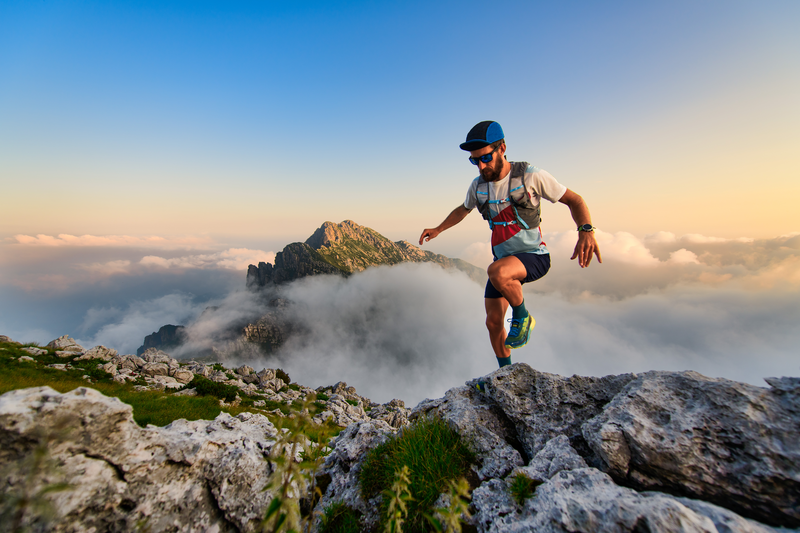
94% of researchers rate our articles as excellent or good
Learn more about the work of our research integrity team to safeguard the quality of each article we publish.
Find out more
ORIGINAL RESEARCH article
Front. Ecol. Evol. , 25 November 2021
Sec. Paleoecology
Volume 9 - 2021 | https://doi.org/10.3389/fevo.2021.771700
This article is part of the Research Topic Lessons from the Past: Linking the Paleofire Record and Fire Management in the Context of a Warming World View all 7 articles
Paleoecology has demonstrated potential to inform current and future land management by providing long-term baselines for fire regimes, over thousands of years covering past periods of lower/higher rainfall and temperatures. To extend this potential, more work is required for methodological innovation able to generate nuanced, relevant and clearly interpretable results. This paper presents records from Cape York Peninsula, Queensland, Australia, as a case study where fire management is an important but socially complex modern management issue, and where palaeofire records are limited. Two new multiproxy palaeofire records are presented from Sanamere Lagoon (8,150–6,600 cal BP) and Big Willum Swamp (3,900 cal BP to present). These records combine existing methods to investigate fire occurrence, vegetation types, and relative fire intensity. Results presented here demonstrate a diversity of fire histories at different sites across Cape York Peninsula, highlighting the need for finer scale palaeofire research. Future fire management planning on Cape York Peninsula must take into account the thousands of years of active Indigenous management and this understanding can be further informed by paleoecological research.
The response of fire under changing climate conditions is an increasingly critical management issue in Australian environments and globally (Commonwealth Scientific and Industrial Research Organisation [CSIRO], 2009, 2021). Paleoecological studies have great potential to provide long-term baselines to better understand “linkages between climate, vegetation, fire regimes and humans” (Gillson et al., 2019, p.1) which is a critical research area worldwide (Bowman et al., 2011). This could improve modeling of future fire conditions under climate change, based on observations of conditions under past climatic shifts, and thereby inform present-day land management. However, the interpretation and application of paleoecological findings to modern and future landscape management is still in its infancy. While regional and global approaches to trends in fire activity are useful for understanding long-term trajectories in wildfire activity (Marlon et al., 2015), more local approaches are needed for studies of past fire to be interpretable in a way that is applicable to modern management issues (e.g., Carter et al., 2021). Delivering on the promise of paleoecology still requires experimentation in Australia. We examine Cape York Peninsula, Queensland, as an ideal location to begin investigating the possibilities of paleoecology within fire-prone tropical savanna environments.
The Cape York Peninsula bioregion covers approximately 122,565 square kilometers (Department of Agriculture, Water and the Environment, n.d.), making up around 6% of Australia’s tropical savanna region (Beringer et al., 2015). Despite ∼40% of the region being grazed (as of 2005; Department of Agriculture, Water and the Environment, 2008), Cape York Peninsula is known as a “pristine wilderness” area (Cape York Sustainable Futures, 2010 p. 13) that has been investigated for possible nomination to World Heritage status for both natural and cultural values (Department of Sustainability, Environment, Water, Population and Communities, 2012).
Fire has been identified as a management issue for the Cape York Peninsula region (Department of Agriculture, Water and the Environment, 2008), with a lack of fire leading to the declining health of heaths and an absence of active fire management leading to large, severe fires and thickening of woody vegetation (Department of National Parks, Sport and Racing, 2013). Fire frequency has also had observed biodiversity effects, such as varied effects on the composition of bird communities (Perry et al., 2011) and feral cat behaviors, with cats showing a preference for recent fire scars (McGregor et al., 2017). Carbon farming initiatives that seek to provide payment for enhanced ecosystem benefits, resulting from controlled early dry season burning, have recently begun in the region (Aboriginal Carbon Foundation, 2019; Queensland Government, 2021), although the relationship between this type of fire regime and possible co-benefits for diversity is potentially complicated (Perry et al., 2016).
Socially, fire management issues on Cape York Peninsula are complex, due to the interplay between the different priorities of pastoralists, Traditional Owners, other industries such as mining, and the Queensland Parks and Wildlife Service (Drucker et al., 2008; Perry et al., 2018; Department of Environment and Science, 2021). Additional factors include fire management policy (e.g., Ockwell, 2008) and general public attitudes, both in favor of, and against, anthropogenic burning (Ockwell and Rydin, 2006). Complexities are not limited to conflicting management priorities but also include differing timings of firing for different vegetation communities across the vast peninsula region; the five vegetation community types and their varied fire requirements identified by Steffensen (2020) in Awu-Laya country (the Laura region of southern Cape York Peninsula) alone demonstrate the need to understand past, present and future fire at finer scales than it is often studied. As Cape York Peninsula is a tropical savanna environment, modeling of fire into the future for this region is also complex, as estimates of future fire activity under warming conditions indicate potentially more fire, less fire, or negligible change (Williams et al., 2001; Pitman et al., 2007; Moritz et al., 2012).
Studies of past fire (palaeofire) can contribute to improving our understanding of both contemporary fire issues and future fire predictions by providing a long-term baseline for fire activity (Lynch et al., 2007). For Cape York Peninsula, this long-term record includes critical information on fire regimes under Indigenous land management prior to European arrival, data which are currently lacking (Crowley, 1995). Disentangling interactions between fire, climate and humans represents a key component of understanding this long fire history (Lynch et al., 2007). Additionally, understanding fire regime changes in the past and into the future under changing climate conditions requires the identification of fire characteristics, not simply the presence or absence of fire. Despite the low number of published palaeofire records in this vast region (5 on the mainland, 7 from offshore Torres Strait islands), Cape York Peninsula is still the best represented area within Australia’s savanna zone for palaeofire research (International Paleofire Network, 2021). The studies that exist primarily use a single fire proxy, microscopic charcoal (Rowe, 2005; Luly et al., 2006; Moss et al., 2015), or a combination of microscopic and macroscopic charcoal (Proske and Haberle, 2012; Stevenson et al., 2015). Previously published records have suggested that lake expansion and stabilization occurred in the region during the mid-Holocene (Luly et al., 2006) and burning increased at several sites in association with human activity (Stephens and Head, 1995; Rowe, 2005, 2007; Proske and Haberle, 2012; Lentfer et al., 2013); this will be described in detail in the Discussion.
This paper suggests that in order to meaningfully contribute to current and future fire problems, palaeofire research must address current limitations to improve the discipline’s provision of more nuanced knowledge for modern and Quaternary sciences (e.g., Woodward and Haines, 2020). For example, for Cape York Peninsula, few studies have tested the applicability of methodologies developed elsewhere to Australian savanna contexts (Rehn et al., 2021; Rehn et al., in press). Lynch et al. (2007) also identify shortcomings of the use of microscopic charcoal as a fire proxy due to interpretive issues, and the need to develop methodologies to better pinpoint human influences. This fits within a broader need for methods that can identify fire characteristics beyond the presence of more or less fire; this is a methodological challenge that can be addressed through a novel combination of existing techniques. Methods that measure the occurrence of fire or amount of biomass burned based on different fire products (charcoal counting and chemical isolation of pyrogenic carbon) can be used together with methods investigating fuel types (charcoal morphology and stable carbon isotopes) to create a more nuanced characterization of past fire.
This study addresses the methodological gap identified above through the application and critical assessment of techniques developed outside of Australian savanna ecosystems. This includes a combination of techniques to investigate factors such as fuel type and spatial differences in fire signal (local versus regional). It also applies the relative fire intensity estimation method presented in Rehn et al. (2021) to Cape York Peninsula to better understand this region’s characteristics of past fire beyond fire incidence, to better identify possible anthropogenic influences. The paper is part of a series of studies presenting new paleoecological records for northern Australia to fill the identified Quaternary knowledge gaps (Rowe et al., 2019, 2020; Rehn et al., 2021). The new data presented here represent a mid-Holocene fire record for Sanamere Lagoon, northern Cape York Peninsula (introduced in Rehn et al., in press), and a late Holocene fire record for Big Willum Swamp, Weipa (previously studied by Stevenson et al., 2015; Figure 1). This region is large but relatively contained (i.e., as a peninsula), is ecologically diverse and, valuably, incorporates a range of site types suitable for paleoecological study. Cape York Peninsula also has complex modern fire issues, both socially and environmentally.
Figure 1. Site locations for new records presented in this paper: (A) Australia with the extent of savanna vegetation indicated by shading (see Fox et al., 2001), showing the study area of Cape York Peninsula, (B) the study area with locations of new records marked with circles, existing record locations marked with diamonds, and the Cape York Peninsula bioregion is shaded in gray (after Department of Agriculture, Water and the Environment, 2021). For a detailed map of paleoenvironmental records across all of northern Australia see Reeves et al. (2013).
Cape York Peninsula forms the northernmost extension of the state of Queensland, Australia. The region is classified as equatorial/tropical savanna with a dry winter under the Köppen-Geiger climate classification (Kottek et al., 2006; Peel et al., 2007). Average annual rainfall across the region is 1450 mm, primarily falling from November to April (Bureau of Meteorology [BOM], 2019) driven by the Australian Monsoon and modulated on inter-annual timescales by the El Niño-Southern Oscillation (ENSO) (Bureau of Meteorology [BOM], 2008). Soils across Cape York Peninsula include 113 identified types, with a predominance of acidic and infertile soil types low in phosphorus (Biggs and Philip, 1995). The region is part of the greater savanna zone of northern Australia; for more detail on the distribution of vegetation types across the north into the Northern Territory and Western Australia, see Fox et al. (2001) and the Department of Environment and Water Resources (2007). The most widespread vegetation type is Eucalyptus woodlands (64% of total area), followed by Melaleuca woodlands (∼14%), and “miscellaneous communities” including mangroves and wetlands (∼7%) (Neldner and Clarkson, 1995). Fire frequency varies across the peninsula, with shorter fire return intervals in western and central regions than in the far north and the eastern coast (Figure 2; Northern Australian Fire Information, 2021), separated by the higher elevations of the most northerly section of the Great Dividing Range (Geoscience Australia, 2015). The western and eastern regions are also distinct in when biomass cures (dries and becomes flammable), with biomass fuels in the west curing earlier in the year than the east (Department of National Parks, Recreation, Sport and Racing, 2013). Parts of the west coastal region burned almost every year between 2000 and 2020, while areas along the east coast burned in five or less of the years in the same period (Northern Australian Fire Information, 2021). The study sites presented in this paper fall within both the broader regions of low fire frequency (Sanamere Lagoon) and high fire frequency (Big Willum Swamp).
Figure 2. Years burnt 2000–2020 for Cape York Peninsula (after Northern Australian Fire Information, 2021).
While the oldest published date for human occupation on Cape York Peninsula is approximately 35,000 BP, the majority of archeological sites and paleoenvironmental records in the region date to the Holocene (Haberle and David, 2004) including initial occupation of Torres Strait (∼8,000 BP; David et al., 2004) and offshore islands (∼4,000 cal BP for Lizard Island; Lambrides et al., 2020). For a detailed map of archeological sites and radiocarbon distributions refer to Williams et al. (2015).
Sanamere Lagoon (11.12°S, 142.35°E; 15 m a.s.l.) is a shallow water body covering approximately 1.5 × 2 km and is located ∼20 km south of Bamaga, northern Cape York Peninsula, Australia (Figure 3). The lagoon is part of the Jardine River Wetlands Aggregation and is classified as a sub-coastal wet heath swamp (Department of Environment and Science, 2018b). Maximum water depth recorded in April 2016 was 1.25 m, and most of the lagoon is perennially wet (from LANDSAT imagery 1970–2018; Department of the Prime Minister and Cabinet, 2019). Sanamere Lagoon lies in an enclosed basin surrounded by higher elevation land and has an approximate catchment area of 9 km2 (Geoscience Australia, 2015).
Figure 3. Satellite imagery and on-site photos of sites presented in this study; (a) Sanamere Lagoon satellite image (Google Earth, 2021b), (b) Sanamere Lagoon dwarf heathland vegetation, (c) open woodland vegetation 300 m from Sanamere Lagoon, (d) Big Willum Swamp satellite image (Google Earth, 2021a), (e) open woodland showing the wet season high water mark of Big Willum Swamp, (f) open woodland vegetation with grassy understorey beyond the Big Willum Swamp high waterline.
The site has a mean annual rainfall of ∼1,750 mm, measured from the nearest weather station on Horn Island ∼55 km north of Sanamere Lagoon, with > 90% occurring between December and April (Bureau of Meteorology [BOM], 2021a). Average daily temperatures range between 25.5 and 30.2°C (Bureau of Meteorology [BOM], 2021a. Wind direction is seasonally variable with predominantly north-northwesterly and southeasterly winds in January and July, respectively (Bureau of Meteorology [BOM], 2021a).
Sanamere Lagoon overlays Pleistocene sand with some silt and clay, bordered by deeply weathered Middle Jurassic to Early Cretaceous quartzose sandstone and micaceous carbonaceous siltstone (“Helby Beds”; Bureau of Mineral Resources, Geology and Geophysics, 1977). Deep bleached sands (Ca43: Uc2.21) and deep sandy mottled yellowish red earths (Mt9: Gn2.64) are dominant soils in the area (Northcote et al., 1960–1968).
Rehn et al. (in press) present a detailed description of vegetation at the site. Vegetation at Sanamere Lagoon can be divided into three main groups. The vegetation at the edge of the lagoon is closed sedgeland with scattered Pandanus and emergent sedges (Eleocharis and Schoenus) extending in bands into the water (Brass, 1953). This sedgeland transitions sharply to open heathland, the dominant vegetation type in the broader Sanamere Lagoon catchment. The heath forms a near treeless shrubland dominated by Neofabricia, Asteromytus, Baeckea, Jacksonia, Hibbertia, Thryptomene, Allocasuarina, and Grevillea (Neldner and Clarkson, 1995; Department of Environment and Science, 2019). Grasses are minimal, with ground cover consisting of clumped Schoenus sedges on wetter soil areas and drainage lines (Rehn et al., in press). The heathland vegetation group extends approximately 300 m from the waterline, transitioning into Eucalyptus woodland with an increase in trees and overall canopy height. The open woodland is dominated by Eucalyptus tetrodonta with Corymbia nesophila codominant (Neldner and Clarkson, 1995; Neldner et al., 2017). Smaller sub-canopy trees include Acacia species, Grevillea glauca, and Grevillea pteridifolia, along with Lomandra and Asteromyrtus species present as shrubs (Rehn et al., in press). Ground cover in this area is dominated by grasses (Poaceae), and leaf litter is present. The area immediately surrounding Sanamere Lagoon burned in 7 of the years from 2000 to 2020, with some areas burning every year at their most frequent (including 2018–2019 and 2019–2020; Northern Australian Fire Information, 2021).
Sanamere Lagoon lies within the Apudthama Land Trust Area (Apudthama Land Trust, 2018) and is immediately adjacent to the Jardine River National Park to the east, gazetted in 1977 (Queensland National Parks and Wildlife Service, 1996). The border of the national park follows the Old Telegraph Track, created in 1887 alongside the overland telegraph line (Horsfall and Morrison, 2010); the Old Telegraph Track was the only access point to the town of Bamaga to the north, established 1949–1952, until development of the Bamaga Road (Queensland Government, 2015). No dated archeological information has been published from the surrounding region.
Big Willum Swamp (12.657°S, 141.998°E; 30 m a.s.l.), also known as Willem Swamp or Waandriipayn (Stevenson et al., 2015), is located near Weipa, western Cape York Peninsula, Australia, within an active bauxite mine (see Figure 3). The swamp covers approximately 250 × 600 m and is classified as a palustrine system (Department of Environment and Science, 2018a). The Traditional Owners of the site are the Peppan people (Stevenson et al., 2015; Queensland Government, 2017). Maximum water depth recorded in July 2017 was 3.5 m, and the swamp is perennially wet (from LANDSAT imagery 1970–2018; Department of the Prime Minister and Cabinet, 2019). The swamp has a small catchment area of ∼0.75 km2 and is surrounded by terrain with limited (∼10 m) elevation variation (Geoscience Australia, 2015).
Big Willum Swamp has a mean annual rainfall of ∼1,790 mm, measured from the nearest weather station Weipa Eastern Ave ∼13 km away, with > 90% occurring between December and April (Bureau of Meteorology [BOM], 2021b). Average daily temperatures range between a mean minimum of 21.8°C and mean maximum of 32.3°C (Bureau of Meteorology [BOM], 2021b). Wind direction is multi-directional and low speed in January while predominantly southeasterly in July (Bureau of Meteorology [BOM], 2021b).
Big Willum Swamp is located on the Weipa Plateau, a Quaternary and Tertiary aluminous laterite including bauxite (Bureau of Mineral Resources, Geology and Geophysics, 1976). The swamp in its current state was formed by the collapse of underlying laterite karst (see Grimes and Spate, 2008). Bauxitic “deep loamy yellowish red earths” are dominant in the surrounding area (Northcote et al., 1960–1968).
The vegetation around Big Willum Swamp is open woodland, and this is also the principal habitat found across the broader Weipa Plateau (Cameron and Cogger, 1992). The site is surrounded by open Eucalyptus woodland with Eucalyptus tetrodonta, Corymbia nesophila codominant (Neldner et al., 2017). The sparse, poorly developed shrub layer contains Coelospermum and Persoonia and the “mid-dense” ground layer of grasses is dominated by Heteropogon, Sarga, and Alloteropsis (Cameron and Cogger, 1992; Neldner et al., 2017). The area surrounding the swamp burned in 6 to 12 of the years 2000–2020, with small patches burning every year at their most frequent, outside of the swamp’s immediate catchment area (Northern Australian Fire Information, 2021).
Extensive archeological sites have been recorded in the Weipa area, including the Wathayn cultural complex four kilometers south of Big Willum Swamp (Shiner et al., 2013). Archeological evidence suggests Indigenous occupation of the Weipa region by ∼2,700 cal BP with an increase in occupational intensity of site use after 1,000 cal BP (Shiner and Morrison, 2009; Morrison, 2014). A Presbyterian mission was established on the Embley River in 1898, named Weipa Mission the following year (Weipa Town Authority, 2014; Queensland Government, 2017). Bauxite mining at Weipa began in 1956, with construction of Weipa township beginning in 1964 (Weipa Town Authority, 2014). Mining leases in the Weipa area span 3,860 km2, including active mining areas and land undergoing rehabilitation (Rio Tinto, 2017).
The new fire records are presented here for Sanamere Lagoon (uppermost samples presented in Rehn et al., in press) and Big Willum Swamp (previously studied by Stevenson et al., 2015; Proske et al., 2017) were created using the following methods (presented in detail in Rehn et al., 2021). Sediment cores were collected during fieldwork in 2016–2017 using a D-section corer at Sanamere Lagoon (SAN1) and a raft-mounted hydraulic corer modified from Eijkelkamp equipment at Big Willum Swamp (BWIL2). Cores were continuously sampled at 0.5 cm intervals to a depth of 24 cm (SAN1) and 13 cm (BWIL2).
Lead-210 and carbon-14 sample preparation and analysis was undertaken at the Australian Nuclear Science and Technology Organisation (ANSTO). Lead-210 dating was conducted only on samples from BWIL2; sufficient sample volumes were not available from the uppermost depths of D-section core SAN1 for lead-210 dating. For details on lead-210 dating methodology see Rehn et al. (in press). Lead-210 dates were converted to calendar years BP (reported as years before 1950 CE) and were combined with radiocarbon dates to create a Bayesian age-depth model using the rBacon package in R (see R Development Core Team, 2013; Blaauw et al., 2019). This process included calibration of radiocarbon dates to cal BP using the Southern Hemisphere calibration curve SHCal20 (Hogg et al., 2020).
Sediment cores were scanned using an Itrax™ μXRF core scanner at ANSTO to provide sediment elemental composition as context for the fire record. Elemental analysis was conducted on core BWIL2 only, as D-section core SAN1 was subsampled in the field. The BWIL2 core was scanned at 1,000 μm intervals with 10 s exposure, using a Molybdenum X-ray tube. Elements of interest were selected from interpreted elements listed by Davies et al. (2015), such as detrital elements Ti, Al, and Fe, including the ratio of incoherent to coherent scatter (Mo ratio) interpreted as an organic signal. Elemental counts were normalized using the procedure described by Weltje et al. (2015), with counts divided by the incoherent scatter for that depth. Measurements included magnetic susceptibility, often associated with erosion and detritus (Davies et al., 2015). Principal components analysis on elemental data was completed using R.
Sample preparation for fossil charcoal particle counting was completed following the procedure described by Stevenson and Haberle (2005) and Rehn et al. (2021). Prepared samples at 0.5 cm intervals were separated into three size fractions by wet sieving: >250 μm, 250–125 μm, and 125–63 μm. Macroscopic charcoal (>125 μm) is broadly interpreted here as representing a local signal, while microscopic charcoal represents a more regional signal; it is not possible to ascribe specific distance sources to these sizes as debate regarding source areas is ongoing (Vachula et al., 2018). Charcoal particles in each size fraction were counted, and measured (maximum length and width) using an eyepiece scale via stereomicroscope. Charcoal particles with length-width ratios of 3.6 or greater were categorized as “elongate” and attributed to grassy fuels (Umbanhowar and McGrath, 1998; Rehn et al., in press).
Sample processing for the determination of pyrogenic carbon content and carbon isotope composition by hydrogen pyrolysis was completed for bulk sediments and the <63 μm fraction, retained from the preparation for charcoal analysis as described above (as per Wurster et al., 2012 and Rehn et al., 2021). Pyrogenic carbon δ13C values were corrected using the formula presented by Wurster et al. (2012), reported against the international V-PDB reference scale with an uncertainty of ± 0.1 ‰. Stable carbon isotope (δ13C) values of −24 ‰ or less are attributed to C3 plants while values of −15 ‰ or greater are attributed to C4 plants (grasses), with values between these end members indicative of mixed C3-C4 biomass contribution (O’Leary, 1988; Wurster et al., 2012; Saiz et al., 2018). Tropical grasses in northern Australia are predominantly C4, allowing for interpretation of the relationship between C3 and C4 values as the relationship between woody fuels and grasses (Saiz et al., 2015). Charcoal counts and pyrogenic carbon abundances were converted to rates of influx using recorded sample volume and mean sedimentation rate derived from the age-depth model. Z-scores were calculated for charcoal and pyrogenic carbon fluxes to determine relative fire intensity, as described in Rehn et al. (2021).
The Sanamere Lagoon record presented here spans the mid-Holocene, from approximately 8,150 to 6,600 cal BP (Supplementary Figure 1), noting sedimentation at the site is documented as having begun in the Pleistocene (Comley, 2017; Rivera Araya et al., 2020). Radiocarbon dates for core SAN1 are presented in the Supplementary Table 1. Charcoal and pyrogenic carbon fluxes peak early in the record, from 8,150 to 7,900 cal BP (Figure 4). High relative fire intensities span approximately 8,050–7,900 cal BP (Figure 5). After 7,900 cal BP, all fire proxies decline sharply and remain at lower levels for the rest of the record, with a minor increase in charcoal fluxes 6,750–6,670 cal BP. Elongate charcoal particles are present throughout the record, which would usually be interpreted as grass input. However, δ13C values are consistently low (<−24 ‰) and suggest input by C3 vegetation with negligible C4 contribution. The elongate charcoal particles in SAN1 are therefore interpreted as deriving from C3 sedges (present at the site today), rather than deriving from grasses which are predominantly C4 in northern Australia (Rehn et al., in press).
Figure 4. Stratigraphic plot of data from Sanamere Lagoon core SAN1, plotted by age. Proxies plotted are sediment moisture (percentage), charcoal fluxes by size fraction, charcoal elongate particle percentages, pyrogenic carbon fluxes by size fraction, pyrogenic carbon δ13C, and Z scores for total charcoal particles and bulk pyrogenic carbon.
Figure 5. Z scores of total charcoal particle influx (gray dashed line, triangle markers) and pyrogenic carbon influx (black line, circle markers) for Sanamere Lagoon core SAN1. High relative fire intensities (red bars) are identified by positive pyrogenic carbon Z scores and low to negative total charcoal Z scores.
The Big Willum Swamp record presented here spans the late Holocene, from approximately 3,900 cal BP to modern (Supplementary Figure 2). Lead-210 and radiocarbon dates for core BWIL2 are presented in the Supplementary Tables 2,3. Elements Al, K, Ca, Ti, Mn, and Fe were identified as detrital in the BWIL2 record by PCA, and are inversely related to organic deposition (Mo ratio) (Supplementary Figure 3). Magnetic susceptibility and all detrital elements decline around 1,700 cal BP. Deposition of organics (indicated by increased Mo inc/coh ratio), charcoal, and pyrogenic carbon increase after ∼1,700 cal BP and peak over the last approximately 600 years (Figure 6). Peak fire occurrence over the last century is considered to be at high relative fire intensities (Figure 7). Trends are comparable across all charcoal size fractions and low levels of macroscopic charcoal are present for most of the record. Elongate charcoal particles are present throughout the record, and δ13C values suggest consistent mixed C3-C4 contribution (−16 ‰ to −20 ‰) for bulk pyrogenic carbon, with −20 ‰ to −27 ‰ for < 63 μm pyrogenic carbon due to the Stable Isotope Disequilibrium Effect (Saiz et al., 2018).
Figure 6. Stratigraphic plot of data from Big Willum Swamp core BWIL2, plotted by age. Proxies plotted are Mo ratio (organic signal), charcoal fluxes by size fraction, charcoal elongate particle percentages, pyrogenic carbon fluxes by size fraction, pyrogenic carbon δ13C, and Z scores for total charcoal particles and bulk pyrogenic carbon.
Figure 7. Z scores of total charcoal particle influx (gray dashed line, triangle markers) and pyrogenic carbon influx (black line, circle markers) for Big Willum Swamp core BWIL2. High relative fire intensities (red bars) are identified by positive pyrogenic carbon Z scores and low to negative total charcoal Z scores.
The Sanamere Lagoon record presents high fire occurrence and relative fire intensity in the mid-Holocene, followed by a decline from 7,900 cal BP. Despite variations in fire occurrence and intensity, vegetation composition at Sanamere Lagoon appears consistently C3-dominated, comparable to the modern dwarf heathland at the site. The Big Willum Swamp record shows markedly increased fire occurrence and relative fire intensity in the historic period, and also shows consistent vegetation composition comparable to modern conditions (mixed trees and grass). The following sections will describe the current state of knowledge of past fire on Cape York Peninsula from existing records before outlining how the Sanamere Lagoon and Big Willum Swamp records contribute to and potentially change this understanding.
Regional syntheses and modeling have characterized the mid-Holocene as warm and wet across northern Australia due to suppression of ENSO and strengthening of the monsoon centered around 6,000 BP (Clement et al., 2000; Liu et al., 2004; McGregor and Gagan, 2004; Zheng et al., 2008; Koutavas and Joanides, 2012; Reeves et al., 2013). The onset of this wet phase appears to have occurred around 8,000 cal BP across Cape York Peninsula, with sedimentation beginning at Big Willum Swamp at this time (Stevenson et al., 2015). Also at this time, rainforest expansion also began at Three-Quarter Mile Lake on eastern Cape York Peninsula along with increasing charcoal, potentially due to the beginning or intensification of Indigenous fire management aimed at maintaining an open landscape in response to encroachment by woody vegetation (Luly et al., 2006; Figures 8, 9). Increased charcoal production and higher frequency burning is also recorded alongside a transition from grassland dominance to thickening woodland on Lizard Island offshore from 7,600 cal BP (Proske and Haberle, 2012). Permanent occupation of the Torres Strait islands also began at this time (David et al., 2004).
Figure 8. Previously published charcoal records from mainland Cape York Peninsula (adapted from Luly et al., 2006; Proske and Haberle, 2012; Lentfer et al., 2013; Stevenson et al., 2015) and the Sanamere Lagoon and Big Willum Swamp records from this study. Black dots represent microscopic charcoal, gray dots represent macroscopic charcoal and red dots represent bulk pyrogenic carbon. Dashed lines indicate interpretable changes in the Sanamere Lagoon and Big Willum Swamp records and show their approximate correspondence with previously published records, with question marks indicating uncertain correspondence where dates are unavailable. Note that scales vary between records and all are plotted relative to depth. Length of Y axis is approximately scaled to maximum age for visual comparison.
Figure 9. A selection of previously published charcoal records from Torres Strait (adapted from Rowe, 2007, 2015); refer to Figure 1 for location of Torres Strait north of Cape York Peninsula. Note that scales vary between records and all are plotted relative to depth. Length of Y axis is approximately scaled to maximum age for visual comparison.
Deepening of Big Willum Swamp between 7,000 and 5,000 cal BP is noted by Stevenson et al. (2015) and Proske et al. (2017) as possibly indicative of higher effective precipitation. A charcoal decline is noted on Lizard Island ∼5,800 cal BP associated with wetter conditions (Proske and Haberle, 2012). A shift from fluctuating brackish conditions to permanent freshwater occurred ∼5,000 cal BP at Three-Quarter Mile Lake attributed to higher effective precipitation, with a continued decline of dryland grasses and increasing woody dominance (Luly et al., 2006).
Mid- to high levels of burning were recorded around 3,800 cal BP on Lizard Island associated with the earliest evidence of human presence (Lentfer et al., 2013). Permanent reoccupation of the Torres Strait islands began shortly after this period (∼3,500–3,000 BP; David et al., 2004), accompanied by increased burning after this time (Rowe, 2005, 2007). Similarly, higher levels of burning and a transition from a shrubby to an increasingly grassy understorey were recorded in association with increased human occupation around Isabella Creek Swamp after 2,700 BP (Stephens and Head, 1995). A hydrological transition from fluctuating to permanent open water conditions occurred at 2,700 cal BP at two swamp sites in Torres Strait on Mua (BG2) and Badu (Bar20) Islands (Rowe, 2015). Increased levels of burning were again recorded at ∼1,500 cal BP on Lizard Island along with Cyclosorus ferns indicative of increased vegetation disturbance (Proske and Haberle, 2012).
Microscopic charcoal increased on Mua and Badu Islands in Torres Strait beginning 1000 cal BP (Rowe, 2015) and a microcharcoal peak was similarly recorded at Big Willum Swamp by Stevenson et al. (2015). Stevenson et al. (2015) noted wet conditions from 600 to 400 cal BP at Big Willum Swamp, overlapping with a period of increased site establishment around Albatross Bay 800–400 cal BP (Morrison, 2014). Higher levels of burning were recorded in Torres Strait from 700 to 500 cal BP with increasing human occupational intensity (Rowe, 2007), promoting an opening of woodlands and encouraging floral diversity (Rowe, 2015).
Climate has been established as wetter than modern in the mid-Holocene, with increased water permanence and woody thickening of savannas and rainforest expansion; coincident with this was increased burning attributed to human attempts to maintain open understoreys. This is seen after 8,000 cal BP and strengthening from 5,000 cal BP at Three-Quarter Mile Lake, and 7,600 cal BP at Lizard Island.
Sanamere Lagoon contributes a record of mid-Holocene fire in a region of northern Cape York Peninsula that currently burns less frequently than areas to the south and southwest. Peak charcoal and pyrogenic carbon fluxes occurred at the start of the SAN1 record from 8,150 to 7,900 cal BP, initially at high intensities, along with consistently low δ13C values for bulk pyrogenic carbon (Figures 4, 5). An abrupt decline in sedimentation rate as well as charcoal and pyrogenic carbon fluxes at Sanamere Lagoon at 7,900 cal BP suggests a potential expansion of the site under the increasingly wet conditions of the mid-Holocene. A peak in < 63 μm pyrogenic carbon at Sanamere Lagoon occurred at ∼7,800 cal BP corresponded to a decrease in δ13C value, potentially reflecting regional burning including of grassy fuels beyond the catchment of the site (due to the Stable Isotope Disequilibrium Effect; Saiz et al., 2015). Charcoal flux increased again at the lagoon from ∼6,800–6,000 cal BP, but at lower levels than those seen prior to 7,900 cal BP.
Climate in the late Holocene was drier and increasingly variable compared to the mid-Holocene, with varied timing of transitions from shrubby to more open and grassy vegetation. Humans also became a more significant ignition source through time. This is recorded on Lizard Island at 3,800 cal BP (more fire attributed to humans) and 1,500 cal BP (more fire and disturbance), Torres Strait after 3,000 cal BP (more fire and opening vegetation) as well as 1,000 cal BP (more fire attributed to humans, also seen regionally at Big Willum Swamp) and 500 cal BP, and Isabella Creek Swamp at 2,700 cal BP (more fire attributed to humans and more grass).
The Big Willum Swamp record presented in this study contributes a multiproxy late Holocene fire record from within a broader region that today burns very frequently, but within a small local area that currently burns less often than the surrounding plateau due to its location within a bauxite mine (Northern Australian Fire Information, 2021). The oldest modeled age for core BWIL2 is ∼3,920 cal BP, associated with low sedimentation rates and minimal charcoal and pyrogenic carbon. Mixed C3-C4 vegetation is indicated throughout the Big Willum Swamp record (Figure 6); this is comparable to modern open woodland at the site and this vegetation type is characteristic of the broader Weipa Plateau (Cameron and Cogger, 1992). Organic input (represented by Mo ratio) and sediment accumulation increased noticeably after ∼1,700 cal BP at Big Willum Swamp, after the site became a permanent deep water body around 2,200 cal BP (Stevenson et al., 2015). Earth mound building at nearby Wathayn (south of the swamp) had begun by this time as well as initiation of most shell mounds in this region (Brockwell et al., 2016; Holdaway et al., 2017). While increased human activity is associated with higher levels of burning elsewhere on Cape York Peninsula (Stephens and Head, 1995; Rowe, 2005, 2007; Proske and Haberle, 2012; Lentfer et al., 2013) this does not appear to occur at Big Willum Swamp.
Peak microscopic charcoal at the swamp at 1,000 cal BP recorded by Stevenson et al. (2015) appears as a minor charcoal peak in the BWIL2 record suggesting a regional increase in fire in this part of Cape York Peninsula. Stevenson et al. (2015) noted wet conditions from 600 to 400 cal BP at Big Willum Swamp, reflected in the BWIL2 record as increased charcoal and pyrogenic carbon fluxes from some time after 600 cal BP coincident with increased sedimentation rates. Fire proxies peak at Big Willum Swamp over the last 100 years, at high relative fire intensities (high pyrogenic carbon, see Figure 7), associated with the establishment of bauxite mining and the Weipa township and cessation of Indigenous burning.
The Big Willum Swamp fire record likely captures a regional fire signal representative of both the Big Willum Swamp catchment and beyond, due to the relatively homogenous vegetation composition across the Weipa Plateau. In contrast, the Sanamere Lagoon fire record appears to capture a local fire signal from within the catchment of the lagoon, with negligible input from the open woodland (mixed C3-C4) vegetation beyond the heathland (Rehn et al., in press). Human presence is difficult to investigate at Sanamere Lagoon due to the absence of documented archeological sites in the Jardine River region, while low charcoal and pyrogenic carbon fluxes for most of the record make identifying changes in relative fire intensity a challenge.
Several trends are apparent from existing palaeofire records on Cape York Peninsula (such as increasing fire occurrence coincident with increasing human occupation intensity). That not all of these trends are reflected within the Sanamere Lagoon and Big Willum Swamp records suggests long-term high spatial variability in fire regimes. Fire is an almost constant presence in most records, including Sanamere Lagoon and Big Willum Swamp. Increasingly wet conditions into the mid-Holocene are supported by the potential expansion of Sanamere Lagoon around 7,900 cal BP. Both sites presented here show relatively stable vegetation composition (C3 versus C4 contribution) in contrast to a trend toward thickening woody vegetation at other sites, particularly through the late Holocene. Similarly, a late Holocene regional trend to increased burning through time, particularly in association with human activity, is not apparent at Big Willum Swamp, despite a well-documented archeological record for the surrounding region. It is possible that the lack of vegetation thickening and lack of increased burning are related at the swamp, with vegetation composition not requiring a change in fire regime to maintain openness, or an unknown external driver influencing both features at other sites but not present at Big Willum.
A dramatic change is apparent in the most recent part of the Big Willum Swamp record, with a large increase in charcoal and pyrogenic carbon fluxes suggesting increased biomass burning at high relative intensities. Despite this major transition in fire regime, vegetation composition has remained stable. While the initiation date of this change is not chronologically well constrained (occurring somewhere between 600 cal BP and 75 cal BP), the new fire regime is sustained through the historical period and most likely represents a change in land management associated with European settlement and accompanying cessation of Indigenous fire management. The absence of any discernible changes in fire regime during earlier phases of presumed intensification of human occupation near Big Willum Swamp makes the recent transition particularly noteworthy.
Insights into modern and future fire issues on Cape York Peninsula can be found among the palaeofire records from the region. While biomass burning has been generally increasing at many sites on Cape York Peninsula during the late Holocene (Stephens and Head, 1995; Proske and Haberle, 2012; Rowe, 2015), recent peaks in fire incidence and intensity at Big Willum Swamp with European settlement exceed anything seen in the last 3,000 years at the site. Increased burning is accompanied at several sites by woody thickening, with this burning interpreted as human attempts to maintain landscape openness; this suggests that understoreys may close in some regions if active fire management is not maintained particularly as atmospheric CO2 continues to rise (Lehmann et al., 2009; cf. Prior et al., 2020). Future fire planning on Cape York Peninsula must acknowledge and account for diversity in fire regimes and thousands of years of active Indigenous fire management shaping the ecosystems found in the region today, despite modern conceptions of Cape York Peninsula as “pristine wilderness” separated from human influence (World Wildlife Fund, 2021).
While the findings of this study are inherently local, as any nuanced understanding of fire for management purposes should be, the multiproxy methodological approach presented in this study may be applied to savanna ecosystems, and potentially other fire-prone ecosystems, outside of Australia. Multiproxy approaches such as that presented by Aleman et al. (2013) have demonstrated the power of these combined techniques to African savannas, and this could be further strengthened with the addition of fire intensity estimation as shown in this study.
Studies of past fire incidence and intensity provide critical information about interactions between fire, vegetation, climate, and people over the longer time scales needed to inform current and future fire management planning. The fire records presented here have helped address spatial and methodological gaps in understanding the Holocene fire history of Australian tropical savannas. The mid-Holocene Sanamere Lagoon record shows high relative fire intensities 8,100–7,900 cal BP followed by a potential expansion of the water body at 7,900 cal BP, and recurring fire in a C3-dominated local environment comparable to the modern dwarf heathland at the site. The late Holocene Big Willum Swamp record shows a minor increase in burning and deposition rates after 1,700 cal BP with no major impact on intensity or vegetation composition. Anthropogenic influence appears most starkly as a sharp increase in burning, at high relative intensities, over the last century associated with European settlement.
Interpreted in the context of existing published palaeofire records for Cape York Peninsula, these records provide the following insights for fire management planning: (1) humans have managed fire in the region for thousands of years and contributed to maintaining landscape openness through burning, (2) a general trend toward vegetation thickening appears across the region over the last ∼4,000 years, and (3) the biomass burning and high fire intensities at Big Willum Swamp since European settlement are unprecedented. Future rehabilitation of the landscape surrounding Big Willum Swamp after mining activities cease will require active fire management, as it has been managed for thousands of years.
These records demonstrate the interpretive power of a multiproxy approach to fire reconstruction and how records of past fire can contribute to modern fire management issues. They also demonstrate the diversity between sites in Australian tropical savannas even in relatively close proximity and therefore the importance of studying more sites in the savanna zone as no one site may be considered representative of the broader region. This fits within broader issues of scale in paleoecological research, and the need for finer spatial scale studies for relevance to modern fire management challenges.
The datasets presented in this study can be found in online repositories. The names of the repository/repositories and accession number(s) can be found below: ER 2019, Ph.D. Dataset: Fire and Environmental Change in Northern Australian Savannas during the Holocene. James Cook University (dataset). http://doi.org/10.25903/5de5fle48e86d.
ER, CR, SU, and MB conceived the research and interpreted the data. ER, CR, and MB undertook fieldwork. ER, PG, AZ, GJ, and CW conducted the laboratory work. ER and CR drafted the manuscript. All authors contributed to the final manuscript.
This project was undertaken with the support of an Australian Research Council Laureate Fellowship to MB (FL140100044) and the Australian Research Council Centres of Excellence for Australian Biodiversity and Heritage (CE170100015). ER acknowledges financial support from the Australian Institute of Nuclear Science and Engineering (Postgraduate Research Award 12143).
The authors declare that the research was conducted in the absence of any commercial or financial relationships that could be construed as a potential conflict of interest.
All claims expressed in this article are solely those of the authors and do not necessarily represent those of their affiliated organizations, or those of the publisher, the editors and the reviewers. Any product that may be evaluated in this article, or claim that may be made by its manufacturer, is not guaranteed or endorsed by the publisher.
We acknowledge the Peppan Traditional Owners of the lands and waters of the Big Willum Swamp region, with thanks to Rio Tinto. We acknowledge the Traditional Owners of the lands and waters of the Sanamere Lagoon region, with many thanks to Charles Woosop and the Apudthama Land Trust. We thank Jennifer Whan, Rainy Comley, Michael Brand, Chris Wurster, and Costjin Zwart for assistance during fieldwork and laboratory analyses at James Cook University, and Sabika Maizma for laboratory assistance at the Centre for Accelerator Science, ANSTO. We acknowledge the financial support from the Australian Government for the Centre for Accelerator Science at ANSTO through the National Collaborative Research Infrastructure Strategy (NCRIS).
The Supplementary Material for this article can be found online at: https://www.frontiersin.org/articles/10.3389/fevo.2021.771700/full#supplementary-material
Aboriginal Carbon Foundation (2019). Carbon Farming on the Cape, Aboriginal Carbon Foundation. Available online at: https://www.abcfoundation.org.au/carbon-farming-on-the-cape (accessed May 26, 2021).
Aleman, J. C., Blarquez, O., Bentaleb, I., Bonté, P., Brossier, B., Carcaillet, C., et al. (2013). Tracking land-cover changes with sedimentary charcoal in the Afrotropics. Holocene 23, 1853–1862. doi: 10.1177/0959683613508159
Beringer, J., Hutley, L. B., Abramson, D., Arndt, S. K., Briggs, P., Bristow, M., et al. (2015). Fire in Australian savannas: from leaf to landscape. Glob. Change Biol. 21, 62–81.
Biggs, A. J. W., and Philip, S. R. (1995). Soils of Cape York Peninsula. Land Resources Bulletin, Queensland Government Technical Report. Brisbane, QLD: Queensland Government.
Blaauw, M., Andres Christen, J., Esuivel Vazquez, J., Belding, T., Theiler, J., Gough, B., et al. (2019). Package ‘rbacon’. Available online at: https://cran.r-project.org/web/packages/rbacon/rbacon.pdf (accessed June 1, 2018).
Bowman, D. M. J. S., Balch, J., Artaxo, P., Bond, W. J., Cochrane, M. A., D’Antonio, C. M., et al. (2011). The human dimension of fire regimes on Earth. J. Biogeogr. 38, 2223–2236. doi: 10.1111/j.1365-2699.2011.02595.x
Brass, L. J. (1953). Results of the Archbold Expeditions No. 68: Summary of the 1948 Cape York (Australia) Expedition – With Notes on the Mammals of Cape York Peninsula by G.H.H. Tate, Vol. 102. New York, NY: Bulletin of the American Museum of Natural History.
Brockwell, S., Foghlú, B. Ó., Fenner, J. N., Stevenson, J., Proske, U., and Shiner, J. (2016). New dates for earth mounds at Weipa, north Queensland, Australia, Archaeol. Oceania 52, 127–134. doi: 10.1002/arco.5118
Bureau of Meteorology [BOM] (2008). The Australian Monsoon. Australian Government. Available online at: http://www.bom.gov.au/climate/about/?bookmark=monsoon (accessed February 18, 2021).
Bureau of Meteorology [BOM] (2019). Regional Weather and Climate Guide: Cape York Peninsula. Australian Government. Available online at: http://www.bom.gov.au/climate/climate-guides/guides/025-Cape-York-QLD-Climate-Guide.pdf (accessed February 4, 2021).
Bureau of Meteorology [BOM] (2021a). Monthly Climate Statistics: Horn Island. Australian Government. Available online at: http://www.bom.gov.au/climate/averages/tables/cw_027058.shtml (accessed April 30, 2021).
Bureau of Meteorology [BOM] (2021b). Monthly Climate Statistics: Weipa Eastern Ave. Available online at: http://www.bom.gov.au/climate/averages/tables/cw_027042.shtml (accessed April 30, 2021).
Bureau of Mineral Resources, Geology and Geophysics (1976). Weipa Sheet SD 54-3, Map. Canberra, ACT: Australian Government.
Bureau of Mineral Resources, Geology and Geophysics (1977). Jardine River Sheet SC 54-15, Map. Canberra, ACT: Australian Government.
Cameron, E. E., and Cogger, H. G. (1992). The Herpetofauna of the Weipa Region, Cape York Peninsula, Technical Reports of the Australian Museum, Vol. 7. Sydney, NSW: The Australian Museum, 1–200. doi: 10.3853/j.1031-8062.7.1992.72
Cape York Sustainable Futures (2010). New Horizons and Opportunities: Cape York Peninsula Investment Prospectus. Brisbane, QLD: Cape York Sustainable Futures and Queensland Government Department of Employment, Economic Development and Innovation.
Carter, V. A., Brunelle, A., Power, M. J., DeRose, R. J., Bekker, M. F., Hart, I., et al. (2021). Legacies of Indigenous land use shaped past wildfire regimes in the Basin-Plateau Region, USA. Commun. Earth Environ. 2:72. doi: 10.1038/s43247-021-00137-3
Clement, A. C., Seager, R., and Cane, M. A. (2000). Suppression of El Niño during the mid-Holocene by changes in the Earth’s orbit. Paleoceanography 15, 731–737. doi: 10.1029/1999PA000466
Comley, R. (2017). The Fire History of Northern Cape York Peninsula: Evidence from the Pyrogenic Carbon Record of Sanamere Lagoon. B.Sc.(Hons) thesis. Cairns, QLD: James Cook University.
Commonwealth Scientific and Industrial Research Organisation [CSIRO] (2009). Bushfires in Australia. Commonwealth Scientific and Industrial Research Organisation. Available online at: https://www.aph.gov.au/DocumentStore.ashx?id=3d4e5dd5-9374-48e9-b3f4-4e6e96da27f5 (accessed November 28, 2018).
Commonwealth Scientific and Industrial Research Organisation [CSIRO] (2021). The 2019-20 Bushfires: A CSIRO Explainer. Commonwealth Scientific and Industrial Research Organisation. Available online at: https://www.csiro.au/en/research/natural-disasters/bushfires/2019-20-bushfires-explainer (accessed May 26, 2021).
Crowley, G. M. (1995). Fire on Cape York Peninsula. Brisbane, QLD: Cape York Peninsula Land Use Strategy Program, Office of the Coordinator General of Queensland.
David, B., McNiven, I., Mitchell, R., Orr, M., Haberle, S., Brady, L., et al. (2004). Badu 15 and the Papuan-Austronesian settlement of Torres Strait. Archaeol. Ocean. 39, 65–78. doi: 10.1002/j.1834-4453.2004.tb00564.x
Davies, S. J., Lamb, H. F., and Roberts, S. J. (2015). “Micro-XRF core scanning palaeolimnology: recent developments,” in Micro-XRF Studies of Sediment Cores: Applications of a Non-Destructive Tool for the Environmental Sciences, Developments in Paleoenvironmental Research, Vol. 17, eds I. W. Croudace and R. G. Rothwell (New Yorkm, NY: Springer).
Department of Agriculture, Water and the Environment (2008). Rangelands 2008 – Taking the Pulse: Cape York Peninsula Bioregion. Canberra ACT: Australian Government.
Department of Agriculture, Water and the Environment (2021). Interim Biogeographic Regionalisation for Australia, Version 7. Australian Government. Available online at: https://www.environment.gov.au/system/files/pages/5b3d2d31-2355-4b60-820c-e370572b2520/files/ibra-regions.pdf (accessed September 16, 2020)
Department of Agriculture, Water and the Environment (n.d.). Australia’s Bioregions (IBRA). Australian Government. Available online at: https://www.environment.gov.au/land/nrs/science/ibra (accessed September 16, 2020).
Department of Environment and Science (2018b). WetlandInfo: Wetland Systems. Queensland Government. Available online at: https://wetlandinfo.des.qld.gov.au/wetlands/what-are-wetlands/definitions-classification/system-definitions.html (accessed 3 December 3, 2018).
Department of Environment and Science (2018a). WetlandInfo. Queensland Government. Available online at: https://wetlandinfo.des.qld.gov.au/wetlands/ (accessed March 7, 2018).
Department of Environment and Science (2019). Jardine River National Park, Heathlands Resources Reserve and Jardine River Resources Reserve: Nature, Culture and History. Queensland Government. Available online at: https://parks.des.qld.gov.au/parks/jardine-river/culture.html (accessed November 21, 2019).
Department of Environment and Science (2021). Cape York Peninsula Parks. Queensland Government. Available online at: https://parks.des.qld.gov.au/parks/cape-york/about (accessed May 26, 2021).
Department of Environment and Water Resources (2007). Australia’s Native Vegetation. Canberra, ACT: Australian Government.
Department of National Parks, Recreation, Sport and Racing (2013). Planned Burn Guidelines: Cape York Peninsula Bioregion of Queensland. Brisbane, QLD: Queensland Government.
Department of National Parks, Sport and Racing (2013). Jardine-Heathlands Aggregation Management Statement 2013. Brisbane, QLD: Queensland Government.
Department of Sustainability, Environment, Water, Population and Communities (2012). A World Heritage Nomination for Cape York Peninsula. Australian Government. Available online at: https://www.environment.gov.au/heritage/places/world-heritage-nomination-cape-york-peninsula (accessed May 26, 2021).
Department of the Prime Minister and Cabinet (2019). NationalMap. Australian Government. Available online at: https://nationalmap.gov.au/ (accessed March 1, 2019).
Drucker, A. G., Garnett, S. T., Luckert, M. K., Crowley, G. M., and Gobius, N. (2008). Manager-based valuations of alternative fire management regimes on Cape York Peninsula, Australia. Int. J. Wildl. Fire 17, 660–673. doi: 10.1071/WF07102
Fox, I. D., Neldner, V. J., Wilson, G. W., and Bannink, P. J. (2001). The Vegetation of the Australian Tropical Savannas. Brisbane, QLD: Queensland Government.
Gillson, L., Whitlock, C., and Humphrey, G. (2019). Resilience and fire management in the Anthropocene. Ecol. Soc. 24:14. doi: 10.5751/ES-11022-240314
Google Earth (2021a). Big Willum Swamp, Weipa, Queensland, 12°39’22”S, 141°59’54”E, Elevation 31m. Available online at: http://www.google.com/earth/index.html (accessed March 3, 2021).
Google Earth (2021b). Sanamere Lagoon, Cape York Peninsula, Queensland, 11°07’19’S, 142°21’32”E Elevation 16m. Available online at: http://www.google.com/earth/index.html (accessed March 3, 2021).
Haberle, S. G., and David, B. (2004). Climates of change: human dimensions of Holocene environmental change in low latitudes of the PEPII transect. Quat. Int. 118–119, 165–179. doi: 10.1016/S1040-6182(03)00136-8
Hogg, A. G., Heaton, T. J., Hua, Q., Palmer, J. G., Turney, C. S. M., Southon, J., et al. (2020). SHCal20 Southern Hemisphere calibration, 0–55,000 years cal BP. Radiocarbon 62, 759–778. doi: 10.1017/RDC.2020.59
Holdaway, S. J., Fanning, P. C., Petchey, F., Allely, K., Shiner, K. I., and Bailey, G. (2017). Temporal variability in shell mound formation at Albatross Bay, northern Australia, PLoS One 12:e0183863. doi: 10.1371/journal.pone.0183863
Horsfall, N., and Morrison, M. (2010). Cape York Peninsula Cultural Story: Non-Indigenous and Shared History. Canberra, ACT: Australian Government.
International Paleofire Network (2021). Global Paleofire Database. International Paleofire Network. Available online at: https://paleofire.org/index.php (accessed April 30, 2021).
Kottek, M., Grieser, J., Beck, C., Rudolf, B., and Rubel, F. (2006). World map of the Köppen-Geiger climate classification updated. Meteorol. Z. 15, 259–263.
Koutavas, A., and Joanides, S. (2012). El Niño-Southern oscillation extrema in the Holocene and Last Glacial Maximum. Paleoceanography 27:A4208. doi: 10.1029/2012PA002378
Lambrides, A. B. J., McNiven, I. J., Aird, S. J., Lowe, K. A., Moss, P., Rowe, C., et al. (2020). Changing use of Lizard Island over the past 4000 years and implications for understanding Indigenous offshore island use on the Great Barrier Reef. Qld. Archaeol. Res. 23, 43–109. doi: 10.25120/qar.23.2020.3778
Lehmann, C. E. R., Prior, L. D., and Bowman, D. M. J. S. (2009). Decadal dynamics of tree cover in an Australian tropical savanna. Aust. Ecol. 34, 601–612. doi: 10.1111/j.1442-9993.2009.01964.x
Lentfer, C. J., Felgate, M. W., Mills, R. A., and Specht, J. (2013). Human history and palaeoenvironmental change at Site 17, Freshwater Beach, Lizard Island, northeast Queensland, Australia. Qld. Archaeol. Res. 16, 141–164. doi: 10.25120/qar.16.2013.227
Liu, Z., Harrison, S. P., Kutzbach, J., and Otto-Bliesner, B. (2004). Global monsoons in the mid-Holocene and oceanic feedback. Clim. Dyn. 22, 157–182. doi: 10.1007/s00382-003-0372-y
Luly, J. G., Grindrod, J. F., and Penny, D. (2006). Holocene palaeoenvironments and change at Three-Quarter Mile Lake, Silver Plains Station, Cape York Peninsula, Australia. Holocene 16, 1085–1094. doi: 10.1177/0959683606069398
Lynch, A. H., Beringer, J., Kershaw, P., Marshall, A., Mooney, S., Tapper, N., et al. (2007). Using the paleorecord to evaluate climate and fire interactions in Australia. Annu. Rev. Earth Planet. Sci. 35, 215–239. doi: 10.1146/annurev.earth.35.092006.145055
Marlon, J. R., Kelly, R., Daniau, A.-L., Vannière, B., Power, M. J., Bartlein, P., et al. (2015). Reconstructions of biomass burning from sediment charcoal records to improve data-model comparisons. Biogeosci. Discuss. 12, 18571–18623. doi: 10.5194/bgd-12-18571-2015
McGregor, H. V., and Gagan, M. K. (2004). Western Pacific coral δ18O records of anomalous Holocene variability in the El Niño-Southern Oscillation. Geophys. Res. Lett. 31:L11204. doi: 10.1029/2004GL019972
McGregor, H. W., Cliff, B., and Kanowski, J. (2017). Habitat preference for fire scars by feral cats in Cape York Peninsula, Australia. Wildl. Res. 43, 623–633. doi: 10.1071/WR16058
Moritz, M. A., Parisien, M.-A., Batllori, E., Krawchuk, M. A., Van Dorn, J., Ganz, D. J., et al. (2012). Climate change and disruptions to global fire activity. Ecosphere 3:49. doi: 10.1890/ES11-00345.1
Morrison, M. (2014). Chronological trends in late Holocene shell mound construction across northern Australia: insights from Albatross Bay, Cape York Peninsula. Aust. Archaeol. 79, 1–13. doi: 10.1080/03122417.2014.11682014
Moss, P., Mackenzie, L., Ulm, S., Sloss, C., Rosendahl, D., Petherick, L., et al. (2015). Environmental context for late Holocene human occupation of the South Wellesley Archipelago, Gulf of Carpentaria, northern Australia. Quat. Int. 385, 136–144. doi: 10.1016/j.quaint.2015.02.051
Neldner, V. J., and Clarkson, J. R. (1995). Vegetation Survey and Mapping of Cape York Peninsula. Brisbane, QLD: Queensland Department of Environment and Heritage.
Neldner, V. J., Niehus, R. E., Wilson, B. A., McDonald, W. J. F., Ford, A. J., and Accad, A. (2017). The Vegetation of Queensland: Descriptions of Broad Vegetation Groups. Brisbane, QLD: Department of Science, Information Technology and Innovation.
Northcote, K. H., Bechmann, G. G., Bettenay, E., Churchward, H. M., Van Dijk, D. C., Dimmock, G. M., et al. (1960–1968). Atlas of Australian Soils, Sheets 1 to 10 With Explanatory Data. Melbourne, VIC: Commonwealth Scientific and Industrial Research Organisation and Melbourne University Press.
Northern Australian Fire Information (2021). Fire History – North Australia and Rangelands Fire Information. Darwin, NT: Charles Darwin University and the Department of the Environment and Energy.
Ockwell, D., and Rydin, Y. (2006). Conflicting discourses of knowledge: understanding the policy adoption of pro-burning knowledge claims in Cape York Peninsula, Australia. Environ. Polit. 15, 379–398. doi: 10.1080/09644010600627659
Ockwell, D. G. (2008). Opening up’ policy to reflexive appraisal: a role for Q methodology? A case study of fire management in Cape York, Australia. Policy Sci. 41, 263–292. doi: 10.1007/s11077-008-9066-y
O’Leary, M. H. (1988). Carbon isotopes in photosynthesis. Bioscience 38, 328–336. doi: 10.2307/1310735
Peel, M. C., Finlayson, B. L., and McMahon, T. A. (2007). Updated world map of the Köppen-Geiger climate classification. Hydrol. Earth Syst. Sci. Discuss. 4, 439–473.
Perry, J. J., Kutt, A. S., Garnett, S. T., Crowley, G. M., Vanderduys, E. P., and Perkins, G. C. (2011). Changes in the avifauna of Cape York Peninsula over a period of 9 years: the relative effects of fire, vegetation type and climate. Emu Aust. Ornithol. 111, 120–131. doi: 10.1071/MU10009
Perry, J. J., Sinclair, M., Wikmunea, H., Wolmby, S., Martin, D., and Martin, B. (2018). The divergence of traditional Aboriginal and contemporary fire management practices on Wik traditional lands, Cape York Peninsula, Northern Australia. Ecol. Manage. Restor. 19, 24–31. doi: 10.1111/emr.12301
Perry, J. J., Vanderduys, E. P., and Kutt, A. S. (2016). Shifting fire regimes from late to early dry-season fires to abate greenhouse emissions does not completely equate with terrestrial vertebrate biodiversity co-benefits on Cape York Peninsula, Australia. Int. J. Wildl. Fire 25, 742–752. doi: 10.1071/WF15133
Pitman, A. J., Narisma, G. T., and McAneney, J. (2007). The impact of climate change on the risk of forest and grassland fires in Australia. Clim. Change 84, 383–401. doi: 10.1007/s10584-007-9243-6
Prior, L. D., Whiteside, T. G., Williamson, G. J., Bartolo, R. E., and Bowman, D. M. J. S. (2020). Multi-decadal stability of woody cover in a mesic eucalypt savanna in the Australian monsoon tropics. Aust. Ecol. 45, 621–635. doi: 10.111/aec.12877
Proske, U., and Haberle, S. G. (2012). Island ecosystem and biodiversity dynamics in northeastern Australia during the Holocene: unravelling short-term impacts and long-term drivers. Holocene 22, 1097–1111. doi: 10.1177/0959683612441840
Proske, U., Stevenson, J., Seddon, A. W. R., and Taffs, K. (2017). Holocene diatom records of wetland development near Weipa, Cape York, Australia. Quat. Int. 440, 42–54. doi: 10.1016/j.quaint.2016.09.014
Queensland Government (2015). Bamaga. Queensland Government. Available online at: https://www.qld.gov.au/atsi/cultural-awareness-heritage-arts/community-histories-bamaga (accessed March 1, 2018).
Queensland Government (2017). Napranum. Queensland Government. Available online at: https://www.qld.gov.au/atsi/cultural-awareness-heritage-arts/community-histories/community-histories-n-p/community-histories-napranum (accessed April 22, 2021).
Queensland Government (2021). The Land Restoration Fund: Pilot Projects. Queensland Government. Available online at: https://www.qld.gov.au/environment/climate/climate-change/land-restoration-fund/funded-projects/pilot-projects (accessed May 26, 2021).
Queensland National Parks and Wildlife Service (1996). Visitor Information: Jardine River National Park. Cairns, QLD: Information brochure, Queensland Department of Environment.
R Development Core Team (2013). R: A Language and Environment for Statistical Computing. Vienna: R Foundation for Statistical Computing.
Reeves, J. M., Bostock, H. C., Ayliffe, L. K., Barrows, T. T., De Deckker, P., Devriendt, L. S., et al. (2013). Palaeoenvironmental change in tropical Australasia over the last 30,000 years – a synthesis by the OZ-INTIMATE group. Quat. Sci. Rev. 74, 97–114. doi: 10.1016/j.quascirev.2012.11.027
Rehn, E., Rowe, C., Ulm, S., Woodward, C., and Bird, M. (2021). A late-Holocene multiproxy fire record from a tropical savanna, eastern Arnhem Land, Northern Territory, Australia. Holocene 31, 870–883. doi: 10.1177/0959683620988030
Rehn, E., Rowe, C., Ulm, S., Woodward, C., Zawadzki, A., Jacobsen, G., et al. (in press). Integrating charcoal morphology and stable carbon isotope analysis to identify non-grass elongate charcoal in tropical savannas. Veg. Hist. Archaeobot. doi: 10.1007/s00334-021-00836-z
Rivera Araya, M., Bird, M., Rowe, C., Ulm, S., and Levchenko, V. (2020). “The reliability of radiocarbon dates of different carbon fractions in the Australian tropical savannas: a case study from Sanamere Lagoon, northeast Australia,” in Proceedings of the 22nd EGU General Assembly 4-8 May. Available online at: https://ui.adsabs.harvard.edu/abs/2020EGUGA.2211983R/abstract (accessed April 1, 2021).
Rowe, C. (2005). A Holocene History of Vegetation Change in the Western Torres Strait Region, Queensland, Australia. Ph.D. thesis. Clayton, VIC: Monash University.
Rowe, C. (2007). A palynological investigation of Holocene vegetation change in Torres Strait, seasonal tropics of northern Australia. Palaeogeogr. Palaeoclimatol. Palaeoecol. 251, 83–103. doi: 10.1016/j.palaeo.2007.02.019
Rowe, C. (2015). Late Holocene swamp transition in the Torres Strait, northern tropical Australia. Quat. Int. 385, 56–68. doi: 10.1016/j.quaint.2014.07.002
Rowe, C., Brand, M., Hutley, L. B., Wurster, C., Zwart, C., Levchenko, V., et al. (2019). Holocene savanna dynamics in the seasonal tropics of northern Australia. Rev. Palaeobot. Palynol. 267, 17–31. doi: 10.1016/j.revpalbo.2019.05.004
Rowe, C., Wurster, C. M., Zwart, C., Brand, M., Hutley, L. B., Levchenko, V., et al. (2020). Vegetation over the last glacial maximum at Girraween Lagoon, monsoonal northern Australia. Quat. Res. 102, 39–52. doi: 10.1017/qua.2020.50
Saiz, G., Goodrick, I., Wurster, C., Nelson, P. N., Wynn, J., and Bird, M. (2018). Preferential production and transport of grass-derived pyrogenic carbon in NE-Australian savanna ecosystems. Front. Earth Sci. 5:115. doi: 10.3389/feart.2017.00115
Saiz, G., Wynn, J. G., Wurster, C. M., Goodrick, I., Nelson, P. N., and Bird, M. I. (2015). Pyrogenic carbon from tropical savanna burning: production and stable isotope composition. Biogeosciences 12, 1849–1863. doi: 10.5194/bg-12-1849-2015
Shiner, J., and Morrison, M. (2009). The contribution of heritage surveys towards understanding the cultural landscape of the Weipa Bauxite Plateau. Aust. Archaeol. 68, 52–55. doi: 10.1080/03122417.2009.11681890
Shiner, J. S., Fanning, P. C., Holdaway, S. J., Petchey, F., Beresford, C., Hoffman, E., et al. (2013). Shell mounds as the basis for understanding human-environment interaction in far north Queensland, Australia. Qld. Archaeol. Res. 16, 65–91. doi: 10.25120/qar.16.2013.224
Steffensen, V. (2020). Fire Country: How Indigenous Fire Management Could Help Save Australia. Richmond, VIC: Hardie Grant Explore.
Stephens, K., and Head, L. (1995). “Palaeoecology of archaeological and swamp sites in SE Cape York Peninsula,” in Quinkan Prehistory: The Archaeology of Aboriginal Art in Southeast Cape York Peninsula, Australia, Tempus, Vol. 3, eds M. J. Morwood and D. R. Hobbs (St. Lucia, QLD: University of Queensland), 18–32.
Stevenson, J., Brockwell, S., Rowe, C., Proske, U., and Shiner, J. (2015). The palaeoenvironmental history of Big Willum Swamp, Weipa: an environmental context for the archaeological record. Aust. Archaeol. 80, 17–31. doi: 10.1080/03122417.2015.11682041
Stevenson, J., and Haberle, S. (2005). Macro Charcoal Analysis: A Modified Technique Used by the Department of Archaeology and Natural History, Palaeoworks Technical Papers 5. Canberra, ACT: Australian National University.
Umbanhowar, C. E., and McGrath, M. J. (1998). Experimental production and analysis of microscopic charcoal from wood, leaves and grasses. Holocene 8, 341–346. doi: 10.1191/095968398666496051
Vachula, R. S., Russell, J. M., Huang, Y., and Richter, N. (2018). Assessing the spatial fidelity of sedimentary charcoal size fractions as fire history proxies with a high-resolution sediment record and historical data. Palaeogeogr. Palaeoclimatol. Palaeoecol. 508, 166–175. doi: 10.1016/j.palaeo.2018.07.032
Weipa Town Authority (2014). Western Cape History. Weipa Town Authority. Available online at: http://www.weipatownauthority.com.au/western-cape-history (accessed July 18, 2018)
Weltje, G. J., Bloemsma, M. R., Tjallingii, R., Heslop, D., Röhl, U., and Croudace, I. W. (2015). “Prediction of geochemical composition from XRF core scanner data: a new multivariate approach including automatic selection of calibration samples and quantification uncertainties,” in Micro-XRF Studies of Sediment Cores: Applications of a Non-Destructive Tool for the Environmental Sciences, Developments in Paleoenvironmental Research, Vol. 17, eds I. W. Croudace and R. G. Rothwell (New York, NY: Springer).
Williams, A. A. J., Karoly, D. J., and Tapper, N. (2001). The sensitivity of Australian fire danger to climate change. Clim. Change 49, 171–191. doi: 10.1023/A:1010706116176
Williams, A. N., Veth, P., Steffen, W., Ulm, S., Turney, C. S. M., Reeves, J. M., et al. (2015). A continental narrative: human settlement patterns and Australian climate change over the last 35,000 years. Quat. Sci. Rev. 123, 91–112. doi: 10.1016/j.quascirev.2015.06.018
Woodward, C., and Haines, H. A. (2020). Unprecedented long-distance transport of macroscopic charcoal from a large, intense forest fire in eastern Australia: implications for fire history reconstruction. Holocene 30, 947–952. doi: 10.1177/0959683620908664
World Wildlife Fund (2021). Cape York Peninsula in Northeastern Australia. World Wildlife Fund. Available online at: https://www.worldwildlife.org/ecoregions/aa0703 (accessed May 26, 2021).
Wurster, C. M., Lloyd, J., Goodrick, I., Saiz, G., and Bird, M. I. (2012). Quantifying the abundance and stable isotope composition of pyrogenic carbon using hydrogen pyrolysis. Rapid Commun. Mass Spectrom. 26, 2690–2696. doi: 10.1002/rcm.6397
Keywords: tropical savannas, charcoal, pyrogenic carbon (PyC), relative fire intensity, holocene, northern Australia, Cape York Peninsula
Citation: Rehn E, Rowe C, Ulm S, Gadd P, Zawadzki A, Jacobsen G, Woodward C and Bird M (2021) Multiproxy Holocene Fire Records From the Tropical Savannas of Northern Cape York Peninsula, Queensland, Australia. Front. Ecol. Evol. 9:771700. doi: 10.3389/fevo.2021.771700
Received: 06 September 2021; Accepted: 01 November 2021;
Published: 25 November 2021.
Edited by:
Pierre Grondin, Ministère des Forêts, de la Faune et des Parcs, CanadaReviewed by:
Triin Reitalu, Tallinn University of Technology, EstoniaCopyright © 2021 Rehn, Rowe, Ulm, Gadd, Zawadzki, Jacobsen, Woodward and Bird. This is an open-access article distributed under the terms of the Creative Commons Attribution License (CC BY). The use, distribution or reproduction in other forums is permitted, provided the original author(s) and the copyright owner(s) are credited and that the original publication in this journal is cited, in accordance with accepted academic practice. No use, distribution or reproduction is permitted which does not comply with these terms.
*Correspondence: Emma Rehn, ZW1tYS5yZWhuQG15LmpjdS5lZHUuYXU=
Disclaimer: All claims expressed in this article are solely those of the authors and do not necessarily represent those of their affiliated organizations, or those of the publisher, the editors and the reviewers. Any product that may be evaluated in this article or claim that may be made by its manufacturer is not guaranteed or endorsed by the publisher.
Research integrity at Frontiers
Learn more about the work of our research integrity team to safeguard the quality of each article we publish.