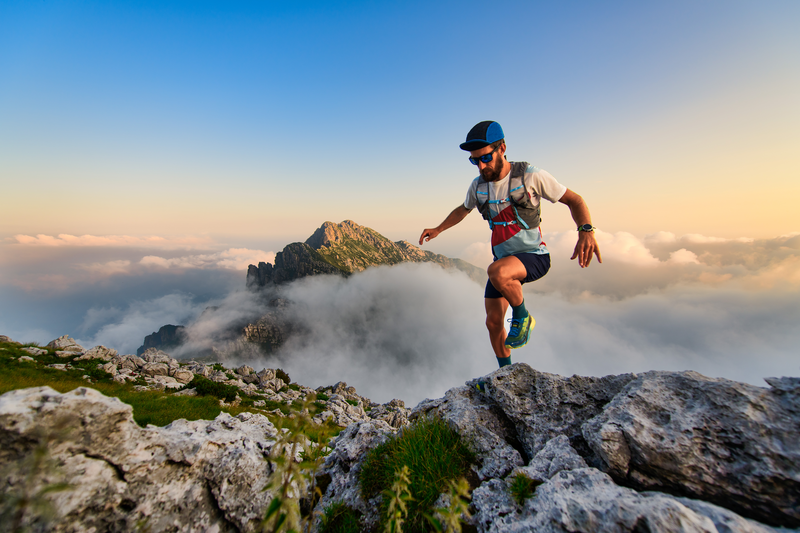
94% of researchers rate our articles as excellent or good
Learn more about the work of our research integrity team to safeguard the quality of each article we publish.
Find out more
ORIGINAL RESEARCH article
Front. Ecol. Evol. , 11 October 2021
Sec. Conservation and Restoration Ecology
Volume 9 - 2021 | https://doi.org/10.3389/fevo.2021.757943
This article is part of the Research Topic Grassland Conservation in Asia: Sustainability Under Climate Change View all 28 articles
Asymmetrical warming between elevations is a common phenomenon and warming magnitude increases with increasing elevations on the Tibetan Plateau, which in turn may reduce temperature differences between elevations. However, it is still unclear how such phenomenon will affect plant community composition in alpine grasslands on the Tibetan Plateau. Therefore, in this study, we performed an experiment at three elevations (i.e., 4,300 m, 4,500 m, and 4,700 m) in alpine grasslands, the Northern Tibetan Plateau since May, 2010. Open top chambers were established at the elevations 4,500 m and 4,700 m. Plant species and phylogenetic composition were investigated in August, 2011–2019. There were no significant differences in plant species and phylogenetic composition, environmental temperature and moisture conditions between the elevation 4,300 m under non-warming conditions and the elevation 4,500 m under warming conditions in 2019. There were also no significant differences in plant species composition, environmental temperature and moisture conditions between the elevation 4,500 m under non-warming conditions and the elevation 4,700 m under warming conditions in 2019. Therefore, the narrowing temperature differences between elevations may result in plant community composition between elevations tending to be similar in alpine grasslands on the Tibetan Plateau under future elevational asymmetrical warming.
Plant community structure is one of the most important plant characteristics, and understanding its changes under environmental changes plays important roles in predicting plant community change under global change (Hautier et al., 2014; Ma et al., 2017). Plant community structure can include species and phylogenetic structure, and they can reflect plant community composition from different aspects (Sun et al., 2021). Climate warming is one of the characteristics of global change and its magnitude can increase with increasing elevation in some regions (e.g., Tibetan Plateau) (Yao et al., 2000; Kuang and Jiao, 2016). This phenomenon can reduce temperature differences between adjacent elevations, which in turn may reduce the differences in plant community composition (i.e., plant β-diversity) between adjacent elevations. Various grasslands are important pastures at different temporal and spatial scales, which in turn provide animal by-products (e.g., milk and beef) for human (Sun et al., 2019). Alpine grasslands, as an important grassland type, may be one of the most sensitive grasslands to climate change and human activities at global and regional scales (Klein et al., 2007). Therefore, it is very important to understand the changes in plant community composition to global change in alpine grasslands.
The Tibetan Plateau, with the important characteristics of high-elevation, cold and dry climate conditions, is one of the key sensitive regions to global change and a global epicenter of global change (Li, 2017). Generally, alpine meadows, alpine steppes and alpine desert steppes are distributed from east to west on the Tibetan Plateau (Wu et al., 2014). Air temperature at a relative higher elevation can tend to be closer and closer to that at a relative lower elevation in alpine grasslands on the Tibetan Plateau under future asymmetrical warming (Yao et al., 2000). More and more studies have investigated the changes of plant community composition under various global climate changes in alpine grasslands on the Tibetan Plateau (Klein et al., 2007; Wang et al., 2012). However, no studies have tried to investigate the effects of reduced temperature differences between elevations on plant community composition in alpine grasslands on the Tibetan Plateau under global warming. Therefore, in this study, we performed an experiment along an elevation gradient from 4,300 m to 4,700 m (i.e., 4,300 m, 4,500 m, and 4,700 m) in alpine grasslands, the Northern Tibetan Plateau. We established four open top chambers, which was used to simulate climate warming, at elevations 4,500 m and 4,700 m, respectively. We compared the plant community composition under the control conditions at the elevation 4,300 m and that under the warming conditions at the elevation 4,500 m, that under control conditions at the elevation 4,300 m and that under the warming conditions at the elevation 4,700 m, and that under control conditions at the elevation 4,500 m and that under the warming conditions at the elevation 4,700 m.
Detailed descriptions on climate, soil and vegetation conditions can be found in our previous studies (Fu et al., 2019). Briefly, the study area was located at the Grassland Station of Damxung County, Lhasa City, Tibet, China. Mean annual temperature and mean annual precipitation was 1.96°C and 476.4 mm in 1963–2019, respectively. This experiment was conducted at three alpine grassland sites (site A: 30°30′ N, 91°04′ E, alpine steppe meadow; site B: 30°31′ N, 91°04′ E, alpine steppe meadow; site C: 30°32′ N, 91°03′ E, alpine meadow) since May 2010. The dominant plant species included Stipa capillacea, Carex montis-everestii and Kobresia pygmaea in the two alpine steppe meadows, and Kobresia pygmaea in the alpine meadow. Open top chambers (bottom diameter: 1.45 m; top diameter: 1.00 m; height: 0.40 m) were used to increase temperature at sites B and C. There were four treatments with four replicates, including the control treatment at site A with an elevation of about 4,300 m (C4300), the control treatment at site B with an elevation of about 4,500 m (C4500), warming treatment at site B with an elevation of about 4,500 m (W4500), and warming treatment at site C with an elevation of about 4,700 m (W4700).
We observed soil temperature (Ts, 5 cm), soil moisture (SM, 10 cm), air temperature (Ta, 15cm) and relative humidity (RH, 15 cm) using HOBO weather stations (Onset Computer, Bourne, MA, United States) during growing season (June–September) in 2011–2019. The sampling frequency and recording interval was 1 min and 30 min, respectively. We also calculated vapor pressure deficit (VPD) from measured Ta and RH using VPD = 0.6108 × exp[(17.27 × Ta)/(Ta + 237.3)] × (1-RH/100).
We performed plant community investigation for each plot in August, 2011–2019. Species coverage and height were recorded for each species within each of the sixteen 50 cm × 50 cm quadrats (four treatments × four replicates = 16 quadrats). We collected topsoil (0–10 cm) samples in August, 2013 and 2015–2019. We sieved these fresh soils through a 1 mm hole and then measured ammonium nitrogen (NH4+-N), nitrate nitrogen (NO3–-N), available phosphorus (AP) and pH.
The “geodist” of SPAA-package was used to obtain the geographic distance between elevations. The important value was calculated from measured species coverage and height. The “vegdist” of the Vegan-package was used to obtain species β-diversity (i.e., βBray, the dissimilarity indices of Bray-Curtis) between two treatments based on the important value. We used the “TPL” and “taxa.table” of the Plantlist-package to obtain the taxonomy data, which were imported into the Phylomatic software to generate the phylogenetic tree. The important value and phylogenetic tree were imported into “comdistnt” of the Picante-package to obtain phylogenetic β-diversity (i.e., βMNTD: beta mean nearest taxon distance) between two treatments. We also used the permutational multivariate analysis of variance to analyze the difference of βBray and βMNTD between treatments for each year. The “varpart” of the Vegan-package was used to partition the variation of the βBray and βMNTD by four explanatory matrices of biotic and/or abiotic variables. All the significant analyses were examined at p < 0.05. The drawing software included the SigmaPlot 12.5 and Microsoft Office Visio 2007.
No differences of multi-year mean SM, Ta and VPD were observed between the C4300 and W4500 treatments, and between the C4500 and W4700 treatments over the nine growing seasons in 2011–2019 (Table 1). The multi-year mean Ts of the W4500 treatment was 0.39°C greater than that of the C4300 treatment over the nine growing seasons in 2011–2019 (Table 1). The multi-year average Ts of the W4700 treatment was 0.56°C lower than that of the C4500 treatment over the nine growing seasons in 2011–2019 (Table 1). The multi-year mean NH4+-N and NO3–-N of the W4500 treatment was 3.96% (0.39 mg kg–1) and 21.33% (2.88 mg kg–1) greater than that of the C4300 treatment, but the multi-year mean pH of the W4500 treatment was 7.22% (0.49) lower than that of the C4300 treatment over the six growing seasons (i.e., 2013 and 2015–2019), respectively (Table 1). The multi-year mean NH4+-N of the W4700 treatment was 117.45% (13.06 mg kg–1) greater than that of the C4500 treatment, but the multi-year mean pH of the W4700 treatment was 10.31% (0.66) lower than that of the C4500 treatment over the six growing seasons (i.e., 2013 and 2015–2019), respectively (Table 1). There were no significances of multi-year mean NO3–-N and AP between the C4300 and W4700 treatments over the six growing seasons (i.e., 2013 and 2015–2019), respectively (Table 1). The multi-year mean Ts, Ta, VPD and pH of the W4700 treatment was 1.49°C, 0.83°C, 0.06 kPa and 1.05 lower than that of the C4300 treatment, respectively (Table 1). The multi-year mean SM and NH4+-N of the W4700 treatment was 0.04 m3 m–3 and 14.32 mg kg–1 greater than that of the C4300 treatment, respectively (Table 1).
Table 1. Comparison of average soil temperature (Ts), soil moisture (SM), air temperature (Ta) and vapor pressure deficit (VPD) across the nine growing seasons (i.e., 2011–2019), ammonium nitrogen (NH4+-N, mg kg–1), nitrate nitrogen (NO3–-N, mg kg–1), available phosphorus (AP, mg kg–1) and pH across the six growing seasons (i.e., 2013 and 2015–2019) between the control treatment at site A (C4300) and the warming treatment at site B (W4500), between C4300 and the warming treatment at site C (W4700), and between the control treatment at site B (C4500) and the W4700 treatment, respectively.
Across all the 9 years, both the species and phylogenetic β-diversity between the C4300 and W4500 treatments, between the C4300 and W4700 treatments, and between the C4500 and W4700 treatments were significant (Table 2). Both the species and phylogenetic β-diversity between the C4300 and W4700 treatments were significant for each year in 2011–2019 (Figure 1). However, the species and phylogenetic β-diversity between the C4300 and W4500 treatments were significant in 2011–2018 but not in 2019 (Figure 1). Meanwhile, the Ts, SM, Ta, and VPD between the C4300 and W4500 treatments were not significant in 2019 (Supplementary Table 1). The species β-diversity between the C4500 and W4700 treatments were significant in 2011–2018 but not in 2019 (Figure 1). Meanwhile, the Ts, SM, Ta, and VPD between the C4500 and W4700 treatments were not significant in 2019 (Supplementary Table 1). The phylogenic β-diversity between the C4500 and W4700 treatments were significant for each year in 2011–2019 (Figure 1).
Table 2. The two-way permutational multivariate analysis of variance (i.e., the “adonis2” of Vegan-package) for analyzing the differences of species β-diversity (βBray) and phylogenetic β-diversity (βMNTD) between C4300 vs. W4500, C4300 vs. W4700, or C4500 vs. W4700), and the effects of measuring year on the βBray and βMNTD across the 9 years in 2011–2019.
Figure 1. Species β-diversity (βBray, A–C) and phylogenetic β-diversity (βMNTD, D–F) between the control treatment at site A vs. the warming treatment at site B (C4300 vs. W4500), the C4300 treatment and the warming treatment at site C (C4300 vs. W4700), and the C4500 treatment and the W4700 treatment (C4500 vs. W4700). ∗ above bars indicates the βBray or βMNTD of the C4300 vs. W4500, C4300 vs. W4700, and C4500 vs. W4700 were significant at p < 0.05.
The species β-diversity increased with the Bray-Curtis dissimilarity index of Ts, SM, Ta, and pH between C4300 vs. W4500, C4300 vs. W4700 and C4500 vs. W4700 (Table 3). The GeoDist.Dur., Temp.Water, soil nutrition and pH together explained about 40% and 46% variations of species and phylogenetic β-diversity, respectively (Figure 2). The exclusive effects on species β-diversity were ordered by pH, Temp.Water, soil nutrition and GeoDist.Dur (Figure 2). The Temp.Water and soil nutrition but not GeoDist.Dur. and pH had exclusive effects on the variations of phylogenetic β-diversity (Figure 2).
Table 3. Correlation analyses of species and phylogenetic β-diversity with the Bray-Curtis dissimilarity index of environmental variables, between the control treatment at site A (C4300) and the warming treatment at site B (W4500), between the C4300 treatment and the warming treatment at site C (W4700), and between the control treatment at site B (C4500) and the W4700 treatment.
Figure 2. Venn plots of variation partitioning analysis, showing the shared and exclusive effects of the geographic distance and warming duration (GeoDist.Dur.), the Bray-Curtis dissimilarity index of temperature and water availability (Temp.Water: soil temperature, soil moisture, air temperature and/or vapor pressure deficit), the Bray-Curtis dissimilarity index of soil nutrition (ammonium nitrogen, nitrate nitrogen, and/or available phosphorus) and the Bray-Curtis dissimilarity index of pH between C4300 vs. W4500, C4300 vs. W4700, and C4500 vs. W4700, on the (A) species β-diversity and (B) phylogenetic β-diversity between C4300 vs. W4500, C4300 vs. W4700, and C4500 vs. W4700. C4300: the control treatment at site A; C4500: the control treatment at site B; W4500: the warming treatment at site B; W4700: the warming treatment at site C.
Our findings showed that plant species composition and even phylogenetic structure may tend to be similar between two adjacent elevations when the differences in environmental temperature and water availability conditions were eliminated. This phenomenon confirmed some previous studies which demonstrated that plant community moved upward under climate warming (Walther et al., 2005; Wang et al., 2019; Cheng et al., 2020). This finding may be attributed to one or more of the following underlying mechanisms. First, trade-off between lifespan and growth rate is a key character of plants, and plant species generally had a longer lifespan but a slower growth under colder climate conditions at high elevations and latitudes (Nobis and Schweingruber, 2013). Climate warming may alleviate low-temperature limitation on the growth of alpine plants and alter the trade-off between lifespan and growth rate, which subsequently may result in similarity of plant communities between the low and high elevations (Lenoir et al., 2008). Second, plant species with narrow niche width of temperature may move upward to avoid or reduce the risk of extinction under climate warming, and warming-tolerant species may move upward for expanding its spatial range (Pauli et al., 1999, 2012; Dolezal et al., 2016). Third, shallow- and deep-root species have different strategies in the utility of soil water, and shallow-root species are more dependent on upper soil water than deep-root species (Hu et al., 2013). Surface soil water may be mainly dependent on precipitation (Golluscio et al., 1998), and precipitation may increase with increasing elevation with a certain elevation range in the Tibet (Zhao et al., 2017). Warming may generally reduce availability water in soils (at least surface soil water) for plant growth and alter re-distribution of soil water at different depths (Wu et al., 2013; Xue et al., 2014). Subsequently, plants, especially shallow-rooted plants, may move upward to avoid or reduce the drought stress caused by climate warming. Fourth, wind stress can cause greater plant dwarfing at higher elevations considering that both wind speed and gale days may increase with increasing elevation (Friend and Woodward, 1990; Yao and Li, 2019). This phenomenon may limit upward migration of some species, although greater wind speed and gale days may increase the dispersal of wind-pollination plants. The reduced in wind speed and gale days may be related to climate warming, and their reduced magnitudes may increase with increasing elevations on the Tibetan Plateau (Yang et al., 2012; Yao and Li, 2019). This phenomenon may alleviate the effect of wind stress on plant community and subsequently increase the potential of some species upward.
Our findings implied that asymmetric warming between elevations/sites may affect the patch, which is one of basic elements of landscape pattern. Thus, such asymmetric warming may affect landscape diversity. However, our findings may have some limitations. First, the elevation only falls within about 4300–4700 m in our study. Thus, it may be necessary to investigate whether asymmetrical warming between elevations may result in similar plant community composition between elevations when the elevations beyond our elevational ranges. Second, our study only focused on two types of alpine grasslands (i.e., alpine steppe meadows and alpine meadows), while there are actually several other types of alpine grasslands (e.g., alpine steppes and alpine desert steppes) on the Tibetan Plateau (Wu et al., 2014). Thus, it is still unclear that asymmetrical warming between elevations can cause similar plant community composition between elevations in other grassland types. In summary, more studies are needed to resolve these uncertainties.
In this study, we simulated the narrowing temperature differences between elevations by setting up open top chambers at higher elevations, and then investigated the responses of plant community composition to such narrowing temperature differences between elevations in alpine grasslands. We then found the species composition and phylogenetic structure of plant community under the warming conditions at an elevation of about 4500 m were not significantly different from those under the control conditions at an elevation of about 4300 m in alpine grasslands in the tenth year. Moreover, the species composition at an elevation of about 4700 m under the warming conditions was also not significantly different from that at an elevation of about 4500 m under the control conditions in the tenth year. Therefore, asymmetrical warming between elevations may result in similar plant community composition between elevations in alpine grasslands on the Tibetan Plateau.
The datasets generated for this study are available on request to the corresponding author.
JW and GF performed the field sampling and wrote the first manuscript. CY revised the manuscript. All authors contributed to the article and approved the submitted version.
This research was supported by the Youth Innovation Promotion Association of Chinese Academy of Sciences (2020054), the National Key Research Projects of China (2017YFA0604801), the Bingwei Outstanding Young Talents Program of Institute of Geographic Sciences and Natural Resources Research, Chinese Academy of Sciences (2018RC202), the National Natural Science Foundation of China (31600432), and the Tibet Science and Technology Major Projects of the Pratacultural Industry (XZ202101ZD0003N).
The authors declare that the research was conducted in the absence of any commercial or financial relationships that could be construed as a potential conflict of interest.
The reviewer DW declared a shared affiliation, though no other collaboration, with one of the authors with the authors, JW, CY, GF, to the handling editor.
All claims expressed in this article are solely those of the authors and do not necessarily represent those of their affiliated organizations, or those of the publisher, the editors and the reviewers. Any product that may be evaluated in this article, or claim that may be made by its manufacturer, is not guaranteed or endorsed by the publisher.
We thank the editor and reviewers for their insightful and valuable comments, which greatly improved the quality of this manuscript.
The Supplementary Material for this article can be found online at: https://www.frontiersin.org/articles/10.3389/fevo.2021.757943/full#supplementary-material
Cheng, Y., Liu, H. Y., Wang, H. Y., Hao, Q., Han, Y., Duan, K. Q., et al. (2020). Climate-Driven Holocene Migration of Forest-Steppe Ecotone in the Tien Mountains. Forests 11:1139. doi: 10.3390/f11111139
Dolezal, J., Dvorsky, M., Kopecky, M., Liancourt, P., Hiiesalu, I., Macek, M., et al. (2016). Vegetation dynamics at the upper elevational limit of vascular plants in Himalaya. Sci. Rep. UK 6:sre24881.
Friend, A., and Woodward, F. (1990). Evolutionary and ecophysiological responses of mountain plants to the growing season environment. Adv. Ecol. Res. 20, 59–124. doi: 10.1016/s0065-2504(08)60053-7
Fu, G., Zhang, H. R., and Sun, W. (2019). Response of plant production to growing/non-growing season asymmetric warming in an alpine meadow of the Northern Tibetan Plateau. Sci. Total Environ. 650, 2666–2673. doi: 10.1016/j.scitotenv.2018.09.384
Golluscio, R. A., Sala, O. E., and Lauenroth, W. K. (1998). Differential use of large summer rainfall events by shrubs and grasses: a manipulative experiment in the Patagonian steppe. Oecologia 115, 17–25. doi: 10.1007/s004420050486
Hautier, Y., Seabloom, E. W., Borer, E. T., Adler, P. B., Harpole, W. S., Hillebrand, H., et al. (2014). Eutrophication weakens stabilizing effects of diversity in natural grasslands. Nature 508, 521–525. doi: 10.1038/nature13014
Hu, J., Hopping, K. A., Bump, J. K., Kang, S. C., and Klein, J. A. (2013). Climate change and water use partitioning by different plant functional groups in a grassland on the Tibetan Plateau. PLoS One 8:0075503. doi: 10.1371/journal.pone.0075503
Klein, J. A., Harte, J., and Zhao, X. Q. (2007). Experimental warming, not grazing, decreases rangeland quality on the Tibetan Plateau. Ecol. Appl. 17, 541–557. doi: 10.1890/05-0685
Kuang, X. X., and Jiao, J. J. (2016). Review on climate change on the Tibetan Plateau during the last half century. J. Geophys. Res. Atmosph. 121, 3979–4007. doi: 10.1002/2015jd024728
Lenoir, J., Gegout, J. C., Marquet, P. A., de Ruffray, P., and Brisse, H. (2008). A significant upward shift in plant species optimum elevation during the 20th century. Science 320, 1768–1771. doi: 10.1126/science.1156831
Li, W. H. (2017). An overview of ecological research conducted on the Qinghai-Tibetan Plateau. J. Resour. Ecol. 8, 1–4. doi: 10.5814/j.issn.1674-764x.2017.01.001
Ma, Z. Y., Liu, H. Y., Mi, Z. R., Zhang, Z. H., Wang, Y. H., Xu, W., et al. (2017). Climate warming reduces the temporal stability of plant community biomass production. Nat. Commu. 8:15378.
Nobis, M. P., and Schweingruber, F. H. (2013). Adult age of vascular plant species along an elevational land-use and climate gradient. Ecography 36, 1076–1085. doi: 10.1111/j.1600-0587.2013.00158.x
Pauli, H., Gottfried, M., and Grabherr, G. (1999). Vascular plant distribution patterns at the low-temperature limits of plant life - the alpine-nival ecotone of Mount Schrankogel (Tyrol, Austria). Phytocoenologia 29, 297–325. doi: 10.1127/phyto/29/1999/297
Pauli, H., Gottfried, M., Dullinger, S., Abdaladze, O., Akhalkatsi, M., Benito Alonso, J. L., et al. (2012). Recent Plant Diversity Changes on Europe’s Mountain Summits. Science 336, 353–355.
Sun, J., Hou, G., Liu, M., Fu, G., Zhan, T. Y., Zhou, H. K., et al. (2019). Effects of climatic and grazing changes on desertification of alpine grasslands, Northern Tibet. Ecol. Indicat. 107:105647. doi: 10.1016/j.ecolind.2019.105647
Sun, W., Li, S., Wang, J., and Fu, G. (2021). Effects of grazing on plant species and phylogenetic diversity in alpine grasslands, Northern Tibet. Ecol. Enginee. 170:106331. doi: 10.1016/j.ecoleng.2021.106331
Walther, G. R., Beissner, S., and Burga, C. A. (2005). Trends in the upward shift of alpine plants. J. Veg. Sci. 16, 541–548. doi: 10.1111/j.1654-1103.2005.tb02394.x
Wang, L., Wang, W. J., Wu, Z. F., Du, H. B., Zong, S. W., and Ma, S. (2019). Potential Distribution Shifts of Plant Species under Climate Change in Changbai Mountains, China. Forests 10:498. doi: 10.3390/f10060498
Wang, S. P., Duan, J. C., Xu, G. P., Wang, Y. F., Zhang, Z. H., Rui, Y. C., et al. (2012). Effects of warming and grazing on soil N availability, species composition, and ANPP in an alpine meadow. Ecology 93, 2365–2376. doi: 10.1890/11-1408.1
Wu, G. L., Ren, G. H., Wang, D., Shi, Z. H., and Warrington, D. (2013). Above- and below-ground response to soil water change in an alpine wetland ecosystem on the Qinghai-Tibetan Plateau, China. J. Hydrol. 476, 120–127. doi: 10.1016/j.jhydrol.2012.10.031
Wu, J. S., Shen, Z. X., Shi, P. L., Zhou, Y. T., and Zhang, X. Z. (2014). Effects of Grazing Exclusion on Plant Functional Group Diversity Alpine Grasslands along a Precipitation Gradient on the Northern Tibetan Plateau. Arct. Antarct. Alp. Res. 46, 419–429. doi: 10.1657/1938-4246-46.2.419
Xue, X., Xu, M. H., You, Q. G., and Peng, F. (2014). Influence of experimental warming on heat and water fluxes of alpine meadows in the Qinghai-Tibet Plateau. Arct. Antarct. Alp. Res. 46, 441–458. doi: 10.1657/1938-4246-46.2.441
Yang, X. M., Li, Z. X., Feng, Q., He, Y. Q., An, W. L., Zhang, W., et al. (2012). The decreasing wind speed in southwestern China during 1969-2009, and possible causes. Quat. Int. 263, 71–84. doi: 10.1016/j.quaint.2012.02.020
Yao, H. R., and Li, D. L. (2019). The Gale Concentration Period and Degree over the Tibetan Plateau and Related Atmospheric Circulation during the Windy Period. J. Desert Res. 39, 122–133.
Yao, T. D., Liu, X. D., Wang, N. L., and Shi, Y. F. (2000). Amplitude of climatic changes in Qinghai-Tibetan Plateau. Chin. Sci. Bull. 45, 1236–1243. doi: 10.1007/bf02886087
Keywords: biodiversity, β-diversity, climate warming, phylogenetic diversity, species composition, Tibetan Plateau (TP)
Citation: Wang J, Yu C and Fu G (2021) Asymmetrical Warming Between Elevations May Result in Similar Plant Community Composition Between Elevations in Alpine Grasslands. Front. Ecol. Evol. 9:757943. doi: 10.3389/fevo.2021.757943
Received: 13 August 2021; Accepted: 21 September 2021;
Published: 11 October 2021.
Edited by:
Jian Sun, Institute of Tibetan Plateau Research, Chinese Academy of Sciences (CAS), ChinaReviewed by:
Da Wei, Chinese Academy of Sciences (CAS), ChinaCopyright © 2021 Wang, Yu and Fu. This is an open-access article distributed under the terms of the Creative Commons Attribution License (CC BY). The use, distribution or reproduction in other forums is permitted, provided the original author(s) and the copyright owner(s) are credited and that the original publication in this journal is cited, in accordance with accepted academic practice. No use, distribution or reproduction is permitted which does not comply with these terms.
*Correspondence: Gang Fu, ZnVnYW5nQGlnc25yci5hYy5jbg==
Disclaimer: All claims expressed in this article are solely those of the authors and do not necessarily represent those of their affiliated organizations, or those of the publisher, the editors and the reviewers. Any product that may be evaluated in this article or claim that may be made by its manufacturer is not guaranteed or endorsed by the publisher.
Research integrity at Frontiers
Learn more about the work of our research integrity team to safeguard the quality of each article we publish.