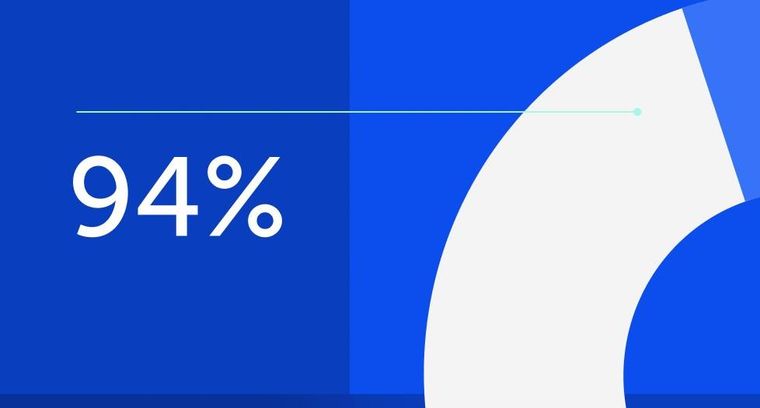
94% of researchers rate our articles as excellent or good
Learn more about the work of our research integrity team to safeguard the quality of each article we publish.
Find out more
ORIGINAL RESEARCH article
Front. Ecol. Evol., 21 October 2021
Sec. Ecophysiology
Volume 9 - 2021 | https://doi.org/10.3389/fevo.2021.767102
This article is part of the Research TopicEcophysiological Analysis of Vulnerability to Climate Warming in EctothermsView all 15 articles
Climate change poses different threats to animals across latitudes. Tropical species have been proposed to be more vulnerable to climate change. However, the responses of animals from tropical mountains to thermal variation and climate change have been scarcely studied. Here, we investigated the thermal biology traits of a tropical lizard (Takydromus kuehnei) distributed at high elevations (>950 m) and evaluated the vulnerabilities of T. kuehnei by thermal biology traits, thermal safety margin, and thermoregulatory effectiveness. The average active body temperatures of T. kuehnei in the field were 26.28°C and 30.65°C in April and June, respectively. The selected body temperature was 33.23°C, and the optimal temperature for locomotion was 30.60°C. The critical thermal minimum and critical thermal maximum temperatures were 4.79°C and 43.37°C, respectively. Accordingly, the thermal safety margin (1.23°C) and thermoregulatory effectiveness (1.23°C) predicted that T. kuehnei distributed in tropical mountains were not significantly depressed by environmental temperatures. This study implies that high-elevation species in tropical regions may not be severely threatened by ongoing climate change and highlights the importance of thermal biology traits in evaluating the vulnerability of species to climate change.
Climate change has negatively affected animal distribution and abundance (Root et al., 2003; Thomas et al., 2004; Medina et al., 2016). Although the latitudinal pattern of the vulnerabilities of animals to climate change is still controversial, increasing investigations claim that tropical animals are profoundly vulnerable to climate change, considering higher metabolic rates (e.g., Dillon et al., 2010), narrow thermal-safety margins (TSM) (e.g., Sunday et al., 2014), and depressed life-history cycles (e.g., Blouin-Demers and Weatherhead, 2001; Deutsch et al., 2008). For instance, the environmental temperature in tropical regions is higher than that in other latitudes, making it possible to exceed the physiological thermal-tolerance limits of animals (Huey et al., 2009; Sunday et al., 2014).
However, animals can migrate toward high latitudes and high elevations to avoid the risk of being threatened by climate change (e.g., Forero-Medina et al., 2011; Freeman et al., 2018). High latitudes and elevations can provide lower average temperatures and more fluctuating temperatures, which provide retreat (i.e., cool places) for thermoregulation. Therefore, tropical mountains may provide refuge for tropical animals to escape from exposure to warming temperatures (Bonebrake and Deutsch, 2012). However, it is largely unknown whether the high-elevation species in tropical regions are depressed by ongoing climate change (Freeman et al., 2018). Understanding the vulnerabilities of animals from tropical mountains is important in not only revealing the thermal adaptation of tropical species at high elevations but also evaluating the availabilities of migrating toward high elevations to escape from climate change (Ghalambor et al., 2006).
As ectotherms, reptiles have been threatened by climate change in the past decades and are projected to encounter more severe threats in the future (Huey et al., 2010; Sinervo et al., 2010; Barnosky et al., 2011; Diele-Viegas et al., 2020; Taylor et al., 2020). Lizards regulate body temperatures by an external heat source, and thus, their biological functions are subject to the effect of thermal environments on body temperatures (Huey, 1982; Hertz et al., 1993). Therefore, compared to other taxa, lizards are particularly sensitive to thermal variations and constitute an appropriate study system for evaluating the vulnerability of animals to climate change (Huey et al., 2012; Taylor et al., 2020). The thermal biology traits, thermal safety margin, and thermoregulation effectiveness are important for thermal adaptation and are also indispensable proxies for determining the vulnerability to climate change in lizards (Huey et al., 2012; Clusella-Trullas et al., 2021). The effects of climate change on lizards depend on the interaction of thermal environments and the integration of thermal biology traits, thermoregulation effectiveness in maintaining body temperature, and thermal safety margins (Sunday et al., 2014; ObregÓn et al., 2020). For example, lizards would maintain their active body temperature in an appropriate range by thermoregulation, which is approximate to the range of optimal body temperatures for various physiological processes (Huey and Kingsolver, 1989; Angilletta et al., 2002). The critical thermal minimum (CTmin) and critical thermal maximum (CTmax) temperatures are thermal limits of permitting performance (Angilletta et al., 2002; Taylor et al., 2020), which generally depend on the thermal environment of species (Ji et al., 1996; Chen et al., 2003; Zhang and Ji, 2004). In addition, the thermal sensitivity of locomotion and resting metabolic rates (RMR) is essential for evaluating the thermal adaptation in ectotherms (e.g., Dillon et al., 2010; Shu et al., 2010; Sun et al., 2014, 2018b). Therefore, thermal biology traits, including body temperature, selected body temperature range, and thermal tolerance, can be integrated to determine the thermoregulatory effectiveness and TSM, which are critical for evaluating the vulnerability of reptiles to climate change (Hertz et al., 1993; Sunday et al., 2014).
The Takydromus lizards have a wide distribution across latitudes in China, ranging from tropical to cold-temperature regions (Zhao, 1993; Lin et al., 2002; Portniagina et al., 2019). Takydromus kuehnei is a small lacertid lizard (snout-vent length, SVL < 60 mm), mainly distributed in tropical regions across southern China. The population on Hainan Island is a typically tropical population in China. According to previous predictions of species vulnerability across latitudes, T. kuehnei of the Hainan population may be the most vulnerable in Takydromus lizards to climate change in China. In the Diaoluoshan Mountains, T. kuehnei is distributed across low to high elevations ranging from 400 to 1000 m (Wang, 2014). Therefore, the high-elevation population of T. kuehnei is an excellent study system for analyzing the response of high-elevation tropical species to thermal variation, considering the interaction between warming climates and thermal biology traits.
With high-elevation population of T. kuehnei from the Diaoluoshan Mountains, this study monitored thermal environments, determined thermal biology traits, and calculated thermoregulatory effectiveness and TSM. By comparing the thermal biology traits, thermoregulatory effectiveness, and TSM with published data across latitudes (e.g., Zhang and Ji, 2004; Sunday et al., 2014; Hao et al., 2020), we aimed to evaluate the vulnerabilities of tropical species (i.e., T. kuehnei in this study) from high elevation to climate change and thus test whether mountains are potential refuges for tropical species under ongoing climate change. Base on the assumption that the ambient temperatures at high elevation in tropical areas are low on average with high variations, which are similar to that from medium and high latitudes (e.g., Ghalambor et al., 2006; Freeman et al., 2018), and the effects of thermal environments on thermal biology traits in Takydromus lizards (Hao et al., 2020), we predicted that the thermal biology traits of T. kuehnei from high elevation are similar to congeners from medium or high latitudes. Accordingly, we also predicted that T. kuehnei are not vulnerable to climate change by high thermoregulation effectiveness and big thermal safety margin. Further, we predicted that the high elevations (i.e., tropical mountains) can be potential refuges for tropical species to escape from exposure to climate change.
Fieldwork was performed at the Mt. Diaoluo National Reserve in Hainan, China (18°43′N, 109°52′E). We collected 20 adult T. kuehnei (12 males and 8 females) from a high-elevation population (height > 900 m) using either a noose or by hand.
During collection, we measured the active body temperature of T. kuehnei once the lizard was captured. We measured cloacal temperatures using an electronic thermocouple within 30 s of capture (UNT T-325, Shanghai, China). The active body temperatures of the lizards were also measured repeatedly in June when they were released.
During lizard collection, we also monitored the operative temperatures (Te) using copper tube models. The copper tube models mimicked the size of the lizards (diameter × length: 15 mm × 70 mm). Each model was inserted with an iButton (DS1921, MAXIM Integrated Products Ltd., United States). We randomly set the copper models exposed to full sun and shaded sites. The iButtons recorded the hourly temperature. We used two iButtons for each site, and the average of two iButtons for each site was calculated as Te. The Te collection lasted from April to June when the lizards were released.
After collection in April, we transported the lizards to the laboratory built in the Mt. Diaoluo National Reserve. We weighed (body mass, ± 0.01 g) and measured (SVL, ± 0.1 mm) the lizards and arranged every five lizards with different sexes in each plastic terrarium (150 cm × 40 cm × 50 cm, length × width × height) with moist soil. The terraria were set in a room where the temperature was maintained at 18 ± 1°C. A supplementary heating lamp was suspended above one end of each terrarium to create temperatures ranging from 18 to 40°C from 06:00 to 20:00. Food (crickets and larval Tenebrio molitor) and water were provided ad libitum. After three days of rearing, we started to determine the thermal biology traits. During the test, if the females were pregnant, we supplemented the test of the traits after they laying the eggs.
The measurements of Tsel were also conducted in a temperature-controlled room at 18 ± 1°C. To create a thermal gradient, we placed a large tank (1000 × 500 × 300 mm, length × width × height) and set a 275 W incandescent lamp above one end of the tank. The heating period was 06:00 to 20:00, which mimicked the natural light period. We followed two established protocols to measure the selected body temperature (Tsel). To facilitate comparison of the Tsel of T. kuehnei to published data from other Takydromus lizards, we first followed an old protocol (Ji et al., 1996; Zhang and Ji, 2004). Briefly, four to five lizards were introduced into the cold end of the terrarium at 16:00 on the first day. The next day, we measured body temperature (cloacae, within 30 s) for each individual at 09:00 and 15:00. The average of two measurements for each lizard was calculated as the selected body temperature (Tsel). To facilitate thermoregulatory accuracy and effectiveness comparison, we also determined the selected temperature range using a new protocol (Li et al., 2017). In brief, we measured body temperatures (cloacae, within 30 s) at each hour from 08:00 to 18:00. The selected temperature range was calculated as the central 50% of all recordings for each lizard.
We determined the critical thermal minimum (CTmin) and critical thermal maximum (CTmax) in a programmed incubator (KB 240, Binder, Germany). Before determination, the candidate was acclimated to 28°C for 2 h. Thereafter, the lizards were cooled/heated from 28°C at a rate of 1°C per 10 min. During the cooling/heating period, we constantly monitored the righting responses of the lizards. Once lizards lost the capacity to right themselves after being turned over, the body temperature (cloacae) was recorded as CTmin/CTmax. Thereafter, the lizards were immediately moved to 28°C for recovery. If lizards could not recover from the test, their records were eliminated from further analysis. In this study, all lizards recovered to normal after cooling or heating.
The locomotor performance of lizards was estimated by sprint speed at six test temperatures ranging from 18 to 38°C (18, 22, 26, 30, 34, and 38°C, in a randomized sequence). Before the measurement, the lizards were acclimated in a temperature-controlled incubator at the test temperature for 2 h. Sprint speed was measured in a customer-made wood racetrack (1200 mm × 100 mm × 150 mm, length × width × height, with intervals marked every 200 mm) in a temperature-controlled room. The lizard was introduced into the racetrack from one end and stimulated by a paintbrush to run through the racetrack. The running processes were recorded using an HD video camera (DCR-SR220E, Sony, Japan). Each lizard was tested twice at the test temperature with an interval of 1 h for rest. We analyzed the video of locomotion with established methods (Shu et al., 2010; Sun et al., 2014). For each lizard, we recorded the fastest speed at 200 mm intervals for each test by raw numbers, and the average of the fastest records from each test was calculated as the sprint speed.
The resting metabolic rate of T. kuehnei was determined at six test temperatures (18, 22, 26, 30, 34, and 38°C) in a random order using a respirometry system (Sable Systems International, Henderson, NV, United States). We estimated the RMR by measuring the CO2 production rate as a proxy for published protocols (Sun et al., 2018b, 2020). The lizards were fasted for at least 12 h before the test and were acclimated for 2 h at the test temperature. The RMR of the lizards was determined using a closed-circuit system (volume = 281.4 mL). The lizard was housed at the test temperature in the chamber, which was placed in a temperature-controlled incubator (MIR554-PC, Sanyo, Japan). First, we set the system open to the air that had sucked up moisture through a tube with 300 ml/min flow rate to stabilize the baseline. After 3 min of opening to the air, the circuit system was transferred to be closed. We continuously recorded the rate of carbon dioxide production (VCO2) for at least 7 min in a closed-circuit system. The RMR was calculated as the carbon dioxide production per gram of body mass per hour (mL g–1 h–1), using the equation RMR = VCO2 × volume/body mass, where VCO2 is the CO2 production rate in percentage (%/h) in the closed-circuit system.
For the calculation of the thermoregulation accuracy (db), we followed published protocols (Li et al., 2017). If Tb was below (or above) the selected temperature range, db was calculated as the difference between the Tb and the lower (or upper) bound of the selected temperature range. Alternatively, if Tb was within the selected temperature range, db was zero. The Te values at day time from 07:00 to 17:00 were used to calculate de. If Te was below (or above) the selected temperature range, de was calculated as the difference between Te and the lower (or upper) bound of the selected temperature range. When Te was within the selected temperature range, de was zero. Finally, we used two indices, E and de – db, to estimate thermoregulatory effectiveness. E was calculated using the equation E = 1 – mean db/mean de, following published methods (Hertz et al., 1993; Li et al., 2017). The thermal safety margins (TSM) were calculated as the difference between Te, max (the mean maximum hourly Te) and CTmax, following the published protocol (Sunday et al., 2014).
The normality and homogeneity were checked using the Shapiro–Wilk test and Bartlett’s test, respectively. First, we analyzed sex differences in Tsel, CTmin, and CTmax by one-way ANOVA. Thereafter, we analyzed the Ta and Te with Kruskal–test between April and June, because they are independent in test time. We analyzed the difference in Te between the open and shaded locations using the Wilcoxon test. We tested between-month differences in Ta, Te, db, and de using the Wilcoxon test. We also analyzed the difference between Tsel and Ta, and Tsel and Te using the Wilcoxon test. For the analysis of locomotion, we used the modified Gaussian regression to fit the dependence of sprint speed on test temperatures using a published method (Hao et al., 2020). Subsequently, the optimal temperature for locomotion was calculated according to the regression. The thermal dependence of the resting metabolic rates was analyzed using the allometric growth curve to body temperature. If there was a significant difference between sexes in thermal biology traits, we supplementarily show the data with males and females separately.
Te differed between open and shaded sites (W = 482732, p < 0.001), and full open sites had significantly higher Te than sites under full shade (full shade, 24.07 ± 0.13°C; full sun, 27.26 ± 0.16°C) (Figure 1A). Te varied over time (Kruskal–Wallis Chi-squared = 1601.4, df = 23, p < 0.001) (Figure 1B). In addition, daily time Te in April (30.19 ± 0.28°C) was significantly higher than that in June (27.78 ± 0.21°C) (W = 227946, p < 0.001).
Figure 1. Hourly (A) and daily average (B) operative temperatures (Te) for high-elevation T. kuehnei in the field. (A) The Orange solid line and blue dash line indicate the Te of open and shade sites, respectively. (B) Orange spots and blue triangles indicate the Te of open and shade sites across the daytime, respectively. Data are shown as mean ± SE.
The Ta of T. kuehnei depended on the time of day in both April (Kruskal–Wallis Chi-squared = 115.62, df = 30, p < 0.001) (Figure 2A) and June (Kruskal–Wallis Chi-squared = 59.814, df = 31, p = 0.001) (Figure 2B). In contrast to the variation in Te between April and June, the Ta in April (26.28 ± 0.21°C) was significantly lower than that in June (30.65 ± 0.22°C) (W = 2051.5, p < 0.001) (Figure 2).
Figure 2. Active body temperatures of T. kuehnei of April (A) and June (B), respectively. The black spot represents the active body temperatures of each individual. The red solid and blue dash lines indicate the maximum and minimum operative temperatures, respectively. The orange shaded area represents the selected temperature range.
The selected body temperature (Tsel) for T. kuehnei was 33.23 ± 0.28°C by the old protocol. The range of Tsel for T. kuehnei was 28.2°C to 36.4°C, with the average value of Tsel being 32.12 ± 0.21°C by the new protocol. Sex did not affect Tsel by the old (F1,17 = 0.705, p = 0.413) or new protocol (F1,68 = 0.495, p = 0.484) (Table 1). The CTmin and CTmax for T. kuehnei were 4.79 ± 0.18°C and 42.37 ± 0.11°C, respectively. We found significant differences in CTmin (F1,17 = 8.828, p = 0.009) and CTmax (F1,17 = 6.241, p = 0.023) between males and females (Table 1).
Table 1. Selected body temperatures (Tsel), the critical thermal minimum (CTrm min), critical thermal maximum (CTmax), thermoregulatory accuracy (db, de), thermoregulatory effectiveness (E), and thermal-safety margins (TSM) of T. kuehnei.
The sprint speed for T. kuehnei was significantly dependent on body temperature; it increased with body temperature within the range of 18 to 30°C and then decreased at higher body temperatures from 30 to 38°C. According to the modified Gaussian regression, the optimal body temperature for maximum sprint speed was 30.60°C (Figure 3A). Metabolic rates were dependent on body temperature, with increasing allometrically against test temperatures (R2 = 0.82, p < 0.0001) (Figure 3B).
Figure 3. Locomotor performance (A) and resting metabolic rates (B) of high-elevation T. kuehnei at different body temperatures. The spots indicate the sprint speed (A) and RMR (B) at each test temperature, and the dash lines indicate the regression. The data are shown as mean ± SE.
Operative temperatures was significantly lower than the average Tsel (W = 20659, p < 0.001). Most Te values (N = 706) were below the lower threshold of the selected temperature range, 156 values were higher than the upper threshold of the selected temperature range, and 380 values were within the range of selected body temperatures. Accordingly, de did not vary between seasons (W = 189527, p = 0.8171). Ta was lower than Tsel in both April (W = 797, p < 0.001) and June (W = 2541, p < 0.001). In April, most Ta values (N = 115) were below the selected temperature range, and the remaining values (N = 28) were within the selected temperature range. In June, most Ta values (N = 109) were in the selected temperature range, and four values were higher than those above the selected temperature range. Accordingly, the db in June was lower than in April (W = 15748, p < 0.001, Table 1). In addition, the de of T. kuehnei was higher than that of the db in April and June. Therefore, the de - db and E values were positive (Table 1). Similarly, all TSM values were positive, and the average TSM for T. kuehnei was 1.23°C (Figure 4 and Table 1).
Figure 4. Thermal-safety margins (TSM) of lizards across latitudes. Each spot indicates a TSM of each species and the red triangle represents the TSM of high-elevation T. kuehnei. The orange line represents the fitting curve of TSM along the global latitude and the gray area represents the 95% confidence interval. The database was collected from published analysis (Sunday et al., 2014).
Tropical (i.e., low latitude) species are proposed to be more vulnerable to climate change than those from subtropical and temperate regions (i.e., medium and high latitudes) (e.g., Deutsch et al., 2008; Dillon et al., 2010; Sunday et al., 2019). However, high-elevation regions (i.e., tropical mountains) are potential refuges for species under climate change (Forero-Medina et al., 2011; Freeman et al., 2018; Meng et al., 2019). Therefore, it is critical to determine the thermal biology and thus the vulnerabilities to climate change of species from tropical mountains (i.e., high elevations), providing a reference for species migration toward high elevations (Huey et al., 2009). According to the thermal biology traits, thermoregulatory effectiveness, and thermal safety margin, we found that the high-elevation population of T. kuehnei is not a severe threat to climate change, implying that tropical mountains are available for tropical species in escaping the exposure to climate change (e.g., Freeman et al., 2018).
As ectotherms, most aspects of behavior and physiology in reptiles are profoundly affected by temperature, making reptiles an important model for studying the vulnerabilities of species to climate change (Huey et al., 2010, 2012). Accordingly, numerous studies have focused on the response of reptiles to thermal variation and climate change (see details in Huey and Berrigan, 2001; Taylor et al., 2020). Comparing biological traits among populations and species is an essential way to evaluate the responses of species to climate change across geographical clues (Bacigalupe et al., 2018; Taylor et al., 2020). Takydromus lizards are distributed from the northernmost Heilongjiang province to the southernmost Hainan province in China (Du et al., 2010; Cai et al., 2012; Ma et al., 2019; Portniagina et al., 2019). Previous studies have summarized the thermal biology traits of the genus Takydromus distributed in China across latitudes (Hao et al., 2020). The Tsel of the genus Takydromus lizards tends to decrease toward low latitudes. However, in contrast to the current pattern, we found that Tsel of T. kuehnei from the high-elevation population is 33.23 ± 0.28°C, which is the highest among the five Takydromus lizards with published data collected by the same protocols (i.e., old protocol in this study) (Ji et al., 1996; Chen et al., 2003; Zhang and Ji, 2004; Hao et al., 2020). Similarly, thermal tolerance does not fit the current latitudinal pattern. Our previous study indicated that the thermal tolerance range increased toward high latitudes by increasing CTmax and decreasing CTmin (Hao et al., 2020). However, in the high-elevation population of T. kuehnei, CTmax and CTmin are similar to those of medium-altitude species (i.e., T. septentrionalis) (Ji et al., 1996; Hao et al., 2020).
In this study, the Ta for T. kuehnei from the high-elevation population was lower than congeners (i.e., T. septentrionalis, Sun et al., 2018a) in both April (26.28°C) and June (30.65°C), although it is a tropical lizard under high Te (Figures 2A,B). In addition, although Ta exhibited significant seasonal variation, only 1.6% (4/246) records were higher than the selected body temperature range, which is opposite to the current situation in tropical species where most body temperatures are near or higher than selected body temperatures (Huey et al., 2009). Furthermore, db in June was lower than in April, indicating that the lizards in June expressed higher thermoregulatory accuracy in maintaining their body temperatures within the range of thermal preference. The seasonal variation in higher Ta under lower Te (Table 1 and Figures 1, 2) appears opposite to the general knowledge that the body temperatures of lizards are subject to ambient temperatures (Huey, 1982). Lizards have different thermoregulatory strategies to adapt to seasonal temperatures (Vicente Liz et al., 2019). Lizards may select a low body temperature in the hot season to avoid exposure to ambient heat temperatures (Firth and Belan, 1998). The higher selected body temperatures in the mild season are probably because mild-season temperatures can relieve lizard exposure to the critical thermal limits (Huey, 1982; Vicente Liz et al., 2019). Accordingly, lizards may enhance activity and facilitate the maintenance of the optimal temperature at a low cost in a mild season (Huey and Slatkin, 1976). In this study, the thermoregulatory effectiveness (i.e., E) of high-elevation T. kuehnei in June was higher than that in April (Table 1), indicating that T. kuehnei in the mild season (i.e., June) allows higher effectiveness of thermoregulation. In comparison, high-elevation T. kuehnei buffers the impacts of warming temperatures by thermoregulation, indicating a high E value (E = 0.48) relative to other tropical lizards. For example, an endemic arboreal lizard from the tropical islands of Mexico, Anolis allisoni (E = −0.3), and Ctenosaura oaxacana (E = 0.13) have low thermoregulatory effectiveness (Valenzuela-Ceballos et al., 2015; Medina et al., 2016).
Thermal-safety margins has been considered as a primary index for evaluating the vulnerability of species to extreme heat (e.g., Deutsch et al., 2008; Sunday et al., 2014). Previous studies revealed that TSM decreased toward low latitudes (i.e., tropical areas), where the TSM of most species was below zero (see details in Sunday et al., 2014). However, this study found that the TSM of high-elevation T. kuehnei is higher than zero, as high as that of lizards from medium and high latitudes around 40° (Figure 4). The TSM was determined using CTmax and Te max. However, the CTmax in lizards is conservative across latitudes because lizards cannot effectively increase the temperature tolerance in the face of rapidly rising temperatures due to climate change (e.g., Sunday et al., 2011; Logan et al., 2014). Therefore, the mild thermal environment, thus Te, can result in a high TSM for high-elevation T. kuehnei.
Generally, high thermoregulatory accuracy and large TSM predict high-elevation T. kuehnei would not be threatened by ongoing climate change in the future. Higher E allows T. kuehnei to regulate body temperatures with high effectiveness under thermal fluctuance (e.g., Li et al., 2017), and large TSM indicates that T. kuehnei is not under the stress of extreme heat (e.g., Sunday et al., 2014).
The primary reason for the lower risk of threat of T. kuehnei in this study may be that the population is collected from high elevations (>900m). In contrast, other Takydromus lizards were collected from low elevations (i.e., ∼200 m to ∼ 290 m) (Hao et al., 2020). High elevation in tropical regions can provide more fluctuant but lower average temperatures than low elevations (e.g., Leuschner, 2000; de Carvalho et al., 2019), which facilitates behavioral thermoregulation and increases the TSM. A large TSM at high elevation is possibly induced by decreased Te max, although normal CTmax (Sunday et al., 2019). The climate variability hypothesis (CVH) posits that tropical organisms in warm and stable thermal environments should possess lower plasticity in their behavioral and physiological responses to thermal variation (Ghalambor et al., 2006; Gaston et al., 2009). In contrast, species from high-latitude or high-elevation habitats in more fluctuating thermal environments, and thus can tolerate or thrive over a broader range of temperatures, indicating a larger thermal safety margin due to being more resilient to climate change (Addobediako et al., 2000; Sunday et al., 2019). For example, the population of Podarcis hispanica from high elevation selected significantly higher body temperatures in the thermal gradient than the population with low elevation (Gabirot et al., 2013).
In summary, T. kuehnei from high elevation expresses similar thermal biology traits to medium-latitude congeners and allows high thermoregulatory accuracy and effectiveness, as well as a large thermal safety margin. Therefore, T. kuehnei could maintain a safe state in tropical regions under climate change. However, this study has some limitations. First, we only focused on the alpine population (>900m), lacking a comparison to the low-elevation population, or some other species with a narrow distribution in elevation/latitude. In the future, further studies with more tropical populations across elevations are needed to understand the vulnerability of tropical species to climate change (Ghalambor et al., 2006). With more intra- and inter-population comparisons, we can also understand the diversity of adaptive strategies to climate change of tropical species (Huey et al., 2009).
The data supporting the conclusions of this article can be found in the Supplementary Material.
The animal study was reviewed and approved by Animal Ethics Committees at the Institute of Zoology, CAS (IOZ14001).
BS, JW, and RZ designed the studies and wrote the draft. ST, KC, XL, and XH collected the data. ST and BS analyzed the data. All authors contributed to revisions of the manuscript.
This work was supported by the National Natural Science Foundation of China (31870391 and 31500324) and Key Program of Zhejiang Provincial Natural Science Foundation of China (LZ17C030001). BS was supported by Youth Innovation Promotion Association CAS (No. 2019085). RZ was supported by Zhejiang Ten Thousand Talents Program (2018R52028).
The authors declare that the research was conducted in the absence of any commercial or financial relationships that could be construed as a potential conflict of interest.
All claims expressed in this article are solely those of the authors and do not necessarily represent those of their affiliated organizations, or those of the publisher, the editors and the reviewers. Any product that may be evaluated in this article, or claim that may be made by its manufacturer, is not guaranteed or endorsed by the publisher.
We thank Teng LI and Tingting Wang for their assistance in the field and Lab.
The Supplementary Material for this article can be found online at: https://www.frontiersin.org/articles/10.3389/fevo.2021.767102/full#supplementary-material
Addobediako, A., Chown, S. L., and Gaston, K. J. (2000). Thermal tolerance, climatic variability and latitude. Proc. Biol. Sci. 267, 739–745. doi: 10.1098/rspb.2000.1065
Angilletta, M. J. Jr., Niewiarowski, P. H., and Navas, C. A. J. (2002). The evolution of thermal physiology in ectotherms. J. Therm. Biol. 27, 249–268. doi: 10.1016/s0306-4565(01)00094-8
Bacigalupe, L. D., Gaitán-Espitia, J. D., Barria, A. M., Gonzalez-Mendez, A., Ruiz-Aravena, M., Trinder, M., et al. (2018). Natural selection on plasticity of thermal traits in a highly seasonal environment. Evol. Appl. 11, 2004–2013. doi: 10.1111/eva.12702
Barnosky, A. D., Matzke, N., Tomiya, S., Wogan, G. O. U., Swartz, B., Quental, T. B., et al. (2011). Has the Earth’s sixth mass extinction already arrived? Nature 471, 51–57. doi: 10.1038/nature09678
Blouin-Demers, G., and Weatherhead, P. J. (2001). Thermal ecology of black rat snakes (Elaphe obsoleta) in a thermally challenging environment. Ecology 82, 3025–3043. doi: 10.2307/2679832
Bonebrake, T. C., and Deutsch, C. A. (2012). Climate heterogeneity modulates impact of warming on tropical insects. Ecology 93, 449–455. doi: 10.1890/11-1187.1
Cai, Y., Yan, J., Xu, X., Lin, Z., and Ji, X. (2012). Mitochondrial DNA phylogeography reveals a west-east division of the northern grass lizard (Takydromus septentrionalis) endemic to China. J. Zool. Syst. Evol. Res. 50, 137–144. doi: 10.1111/j.1439-0469.2012.00655.x
Chen, X., Xu, X., and Ji, X. (2003). Influence of body temperature on food assimilation and locomotor performance in white-striped grass lizards, Takydromus wolteri (Lacertidae). J. Therm. Biol. 28, 385–391. doi: 10.1016/s0306-4565(03)00022-6
Clusella-Trullas, S., Garcia, R. A., Terblanche, J. S., and Hoffmann, A. A. (2021). How useful are thermal vulnerability indices? Trends Ecol. Evol. doi: 10.1016/j.ree.2021.07.001 [Epub ahead of print].
de Carvalho, W. D., Martins, M. A., Esbérard, C. E. L., and Palmeirim, J. M. (2019). Traits that allow bats of tropical lowland origin to conquer mountains: bat assemblages along elevational gradients in the South American Atlantic Forest. J. Biogeogr. 46, 316–331. doi: 10.1111/jbi.13506
Deutsch, C. A., Tewksbury, J. J., Huey, R. B., Sheldon, K. S., Ghalambor, C. K., Haak, D. C., et al. (2008). Impacts of climate warming on terrestrial ectotherms across latitude. Proc. Natl. Acad. Sci. U.S.A. 105, 6668–6672.
Diele-Viegas, L. M., Figueroa, R. T., Vilela, B., and Rocha, C. F. D. (2020). Are reptiles toast? A worldwide evaluation of Lepidosauria vulnerability to climate change. Clim. Change. 159, 581–599. doi: 10.1007/s10584-020-02687-5
Dillon, M. E., Wang, G., and Huey, R. B. (2010). Global metabolic impacts of recent climate warming. Nature 467, 704–706. doi: 10.1038/nature09407
Du, W., Ji, X., Zhang, Y., Lin, Z., and Xu, X. (2010). Geographic variation in offspring size of a widespread lizard (Takydromus septentrionalis): importance of maternal investment. Biol. J. Linnean Soc. 101, 59–67.
Firth, B. T., and Belan, I. J. P. Z. (1998). Daily and seasonal rhythms in selected body temperatures in the Australian lizard Tiliqua rugosa (Scincidae): field and laboratory observations. Physiol. Zool. 71, 303–311. doi: 10.1086/515919
Forero-Medina, G., Joppa, L., and Pimm, S. L. (2011). Constraints to species’ elevational range shifts as climate changes. Conserv. Biol. 25, 163–171. doi: 10.1111/j.1523-1739.2010.01572.x
Freeman, B. G., Scholer, M. N., Ruiz-Gutierrez, V., and Fitzpatrick, J. W. (2018). Climate change causes upslope shifts and mountaintop extirpations in a tropical bird community. Proc. Natl. Acad. Sci. U.S.A. 115, 11982–11987.
Gabirot, M., Balleri, A., López, P., and José, M. (2013). Differences in thermal biology between two morphologically distinct populations of Iberian wall lizards inhabiting different environments. Ann. Zool. Fennici 50, 225–236. doi: 10.5735/085.050.0406
Gaston, K. J., Chown, S. L., Calosi, P., Bernardo, J., Bilton, D. T., Clarke, A., et al. (2009). Macrophysiology: a conceptual reunification. Am. Natt. 174, 595–612. doi: 10.1086/605982
Ghalambor, C. K., Huey, R. B., Martin, P. R., Tewksbury, J. J., and Wang, G. (2006). Are mountain passes higher in the tropics? Janzen’s hypothesis revisited. Integr. Comp. Biol. 46, 5–17. doi: 10.1093/icb/icj003
Hao, X., Tao, S., Meng, Y., Liu, J., Cui, L., Liu, W., et al. (2020). Thermal biology of cold-climate distributed Heilongjiang grass lizard, Takydromus amurensis. Asian Herpetol. Res. 11, 350–359.
Hertz, P. E., Huey, R. B., and Stevenson, R. (1993). Evaluating temperature regulation by field-active ectotherms: the fallacy of the inappropriate question. Am. Nat. 142, 796–818. doi: 10.1086/285573
Huey, R. B., and Berrigan, D. (2001). Temperature, demography, and ectotherm fitness. Am. Nat. 158, 204–210. doi: 10.2307/3079165
Huey, R. B., and Kingsolver, J. G. (1989). Evolution of thermal sensitivity of ectotherm performance. Trends Ecol. Evol. 4, 131–135. doi: 10.1016/0169-5347(89)90211-5
Huey, R. B., Deutsch, C. A., Tewksbury, J. J., Vitt, L. J., Hertz, P. E., Álvarez Pérez, H. J., et al. (2009). Why tropical forest lizards are vulnerable to climate warming. Proc. R. Soc. B Biol. Sci. 276, 1939–1948. doi: 10.1098/rspb.2008.1957
Huey, R. B., Kearney, M. R., Krockenberger, A., Holtum, J. A. M., Jess, M., and Williams, S. E. (2012). Predicting organismal vulnerability to climate warming: roles of behaviour, physiology and adaptation. R. Soc. Philos. Trans. Biol. Sci. 367, 1665–1679. doi: 10.1098/rstb.2012.0005
Huey, R. B., Losos, J. B., and Moritz, C. (2010). Are lizards toast? Science. 328, 832–833. doi: 10.1126/science.1190374
Huey, R., and Slatkin, M. (1976). Cost and benefits of lizard thermoregulation. Q. Rev. Biol. 51, 363–384. doi: 10.1086/409470
Ji, X., Du, W., and Sun, P. (1996). Body temperature, thermal tolerance and influence of temperature on sprint speed and food assimilation in adult grass lizards, Takydromus septentrionalis. J. Therm. Biol. 21, 155–161. doi: 10.1016/0306-4565(95)00037-2
Leuschner, C. (2000). Are high elevations in tropical mountains arid environments for plants? Ecology 81, 1425–1436. doi: 10.1890/0012-9658(2000)081[1425:aheitm]2.0.co;2
Li, S. R., Wang, Y., Ma, L., Zeng, Z. G., Bi, J. H., and Du, W. G. (2017). Thermal ecology of three coexistent desert lizards: implications for habitat divergence and thermal vulnerability. J. Comp. Physiol. B Biochem. Syst. Environ. Physiol. 187, 1009–1018. doi: 10.1007/s00360-017-1087-4
Lin, S., Chen, C. A., and Lue, K. (2002). Molecular phylogeny and biogeography of the grass lizards genus Takydromus (Reptilia: Lacertidae) of East Asia. Mol. Phylogenet. Evol. 22, 276–288. doi: 10.1006/mpev.2001.1059
Logan, M. L., Cox, R. M., and Calsbeek, R. (2014). Natural selection on thermal performance in a novel thermal environment. Proc. Natl. Acad. Sci. U.S.A. 111, 14165–14169. doi: 10.1073/pnas.1404885111
Ma, L., Liu, P., Su, S., Luo, L. G., Zhao, W. G., and Ji, X. (2019). Life-history consequences of local adaptation in lizards: Takydromus wolteri (Lacertidae) as a model organism. Biol. J. Linnean Soc. 127, 88–99. doi: 10.1093/biolinnean/blz024
Medina, M., Fernandez, J. B., Charruau, P., De La Cruz, F. M., and Ibargungoytia, N. (2016). Vulnerability to climate change of Anolis allisoni in the mangrove habitats of Banco Chinchorro Islands, Mexico. J. Therm. Biol. 58, 8–14. doi: 10.1016/j.jtherbio.2016.02.005
Meng, H. H., Zhou, S. S., Jiang, X. L., Gugger, P. F., Li, L., Tan, Y. H., et al. (2019). Are mountaintops climate refugia for plants under global warming? A lesson from high-mountain oaks in tropical rainforest. Alpine Bot. 129, 175–183. doi: 10.1007/s00035-019-00226-2
ObregÓn, R. L., Scolaro, J. A., IbargÜengoytÍa, N. R., and Medina, M. (2020). Thermal biology and locomotor performance in Phymaturus calcogaster: are Patagonian lizards vulnerable to climate change? Integr. Zool. 16, 53–66. doi: 10.1111/1749-4877.12481
Portniagina, E. Y., Maslova, I. V., and Han, S. H. (2019). Habitat and altitudinal distribution of two lizard species of genus Takydromus from the Northeast Asia (Far East of Russia, Republic of Korea). Russ. J. Herpetol. 26, 8–16.
Root, T. L., Price, J. T., Hall, K. R., Schneider, S. H., Rosenzweig, C., and Pounds, J. A. (2003). Fingerprints of global warming on wild animals and plants. Nature 421, 57–60. doi: 10.1038/nature01333
Shu, L., Sun, B., and Du, W. (2010). Effects of temperature and food availability on selected body temperature and locomotor performance of Plestiodon (Eumeces) chinensis (Scincidae). Anim. Biol. 60, 337–347. doi: 10.1163/157075610x516547
Sinervo, B., Mendez-De-La-Cruz, F., Miles, D. B., Heulin, B., Bastiaans, E., Villagran-Santa Cruz, M., et al. (2010). Erosion of lizard diversity by climate change and altered thermal niches. Science 328, 894–899. doi: 10.1126/science.1184695
Sun, B. J., Huebner, C., Treidel, L. A., Clark, R. M., Roberts, K. T., Kenagy, G. J., et al. (2020). Nocturnal dispersal flight of crickets: behavioural and physiological responses to cool environmental temperatures. Funct. Ecol. 34, 1907–1920. doi: 10.1111/1365-2435.13615
Sun, B. J., Ma, L., Li, S. R., Williams, C. M., Wang, Y., Hao, X., et al. (2018b). Phenology and the physiological niche are co-adapted in a desert-dwelling lizard. Funct. Ecol. 32, 2520–2530. doi: 10.1111/1365-2435.13201
Sun, B. J., Tang, W. Q., Zeng, Z. G., and Du, W. G. (2014). The seasonal acclimatisation of locomotion in a terrestrial reptile, Plestiodon chinensis (Scincidae). Asian Herpetol. Res. 5, 197–203. doi: 10.3724/sp.j.1245.2014.00197
Sun, B. J., Wang, Y., Wang, Y., Lu, H. L., and Du, W. G. (2018a). Anticipatory parental effects in a subtropical lizard in response to experimental warming. Front. Zool. 15:51.
Sunday, J. M., Bates, A. E., and Dulvy, N. K. (2011). Global analysis of thermal tolerance and latitude in ectotherms. Proc. R. Soc. B Biol. Sci. 278, 1823–1830. doi: 10.1098/rspb.2010.1295
Sunday, J. M., Bates, A. E., Kearney, M. R., Colwell, R. K., Dulvy, N. K., Longino, J. T., et al. (2014). Thermal-safety margins and the necessity of thermoregulatory behavior across latitude and elevation. Proc. Natl. Acad. Sci. U.S.A. 111, 5610–5615.
Sunday, J., Bennett, J. M., Calosi, P., Clusella-Trullas, S., Gravel, S., Hargreaves, A. L., et al. (2019). Thermal tolerance patterns across latitude and elevation. Philos. Trans. R. Soc. Lond. B Biol. Sci. 374:20190036. doi: 10.1098/rstb.2019.0036
Taylor, E. N., Diele-Viegas, L. M., Gangloff, E. J., Hall, J. M., Halpern, B., Massey, M. D., et al. (2020). The thermal ecology and physiology of reptiles and amphibians: a user’s guide. J. Exp. Zool. Part A Ecol. Integr. Physiol. 335, 13–44.
Thomas, C. D., Cameron, A., Green, R. E., Bakkenes, M., Beaumont, L. J., Collingham, Y. C., et al. (2004). Extinction risk from climate change. Nature 427, 145–148.
Valenzuela-Ceballos, S., Castañeda, G., Rioja-Paradela, T., Carrillo-Reyes, A., and Bastiaans, E. J. J. O. T. B. (2015). Variation in the thermal ecology of an endemic iguana from Mexico reduces its vulnerability to global warming. J. Therm. Biol. 48, 56–64. doi: 10.1016/j.jtherbio.2014.12.011
Vicente Liz, A., Santos, V., Ribeiro, T., Guimarães, M., and Verrastro, L. (2019). Are lizards sensitive to anomalous seasonal temperatures? Long-term thermobiological variability in a subtropical species. PLos One 14:e0226399. doi: 10.1371/journal.pone.0226399
Wang, J. (2014). Wild Vertebrate in Diaoluoshan, Hainan, China (In Chinese). Beijing: China Forestry Publishing House.
Zhang, Y., and Ji, X. (2004). The thermal dependence of food assimilation and locomotor performance in southern grass lizards, Takydromus sexlineatus (Lacertidae). J. Therm. Biol. 29, 45–53. doi: 10.1016/j.jtherbio.2003.10.007
Keywords: climate change, tropical mountains, lizards, thermal biology, thermoregulatory effectiveness, thermal safety margin, Takydromus kuehnei, high elevation
Citation: Tao S, Cheng K, Li X, Han X, Wang J, Zheng R and Sun B (2021) The Thermal Biology of Takydromus kuehnei Indicates Tropical Lizards From High Elevation Have Not Been Severely Threatened by Climate Change. Front. Ecol. Evol. 9:767102. doi: 10.3389/fevo.2021.767102
Received: 30 August 2021; Accepted: 27 September 2021;
Published: 21 October 2021.
Edited by:
Giovanna Battipaglia, University of Campania Luigi Vanvitelli, ItalyReviewed by:
Liyun Yin, Hebei Normal University, ChinaCopyright © 2021 Tao, Cheng, Li, Han, Wang, Zheng and Sun. This is an open-access article distributed under the terms of the Creative Commons Attribution License (CC BY). The use, distribution or reproduction in other forums is permitted, provided the original author(s) and the copyright owner(s) are credited and that the original publication in this journal is cited, in accordance with accepted academic practice. No use, distribution or reproduction is permitted which does not comply with these terms.
*Correspondence: Jichao Wang, d2pjQGhhaW5udS5lZHUuY24=; Rongquan Zheng, emhlbmdycUB6am51LmNu
†These authors have contributed equally to this work
Disclaimer: All claims expressed in this article are solely those of the authors and do not necessarily represent those of their affiliated organizations, or those of the publisher, the editors and the reviewers. Any product that may be evaluated in this article or claim that may be made by its manufacturer is not guaranteed or endorsed by the publisher.
Research integrity at Frontiers
Learn more about the work of our research integrity team to safeguard the quality of each article we publish.