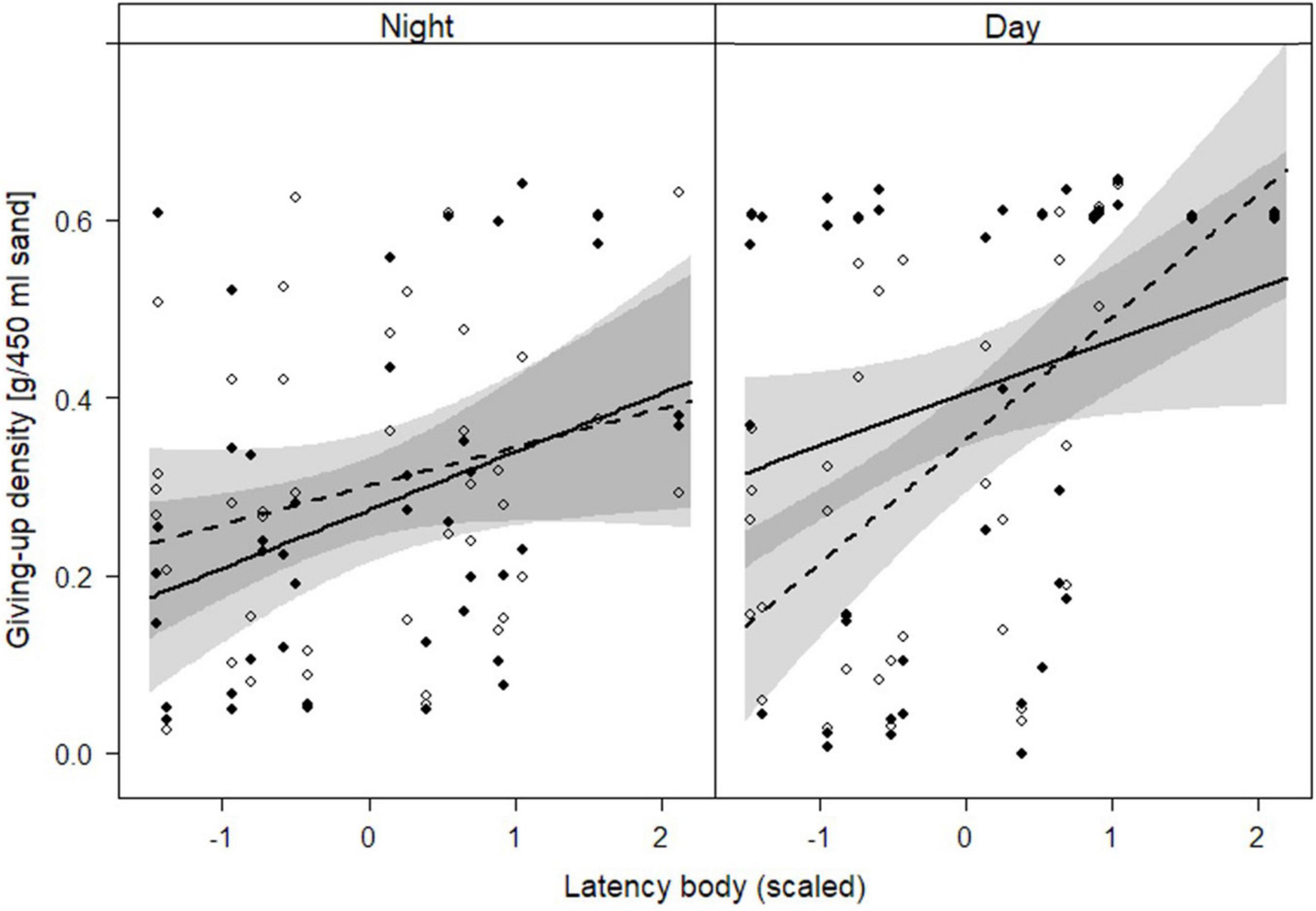
Figure 5. Influence of light treatment, daytime and latency body (scaled) on giving-up density of seed trays. Both sides of the experimental arena contained two seed trays filled with millet and sand. Black dots and the solid line show raw data from the dark side of the arena and the prediction line from the linear mixed effects model, respectively. White dots and the dashed show raw data from the illuminated side of the arena and the prediction line from the model. Gray areas represent 95%-confidence intervals. The higher the latency body, the shyer is the animal.
Exploitation Efficiency
To investigate differences in exploitation efficiency, we tried to fit population-level harvesting curves to total seeds taken out of the seed trays depending on the cumulative time animals spend in the tray for dark and illuminated trays at night and the subsequent day. However, we were not able to fit functions that explained enough variance (Supplementary Material 3).
Body Mass Change
Animals experienced a change in body mass of −1.62 ± 1.37 g over the course of the experiment. Body mass change did not differ depending on boldness (latency to emerge; t = −1.36, df = 19, P = 0.191).
Grassland Enclosure Experiment
Side Distribution
On average, 61.2 ± 36.6% (N = 44) of locations of voles living in partially illuminated enclosures were within the illuminated part of the enclosure. Rates did not differ from expected 50% during the day and during the night at half moon (Uday = 29, P = 0.496; Unight = 20, P = 0.812; N = 9) and new moon (Uday = 61, P = 0.293; Unight = 68, P = 0.122; N = 13). Additionally, rates did not differ at new moon between animals living in partially illuminated enclosures and animals living under uniformly dark or illumination conditions during day (Uday = 27, P = 0.313; NPartial = 13, NUniform = 6) and night (Uday = 35, P = 0.757; NPartial = 13, NUniform = 6).
Discussion
We found that light treatment and individual boldness influenced the behavioral variables measured in the laboratory experiment. Animals tended to visit dark seed trays more often than illuminated trays during the night and tended to visit previously illuminated seed trays more often than previously dark trays during the day. Overall, boldness influenced foraging behavior more during day than during night with bold individuals spending more time in seed trays, visiting seed trays more often and having lower GUDs than shy individuals. While GUD was similar between dark and illuminated seed trays within individuals of varying boldness during the night, bold individuals had lower GUDs in previously illuminated trays than in dark trays during the day. In contrast, animals did not show avoidance or preference of the illuminated side in the naturally vegetated grassland enclosures.
While moonlight and skyglow (the reflection and scattering of ALAN by molecules or aerosols in the atmosphere) elicit a dim and spatially relatively homogeneous nighttime surface illumination (Kyba and Hölker, 2013), direct light sources often create a spatially heterogeneous “nightscape” with higher light intensities (Kuechly et al., 2012; Hale et al., 2013). We can show that small mammals adjust their foraging behavior to the artificial light conditions at the foraging side. Our findings of a reduced number of tray visits at illuminated trays at night are in accordance with Bird et al. (2004) who found that Beach mice decreased the number of patches they foraged in with increasing proximity to artificial light sources. On the other hand, time spent in trays and GUDs between dark and illuminated trays were similar at night. Animals might have reduced their movement between seed trays in the area subjected to ALAN to decrease visibility for predators. Nevertheless, they have to fulfill their energetic demands through foraging and might not be able to reduce the time they spent at the foraging site any further.
Shy individuals avoided foraging during the day more than bold individuals, leading to a reduced time spent in seed trays and number of tray visits. The arena provided no shelter except the nest box so that bank voles, which are common prey for a large variety of ground and aerial predators (Halle, 1993), might have experienced an increased perceived predation risk with increasing illumination. As the highest illumination level was present during the day, shy individuals who are more risk-averse might have reduced their diurnal foraging activity to a minimum while bold individuals did not reduce the time they spent in seed trays and the number of visits to trays. Thus, animals with varying personalities may differ in their perception of temporal risk distribution and in their risk allocation (Lima and Bednekoff, 1999). Alternatively, bold individuals might have to maximize foraging as they are shown to have a higher metabolism than shy individuals (Binder et al., 2016; von Merten et al., 2020). In that case, they are forced to show higher foraging activity even though perceived predation risk is high to fulfill their energetic needs.
Additionally, we can show a carry-over effect from the nighttime illumination to the foraging behavior shown at day as animals tended to visit seed trays more that were illuminated at night than those that previously were dark. This way, they could try to compensate for reduced nocturnal foraging. However, GUDs during the day differed depending on light treatment and boldness of the individual. With increasing boldness, the GUDs in the previously illuminated seed trays strongly decreased, suggesting that bold individuals try to maximize food consumption to compensate for missed foraging opportunities at night while shy individuals spent even more time on vigilance behavior. This might be a result of a so called “memory window” (Hughes et al., 1992). A foragers’ behavior is influenced by its recent experiences in that environment. Perhaps shy individuals reduce their foraging at previously illuminated seed trays during the day as they remember the increased perceived predation risk during night and might attach more importance to that memory than bold individuals.
Depending on the behavioral changes caused by ALAN in predators of small mammals, the different responses of bold and shy individuals to spatially heterogeneous nighttime illumination can lead to a selection of a certain behavioral type and therefore to a loss of behavioral variation. If predators are avoiding illuminated sites, bold individuals should be at an advantage as they face no increased predation risk while having a higher access to food than shy individuals. On the other hand, studies show that aerial predators such as owls are able to increase hunting efficiency under increased nighttime illumination (Clarke, 1983). In that case, shy individuals might be at an advantage as their risk of being predated upon is lower than for bold individuals as they avoid illuminated habitat patches. Either way, ALAN has the potential to dramatically shift species interactions by a change in behavior of important prey species and thus can affect several trophic levels.
In contrast to the laboratory, we could not find an influence of partial nighttime illumination on the distribution of animals within grassland enclosures. In a previous study, we had found that animals increased their activity in illuminated enclosures compared to dark enclosures (Hoffmann et al., 2019), however, offering partial illumination in this study, we could not find a preference or avoidance for the illuminated part. A possible explanation for this could be that animals were able to avoid dim nighttime illumination by using vegetation cover and burrows. Furthermore, the light emitted by the solar garden lamps was more than ten times lower than the light used in the laboratory experiment. It resulted in surface illumination levels 2–3 times brighter than the maximum full moon illuminance on Earth (hypothetical value: 0.3 lx) down to a typical summer full moon in Berlin/Brandenburg (∼ 0.1 lx, Jechow et al., 2020) in only small parts of the enclosure while in most areas artificial illumination levels were below that level. The presence of high vegetation in April might have been sufficient to diminish the effect of increased predation risk sufficiently so that animals did not need to adjust their space use behavior. However, it may be interesting to investigate in future studies whether ALAN affects small-scale behavioral traits, such as time spent foraging above-ground, time spent hiding in burrows and burrowing activity or time spend foraging directly under lamps.
This study shows that partial nighttime illumination can affect night- and daytime foraging behavior of small mammals and provides further insights into how animal personality is related to behavioral changes caused by ALAN. While we can show that ALAN has carry-over effects regarding movement and foraging behavior into the daytime, it remains unclear how these affect interspecific interactions with diurnal species on the same and higher trophic levels. Thus, future studies on the effects of ALAN should extent their view from the night into the day to be able to better estimate the consequences of increasing nighttime illumination for ecosystems.
Data Availability Statement
The raw data supporting the conclusions of this article will be made available by the authors, without undue reservation.
Ethics Statement
The animal study was reviewed and approved by the Landesamt für (Arbeitsschutz), Verbraucherschutz und Gesundheit, Brandenburg (reference numbers: LAVG 2347-27-2017 and LUGV V3-2347-44-2011). Keeping and breeding of animals was permitted by the Stadtverwaltung der Landeshauptstadt Potsdam, Bereich Veterinärwesen und Lebensmittelüberwachung (AZ 386-1).
Author Contributions
JH designed the laboratory experiment. JE and JH designed the field experiment. JH secured the funding, supervised the collection of data, and analyzed the data with feedback from JE and FH. JH wrote the draft of the manuscript, which was further edited by FH and JE. All authors contributed to the article and approved the submitted version.
Funding
The Deutsche Bundesstiftung Umwelt (DBU) funded JHs studies on effects of light pollution (reference number 20015/374).
Conflict of Interest
The authors declare that the research was conducted in the absence of any commercial or financial relationships that could be construed as a potential conflict of interest.
Publisher’s Note
All claims expressed in this article are solely those of the authors and do not necessarily represent those of their affiliated organizations, or those of the publisher, the editors and the reviewers. Any product that may be evaluated in this article, or claim that may be made by its manufacturer, is not guaranteed or endorsed by the publisher.
Acknowledgments
We thank Andreas Rauch for his contribution during the laboratory experiment. We also thank Julia Köchling and Anne Gärtner for conducting field work and Elke Seydewitz for the analysis of telemetry data.
Supplementary Material
The Supplementary Material for this article can be found online at: https://www.frontiersin.org/articles/10.3389/fevo.2021.779825/full#supplementary-material
References
Baker, B. J., and Richardson, J. M. L. (2006). The effect of artificial light on male breeding-season behaviour in green frogs, Rana clamitans melanota. Can. J. Zool. 84, 1528–1532. doi: 10.1139/z06-142
Ballew, N. G., Mittelbach, G. G., Scribner, K. T., van Doorn, G. S., and Winn, A. A. (2017). Fitness consequences of boldness in juvenile and adult largemouth bass. Am. Nat. 189, 396–406. doi: 10.1086/690909
Bates, D. M. (2010). lme4: Mixed-Effects Modeling with R. Available online at: http://lme4.r-forge.r-project.org/book (accessed March 3, 2016).
Binder, T. R., Wilson, A. D. M., Wilson, S. M., Suski, C. D., Godin, J.-G. J., and Cooke, S. J. (2016). Is there a pace-of-life syndrome linking boldness and metabolic capacity for locomotion in bluegill sunfish? Anim. Behav. 121, 175–183. doi: 10.1016/j.anbehav.2016.09.006
Bird, B. L., Branch, L. C., and Miller, D. L. (2004). Effects of coastal lighting on foraging behavior of beach mice. Conserv. Biol. 18, 1435–1439. doi: 10.1111/j.1523-1739.2004.00349.x
Bowers, M. A. (1988). Seed removal experiments on desert rodents: the microhabitat by moonlight effect. J. Mammal. 69, 201–204. doi: 10.2307/1381778
Canário, F., Hespanhol Leitão, A., and Tomé, R. (2012). Predation attempts by short-eared and long-eared owls on migrating songbirds attracted to artificial lights. J. Raptor Res. 46, 232–234. doi: 10.3356/JRR-11-15.1
Clarke, J. A. (1983). Moonlight’s influence on predator/prey interactions between short-eared owls (Asio flammeus) and deermice (Peromyscus maniculatus). Behav. Ecol. Sociobiol. 13, 205–209. doi: 10.1007/BF00299924
Curtis, D. J., and Rasmussen, M. A. (2006). The evolution of Cathemerality in primates and other mammals: a comparative and chronoecological approach. Folia Primatol. (Basel) 77, 178–193. doi: 10.1159/000089703
Daly, M., Behrends, P. R., Wilson, M. I., and Jacobs, L. F. (1992). Behavioural modulation of predation risk: moonlight avoidance and crepuscular compensation in a nocturnal desert rodent, Dipodomys merriami. Anim. Behav. 44, 1–9. doi: 10.1016/S0003-3472(05)80748-1
Davies, T. W., Bennie, J., Cruse, D., Blumgart, D., Inger, R., and Gaston, K. J. (2017). Multiple night-time light-emitting diode lighting strategies impact grassland invertebrate assemblages. Global Change Biol. 23, 2641–2648. doi: 10.1111/gcb.13615
de Jong, M., Caro, S. P., Gienapp, P., Spoelstra, K., and Visser, M. E. (2017). Early birds by light at night: effects of light color and intensity on daily activity patterns in blue tits. J. Biol. Rhythms 32, 323–333. doi: 10.1177/0748730417719168
Donatello, S., Rodríguez, R., Quintero, M. G. C., Jrc, O. W., Van Tichelen, P., Van, V., et al. (2019). Revision of the EU Green Public Procurement Criteria for Street Lighting and Traffic Signals: Technical Report and Criteria Proposal. Luxembourg: Publications Office of the European Union.
Eccard, J. A., Scheffler, I., Franke, S., and Hoffmann, J. (2018). Off-grid: solar powered LED illumination impacts epigeal arthropods. Insect Conserv. Div. 11, 600–607. doi: 10.1111/icad.12303
Falchi, F., Cinzano, P., Duriscoe, D., Kyba, C. C. M., Elvidge, C. D., Baugh, K., et al. (2016). The new world atlas of artificial night sky brightness. Sci. Adv. 2:e1600377. doi: 10.1126/sciadv.1600377
Gaston, K. J., Bennie, J., Davies, T. W., and Hopkins, J. (2013). The ecological impacts of nighttime light pollution: a mechanistic appraisal: nighttime light pollution. Biol. Rev. 88, 912–927. doi: 10.1111/brv.12036
Gosling, S. D. (2001). From mice to men: what can we learn about personality from animal research? Psychol. Bull. 127, 45–86. doi: 10.1037/0033-2909.127.1.45
Gutman, R., Dayan, T., Levy, O., Schubert, I., and Kronfeld-Schor, N. (2011). The effect of the lunar cycle on fecal cortisol metabolite levels and foraging ecology of nocturnally and diurnally active spiny mice. PLoS One 6:e23446. doi: 10.1371/journal.pone.0023446
Hale, J. D., Davies, G., Fairbrass, A. J., Matthews, T. J., Rogers, C. D. F., and Sadler, J. P. (2013). Mapping lightscapes: spatial patterning of artificial lighting in an urban landscape. PLoS One 8:e61460. doi: 10.1371/journal.pone.0061460
Halle, S. (1993). Diel pattern of predation risk in microtine rodents. Oikos 68:510. doi: 10.2307/3544919
Halle, S. (2006). Polyphasic activity patterns in small mammals. Folia Primatol. 77, 15–26. doi: 10.1159/000089693
Hansson, L., and Larsson, T.-B. (1978). Vole diet on experimentally managed reforestation areas in northern Sweden. Ecography 1, 16–26. doi: 10.1111/j.1600-0587.1978.tb00934.x
Herde, A., and Eccard, J. A. (2013). Consistency in boldness, activity and exploration at different stages of life. BMC Ecol. 13:49. doi: 10.1186/1472-6785-13-49
Herring, H., and Roy, R. (2007). Technological innovation, energy efficient design and the rebound effect. Technovation 27, 194–203. doi: 10.1016/j.technovation.2006.11.004
Hoffmann, J., Palme, R., and Eccard, J. A. (2018). Long-term dim light during nighttime changes activity patterns and space use in experimental small mammal populations. Environ. Poll. 238, 844–851. doi: 10.1016/j.envpol.2018.03.107
Hoffmann, J., Rüsen, L., Schirmer, A., and Eccard, J. A. (2020). A Little is too Much: Already Low Levels of Artificial Light at Night Influence the Behavior of Small Mammals. Animal Ecology. Potsdam: University of Potsdam.
Hoffmann, J., Schirmer, A., and Eccard, J. A. (2019). Light pollution affects space use and interaction of two small mammal species irrespective of personality. BMC Ecol. 19:26. doi: 10.1186/s12898-019-0241-0
Hölker, F., Moss, T., Griefahn, B., Kloas, W., Voigt, C. C., Henckel, D., et al. (2010a). The dark side of light: a transdisciplinary research agenda for light pollution policy. Ecol. Soc. 15:13. doi: 10.5751/ES-03685-150413
Hölker, F., Wolter, C., Perkin, E. K., and Tockner, K. (2010b). Light pollution as a biodiversity threat. Trends Ecol. Evol. 25, 681–682.
Hughes, R. N., Kaiser, M. J., Mackney, P. A., and Warburton, K. (1992). Optimizing foraging behaviour through learning. J. Fish Biol. 41, 77–91. doi: 10.1111/j.1095-8649.1992.tb03870.x
Jacob, J., and Brown, J. S. (2000). Microhabitat use, giving-up densities and temporal activity as short- and long-term anti-predator behaviors in common voles. Oikos 91, 131–138. doi: 10.1034/j.1600-0706.2000.910112.x
Jechow, A., Kyba, C. C. M., and Hölker, F. (2020). Mapping the brightness and color of urban to rural skyglow with all-sky photometry. J. Quant. Spectrosc. Radiat. Transfer 250:106988. doi: 10.1016/j.jqsrt.2020.106988
Kashon, E. A. F., and Carlson, B. E. (2018). Consistently bolder turtles maintain higher body temperatures in the field but may experience greater predation risk. Behav. Ecol. Sociobiol. 72:9. doi: 10.1007/s00265-017-2428-8
Korpela, K., Sundell, J., and Ylönen, H. (2011). Does personality in small rodents vary depending on population density? Oecologia 165, 67–77. doi: 10.1007/s00442-010-1810-2
Kotler, B. P., Brown, J., Mukherjee, S., Berger-Tal, O., and Bouskila, A. (2010). Moonlight avoidance in gerbils reveals a sophisticated interplay among time allocation, vigilance and state-dependent foraging. Proc. R. Soc. Lond. B Biol. Sci. 277, 1469–1474. doi: 10.1098/rspb.2009.2036
Kronfeld-Schor, N., Dominoni, D., de la Iglesia, H., Levy, O., Herzog, E. D., Dayan, T., et al. (2013). Chronobiology by moonlight. Proc. R. Soc. Lond. B Biol. Sci. 280:20123088. doi: 10.1098/rspb.2012.3088
Kuechly, H. U., Kyba, C. C. M., Ruhtz, T., Lindemann, C., Wolter, C., Fischer, J., et al. (2012). Aerial survey and spatial analysis of sources of light pollution in Berlin, Germany. Remote Sens. Environ. 126, 39–50. doi: 10.1016/j.rse.2012.08.008
Kurvers, R. H. J. M., and Hölker, F. (2015). Bright nights and social interactions: a neglected issue. Behav. Ecol. 26, 334–339. doi: 10.1093/beheco/aru223
Kurvers, R. H. J. M., Drägestein, J., Hölker, F., Jechow, A., Krause, J., and Bierbach, D. (2018). Artificial Light at night affects emergence from a refuge and space use in guppies. Sci. Rep. 8:14131. doi: 10.1038/s41598-018-32466-3
Kyba, C. C. M., and Hölker, F. (2013). Do artificially illuminated skies affect biodiversity in nocturnal landscapes? Landsc. Ecol. 28, 1637–1640. doi: 10.1007/s10980-013-9936-3
Kyba, C. C., Hänel, A., and Hölker, F. (2014). Redefining efficiency for outdoor lighting. Energy Environ. Sci. 7, 1806–1809. doi: 10.1039/C4EE00566J
Kyba, C. C., Kuester, T., de Miguel, A. S., Baugh, K., Jechow, A., Hölker, F., et al. (2017). Artificially lit surface of Earth at night increasing in radiance and extent. Sci. Adv. 3:e1701528.
Laforge, A., Pauwels, J., Faure, B., Bas, Y., Kerbiriou, C., Fonderflick, J., et al. (2019). Reducing light pollution improves connectivity for bats in urban landscapes. Landsc. Ecol. 34, 793–809. doi: 10.1007/s10980-019-00803-0
Lima, S. L., and Bednekoff, P. A. (1999). Temporal variation in danger drives antipredator behavior: the predation risk allocation hypothesis. Am. Nat. 153, 649–659. doi: 10.1086/303202
Lima, S. L., and Dill, L. M. (1990). Behavioral decisions made under the risk of predation: a review and prospectus. Can. J. Zool. 68, 619–640. doi: 10.1139/z90-092
Martínez, H. R. (2015). Analysing Interactions of Fitted Models. Available online at: https://cran.r-project.org/web/packages/phia/vignettes/phia.pdf (accessed November 7, 2015).
Mazza, V., Eccard, J. A., Zaccaroni, M., Jacob, J., and Dammhahn, M. (2018). The fast and the flexible: cognitive style drives individual variation in cognition in a small mammal. Anim. Behav. 137, 119–132. doi: 10.1016/j.anbehav.2018.01.011
Merrick, M. J., and Koprowski, J. L. (2017). Should we consider individual behavior differences in applied wildlife conservation studies? Biol. Conserv. 209, 34–44. doi: 10.1016/j.biocon.2017.01.021
Nakagawa, S., and Schielzeth, H. (2013). A general and simple method for obtaining R 2 from generalized linear mixed-effects models. Methods Ecol. Evol. 4, 133–142. doi: 10.1111/j.2041-210x.2012.00261.x
Negro, J. J., Bustamante, J., Melguizo, C., Ruiz, J. L., and Grande, J. M. (2000). Nocturnal activity of Lesser Kestrels under artificial lighting conditions in Seville, Spain. J. Raptor Res. 34, 327–329.
Norrdahl, K., and Korpimäki, E. (1995). Mortality factors in a cyclic vole population. Proc. R. Soc. Lond. B 261, 49–53. doi: 10.1098/rspb.1995.0116
Perea, R., González, R., San Miguel, A., and Gil, L. (2011). Moonlight and shelter cause differential seed selection and removal by rodents. Anim. Behav. 82, 717–723. doi: 10.1016/j.anbehav.2011.07.001
R Core Team (2018). R: A Language and Environment for Statistical Computing. Vienna: R Foundation for Statistical Computing.
R Core Team (2020). R: A Language and Environment for Statistical Computing. Vienna: R Foundation for Statistical Computing.
Réale, D., Gallant, B. Y., Leblanc, M., and Festa-Bianchet, M. (2000). Consistency of temperament in bighorn ewes and correlates with behaviour and life history. Anim. Behav. 60, 589–597. doi: 10.1006/anbe.2000.1530
Russ, A., Luèenièová, T., and Klenke, R. (2017). Altered breeding biology of the European blackbird under artificial light at night. J. Avian Biol. 48, 1114–1125. doi: 10.1111/jav.01210
Schirmer, A., Herde, A., Eccard, J. A., and Dammhahn, M. (2019). Individuals in space: personality-dependent space use, movement and microhabitat use facilitate individual spatial niche specialization. Oecologia 189, 647–660. doi: 10.1007/s00442-019-04365-5
Šíchová, K., Koskela, E., Mappes, T., Lantová, P., and Boratyński, Z. (2014). On personality, energy metabolism and mtDNA introgression in bank voles. Anim. Behav. 92, 229–237. doi: 10.1016/j.anbehav.2014.04.011
Sih, A. (1980). Optimal behavior: can foragers balance two conflicting demands? Science 210, 1041–1043. doi: 10.1126/science.210.4473.1041
Smith, B. R., and Blumstein, D. T. (2008). Fitness consequences of personality: a meta-analysis. Behav. Ecol. 19, 448–455. doi: 10.1093/beheco/arm144
Stone, E. L., Harris, S., and Jones, G. (2015). Impacts of artificial lighting on bats: a review of challenges and solutions. Mammal. Biol. 80, 213–219. doi: 10.1016/j.mambio.2015.02.004
van Grunsven, R. H. A., Jähnichen, D., Grubisic, M., and Hölker, F. (2018). Slugs (Arionidae) benefit from nocturnal artificial illumination. J. Exp. Zool. A 329, 429–433. doi: 10.1002/jez.2170
von Merten, S., Dingemanse, N. J., Mathias, M., da, L., and Rychlik, L. (2020). Individual behavior, behavioral stability, and pace of life within and among five shrew species. Behav. Ecol. Sociobiol. 74:15. doi: 10.1007/s00265-019-2793-6
Willmott, N. J., Henneken, J., Elgar, M. A., and Jones, T. M. (2019). Guiding lights: foraging responses of juvenile nocturnal orb-web spiders to the presence of artificial light at night. Ethology 125, 289–297. doi: 10.1111/eth.12852
Keywords: light pollution, inter-individual differences, animal personality, Myodes glareolus, ALAN
Citation: Hoffmann J, Hölker F and Eccard JA (2022) Welcome to the Dark Side: Partial Nighttime Illumination Affects Night-and Daytime Foraging Behavior of a Small Mammal. Front. Ecol. Evol. 9:779825. doi: 10.3389/fevo.2021.779825
Received: 19 September 2021; Accepted: 29 November 2021;
Published: 04 January 2022.
Edited by:
Jennifer N. Phillips, Texas A&M University-San Antonio, United StatesReviewed by:
Kylie A. Robert, La Trobe University, AustraliaCaroline Habold, UMR7178 Institut Pluridisciplinaire Hubert Curien (IPHC), France
Copyright © 2022 Hoffmann, Hölker and Eccard. This is an open-access article distributed under the terms of the Creative Commons Attribution License (CC BY). The use, distribution or reproduction in other forums is permitted, provided the original author(s) and the copyright owner(s) are credited and that the original publication in this journal is cited, in accordance with accepted academic practice. No use, distribution or reproduction is permitted which does not comply with these terms.
*Correspondence: Jana A. Eccard, eccard@uni-potsdam.de