- Instituto Valenciano de Investigaciones Agrarias (IVIA), Centro de Protección Vegetal y Biotecnología, Moncada, Spain
Insect herbivory activates plant defense mechanisms and releases a blend of herbivore-induced plant volatiles (HIPVs). These volatile compounds may be involved in plant-plant communication and induce defense response in undamaged plants. In this work, we investigated whether the exposure of sweet pepper plants to HIPVs [(Z)-3-hexenol, (Z)-3-hexenyl acetate, (Z)-3-hexenyl propanoate, (Z)-3-hexenyl butanoate, hexyl butanoate, methyl salicylate and methyl jasmonate] activates the sweet pepper immune defense system. For this, healthy sweet pepper plants were individually exposed to the each of the above mentioned HIPVs over 48 h. The expression of jasmonic acid and salicylic acid related genes was quantified. Here, we show that all the tested volatiles induced plant defenses by upregulating the jasmonic acid and salicylic acid signaling pathway. Additionally, the response of Frankliniella occidentalis, a key sweet pepper pest, and Orius laevigatus, the main natural enemy of F. occidentalis, to HIPV-exposed sweet pepper plants were studied in a Y-tube olfactometer. Only plants exposed to (Z)-3-hexenyl propanoate and methyl salicylate repelled F. occidentalis whereas O. laevigatus showed a strong preference to plants exposed to (Z)-3-hexenol, (Z)-3-hexenyl propanoate, (Z)-3-hexenyl butanoate, methyl salicylate and methyl jasmonate. Our results show that HIPVs act as elicitors to sweet pepper plant defenses by enhancing defensive signaling pathways. We anticipate our results to be a starting point for integrating HIPVs-based approaches in sweet pepper pest management systems which may provide a sustainable strategy to manage insect pests in horticultural plants.
Introduction
Plants can communicate to each other by means of volatile organic compounds (VOCs) (Baldwin et al., 2002; Heil and Karban, 2010). Those chemical blends are intercepted as warning signal by undamaged plants which neighbor attacked plants. Thus, the undamaged plants’ defenses are triggered in advance and the herbivore damage is potentially minimized (Kost and Heil, 2006; Frost et al., 2008a). VOCs are also involved in many multitrophic interactions including intra-plant signaling, flower-pollinators interaction and herbivore’s natural enemies’ attraction (Kessler et al., 2006; Heil, 2008; War et al., 2011).
When attacked by herbivores, plants activate their mechanisms of defense which involve different transduction pathways regulated by phytohormones, such as salicylic acid (SA), jasmonic acid (JA), abscisic acid (ABA), and ethylene (ET) (Bari and Jones, 2009; Dicke and van Loon, 2014). Herbivore feeding activity stimulates the release of herbivore-induced plant volatiles (HIPVs) (War et al., 2011) which indirectly influences herbivores by attracting their predators and/or parasitoids (Heil, 2008). The HIPVs blend is dominated by esters, terpenes, aldehydes, alcohols and aromatic compounds (Arimura et al., 2009).
In the case of sweet pepper plants, the phytophagous behavior of two of the most commonly used biological control agents, the zoophytophagous mirids Nesidiocoris tenuis (Reuter) and Macrolophus pygmaeus (Rambur) (Hemiptera: Miridae), have been demonstrated to induce plant defenses and enhance the emission of HIPVs which repel two arthropod key pests for this crop, Frankliniella occidentalis (Pergande) (Thysanoptera: Thripidae) and Bemisia tabaci (Gennadius) (Hemiptera: Aleyrodidae). Furthermore, these HIPVs attract the whitefly parasitoid Encarsia formosa (Gahan) (Hymenoptera: Aphelinidae) (Bouagga et al., 2018). Bouagga et al. (2017) also demonstrated that the zoophytophagous minute pirate bug Orius laevigatus (Fieber) (Hemiptera: Anthocoridae) feeding on sweet pepper plants induced the plant’s response, stimulating the release of HIPVs and activating the defensive transduction pathways regulated by jasmonic acid (JA) and salicylic acid (SA) phytohormones. This array of HIPVs, which principally belong to terpenoid and fatty acid derived green leaf volatile (GLVs) classes, were responsible for repelling F. occidentalis and B. tabaci.
Taking advantage of HIPVs in plant-plant communication, Pérez-Hedo et al. (2021a) studied the effect of separate exposure of tomato plants to seven mirid-induced volatiles [1-hexanol, (Z)-3-hexenol, (Z)-3-hexenyl acetate, (Z)-3-hexenyl propanoate, (Z)-3-hexenyl butanoate, hexyl butanoate, and methyl salicylate] together with one of the most studied plant defense activators methyl jasmonate, over 24 h on their defense induction. All HIPVs were capable of activating defensive response in tomato plants and upregulating the genes of defense; proteinase Inhibitor II (PIN2), pathogenesis-related protein precursor (PR1) and Sl-PI-I marker genes for the JA, SA and plant Proteinase Inhibitor I signaling pathway, respectively. Furthermore, the repellence effect of two potentially selected volatiles [(Z)-3-hexenyl propanoate and methyl salicylate] on tomato pests F. occidentalis, B. tabaci and Tuta absoluta (Meyrick) (Lepidoptera: Gelechiidae) and the attraction effect to the parasitoid E. formosa were revealed. Nevertheless, in sweet pepper plants this approach has not been studied and the already characterized HIPVs (Bouagga et al., 2017, 2018) have not yet been tested for their effect in plant defense induction through exposure. For this reason, in this study we aim to investigate the potential of five previously identified HIPVs emitted from herbivore induced-sweet pepper plants [(Z)-3-hexenol, (Z)-3-hexenyl acetate, (Z)-3-hexenyl propanoate, (Z)-3-hexenyl butanoate and methyl salicylate) (Bouagga et al., 2017, 2018)] to induce plant defenses through exposure of intact sweet pepper plants. In addition to the five volatiles described above, we decided to test two other HIPV’s (hexyl butanoate and methyl jasmonate) due to their interest as elicitors found in the literature (Pérez-Hedo et al., 2021a). Subsequently, individual sweet pepper plants were exposed separately to each of the above seven HIPVs over 48 h, and then the quantification of the expression level of the defensive gene markers were performed. Using a Y-tube olfactometer, we also tested the olfactive response of the key sweet pepper pest F. occidentalis and its arthropod predator O. laevigatus to sweet pepper plants that were exposed individually to HIPVs over 48 h and unexposed sweet pepper plants. Finally, we discussed the potential of HIPV(s) to stimulate plant defenses, which should be tested at field level for their future use as new and sustainable tool for sweet pepper biological control strategies.
Materials and Methods
Insects and Plants
Pesticide-free Capsicum annum (Solanaceae) cv. (Lipari) (Dulce Italiano, Mascarell Semillas S.L., Valencia, Spain) plants were used in all the experiments. Two weeks after germination, plants were individually transplanted into plastic pots (8 × 8 × 8 cm) and maintained in a climatic chamber at Instituto Valenciano de Investigaciones Agrarias (IVIA) at 25 ± 2°C, relative humidity (RH) of 65 ± 10% and a photoperiod of 14:10 h (L:D). Six leaf fully developed plants (20 cm height approximately) were used for the experiments.
Orius laevigatus adults were supplied by Koppert Biological Systems, S.L. (Águilas, Murcia, Spain). Upon reception, adults were released and maintained on green bean pods (Phaseolus vulgaris L. Fabales: Fabaceae) in a plastic cage (30 × 30 × 30 cm) (BugDorm-1 Insect Tents; MegaView Science Co., Ltd., Taichung, Taiwan) with Ephestia kuehniella (Zeller) (Lepidoptera: Pyralidae) as supplementary food until use. From there, O. laevigatus females were selected and transferred into a plastic cage (30 × 30 × 30 cm) (BugDorm-1 Insect Tents) with water supplied on soaked cotton plugs and starved for 24 h. Frankliniella occidentalis adults were obtained from colony reared on green bean pods in growth chamber at 25 ± 2°C, 65 ± 10% RH and 14:10 h (L:D) photoperiod at IVIA. In the case of F. occidentalis, females were enclosed in a Petri dish (9 cm in diameter) and also starved for 24 h before use. Females of both species, always less than 5 days-old, were tested for the Y-tube experiments.
Sweet Pepper Plants Exposure to Herbivore-Induced Plant Volatiles and Plant Gene Expression Analysis
Synthetic standards of volatiles compounds: (Z)-3-hexenol [(Z)-3-H], (Z)-3-hexenyl acetate [(Z)-3-HA], (Z)-3-hexenyl propanoate [(Z)-3-HP], (Z)-3-hexenyl butanoate [(Z)-3-HB], hexyl butanoate (HB), methyl salicylate (MeSA) and methyl jasmonate (MeJA) were purchased from Sigma-Aldrich (St. Louis, MO, United States). Plants were exposed to pure volatiles using a polymeric low-density dispenser (Pérez-Hedo et al., 2021b). These dispensers, which guarantee a constant release rate of 9.6 mg/day, were filled with cotton soaked with 1 ml of each volatile and then placed in 60 × 60 × 60 plastic cage (BugDorm-2 insect tents; MegaView Science Co., Ltd., Taichung, Taiwan). Eight sweet pepper plants were placed in a plastic cage and exposed to one of the tested volatiles over 48 h in a climatic chamber at 25 ± 2°C, 65 ± 10% RH and 14:10 h (L:D) photoperiod. Similarly, eight unexposed plants were isolated in other climatic chamber at same conditions over 48 h.
Following this procedure described above to expose the HIPVs, two young apical leaves from eight exposed plants per volatile and eight unexposed plants were used to quantify the expression of defensive gene markers: PIN2 (proteinase inhibitor II) JA-gene marker, AMP1 (antimicrobial peptide 1) SA-gene marker and ABA-gen marker ASR1 (ABA stress ripening protein 1) (Pérez-Hedo et al., 2015; Bouagga et al., 2017, 2018).
Samples of the apical part of volatile-exposed sweet pepper plants and unexposed plants were collected and ground in liquid nitrogen for NZYol (NZYTech, Lisboa, Portugal) based RNA extraction. 1 μg of each RNA sample was treated with TURBO DNA-free™ Kit (Ambion®, Life Technologies, CA, United States) to remove contaminating DNA. Reverse transcription RT proceeded and cDNA was synthesized using Prime Script™ RT Reagent Kit (TAKARA Bio, CA, United States). Real-time PCR amplification was performed in LightCycler® 480 System (Roche Molecular Systems, Inc., Switzerland), using NZYSpeedy qPCR Green Master Mix (2x) (NZYTech, Lisboa, Portugal) as described by Pérez-Hedo et al. (2015). Primers sequences of defensive genes PIN2, AMP1, ASR1 and the housekeeping gen EF1 (Elongator factor 1) used as standard control gene for normalization are represented in Table 1.
Y-Tube Bioassays
A Y-tube olfactometer bioassay was conducted to test F. occidentalis and O. laevigatus female’s response to two odor sources (Volatle-exposed plants and unexposed plants) using 20 cm height sweet pepper plants. We tested females since they are the responsible for selecting the host for oviposition. Eight sweet pepper plants were exposed to one of the tested volatiles over 48 h in a plastic cage (60 × 60 × 60 cm; BugDorm-2 insect tents) in a climatic chamber at 25 ± 2°C, 65 ± 10% RH and 14:10 h (L:D) photoperiod. Similarly, eight unexposed plants were isolated in other climatic chamber at same conditions over 48 h. The Y-tube olfactometer (Analytical Research Systems, Gainesville, FL) consisted of a Y-shaped glass tube (2.4 cm on diameter, 13.5 cm length base and two arms 5.75 cm length each) which was connected via high density polyethylene (HDPE) tubes to two 5 L glass jars. Each jar contained an odor source (volatile-exposed plant or unexposed plant which were immediately transferred from exposition cages) and was connected to a unidirectional air pump with 150 ml/min airflow rate. The experiment environmental conditions were 23 ± 2°C, RH 60% ± 10 and light intensity of 2516 lux (Pérez-Hedo and Urbaneja, 2015).
Each individual female F. occidentalis or O. laevigatus were introduced in the entry base arm and observed for a maximum of 15 min. Once the insect walked at least 3 cm up one of the arms the time was recorded and the individual considered a “responder.” If after 15 min the individual did not make a choice between either arm, it was excluded from the data analysis. Each individual was used only once and the total number of replicates per species was 40–50 responder individuals. After five females were tested, the right and left arms were switched to avoid any spatial effect. After testing 10 females the Y-tube glass material (jars and Y-arm) was rinsed with soap, water and acetone and was dried for 5 min and new plants (exposed and unexposed) were put in place.
Data Analysis
Data obtained from Y-tube olfactometer experiment were statically analyzed by chi-square goodness of fit test based on a null model in which the two odor sources are selected with equal frequency. Data of gene expression quantification were modified using logarithmic transformation and then analyzed by one-way analysis of variance (ANOVA). Tukey’s test was used for mean comparison at α < 0.05. All statistical analyses were performed using GraphPad Prism 9 for Windows (GraphPad Software, San Diego, California United States).1
Results
Plant Gene Expression
The quantification of marker genes PIN2 [F(7, 48) = 17.19; P < 0.0001] and AMP1 [F(7, 48) = 9.565; P < 0.0001] involved, respectively, in JA and SA signaling pathways were upregulated in all plants exposed individually to each of the seven volatiles tested in comparison to unexposed plants (Figure 1). In a similar way, all the volatiles activated the SA mechanism overexpressing the defensive gene AMP1 (Figure 1B). However, the volatile (Z)-3-hexenol, hexyl butanoate and methyl salicylate induced higher expression of PIN2 than all other volatiles (Figure 1A). On the other hand, the ABA pathway marked by the gen ASR1 was not overexpressed in sweet pepper plants exposed to volatiles neither in intact plants [F(7, 48) = 1.546; P = 0.1749] (Figure 1C).
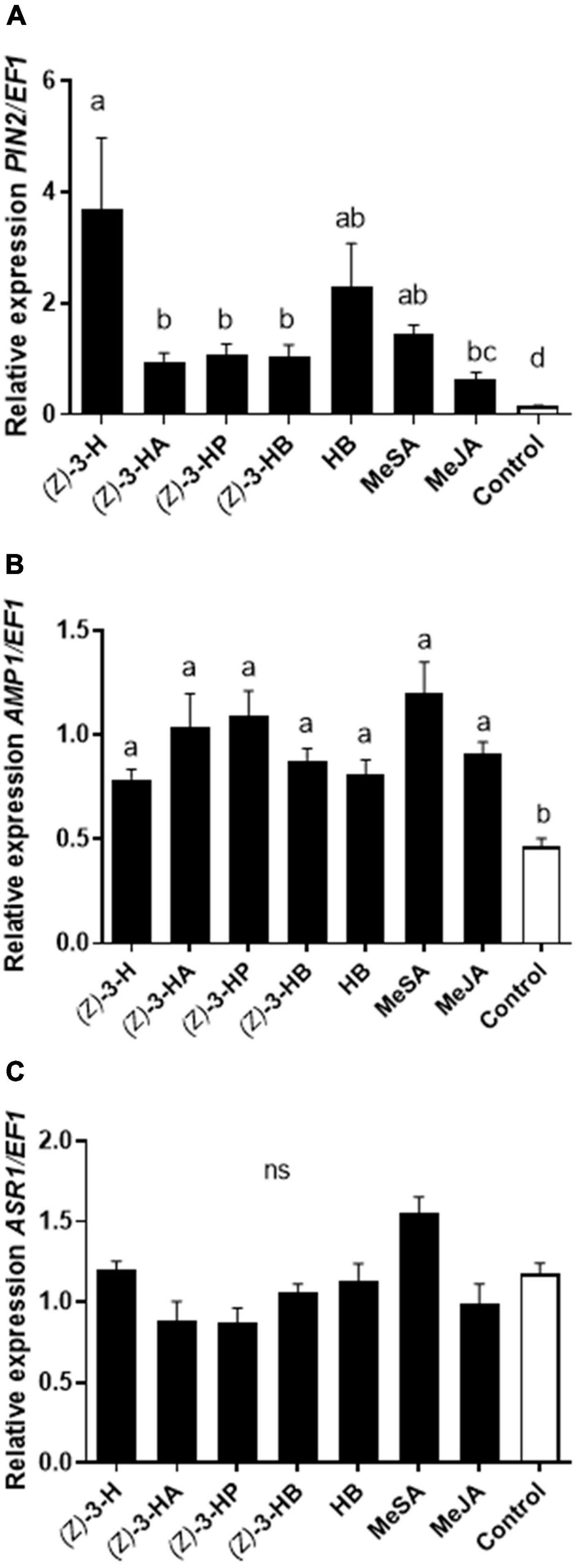
Figure 1. Transcriptional response of the defensive genes PIN2 (A), AMP1 (B) and ASR1 (C) markers of JA, SA, and ABA signaling pathways, respectively, in sweet pepper plants exposed to HIPVs: (Z)-3-hexenol [(Z)-3-H], (Z)-3-hexenyl acetate [(Z)-3-HA], (Z)-3-hexenyl propanoate [(Z)-3-HP], (Z)-3-hexenyl butanoate [(Z)-3-HB], hexyl butanoate (HB), methyl salicylate (MeSA) and methyl jasmonate (MeJA). Transcription levels were normalized to the expression of the housekeeping gene EF1 measured in the same sample. Data are represented as the mean of eight independent analysis of transcript expression relative to EF1 ± SE (n = 8). Significant differences based on ANOVA and Tukey’s multiple comparison test are represented in different letters (P < 0.05).
Y-Tube Bioassays
Females F. occidentalis did not show a preference to odors from intact sweet pepper plants and plants exposed to (Z)-3-H (χ2 = 2.5; P = 0.1138), (Z)-3-HA (χ2 = 0.21; P = 0.6473), HB (χ2 = 0.56; P = 0.4561), and MeJA (χ2 = 1.60; P = 0.2059) (Figure 2A). However, sweet pepper plants exposed to (Z)-3-HP and MeSA were more repellent to F. occidentalis than intact plants (χ2 = 4.25, P = 0.0394 and χ2 = 7.08, P = 0.0078, respectively). Plants exposed to (Z)-3-HB showed also a trend to be repellent against F. occidentalis but statistical differences were not found (χ2 = 3.81; P = 0.0508).
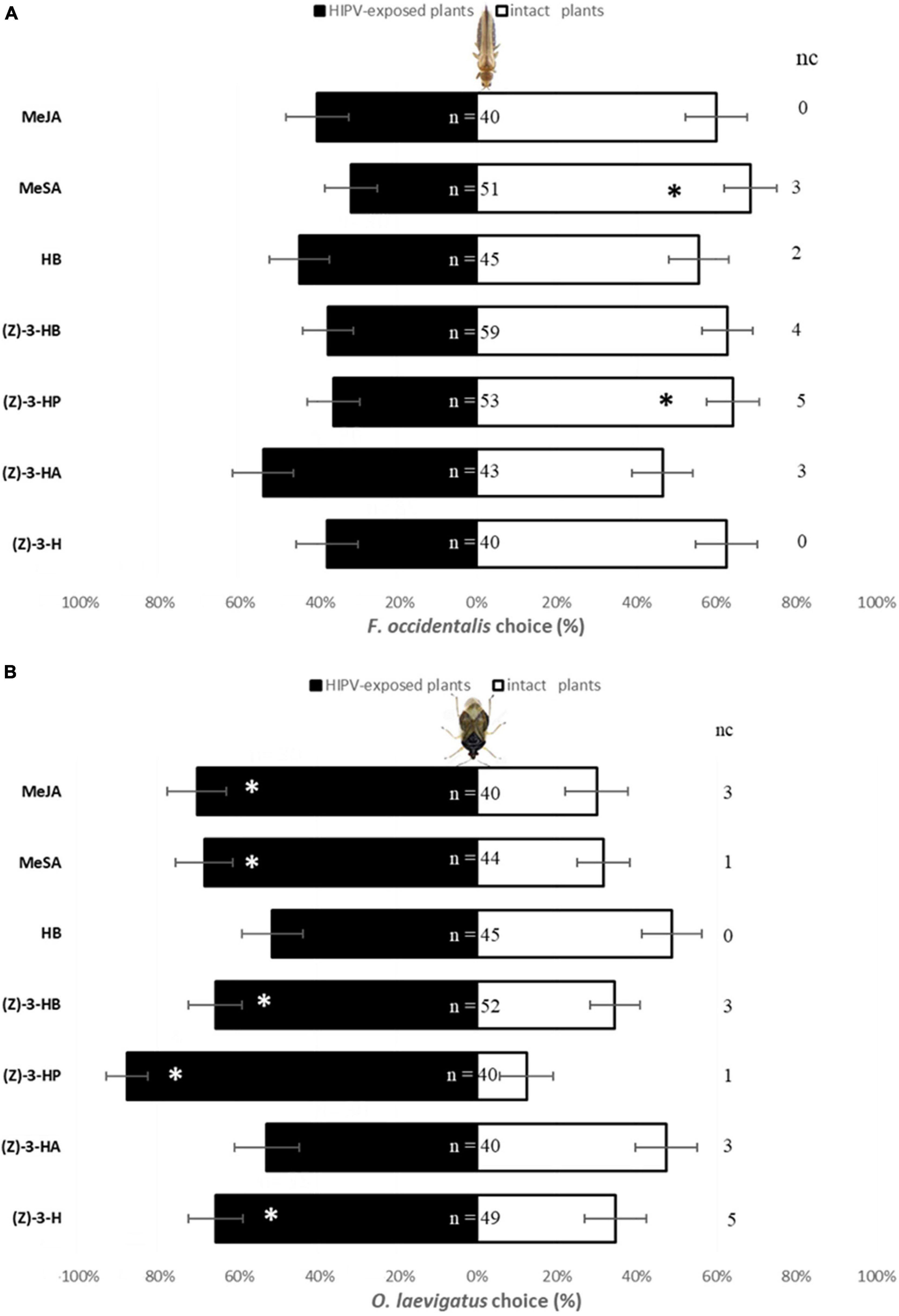
Figure 2. Response (% ± SE) in Y-Tube Olfactometer of F. occidentalis (A) and O. laevigatus (B) females to two sweet pepper odor sources: intact plants and 48 h-exposed plants to (Z)-3-hexenol [(Z)-3-H], (Z)-3-hexenyl acetate [(Z)-3-HA], (Z)-3-hexenyl propanoate [(Z)-3-HP], (Z)-3-hexenyl butanoate [(Z)-3-HB], hexyl butanoate (HB), methyl salicylate (MeSA) and methyl jasmonate (MeJA) separately. n is total number of responder females and nc (no choice) is number of individuals who didn’t make a choice. Significant differences based on χ2-tests are marked using asterisk * (P < 0.05).
Orius laevigatus females were more attracted to odors from plants exposed to (Z)-3-H (χ2 = 4.59; P = 0.0321), (Z)-3-HP (χ2 = 22.5; P < 0.0001), (Z)-3-HB (χ2 = 4.92; P = 0.0265), MeSA (χ2 = 5.82; P = 0.0159) and MeJA (χ2 = 6.4; P = 0.0114) than to sweet pepper intact plants (Figure 2B). Nevertheless, when O. laevigatus females were offered a choice between odors from intact plants and plants exposed to (Z)-3-HA (χ2 = 0.1; P = 0.7518) and HB (χ2 = 0.02; P = 0.8815) they showed no preference.
Discussion
The individual exposure of sweet pepper plants to herbivore-induced plants volatiles i.e., methyl jasmonate, methyl salicylate and five GLVs has been shown to induce defenses in HIPVs-exposed plants. All tested synthetic volatiles significantly upregulated the expression of defense-related genes PIN2 and AMP1 (Figure 1). Despite the similarity in overall upregulation, some volatiles have overexpressed more than others the transcriptional synthesis and transduction signaling. Hence, the green leaf volatile (Z)-3-hexenol [(Z)-3-H] was the major volatile compound upregulating PIN2. Indeed, GLVs being the early and rapid compounds synthetized under herbivore attack, are well-reported for their ability to induce plant defenses to biotic as well as abiotic stresses (Scala et al., 2013; Yamauchi et al., 2015; Cofer et al., 2018). They have been found to prime JA related gene expression and to trigger the release of VOCs (Engelberth et al., 2004; Frost et al., 2008b; Timilsena et al., 2020). When healthy intact maize plants were exposed separately to (Z)-3-hexenol and other C6 compounds, the JA signaling pathway was activated which stimulated an increased level of jasmonates molecules (Engelberth et al., 2004; Ruther and Kleier, 2005). Besides, it has been reported that (Z)-3-hexenol exposure upregulated genes associated with salicylic acid activation pathway and calcium transcriptional regulation (Engelberth et al., 2013).
Many studies have demonstrated that the combined use of two or more volatile compounds mediates a specific and adaptive defensive response which further enhances both direct and indirect defenses (Ruther and Kleier, 2005; Hu et al., 2019), which could be of great benefit when incorporated to pest management programs. In the case of maize, the associated application of volatile hormone ethylene along with GLVs synergistically increased plant induction and VOCs emission (Ruther and Kleier, 2005). Moreover, Hu et al. (2019) have shown that the simultaneous exposure of maize plants to the green leaf volatile (Z)-3-hexenyl acetate and the aromatic volatile indole have additively primed specific defensive response and elicited in advantage JA signaling pathway in comparison to individual exposure to both volatiles. However, this dual-exposure has resulted in an antagonistic effect on the composition of the volatiles induced by exposure, which was qualitatively reduced than single exposures. Further studies aiming to understand the potentiality and reliability of volatiles in combination and how plants integrate multiple volatile cues into hormonal responses are important for improving plant defenses strategies.
HIPVs are known to induce defenses and to trigger stress metabolic biosynthesis in healthy plants (Pérez-Hedo et al., 2021b). Here, both defensive genes PIN2 and AMP1 were significantly overexpressed in volatile-exposed plants (Figure 1). AMP1 is a gene of the antimicrobial peptides immunity network that is implicated in broad-spectrum resistance to bacterial and fungal pathogens (Lee et al., 2008). The overexpression of AMP1 stimulates the SA-dependent pathway (Bolouri-Moghaddam et al., 2016; Niu et al., 2020). The SA defensive signaling pathway is reported as an essential mechanism of plant defense against biotrophic pathogens and phloem-feeding herbivores (Erb et al., 2012) and it has been well-established for its crucial and functional role in the regulation of signal transduction pathways and the activation of local and systemic-acquired resistance (Gaffney et al., 1993). Whereas, JA signaling is known to modulate defenses against necrotrophic pathogens and chewing insects (Erb et al., 2012). It has been speculated that SA interact with and may evolve cross talks with JA signaling (Robert-Seilaniantz et al., 2011; Li et al., 2019). Elevated chewing herbivore-resistance lead to enhanced susceptibility to phloem-feeding insects. However, the JA and SA correlation either antagonistic or synergistic is pathogen/herbivore dependent (Spoel et al., 2003; Beckers and Spoel, 2006). Here, both jasmonic acid JA and salicylic acid SA signaling pathways were synergistically upregulated in sweet pepper plants exposed to HIPVs (Figure 1). Similarly the exposure of tomato plants to the same volatiles compounds (Pérez-Hedo et al., 2021a) activated both JA and SA mediated defense response. The role of the phytohormones SA and JA in endogenous regulation of plant defense related-traits is well documented. The exogenous application of MeSA and MeJA have been demonstrated to elicit plant defenses and potentially manage herbivore pests (Vallad and Goodman, 2004; Erb et al., 2012; Santino et al., 2013; Cao et al., 2014; Freitas et al., 2018).
The ABA metabolic pathway did not seem to be elicited by the seven volatile compounds in this experiment. Likewise in corn plants exposed to aromatic volatile indole (Ye et al., 2019), the ABA accumulation was not affected by the exposure. In contrast the exposure of maize plants to same volatile, i.e., indole, enhanced the phytohormone ABA production (Erb et al., 2015). These differences in HIPVs mediated hormonal response may be due to plant species diversity or to the specificity of volatiles in triggering signaling cascades (Engelberth et al., 2013).
The induction of plant defenses by volatiles exposure mediates direct (e.g., repelling of insect feeding or oviposition) and indirect effects (e.g., attracting predators or parasitoids) on insect pests. In this work, the olfactory behavior analysis demonstrated that plants exposed to volatiles (Z)-3-HP and MeSA were repellent to herbivore F. occidentalis (Figure 2A). Yet, the predator O. laevigatus was highly attracted to plants exposed to (Z)-3-H, (Z)-3-HP, (Z)-3-HB, MeSA and MeJA (Figure 2B). In many crop species, it has been reported that the exposure of plants to volatiles decreases pest performance and oviposition, while attracting natural enemies. For example, tomato plants exposed to (Z)-3-HP and MeSA effected their attractiveness to the tomato pests T. absoluta, B. tabaci, and F. occidentalis (Pérez-Hedo et al., 2021a). Also, tomato plants exposure to (Z)-3-hexenol decreased oviposition rate and reduced feeding activity of B. tabaci, while it increased the attraction of its parasitoid E. formosa (Yang et al., 2020). In corn, as well, previous studies have demonstrated that indole volatile can elicit direct defenses in maize, repelling Spodoptera littoralis Boisduval (Lepidoptera: Noctuidae) and increasing juvenile mortality (Veyrat et al., 2016; Ye et al., 2019).
Plant exposure to HIPVs makes defense mechanisms stronger and more robust. For instance, the composition and the amount of plant volatiles emitted by tomato plants after exposure to HIPVs was higher (Pérez-Hedo et al., 2021a) than those induced by herbivore attack (Pérez-Hedo et al., 2018). The increased quantity of emitted volatiles in HIPV-induced plants may antagonize the plant constitutive response (Zhang et al., 2020). In fact, plants when exposed to volatiles induced by the herbivory of Spodoptera exigua (Hübner) (Lepidoptera: Noctuidae) allocate more recourses in induced defenses by priming JA and proteinase inhibitor protein (PI-II) accumulation and enhancing chemical compounds emission, while the constitutive defense modulated by PI-II production and S. exigua weight gain was limited. Although, many studies have reported that the induced defenses come at a cost to some physiological mechanisms and so negatively impact plant performance and fitness (Baldwin, 1998; Heil and Baldwin, 2002).
Bouagga et al. (2017, 2018) identified the set of volatiles emitted upon feeding of O. laevigatus, M. pygmaeus and N. tenuis, which belong to GLVs esters, terpenes and methyl salicylate. However, in this work the HIPVs emitted after plant defense induction through exposure to volatiles have not been characterized. Therefore, it would be important to identify which are these volatiles, measure the emission level and also to study the costs/benefits of plant defenses induction through HIPVs expore.
In summary, our results demonstrate that the HIPVs exposure can elicit resistant response in sweet pepper via the induction of JA and SA signaling pathways. HIPVs can enhance plant resistance to herbivores (i.e., throughout repellence) and may mediate herbivores susceptibility to natural biological control agents. Yet, many questions remain open; which are the HIPVs emitted by HIPVs exposed-plants? HIPVs could influence other sweet pepper pest’ behavior? In addition to chemical defenses (release of VOCs), may HIPV exposure trigger the structural-based defenses enhancing glandular trichomes density known by their role in secondary metabolites secretion and plant protection? How can multiple volatiles interact to prime plant response? How do plants integrate chemical cues to target metabolic mechanisms? Is there an equilibrated cost/benefit of the activation of induced-defense cascades against herbivores? Answering these questions will help to understand the effectiveness of HIPVs in defense induction, consequently, providing a reliable and sustainable approach for pest management.
Data Availability Statement
The raw data supporting the conclusions of this article will be made available by the authors, without undue reservation.
Author Contributions
MP-H and AU conceived the idea. CR, MA-V, MP-H, and AU designed the research methodology. CR and JG-R performed the experiments. CR and MP-H analyzed the data. All authors discussed the drafts, took part in writing the manuscript and gave final approval for publication.
Funding
The research leading to these results was partially funded by the Spanish Ministry of Economy and Competitiveness MINECO (RTA2017-00073-00-00 and PID2020-113234RR-I00) and the Conselleria d’Agricultura, Pesca i Alimentació de la Generalitat Valenciana. CR was supported by grant from INIA (PRE2018-084631).
Conflict of Interest
MP-H, MA-V, and AU were inventors on the Spanish Patent No. P202030330 entitled “Uso de ésteres de (Z)-3-hexenilo y método para proteger plantas frente a plagas”.
The remaining authors declare that the research was conducted in the absence of any commercial or financial relationships that could be construed as a potential conflict of interest.
Publisher’s Note
All claims expressed in this article are solely those of the authors and do not necessarily represent those of their affiliated organizations, or those of the publisher, the editors and the reviewers. Any product that may be evaluated in this article, or claim that may be made by its manufacturer, is not guaranteed or endorsed by the publisher.
Acknowledgments
We thank Alice Mockford (University of Worcester) for helpful comments on earlier versions of the manuscript, to Dr. Javier Calvo (Koppert Biological Systems, S.L., Spain) for supplying the insects and Jose Catalán (IVIA) for arthropod colonies.
Footnotes
References
Arimura, G. I., Matsui, K., and Takabayashi, J. (2009). Chemical and molecular ecology of herbivore-induced plant volatiles: proximate factors and their ultimate functions. Plant Cell Physiol. 50, 911–923. doi: 10.1093/pcp/pcp030
Baldwin, I. T. (1998). Jasmonate-induced responses are costly but benefit plants under attack in native populations. Proc. Natl. Acad. Sci. U.S.A. 95, 8113–8118. doi: 10.1073/pnas.95.14.8113
Baldwin, I. T., Kessler, A., and Halitschke, R. (2002). Volatile signaling in plant–plant–herbivore interactions: what is real? Curr. Opin. Plant Biol. 5, 351–354. doi: 10.1016/s1369-5266(02)00263-7
Bari, R., and Jones, J. D. G. (2009). Role of plant hormones in plant defence responses. Plant Mol. Biol. 69, 473–488. doi: 10.1007/s11103-008-9435-0
Beckers, G. J. M., and Spoel, S. H. (2006). Fine-tuning plant defence signalling: salicylate versus jasmonate. Plant Biol. 8, 1–10. doi: 10.1055/s-2005-872705
Bolouri-Moghaddam, M. R., Vilcinskas, A., and Rahnamaeian, M. (2016). Cooperative interaction of antimicrobial peptides with the interrelated immune pathways in plants. Mol. Plant Pathol. 17, 464–471. doi: 10.1111/mpp.12299
Bouagga, S., Urbaneja, A., Rambla, J. L., Flors, V., Granell, A., Jaques, J. A., et al. (2018). Zoophytophagous mirids provide pest control by inducing direct defences, antixenosis and attraction to parasitoids in sweet pepper plants. Pest Manage. Sci. 74, 1286–1296. doi: 10.1002/ps.4838
Bouagga, S., Urbaneja, A., Rambla, J. L., Granell, A., and Pérez-Hedo, M. (2017). Orius laevigatus strengthens its role as a biological control agent by inducing plant defenses. J. Pest Sci. 91, 55–64. doi: 10.1007/s10340-017-0886-4
Cao, H., Wang, S., and Liu, T. (2014). Jasmonate- and salicylate-induced defenses in wheat affect host preference and probing behavior but not performance of the grain aphid, Sitobion avenae. Insect Sci. 21, 47–55. doi: 10.1111/1744-7917.12023
Cofer, T. M., Engelberth, M., and Engelberth, J. (2018). Green leaf volatiles protect maize (Zea mays) seedlings against damage from cold stress. Plant Cell Environ. 41, 1673–1682. doi: 10.1111/pce.13204
Dicke, M., and van Loon, J. J. A. (2014). Chemical ecology of phytohormones: how plants integrate responses to complex and dynamic environments. J. Chem. Ecol. 40, 653–656. doi: 10.1007/s10886-014-0479-0
Engelberth, J., Alborn, H. T., Schmelz, E. A., and Tumlinson, J. H. (2004). Airborne signals prime plants against insect herbivore attack. Proc. Natl. Acad. Sci. U.S.A. 101, 1781–1785. doi: 10.1073/pnas.0308037100
Engelberth, J., Contreras, C. F., Dalvi, C., Li, T., and Engelberth, M. (2013). Early transcriptome analyses of Z-3-Hexenol-treated Zea mays revealed distinct transcriptional networks and anti-herbivore defense potential of green leaf volatiles. PLoS One 8:e77465. doi: 10.1371/journal.pone.0077465
Erb, M., Meldau, S., and Howe, G. A. (2012). Role of phytohormones in insect-specific plant reactions. Trends Plant Sci. 17, 250–259. doi: 10.1016/j.tplants.2012.01.003
Erb, M., Veyrat, N., Robert, C. A. M., Xu, H., Frey, M., Ton, J., et al. (2015). Indole is an essential herbivore-induced volatile priming signal in maize. Nat. Commun. 6:6273. doi: 10.1038/ncomms7273
Freitas, T., Stout, M., and Sant’Ana, J. (2018). Effects of exogenous methyl jasmonate and salicylic acid on rice resistance to Oebalus pugnax. Pest Manag. Sci. 75, 744–752. doi: 10.1002/ps.5174
Frost, C. J., Mescher, M. C., Carlson, J. E., and De Moraes, C. M. (2008a). Plant defense priming against herbivores: getting ready for a different battle. Plant Physiol. 146, 818–824. doi: 10.1104/pp.107.113027
Frost, C. J., Mescher, M. C., Dervinis, C., Davis, J. M., Carlson, J. E., and De Moraes, C. M. (2008b). Priming defense genes and metabolites in hybrid poplar by the green leaf volatile cis-3-hexenyl acetate. New Phytol. 180, 722–734. doi: 10.1111/j.1469-8137.2008.02599.x
Gaffney, T., Friedrich, L., Vernooij, B., Negrotto, D., Nye, G., Uknes, S., et al. (1993). Requirement of salicylic acid for the induction of systemic acquired resistance. Science 261, 754–756. doi: 10.1126/science.261.5122.754
Heil, M. (2008). Indirect defence via tritrophic interactions. New Phytol. 178, 41–61. doi: 10.1111/j.1469-8137.2007.02330.x
Heil, M., and Baldwin, I. T. (2002). Fitness costs of induced resistance: emerging experimental support for a slippery concept. Trends Plant Sci. 7, 61–67. doi: 10.1016/s1360-1385(01)02186-0
Heil, M., and Karban, R. (2010). Explaining evolution of plant communication by airborne signals. Trends Ecol. Evol. 25, 137–144. doi: 10.1016/j.tree.2009.09.010
Hu, L., Ye, M., and Erb, M. (2019). Integration of two herbivore-induced plant volatiles results in synergistic effects on plant defence and resistance. Plant Cell Environ. 42, 959–971. doi: 10.1111/pce.13443
Kessler, A., Halitschke, R., Diezel, C., and Baldwin, I. T. (2006). Priming of plant defense responses in nature by airborne signaling between Artemisia tridentata and Nicotiana attenuata. Oecologia 148, 280–292. doi: 10.1007/s00442-006-0365-8
Kost, C., and Heil, M. (2006). Herbivore-induced plant volatiles induce an indirect defence in neighbouring plants. J. Ecol. 94, 619–628. doi: 10.1111/j.1365-2745.2006.01120.x
Lee, S. C., Hwang, I. S., Choi, H. W., and Hwang, B. K. (2008). Involvement of the pepper antimicrobial protein CaAMP1 gene in broad spectrum disease resistance. Plant Physiol. 148, 1004–1020. doi: 10.1104/pp.108.123836
Li, N., Han, X., Feng, D., Yuan, D., and Huang, L. J. (2019). Signaling crosstalk between salicylic acid and ethylene/jasmonate in plant defense: do we understand what they are whispering? Int. J. Mol. Sci. 20:671. doi: 10.3390/ijms20030671
Niu, L., Zhong, X., Zhang, Y., Yang, J., Xing, G., Li, H., et al. (2020). Enhanced tolerance to Phytophthora root and stem rot by over-expression of the plant antimicrobial peptide CaAMP1 gene in soybean. BMC Genet. 21:1–10. doi: 10.1186/s12863-020-00872-0
Pérez-Hedo, M., Alonso-Valiente, M., Vacas, S., Gallego, C., Pons, C., Arbona, V., et al. (2021b). Plant exposure to herbivore-induced plant volatiles: a sustainable approach through eliciting plant defenses. J. Pest Sci. 94, 1221–1235. doi: 10.1007/s10340-021-01334-x
Pérez-Hedo, M., Alonso-Valiente, M., Vacas, S., Gallego, C., Rambla, J. L., Navarro-Llopis, V., et al. (2021a). Eliciting tomato plant defenses by exposure to herbivore induced plant volatiles. Entomologia Generalis 41, 209–218. doi: 10.1111/pce.14209
Pérez-Hedo, M., Arias-Sanguino, ÁM., and Urbaneja, A. (2018). Induced tomato plant resistance against Tetranychus urticae triggered by the phytophagy of Nesidiocoris tenuis. Front. Plant Sci. 9:1419. doi: 10.3389/fpls.2018.01419
Pérez-Hedo, M., and Urbaneja, A. (2015). Prospects for predatory mirid bugs as biocontrol agents of aphids in sweet peppers. J. Pest Sci. 88, 65–73. doi: 10.1007/s10340-014-0587-1
Pérez-Hedo, M., Urbaneja-Bernat, P., Jaques, J. A., Flors, V., and Urbaneja, A. (2015). Defensive plant responses induced by Nesidiocoris tenuis (Hemiptera: Miridae) on tomato plants. J. Pest Sci. 88, 543–554. doi: 10.1007/s10340-014-0640-0
Robert-Seilaniantz, A., Grant, M., and Jones, J. D. G. (2011). Hormone crosstalk in plant disease and defense: more than just jasmonate-salicylate antagonism. Annu. Rev. Phytopathol. 49, 317–343. doi: 10.1146/annurev-phyto-073009-114447
Ruther, J., and Kleier, S. (2005). Plant-plant signaling: ethylene synergizes volatile esmission in Zea mays induced by exposure to (Z)-3-hexen-1-ol. J. Chem. Ecol. 31, 2217–2222. doi: 10.1007/s10886-005-6413-8
Santino, A., Taurino, M., De Domenico, S., Bonsegna, S., Poltronieri, P., Pastor, V., et al. (2013). Jasmonate signaling in plant development and defense response to multiple (a)biotic stresses. Plant Cell Rep. 32, 1085–1098. doi: 10.1007/s00299-013-1441-2
Scala, A., Allmann, S., Mirabella, R., Haring, M. A., and Schuurink, R. C. (2013). Green leaf volatiles_ a plant’s multifunctional weapon against herbivores and pathogens. Int. J. Mol. Sci. 14, 17781–17811. doi: 10.3390/ijms140917781
Spoel, S. H., Koornneef, A., Claessens, S. M. C., Korzelius, J. P., Van Pelt, J. A., Mueller, M. J., et al. (2003). NPR1 modulates cross-talk between salicylate- and jasmonate-dependent defense pathways through a novel function in the cytosol. Plant Cell 15, 760–770. doi: 10.1105/tpc.009159
Timilsena, B. P., Seidl-Adams, I., and Tumlinson, J. H. (2020). Herbivore-specific plant volatiles prime neighboring plants for nonspecific defense responses. Plant Cell Environ. 43, 787–800. doi: 10.1111/pce.13688
Vallad, G., and Goodman, R. (2004). Systemic acquired resistance and induced systemic resistance in conventional agriculture. Crop Sci. 44, 1920–1934. doi: 10.2135/cropsci2004.1920
Veyrat, N., Robert, C. A. M., Turlings, T. C. J., and Erb, M. (2016). Herbivore intoxication as a potential primary function of an inducible volatile plant signal. J. Ecol. 104, 591–600. doi: 10.1111/1365-2745.12526
War, A. R., Sharma, H. C., Paulraj, M. G., YousfWar, M., and Ignacimuthu, S. (2011). Herbivore induced plant volatiles: their role in plant defense for pest management. Plant Signal. Behav. 6, 1973–1978. doi: 10.4161/psb.6.12.18053
Yamauchi, Y., Kunishima, M., Mizutani, M., and Sugimoto, Y. (2015). Reactive short-chain leaf volatiles act as powerful inducers of abiotic stress-related gene expression. Sci. Rep. 5:8030. doi: 10.1038/srep08030
Yang, F., Zhang, Q., Yao, Q., Chen, G., Tong, H., Zhang, J., et al. (2020). Direct and indirect plant defenses induced by (Z)-3-hexenol in tomato against whitefly attack. J. Pest Sci. 93, 1243–1254. doi: 10.1007/s10340-020-01234-6
Ye, M., Glauser, G., Lou, Y., Erb, M., and Hu, L. (2019). Molecular dissection of early defense signaling underlying volatile-mediated defense regulation and herbivore resistance in rice. Plant Cell 31, 687–698. doi: 10.1105/tpc.18.00569
Keywords: HIPVs, Frankliniella occidentalis, Orius laevigatus, gene expression, behavioral response
Citation: Riahi C, González-Rodríguez J, Alonso-Valiente M, Urbaneja A and Pérez-Hedo M (2022) Eliciting Plant Defenses Through Herbivore-Induced Plant Volatiles’ Exposure in Sweet Peppers. Front. Ecol. Evol. 9:776827. doi: 10.3389/fevo.2021.776827
Received: 14 September 2021; Accepted: 19 November 2021;
Published: 04 January 2022.
Edited by:
Anne Marie Cortesero, University of Rennes 1, FranceReviewed by:
Jorge Alberto Zavala, Consejo Nacional de Investigaciones Científicas y Técnicas (CONICET), ArgentinaIslam S. Sobhy, Keele University, United Kingdom
Copyright © 2022 Riahi, González-Rodríguez, Alonso-Valiente, Urbaneja and Pérez-Hedo. This is an open-access article distributed under the terms of the Creative Commons Attribution License (CC BY). The use, distribution or reproduction in other forums is permitted, provided the original author(s) and the copyright owner(s) are credited and that the original publication in this journal is cited, in accordance with accepted academic practice. No use, distribution or reproduction is permitted which does not comply with these terms.
*Correspondence: Meritxell Pérez-Hedo, perez_merhed@gva.es