- Department of Ecology and Evolution, University of Lausanne, Lausanne, Switzerland
Salmonid fish have become important models in evolution and ecology, but possible effects of embryo or larval sex are mostly ignored, probably because morphological gonad formation starts only months after hatching and sexual maturation years later. However, recent gene expression studies and first observations in domestic strains suggest that sex-specific life histories could already start at an embryonic stage. Here we test this hypothesis in embryos and larvae of lake char (Salvelinus umbla). We sampled wild char and used their gametes to produce embryos of 40 different families. Embryos were raised singly in a stress or a non-stress environment until a late larval stage (stress was induced by allowing remainders of ovarian fluids to support microbial growth). Genetic markers were then used to sex the fish and reconstruct paternity (N = 1,463, including dead embryos). Primary sex ratio did not differ among families and was about 1:1. Female embryos hatched on average later and showed lower stress tolerance than male embryos. There were significant parental effects on offspring growth and mortality, but the sex differences in embryo performance were not family specific. We conclude that the sexes differ in their life history and susceptibilities to environmental stress already at embryonic stages. Environmental stress during incubation can therefore affect population sex ratio and hence population growth and genetics.
Introduction
Salmonid fish usually reach sexual maturity after their second year of life or later. At this stage, male and female differ significantly in behavior, life history, and morphology. There can also be sex differences in juveniles of migratory salmonids, with females being typically more inclined to become migratory than males (Klemetsen et al., 2003; Pearse et al., 2019). Apart from that, little is known about sex differences at embryo and juvenile stages, especially also of non-migratory salmonids. Population models typically assume that such differences do not exist or are of little relevance for demography and population growth (e.g., Nislow and Armstrong, 2012; Tsuboi et al., 2013; Louison and Stelzer, 2016). However, Morán et al. (2016) found variation in family sex ratios in crosses of three domestic brown trout (Salmo trutta) populations that they speculated could be due to sex-specific mortalities during embryogenesis. In natural populations of the same species, juvenile males were found to be more aggressive and having a lower perception of predation risks than juvenile females (Johnsson et al., 2001) and they sometimes also showed sex-specific habitat preferences (Giller and Greenberg, 2015). Bylemans et al. (in preparation) found that sex ratios in about half-year-old brown trout caught from a streamlet depended on whether the fish were hatchery or wild born. Any such early sex differences in behavior and life history can affect tolerance to various types of environmental stressors, including new ones that are linked to, for example, different types of pollution or climate change (Geffroy and Douhard, 2019). They therefore have the potential to affect adult sex ratio and hence population growth, genetics, and evolutionary potential.
Salmonid populations are often monitored over decades because of their ecological, cultural, and economic relevance. Some of these monitoring programs revealed changes in sex ratios due to, for example, sex-specific mortality during migration (Thompson et al., 2016). Others revealed significant changes in sex ratios that could statistically be linked to environmental factors such as changes in water temperatures (Geffroy and Wedekind, 2020). In the late 1980s, for example, mean temperatures changed abruptly in Europe and remained elevated since then (Reid et al., 2016). This sudden temperature change seemed to be responsible for a significant male bias in a wild and protected population of European grayling (Thymallus thymallus) (Wedekind et al., 2013). The distorted sex ratio has remained largely unchanged until today (Wedekind, unpublished observations). Interestingly, the distortion of the adult sex ratio was recorded among mostly 5-year old fish and started only 5 years after the change of the mean water temperatures (Wedekind et al., 2013). This observation, combined with the fact that grayling sex determination is genetic (Yano et al., 2013) and stable under various temperature conditions (Pompini et al., 2013) suggested that the changed environment affects sex-specific survival mainly during the first year of life, i.e., long before sex differences in behavior or life history are obvious in this species. A closer look at the timing of gonad development then revealed that the two sexes differ significantly during their first summer: sex differentiation starts early in genetic females and it is much delayed in genetic males who instead grow faster during that time (Maitre et al., 2017). Gene expression studies revealed that the sexes differ already at an embryonic stage (Maitre et al., 2017), long before morphological gonad development. Selmoni et al. (2019) therefore tested whether sex-specific gene expression during such early stages could be affected by a typical anthropogenic change of the environment, namely pollution by a synthetic estrogen at ecologically relevant concentration. They found that this form of pollution can indeed affect sex-specific gene expression at ontogenetic stages as early as the embryo and yolk-sac stages. Their findings suggest that the genetic sexes can differ in their response to environmental challenges from the earliest stages on, and that environmental conditions that affect embryo development can therefore have long-lasting effects on population sex ratios that may then potentially affect, for example, spawning behavior, population genetics, and population growth. Sex differences and their link to environmental conditions therefore need to be studied already at early developmental stages.
Here we focus on embryos and yolk-sac larvae of another salmonid, the lake char (Salvelinus umbla) from Lake Geneva (Switzerland). This population has been exposed to the typical challenges of lakes of the Swiss Plateau that include eutrophication (Feulner and Seehausen, 2019), pollution by toxic metals or organic matter deposition (Diez et al., 2017), and a significant increase in winter temperatures (Kelly et al., 2020) that not only affects embryo development rates but also sediment load and its effects on embryos (Mari et al., 2016). We test whether the genetic sexes differ in mortality, embryo development, timing of hatching, and larval growth in a stress and a non-stress environment. Previous experiments on other salmonids established that exposure of embryos to organic nutrients supports growth of symbiotic microbial communities (Wedekind et al., 2010) and affects their compositions and functional pathways (Wilkins et al., 2016). Such nutrient-induced changes then leads to net increases in total virulence (Jacob et al., 2010; Wedekind et al., 2010; Wilkins et al., 2016, 2017), either because of additive effects linked to increased microbial density or changed microbial compositions, or because of density-dependent transitions of microbial life histories (Diggle et al., 2007). Because ovarian fluids are rich of carbohydrates, amino acids, and other organic and inorganic components that support for the growth of microbial communities (Lahnsteiner et al., 1995), we induced such environmental stress by varying the level of remainders of ovarian fluids on freshly fertilized eggs.
Materials and Methods
Sampling of Breeders and Production Families
Ten adult males and 4 females were caught during their spawning season in Lake Geneva. Their gametes were stripped and used in a study on male and female reproductive strategies (Nusbaumer et al., 2021b). Briefly, the eggs and ovarian fluids of a female were stripped into a container from which ovarian fluids (as much as possible) were extracted with a syringe before washing the eggs twice with Ovafish® (IMV Technologies, l’Aigle, France; the content of the container was each topped up to 200 mL before discarding the Ovafish). Twenty batches of 24 eggs per female (80 batches in total) were then separated in wells of 6-well plates (Falcon, BD Biosciences, Allschwil, Switzerland). The ovarian fluids of each female were diluted with standardized water to a ratio of 1:2 (ovarian fluid to water). The milt of each male was diluted in the inactivating medium Storfish (IMV Technologies, France) to 50 million active sperm per mL. The males were assigned to 5 haphazardly chosen pairs of competitors, and the milt of each pair of competitors was mixed equally. Each batch of 24 eggs was then exposed to 1 mL of such a mix of milt that had been then activated in either 4 mL of standardized water or in 4 mL of diluted ovarian fluids (of the female the eggs were from). After 2 min (when fertilization can be expected to have happened), 16.8 mL of standardized water was added to each well and the eggs were left undisturbed for 2 h to allow for hardening before immediate transport to the laboratory.
This experimental design of these sperm competition trials was full-factorial and fully balanced and finally led to 40 different full-sib families (each female crossed with each male) after paternity had been determined with genetic markers (see below). However, 10 of the 80 batches (all of the same female, prepared to be exposed to sperm activated in water only) were lost due to a handling accident, leaving 1,680 eggs that could be monitored.
Monitoring of Embryos and Larvae
After fertilization, the freshly fertilized eggs were transported to the laboratory to be incubated for later parental analyses (Nusbaumer et al., 2021b). Briefly, eggs were rinsed for 30 s under cold running tap water (4 L/min) in a sterilized tea strainer before being distributed singly in 24 well plates (Falcon, BD Biosciences, Allschwil, Switzerland) filled with 1.8 mL of autoclaved standardized water (OECD, 1992), and incubated at 4.5°C in a 12 h:12 h light-dark cycle (to simplify monitoring and minimize variation of light conditions) until 14 days post-hatching as in von Siebenthal et al. (2009). Under these environmental conditions, embryo mortality until hatching is typically < 3% in similar-sized eggs of brown trout (Nusbaumer et al., 2021c). We considered a short rinsing of the eggs necessary because we had previously observed that not rinsing eggs before incubation in 24-well plates can lead to very high embryo mortalities (Wedekind, unpublished observations).
Two weeks after fertilization, fertilization success was estimated by the presence of absence of a neurula in the egg, and embryo mortality was monitored from then on. At the day of hatching, yolk-sac larvae were transferred to 12-well plates filled with 5 mL autoclaved standardized water and larval mortality and malformations (Supplementary Figure 1) were recorded until 14 days after hatching. Dead embryos and dead larvae were transferred to 2 mL microtubes filled with 1 mL 96% ethanol, and stored at −20°C for further analysis.
On the day of hatching and 14 days later, larvae were photographed in a custom-made photo box with a digital camera from below the 12-well plate (Canon 70D, 50 mm, f/3.2, 1/400 s, ISO 200, WB 6000 K, RAW format 24 Mpx). After the second photo was taken, larvae were euthanized with a spike of 100 μL of Koi Med® Sleep 4.85% (Koi & Bonsai Zimmermann, Germany), transferred to 2 mL microtubes filled with 1 mL 96% ethanol, and stored at −20°C for further analysis. Photos were analyzed in ImageJ (Schneider et al., 2012). At each stage (hatching day, 14 days post-hatching), the standard body length was measured (from snout to the base of the caudal fin), and the minor and major axis of the yolk sac were measured to infer yolk sac volume as in Jensen et al. (2008).
Paternity Analyses and Genetic Sexing
In total 1,475 embryos and larvae of known maternal origin were genotyped in one or two multiplexes that each contained 3 polymorphic microsatellite markers (Savary et al., 2017). The second multiplex was only used if the first one was not sufficient yet for identifying the paternal origin. The detailed protocol is described in Nusbaumer et al. (2021b). Briefly, DNA was extracted from unhatched embryos, larvae and parents. Genotypes were read using Genemapper v4.0 (Applied Biosystems, United States), and paternity was assigned using CERVUS v3.0 (Kalinowski et al., 2007). Peak heights in adult males (n = 10) that were genotyped twice ranged from 1,513 to 3,743 in replicated PCRs and showed high repeatability (r2 = 0.95).
The assignment to fathers was used in Nusbaumer et al. (2021b) to evaluate the competitiveness of sperm. In the present study, the family assignments are used to test for the maternal and paternal influence on the performance of male and female offspring. An sdY sex marker (226 bp; primers: 5′-CCCAGCACTGTTTTCTTGTCTCA-3′ and 5′-CTTAAAACCA CTCCACCCTCCAT-3′) (Yano et al., 2013) was therefore added to the first multiplex to identify the genetic sex of each embryo or larva. Successful amplification of the sdY marker as observed in Genemapper was interpreted as male, while no amplification of the sdY marker was interpreted as female if the three other loci of the multiplex were successfully amplified. In total 1,463 offspring could be assigned to both parents and genetically sexed (amplification of the first multiplex that included the sdY marker failed for one offspring that could nevertheless be assigned to a father with the second multiplex).
Statistical Analyses
All analyses were done in RStudio (R Development Core Team, 2015) and JMP 15.2.1. General (GLMM) and linear (LMM) mixed-effects models were run in the package lme4 (Bates et al., 2015). Categorical traits such as sex, mortality until hatching, and malformation were analyzed in GLMM fitted with a binomial error distribution. Continuous variables were analyzed in LMM fitted with maximum likelihood. For all models, the model assumptions were visually checked in Q-Q plots and residuals plots. Sex and experimental treatment (presence or absence of ovarian fluids during fertilization) were entered as fixed effects, while sire and dam identities were random effects. In models on embryo growth, length at hatching was added as fixed effect to account for variability in initial length. The significance of model terms was evaluated in likelihood ratio tests. Terms of interest were dropped or added and the new model was compared to the reference model using likelihood ratio tests. Adding terms of interests allowed testing for possible effects of two-way interactions, while higher level interactions were not tested. Larval mortality was not further analyzed because only 13 larvae died during the observational period (<1%).
Results
Sex Ratios
The 1,463 embryos that could be successfully assigned to the 40 experimental families were represented by 36.6 ± 15.8 (± SD, range 6–71) offspring per full-sib family. The overall sex ratio was 49.96% males, did not differ among paternal or maternal sib groups and was not affected by the presence or absence of ovarian fluids during fertilization (Table 1 and Figure 1). There were also no significant effects of any possible interactions between parents and treatment on sex ratio (Table 1).
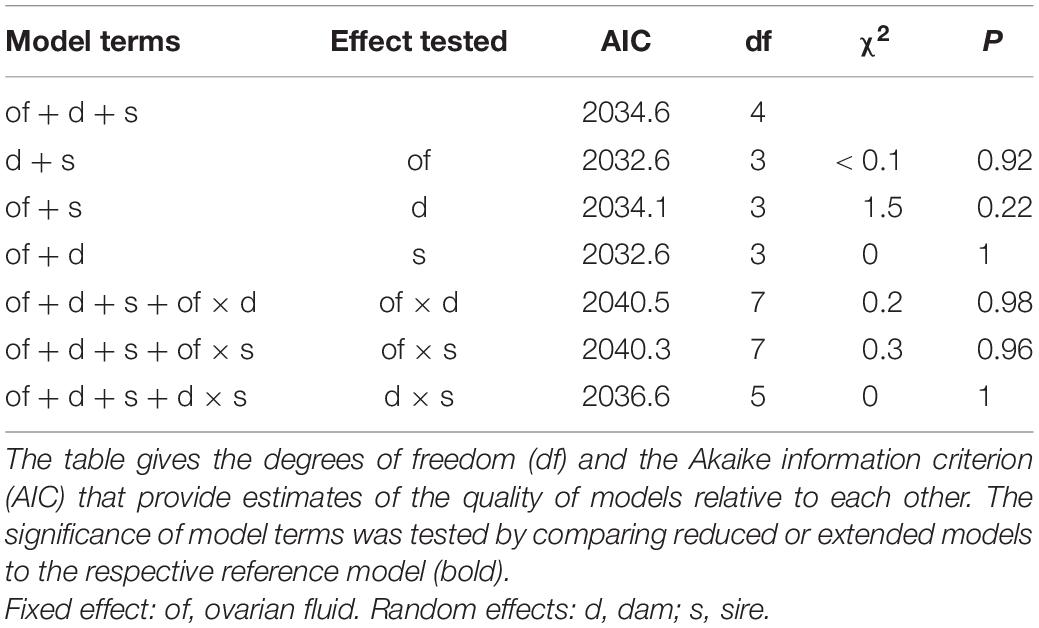
Table 1. Likelihood ratio tests on generalized mixed-effects models testing for parental and treatment effects (fertilization based on sperm competition with or without ovarian fluids) on primary sex ratios.
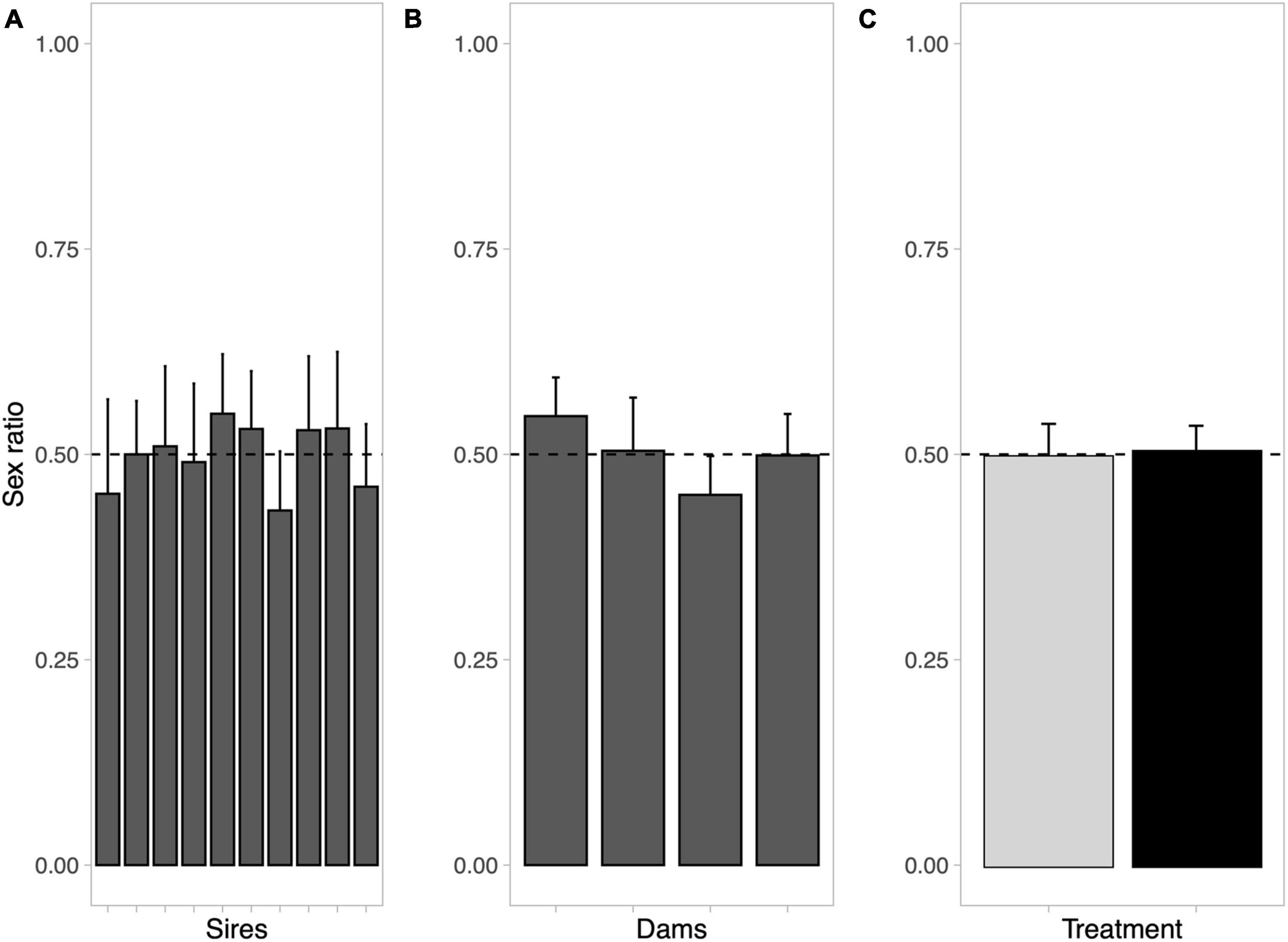
Figure 1. Average sex ratios of (A) all paternal sib groups, (B) all maternal sib groups, and (C) of embryos fertilized with water only (gray bar) or with diluted ovarian fluid (black bar). Bars indicate means per full-sib family and treatment, with 95% confidence intervals. Sex ratio range from all females (0) to all males (1). The horizontal dashed lines indicate even sex ratio.
Sex Differences in Embryo Performance
Offspring sex played a role in embryo development in the two experimental groups. There was a non-significant tendency for males to reach higher hatching rates than females (i.e., males tend to have lower embryo mortality; Table 2A). In total 38 embryos were classified as malformed (2.6%) of which 34 hatched. These malformed fish hatched on average earlier than non-malformed ones (t = −2.0, p = 0.046). Females suffered from higher malformation rates but hatched on average later than males (Tables 2B,C and Figures 2B,C). Despite the later hatching, freshly hatched females did not differ from males in body length, yolk sac volume or growth during than first 14 days after hatching (Tables 2D–F and Figures 2D–F).
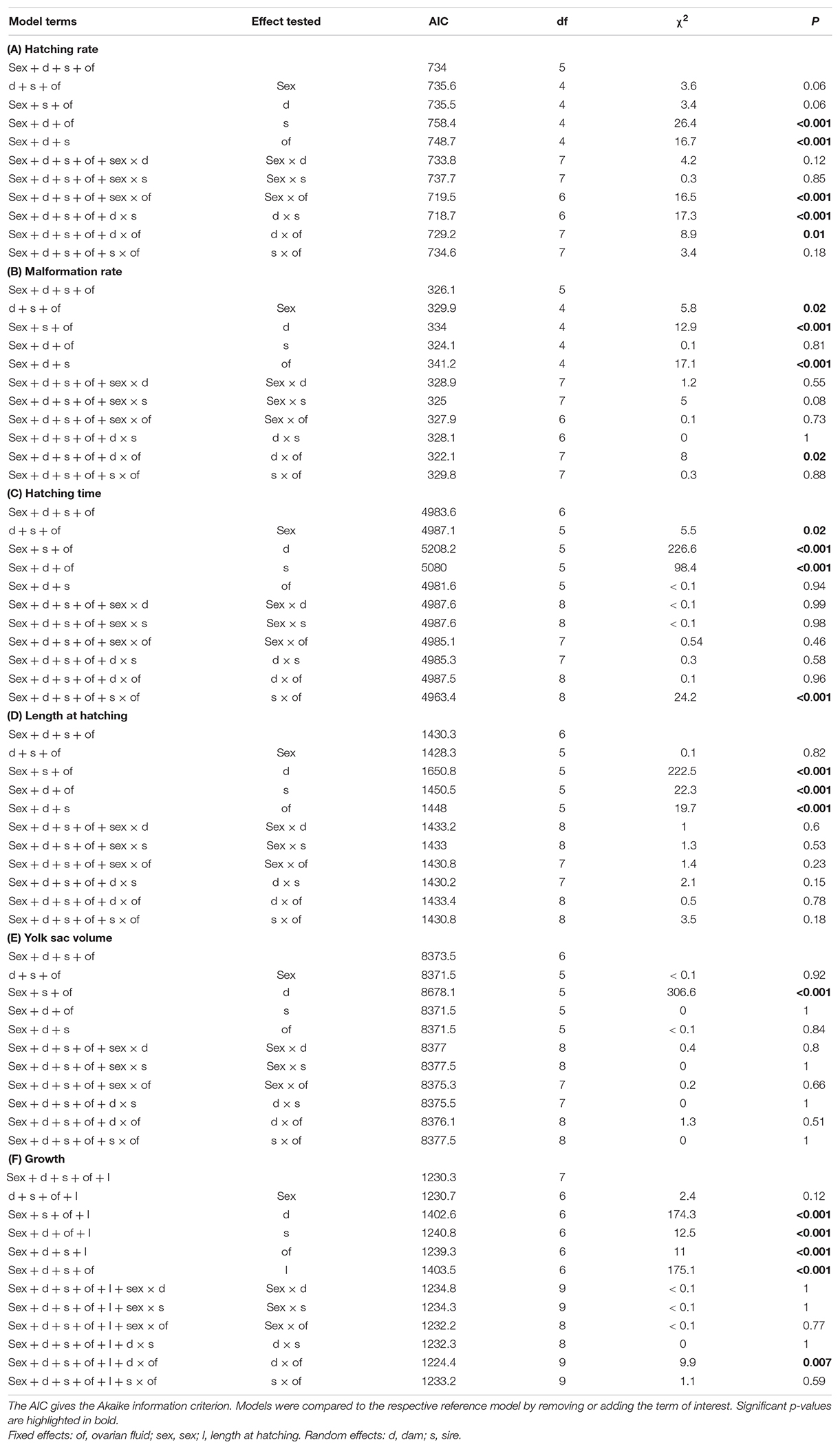
Table 2. Likelihood ratio tests on generalized mixed-effects models on (A) embryo hatching rate and (B) malformation rate, and likelihood ratio tests on linear mixed-effects models on (C) hatching time, (D) larval length at hatching, (E) yolk sac volume at hatching, and (F) increase in larval length during the first 14 days after hatching.
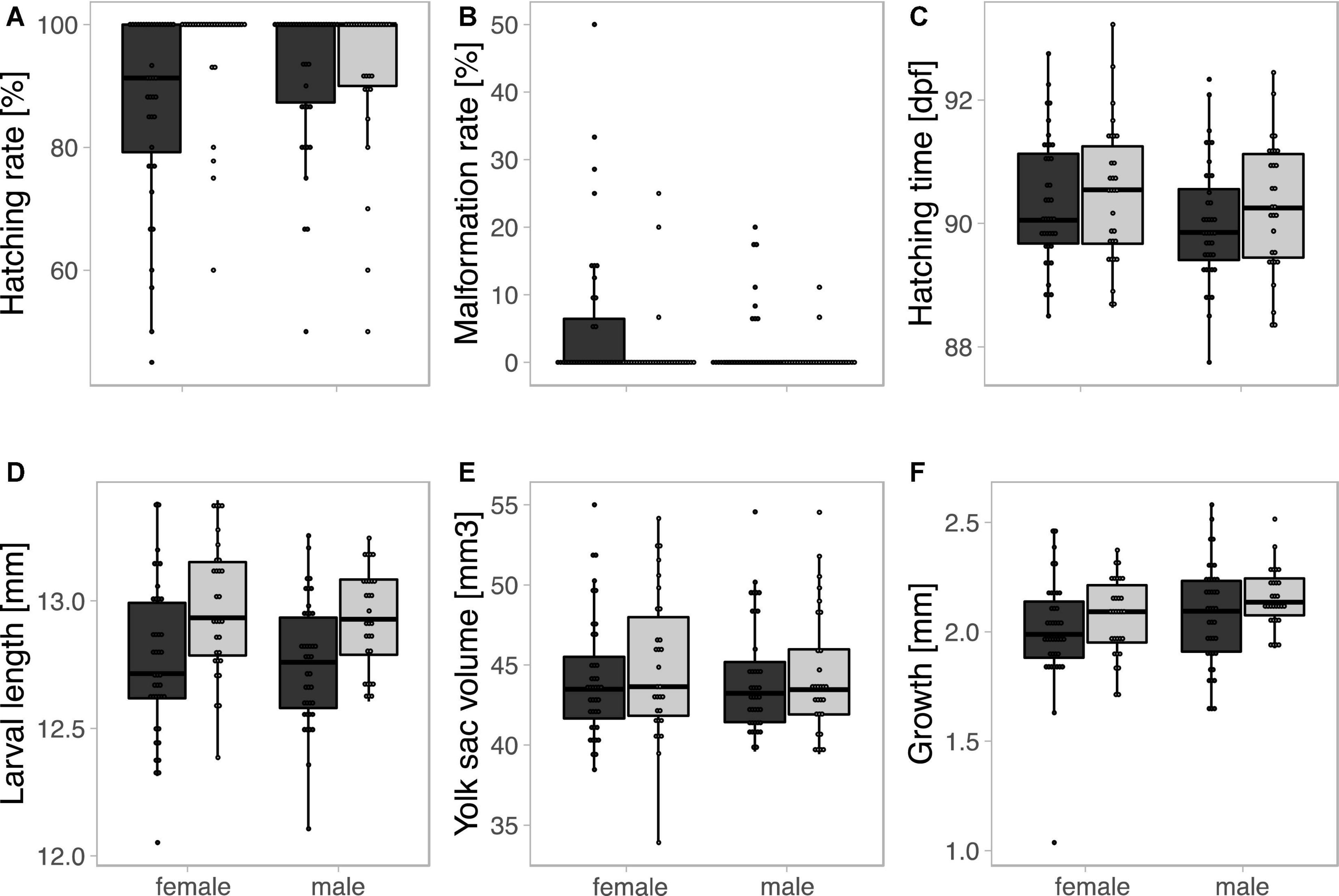
Figure 2. Effects of genetic sex and environment on (A) hatching rate (as measure of embryo survival), (B) rate of malformations among hatchlings, (C) the timing of hatching (dpf = days post-fertilization), (D) larval length at hatching, (E) yolk sac volume at hatching, and (F) increase of larval length during the first 14 days after hatching. Tukey outlier boxplots with quartiles, whiskers, and jittered family means for female and male embryos. Black and gray symbols represent stressful and non-stressful environments, respectively. See Table 2 for statistics.
The experimental treatment during gamete fusion produced significant effects on offspring performance: Embryos that resulted from fertilization in diluted ovarian fluids suffered from lower hatching rates, higher malformation rates, hatched at smaller size, and then grew slower than larvae that resulted from fertilization in water only (Table 2 and Figure 2). The two sexes reacted differently to this treatment: While hatching rates appeared unaffected in males, they significantly declined in females that resulted from gamete fusion in the ovarian fluids treatment (sex × treatment interaction in Table 2A and Figure 2A). However, the sexes’ reactions to the treatment did not produce any significant effects on embryo growth and development (Tables 2B–F and Figure 2).
Parental Effects on Embryo Performance
There were significant parental effects on all measures of embryo performance (see significant main effects of sire and dam in Table 2, and the significant sire × dam interaction in Table 2A). Sires differed in embryo survival, the timing of hatching, larval size at hatching, and larval growth after hatching (Table 2), while dams differed significantly in offspring malformation rates, timing of hatching, larval size and yolk sac volume at hatching, and larval growth after hatching (Table 2). Embryo mortality was also dependent on the combination of sire and dam (see dam × sire interaction in Table 2A).
There were significant parental effects on the strength of the treatment effects, i.e., significant dam × treatment interactions on embryo mortality, malformation rates, and larval growth after hatching (Tables 2A,B,F), and a significant sire × treatment interaction on the timing of hatching (Table C). However, effects of offspring sex were never significantly influenced by parental origin (see non-significant sex × dam and sex × sire interactions in Table 2).
Discussion
The primary sex ratio of fish with genetic sex determination can be expected to be around 1:1, i.e., 50% males in all families (Fisher, 1930). This is what we observed in our study population when sexing all offspring, including those that had died before hatching. With on average 36.6 sexed offspring per family and 40 full-sib families in total, we had much statistical power to detect potential variation in family sex ratios. We therefore conclude that the primary sex ratio is not different to 1:1 in this population of lake char. There were, however, increased rates of malformations in female larvae. We also found that the sexes differed in their susceptibility to environmental stress, i.e., that sex-specific embryo mortality depended on the rearing environment. Therefore, sex-specific embryo mortality needs to be controlled for when testing for variation in primary family sex ratios.
We found that female larvae hatched on average later than male larvae (even if they had higher rates of malformed fish that hatched earlier than non-malformed fish). Despite this difference in hatching date, both sexes hatched at similar body sizes and similar yolk sac volumes. We therefore conclude that male embryos developed faster than female embryos. After hatching, male larvae also tended to grow faster than females, but this difference was not significant.
The genetic differences between the sexes are small in salmonids and seem to be restricted to the sdY master sex determining gene that Guiguen et al. (2019b) described as “… the first example of a totally de novo evolved gene functionally capable of hijacking the leadership of the gonadal gene regulatory network” (p. 47). This novelty may at least partly explain why, in salmonids, the Y chromosomes that bear the sdY gene have not significantly degenerated as would generally be expected from Y chromosomes (Bachtrog, 2013). In rainbow trout (Oncorhynchus mykiss), the sdY gene has been found to be expressed already around hatching, i.e., long before the initiation of histological sex differentiation (Guiguen et al., 2019a). Our results suggest that sex-specific gene expression happens even earlier in lake char, as it does in grayling (Maitre et al., 2017).
Females vary in the amount and viscosity of ovarian fluids (Lahnsteiner et al., 1995; Turner and Montgomerie, 2002), and during natural spawning, eggs are swirled around to various degrees depending, for example, on male and female movements during the spawning. Gamete fusion is therefore expected to happen in various concentrations of ovarian fluids. This is what we simulated in our experimental treatment that revealed significant effects of ovarian fluids on sperm velocity and the outcome of sperm competition (Nusbaumer et al., 2021b). Here we concentrate on what happens after gamete fusion when eggs may be contaminated to various degrees by viscous and somewhat sticky residues of ovarian fluids.
Ovarian fluids of char and other salmonids contain high levels of carbohydrates including glucose and fructose, proteins and free amino acids, lactate, phospholipids, cholesterol, and various inorganic components (Lahnsteiner et al., 1995). Residues of ovarian fluids are therefore expected to support the growth of symbiotic microbial communities on freshly fertilized eggs, even after much of the ovarian fluids had been washed off the eggs before incubation (in order to avoid the very high embryo mortalities that could be expected when not rinsing eggs before incubation; Wedekind, unpublished observations). We found that embryos that resulted from fertilization trials with ovarian fluids suffered indeed from increased mortalities and malformation rates and showed reduced growth at the embryo and larval stages than eggs of which ovarian fluids had been washed of before fertilization. Analogous detrimental effects have previously been observed when nutrient broth was experimentally added to eggs of the whitefish Coregonus suitieri (Wedekind et al., 2010) or to eggs of brown trout (Jacob et al., 2010; Wilkins et al., 2016, 2017). Organic pollution, therefore, creates a stressful environment to salmonid embryos, and contamination by residues of ovarian fluids is expected to be a stressor under hatchery and laboratory conditions. For this reason, salmonids eggs are often separated from ovarian fluids in aquaculture production. It remains to be tested whether contamination by residues of ovarian fluids can also be a stressor of ecological relevance at the natural spawning ground.
We found significant parental effects on all offspring characteristics that we determined. We also found that parental effects affected the tolerance to the environmental stress that has been inflicted by the experimental treatment. Many of these effects were very strong, as expected from estimates of parental effects in other salmonids (see below). However, despite all these effects of parental origin on embryo performance, and despite the high number of replicates that we used here and that provided much statistical power, there seemed to be no parental effect on sex-specific embryo performance. The families did not differ in sex ratios and no family effects on sex-specific growth and life history were detected.
Parental effects on embryo and larval performance have been repeatedly analyzed in other salmonids, usually based on full-factorial breeding experiments with sufficient replication on the maternal and the paternal sides and large numbers of embryos that were often singly reared as in the present study (recent examples include Clark et al., 2016; Forest et al., 2016; Marques da Cunha et al., 2019; Nusbaumer et al., 2021c). Such experiments allow separating and quantifying the variance components and hence testing, for example, for the evolutionary potential of natural populations to adapt to changing environments (Lynch and Walsh, 1998). Sire effects on offspring traits then directly reveal additive genetic effects, while dam effects are a mixture of maternal environmental and genetic effects, and dam × sire interaction effects provide a good estimate of non-additive genetic effects. Our experimental treatment led to 40 different full-sib families, i.e., all families that are possible with gametes from 10 males and 4 females, and we also raised large numbers of embryos singly in 24-well plates. However, our breeding design is different from the full-factorial breeding that was used in previous experiments on salmonid fish, because the families resulted from sperm competition experiments. Sperm competitiveness can vary within the milt of a male (Sutter and Immler, 2020) and has been shown to affect offspring traits (Immler et al., 2014). We can therefore not exclude that sperm competitiveness is linked to genetic quality. If so, our experiment would not provide reliable estimates of the variance components that would result from non-competitive breeding experiments. Instead, it could provide a first estimate of variance components affected by sperm competition. In future studies, such estimates could be compared to estimates that result from non-competitive breeding to discuss the effects of sperm competition on population genetics.
Conclusion
In conclusion, we found that male and female lake char embryos differ significantly in the timing of hatching and in their tolerance to an ecologically relevant stress. These sex-specific differences happen long before histological sex differentiation starts, and years before the sexes assume their different role at the spawning place. Sex-specific susceptibilities to environmental challenges can affect growth and survival and hence adult population sex ratios. They can therefore be a reason for sex-ratio biases that may not only affect population growth (e.g., if population growth is limited by the number of females) but also population genetics by reducing the genetically effective population size and hence increasing average inbreeding coefficients. Our findings demonstrate that sex-specific susceptibilities to environmental factors can already be relevant as early as during egg incubation on the spawning ground.
Data Availability Statement
Data can be downloaded from the Dryad depository (Nusbaumer et al., 2021a).
Ethics Statement
Ethical review and approval was not required for the animal study because data were taken from embryos and larvae before the onset of exogenous feeding.
Author Contributions
DN and CW designed the study. LG and DN organized and conducted fieldwork. DN performed the in vitro fertilization, reared, and monitored embryos and larvae, did the genotyping, and analyzed the data. LG and LA measured the larvae. CW wrote a first draft of the manuscript that was revised by all authors.
Funding
Funding was provided by the Swiss National Science Foundation (31003A_159579 and 31003A_182265).
Conflict of Interest
The authors declare that the research was conducted in the absence of any commercial or financial relationships that could be construed as a potential conflict of interest.
Publisher’s Note
All claims expressed in this article are solely those of the authors and do not necessarily represent those of their affiliated organizations, or those of the publisher, the editors and the reviewers. Any product that may be evaluated in this article, or claim that may be made by its manufacturer, is not guaranteed or endorsed by the publisher.
Acknowledgments
We thank G. Levray from the Pistolet fishery for catching the fish, L. Adhia Eya, A. Atherton, C. Berney, C. de Guttry, F. Dolivo, L. Espinat, S. Kreuter, E. Longange, L. Marques da Cunha, N. Sironi and for assistance in the field or in the lab, F. Schütz for discussion on statistics, and E. Lasne for discussion and access to the INRA facilities.
Supplementary Material
The Supplementary Material for this article can be found online at: https://www.frontiersin.org/articles/10.3389/fevo.2021.768263/full#supplementary-material
References
Bachtrog, D. (2013). Y-chromosome evolution: emerging insights into processes of Y-chromosome degeneration. Nat. Rev. Genet. 14, 113–124. doi: 10.1038/nrg3366
Bates, D., Mächler, M., Bolker, B., Walker, S. (2015). Fitting linear mixed-effects models using lme4. J. Stat. Softw. 67, 1–48. doi: 10.18637/jss.v067.i01
Clark, E. S., Pompini, M., Uppal, A., and Wedekind, C. (2016). Genetic correlations and little genetic variance for reaction norms may limit potential for adaptation to pollution by ionic and nanoparticulate silver in a whitefish (Salmonidae). Ecol. Evol. 6, 2751–2762. doi: 10.1002/ece3.2088
Diez, E. G., Corella, J. P., Adatte, T., Thevenon, F., and Loizeau, J. L. (2017). High-resolution reconstruction of the 20th century history of trace metals, major elements, and organic matter in sediments in a contaminated area of Lake Geneva, Switzerland. Appl. Geochem. 78, 1–11. doi: 10.1016/j.apgeochem.2016.12.007
Diggle, S. P., Griffin, A. S., Campbell, G. S., and West, S. A. (2007). Cooperation and conflict in quorum-sensing bacterial populations. Nature 450, 411–417. doi: 10.1038/nature06279
Feulner, P. G. D., and Seehausen, O. (2019). Genomic insights into the vulnerability of sympatric whitefish species flocks. Mol. Ecol. 28, 615–629. doi: 10.1111/mec.14977
Forest, A. R., Semeniuk, C. A., Heath, D. D., and Pitcher, T. E. (2016). Additive and non-additive genetic components of the jack male life history in Chinook salmon (Oncorhynchus tshawytscha). Genetica 144, 477–485. doi: 10.1007/s10709-016-9917-y
Geffroy, B., and Douhard, M. (2019). The adaptive sex in stressful environments. Trends Ecol. Evol. 34, 628–640. doi: 10.1016/j.tree.2019.02.012
Geffroy, B., and Wedekind, C. (2020). Effects of global warming on sex ratios in fishes. J. Fish Biol. 97, 596–606. doi: 10.1111/jfb.14429
Giller, P., and Greenberg, L. (2015). The relationship between individual habitat use and diet in brown trout. Freshw. Biol. 60, 256–266. doi: 10.1111/fwb.12472
Guiguen, Y., Bertho, S., Herpin, A., and Fostier, A. (2019a). “Sex determination and sex control in salmonidae,” in Sex Control in Aquaculture, Vol. 1, eds H.-P. Wang, F. Piferrer, S.-L. Chen, and Z.-G. Shen (Hoboken, NJ: John Wiley & Sons Ltd.), 251–280.
Guiguen, Y., Fostier, A., and Herpin, A. (2019b). “Sex determination and differentiation in fish: genetic, genomic, and endocrine aspects,” in Sex Control in Aquaculture, Vol. 1, eds H.-P. Wang, F. Piferrer, S.-L. Chen, and Z.-G. Shen (Hoboken, NJ: John Wiley & Sons Ltd), 35–63. doi: 10.1002/9781119127291.ch2
Immler, S., Hotzy, C., Alavioon, G., Petersson, E., and Arnqvist, G. (2014). Sperm variation within a single ejaculate affects offspring development in Atlantic salmon. Biol. Lett. 10:20131040. doi: 10.1098/rsbl.2013.1040
Jacob, A., Evanno, G., von Siebenthal, B. A., Grossen, C., and Wedekind, C. (2010). Effects of different mating scenarios on embryo viability in brown trout. Mol. Ecol. 19, 5296–5307. doi: 10.1111/j.1365-294X.2010.04884.x
Jensen, L. F., Hansen, M. M., Pertoldi, C., Holdensgaard, G., Mensberg, K.-L. D., and Loeschcke, V. (2008). Local adaptation in brown trout early life-history traits: implications for climate change adaptability. Proc. R. Soc. B Biol. Sci. 275, 2859–2868. doi: 10.1098/rspb.2008.0870
Johnsson, J. I., Sernland, E., and Blixt, M. (2001). Sex-specific aggression and antipredator behaviour in young brown trout. Ethology 107, 587–599. doi: 10.1046/j.1439-0310.2001.00682.x
Kalinowski, S. T., Taper, M. L., and Marshall, T. C. (2007). Revising how the computer program CERVUS accommodates genotyping error increases success in paternity assignment. Mol. Ecol. 16, 1099–1106. doi: 10.1111/j.1365-294X.2007.03089.x
Kelly, S., Moore, T. N., de Eyto, E., Dillane, M., Goulon, C., Guillard, J., et al. (2020). Warming winters threaten peripheral Arctic charr populations of Europe. Clim. Change 163, 599–618. doi: 10.1007/s10584-020-02887-z
Klemetsen, A., Amundsen, P. A., Dempson, J. B., Jonsson, B., Jonsson, N., O’Connell, M. F., et al. (2003). Atlantic salmon Salmo salar L., brown trout Salmo trutta L. and Arctic charr Salvelinus alpinus (L.): a review of aspects of their life histories. Ecol. Freshw. Fish 12, 1–59. doi: 10.1034/j.1600-0633.2003.00010.x
Lahnsteiner, F., Weismann, T., and Patzner, R. A. (1995). Composition of the ovarian fluid in 4 salmonid species: Oncorhynchus mykiss, Salmo trutta f lacustris, Saivelinus alpinus and Hucho hucho. Reprod. Nutr. Dev. 35, 465–474. doi: 10.1051/rnd:19950501
Louison, M. J., and Stelzer, R. S. (2016). Use of first-order tributaries by brown trout (Salmo trutta) as nursery habitat in a cold water stream network. Ecol. Freshw. Fish 25, 133–140. doi: 10.1111/eff.12197
Lynch, M., and Walsh, B. (1998). Genetics and Analysis of Quantitative Traits. Sunderland, MA: Sinauer Associates Inc.
Maitre, D., Selmoni, O. M., Uppal, A., Marques da Cunha, L., Wilkins, L. G. E., Roux, J., et al. (2017). Sex differentiation in grayling (Salmonidae) goes through an all-male stage and is delayed in genetic males who instead grow faster. Sci. Rep. 7:15024. doi: 10.1038/s41598-017-14905-9
Mari, L., Garaud, L., Evanno, G., and Lasne, E. (2016). Higher temperature exacerbates the impact of sediments on embryo performances in a salmonid. Biol. Lett. 12:20160745. doi: 10.1098/rsbl.2016.0745
Marques da Cunha, L., Maitre, D., and Wedekind, C. (2019). Low adaptive potential for tolerance to ethynylestradiol, but also low toxicity, in a grayling population (Thymallus thymallus). BMC Evol. Biol. 19:227. doi: 10.1186/s12862-019-1558-1
Morán, P., Labbé, L., and Garcia de Leaniz, C. (2016). The male handicap: male-biased mortality explains skewed sex ratios in brown trout embryos. Biol. Lett. 12:20160693. doi: 10.1098/rsbl.2016.0693
Nislow, K. H., and Armstrong, J. D. (2012). Towards a life-history-based management framework for the effects of flow on juvenile salmonids in streams and rivers. Fish. Manag. Ecol. 19, 451–463. doi: 10.1111/j.1365-2400.2011.00810.x
Nusbaumer, D., Garaud, L., de Guttry, C., Ançay, L., and Wedekind, C. (2021b). Sperm of more colorful males are better adapted to ovarian fluids in lake char (Salmonidae). bioRxiv [Preprint]. doi: 10.1101/2021.10.02.462848
Nusbaumer, D., Marques da Cunha, L., and Wedekind, C. (2021c). Testing for population differences in evolutionary responses to pesticide pollution in brown trout (Salmo trutta). Evol. Appl. 14, 462–475. doi: 10.1111/eva.13132
Nusbaumer, D., Garaud, L., Ançay, L., and Wedekind, C. (2021a). Data from: sex-specific stress tolerance in embryos of lake char (Salvelinus umbla). Dryad. doi: 10.5061/dryad.gqnk98sp3
OECD (1992). Guideline for Testing of Chemicals 203 (Fish Acute Toxicity Test). Paris: OECD Publishing.
Pearse, D. E., Barson, N. J., Nome, T., Gao, G., Campbell, M. A., Abadía-Cardoso, A., et al. (2019). Sex-dependent dominance maintains migration supergene in rainbow trout. Nat. Ecol. Evol. 3, 1731–1742. doi: 10.1038/s41559-019-1044-6
Pompini, M., Buser, A. M., Thali, M. R., von Siebenthal, B. A., Nusslé, S., Guduff, S., et al. (2013). Temperature-induced sex reversal is not responsible for sex-ratio distortions in grayling Thymallus thymallus or brown trout Salmo trutta. J. Fish Biol. 83, 404–411. doi: 10.1111/jfb.12174
R Development Core Team (2015). R: A Language and Environment for Statistical Computing. Vienna: R Foundation for Statistical Computing.
Reid, P. C., Hari, R. E., Beaugrand, G., Livingstone, D. M., Marty, C., Straile, D., et al. (2016). Global impacts of the 1980s regime shift. Glob. Chang. Biol. 22, 682–703. doi: 10.1111/gcb.13106
Savary, R., Dufresnes, C., Champigneulle, A., Caudron, A., Dubey, S., Perrin, N., et al. (2017). Stocking activities for the Arctic charr in Lake Geneva: genetic effects in space and time. Ecol. Evol. 7, 5201–5211. doi: 10.1002/ece3.3073
Schneider, C. A., Rasband, W. S., and Eliceiri, K. W. (2012). NIH Image to ImageJ: 25 years of image analysis. Nat. Methods 9, 671–675. doi: 10.1038/nmeth.2089
Selmoni, O. M., Maitre, D., Roux, J., Wilkins, L. G. E., Marques da Cunha, L., Vermeirssen, E. L. M., et al. (2019). Sex-specific changes in gene expression in response to estrogen pollution around the onset of sex differentiation in grayling (Salmonidae). BMC Genomics 20:583. doi: 10.1186/s12864-019-5955-z
Sutter, A., and Immler, S. (2020). Within-ejaculate sperm competition. Philos. Trans. R. Soc. B Biol. Sci. 375:20200066. doi: 10.1098/rstb.2020.0066
Thompson, N. F., Leblanc, C. A., Romer, J. D., Schreck, C. B., Blouin, M. S., and Noakes, D. L. G. (2016). Sex-biased survivorship and differences in migration of wild steelhead (Oncorhynchus mykiss) smolts from two coastal Oregon rivers. Ecol. Freshw. Fish 25, 642–651. doi: 10.1111/eff.12242
Tsuboi, J. I., Iwata, T., Morita, K., Endou, S., Oohama, H., and Kaji, K. (2013). Strategies for the conservation and management of isolated salmonid populations: lessons from Japanese streams. Freshw. Biol. 58, 908–917. doi: 10.1111/fwb.12096
Turner, E., and Montgomerie, R. (2002). Ovarian fluid enhances sperm movement in Arctic charr. J. Fish Biol. 60, 1570–1579. doi: 10.1111/j.1095-8649.2002.tb02449.x
von Siebenthal, B. A., Jacob, A., and Wedekind, C. (2009). Tolerance of whitefish embryos to Pseudomonas fluorescens linked to genetic and maternal effects, and reduced by previous exposure. Fish Shellfish Immunol. 26, 531–535. doi: 10.1016/j.fsi.2009.02.008
Wedekind, C., Evanno, G., Székely, T., Pompini, M., Darbellay, O., and Guthruf, J. (2013). Persistent unequal sex ratio in a population of grayling (Salmonidae) and possible role of temperature increase. Conserv. Biol. 27, 229–234. doi: 10.1111/j.1523-1739.2012.01909.x
Wedekind, C., Gessner, M. O., Vazquez, F., Maerki, M., and Steiner, D. (2010). Elevated resource availability sufficient to turn opportunistic into virulent fish pathogens. Ecology 91, 1251–1256. doi: 10.1890/09-1067.1
Wilkins, L. G. E., Fumagalli, L., and Wedekind, C. (2016). Effects of host genetics and environment on egg-associated microbiotas in brown trout (Salmo trutta). Mol. Ecol. 25, 4930–4945. doi: 10.1111/mec.13798
Wilkins, L. G. E., Marques da Cunha, L., Glauser, G., Vallat, A., and Wedekind, C. (2017). Environmental stress linked to consumption of maternally derived carotenoids in brown trout embryos (Salmo trutta). Ecol. Evol. 7, 5082–5093. doi: 10.1002/ece3.3076
Keywords: fish, genetic sexing, embryo, hatching, yolk-sac larvae, environmental stress, ovarian fluid, salmonid
Citation: Nusbaumer D, Garaud L, Ançay L and Wedekind C (2021) Sex-Specific Stress Tolerance in Embryos of Lake Char (Salvelinus umbla). Front. Ecol. Evol. 9:768263. doi: 10.3389/fevo.2021.768263
Received: 31 August 2021; Accepted: 25 October 2021;
Published: 17 November 2021.
Edited by:
Ákos Pogány, Eötvös Loránd University, HungaryReviewed by:
Franz Lahnsteiner, Bundesamt für Wasserwirtschaft, AustriaNico K. Michiels, University of Tübingen, Germany
Copyright © 2021 Nusbaumer, Garaud, Ançay and Wedekind. This is an open-access article distributed under the terms of the Creative Commons Attribution License (CC BY). The use, distribution or reproduction in other forums is permitted, provided the original author(s) and the copyright owner(s) are credited and that the original publication in this journal is cited, in accordance with accepted academic practice. No use, distribution or reproduction is permitted which does not comply with these terms.
*Correspondence: Claus Wedekind, Y2xhdXMud2VkZWtpbmRAdW5pbC5jaA==; orcid.org/0000-0001-6143-4716