- 1Department of Zoology and Entomology, University of Pretoria, Pretoria, South Africa
- 2Environment and Sustainability Institute, University of Exeter, Exeter, United Kingdom
- 3Wits Research Institute for Malaria, School of Pathology, Faculty of Health Sciences, University of the Witwatersrand, Johannesburg, South Africa
- 4Centre for Emerging Zoonotic and Parasitic Diseases, National Institute for Communicable Diseases of the National Health Laboratory Service, Johannesburg, South Africa
- 5School of Health Systems and Public Health, University of Pretoria Institute for Sustainable Malaria Control (UP ISMC), University of Pretoria, Pretoria, South Africa
- 6Scientific Services, South African National Parks, Skukuza, South Africa
Light is a fundamental cue regulating a host of biological responses. The artificial modification thereof demonstrably impacts a wide range of organisms. The use of artificial light is changing in type, extent and intensity. Insect vector-borne diseases remain a global scourge, but surprisingly few studies have directly investigated the interactions between artificial light and disease vectors, such as mosquitoes. Here we briefly overview the progress to date, which highlights that artificial light must be considered as a modulator of mosquito-borne disease risk. We discuss where the mechanisms may lie, and where future research could usefully be directed, particularly in advancing understanding of the biological effects of the light environment. Further understanding of how artificial light may modulate mosquito-borne disease risk may assist in employing and redesigning light regimes that do not increase, and may even mitigate, already significant disease burdens, especially in the developing world.
Introduction
Research into mosquito-borne disease risk covers a rich tapestry of approaches and fields. The work has tackled this pernicious societal problem in a variety of ways. Chiefly amongst these are advances in understanding of mosquito and parasite biology, ecology, biochemistry, genetics, control strategies and how these interact with human activities (White et al., 2011; Gatton et al., 2013; Caminade et al., 2014; Neafsey et al., 2015; Otto et al., 2018; Mordecai et al., 2019; Mozūraitis et al., 2020). Nonetheless, a new frontier of work is exploring the ways in which light, and the Artificial Light at Night (ALAN) from human forcing, may alter mosquito borne-disease risk. We here explore such advances, and highlight the complex ways in which artificially lit environments may change human-vector interactions and in consequence alter mosquito borne-disease risk.
Artificial Light at Night is produced from a range of anthropogenic sources, and is increasingly recognized as a global change driver (Davies and Smyth, 2018). Light regulates a range of physiological and behavioral responses, and so ultimately can also influence the fitness of species. ALAN therefore has impacts across the biological organizational hierarchy, from genes to communities, and across a diversity of taxonomic groups, from bacteria to higher vertebrates (Gaston et al., 2013; Sanders et al., 2021).
The interactions of artificial light with a host of aspects of insect biology are now well established (Wakefield et al., 2016; Knop et al., 2017; Owens et al., 2020; Sanders et al., 2021). If ALAN increases mosquito fitness and biting behavior, it may increase mosquito-borne disease risk (Barghini and de Medeiros, 2010; Martinez-Bakker and Helm, 2015; Kernbach et al., 2018). Conversely, if ALAN suitably changes fitness and feeding behavior it could be implemented and operationalized as an additional mosquito-control strategy to reduce mosquito-borne diseases (Barghini and de Medeiros, 2010; Martinez-Bakker and Helm, 2015; Kernbach et al., 2018). With mosquito-borne diseases, such as malaria, West Nile, chikungunya, dengue, lymphatic filariasis and zika, accounting for 17% of all global infectious diseases and cause an estimated 7,00,000 human deaths annually (WHO, 2021a), artificial drivers are integral aspects to understand. From a research and monitoring perspective, it is also critical to understand how mosquito trapping techniques using light for monitoring purposes may be optimized to ensure a broader suite of species are captured with greater reliability (Wilson et al., 2021). For example, Culex pipiens shows negative phototaxis, and so removal of light from regularly used CDC light traps may improve collection efficiency and understanding of species ecology (Boze et al., 2021).
Here, we consider artificial light as a modulator of mosquito-borne disease transmission. First, we touch on the burgeoning literature demonstrating that artificial light is affecting mosquito-borne disease risk. Then, we discuss the mechanisms that may underpin how ALAN is altering mosquito biology by highlighting how biological responses interact with the characteristics of the light environment and how these may influence responses of mosquitoes to light regimes. Throughout, we discuss where ongoing research in this critical field may be usefully focused, particularly advancing understanding of the biological effects of light and the characteristics of the light environment itself.
Mosquitoes and Artificial Light
Light at night as a modulator of circadian rhythms in anophelines was first experimentally demonstrated in 1966, where recording the flight activity of Anopheles gambiae under different light regimes showed that light can have an inhibitory effect on activity (Jones et al., 1966). Since then, a cohesive quantitative link between artificial light and mosquito biology has emerged, although the work is scattered across a range of different responses, from attraction and changes in biting rates, to impacts on different aspects of the biology of mosquitoes, and from genes to organismal physiology. We highlight some examples below, and note that unless otherwise stated, all studies used white Light Emitting Diode (LED) lights in cases where experimental treatments are used.
• There is extensive circadian and light regulation of the transcriptome (i.e., sum of all the messenger RNA) in An. gambiae, influencing genes from metabolic detoxification, immunity to nutrient sensing (Rund et al., 2013) and clock gene expression in Culex pipiens f. molestus (Honnen et al., 2019).
• Acute and sustained photic suppression of biting activity in An. gambiae (Sheppard et al., 2017), and their blood feeding is modulated by light (Das and Dimopoulos, 2008).
• Aedes aegypti may increase its biting rate under 50 lux incandescent lights (Rund et al., 2020), while ultraviolet (UV) light suppresses An. gambiae activity compared to no night time light (Baik et al., 2020).
• Culex mosquitoes in areas with even dim skyglow ALAN (∼4 lx) reproduce and bite later into the season, thus extending the period of disease risk for urban residents (Fyie et al., 2021).
• Artificial Light at Night reduces diapause incidence in Aedes albopictus by 40% (Westby and Medley, 2020; high-pressure sodium lights).
• Artificial Light at Night can alter West Nile virus exposure risk across Florida (Kernbach et al., 2021) and increases virus competence in its ubiquitous passerine reservoir species (Kernbach et al., 2019); both studies investigated ALAN at broad spatial scales.
Although such work takes a range of forms and has little consistency in the responses measured, there is now consensus that ALAN is altering mosquito biology (Longcore and Rich, 2004; Rund et al., 2016; Wilson et al., 2021).
Biological Effects of Artificial Light
Artificial Light at Night may modulate disease vector biology and hence disease risk (Figure 1). Organisms either use light as a resource (e.g., partitioning, and thus activity) or use light as an information source [e.g., for vision, circadian clocks and photoperiodism; following Gaston et al. (2013)]. In consequence, the characteristics of the light environment can modulate biological responses. We broadly focus on such patterns – but we note that intra- and interspecific differences also need to be accounted for. For instance, light responses in both Anopheles and Aedes are strongly affected by sex (Honnen et al., 2016; Baik et al., 2020). For this reason, we caution against generalizations between different species. At this stage, in our assessment of the literature, the understanding of the impacts of ALAN on mosquitoes is still incomplete. Only some aspects have been touched on thus far, which we highlight below, and also where work can usefully be expanded.
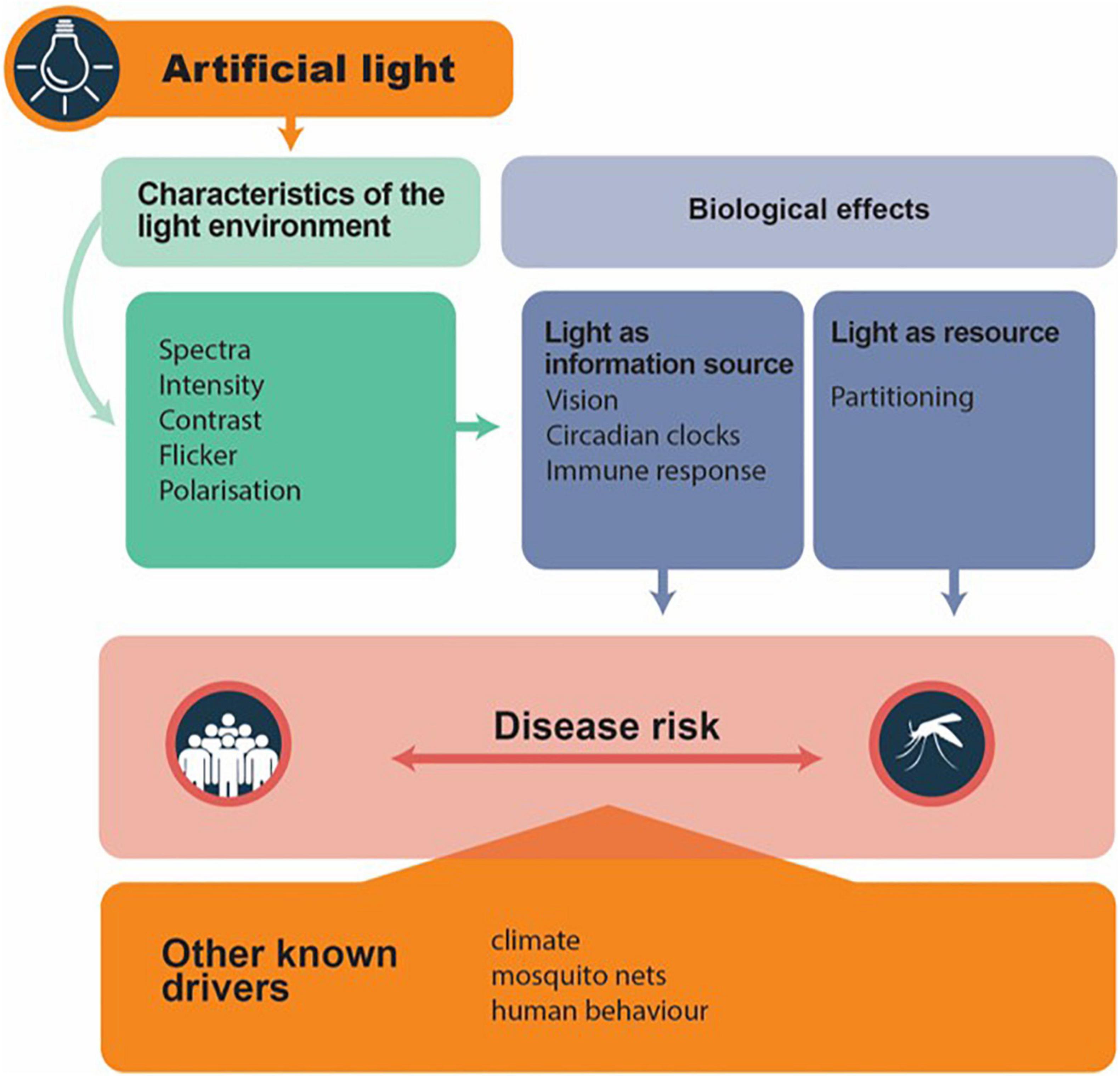
Figure 1. Conceptual illustration to predict how artificial light may act as a modulator to mosquito-borne disease transmission. It captures the characteristics of the light environment which alter species biologically respond to light, which then may modulate disease transmission risk. Note that all the other known drivers can interact with that of artificial light to influence disease transmission risk. Simplified from Gaston et al. (2013).
Much attention has been paid to the circadian rhythms of vectors since endogenous circadian clocks regulate many aspects of behavior, physiology and metabolism (Rund et al., 2013; Prior et al., 2019; Baik et al., 2020). There is extensive circadian and light regulation of the transcriptome in An. gambiae (Rund et al., 2013), and host circadian rhythms are disrupted during malaria infection (Prior et al., 2019). Circadian rhythms also alter detoxification and insecticide resistance in the malaria mosquito An. gambiae (Balmert et al., 2014). In humans, artificial lights have altered and extended human activity beyond strictly diurnal into extensive night time hours (Chepesiuk, 2009). The causes and consequences of daily rhythms in the interactions between vectors, their hosts and the parasites they transmit is an emerging research area [most recently reviewed by Rund et al. (2016)].
Increases in human activity into the nighttime mean that nocturnal mosquitoes have a greater chance of obtaining a blood meal, if it extends human activity outside of other prevention methods, such as bed-net use (Barghini and de Medeiros, 2010). In the Bolivian Amazon, 48% of An. darlingi total night biting takes place from 19:00 to 21:00pm (Harris et al., 2006), and there is a similarly early biting time in other anophelines in Kenya (Cooke et al., 2015). Furthermore, Anopheles and Culex are predominantly crepuscular and nocturnal, while Aedes spp. are predominantly diurnal (Honnen et al., 2019; Baik et al., 2020). As such, the timing of artificial light outside of natural regimes is a key aspect that alters mosquito biology (Sheppard et al., 2017). As one might expect, in the nocturnal An. gambiae biting rates are suppressed under artificial light (Sheppard et al., 2017), particularly UV light (Baik et al., 2020). Conversely, under LED lights, Ae. aegypti may increase their biting rates on humans (Rund et al., 2020). This limited evidence suggests that ALAN reduces biting rates in nocturnal mosquitoes, but that it may increase those of diurnal ones. How increased biting rates translate into mosquito fitness and disease prevalence can be further explored, especially in both laboratory and field settings.
From a community ecology perspective, alterations to mosquito composition and behavior due to artificial lights may conceivably alter competitive interactions, community assembly and predation risk (for a recent review see Grubisic and van Grunsven, 2021). How artificial light may interact with various aspects of mosquito ecology, and how this alters their fitness and potentially disease risk, could be usefully advanced. At the moment, however, the theoretical framework on how ALAN impacts insect communities and populations is poorly developed (Grubisic and van Grunsven, 2021).
Light is also used as an information source via the insect eye which can affect their visual ecology in complex ways (Land and Nilsson, 2012). Detailed modeling of spectra, intensity, reflectance and the vision of hawk-moths (Deilephila elpenor), revealed that artificial lighting can disrupt the visual ecology links between flowers, pollinators and predators (Briolat et al., 2021). Such detailed work does not exist for mosquitoes, and so the role of mosquito vision in increasing vector disease transmission is currently not well known (Gibson, 1995; Land et al., 1997; Moon et al., 2015). Light is known to drive both rhodopsin maturation and recycling (Metoxen et al., 2016) and Op10 rhodopsin is co-expressed with Op8 rhodopsin in Ae. aegypti and An. gambiae R7 photoreceptor cells (Hu et al., 2014; Zhan et al., 2020). Anopheles gambiae, as well as other disease vectors, may be particularly sensitive to even small fluctuations in the night environment. There are fundamental structural differences in the eyes of diurnal and nocturnal mosquitoes, illustrating that it’s a key morphological trait responding to the light environment (Land et al., 1999; Land and Nilsson, 2012). While most nocturnal insects have superposition eyes, curiously, An. gambiae has retained structures more akin to an apposition eye more typical for diurnal species, such as a wide conical lens and short thick rhabdoms (Land et al., 1999). Its eye is adapted for high sensitivity in dim environments, where selectivity to, and a high ability for, photon capture takes precedence over finer resolutions in vision. This means even small fluctuations in the light environment may therefore influence behavior and hence modulate risk for disease transmission (Land et al., 1999; Barghini and de Medeiros, 2010; Land and Nilsson, 2012; Martinez-Bakker and Helm, 2015; Kernbach et al., 2018).
Mosquitoes make use of an attraction cascade to find potential hosts, relying on a range of sensory cues, using vision to associate odor plumes with thermal targets (Van Breugel et al., 2015; Zhan et al., 2020). The range of attraction, the maximum distance at which light may attract insects, is variable but generally low in mosquitoes. In Anopheles mosquitoes, it is less than 5 m (Costantini et al., 1998), and more variable in Culicoides at 10–30 m (Rigot and Gilbert, 2012; Kirkeby et al., 2013; Wilson et al., 2021). However, of greater importance may be how mosquitoes perceive their lit environment, as light from a source (radiance) is not akin to light reflected off a surface (irradiance). For instance, red objects are attractive to mosquitoes but not red light (Wilson et al., 2021).
The use of ALAN may increase transmission risk if it is occurs at times and places that attract vectors, and so increases the potential for disease transfer. How typical objects in human environments reflect lights in different artificially lit environments, and how this may attract or repel mosquito behavior, may be another fruitful research avenue.
Artificial light may suppress the immune responses of a range of mammals, including humans (Navara and Nelson, 2007; Gaston et al., 2013; Fonken and Nelson, 2014). Exposure to artificial light alters hormone production, in particular by interfering with the regular expression of melatonin (Navara and Nelson, 2007; Gaston et al., 2013; Fonken and Nelson, 2014). Since it is critical to the sleep cycle and removal of free-radicals, changes in melatonin may alter an organism’s ability to fight off diseases. Melatonin itself may also modulate the Plasmodium (malaria parasite) cell cycle (Lima et al., 2013). Melatonin expression in mosquitoes is less well known, but it seems likely that it will also have a sensitivity to blue wavelengths, and may similarly be involved in regulating immune responses to infection from parasites like Plasmodium. c.
Characteristics of the Light Environment
Expanding understanding of how light may modulate the above biological responses and alters mosquito-borne disease risk requires increased attention. This includes the impact of the characteristics of the light environment (spectra, intensity, contrast, flicker, and polarization) on mosquito species and their biology.
The spectrum of light is arguably its most fundamental characteristic and is often included as a key characteristic in ALAN research. Mosquitoes are known to respond to light of different spectra. For instance, electroretinograms show that both female Aedes (Muir et al., 1992) and female Culex spp. (Peach et al., 2019) have dual peaks of sensitivity of insect eye receptors to UV and blue/green light, respectively. Anopheles spp., especially females, show a strong aversion for light in the UV and bluer spectra (Baik et al., 2020). This likely indicates fewer photoreceptors in mosquitoes than other groups (Van Der Kooi et al., 2021), which may in turn indicate that lighting approaches can be devised that do not attract them by being outside of their visual spectrum, but that appear as regular lighting to humans. Conceptually, light in the red spectrum could be less attractive to these groups (Wilson et al., 2021). However, given the diversity of responses from earlier studies, no single spectral solution will act as a deterrent for different species of mosquitoes, but there may be opportunities for exploring more targeted species-specific approaches (Wilson et al., 2021).
Insights into how spectra and intensity interact to alter mosquito behavior can also be advanced. Different intensities do alter behavior in Anophelines (Sheppard et al., 2017). Two lights that appear equally bright to humans may look fundamentally different to insects. We posit that laboratory-reared mosquitoes are typically also kept in environments darker than even the faint celestial light and lunar cycles that would occur naturally, and so at very low light intensities. How this alters circadian rhythms over time, and inferences made from laboratory populations, could be usefully investigated.
Less well studied than light spectra and intensity is the influence of contrast, flicker and polarization. Objects in the environment reflect different wavelengths of lights to different extents, which means the spectral environment experienced by an individual mosquito may differ depending on the makeup of objects and their contrast with each other, and the composition of different artificial lights in the local environment (Land and Nilsson, 2012; Wilson et al., 2021). For Ae. aegypti, it has been shown that mosquito attraction to the thermal and odorant cues of host decoy traps is modulated by visual information, and changes with the color of the traps (Tang et al., 2021). In the context of mosquitoes, this may be particularly important in how they respond to isolated lights vs. more diversely lit environments.
Flicker frequency, aka critical fusion frequency (CFF), is the frequency at which flickering light appears as a continuous light source to the observer. For instance, human CFF is around 50–60 Hz, but in nocturnal insects it averages around 70 Hz (Barroso et al., 2017). In consequence, insects may observe flickering differently to other organisms and so it may affect light attractiveness and/or repulsion (Wilson et al., 2021). Indeed, manipulating flicker frequency of UV LED light can improve the capability of light traps to attract mosquitoes (Liu et al., 2017), but it nonetheless remains an understudied component of artificial light.
Navigating by polarized light is important to many aquatic insects. However, no evidence for positive polarotaxis was found in Ae. aegypti (Bernáth et al., 2008), and so other mainly olfactory cues are thought to be of greater importance for finding suitable egg-laying water in mosquitoes (Heinloth et al., 2018). Nevertheless, as with contrast and CFF, the role of polarized light in mosquito biology may be overlooked as it has rarely been explicitly considered in previous studies.
Conclusion
Research conducted to date on disease transmission and risk has mainly focused on the impacts of artificial light on vectors in laboratory settings rather than on how real-world environments are artificially lit, and how humans and vectors respond and interact in those environments. While the evidence-base for artificial light as a modulator of mosquito-borne diseases is now established, there are still many unanswered questions and future research can be informed by what has already been learned from laboratory experiments and responses in other taxonomic groups. Many questions may be answered by testing how different characteristics of the lit environment may be translated into the myriad of biological effects that it influences. New approaches can quantify the key features of lit environments and the generally overlooked components we mentioned above (Nilsson and Smolka, 2021). Of course, ALAN can interact with other, better known anthropogenic drivers of increased disease risk like land transformation (Meyer Steiger et al., 2016; Lee et al., 2020) and changing climatic conditions (Medlock and Leach, 2015). Teasing their individual contributions apart experimentally will advance understanding into disease risk. Popular disease frameworks such as R0 equations (Hartemink et al., 2015), Dynamic SEIR (Susceptible, Exposed, Infectious, Removed) compartmental models (Getz et al., 2019) and/or agent based models (Smith et al., 2018), could usefully incorporate the impacts of artificial light on vector biology. However, a range of new data from laboratory and field studies will be required to parametrize such models, and much work remains to be done to develop them.
At present, the World Health Organization (WHO, 2021b) does not recognize artificial light as a modulator of vector borne disease risk. The extent to which artificial light may be used as a vector control strategy remains unknown (Wilson et al., 2021). The burgeoning scientific study of the interactions of artificial light and mosquitoes now clearly demonstrates that it needs greater consideration as such. Only once the consequences of artificial lighting technologies are fully understood can we ensure that current developmental expansion does not unexpectedly compromise human health by increasing disease risk. Mosquito-borne diseases compromise the attainment of the Sustainable Development Goals (SDGs) and artificial lights of the correct kinds, used in optimal places and times, could help reduce vector-borne disease burdens.
Author Contributions
BC, KG, and IS conceptualized the study. BC wrote the first draft. All authors then contributed and wrote all subsequent drafts.
Funding
The Jennifer Ward Oppenheimer Research Grant funds BC and LK is supported by a DST/NRF Research Chair Initiative grant award (UID: 64763).
Conflict of Interest
The authors declare that the research was conducted in the absence of any commercial or financial relationships that could be construed as a potential conflict of interest.
Publisher’s Note
All claims expressed in this article are solely those of the authors and do not necessarily represent those of their affiliated organizations, or those of the publisher, the editors and the reviewers. Any product that may be evaluated in this article, or claim that may be made by its manufacturer, is not guaranteed or endorsed by the publisher.
References
Baik, L. S., Nave, C., Au, D. D., Guda, T., Chevez, J. A., Ray, A., et al. (2020). Circadian regulation of light-evoked attraction and avoidance behaviors in daytime- versus nighttime-biting mosquitoes. Curr. Biol. 30, 3252–3259.e3. doi: 10.1016/j.cub.2020.06.010
Balmert, N. J., Rund, S. S. C., Ghazi, J. P., Zhou, P., and Duffield, G. E. (2014). Time-of-day specific changes in metabolic detoxification and insecticide resistance in the malaria mosquito Anopheles gambiae. J. Insect Physiol. 64, 30–39. doi: 10.1016/j.jinsphys.2014.02.013
Barghini, A., and de Medeiros, B. A. S. (2010). Artificial lighting as a vector attractant and cause of disease diffusion. Environ. Health Perspect. 118, 1503–1506. doi: 10.1289/ehp.1002115
Barroso, A., Haifig, I., Janei, V., Da Silva, I., Dietrich, C., and Costa-Leonardo, A. M. (2017). Effects of flickering light on the attraction of nocturnal insects. Light. Res. Technol. 49, 100–110. doi: 10.1177/1477153515602143
Bernáth, B., Horváth, G., Gál, J., Fekete, G., and Meyer-Rochow, V. B. (2008). Polarized light and oviposition site selection in the yellow fever mosquito: no evidence for positive polarotaxis in Aedes aegypti. Vision Res. 48, 1449–1455. doi: 10.1016/j.visres.2008.04.007
Boze, B. G. V., Renfro, K., Markowski, D., and Lozano-Fuentes, S. (2021). Effect of incandescent light on collection of West Nile Virus vectors using CDC miniature light traps in Northern Colorado. J. Insect Sci. 21, 21–24. doi: 10.1093/jisesa/ieab069
Briolat, E. S., Gaston, K. J., Bennie, J., Rosenfeld, E. J., and Troscianko, J. (2021). Artificial nighttime lighting impacts visual ecology links between flowers, pollinators and predators. Nat. Commun. 12:4163. doi: 10.1038/s41467-021-24394-0
Caminade, C., Kovats, S., Rocklov, J., Tompkins, A. M., Morse, A. P., Colón-González, F. J., et al. (2014). Impact of climate change on global malaria distribution. Proc. Natl. Acad. Sci. U.S.A. 111, 3286–3291. doi: 10.1073/pnas.1302089111
Chepesiuk, R. (2009). Missing the dark: health effects of light pollution. Environ. Health Perspect. 117, 20–27. doi: 10.1289/ehp.117-a20
Cooke, M. K., Kahindi, S. C., Oriango, R. M., Owaga, C., Ayoma, E., Mabuka, D., et al. (2015). “A bite before bed”: exposure to malaria vectors outside the times of net use in the highlands of western Kenya. Malar. J. 14:259. doi: 10.1186/s12936-015-0766-4
Costantini, C., Sagnon, N., della Torre, A., Diallo, M., Brady, J., Gibson, G., et al. (1998). Odor-mediated host preferences of West African mosquitoes, with particular reference to malaria vectors. Am. J. Trop. Med. Hyg. 58, 56–63. doi: 10.4269/ajtmh.1998.58.56
Das, S., and Dimopoulos, G. (2008). Molecular analysis of photic inhibition of blood-feeding in Anopheles gambiae. BMC Physiol. 8:23. doi: 10.1186/1472-6793-8-23
Davies, T. W., and Smyth, T. (2018). Why artificial light at night should be a focus for global change research in the 21st century. Glob. Chang. Biol. 24, 872–882. doi: 10.1111/gcb.13927
Fonken, L. K., and Nelson, R. J. (2014). The effects of light at night on circadian clocks and metabolism. Endocr. Rev. 35, 648–670. doi: 10.1210/er.2013-1051
Fyie, L. R., Gardiner, M. M., and Meuti, M. E. (2021). Artificial light at night alters the seasonal responses of biting mosquitoes. J. Insect Physiol. 129:104194. doi: 10.1016/j.jinsphys.2021.104194
Gaston, K. J., Bennie, J., Davies, T. W., and Hopkins, J. (2013). The ecological impacts of nighttime light pollution: a mechanistic appraisal. Biol. Rev. 88, 912–927. doi: 10.1111/brv.12036
Gatton, M. L., Chitnis, N., Churcher, T., Donnelly, M. J., Ghani, A. C., Godfray, H. C. J., et al. (2013). The importance of mosquito behavioural adaptations to malaria control in Africa. Evolution (N. Y). 67, 1218–1230. doi: 10.1111/evo.12063
Getz, W. M., Salter, R., and Mgbara, W. (2019). Adequacy of SEIR models when epidemics have spatial structure: ebola in Sierra Leone. Philos. Trans. R. Soc. Lond. B Biol. Sci. 374:20180282. doi: 10.1098/rstb.2018.0282
Gibson, G. (1995). A behavioural test of the sensitivity of a nocturnal mosquito, Anopheles gambiae, to dim white, red and infra-red light. Physiol. Entomol. 20, 224–228. doi: 10.1111/j.1365-3032.1995.tb00005.x
Grubisic, M., and van Grunsven, R. H. (2021). Artificial light at night disrupts species interactions and changes insect communities. Curr. Opin. Insect Sci. 47, 136–141. doi: 10.1016/j.cois.2021.06.007
Harris, A. F., Matias-Arnéz, A., and Hill, N. (2006). Biting time of Anopheles darlingi in the Bolivian Amazon and implications for control of malaria. Trans. R. Soc. Trop. Med. Hyg. 100, 45–47. doi: 10.1016/j.trstmh.2005.07.001
Hartemink, N., Cianci, D., and Reiter, P. (2015). R0 for vector-borne diseases: impact of the assumption for the duration of the extrinsic incubation period. Vector Borne Zoonotic Dis. 15, 215–217. doi: 10.1089/vbz.2014.1684
Heinloth, T., Uhlhorn, J., and Wernet, M. F. (2018). Insect responses to linearly polarized reflections: orphan behaviors without neural circuits. Front. Cell. Neurosci. 12:50. doi: 10.3389/fncel.2018.00050
Honnen, A. C., Kypke, J. L., Hölker, F., and Monaghan, M. T. (2019). Artificial light at night influences clock-gene expression, activity, and fecundity in the mosquito Culex pipiens f. molestus. Sustainability 11:6220. doi: 10.3390/su11226220
Honnen, A., Johnston, P. R., and Monaghan, M. T. (2016). Sex-specific gene expression in the mosquito Culex pipiens f. molestus in response to artificial light at night. BMC Genomics 17:22. doi: 10.1186/s12864-015-2336-0
Hu, X., Leming, M. T., Whaley, M. A., and O’Tousa, J. E. (2014). Rhodopsin coexpression in UV photoreceptors of Aedes aegypti and Anopheles gambiae mosquitoes. J. Exp. Biol. 217, 1003–1008. doi: 10.1242/jeb.096347
Jones, M. D. R., Ford, M., and Gillet, J. D. (1966). Light-on and light-off effects on the ciracadian flight activity in the mosquito Anopheles gambia. Nature 211, 871–872.
Kernbach, M. E., Hall, R. J., Burkett-Cadena, N. D., Unnasch, T. R., and Martin, L. B. (2018). Dim light at night: physiological effects and ecological consequences for infectious disease. Integr. Comp. Biol. 58, 995–1007. doi: 10.1093/icb/icy080
Kernbach, M. E., Martin, L. B., Unnasch, T. R., Hall, R. J., Jiang, R. H. Y., and Francis, C. D. (2021). Light pollution affects West Nile virus exposure risk across Florida. Proc. R. Soc. Lond. B Biol. Sci. 288:20210253. doi: 10.1098/rspb.2021.0253
Kernbach, M. E., Newhouse, D. J., Miller, J. M., Hall, R. J., Gibbons, J., Oberstaller, J., et al. (2019). Light pollution increases West Nile virus competence of a ubiquitous passerine reservoir species. Proc. R. Soc. Lond. B Biol. Sci. 286:20191051. doi: 10.1098/rspb.2019.1051
Kirkeby, C., Græsbøll, K., Stockmarr, A., Christiansen, L. E., and Bødker, R. (2013). The range of attraction for light traps catching Culicoides biting midges (Diptera: Ceratopogonidae). Parasit. Vectors 6:67. doi: 10.1186/1756-3305-6-67
Knop, E., Zoller, L., Ryser, R., Gerpe, C., Hörler, M., and Fontaine, C. (2017). Artificial light at night as a new threat to pollination. Nature 548, 206–209. doi: 10.1038/nature23288
Land, M. F., Gibson, G., and Horwood, J. (1997). Mosquito eye design: conical rhabdoms are matched to wide aperture lenses. Proc. R. Soc. Lond. B Biol. Sci. 264, 1183–1187. doi: 10.1098/rspb.1997.0163
Land, M. F., Gibson, G., Horwood, J., and Zeil, J. (1999). Fundamental differences in the optical structure of the eyes of nocturnal and diurnal mosquitoes. J. Comp. Physiol. 185, 91–103. doi: 10.1007/s003590050369
Lee, J. M., Wasserman, R. J., Gan, J. Y., Wilson, R. F., Rahman, S., and Yek, S. H. (2020). Human activities attract harmful mosquitoes in a tropical urban landscape. Ecohealth 17, 52–63. doi: 10.1007/s10393-019-01457-9
Lima, W. R., Holder, A. A., and Garcia, C. R. S. (2013). Melatonin signaling and its modulation of PfNF-YB transcription factor expression in Plasmodium falciparum. Int. J. Mol. Sci. 14, 13704–13718. doi: 10.3390/ijms140713704
Liu, Y. N., Liu, Y. J., Chen, Y. C., Ma, H. Y., and Lee, H. Y. (2017). Enhancement of mosquito trapping efficiency by using pulse width modulated light emitting diodes. Sci. Rep. 7:40074. doi: 10.1038/srep40074
Longcore, T., and Rich, C. (2004). Ecological light pollution. Front. Ecol. Environ. 2, 191–198. doi: 10.1890/1540-9295(2004)002[0191:ELP]2.0.CO;2
Martinez-Bakker, M., and Helm, B. (2015). The influence of biological rhythms on host-parasite interactions. Trends Ecol. Evol. 30, 314–326. doi: 10.1016/j.tree.2015.03.012
Medlock, J. M., and Leach, S. A. (2015). Effect of climate change on vector-borne disease risk in the UK. Lancet Infect. Dis. 15, 721–730. doi: 10.1016/S1473-3099(15)70091-5
Metoxen, A. J., Leming, M. T., Hu, X., Whaley, M. A., and O’Tousa, J. E. (2016). Light-driven processes control both rhodopsin maturation and recycling in mosquito photoreceptors. J. Neurosci. 36, 11051–11058. doi: 10.1523/JNEUROSCI.1754-16.2016
Meyer Steiger, D. B., Ritchie, S. A., and Laurance, S. G. W. (2016). Mosquito communities and disease risk influenced by land use change and seasonality in the Australian tropics. Parasit. Vectors 9:387. doi: 10.1186/s13071-016-1675-2
Moon, Y. M., Metoxen, A. J., Leming, M. T., Whaley, M. A., and Tousa, J. E. O. (2015). Rhodopsin management during the light-dark cycle of Anopheles gambiae mosquitoes. J. Insect Physiol. 70, 88–93. doi: 10.1016/j.jinsphys.2014.09.006.Rhodopsin
Mordecai, E. A., Caldwell, J. M., Grossman, M. K., Lippi, C. A., Johnson, L. R., Neira, M., et al. (2019). Thermal biology of mosquito-borne disease. Ecol. Lett. 22, 1690–1708. doi: 10.1111/ele.13335
Mozūraitis, R., Hajkazemian, M., Zawada, J. W., Szymczak, J., Pålsson, K., Sekar, V., et al. (2020). Male swarming aggregation pheromones increase female attraction and mating success among multiple African malaria vector mosquito species. Nat. Ecol. Evol. 4, 1395–1401. doi: 10.1038/s41559-020-1264-9
Muir, L. E., Thorne, M. J., and Kay, B. H. (1992). Aedes aegypti (Diptera: Culicidae) vision: spectral sensitivity and other perceptual parameters of the female eye. J. Med. Entomol. 29, 278–281. doi: 10.1093/jmedent/29.2.278
Navara, K. J., and Nelson, R. J. (2007). The dark side of light at night: physiological, epidemiological, and ecological consequences. J. Pineal Res. 43, 215–224. doi: 10.1111/j.1600-079X.2007.00473.x
Neafsey, D. E., Waterhouse, R. M., Abai, M. R., Aganezov, S. S., Alekseyev, M. A., Allen, J. E., et al. (2015). Highly evolvable malaria vectors: the genomes of 16 Anopheles mosquitoes. Science 347:1258522. doi: 10.1126/science.1258522
Nilsson, D. E., and Smolka, J. (2021). Quantifying biologically essential aspects of environmental light. J. R. Soc. Interface 18:20210184. doi: 10.1098/rsif.2021.0184
Otto, T. D., Gilabert, A., Crellen, T., Böhme, U., Arnathau, C., Sanders, M., et al. (2018). Genomes of all known members of a Plasmodium subgenus reveal paths to virulent human malaria. Nat. Microbiol. 3, 687–697. doi: 10.1038/s41564-018-0162-2
Owens, A. C. S., Cochard, P., Durrant, J., Farnworth, B., Perkin, E. K., and Seymoure, B. (2020). Light pollution is a driver of insect declines. Biol. Conserv. 241:108259. doi: 10.1016/j.biocon.2019.108259
Peach, D. A. H., Ko, E., Blake, A. J., and Gries, G. (2019). Ultraviolet inflorescence cues enhance attractiveness of inflorescence odour to Culex pipiens mosquitoes. PLoS One 14:e0217484. doi: 10.1371/journal.pone.0217484
Prior, K. F., O’Donnell, A. J., Rund, S. S. C., Savill, N. J., van der Veen, D. R., and Reece, S. E. (2019). Host circadian rhythms are disrupted during malaria infection in parasite genotype-specific manners. Sci. Rep. 9:10905. doi: 10.1038/s41598-019-47191-8
Rigot, T., and Gilbert, M. (2012). Quantifying the spatial dependence of Culicoides midge samples collected by Onderstepoort-type blacklight traps: an experimental approach to infer the range of attraction of light traps. Med. Vet. Entomol. 26, 152–161. doi: 10.1111/j.1365-2915.2011.00988.x
Rund, S. S. C., Gentile, J. E., and Duffield, G. E. (2013). Extensive circadian and light regulation of the transcriptome in the malaria mosquito Anopheles gambiae. BMC Genomics 14:218. doi: 10.1186/1471-2164-14-218
Rund, S. S. C., Labb, L. F., Benefiel, O. M., and Duffield, G. E. (2020). Artificial light at night increases Aedes aegypti mosquito biting behavior with implications for arboviral disease transmission. Am. J. Trop. Med. Hyg. 103, 2450–2452. doi: 10.4269/ajtmh.20-0885
Rund, S. S. C., O’Donnell, A. J., Gentile, J. E., and Reece, S. E. (2016). Daily rhythms in mosquitoes and their consequences for malaria transmission. Insects 7:14. doi: 10.3390/insects7020014
Sanders, D., Frago, E., Kehoe, R., Patterson, C., and Gaston, K. J. (2021). A meta-analysis of biological impacts of artificial light at night. Nat. Ecol. Evol. 5, 74–81. doi: 10.1146/annurev-ecolsys-110316-022745
Sheppard, A. D., Rund, S. S. C., George, G. F., Clark, E., Acri, D. J., and Duffield, G. E. (2017). Light manipulation of mosquito behaviour: acute and sustained photic suppression of biting activity in the Anopheles gambiae malaria mosquito. Parasit. Vectors 10:255. doi: 10.1186/s13071-017-2196-3
Smith, N. R., Trauer, J. M., Gambhir, M., Richards, J. S., Maude, R. J., Keith, J. M., et al. (2018). Agent-based models of malaria transmission: a systematic review. Malar. J. 17:299. doi: 10.1186/s12936-018-2442-y
Tang, J. Y., Kosgei, J., Ochomo, E., Ndenga, B. A., Ghiaseddin, R., Lobo, N. F., et al. (2021). Impact of visual features on capture of Aedes aegypti with host decoy traps (HDT). Med. Vet. Entomol. 35, 169–176. doi: 10.1111/mve.12482
Van Breugel, F., Riffell, J., Fairhall, A., and Dickinson, M. H. (2015). Mosquitoes use vision to associate odor plumes with thermal targets. Curr. Biol. 25, 2123–2129. doi: 10.1016/j.cub.2015.06.046
Van Der Kooi, C. J., Stavenga, D. G., Arikawa, K., Belušič, G., and Kelber, A. (2021). Evolution of insect color vision: from spectral sensitivity to visual ecology. Annu. Rev. Entomol. 66, 435–461. doi: 10.1146/annurev-ento-061720-071644
Wakefield, A., Broyles, M., Stone, E. L., Jones, G., and Harris, S. (2016). Experimentally comparing the attractiveness of domestic lights to insects: do LEDs attract fewer insects than conventional light types? Ecol. Evol. 6, 8028–8036. doi: 10.1002/ece3.2527
Westby, K. M., and Medley, K. A. (2020). Cold nights, city lights: artificial light at night reduces photoperiodically induced diapause in urban and rural populations of Aedes albopictus (Diptera: Culicidae). J. Med. Entomol. 57, 1694–1699. doi: 10.1093/jme/tjaa139
White, B. J., Collins, F. H., and Besansky, N. J. (2011). Evolution of Anopheles gambiae in relation to humans and malaria. Annu. Rev. Ecol. Evol. Syst. 42, 111–132. doi: 10.1146/annurev-ecolsys-102710-145028
WHO (2021a). Vector-Borne Diseases Factsheet. Available online at: https://www.who.int/news-room/fact-sheets/detail/vector-borne-diseases (accessed August 31, 2021).
WHO (2021b). Handbook of Integrated Vector Management. Available online at: https://www.euro.who.int/en/health-topics/communicable-diseases/vector-borne-and-parasitic-diseases/integrated-vector-management-ivm (accessed August 31, 2021).
Wilson, R., Wakefield, A., Roberts, N., and Jones, G. (2021). Artificial light and biting flies: the parallel development of attractive light traps and unattractive domestic lights. Parasit. Vectors 14:28. doi: 10.1186/s13071-020-04530-3
Keywords: ALAN, light emitting diode, vector control, sustainable development goals, disease vectors
Citation: Coetzee BWT, Gaston KJ, Koekemoer LL, Kruger T, Riddin MA and Smit IPJ (2022) Artificial Light as a Modulator of Mosquito-Borne Disease Risk. Front. Ecol. Evol. 9:768090. doi: 10.3389/fevo.2021.768090
Received: 31 August 2021; Accepted: 24 November 2021;
Published: 25 January 2022.
Edited by:
Davide M. Dominoni, University of Glasgow, United KingdomReviewed by:
Giles Duffield, University of Notre Dame, United StatesSamuel Rund, University of Notre Dame, United States
Copyright © 2022 Coetzee, Gaston, Koekemoer, Kruger, Riddin and Smit. This is an open-access article distributed under the terms of the Creative Commons Attribution License (CC BY). The use, distribution or reproduction in other forums is permitted, provided the original author(s) and the copyright owner(s) are credited and that the original publication in this journal is cited, in accordance with accepted academic practice. No use, distribution or reproduction is permitted which does not comply with these terms.
*Correspondence: Bernard W. T. Coetzee, bernard.coetzee@up.ac.za