- 1State Key Laboratory of Biocontrol and Guangdong Provincial Key Laboratory of Plant Resources, School of Life Sciences, Sun Yat-sen University, Guangzhou, China
- 2Geological Institute, Russian Academy of Sciences, Moscow, Russia
- 3Borissiak Paleontological Institute, Russian Academy of Sciences, Moscow, Russia
- 4School of Ecology, Sun Yat-sen University, Guangzhou, China
- 5State Key Laboratory of Palaeobiology and Stratigraphy, Nanjing Institute of Geology and Palaeontology, Chinese Academy of Sciences, Nanjing, China
A first occurrence of the genus Meliosma (Sabiaceae) is reported from the upper Eocene of the Maoming Basin of South China. This fossil is one of the oldest reliable records of the genus within its modern center of diversity. Fossil leaves are assigned to a new species, Meliosma eosinica sp. nov. based on leaf morphology and epidermal characters. The leaf epidermal anatomy of fossil Meliosma is illustrated for the first time. We also provide the first SEM observation of pollen grains associated with Meliosma. This study also documents an occurrence of mites within the leaf domatia previously unknown from the fossil record. We presume that the studied mite belongs to the superfamily Eupodoidea (Arthropoda), and probably the family Eupodidae, which comprises very small soft-bodied cosmopolitan mites occupying a wide range of terrestrial habitats. Additionally, we analyze the damage types on the fossil leaves of Meliosma. They exhibit exclusively external foliage feeding damage caused by arthropods and traces of probable fungal infection. A review of currently known fossil occurrences of leaves, fruits, and wood of Meliosma provides evidence for the geological and geographical distribution of the genus.
Introduction
Sabiaceae are a small family of trees, shrubs, and lianas comprising four genera: Meliosma Blume, Kingsboroughia Liebm., Sabia Colebr., and Ophiocaryon Endl. (Yang et al., 2018). The family is distributed in tropical and subtropical regions of eastern and south eastern Asia and Central and South America. The most diverse and widely distributed genus in the family is Meliosma (Figure 1). The number of species within Meliosma is uncertain and between different publications varies considerably from 25 species (Van Beusekom, 1971) to 80 species (Stevens, 2017) or even 124 species (World Checklist of Selected Plant Families, 2021). In China, 29 species are currently known (Law and Wu, 1982; Guo and Brach, 2007) and over 70 species are estimated for the Neotropical region (Cornejo, 2008, 2009; Ramos and Cornejo, 2012).
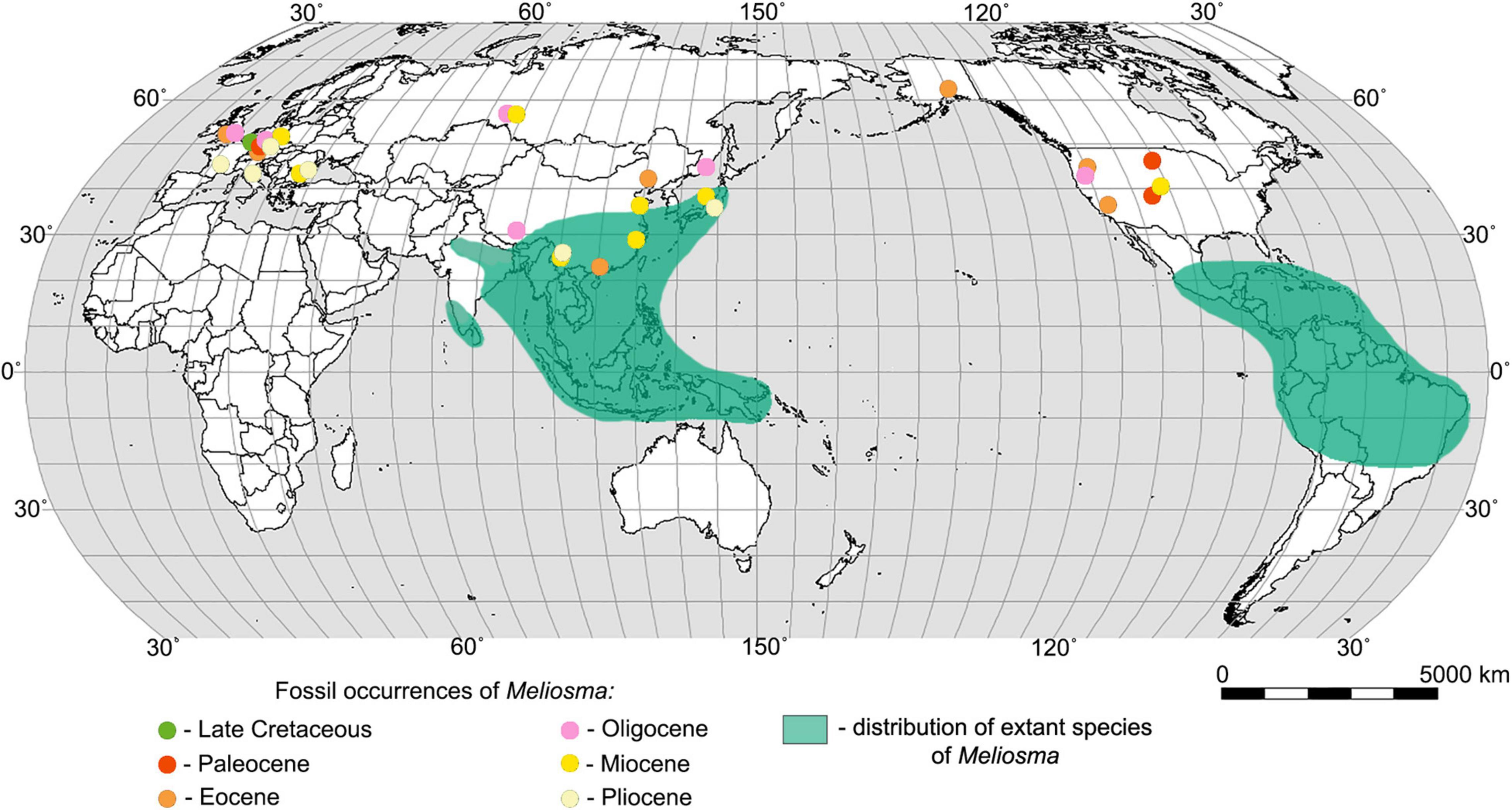
Figure 1. World map showing the distribution of extant species of Meliosma (according to Van Beusekom, 1971) and the main fossil sites of Meliosma.
The genus Meliosma, which in the Paleogene and Neogene was quite widespread in Europe, Asia, and America, now has a disjunct distribution with centers of diversity in southeast Asia (southern China, Myanmar, Thailand, Vietnam, Indonesia), Central America, and in northwest South America (Figure 1). Based on phylogenetic and biogeographic studies of Sabiaceae, including the consideration of the fossil record, it is presumed that Meliosma originated in Asia, subsequently migrated to Europe and then through the North Atlantic Land Bridge (Raven and Axelrod, 1974; Zúñiga, 2014) or the Bering Land Bridge (Van Beusekom, 1971) moved to America. Molecular dating and ancestral area reconstruction suggest a Eurasian origin of Sabiaceae in the Late Cretaceous followed by a boreotropical range expansion during the Paleogene (Yang et al., 2018).
The family Sabiaceae has an uncertain position in modern plant systematics. Earlier classifications placed it in the Sapindales (Bentham and Hooker, 1862; Takhtajan, 1966, 1987; Dahlgren, 1980), in the Berberidales (Cronquist, 1957), or the Ranunculales, close to Menispermaceae (Cronquist, 1981). Later, Takhtajan (1997) distinguished a separate order, Sabiales. The position of the family relative to orders Proteales and Buxales, or core eudicots has been debated in many publications (Hilu et al., 2003; Soltis et al., 2003; Furness et al., 2007; Wanntorp and Ronse de Craene, 2007; Worberg et al., 2007; Ronse de Craene and Wanntorp, 2008; Zúñiga, 2015; Yang et al., 2018). According to recent phylogenetic studies based on molecular data, the family is placed in Proteales (Angiosperm Phylogeny Group, 2016; Stevens, 2017; Yang et al., 2018). This placement received moderate (74%) bootstrap support, and is corroborated by various alternative hypothesis tests (Yang et al., 2018).
The most comprehensive research on living and fossil representatives of the genus Meliosma is the revision of Van Beusekom (1971). He proposed an infrageneric classification of Meliosma based primarily on endocarp morphology. The genus was subdivided into subgenera Kingsboroughia and Meliosma, with the sections Hendersonia and Kingsboroughia, and Meliosma and Lorenzanea, respectively. In his monograph, the extant species of Meliosma (with the exception of Neotropical sect. Lorenzanea) were taxonomically revised and all known fossil species of the genus were evaluated.
The earliest records assigned to the genus Meliosma (represented by endocarps) so far reported come from the Maastrichtian of Europe (Knobloch and Mai, 1986). To date, about 15 reliable species of fossil fruits (endocarps) of the genus, as well as 4 species of fossil wood, have been described (see Supplementary Table 1; Supplementary Figure 1). Publications with detailed descriptions and illustrations of Meliosma fossil leaves are quite rare. To date, more than 18 species of fossil leaves attributed to the genus have been reported from the Paleocene to the Pliocene (e.g., Van Beusekom, 1971; Hickey, 1977; Wolfe, 1977; WGCPC (Writing Group of Cenozoic Plants of China), 1978; Manchester, 1981; Peppe and Hickey, 2014). However, the generic assignment of these fossils is often questionable and based on fragmentary material. Van Beusekom (1971, p. 384) remarked that “in Meliosma the leaf-types are generally not very characteristic. Especially when they have an entire margin, they may easily be confused with leaves of other taxa. In fact, it is only the obovate-oblanceolate leaf with dentate margins, such as is found in several subspecies of Meliosma simplicifolia (Roxb.) Walp. which, with the help of its rather characteristic nervation pattern, may be referred with reasonable certainty to Meliosma. Indeed, it is this type of leaf which predominantly figures in paleobotanical literature under the name of Meliosma.”
Here, we report well-preserved leaves from the upper Eocene of South China confidently assigned to the genus Meliosma based on a detailed comparison of leaf morphology and epidermal characters with those of extant representatives of the genus. Fossil leaves from the Maoming Basin are described as a new species. The leaf epidermal features of fossil Meliosma are depicted for the first time. We examine fossil pollen grains found on the leaf of Meliosma and compare them with pollen grains of several extant species of this genus using SEM. One of the purposes of this study is to identify the mite found in the fossil leaf domatium and evaluate its possible adaptive relationship. In addition, we document the fossil leaf damage types.
Materials and Methods
Ten specimens studied here were collected by J. Jin (Sun Yat-sen University, Guangzhou, China) and his colleagues and students during the 2010s from the Huangniuling Formation of the Maoming Basin, located in southwest Guangdong Province, South China (Figure 2). The leaf fossils preserved as impressions/compressions were recovered from a lens of mudstones in the upper part of the formation together with hundreds of other plant fossils. The Huangniuling flora is highly diverse and has yielded more than 150 species of angiosperms and rare ferns and gymnosperms (e.g., Spicer et al., 2017). The Huangniuling Formation is considered to be late Eocene in age based on paleomagnetic and palynological data (Wang et al., 1994; Aleksandrova et al., 2015). The specimens are housed in the Museum of Biology, Sun Yat-sen University, Guangzhou, China with collection numbers beginning MMJ3.
The morphological study of both fossil and modern leaves was performed using a dissecting microscope (Carl Zeiss Stemi 2000 C) equipped with a Canon G10 Power Shot digital camera. The leaf specimens were photographed under low-angle incident light using a Panasonic GX7 digital camera with Leica DG Macro-Elmarit 1:2.8/45 mm macro lens. Fossil leaf drawings were made by tracing over photographs using a Wacom Intuos3 tablet. For the leaf descriptions, we used a terminology devised by Ellis et al. (2009). The types of insect damage on the fossil leaves were identified following the “Guide to Insect (and Other) damage types on compressed plant fossils” (Labandeira et al., 2007).
The fossil leaves of Meliosma studied here almost lack cuticles except for small fragments from the midrib and leaf domatia. These fragments were macerated using Schulze solution (70% HNO3 and saturated KClO3 with a volume ratio of 3:1) and ammonium hydroxide and then mounted on glass slides and SEM stubs. We examined the material with a light microscope (LM) Zeiss Axiostar plus at the Geological Institute, Russian Academy of Sciences (GIN RAS), and with an SEM (Tescan Vega) at the Borissiak Paleontological Institute, Russian Academy of Sciences (PIN RAS).
A cluster of dispersed pollen grains similar in morphology to those of extant species of Meliosma was found on the fossil leaf (specimen MMJ3-837-3a). Two mites (one of them was later lost) were obtained from the leaf domatium during maceration. Pollen grains and fragments of mite cuticle were mounted on an SEM stub, sputtered with gold, and observed with a Tescan, at accelerating voltage 20 kV.
We examined the leaves and pollen of the extant species of Meliosma using specimens available in the Herbarium of Sun Yat-sen University (SYS), Guangzhou, China, as well as specimens collected by S.R. Manchester (Florida Museum of Natural History, Gainesville, United States) in the Xishuangbanna Tropical Botanical Garden (XTBG) and kindly donated to us. The cuticles from small fragments of extant leaves were prepared by immersing them in 70% nitric acid, followed by treatment with ammonium hydroxide for complete removal of the leaf mesophyll. The leaf cuticles and parts of anthers of extant Meliosma species were mounted on an SEM stub with nail varnish, sputtered with gold and palladium, and observed using SEM, at accelerating voltage 20 kV, at PIN RAS.
Results
Order PROTEALES Juss. ex Bercht. et J. Presl, 1820
Family SABIACEAE Blume, 1851
Genus Meliosma Blume, 1823
Species Meliosma eosinica Moiseeva, Kodrul et Jin (Figures 3A–H, 4A–F, 5A–H, 6A,D,F,H, 7A–E, 8A–C, 9A–E,G,H).
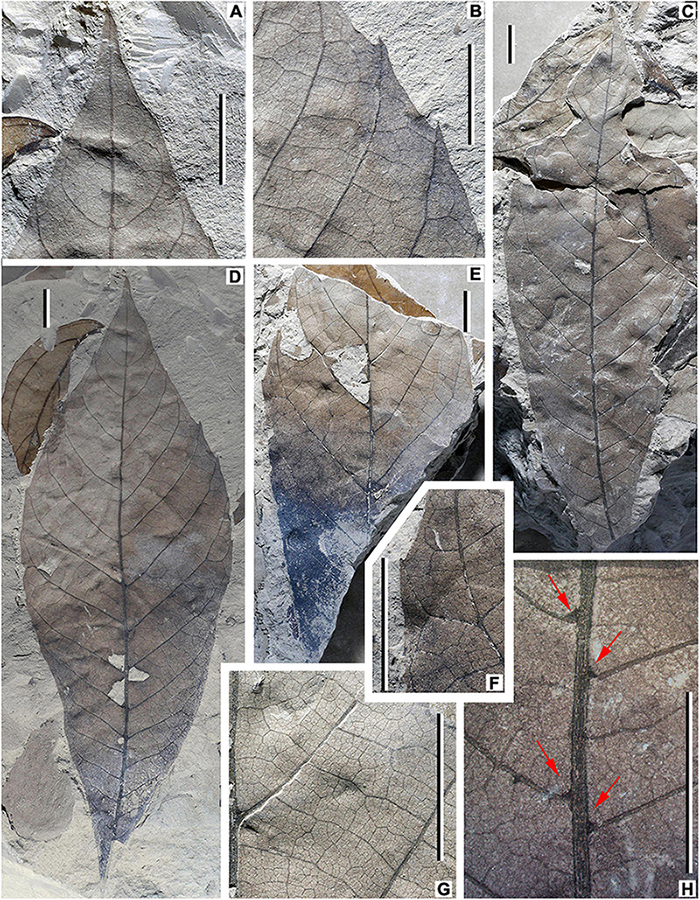
Figure 3. Fossil leaves of Meliosma eosinica sp. nov. from the Eocene Huangniuling Formation of the Maoming Basin, South China. (A,B,D) Holotype, MMJ3-008-1a. Note insect leaf-feeding damage in (D) small circular hole (damage type DT2) and a cluster of three holes located at the divergence point of secondary veins from primary veins (DT57) with a narrow reaction rim. (C) Untoothed leaf, MMJ3-2175-1a. Note polylobate insect feeding holes (DT3) with weakly developed reaction tissue. (E) Incompletely preserved leaf showing insect marginal feeding (DT15), hole feeding (DT5), and surface feeding (DT31) traces, MMJ3-837-3a. (F,G) Enlargement of leaf in (E). (H) Enlargement of leaf in (C) showing domatia (arrows). Scale bars = 1 cm.
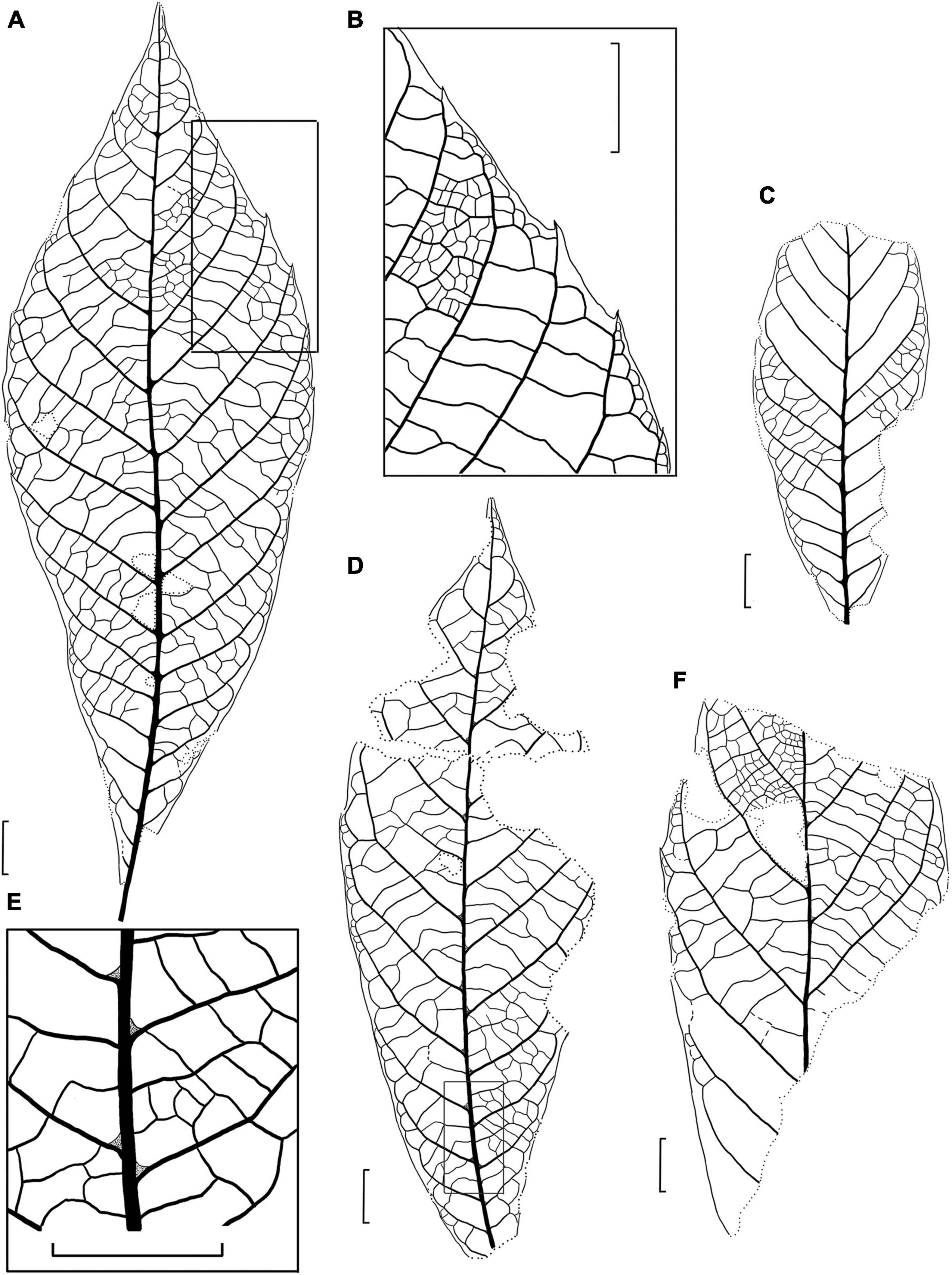
Figure 4. Drawings of the fossil leaves of M. eosinica sp. nov. from the Huangniuling Formation of the Maoming Basin, South China. (A) Holotype, MMJ3-008-1a. (B) Higher magnifications of the venation near the leaf margin in (A). (C) Leaf with untoothed margin, MMJ3-2207-2. (D) Leaf with untoothed margin, MMJ3-2175-1a. (E) Higher magnifications of the leaf domatia and venation near midvein in (D). (F) Incomplete leaf, MMJ3-837-3a. Scale bars = 1 cm.
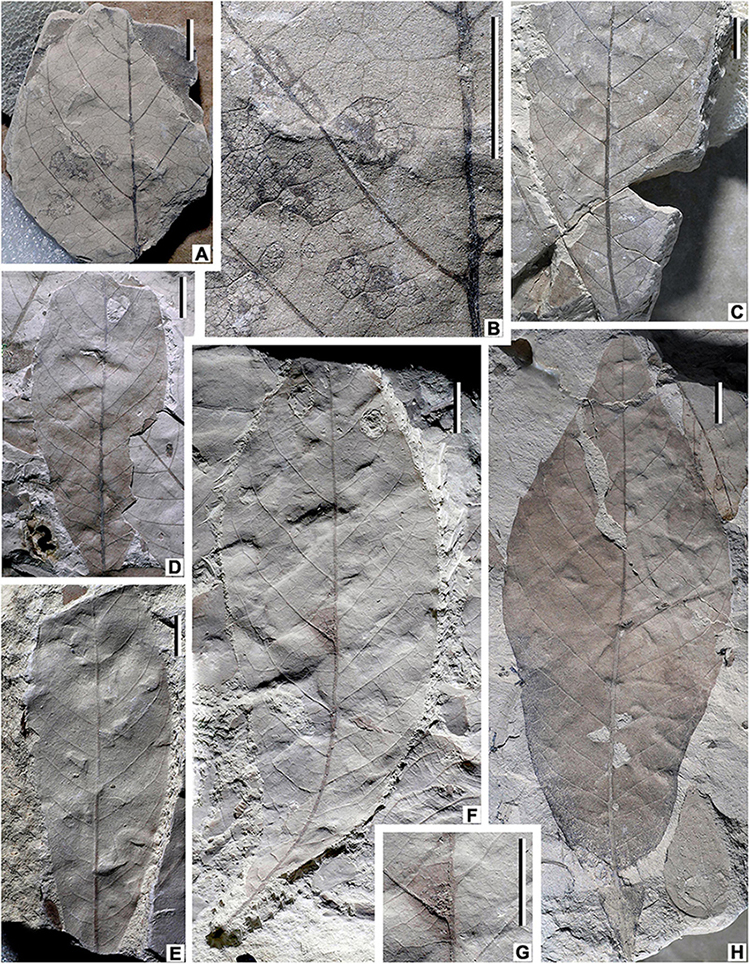
Figure 5. Fossil leaves of M. eosinica sp. nov. from the Eocene Huangniuling Formation of the Maoming Basin, South China. (A) Incomplete leaf showing skeletonization insect damage (DT17), MMJ3-321. (B) Enlargement of leaf in (A), polygonal skeletonized patches with reaction tissue bounded by tertiary veins. (C) Lower part of the leaf, MMJ3-549-1. (D) Untoothed leaf with large polylobate perforation (DT5) caused by an insect, MMJ3-2207-2. (E) Untoothed leaf, MMJ3-2205-1. (F) Leaf with small teeth in the upper third of lamina, MMJ3-2206-2. Note circular holes (DT2, DT4) caused by insects and probable fungal damage in the domatium zone. (G) Enlargement of domatium zone in (F). (H) Holotype (counterpart), MMJ3-008-1b. Scale bars = 1 cm.
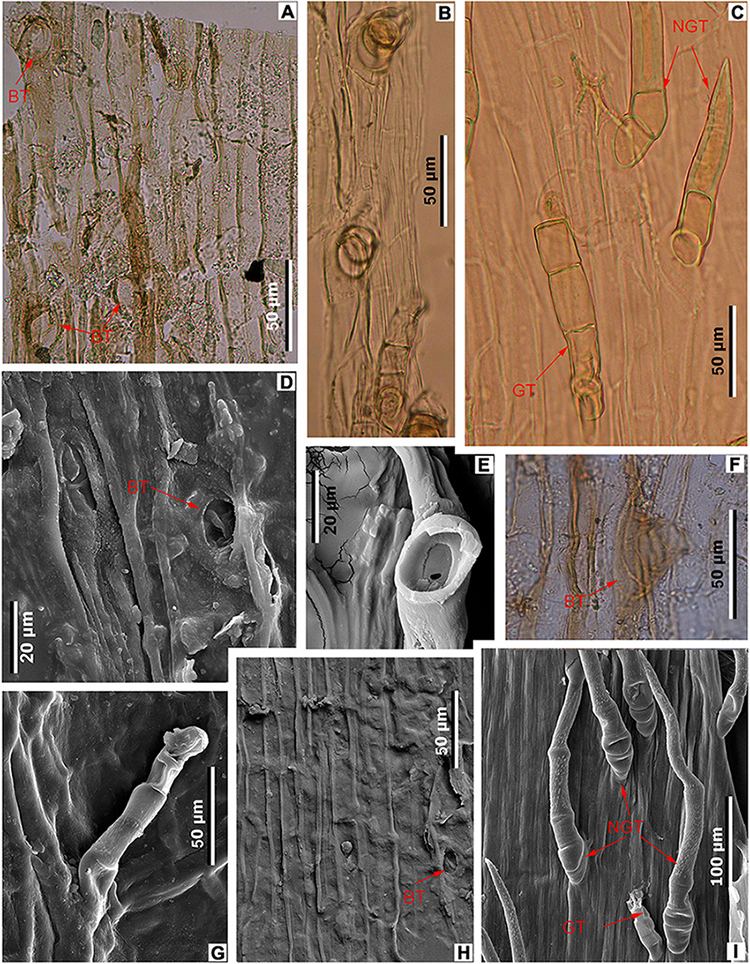
Figure 6. Midvein epidermal characters in fossil and extant Meliosma leaves. M. eosinica sp. nov., holotype, MMJ3-008-1a (A,D,F,H). (A) Trichome bases (BT), light microscopy (LM). (D) Trichome base, SEM. (F) Glandular(?) trichome base, LM. (H) Ordinary cells of the costal area and trichome base, internal view, SEM. M. rigida Siebold et Zucc., SYS00085214 (B,E). (B) Trichomes, LM. (E) Base of trichome, external view, SEM. M. simplicifolia (Roxb.) Walp., Xishuangbanna Tropical Botanical Garden (XTBG) (C,G,I). (C) Glandular (GT) and non-glandular (NGT) trichomes, LM. (G) Glandular trichome (GT), external view, SEM. (I) Glandular (GT) and non-glandular trichomes (NGT), external view, SEM.
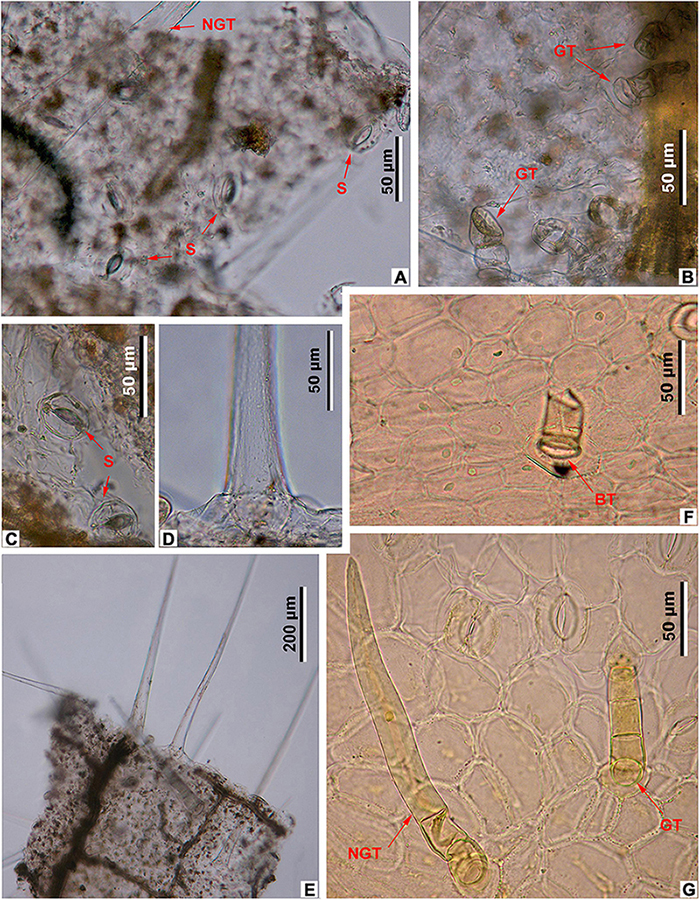
Figure 7. Leaf epidermal characters of fossil and extant Meliosma, LM. M. eosinica sp. nov., MMJ3-837-3a (A–E). (A) Abaxial surface with stomata (S) and non-glandular trichomes (NGT). (B) Adaxial surface with glandular trichomes (GT). (C) Abaxial surface with stomata (S). (D) The base of unicellular non-glandular trichome (NGT). (E) Long unicellular trichomes. Extant M. simplicifolia (Roxb.) Walp., XTBG (F,G). (F) Adaxial surface with the base of glandular trichome (BT). (G) Abaxial surface with stomata, multicellular glandular (GT), and non-glandular (NGT) trichomes.
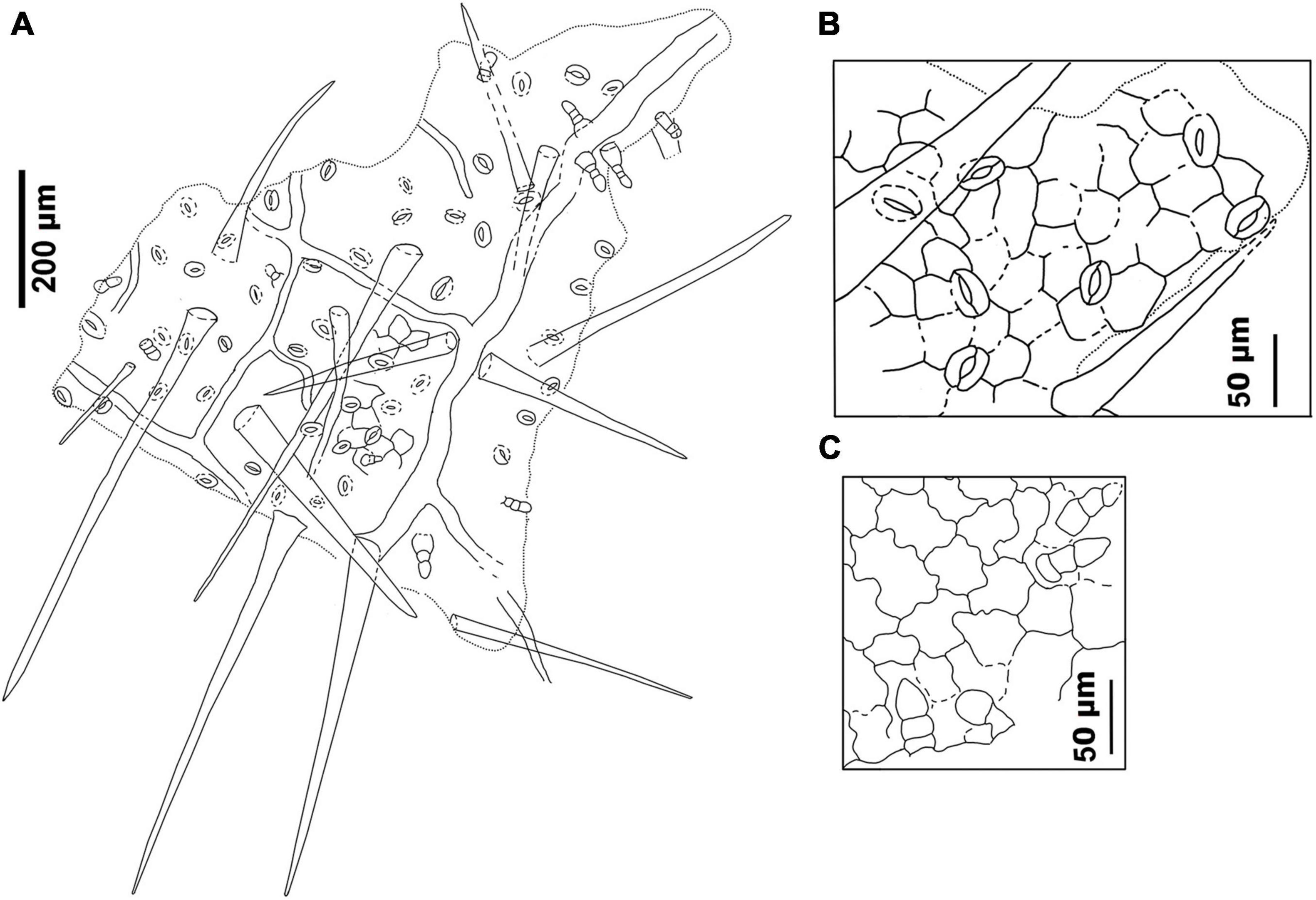
Figure 8. Drawings of the leaf epidermal characters of M. eosinica sp. nov. from the Huangniuling Formation of the Maoming Basin, South China, MMJ3-837-3a. (A) Leaf abaxial surface showing stomata, long unicellular non-glandular trichomes, and multicellular glandular trichomes. (B) Higher magnifications of the abaxial surface. (C) Leaf adaxial surface showing glandular trichomes.
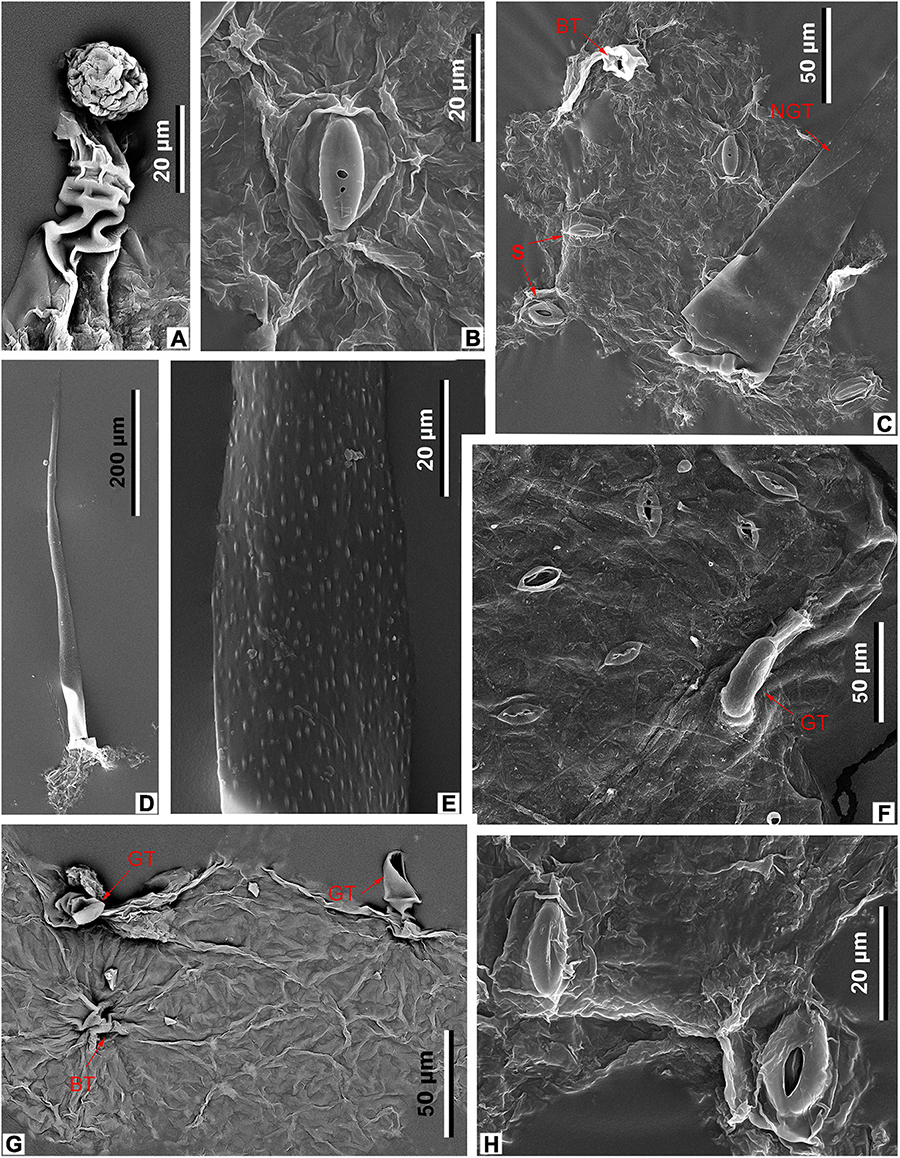
Figure 9. Leaf epidermal characters of M. eosinica sp. nov., MMJ3-837-3a (A–E,G,H), and extant M. simplicifolia (Roxb.) Walp., SYS00085373 (F), SEM. (A) Glandular trichome. (B) Stoma. (C) Abaxial surface with stomata (S), unicellular non-glandular trichome (NGT), and base of glandular(?) trichome (BT), external view. (D) Abaxial surface with long unicellular non-glandular trichome. (E) The surface of non-glandular trichome (NGT). (F) Abaxial surface with stomata (S) and glandular trichome (GT), external view. (G) Adaxial surface with glandular trichomes (GT), internal view. (H) Abaxial surface with two stomata, external view.
Holotype
Leaf, specimen no. MMJ3-008-1a (part) and MMJ3-008-1b (counterpart), designated here (Figures 3A,B,D, 4A,B, 5H, 6A,D,F,H).
Repository
The Museum of Biology of the Sun Yat-sen University, Guangzhou, China.
Type Locality
Maoming Basin, Guangdong Province, China.
Stratigraphic Horizon
The Huangniuling Formation, upper Eocene.
Etymology
From Eocene and Sina (lat.) – China.
Diagnosis
Leaves are simple, petiolate, laminar size mesophyll, length to width ratio about 3:1. Lamina is obovate, slightly asymmetrical at the base. Laminar apex angle acute with straight or acuminate apex shape, base angle acute with cuneate shape. Primary venation pinnate. The surface of the primary vein is pubescent. Major secondary veins alternate or occasionally opposite, semicraspedodromous or brochidodromous with spacing decreasing proximally. In the axils of primary and secondary veins leaf domatia developed. Leaf margin untoothed undulate or irregularly serrate in the upper half of lamina. Tooth spacing is irregular with one order of teeth. Sinus shape rounded, tooth shapes typically concave/convex or concave/flexuous. Leaves hypostomatic. Abaxial epidermis is composed of polygonal ordinary cells, anomocytic stomata, and trichomes of two types: simple elongate unicellular and glandular with uniseriate 2–3-celled stalk and single-celled head. Adaxial epidermis is composed of isodiametric ordinary cells with glandular trichomes.
Description
The leaves are simple, petiolate, with marginal petiole attachment. The incompletely preserved petiole is up to 0.8 cm long and 0.15 cm wide. Laminar size is mesophyll. The biggest specimen is 17.5 cm long and up to 6.1 cm wide, the length-to-width (L/W) ratio is approximately 3:1. The laminar shape is obovate. The leaf apex is acute, straight, or acuminate in shape. The leaf base is slightly asymmetrical, with an acute angle and cuneate shape.
Primary venation is pinnate. The primary vein is straight or slightly curved at the base, thick in the basal half and tapering upward, terminating at the apex. The surface of the primary vein is pubescent. Major secondary veins (14–17 pairs) are alternate or occasionally opposite, mixed semicraspedodromous or brochidodromous (mostly in the lower part of the lamina and near the leaf apex) with spacing that decreases proximally. The secondary vein angle is smoothly increasing proximally (from 45 to 50° in the upper part of the lamina to 70° in the lower part). The leaves possess numerous leaf domatia in the axils between primary and secondary veins (Figures 3H, 4E). Interior secondaries are absent. Minor secondary veins, if present, are semicraspedodromous. Intersecondary veins are occasionally present, usually, their length is less than 50% of the subjacent secondary vein, the intersecondary proximal course is almost perpendicular to midvein, while the intersecondary distal course is perpendicular to subjacent secondary vein. Intercostal tertiary veins are mixed percurrent with an obtuse angle to midvein and consistent vein angle variability. Epimedial tertiary veins are mixed percurrent with proximal course perpendicular to the midvein and distal course parallel to intercostal tertiaries. Exterior tertiaries are looped. Quaternary vein fabric is an alternate percurrent. Quintarnary vein fabric is irregular reticulate. Areolation is well developed. Freely ending veinlets are unbranched. Marginal ultimate venation is looped.
The leaf margin is untoothed undulate or irregularly serrate in the upper half of leaf lamina. Tooth spacing is irregular with one order of teeth and usually less than one tooth per 1 cm. Teeth are small and 1–1.5 mm high. Sinus shape is rounded, tooth shapes are concave/convex or concave/flexuous. The principal vein terminates at the tooth apex.
The leaf epidermal characters were obtained from the cuticle fragmentary preserved on the midvein and near domatia. The cuticle is very thin. Ordinary epidermal cells of a costal area are rectangular in shape, with straight anticlinal walls, 40–70 μm long and 9–15 μm wide, bearing numerous trichome bases (Figures 6A,D,F,H).
The epidermis of the leaf abaxial surface is composed of polygonal ordinary cells, randomly oriented stomata, and trichomes of two types: simple elongate unicellular and glandular (Figures 7A,C, 8A,B). The length of the unicellular non-glandular trichomes is up to 600 μm, their diameter at the base is approximately 20–30 μm (Figures 7D,E, 8A, 9C–E). Glandular trichomes have uniseriate stalks that usually consist of 2–3 cells and a single-celled head, the length of trichomes is 45–60 μm, their diameter at the base is about 20 μm (Figure 8A). Stomata are anomocytic with four to six subsidiary cells (Figures 8B, 9B,C,H), stomatal size ranges between 28 and 33 μm in length and between 25 and 28 μm in width.
The epidermis of the adaxial surface consists of isodiametric cells, usually pentagonal with undulate anticlinal cell walls, 37–60 μm long, 23–36 μm wide (Figure 8C). There are only glandular trichomes of the same morphology as on the abaxial surface (Figures 7B, 9A,G).
Material
MMJ3-008-1a, b; MMJ3-321; MMJ3-549-1; MMJ3-837-3a, b; MMJ3-898; MMJ3-2175-1a, b; MMJ3-2205-1; MMJ3-2206-2; MMJ3-2207-1; MMJ3-2207-2.
Discussion
Comparison With the Fossil Species of Meliosma
Leaves of the new species are most similar to the fossil leaves of Meliosma sp. cf. M. simplicifolia from the Eocene Clarno Formation of Oregon, United States (Manchester, 1981) in the size and shape of the lamina, the number of secondary veins, as well as the tertiary and higher order venation patterns. Meliosma eosinica sp. nov. differs from this species mostly in terms of the leaf margin characters: fossil leaves from the Maoming Basin have irregular small teeth only in the upper half of the lamina or untoothed leaf margin. By contrast, leaves from the Clarno Formation are characterized by more prominent teeth arranged almost along the whole leaf margin. However, fossil leaves from the Clarno Formation have not been described in detail.
The leaves of M. eosinica are also very similar in general morphology to the fossil leaves M. shanwangensis Hu et Chaney originally described from the lower-middle Miocene of Shandong, eastern China (Hu and Chaney, 1940). Both species are characterized by an obovate shape of the leaf lamina with an acute or acuminate apex and cuneate base, a similar number of secondary veins (12–16 pairs in M. shanwangensis and 14–17 pairs in M. eosinica) arising at angles of 50–70° from the midvein and distally curving up to the leaf margin, and a slightly asymmetrical base. Leaves of M. shanwangensis differ in having remotely serrate margin and serration starting in some cases from the leaf base.
Leaves of M. eosinica resemble leaves of M. obtusifolia Tao described also from the lower-middle Miocene of Shandong, eastern China (WGCPC (Writing Group of Cenozoic Plants of China), 1978), in respect of their obovate lamina shape and irregularly serrate margin, but differ by having an acute apex. Tao compared these fossil leaves with the leaves of extant M. parviflora Lecomte, which also possess an obovate lamina with an obtuse rounded apex. In addition, leaves of M. eosinica differ from those of M. obtusifolia by their larger lamina size and their greater number of secondary veins (14–17 pairs compared to 10–13 in Meliosma obtusifolia).
The leaves of M. eosinica are similar to those of M. longifolia (Heer) Hickey from the Golden Valley Formation (lower Eocene) of Western Dakota, United States (Hickey, 1977), in the number of secondary veins and their angle of divergence. However, M. eosinica leaves can be distinguished by their obovate shape and irregularly serrate margin.
Unlike the leaves of two species, M. duktotensis Wolfe and M. kushtakensis Wolfe, described from the Eocene Kulthieth and Kushtaka formations of the Gulf of Alaska (Wolfe, 1977), M. eosinica leaves are characterized by a larger lamina size, a greater number of secondary veins, and an irregularly serrate or undulate leaf margin.
The leaves of M. eosinica differ significantly from the leaves of two Paleocene species from North Dakota, United States, M. thriviensis Peppe et Hickey from the lower Paleocene Ludlow Member of the Fort Union Formation and M. vandaelium Peppe et Hickey from the middle Paleocene Tongue River Member of the Fort Union Formation (Peppe and Hickey, 2014). Both American species are characterized by a small number of secondary veins, 9–10 pairs in M. vandaelium and 8–14 (average 10) in M. thriviensis, compared with 14–17 pairs in M. eosinica. The secondary vein angle in M. eosinica is smoothly increasing proximally (from 45 to 50° in the upper part to 70° in the lower part of the lamina) whereas in M. vandaelium the secondary vein angle decreases basally, and in M. thriviensis secondary vein spacing and angle are uniform. These species also differ in their leaf margin characteristics. In M. eosinica, the teeth are sparse, developed mainly in the upper part of the leaf or the leaf margin is untoothed, while in M. vandaelium the margin is serrate to dentate throughout the entire leaf, the teeth are smaller and more frequent (three teeth per 1 cm). In M. thriviensis the teeth are uniformly spaced along the entire lamina (one to two teeth per 1 cm).
The only fossil leaves of Meliosma with studied epidermal characters were reported from the upper Miocene of Tiantai, Zhejiang, China in a conference abstract (Jia and Sun, 2011). Leaves of M. eosinica differ from those of M. cf. flexuosa Pamp. from Zhejiang in having a larger lamina size (6.2–9.8 cm long and 2.2–4.2 cm wide in M. cf. flexuosa), an acute apex (obtuse or narrowly acuminate in M. cf. flexuosa), and smaller irregular teeth (compared with sharp coarse marginal teeth in M. cf. flexuosa). Meliosma cf. flexuosa also differs in having only sparse multicellular hairs composed of 6–7 cells and smaller stomata (15–20 μm long, 12–15 μm wide). However, these Zhejiang fossils were not subsequently published in full.
Comparison With Modern Meliosma Species
Within living species of Meliosma, leaves of M. rigida Siebold et Zucc., M. fordii Hemsl., and M. simplicifolia have the most similar leaf architecture to those of M. eosinica. Van Beusekom (1971) treated these species as subspecies of one highly variable species M. simplicifolia, but later they were considered as individual species (Guo and Brach, 2007). The leaves of these three extant species are very similar to each other; and like M. eosinica, they are obovate or elliptic in shape with an acute or acuminate apex and a cuneate base, pinnate semicraspedodromous or brochidodromous venation with distinctly ascending secondary veins, and untoothed or serrate margins usually toothed in the upper half of lamina (Figures 10A–G,I,L). Compared with the new fossil species, M. simplicifolia differs in having larger leaves (15–40 × 4–16 cm) and a greater number of secondary veins (15–28 pairs) (Guo and Brach, 2007). The leaves of M. rigida and M. fordii are usually less than 15 cm long and 5 cm wide, the number of secondary veins is often fewer than 15 pairs. In addition, despite the certain morphological similarity of leaves, extant taxa also differ from each other and fossil species in leaf epidermal features (Figures 6B,C,E,G,I, 11A,D–G, 12).
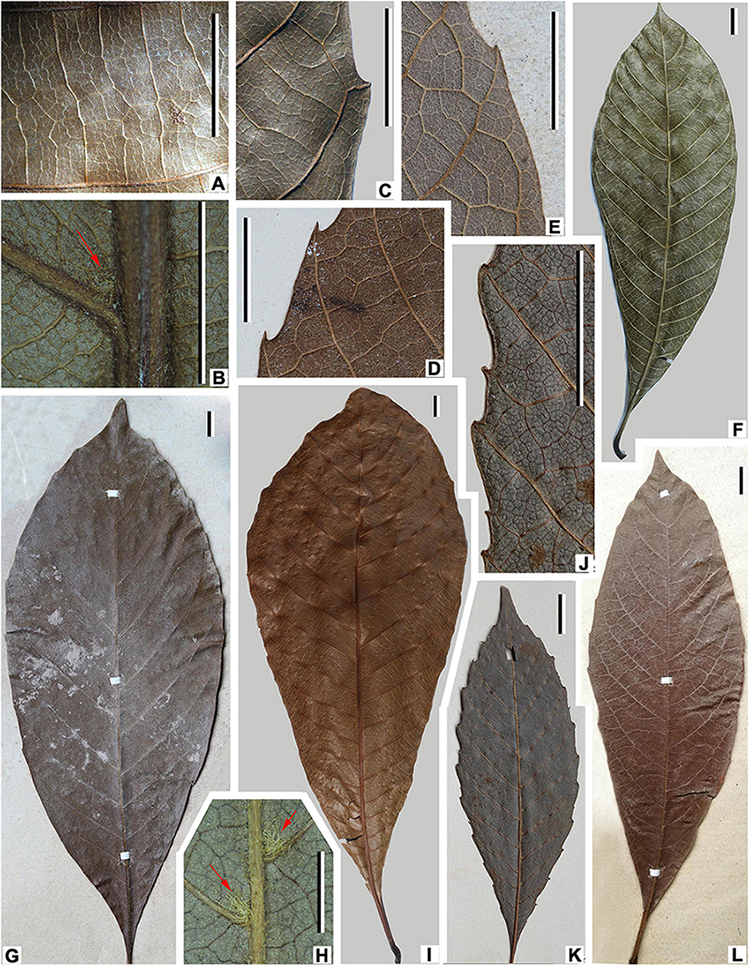
Figure 10. Leaf morphology of extant Meliosma species. M. simplicifolia (Roxb.) Walp., XTBG (A–C,F,I). (A) Tertiary and higher-order venation. (B) Leaf domatium (arrow) in the axil of midvein and secondary vein. (C) Marginal tooth. (F) Leaf with entire margin. (I) Leaf with an irregularly serrate margin. M. fordii Hemsl. (D,L). (D) Marginal tooth, SYS00085158. (L) Leaf with irregularly serrate margin, SYS00085144. M. rigida Siebold et Zucc., SYS00085219 (E,G). (E) Marginal tooth. (G) Leaf with an irregularly serrate margin. M. tenuis Maxim., SYS00085289 (H,J,K). (H) Tuft of long trichomes (domatia) in axils of midvein and secondary veins. (J) Marginal teeth of two orders. (K) Leaf with biserrate margin. Scale bars = 1 cm.
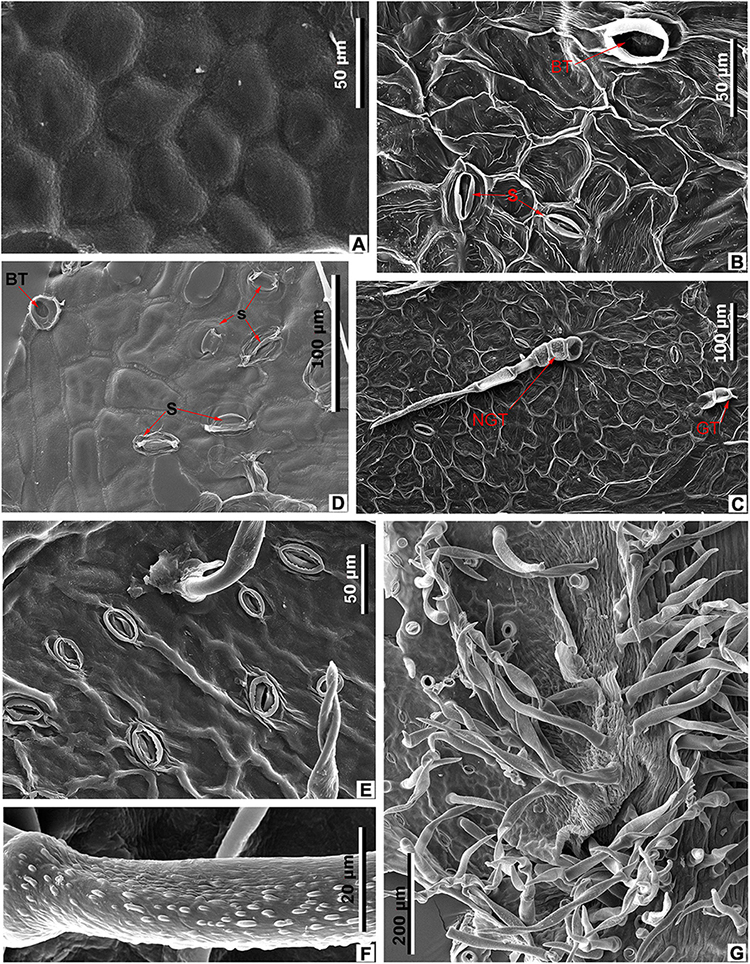
Figure 11. Leaf epidermal characters of extant Meliosma, SEM. M. simplicifolia (Roxb.) Walp., XTBG (A,D–G). (A) Ordinary cells on adaxial surface, internal view. (D) Stomata (S) and trichome base (BT) on abaxial surface, internal view. (E) Stomata and trichome base on abaxial surface, external view. (F) Trichome surface. (G) Leaf domatium. M. tenuis Maxim. SYS00085289 (B,C). (B) Stomata (S) and trichome base (BT) on abaxial surface, internal view. (C) Abaxial surface with stomata, spinose multicellular non-glandular (NGT) and multicellular glandular (GT) trichomes, external view.
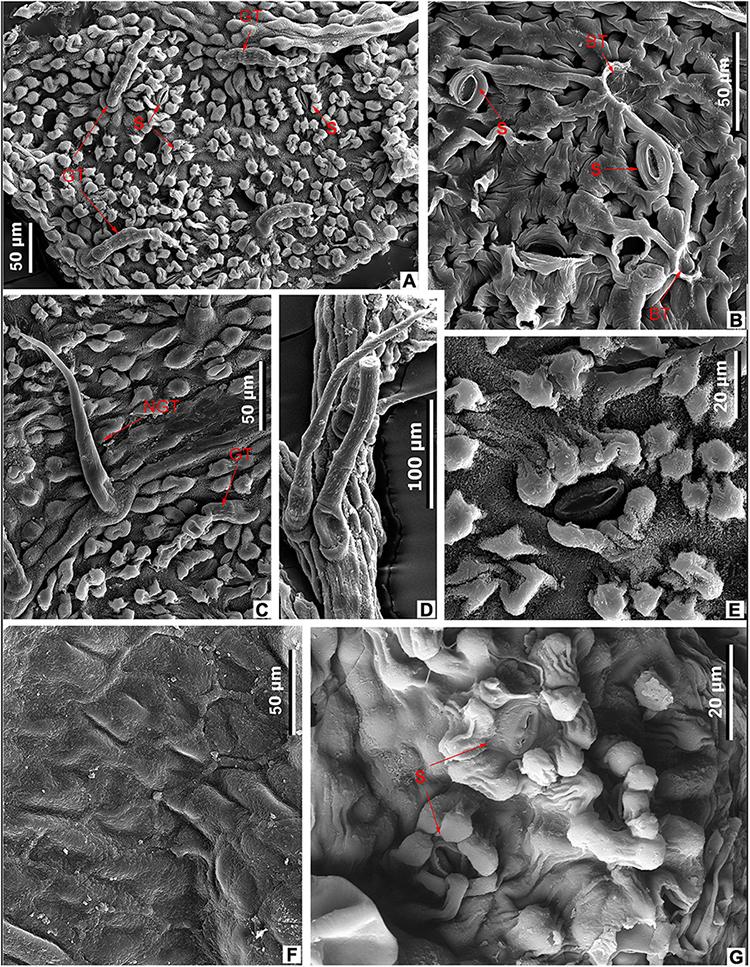
Figure 12. Leaf epidermal characters of extant Meliosma, SEM. M. fordii Hemsl., SYS00085139 (A–F). (A) Abaxial surface with papillate cells, stomata (S), and glandular trichomes (GT), external view. (B) Abaxial surface with stomata (S) and trichome base (BT), internal view. (C) Abaxial surface with glandular (GT) and unicellular non-glandular (NGT) trichomes, external view. (D) Multicellular non-glandular trichomes. (E) Stomata surrounded by papillae, external view. (F) Ordinary cells on adaxial surface, internal view. (G) M. rigida Siebold et Zucc., SYS00085214, stomata (S) with papillae on abaxial surface, external view.
The epidermal characters of the new fossil species M. eosinica are most similar to those of M. simplicifolia (Figures 6C,G,I, 7F,G, 9F, 11A,D–G; Supplementary Table 2). The epidermis of the leaf abaxial surface in both extant and fossil species is composed of ordinary cells lacking papillae, with randomly orientated anomocytic stomata and trichomes of two types: non-glandular and glandular, which are especially numerous in the axils between primary and secondary veins forming domatia of hair-tuft type (e.g., O’Dowd and Willson, 1989). On the leaf adaxial surface of both species, mostly glandular trichomes of a similar morphology are presented. The stomata in the modern species are slightly larger, 38–45 μm long and 35–37 μm wide, compared with 28–33 μm long and 25–28 μm wide in M. eosinica. M. eosinica differs from M. simplicifolia in having unicellular non-glandular trichomes. The non-glandular trichomes of the modern species consist of 2–5 cells (Figures 6C,I), and the length of trichomes varies from 100 to 500 μm. The surface of non-glandular trichomes in the new species is smoother (Figures 9E, 11F). The glandular trichomes of M. eosinica are smaller than those of M. simplicifolia, in that they consist of 2–3 cells and are up to 60 μm long (compared to 4–5 cells in the trichomes of usually more than 100 μm long in M. simplicifolia).
Unlike M. eosinica, on the leaf abaxial surface of M. rigida, all epidermal cells have papillae, the stomata are smaller (10–14 μm long and 7–9 μm wide compared with 28–33 μm long and 25–28 μm wide in M. eosinica), sunken, and surrounded by subsidiary cells with large papillae which partly cover the stomatal pit (Figure 12G; Supplementary Table 2). The epidermal characters similar to those of M. rigida are observed also in the leaves of M. fordii (Figures 12A–E; Supplementary Table 2): all epidermal cells of the abaxial surface are papillate, the stomata are relatively small (17–23 μm long and 15–18 μm wide), and the ordinary cells of the adaxial surface are non-papillate, polygonal, with straight anticlinal walls, 40–50 μm long, and 26–30 μm wide (Figure 12F). Similar to the fossil leaves of M. eosinica, numerous non-glandular and glandular trichomes are present on the leaf abaxial surface of both modern species, particularly on the midvein (Figures 12A,C). As in M. eosinica, on the leaves of M. fordii and M. rigida, simple unicellular non-glandular trichomes are sometimes present (Figure 12C), although more often, they consist of several cells (Figure 12D). Non-glandular trichomes on M. rigida leaves are usually elongated with a short basal cell and a cylindrical terminal cell.
Leaves of the extant Japanese species M. tenuis Maxim. differ from those of M. eosinica in being of smaller leaf size, having a mainly elliptic (not obovate) lamina shape, straight secondary veins craspedodromously terminated at the tooth apex and a biserrate leaf margin toothed along the entire length of the lamina (Figures 10H,J,K). The leaf epidermal features of M. eosinica are similar to those of M. tenuis in stomatal size (26–37 μm long and 15–20 μm wide in M. tenuis compared to 28–33 μm long and 25–28 μm wide in M. eosinica), with non-papillate ordinary cells on abaxial and adaxial surfaces and numerous trichomes of two types (Figures 11B,C; Supplementary Table 2). However, in contrast to M. eosinica, the non-glandular trichomes of M. tenuis are much longer (up to 1,000–1,100 μm) and consist of several cells.
Pollen Associated With Fossil Meliosma Leaves
Pollen grains recovered from the leaf surface of M. eosinica are small, prolate to subprolate, tricolpate (probably, tricolpate but this is not detectable on available SEM photos), and perforate (Figure 13). Perforations are distributed more or less regularly, at a distance 3–5 times greater than the perforation diameter. They become smaller on apocolpium regions and toward apertures; there is a narrow smooth margo along the aperture. The aperture membrane is granular. The colpus ends are pointed. The pollen outline is elongated in equatorial view and rounded in polar view (the polar outline is based on one pressed pollen grain and might be incorrect). The polar axis is 16.2–19.2 μm, the equatorial diameter is 10.5–12.5 μm, and the colpus length is 11.2–13.6 μm (based on three pollen grains).
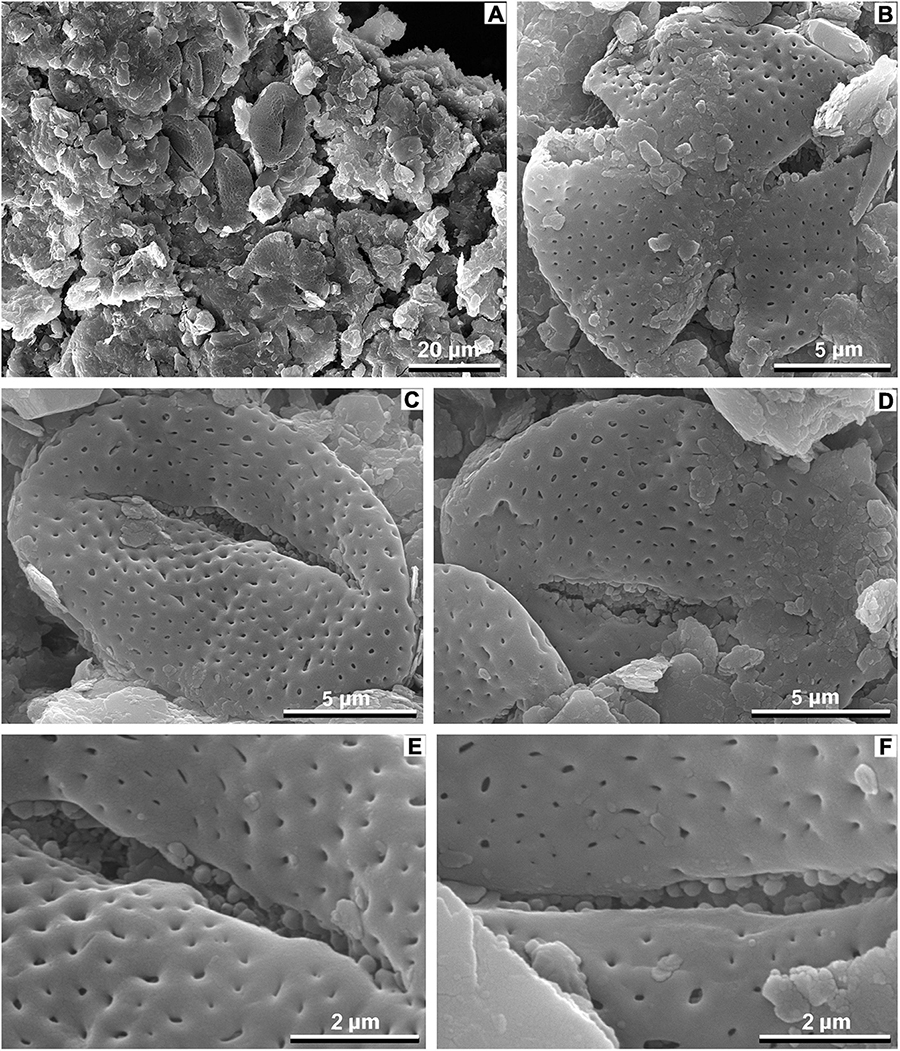
Figure 13. Fossil pollen associated with M. eosinica sp. nov., MMJ3-837-3a, SEM. (A) A cluster of pollen grains found on the leaf of Meliosma. (B) A polar view of the single pollen grain, a smooth margo along the colpus is seen. (C,D) Single pollen grain, equatorial view, granular aperture membrane, and probable os in (C) are seen. (E,F) Close-ups with exine and aperture sculpture.
We found no previous data on fossil Meliosma pollen. Studies on the pollen grains of extant Meliosma species are relatively few with the most detailed study SEM and transmission electron microscopy (TEM) by Furness et al. (2007), and short descriptions of SEM data by Li et al. (2011) and Miyochi et al. (2011). Earlier publications incorporating data from LM (see review in Mondal, 1983) are insufficient for a reliable determination of fossil Meliosma pollen. The pollen grains of extant Meliosma are small, spheroidal to subprolate, tricolporate, perforate to microreticulate; the exine sculpturing often becomes finer toward the colpi or forms a smooth margo along the colpus. Among the studied species, our fossil pollen grains show the most similarity to M. pinnata ssp. macrophylla (Merr.) Beusekom (Furness et al., 2007), M. rhoifolia Maxim. (Figures 14A,B, this study), and M. rigida (Figure 14C, this study). To a lesser extent, due to somewhat larger perforations, the studied fossil pollen grains also resemble M. buchananifolia Merr. (Li et al., 2011) and M. angustifolia Merr. (Figure 14D, this study). The pollen grains of M. fruticosa Blume (Figure 14E) and M. simplicifolia (Figure 14F) show larger perforations (microreticulation) and no smooth margo along the colpi that differentiate the here studied fossils. However, the pollen type and exine sculpturing of the found fossil pollen is rather common for eudicot angiosperms and indistinguishable from several other taxa (e.g., some Menispermaceae taxa, Harley and Ferguson, 1982).
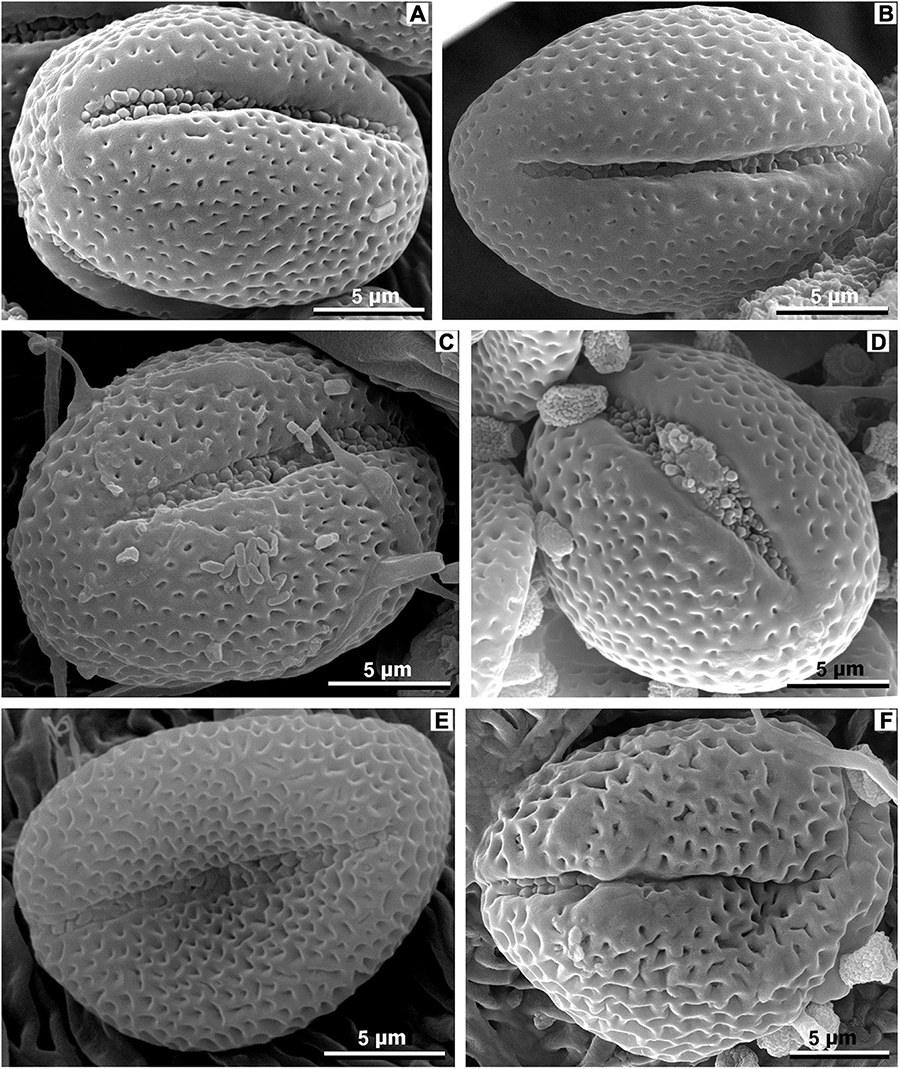
Figure 14. Pollen of extant species of Meliosma, equatorial view, SEM. (A,B) M. rhoifolia Maxim., SYS00085206. (C) M. rigida Siebold et Zucc., SYS00085214. (D) M. angustifolia Merr., SYS00085105. (E) M. fruticosa Blume, SYS00085274. (F) M. simplicifolia (Roxb.) Walp., SYS00085273.
Leaf Domatia and Associated Mites
Leaf domatia are tiny structures located on the underside of leaves, usually in the vein axils (Figure 3H). The main types of domatia of woody angiosperms are represented by cavities between veins (pockets), partial destruction of the external tissue near the vein bifurcation (pits), and hair tufts covering the area between veins. The combination of hair-tufts with pockets or pits is common for many plants (Nishida et al., 2005; Romero and Benson, 2005). Leaf domatia occur in woody angiosperms of over 80 families, including Sabiaceae, in both temperate and tropical regions but are most abundant in tropical and subtropical zones of Australia, Korea, China, Japan, New Zealand, Africa, and the United States, being limited mainly to humid areas (O’Dowd and Willson, 1989, 1991; Pemberton and Turner, 1989; O’Dowd et al., 1991; Ryu and Ehara, 1991; Willson, 1991; O’Dowd and Pemberton, 1994; Situngu and Barker, 2017). The function of leaf domatia has been debated for a long time (e.g., O’Dowd and Willson, 1989, 1991; Pemberton and Turner, 1989; Walter and O’Dowd, 1992a,b; O’Dowd and Pemberton, 1994; Agrawal, 1997; Norton et al., 2000, 2001; Romero and Benson, 2005; Ferreira et al., 2008; Rowles and O’Dowd, 2009; Schmidt, 2014; Situngu and Barker, 2017). Leaf domatia are often called acarodomatia because they are inhabited by mites (e.g., O’Dowd et al., 1991; O’Dowd and Pemberton, 1998; Norton et al., 2000; Romero and Benson, 2004, 2005; Situngu and Barker, 2017; Maccracken et al., 2019). The diversity and reproduction of mites are greater on leaves with domatia (e.g., Walter and O’Dowd, 1992a,b; O’Dowd and Pemberton, 1994; O’Connell et al., 2010). The majority of mites inhabiting leaf domatia are either predators or fungivores (e.g., O’Dowd and Willson, 1989, 1991; Pemberton and Turner, 1989; Walter and Denmark, 1991; Willson, 1991; Walter and O’Dowd, 1992a,b; Nishida et al., 2005), but saprophagous mites also utilize domatia (O’Dowd and Willson, 1989). Studies on leaves with several types of domatia revealed that different mite taxa dominate different domatia, even on the same leaf (Nishida et al., 2005). Since numerous tiny mites are characterized by a thin weakly chitinised integument, dry air, and high temperatures are very harmful to them. Domatia help to reduce moisture loss and maintain a relatively constant temperature, thereby preventing the desiccation of mite eggs and mite bodies during molting (Pemberton and Turner, 1989; Willson, 1991; Agrawal, 1997; Norton et al., 2000; Romero and Benson, 2005). Domatia also provide mites with refuges from predatory arthropods (e.g., O’Dowd and Willson, 1989; Agrawal, 1997; Norton et al., 2001; Romero and Benson, 2005; Schmidt, 2014; Situngu and Barker, 2017) and protect young predatory mites from cannibalism (Ferreira et al., 2008). Furthermore, domatia functions as pollen and fungal spore traps, providing some mites (e.g., some Phytoseiidae, Eupodidae) with additional food sources (e.g., Kreiter et al., 2002; Duso et al., 2004; Romero and Benson, 2005; Schmidt, 2014). In return, predaceous and fungivorous mites may protect leaves from epiphyllous pathogenic agents, including herbivorous arthropods and fungi (e.g., Pemberton and Turner, 1989; O’Dowd and Willson, 1991; Agrawal, 1997; Norton et al., 2000; Romero and Benson, 2004; Monks et al., 2007). Thus, leaf domatia facilitate a protective mutualism between mites and plants (Norton et al., 2000; O’Connell et al., 2010; Walter, 2017).
While studies of the modern mite-plant associations are relatively abundant, the fossil record of leaf domatia considered to be inhabited by mites is sporadic. The oldest acrodomatia in the fossil record are known from the Upper Cretaceous of western North America (Maccracken et al., 2019). Fossil acrodomatia have also been described from the middle and upper Eocene of Victoria, Australia (O’Dowd et al., 1991), the middle Eocene of western North America (Liu et al., 2014), and the Miocene of New Zealand (Lee et al., 2010, 2012; Kaulfuss et al., 2015; Conran et al., 2016). However, the interaction between domatia-bearing plants and mites in the fossil record has not been proven by the presence of mites in domatia. In this paper, we report the first occurrence of fossil mites in a leaf domatium of M. eosinica (Figure 15). The recovered mite exoskeleton is considerably damaged, the covering was apparently soft, weakly sclerotized, without pronounced shields, and with numerous cuticular papillae and the thinnest of grooves (Figures 15C,D). Several characteristic densely pilose setae are preserved (Figures 15A–D), but a chaetome cannot be reconstructed due to incomplete preservation of the exoskeleton. Preserved legs are partly deformed, but presumably, they were longer than the body (Figures 15A,B). The gnathostome is not preserved, therefore the type of nutrition is not known with certainty. Based on all visible characters, we interpreted the studied mite as belonging to the superfamily Eupodoidea (Trombidiformes, Arachnida, Arthropoda), and probably the family Eupodidae, which comprises very small (up to 200 μm long) free-living mites with soft and weakly sclerotized bodies. Eupodids can be distinguished from other mites of the superfamily by chaetotaxy, setal and integument ornamentations, and the number of rhagidial solenidia (Strandtmann, 1971; Baker, 1990; Baker and Lindquist, 2002; Krantz and Walter, 2009; Khaustov, 2015a,b).
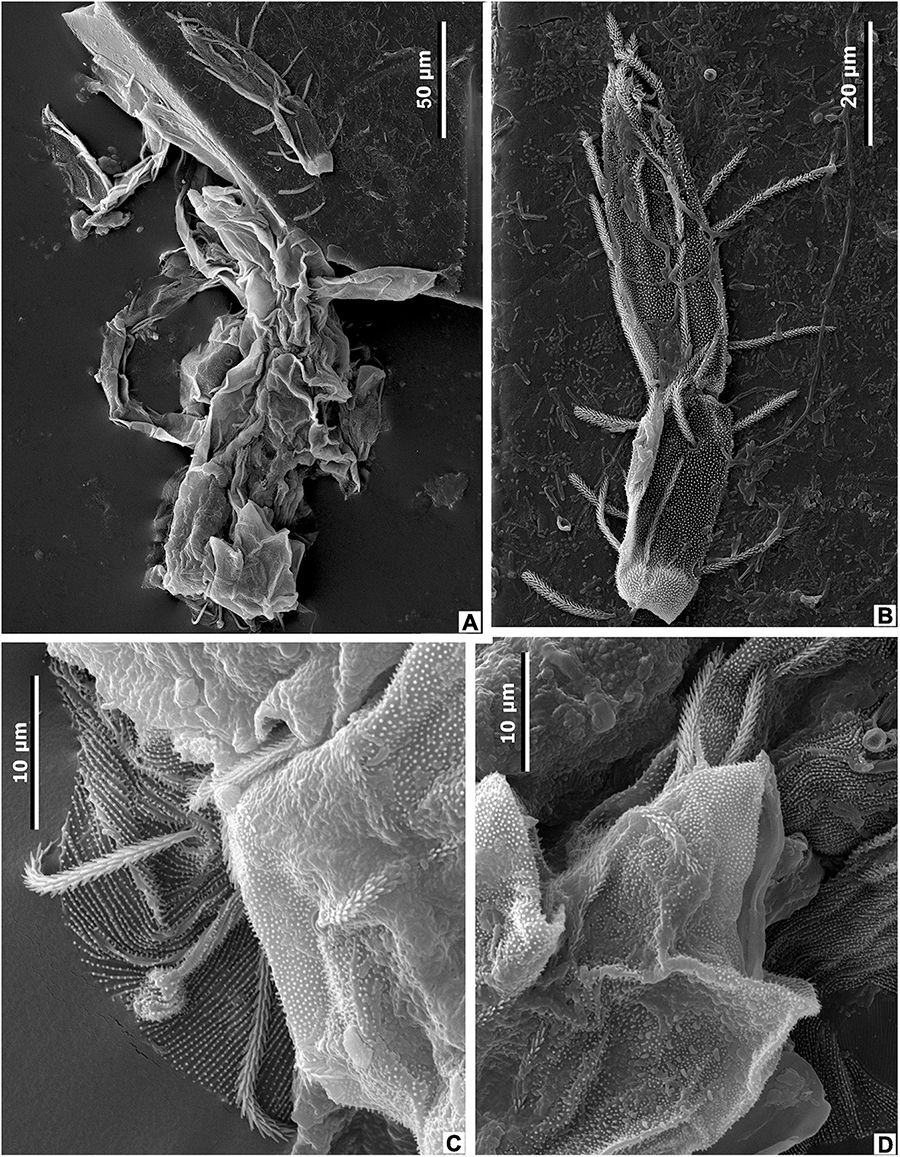
Figure 15. Mite from the leaf domatia of M. eosinica sp. nov., MMJ3-837-3a, SEM. (A) Incomplete mite exoskeleton, general view. (B) Mite leg. (C,D) Details of mite exoskeleton.
Eupodoidea has a global distribution, including both polar regions (e.g., Strandtmann, 1971; Olivier and Theron, 2000; Baker and Lindquist, 2002; Booth et al., 2009). The most described species of extant mites inhabit temperate to cold regions, whereas mites from tropical areas are much less understood (Baker and Lindquist, 2002; Booth et al., 2009). In China, extant Eupodidae mites were studied from the Shanghai City (Zha et al., 2003), Sichuan (He et al., 2012), Fujian (Zhang and Lin, 2012), and Jiangxi (Ding et al., 2008) provinces.
The greatest diversity and frequency of extant Eupodoidea mites are reported from the soil, leaf litter, mosses, lichens, decomposing wood, grasses, and shrubs where they may act as fungivores, predators, bryophytes, or phytophages (e.g., Baker, 1990; Krantz and Walter, 2009; Bizarro et al., 2020). Moreover, abundant Eupodoidea mites inhabit canopies from temperate to tropical forests living on the bark and under the bark of trees, as well as on leaves and stems (e.g., Walter and Behan-Pelletier, 1999; Romero and Benson, 2004; Situngu and Barker, 2017). Mites of the family Eupodidae are often very abundant in soil habitats. They are typically found in moist microhabitats, many of them are fungivores (Cowles, 2018). Most bark- and leaf-dweller eupodids are algivores, fungivores, and pollen-feeders (Walter and Behan-Pelletier, 1999).
We suppose that the fossil mites studied here lived in the forest canopy occupying the tomentous leaf surface and the domatia of the hair-tuft type.
Types of Arthropod and Fungal Damage on the Leaves of Meliosma
Analysis of plant interactions with arthropods and plant pathogens (especially fungi) in the fossil record provides recent ecological and evolutionary studies with comparative long-term data on the influence of climate change on the ecosystem structure and food-chain relationships (Currano et al., 2008; Wilf, 2008; Wappler, 2010; Wappler et al., 2012; Wappler and Grímsson, 2016), recovery of ecosystems after environmental crises and mass extinctions (Labandeira et al., 2002; Wilf et al., 2006; Wilf, 2008; Donovan et al., 2014, 2016), and their evolutionary implications (Labandeira, 2013, 2021; Labandeira and Currano, 2013).
Damage traces on the leaves of M. eosinica are caused mainly by external feeders and, more rarely, by fungi. Insect damage types (DTs) from the external foliage feeding group can be assigned to hole feeding, margin feeding, skeletonization, and surface feeding. The most common DTs are hole feeding types. Medium-sized circular and polylobate holes (DT2, DT3) range in maximum diameter between 2 and 5 mm; with weakly developed reaction tissue present along the edges of polylobate holes (Figures 3C,D, 5F,H). Larger circular holes (DT4) reach 6.5 mm in diameter (Figure 5F). Large-sized polylobate perforations (DT5) showing a narrow reaction rim are confined to the axils between the midvein and secondary veins (Figures 3E, 5D). A cluster of three holes located at the divergence point of secondary veins from primary vein is assigned to DT57 (Figures 3D, 5H). Margin feeding damage (DT15) is present as incisions (up to 7 mm wide and 10 mm deep) extending toward the midvein and showing a distinct reaction rim (Figure 3E). One leaf is skeletonized (Figures 5A,B). Polygonal patches of skeletonized tissue (DT17) are bounded by tertiary veins, with one elongate skeletonized zone adjacent to a secondary vein. Reaction tissue is usually present along the edges of the patches, and high order venation is preserved within skeletonized areas. Surface feeding damage (DT31) recorded on only one leaf is characterized by a circular shape and a distinct reaction rim, and measures 5 mm in diameter (Figure 3E).
A probable fungal infection mark with a wide diffuse reaction front bounded on one side by a tertiary vein is evident in the axillary zone of the primary vein and a secondary vein where domatia are developed (Figures 5F,G).
Review of the Meliosma Fossils (Including Leaves, Fruits, and Wood) and Their Geographical Distribution
Meliosma has a rather long and well-documented fossil history and was widespread both in North America and Eurasia during most of the Paleogene and Neogene (Supplementary Table 1; Supplementary Figure 1). The earliest records assigned to the genus Meliosma have been reported from the Upper Cretaceous (Maastrichtian) of Europe (Knobloch and Mai, 1986). Endocarps of M. praealba Knobloch et Mai were described from Walbeck, Germany; this species corresponds to the subgenus Kingsboroughia according to the classification of Van Beusekom (1971). In North America, the presence of Meliosma is confirmed by endocarps of M. rostellata (Lesquereux) Crane, Manchester et Dilcher from the uppermost Cretaceous or lowermost Paleocene Denver Formation near Golden, Colorado, and the Paleocene Sentinel Butte Member of Fort Union Formation near Almont, North Dakota (Crane et al., 1990). Paleocene endocarps are also known in Europe. Mai (1987) described two species, M. gonnensis Mai and M. sheppeyensis Reid et Chandler, from the lower and upper Paleocene of Gonna near Sangerhausen, Germany. According to Mai (1987), the endocarps of M. sheppeyensis are very similar to those from the Lower Eocene London Clay Formation of southern England (Reid and Chandler, 1933).
The oldest occurrence of fossil leaves assigned to the genus Meliosma is from the Paleocene of Williston Basin, North Dakota, United States (Supplementary Figure 1). The species M. thriviensis was recorded from the lower Paleocene Ludlow Member of the Fort Union Formation, while M. vandaelium comes from the middle Paleocene Tongue River Member of the Fort Union Formation (Peppe and Hickey, 2014). Unlike most other Meliosma species, these two species are characterized by a relatively small number of secondary veins, 9–10 pairs in M. vandaelium and 8–14 in M. thriviensis. Peppe and Hickey (2014) compare these species with M. longifolia from the lower Eocene Golden Valley Formation of North Dakota, United States, and note a trend toward an increasing number of secondary vein pairs. They suggest that M. thriviensis, M. vandaelium, and M. longifolia might represent a pattern of evolutionary change within the genus. M. thriviensis could represent an evolutionary precursor to M. vandaelium, and M. vandaelium, in turn, could be a precursor to M. longifolia (Peppe and Hickey, 2014).
In the Eocene, the genus Meliosma reaches a wide distribution and diversity, confirmed by numerous occurrences of fossil fruits, leaves, and wood, including leaves of the new species M. eosinica and associated pollen grains described in this paper. Three species of endocarps, M. sheppeyensis Reid et Chandler, M. cantiensis Reid et Chandler, and M. jenkinsii Reid et Chandler, have been described from the lower Eocene London Clay Formation of southern England (Reid and Chandler, 1933; Chandler, 1961; Collinson, 1983). M. jenkinsii also has been reported from the middle Eocene Geiseltal Flora of eastern Germany (Mai, 1976).
The middle Eocene Nut Beds assemblage from the Clarno Formation of Oregon, United States is one of the most interesting fossil floras, which includes five species of Meliosma based on endocarps (Manchester, 1994), three species of woods (Wheeler and Manchester, 2002), and leaf species (Manchester, 1981). Manchester (1994) described four species of fossil endocarps, M. beusekomii Manchester, M. bonesii Manchester, M. elongicarpa Manchester, and M. leptocarpa Manchester, which correspond to the subgenus Meliosma, section Meliosma, and one species, M. cf. jenkinsii Reid et Chandler, corresponds to the subgenus Kingsboroughia. The Clarno Formation fossil wood is the oldest known wood with anatomical characteristics similar to those of extant genus Meliosma. There are three types of Meliosma wood in the Clarno Formation assemblage, M. brehmii Wheeler et Manchester, M. deweyii Wheeler et Manchester, and M. dodsonii Wheeler et Manchester, distinguished by variation in porosity, ray size, and ray cellular composition. All Meliosma woods have characteristics of the subgenus Meliosma, section Meliosma (Wheeler and Manchester, 2002). Only one well-preserved fossil leaf of Meliosma from this locality has been illustrated but so far has not been described (Manchester, 1981). The leaf from the Clarno Formation is most similar in morphology to those of extant M. simplicifolia.
Fossil leaves more or less similar in morphology to those of extant Meliosma have been described from several Eocene localities in North America and Asia. Two species, M. duktotensis and M. kushtakensis, were reported from the middle-upper Eocene Kulthieth and Kushtaka formations of the Gulf of Alaska (Wolfe, 1977). M. longifolia comes from the lower Eocene Golden Valley Formation of North Dakota, United States (Hickey, 1977). Previously, parts of these same leaves were assigned to Quercus sullyi Newberry by Brown (1962). According to Crane et al. (1990) and Manchester (2014), the leaves illustrated by Hickey, and most of those treated as Q. sullyi by Brown, appear to represent the extinct genus Dyrana Golovneva (Golovneva, 1994; Budantsev and Golovneva, 2009). The glandular (rather than spinose) marginal teeth with rounded sinuses may indicate that these leaves represent Platanaceae, rather than Sabiaceae (Manchester, 2014).
A single leaf from the Chalk Bluffs assemblage, Sierra Nevada, California (United States) assigned to M. truncata MacGinitie (1941) is similar in morphology to the leaves of extant M. parviflora (Van Beusekom, 1971), but in fact, such an important diagnostic feature as the shape of the leaf apex is unknown, since the apex has not been preserved.
In China, within the distribution area of extant Meliosma, occurrences of Eocene Meliosma-like leaves have been also repeatedly mentioned (Supplementary Table 1), but fragmentary preservation does not afford confidence in determining their taxonomic affinity. Among them, M. rigidofolia Guo was described based on one small incomplete leaf from the Guchengzi Formation of Fushun coal mine near Fushun City, Liaoning, northeastern China (WGCPC (Writing Group of Cenozoic Plants of China), 1978). Two poorly preserved incomplete leaves from the Shinao Basin, Panxian County, Guizhou, southwest China (Zhang, 1983) and one fossil leaf from the Nadu Formation, Fengling Town, Nanning City, Guangxi, South China (Feng et al., 1977) were assigned to Meliosma sp. M. augusta auct. was listed in the assemblage of presumably early Eocene in age from the Yilan coal mine, Heilongjiang, northernmost China, but this plant fossil has not been described and figured (He and Tao, 1997).
Oligocene occurrences of leaf and fruit fossils of Meliosma are less common. Three species of fossil leaves morphologically similar to extant Meliosma were reported from the Goshen flora (early Oligocene), Lane County, Oregon (United States): M. rostrata Chaney et Sanborn (Chaney and Sanborn, 1933, depicted in Van Beusekom, 1971, p. 403, Figures 14-C 1, 2, M. goshenensis Chaney et Sanborn (Chaney and Sanborn, 1933, depicted in Van Beusekom, 1971, p. 399, Figure 10-A), and M. aesculifolia Chaney et Sanborn (Chaney and Sanborn, 1933, depicted in Van Beusekom, 1971, p. 391, Figure 14-B). M. goshenensis, according to Van Beusekom (1971), is very similar in morphology to the extant species M. rigida and M. fordii. Small lanceolate leaves (or leaflets?) that serrate along the whole lamina margin were described as M. nevolinae Pavlyutkin from the lower Oligocene Fatashinskaya Formation of South Primorye, Russia (Pavlyutkin et al., 2014). Furthermore, a single small leaf lacking an apex, probably pubescent, with a serrate margin in the upper part of the lamina, was described as Meliosma sp. from the same deposits (Pavlyutkin et al., 2014). A single incomplete leaf of Meliosma sp. was described (but not pictured) from the Liuqu Formation of Lazi County, Xizang, southwestern China (Tao, 1988). The age of this formation, according to various reports, has been thought to be Oligocene (Wei et al., 2011), Oligocene–Miocene (Li et al., 2015), or late Paleocene (Ding et al., 2017), this most recent determination being based on radiometric analysis of contained volcanic ashes.
Several Meliosma species based on endocarps are known from the Oligocene of Europe. M. reticulata (C. et E.M. Reid) Chandler was originally described from Bovey Tracey, Devonshire, England, and subsequently also recovered from the Haselbach member, Saxony, Germany (Chandler, 1957; Mai and Walther, 1978). Endocarps of M. europaea C. et E.M. Reid have been reported from several Oligocene and Miocene localities in Western Siberia (Dorofeev, 1963). According to Mai (1964), the older epithet wetteraviensis has priority over europaea, and, consequently, M. europaea was placed by Mai in the synonymy of M. wetteraviensis (Ludw.) Mai. This species is also known from the Miocene and numerous Pliocene localities of Europe (Supplementary Table 1). Van Beusekom (1971) noted that the similarity of these endocarps (especially from Pliocene) to modern ones of M. veitchiorum Hemsl. is very close; they are probably best attributed to M. veitchiorum.
Several species of Meliosma endocarps have been also found both in the Miocene and Pliocene of Europe. M. miessleri Mai was described from the Miocene of Germany, the Czech Republic, and the Pliocene of Italy (Supplementary Table 1; Mai, 1964; Van Beusekom, 1971; Martinetto, 1998; Holý et al., 2012), and M. pliocaenica (Szafer) Gregor has been recorded from the upper Miocene Sandanski Formation and upper Miocene–lower Pliocene Noviiskar Formation in Bulgaria (Mai and Palamarev, 1997).
Silicified wood of Meliosma mio-oldhami Suzuki et Watari is known from the Lower Miocene Nawamata Formation of central Japan (Watari, 1949; Suzuki and Watari, 1994). This species is similar to the extant species M. oldhami Miq. ex Maxim. in wood anatomy.
The only species of Meliosma leaves in the upper Miocene of North America, described as M. predentata MacGinitie, was reported from the Kilgore flora of Nebraska, United States (Van Beusekom, 1971, p. 402, Figure 10-D).
Fossil leaves of M. obtusifolia and M. shanwangensis have been described from the lower-middle Miocene Shanwang Formation of Shandong, eastern China (Hu and Chaney, 1940; WGCPC (Writing Group of Cenozoic Plants of China), 1978). Species M. shanwangensis are also known from the Miocene of Kobe Group, Japan, and South Primorye, Russia (Hori, 1983; Pavlyutkin et al., 2012). Miocene–Pliocene leaves from southwestern China were assigned to M. lincangensis Tao et Chen from the Lincang Region, Yunnan (Tao and Chen, 1983; Tao et al., 2000), and extant species M. buchananifolia (younger synonym of M. thorelii Lecomte) from Tengchong County, Yunnan (Tao and Du, 1982; Tao et al., 2000; Li et al., 2004). Meliosma sp. based on a single incomplete leaf was reported from the Tatsumitoge flora (late Miocene), Tottori Prefecture, Southwest Honshu, Japan (Ozaki, 1980).
The only fossil leaves with known epidermal characters assigned to the extant species M. cf. flexuosa were reported from the upper Miocene Shengxian Formation of Zhejiang Province, eastern China (Jia and Sun, 2011).
Pliocene endocarps of M. canavesana Martinetto, originally described as Meliosma aff. reticulata (Martinetto, 1998), have been reported from several fossil sites in northwestern and Central Italy (Martinetto, 2001a). Two species of fossil leaves similar to the leaves of modern species M. myriantha Siebold et Zucc. and M. tenuis were identified from the Kabutoiwa Formation of Central Honshu, Japan (Ozaki, 1991). Fossil endocarps of Meliosma cf. rigida Siebold et Zucc. were reported from the lower Pliocene of Hatagoya, Central Honshu, Japan (Miki, 1941; Van Beusekom, 1971, p. 402, Figures 13-E 1, 2).
Conclusion
The new species of M. eosinica from the Eocene of the Maoming Basin adds to the diversity of the genus in the Paleogene and extends its paleogeographical range to South China. The leaves of the new fossil species are morphologically most similar to those of the extant Asian species M. rigida, M. fordii, and M. simplicifolia, but extant taxa differ from the fossil species, and each other, in leaf epidermal characters. Pollen grains found on the leaf surface of M. eosinica sp. nov. resemble those of the studied extant species M. pinnata ssp. macrophylla (Furness et al., 2007), M. rhoifolia, and M. rigida. The first fossil record of a mite associated with leaf domatia increases understanding of co-evolutionary relationships between plants and mites in deep time.
Data Availability Statement
The original contributions presented in the study are included in the article/Supplementary Material, further inquiries can be directed to the corresponding author.
Author Contributions
MM, TK, and JJ conceived the project and designed the research. JJ organized field work and led the data acquisition. MM prepared, imaged fossil and modern specimens, and prepared and studied the cuticles with LM and SEM. MT analyzed and interpreted data on fossil and modern pollen. NM and TK analyzed and interpreted data on fossil mite. MM, TK, MT, NM, XW, and JJ contributed to initial manuscript preparation. All authors contributed to the discussion, final manuscript preparation, and approved submission.
Funding
This study was supported by the National Natural Science Foundation of China (Grant Nos. 41872015, 42111530024 to JJ), State Key Laboratory of Palaeobiology and Stratigraphy (Nanjing Institute of Geology and Palaeontology, CAS) (Grant No. 193118 to JJ), the Fundamental Research Funds for the Central Universities (Grant No. 2021qntd18 to XW), and the Russian Foundation for Basic Research (Grant Nos. 19-04-00046 for NM and 21-54-53001 for MT, NM). The work was carried out within the framework of the State program 0135-2019-0045 GIN RAS.
Conflict of Interest
The authors declare that the research was conducted in the absence of any commercial or financial relationships that could be construed as a potential conflict of interest.
Publisher’s Note
All claims expressed in this article are solely those of the authors and do not necessarily represent those of their affiliated organizations, or those of the publisher, the editors and the reviewers. Any product that may be evaluated in this article, or claim that may be made by its manufacturer, is not guaranteed or endorsed by the publisher.
Acknowledgments
We would like to thank Philipp E. Chetverikov (Zoological Institute RAS, Saint Petersburg, Russia) and Alexander A. Khaustov (Tyumen State University, Russia) for their assistance in the identification of the fossil mite. We thank Galina N. Aleksandrova (Geological Institute RAS, Moscow, Russia) for valuable discussions on fossil pollen, Elena I. Kostina (Geological Institute RAS, Moscow, Russia) for her help and advice on macerating fossil and modern leaf cuticles, Robert A. Spicer (School of Environment, Earth and Ecosystem Sciences, The Open University, Milton Keynes, United Kingdom) for valuable advice, and Steven R. Manchester (Florida Museum of Natural History, Gainesville, FL, United States) for helpful comments, donated leaves of Meliosma from his own herbarium, and unpublished photographs of fossils from the Clarno Formation for comparison. We are grateful to Roman A. Rakitov for his help during the SEM examination. We also thank Alexei B. Herman (Geological Institute RAS, Moscow, Russia) for his helpful suggestions on this study. We thank the editor and two reviewers for their constructive comments and useful suggestions.
Supplementary Material
The Supplementary Material for this article can be found online at: https://www.frontiersin.org/articles/10.3389/fevo.2021.770687/full#supplementary-material
Supplementary Figure 1 | Plate tectonic maps showing the main fossil occurrences of the Meliosma endocarps (blue), leaves (red), and woods (green). Localities: (1). Walbeck (Germany); (2). Almont and Williston Basin, North Dakota (United States); (3). Golden, Colorado (United States); (4). Gonna (Germany); (5). London Clay (southern England); (6). Geiseltal (Germany); (7). Messel (Germany); (8). Gulf of Alaska Region (United States); (9). Nut Beds, Oregon (United States); (10). Chalk Bluffs, Sierra Nevada, California (United States); (11). Fushun coal mine, Liaoning (China); (12). Maoming Basin, Guangdong (South China); (13). Goshen, Oregon (United States); (14). Kraskino, South Primorye (Russia); (15). Lazi County, Xizang, Tibet (China); (16). Bovey Tracey and Heathfield, Devonshire (England); (17). Haselbach, Saxony (Germany); (18). Omsk Region, Western Siberia (Russia); (19). Monzen, Noto Peninsula (Japan); (20). Shandong (eastern China); (21). Zhejiang (eastern China); (22). Kilgore, Nebraska (United States); (23). Tengchong County and Lincang Region, Yunnan (southwestern China); (24). Hartau near Zittau (eastern Germany) and Kristina Mine (Czech Republic); (25). Sento, Lanzo (northern Italy); (26). Central Italy; (27). Bulgaria; (28). Western Germany; (29). Cantal, France; (30). Central Honshu, Japan. Plate tectonic maps according to Hay et al. (1999).
Supplementary Table 1 | Fossil record of Meliosma.
Supplementary Table 2 | Leaf epidermal characters of Meliosma eosinica and similar extant species of Meliosma.
References
Agrawal, A. (1997). Do leaf domatia mediate a plant-mite mutualism? An experimental test of the effects on predators and herbivores. Ecol. Entomol. 22, 371–376. doi: 10.1046/j.1365-2311.1997.00088.x
Aleksandrova, G. N., Kodrul, T. M., and Jin, J. H. (2015). Palynological and paleobotanical investigations of Paleogene sections in the Maoming Basin, South China. Stratigr. Geol. Correl. 23, 300–325. doi: 10.1134/S0869593815030028
Angiosperm Phylogeny Group (2016). An update of the Angiosperm Phylogeny Group classification for the orders and families of flowering plants: APG IV. Bot. J. Linn. Soc. 181, 1–20. doi: 10.1111/boj.12385
Baker, A. S. (1990). A survey of external morphology of mites of the superfamily Eupodoidea Banks, 1894 (Acari: Acariformes). J. Nat. Hist. 24, 1227–1261. doi: 10.1080/00222939000770741
Baker, A. S., and Lindquist, E. E. (2002). Aethosolenia laselvensis gen. nov., sp. nov., a new eupodoid mite from Costa Rica (Acari: Prostigmata). Syst. Appl. Acarol. Spec. Publ. 11, 1–11. doi: 10.11158/saasp.11.1.1
Bentham, G., and Hooker, J. D. (1862). Genera Plantarum, Part I. London: Reeve & CC, Williams & Norgate.
Bizarro, G. L., Wurlitzer, W. B., Britto, E. P. J., Johann, L., Ferla, N. J., de Moraes, G. J., et al. (2020). Two new species (Acari: Tydeidae: Eupodidae) from Mato Grosso. Brazil. Intern. J. Acarol. 46, 538–543. doi: 10.1080/01647954.2020.1825527
Booth, R. G., Edwards, M., and Usher, M. B. (2009). Mites of the genus Eupodes (Acari, Prostigmata) from maritime Antarctica: a biometrical and taxonomic study. J. Zool. 207, 381–406. doi: 10.1111/j.1469-7998.1985.tb04939.x
Brown, R. W. (1962). Paleocene flora of the Rocky Mountains and Great Plains. U.S.Geol. Surv. Prof. Pap. 375:119. doi: 10.3133/pp375
Budantsev, L. Y., and Golovneva, L. B. (2009). Iskopaemye flory Arktiki II – Paleogenovaja flora Shpizbergena. Russian Academy of Sciences, Komarov Botanical Institute. Saint Petersburg: Marafon.
Chandler, M. E. J. (1957). The Oligocene flora of the Bovey Tracey lake basin, Devonshire. Bull. Bri. Mus. (Natural History) Geol. 3, 71–123. doi: 10.5962/p.313850
Chandler, M. E. J. (1961). The Lower Tertiary Floras of Southern England. 1. Paleocene Floras. London Clay Flora (Suppl.). Text and atlas. London: Trustees of the British Museum, doi: 10.5962/bhl.title.110079
Chaney, R. W., and Sanborn, E. I (1933). The Goshen Flora of West Central Oregon. Washington DC: Publ. Carnegie Inst. Wash.
Collinson, M. E. (1983). Fossil Plants of the London Clay. Palaeontological Association Field Guide to Fossils. Number 1. Oxford: Oxford University Press.
Collinson, M. E., Manchester, S. R., and Wilde, V. (2012). Fossil fruits and seeds of the Middle Eocene Messel biota, Germany. Abh. Senckenb. Naturforsch. Ges. 570, 1–249.
Conran, J. G., Lee, D. E., and Reichgelt, T. (2016). Malloranga dentata (Euphorbiaceae: Acalyphoideae): a new fossil species from the Miocene of New Zealand. Rev. Palaeobot. Palynol. 226, 58–64. doi: 10.1016/j.revpalbo.2015.12.007
Cornejo, X. (2008). Four new species of Meliosma (Sabiaceae) from Ecuador and Bolivia. Harvard Pap. Bot. 13, 93–102.
Cornejo, X. (2009). Meliosma longepedicellata (Sabiaceae): a new species from southeastern Ecuador. Harvard Pap. Bot. 14, 93–96. doi: 10.3100/025.014.0216
Cowles, J. (2018). Amazing Arachnids. Princeton, NJ: Princeton University Press, doi: 10.1515/9781400890187
Crane, P. R., Manchester, S. R., and Dilcher, D. L. (1990). A preliminary survey of fossil leaves and well-preserved reproductive structures from the Sentinel Butte Formation (Paleocene) near Almont, North Dakota. Fieldiana Geol. Publ. 14:63. doi: 10.5962/bhl.title.100826
Cronquist, A. (1957). Outline of a new system of families and orders of dicotyledons. Bull. Jard. Bot. l’État Brux. 27, 13–40. doi: 10.2307/3666976
Cronquist, A. (1981). An Integrated System of Classification of Flowering Plants. New York, NY: Columbia University Press.
Currano, E. D., Wilf, P., Wing, S. L., Labandeira, C. C., Lovelock, E. C., and Royer, D. L. (2008). Sharply increased insect herbivory during the Paleocene-Eocene Thermal Maximum. Proc. Natl. Acad. Sci. U.S.A. 105, 1960–1964. doi: 10.1073/pnas.0708646105
Dahlgren, R. M. T. (1980). A revised system of classification of the angiosperms. Bot. J. Linn. Soc. 80, 91–124. doi: 10.1111/j.1095-8339.1980.tb01661.x
Ding, C.-C., Dai, Z.-K., Xue, X.-F., Li, H.-X., Liu, M.-Q., Chen, X.-Y., et al. (2008). Effects of vegetations restoration on soil mite community in degraded red soil, Subtropical China. Acta Ecol. Sin. 28, 4771–4781.
Ding, L., Spicer, R. A., Yang, J., Xu, Q., Cai, F. L., Li, S., et al. (2017). Quantifying the rise of the Himalaya orogen and implications for the South Asian monsoon. Geology 45, 215–218. doi: 10.1130/G38583.1
Donovan, M. P., Iglesias, A., Wilf, P., Labandeira, C. C., and Cúneo, N. R. (2016). Rapid recovery of Patagonian plant-insect associations after the end-Cretaceous extinction. Nat. Ecol. Evol. 1:12. doi: 10.1038/s41559-016-0012
Donovan, M. P., Wilf, P., Labandeira, C. C., Johnson, K. R., and Peppe, D. J. (2014). Novel insect leaf-mining after the end-Cretaceous extinction and the demise of Cretaceous leaf miners, Great Plains, USA. PLoS One 9:e103542. doi: 10.1371/journal.pone.0103542
Duso, C., Malagnini, V., Paganelli, A., Aldegheri, L., Bottini, M., and Otto, S. (2004). Pollen availability and abundance of predatory phytoseiid mites on natural and secondary hedgerows. BioControl 49, 397–415. doi: 10.1023/B:BICO.0000034601.95956.89
Ellis, B., Daly, D. C., Hickey, L. J., Johnson, K. R., Mitchel, J. D., Wilf, P., et al. (2009). Manual of Leaf Architecture. Ithaca, NY: Cornell University Press.
Feng, S. N., Meng, F. S., Chen, G. X., Xi, Y. H., Zhang, C. F., and Liu, Y. A. (1977). “Plant kingdom”, in Palaeontological atlas of Central South China, vol. 3, Mesozoic and Cenozoic, Hubei Institute of Geological Science, Henan Bureau of Geology and Mineral Resources. Beijing: Geological Publishing House, 195–262.
Ferreira, J. A. M., Eshuis, B., Janssen, A., and Saabelis, M. W. (2008). Domatia reduce larval cannibalism in predatory mites. Ecol. Entomol. 33, 374–379. doi: 10.1111/j.1365-2311.2007.00970.x
Furness, C. A., Magallón, S., and Rudall, P. J. (2007). Evolution of endoapertures in early-divergent eudicots, with particular reference to pollen morphology in Sabiaceae. Plant Syst. Evol. 263, 77–92. doi: 10.1007/s00606-006-0477-y
Golovneva, L. B. (1994). Maastriht – Datskie Flory Korjakskogo Nagor’ja. Proc. Komarov Botanical Institute. Issue 13. Saint Petersburg: BIN RAN.
Guo, L. X., and Brach, A. R. (2007). “Sabiaceae,” in Flora of China, eds Z. Y. Wu, P. H. Raven, and D. Y. Hong (Beijing: Science Press), 25–42.
Harley, M. M., and Ferguson, I. K. (1982). Pollen morphology and taxonomy of the tribe Menispermeae (Menispermaceae). Kew Bull. 37, 353–366. doi: 10.2307/4110032
He, C.-X., and Tao, J.-R. (1997). A study on the Eocene flora in Yilan County, Heilongjiang, China. J. Syst. Evol. 35, 249–256.
He, X. J., Wu, P. F., Cui, L. W., and Zhang, H. Z. (2012). Effects of slope gradient on the community structures and diversities of soil fauna. Acta Ecol. Sinica 32, 3701–3713. doi: 10.5846/stxb201112081879
Hickey, L. J. (1977). Stratigraphy and paleobotany of the Golden Valley Formation (Early Tertiary) of western North Dakota. Geol. Soc. Am. Mem. 150:296.
Hilu, K. W., Borsch, T., Muller, K., Soltis, D. E., Soltis, P. S., Savolainen, V., et al. (2003). Angiosperm phylogeny based on matK sequence information. Am. J. Bot. 90, 1758–1776. doi: 10.3732/ajb.90.12.1758
Holý, F., Kvaček, Z., and Teodoridis, V. (2012). A review of the early Miocene mastixioid flora of the Kristina Mine at Hrádek nad Nisou in North Bohemia (Czech Republic). Acta Mus. Nat. Pragae, Ser. B Hist. Nat. 68, 53–118.
Hori, J. (1983). Kobe’s Plant Fossils. Kobe: Kobe Shimbun Publ. Center, doi: 10.1295/kobunshi.32.196
Hu, H. H., and Chaney, R. W. (1940). A Miocene Flora From Shantung Province, China. Washington DC: Carnegie Institution of Washington Publication.
Jia, H., and Sun, B. N. (2011). “Leaves of Meliosma cf. flexuosa from upper Miocene of Tiantai, Zhejiang and its microstructure,” in Proccedings of the Abstract volume of the 26th Annual Conference of Palaeontological Society of China, October 2011. Guanling of Guizhou, China, (Guizhou), 245–246.
Kaulfuss, U., Lee, D. E., Barratt, B. I., Leschen, R. A., Larivière, M. C., Dlussky, G. M., et al. (2015). A diverse fossil terrestrial arthropod fauna from New Zealand: evidence from the early Miocene Foulden Maar fossil lagerstätte. Lethaia 48, 299–308. doi: 10.1111/let.12106
Khaustov, A. A. (2015a). A new genus and species of the family Rhagidiidae (Acari: Eupodoidea) from Crimea. Int. J. Acarology 41, 617–623. doi: 10.1080/01647954.2015.1085899
Khaustov, A. A. (2015b). A new genus of the family Eupodidae (Acari: Eupodoidea) with the description of a new species from Crimea. Syst. Appl. Acarol. 20, 927–936. doi: 10.11158/saa.20.8.8
Knobloch, E., and Mai, D. H. (1986). Monographie der Früchte und Samen in der Kreide von Mitteleuropa. Praha: Vydal Ustredni ustav geol.
Krantz, G. W., and Walter, D. E. (2009). A manual of acarology, 3rd Edn. Lubbock, TX: Texas Tech University Press.
Kreiter, S., Tixier, M.-S., Croft, B. A., Auger, P., and Barret, D. (2002). Plants and leaf characteristics influencing the predaceous mite Kampimodromus aberrans (Acari: Phytoseiidae) in habitats surrounding vineyards. Environ. Entomol. 31, 648–660. doi: 10.1603/0046-225X-31.4.648
Labandeira, C. C. (2013). A paleobiologic perspective on plant–insect interactions. Curr. Opin. Plant Biol. 16, 414–421. doi: 10.1016/j.pbi.2013.06.003
Labandeira, C. C. (2021). Ecology and evolution of gall-inducing arthropods: the pattern from the terrestrial fossil record. Front. Ecol. Evol. 9:632449. doi: 10.3389/fevo.2021.632449
Labandeira, C. C., and Currano, E. D. (2013). The fossil record of plant-insect dynamics. Annu. Rev. Earth Planet. Sci. 41, 287–311. doi: 10.1146/annurev-earth-050212-124139
Labandeira, C. C., Johnson, K. R., and Wilf, P. (2002). Impact of the terminal Cretaceous event on plant–insect associations. Proc. Natl. Acad. Sci. U.S.A. 99, 2061–2066. doi: 10.1073/pnas.042492999
Labandeira, C. C., Wilf, P., Johnson, K. R., and Marsh, F. (2007). Guide to Insect (and other) Damage Types on Compressed Plant Fossils, Version 3.0. Washington, DC: Smithsonian Institution.
Law, Y.-W., and Wu, Y.-F. (1982). A preliminary study on Sabiaceae of China. J. Syst. Evol. 20, 421–438.
Lee, D. E., Bannister, J. M., Raine, J. I., and Conran, J. G. (2010). Euphorbiaceae: acalyphoideae fossils from early Miocene New Zealand: Mallotus–Macaranga leaves, fruits, and inflorescence with in situ Nyssapollenites endobalteus pollen. Rev. Palaeobot. Palynol. 163, 127–138. doi: 10.1016/j.revpalbo.2010.10.002
Lee, D. E., Conran, J. G., Lindqvist, J. K., Bannister, J. M., and Mildenhall, D. C. (2012). New Zealand Eocene, Oligocene and Miocene macrofossil and pollen records and modern plant distributions in the Southern Hemisphere. Bot. Rev. 78, 235–260. doi: 10.1007/s12229-012-9102-7
Li, G., Kohn, B., Sandiford, M., Xu, Z., and Wei, L. (2015). Constraining the age of Liuqu Conglomerate, southern Tibet: implications for evolution of the India–Asia collision zone. Earth Planet. Sci. Lett. 426, 259–266. doi: 10.1016/j.epsl.2015.06.010
Li, T. Q., Cao, H. J., Kang, M. S., Zhang, Z. X., Zhao, N., and Zhang, H. (2011). Pollen Flora of China. Woody Plants by SEM. Beijing: Science Press.
Li, X.-K., Tan, X. H., Gao, Z. Y., and Yao, J. C. (2004). The geological period and sedimentation environment Mangbang formation in Tengchong area, Yunnan. Yunnan Geol. 23, 241–251.
Liu, X., Manchester, S. R., and Jin, J. (2014). Alnus subgenus Alnus in the Eocene of western North America based on leaves, associated catkins, pollen, and fruits. Am. J. Bot. 101, 1925–1943. doi: 10.3732/ajb.1400228
Maccracken, S. A., Miller, I. M., and Labandeira, C. C. (2019). Late Cretaceous domatia reveal the antiquity of plant-mite mutualisms in flowering plants. Biol. Lett. 15:20190657. doi: 10.1098/rsbl.2019.0657
MacGinitie, H. D. (1941). A Middle Eocene Flora From the Central Sierra Nevada. Washington, DC: Carnegie Inst. Wash. Publ.
MacGinitie, H. D. (1962). The Kilgore flora. A Late Miocene flora from northern Nebraska. Univ. Calif. Publ. Geol. Sci. 35, 67–158.
Mai, D. H. (1964). Die Mastixioideen-Floren im Tertiär der Oberlausitz. Paläontol. Abh. Abt. B 2, 1–192.
Mai, D. H. (1973). Die Revision der Originale von R. Ludwig 1857 – ein Beitrag zur Flora des unteren Villafranchien. Acta Palaeobot. 14, 89–117.
Mai, D. H. (1976). Fossile Früchte und Samen aus dem Mitteleozän des Geiseltales. Abh. Zent. Geol. Inst. Berlin. 26, 93–149.
Mai, D. H. (1987). Neue Früchte und Samen aus paläozänen Ablagerungen Mitteleuropas. Feddes Report 98, 197–229. doi: 10.1002/fedr.4910980303
Mai, D. H., and Palamarev, E. (1997). Neue Paläofloristische Funde aus Kontenentalen und Brackischen Tertiärformationen in Bulgarien. Feddes Rep. 108, 481–506. doi: 10.1002/fedr.19971080702
Mai, D. H., and Walther, H. (1978). Die Floren der Haselbacher Serie im Weißelster-Becken (Bezirk Leipzig, DDR). Abhandlungen Staatlichen Mus. Mineralogie Geologie Dresden 28, 1–200.
Manchester, S. R. (1994). Fruits and Seeds of the Middle Eocene Nut Beds Flora, Clarno Formation, Oregon. Palaeontogr. Am. 58:205.
Manchester, S. R. (2014). Revisions to Roland Brown’s North American Paleocene flora. Acta Mus. Nat. Pragae Ser. B Hist. Nat. 70, 153–210. doi: 10.14446/AMNP.2014.153
Manchester, S. R., and McIntosh, W. C. (2007). Late Eocene silicified fruits and seeds from the John Day Formation near Post, Oregon. PaleoBios 27, 7–17.
Martinetto, E. (1994). Analisi Paleocarpologica dei depositi continentali Pliocenici della Stura di Lanzo. Boll. Mus. Regionale Sci. Nat. Torino 12, 137–172.
Martinetto, E. (1998). “East Asian elements in the Plio–Pleistocene floras of Italy,” in Proc. Int. Symp. Floristic charact. Diversity East Asian Plants, eds A. Zhang and W. Sugong (Berlin: Spring–Verlag), 71–87.
Martinetto, E. (2001a). Studies on some exotic elements of the Pliocene floras of Italy. Palaeontogr. B 259, 149–166.
Martinetto, E. (2001b). The role of Central Italy as a centre of refuge for thermophilous plants in the Late Cenozoic. Acta Palaeobot. 41, 299–319.
Miki, S. (1941). On the change of flora in eastern Asia since the Tertiary Period (1). The clay or lignite beds flora in Japan with special reference to the Pinus trifolia beds in Central Hondo. Jpn J. Bot. 11, 237–303.
Miyochi, N., Fujiki, T., and Kimura, H. (2011). Pollen flora of Japan. Sapporo: Hokkaido University Press.
Mondal, M. (1983). Pollen Morphology and Systematic Relationships of Families Sabiaceae (s.l.) and Connaraceae. PhD thesis. Calcutta: University of Calcutta.
Monks, A., O’Connell, D. M., Lee, W. G., Bannister, J. M., and Dickinson, K. J. M. (2007). Benefits associated with the domatia mediated tritrophic mutualism in the shrub Coprosma lucida. Oikos 116, 873–881. doi: 10.1111/j.0030-1299.2007.15654.x
Nishida, S., Naiki, A., and Nishida, T. (2005). Morphological variation in leaf domatia enables coexistence of antagonistic mites in Cinnamomum camphora. Canad. J. Bot. 83, 93–101. doi: 10.1139/b04-152
Norton, A. P., English-Loeb, G., and Belden, E. (2001). Host plant manipulation of natural enemies: leaf domatia protect beneficial mites from insect predators. Oecologia 126, 535–542. doi: 10.1007/s004420000556
Norton, A. P., English-Loeb, G., Gadoury, D., and Seem, R. C. (2000). Mycophagous mites and foliar pathogens: leaf domatia mediate tritrophic interactions in grapes. Ecology 81:490.
O’Connell, D. M., Lee, W. G., Monks, A., and Dickinson, K. J. M. (2010). Does microhabitat structure affect foliar mite assemblages? Ecol. Entomol. 35, 317–328. doi: 10.1111/j.1365-2311.2010.01185.x
O’Dowd, D. J., Brew, C. R., Christophel, D. C., and Norton, R. A. (1991). Mite-plant associations from the Eocene of southern Australia. Science 252, 99–101. doi: 10.1126/science.252.5002.99
O’Dowd, D. J., and Pemberton, R. W. (1994). Leaf domatia in Korean plants: floristics, frequency, and biogeography. Vegetatio 114, 137–148.
O’Dowd, D. J., and Pemberton, R. W. (1998). Leaf domatia and foliar mite abundance in broadleaf deciduous forest of north Asia. Am. J. Bot. 85, 70–78. doi: 10.2307/2446556
O’Dowd, D. J., and Willson, M. F. (1989). Leaf domatia and mites on Australasian plants: ecological and evolutionary implications. Biol. J. Linn. Soc. 37, 191–236. doi: 10.1111/j.1095-8312.1989.tb01901.x
O’Dowd, D. J., and Willson, M. F. (1991). Associations between mites and leaf domatia. Trends Ecol. Evol. 6, 179–182. doi: 10.1016/0169-5347(91)90209-G
Olivier, P. A. S., and Theron, P. D. (2000). Pentapalpidae, a new family of eupodoid mites (Prostigmata: Eupodoidea) from South Africa. Acarologia 40, 385–392.
Ozaki, K. (1980). Late Miocene Tatsumitoge flora of Tottori Prefecture, Southwest Honshu (III). Sci. Rep. Yokohama Nat. Univ. Sec. 2, 19–45.
Ozaki, K. (1991). Late Miocene and Pliocene Floras in Central Honshu, Japan. Yokohama: Bull. Kanagawa Pref. Mus. Nat. Sci., Spec. Iss. Yokohama.
Pavlyutkin, B. I, Chekryzhov, I. Y., and Petrenko, T. I (2012). Geologija i Flora Nizhnego Miocena Juga Primor’ja. Vladivostok: Dalnauka.
Pavlyutkin, B. I., Chekryzhov, I. U., and Petrenko, T. I. (2014). Geologija i Flory Nizhnego Oligocena Primor’ja. Vladivostok: Dalnauka.
Pemberton, R. W., and Turner, C. E. (1989). Occurrence of predatory and fungivorous mites in leaf domatia. Am. J. Bot. 76, 105–112. doi: 10.1002/j.1537-2197.1989.tb11290.x
Peppe, D. J., and Hickey, L. J. (2014). Fort Union Formation fossil leaves (Paleocene, Williston Basin, North Dakota, USA) indicate evolutionary relationships between Paleocene and Eocene plant species. Bull. Peabody Mus. Nat. Hist. 55, 171–189. doi: 10.3374/014.055.0209
Ramos, E., and Cornejo, X. (2012). Three new taxa of Meliosma (Sabiaceae) Neotropical Sabiaceae: three new species and a new variety of Meliosma. Harvard Pap. Bot. 17, 283–293. doi: 10.3100/025.017.0208
Raven, P. H., and Axelrod, D. I. (1974). Angiosperm biogeography and past continental movements. Ann. Miss. Bot. Gard. 61, 539–673. doi: 10.2307/2395021
Reid, E. M., and Chandler, M. E. J. (1933). The London Clay flora. London: Brit. Mus. Nat. Hist, doi: 10.5962/bhl.title.110147
Romero, G. Q., and Benson, W. W. (2004). Leaf domatia mediate mutualism between mites and a tropical tree. Oecologia 140, 609–616. doi: 10.1007/s00442-004-1626-z
Romero, G. Q., and Benson, W. W. (2005). Biotic interactions of mites, plants and leaf domatia. Curr. Opp. Plant Biol. 8, 436–440. doi: 10.1016/j.pbi.2005.05.006
Ronse de Craene, L. P., and Wanntorp, L. (2008). Morphology and anatomy of the flower of Meliosma (Sabiaceae): implications for pollination biology. Plant Syst. Evol. 271, 79–91. doi: 10.1007/s00606-007-0618-y
Rowles, A. D., and O’Dowd, D. J. (2009). Leaf domatia and protection of a predatory mite Typhlodromus doreenae Schicha (Acari: Phytoseiidae) from drying humidity. Austral. J. Entomol. 48, 276–281. doi: 10.1111/j.1440-6055.2009.00716.x
Ryu, M. O., and Ehara, S. (1991). Three phytoseiid mites from Korea (Acari: Phytoseiidae). Acta Arachnol. 40, 23–30. doi: 10.2476/asjaa.40.23
Schmidt, R. A. (2014). Leaf structures affect predatory mites (Acari: Phytoseiidae) and biological control: a review. Exp. App. Acarol. 62, 1–17. doi: 10.1007/s10493-013-9730-6
Situngu, S., and Barker, N. P. (2017). Position, position, position: Mites occupying leaf domatia are not uniformly distributed in the tree canopy. South Afr. J. Bot. 108, 23–28. doi: 10.1016/j.sajb.2016.09.012
Soltis, D. E., Senters, A. E., Zanis, M. J., Kim, S., Thompson, J. D., Soltis, P. S., et al. (2003). Gunnerales are sister to other core eudicots: implications for the evolution of pentamery. Am. J. Bot. 90, 461–470. doi: 10.3732/ajb.90.3.461
Spicer, R. A., Yang, J., Herman, A. B., Kodrul, T. M., Aleksandrova, G. N., Maslova, N. P., et al. (2017). Paleogene monsoons across India and South China: drivers of biotic change. Gondwana Res. 49, 350–363.
Stevens, P. F. (2017). Angiosperm Phylogeny Website. Version 14, July 2017. Available online at: http://www.mobot.org/MOBOT/research/APweb (accessed August 25, 2021).
Suzuki, M., and Watari, S. (1994). Fossil wood flora of the early Miocene Nawamata formation of Monzen, Noto Peninsula, central Japan. J. Plant Res. 107, 63–76. doi: 10.1007/BF02344531
Takhtajan, A. L. (1966). Sistema i Filogeniia Tsvetkovykh Rastenii. Systema et Phylogenia Magnoliophytorum. Moscow: Nauka.
Takhtajan, A. L. (1997). Diversity and Classification of Flowering Plants. New York, NY: Columbia Univ. Pres.
Tao, J. R. (1988). Plant Fossils From Liuqu Formation in Lhaze County, Xizang and their Paleoclimatological Significances. The paper collection of the Institute of Geology, Chinese Academy of Sciences, No. 3. Beijing: Science Press, 223–238.
Tao, J. R., and Chen, M. H. (1983). Neogene Flora of south part of the watershed of Salween-Mekong-Yangtze Rivers (the Lincang Region), Yunnan, in Studies in Qinghai-Xizang Plateau– Comprehensive Scientific Expedition to the Qinghai-Xizang Plateau, special Issue of Hengduan Mountains Scientific Expedition (I). Kunming: Chinese Academy of Sciences (Yunnan People’s Press), 74–89.
Tao, J. R., and Du, N. Q. (1982). Neogene Flora of Tengchong Basin in eastern Yunnan, China. Acta Bot. Sin. 24, 273–281.
Tao, J. R., Zhou, Z. K., and Liu, Y. S. (2000). The Evolution of the Late Cretaceous–Cenozoic Floras in China. Beijing: Science Press.
Van Beusekom, C. F. (1971). Revision of Meliosma (Sabiaceae), sect. Lorenzanea excepted, living and fossil, geography and phylogeny. Blumea 19, 355–529.
Walter, D. (2017). Little houses in big trees: an Aesop’s fable with plants. Wildlife Austral. 54, 26–31.
Walter, D. E., and Behan-Pelletier, V. (1999). Mites in forest canopies: filling the size distribution shortfall? Annu. Rev. Entomol. 44, 1–19. doi: 10.1146/annurev.ento.44.1.1
Walter, D. E., and Denmark, H. A. (1991). Use of leaf domatia on wild grape (Vitis munsoniana) by arthropods in Central Florida. Florida Entomol. 74, 440–446. doi: 10.2307/3494838
Walter, D. E., and O’Dowd, D. J. (1992a). Leaf morphology and predators: effect of leaf domatia on the abundance of predatory mites (Acari: Phytoseiidae). Environ. Entomol. 21, 478–484. doi: 10.1093/ee/21.3.478
Walter, D. E., and O’Dowd, D. J. (1992b). Leaves with domatia have more mites. Ecology 73, 1514–1518. doi: 10.2307/1940694
Wang, J. D., Li, H. M., Zhu, Z. Y., Maurice, K. S., Yang, J. F., and Zhang, G. M. (1994). Magnetostratigraphy of Tertiary rocks from Maoming Basin, Guangdong Province, China. Chin. J. Geochem. 13:166. doi: 10.1007/BF02838516
Wanntorp, L., and Ronse de Craene, L. P. (2007). Flower development of Meliosma (Sabiaceae): Evidence for multiple origins of pentamery in the eudicots. Am. J. Bot. 94, 1828–1836. doi: 10.3732/ajb.94.11.1828
Wappler, T. (2010). Insect herbivory close to the Oligocene–Miocene transition — a quantitative analysis. Palaeogeogr. Palaeoclimatol. Palaeoecol. 292, 540–550. doi: 10.1016/j.palaeo.2010.04.029
Wappler, T., and Grímsson, F. (2016). Before the ‘Big Chill’: Patterns of plant-insect associations from the Neogene of Iceland. Glob. Planet. Change 142, 73–86. doi: 10.1016/j.gloplacha.2016.05.003
Wappler, T., Labandeira, C. C., Rust, J., Frankenhäuser, H., and Wilde, V. (2012). Testing for the effects and consequences of mid Paleogene climate change on insect herbivory. PLoS One 7:e40744. doi: 10.1371/journal.pone.0040744
Watari, S. (1949). Studies on the fossil woods from the Tertiary of Japan. VI. Meliosma oldhami Miquel from the Miocene of Hanenisi, Simane Prefecture. Bot. Mag. Tokyo 62, 83–86. doi: 10.15281/jplantres1887.62.83
Wei, L. J., Liu, X. H., Yan, F. H., Mai, X. S., Li, G. W., Liu, X. B., et al. (2011). Palynological evidence sheds new light on the age of the Liuqu Conglomerates in Tibet and its geological significance. Sci. China Earth Sci. 54, 901–911. doi: 10.1007/s11430-010-4149-y
WGCPC (Writing Group of Cenozoic Plants of China) (1978). Cenozoic Plants of China. Beijing: Science Press.
Wheeler, E. A., and Manchester, S. R. (2002). Woods of the Eocene Nut Beds Flora, Clarno Formation, Oregon, USA. IAWA J. Suppl. 3, 1–188.
Wilf, P. (2008). Insect-damaged fossil leaves record food web response to ancient climate change and extinction. New Phytol. 178, 486–502. doi: 10.1111/j.1469-8137.2008.02395.x
Wilf, P., Labandeira, C. C., Johnson, K. R., and Ellis, B. (2006). Decoupled plant and insect diversity after the end-Cretaceous extinction. Science 313, 1112–1115. doi: 10.1126/science.1129569
Willson, M. F. (1991). Foliar shelters for mites in the Eastern deciduous forest. Am. Midland Natur. 126, 111–117. doi: 10.2307/2426155
Wolfe, J. A. (1977). Paleogene floras from the Gulf of Alaska region. Geol. Surv. Prof. Pap. 99, 1–108. doi: 10.3133/pp997
Worberg, A., Quandt, D., Barniske, A.-M., Löhne, C., Hilu, K. W., and Borsch, T. (2007). Phylogeny of basal eudicots: insights from non-coding and rapidly evolving DNA. Org. Divers. Evol. 7, 55–77. doi: 10.1016/j.ode.2006.08.001
World Checklist of Selected Plant Families (2021). Facilitated by the Royal Botanic Gardens, Kew. Available online at: http://wcsp.science.kew.org/ (accessed August 25, 2021).
Yang, T., Lu, L.-M., Wang, W., Li, J.-H., Manchester, S. R., Wen, J., et al. (2018). Boreotropical range expansion and long-distance dispersal explain two amphi-Pacific tropical disjunctions in Sabiaceae. Mol. Phylogenetics Evol. 124, 181–191. doi: 10.1016/j.ympev.2018.03.005
Zha, G.-C., Liang, L.-R., and Zhou, C.-Q. (2003). Community structure and diversity of arthropods in moss soil. Acta Ecol. Sinica 23, 1057–1062.
Zhang, D.-Z., and Lin, N.-Q. (2012). Preliminary investigation and systematics on the beneficial mites (Acari: Prostigmata and Endeostigmata) at tea gardens of Fujian. Wuyi Sci. J. 28, 13–22.
Zhang, J. H. (1983). Discovery of old Tertiary flora from Panxian of Guizhou and its significance. Pap. Stratigr. Paleontol. Guizhou 1, 133–141. (in Chinese, with English Abstr.),
Zúñiga, J. D. (2014). Systematics, Biogeography and Petiole Anatomy of Sabiaceae with Emphasis on Meliosma. PhD thesis. Claremont, CA: Claremont Graduate University.
Keywords: plant fossils, Meliosma, mites, Eocene, China
Citation: Moiseeva MG, Kodrul TM, Tekleva MV, Maslova NP, Wu X and Jin J (2022) Fossil Leaves of Meliosma (Sabiaceae) With Associated Pollen and a Eupodid Mite From the Eocene of Maoming Basin, South China. Front. Ecol. Evol. 9:770687. doi: 10.3389/fevo.2021.770687
Received: 04 September 2021; Accepted: 30 November 2021;
Published: 03 January 2022.
Edited by:
Daniel J. Peppe, Baylor University, United StatesReviewed by:
Ari Iglesias, Consejo Nacional de Investigaciones Científicas y Técnicas (CONICET), ArgentinaNathan Jud, William Jewell College, United States
Copyright © 2022 Moiseeva, Kodrul, Tekleva, Maslova, Wu and Jin. This is an open-access article distributed under the terms of the Creative Commons Attribution License (CC BY). The use, distribution or reproduction in other forums is permitted, provided the original author(s) and the copyright owner(s) are credited and that the original publication in this journal is cited, in accordance with accepted academic practice. No use, distribution or reproduction is permitted which does not comply with these terms.
*Correspondence: Jianhua Jin, bHNzampoQG1haWwuc3lzdS5lZHUuY24=