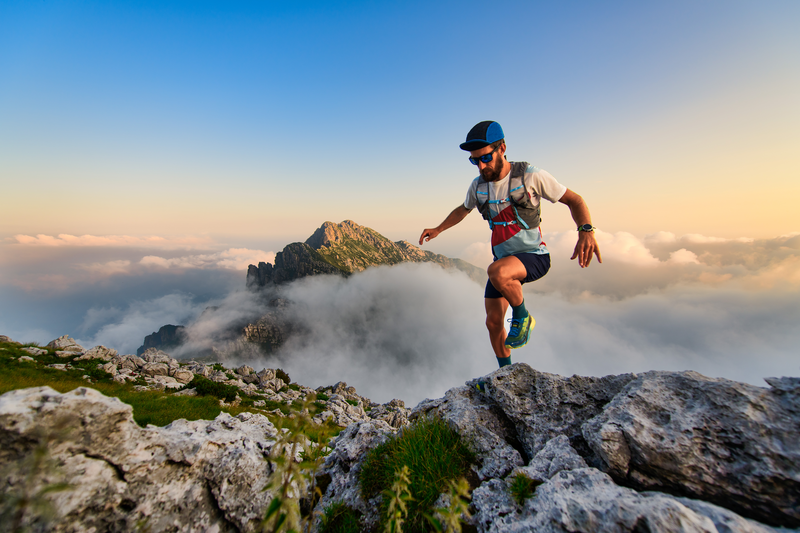
94% of researchers rate our articles as excellent or good
Learn more about the work of our research integrity team to safeguard the quality of each article we publish.
Find out more
ORIGINAL RESEARCH article
Front. Ecol. Evol. , 08 November 2021
Sec. Behavioral and Evolutionary Ecology
Volume 9 - 2021 | https://doi.org/10.3389/fevo.2021.751072
This article is part of the Research Topic Effects of Artificial Light at Night on Organisms: From Mechanisms to Function View all 13 articles
Artificial light at night (LAN) alters the physiology and behavior of an organism; however, very little is known about phase-dependent effects of LAN, particularly, in night migratory songbirds. Therefore, in this study, we investigated whether the effects of LAN on daily activity and photoperiodic responses in the Palearctic Indian migratory songbird, redheaded buntings (Emberiza bruniceps), is dependent on the different phases of the night. Male buntings maintained under short photoperiod (8L:16D; L = 100 lux, D < 0.1 lux) in individual activity cages were exposed to LAN (2 lux) for 6 weeks either in 4 h bin given at the different phases of 16 h night (early, mid, or late at ZT 08–12, ZT 14–18, or ZT 20–24, respectively; n = 9 each group) or throughout 16 h night (all night light, n = 6, ZT 08–24, the time of lights ON was considered as Zeitgeber time 0, ZT 0). A group (n = 6) with no LAN served as control. The results showed that LAN at the different phases of night induced differential effects as shown by an intense activity during the night, altered melatonin and temperature rhythms, and showed an increase in body mass and body fattening, food intake, and gonadal size. Midnight light exposure has a greater impact on migration and reproduction linked phenotypes, which is similar to the ones that received light throughout the night. The highlights of this study are that (i) LAN impacts day-night activity behavior, (ii) its continuity with the day alters the perception of day length, (iii) birds showed differential sensitivity to LAN in a phase-dependent manner, (iv) the direction of placing LAN affects the daily responses, e.g., LAN in the early night was “accepted” as extended dusk but the late night was considered as early dawn, and (v) midnight LAN was most effective and induced similar responses as continuous LAN. Overall, LAN induces long day responses in short days and shows differential sensitivity of the different phases of the night toward the light. This information may be valuable in adopting a part-night lighting approach to help reduce the physiological burden, such as early migration and reproduction, of artificial lighting on the nocturnal migrants.
In nature, the day-night cycles are provided by bright sunlight and dim moon and starlight, respectively. These cycles are very consistent with no interannual variations and act as the most reliable cue to regulate daily and seasonal responses in the animals. However, the advent of artificial light and its use at night owing to various anthropogenic activities, such as industries, call centers, and the operation of a long-distance flight, have disrupted the consistent pattern of light:dark cycle. The artificial light at night (LAN) is of relatively high intensity than natural light, therefore, may alter the perception of day length (Kumar et al., 2018). Also, it is rich in the blue portion of the light spectrum, which could be perceived as the day (Navara and Nelson, 2007; Gaston et al., 2013; Yadav et al., 2015). Because the light synchronizes avian circadian and seasonal functions (Berson et al., 2002; Foster and Kreitzmann, 2004), changes in the light environment at night are likely to affect the expression of several biological functions. The artificial LAN is linked with changes in the locomotor activity and feeding behavior in both vertebrates (Fonken et al., 2010; Santos et al., 2010; Polak et al., 2011; Titulaer et al., 2012; Dominoni et al., 2014) and invertebrates (Moore et al., 2000; Eisenbeis and Hanel, 2009). The ecological consequences of increasing LAN are well-documented in different birds and mammals (Rich and Longcore, 2006; Navara and Nelson, 2007; Dominoni et al., 2014).
The effect of LAN seems to be more prominent in migratory birds because most of them are nocturnal migrants. The bird migration represents enduring flights in time and space that may range from non-stop trans-hemispheric flights to days- or months-long journeys (Conklin et al., 2017). Any disruption in this flight may induce harmful effects at individual and population levels (Alerstam and Lindström, 1990; Liechti, 2006; Hewson et al., 2016; Kelly et al., 2016; Cohen et al., 2017). Several studies have shown the role of different environmental factors in regulating nocturnal flight (Alerstam and Lindström, 1990; Richardson, 1990; Marra et al., 2005; Liechti, 2006; Kelly et al., 2016), including its orientation and navigation at night (Hiscock et al., 2016; Mouritsen et al., 2016). Because visual cues are important for the night flight, it is possible that brighter night affects abilities of birds to orient and navigate (Cochran and Graber, 1958; Day et al., 2015; Vincze et al., 2015) during a migration. It may also interfere with the geomagnetic compass, which tells the birds about the spatial maps (Kishkinev et al., 2015; Hiscock et al., 2016; Mouritsen et al., 2016).
Recent studies have shown the physiological mechanisms and underlying effects of LAN on daily and seasonal responses in birds. It is being observed that LAN disrupts their circadian rhythms and changes their flight behavior, which may lead to disorientation. It may alter their reproductive physiology (Larkin and Frase, 1988; Wiltschko et al., 1993; Bruderer et al., 1999; Dominoni et al., 2013c,d; Dominoni and Partecke, 2015; Raap et al., 2015; de Jong et al., 2016). The sensitivity of the circadian system to LAN may influence the overall physiology and behavior, making it vulnerable to the circadian disruption of the melatonin profile, metabolic functions, and other hormonally driven systems. Melatonin is produced in the dark, it tracks the night length and “informs” the animal about the time of the day and/or year. It helps in the synchronization of the circadian clock to the light:dark (L:D) cycle and is shown to be required for the persistence of circadian rhythms in an aperiodic environment (Gwinner et al., 1997; Gwinner and Brandstaetter, 2001; Rani et al., 2005a). Besides being a “read out” of physiological day and night, melatonin exhibits a phase relationship with the daily rhythms in cortisol, body temperature, and sleep (Gwinner et al., 1997; Arendt and Skene, 2005). Thus, any alteration in the external environment may alter this relationship (Yadav et al., 2015). Thus, a 24-h melatonin rhythm acts as a strong marker to assess the impact of LAN on daily rhythms.
Although several studies have shown the effect of LAN on daily and seasonal behaviors, there are still gaps, e.g., most of the studies have been done on resident bird species and almost none has demonstrated the differential sensitivity of the different phases of the night toward LAN. In this light, we chose a migratory species (redheaded bunting; Emberiza bruniceps) to conduct this study. Buntings proved to be a good model system for such studies due to the following reasons (i) they are long-distance latitudinal migrants representing the Palearctic Indian migratory system, (ii) show distinct life history stages (photosensitive overwintering, spring migration, photostimulated breeding, and autumn migration) in their annual cycle, (iii) in a laboratory condition under captivity, on exposure to increasing day lengths they exhibit nighttime migratory restlessness (Zugunruhe), and (iv) they are long-day breeders, and an increasing photoperiod of ≥12 h per day stimulates migratory and reproductive phenotypes present in them. Thus, an obvious question would be to study the effect of LAN using a non-stimulatory photoperiod (e.g., 8L:16D). The expectation is that if the birds are sensing the LAN, they would respond to it, as if, they have been exposed to long stimulatory day lengths. In view of this, we aimed to investigate the effect of LAN on circadian (daily activity rest pattern, body temperature, and melatonin rhythm) and seasonal (changes in migration and reproduction-linked phenologies) responses in migratory buntings and also to study the differential sensitivity of the different phases of the night toward LAN.
This study was done on Palearctic Indian migratory songbirds, the redheaded bunting (E. bruniceps). They migrate two times a year between their breeding (∼40°N; in South-Eastern Europe, Asia Minor, Palestine, Syria, Upper Mesopotamia, and Persia) and wintering grounds (∼25°N; spreading throughout western and central India, chiefly in Rajasthan, Madhya Pradesh, Gujarat, Maharashtra, and Karnataka) representing autumn and spring migration, respectively. They come to their wintering grounds in the autumn (late July and early August) and return to their breeding grounds in late March and early April (prevailing day length ≥ 12 h light per day). It is estimated that these species spend a total of about 96 days (48 days each way), covering a migratory journey of about 7,000 km in both the ways and of ∼90 days at breeding grounds; the remaining almost half of the year they spend at their wintering grounds (Ali and Ripley, 1974). Buntings are long-day breeders, and in captivity, the day length of ≥12 h light per day induces hyperphagia, body mass gain, fat deposition, gonadal recrudescence, and the nighttime migratory restlessness, Zugunruhe (Jain and Kumar, 1995).
Adult male buntings were captured in mid-February 2015 from the overwintering flocks near Lucknow (25°N) using the mist net. They were brought to the laboratory and acclimatized in an outdoor aviary (size = 3 m × 2.5 m × 2.5 m) for a week under a natural day length condition. Thereafter, they were transferred to an indoor aviary under short day length (8L:16D; L = ∼100 lux and D ≤ 0.1 lux, closer to dark) and constant temperature (22°C ± 2°C) conditions. Food (seeds of Setaria italica) and water were available ad libitum and replenished during the light phase.
The buntings (n = 39) maintained in an indoor aviary were transferred to individual activity cages (60 cm × 35 cm × 45 cm) placed singly in a light-tight photoperiodic chamber (75 cm × 50 cm × 70 cm). After more than a week under 8L:16D, the birds were given LAN (2 lux) for 6 weeks either in 4 h bin given at the different phases of 16 h night (early, mid, or late at ZT 08–12, ZT 14–18, or ZT 20–24, respectively; n = 9 each group) or throughout night (all night, n = 6, ZT 08–24, the time of lights ON was considered as Zeitgeber time 0, ZT 0). A group (n = 6) with no LAN served as control. The light:dark cycles were given by compact fluorescent lamps (CFL; 14 W, 230 V, Phillips, India). Desired light intensity was obtained by covering the CFL with a black paper sheet having small holes on the side facing the roof so that a bird received a diffused light from the light source.
We measured migratory and reproductive phenotypes, such as locomotor activity and change in food intake, body mass, body fattening, and gonadal size, along with the body temperature and melatonin levels.
To collect the general activity of the birds, each cage was equipped with an IR motion sensor (Conrad Electronic, Hirschau, Germany, Haustier PIR-Melder). The activity was collected and analyzed by Chronobiology kit; Stanford Software Systems, Stanford, CA, United States (Malik et al., 2004). The activity records (actograms) were double plotted, and the hourly activity counts for 24 h were calculated for the selected duration of an experiment. The activities in the second week and last week of LAN exposure were considered as beginning and the end, respectively. The daily activity profile was plotted as mean (±SEM).
To measure the plasma melatonin levels, blood samples from the birds of different groups (n = 5 each) were taken four times of the day (ZT 04, mid-day; ZT 10, early night; ZT 16, midnight; and ZT 22, late light) covering the midpoints of day and night, and of all the LAN phases, to make the data comparable. Blood was taken by puncturing the brachial wing vein. To avoid any stress to the birds, sampling was scattered over a period of 13 days (days 25–37 of night light treatment). All birds were bled for all the time points. Nighttime samples were collected in a dim green light (500 nm) from a KL1500 cold light source focused on the wing vein for not more than half-a-minute. This had shown no effect on the nocturnal melatonin secretion (Cassone et al., 2008). Each time about 200 μl of blood was collected by heparinized capillaries in the vials and immediately centrifuged at 3,000 rpm for 10 min to collect the plasma, which was stored at −20°C until assayed for melatonin.
Plasma melatonin assay was done by ELISA using a specific melatonin kit (product no. RE54021, IBL International GmbH, Hamburg, Germany). This assay was previously standardized and used for the measurement of plasma melatonin in several studies (Lahiri et al., 2004; Terzieva et al., 2009), including the studies from our laboratory (Singh et al., 2012; Yadav et al., 2015; Kumar et al., 2018). Melatonin was extracted and assayed from each plasma sample as per the protocol and instructions from the manufacturer. Briefly, 100 μl of experimental samples, standards, and controls were methanol extracted and dried by a vacuum concentrator. The obtained pellet was reconstituted in 150 μl of double-distilled water. Then, 50 μl of each reconstituted sample volume and 50 μl melatonin antiserum (rabbit, polyclonal) were incubated in a 96-well plate at 4°C for 20 h. Thereafter, 150 μl of a freshly prepared enzyme conjugate was added and incubated for 2 h at room temperature with continuous shaking (500 rpm). Then, 200 μl of a freshly prepared p-nitrophenyl phosphate (PNPP) substrate solution was added to each well and incubated for another 40 min. The reaction was stopped by adding 50 μl of PNPP stop solution to each well, and optical density (OD) was measured at 405 nm, using 650 nm wavelength as a reference. The ODs of standard samples were plotted to make a standard curve, and the concentration of melatonin in experimental samples was calculated with a reference to values in the standard curve. Individual values of each bird were used to calculate mean ± SEM melatonin levels (pg/ml) for a group. The analytical sensitivity (limit of detection) of the assay was 1.6 pg/ml, and the % CV for intra- and inter-assay was 7.69, 7.33, 6.59, and 9.67, respectively.
The body temperature in each group was measured by a quick shot IR ThermoScan, India (Model: EXP-01B) from the flank area of the birds, just before the blood sampling at ZT 04, 10, 16, and 22.
Body mass was recorded using a top pan balance up to an accuracy of 0.1 g. The food intake (g)/bird/day was measured as described by Kumar et al. (2001). It was calculated as the difference between the food supplied and food recovered after 24 h. Body fattening (fat deposition in furcular, scapular, and abdominal areas) was assessed as the fat score on a scale of 0–5, where 0 represents no fat and 5 represents the maximum deposition of fat (Kumar et al., 2001). The testis size was measured by laparotomy performed under general anesthesia (Kumar et al., 2002) using the formula 4/3 π ab2, where a and b denote one-half of long (length) and short (width) axes, respectively (Kumar et al., 2002). The physiological parameters were measured before the LAN treatment (baseline data) and in the sixth week of LAN treatment, and a change was plotted as mean (±SEM).
Data on activity profile, plasma melatonin, body temperature, and physiological parameters (food intake, body mass, body fattening, and testis volume) were plotted as mean ± SEM while the actograms given are of a representative bird in each treatment. We used a one-way ANOVA with or without a repeated measure (RM) (one-way RM ANOVA and one-way ANOVA, respectively) followed by the Newman-Keuls multiple comparison post hoc test to determine the effect of LAN on plasma melatonin levels and body temperature within the group and between the groups. We also used a one-way ANOVA without an RM (one-way ANOVA) followed by the Newman-Keuls multiple comparison post hoc test to determine the effect of LAN on physiological parameters. A significance was considered at p < 0.05. All data analyses were done using GraphPad Prism software version 5.0 (San Diego, CA, United States).
The results showed that LAN had an impact on both circadian and seasonal responses in a phase-dependent manner (Figures 1–3).
Figure 1. Double plotted representative actograms showing the impact of light at night (LAN) in migratory redheaded bunting (Emberiza bruniceps). Birds, which are maintained under non-stimulatory short days (8L:16D; L = 100 lux, D < 0.1 lux), were given LAN (∼2 lux) either in 4 h bin placed at different phases of 16 h night (early, mid, or late at ZT08–12, ZT14–18, or ZT20–24 respectively; n = 9 each group) or throughout the night (all night, n = 6, ZT 08–24). A group (n = 6) with no LAN served as control. Note that early night and late night LAN evoke a response similar to dusk and dawn, respectively. Mid night LAN shows the fragmentation of activity or rest during the night, whichever applicable. In general, all the groups except the control show long day effects. The lower panel (A–E) shows a 24-h activity profile in the beginning (α) and end (β) of LAN treatment. Shaded and dark areas in the figure represent the LAN and dark phases of the night, respectively.
Figure 2. An effect of LAN (∼2 lux) on plasma melatonin (pg/ml) and body temperature (°C). Birds exposed to different light at night (LAN) treatments (A–E) were sampled for plasma melatonin levels and scanned for body temperature during mid-day and middle of each LAN treatment (ZT 04, 10, 16, and 22). English or Greek alphabets on the point symbol (Mean ± SEM, n = 5) indicate a statistical analysis for melatonin and temperature, respectively. Similar alphabets indicate no difference, whereas different alphabets indicate a significant difference (p < 0.05; the Newman–Keuls multiple comparison post hoc test) between ZTs. It is noted that melatonin and temperature rhythms are antiphasic and LAN alters their shape of the curve.
Figure 3. An effect of light at night (LAN) (2 lux) exposure on seasonal responses in migratory redheaded bunting (Emberiza bruniceps). Changes (mean ± SEM) in (A) food intake, (B) body mass, (C) body fattening, and (D) testis volume under different LAN treatments. Similar alphabets on a bar indicate no difference, whereas different alphabets indicate a significant difference (p < 0.05; the Newman–Keuls multiple comparison post hoc test) between groups. It is noted that midnight LAN behaves similar to all night LAN but with greater intensity.
Buntings are diurnal birds, therefore, their activity is restricted only within a day time of 8L:16D; however, during LAN treatment the daytime activity either extended into the dark (early night group) or start early (late night group). The buntings in the control group (8L:16D) showed no nighttime activity, but in the all night group (ZT 08–24), they developed a prominent nighttime activity. The groups that received LAN for 4 h at the different phases of night evoked different responses. In the “early night” group, the light given at ZT 08–12, delayed the end but in the “late night” group light at ZT 20–24 advanced the onset of the day. Contrarily, birds in the “midnight” group (ZT 14–18) showed a different pattern of activity in the night. In these birds, initially, the activity was restricted to 8 h day and 4 h segment of LAN but one time the intense nighttime activity; Zugunruhe, developed, the activity during LAN was compromised. This period of less activity was flanked by an intense nighttime activity (Figure 1C). Besides, the birds in the “late night” group (ZT 20–24) showed a mixed response. All the birds (100%) of this group were active during 8 h day, but 55.56% of the birds were active in a 4-h LAN segment too. Birds under the different phases of LAN developed an intense nighttime activity. In early and late night groups, 44.44% of birds and in midnight and all night groups, 100% of birds developed an intense nighttime activity, respectively.
Figure 2 shows the plasma melatonin levels and body temperature in different groups experiencing LAN at the different phases of the night. LAN provided at the different phases of night altered their melatonin and temperature profiles. In general, the melatonin levels showed a rhythmic pattern in all the groups (control group: F3, 12 = 8.827, p = 0.0023; early night group: F3, 12 = 10.51, p = 0.0011; midnight group: F3, 12 = 4.394, p = 0.0264; late night group: F3, 12 = 10.10, p = 0.0013; all night group: F3, 12 = 5.009, p = 0.0177; one-way RM ANOVA; Figures 2A–E). Levels were minimum during the midday (ZT 04) and significantly elevated during the night except in the period of 4 h LAN when the melatonin was significantly lower and similar to day time levels (Figures 2A–D). Contrarily, melatonin levels in the all night group (Figure 2E) did not show a high amplitude rhythm. Levels were low throughout the night except at the end (ZT 22) when it became significantly high (all night group: F3, 12 = 5.009, p = 0.0177; one-way RM ANOVA; Figure 2E).
Body temperature also followed a rhythmic pattern as that of melatonin although in antiphase (control group: F3, 12 = 3.526, p = 0.0487; early night group: F3, 12 = 5.013, p = 0.0176; midnight group: F3, 12 = 19.60, p < 0.0001; late night group: F3, 12 = 7.254, p = 0.0049; all night group: F3, 12 = 0.3900, p = 0.7624; one-way RM ANOVA; Figures 2A–E). In general, the temperature was high during day (ZT 04) and at the 4-h LAN phase. Birds exposed to the all night group showed no change in their body temperature throughout 24 h (Figure 2E).
Light at night-induced photoperiodic physiological responses, such as food intake, body mass, body fattening, and testis recrudescence, are shown in Figure 3. All groups (except control) showed the effects of LAN, the responses were dependent on the different phases of LAN exposure. The change in food intake is significantly high in buntings of all the four LAN-treated groups in comparison to the birds of the control group (change in food intake: F4, 34 = 9.654, p < 0.0001; one-way ANOVA; Figure 3A). A change in body mass is high in the birds of LAN-exposed groups but midnight and all night groups have a significant difference with control and early night groups (change in body mass: F4, 34 = 6.683, p = 0.0004; one-way ANOVA; Figure 3B). A change in the fat score is significantly high in midnight, late night, and all night groups in comparison to the birds of the control group (a change in the fat score: F4, 34 = 5.871, p = 0.0011; one-way ANOVA; Figure 3C). Testis recrudescence takes place in all the four LAN-treated groups, but the change in testis volume is significantly higher in the birds of midnight group with respect to the rest of the groups (a change in the testicular volume: F4, 34 = 13.18, p < 0.0001; one-way ANOVA; Figure 3D).
The results show that exposure to LAN even for a small duration of 4 h at an intensity of ∼2 lux induces photoperiodic responses in bunting maintained under short days, which is otherwise a non-stimulatory photoperiod for them. Irrespective of time, early night, midnight, or late night, the 4 h LAN could induce migratory restlessness in all the groups along with hyperphagia, an increase in body fattening, and the testis size. It is established if they are held captive in a caged condition and not allowed to fly (migrate) during their migratory season, they show a strong motivation to migrate, generally known as migratory restlessness, Zugunruhe (Gwinner, 1990).
The results thus suggest that the short-duration LAN was able to change the perception of day length in bunting. The change in the perception could be due to a subjective interpretation of different light intensities (Kumar et al., 1992, 2007; Foster and Kreitzmann, 2004; Longcore and Rich, 2004; Dominoni et al., 2013a) or a changed melatonin profile (Cassone et al., 2008). In black-headed bunting exposed to a combination of the bright and dim day:night (LD) cycle of 10 and 2 lux light intensities, respectively, the dim light period was perceived as night (Kumar et al., 1992). Previous studies on stonechats and baya weaver when exposed to the dim:bright LD also showed a change in the perception of day length and accordingly changed their melatonin profile (Kumar et al., 2007; Singh et al., 2012).
In this study, the birds were experiencing the three grades of light intensities, such as bright (100 lux) in 8L, dim (2 lux) in 4 h LAN, and close to dark (0.1 lux) at the night, but the transition of light intensities from the day to night was different in different groups. For example, in the early night group, the transition was from bright to dim to dark, in the midnight group, from bright to dark interrupted by dim, in the late night group, bright to dark to dim, and in all night group, from bright to dim. These transitions could have re-entrained the endogenous rhythm of photoperiodic photosensitivity as it is evident from their differential responses. A maximum response in the midnight group suggests that birds interpreted it as a lighting regimen of 18L:6D (8L + 6D + 4 h LAN), which is strongly stimulatory (Singh et al., 2002). There were a moderate response in the early night and late night groups, which suggests that birds interpreted it as a lighting regimen of 12L:12D (8L + 4 h LAN or 4 h LAN + 8L), which is a marginally inductive photoperiod for bunting. In a previous study on bunting (Singh et al., 2002), similar observations were made. The bunting exposed to 1L:8D:1L:14D and 1L:8D:10L:5D seemed to be re-entrained, and they interpreted the abovementioned lighting regimen as 10L:14D and 19L:5D (Singh et al., 2002).
There are a few studies on redheaded bunting (E. bruniceps), which are suggestive of the photoperiod-dependent effects on seasonal responses such as body fattening and testicular growth (Rani et al., 2005b). When exposed to increasing photoperiods such as 11.5L:12.5D, 12L:12D, 12.5L:11.5D, 13L:11D, 14L:10D, and 18L:6D, the buntings responded in a photoperiod-dependent manner and underwent growth and regression cycle under photoperiods ≥ 12 h per day (Rani et al., 2005b). A previous study on Japanese quail has also established a relationship between the length of the photoperiod and the rate of the photoperiodic induction. The rate of testicular growth was slower under 12L:12D as compared to 13L:11D, 14L:10D, 16L:8D, and 20L:4D photoperiods, which showed a maximum growth (Follett and Maung, 1978). The light intensity is suggestive of affecting the photoperiodic response as observed in the case of European starlings (Sturnus vulgaris). The starlings exposed to an 18L:6D photoperiod at 3-, 13-, 45-, and 108-lux light intensities responded differently, and it appeared as if they were exposed to different photoperiods such as 11L:13D, 13L:11D, 16L:8D, and 18L:6D, respectively (Bentley et al., 1998). Similarly, the buntings kept under a stimulatory photoperiod of 13L:11D and at the intensities of 50, 100, 400, 800, and 1,000 lux also showed an intensity-dependent photoperiodic induction (Misra et al., 2004).
In our study, the onset and end of a daytime activity were affected by the phase of LAN. The birds, which received 4 h LAN in the early night, extended their daytime activity into the night, and the ones, which received at late night, showed an early onset of morning activity, which extended their nighttime activity into the day. In midnight and all night groups, the onset of morning activity was not affected by LAN. Our results are slightly different from those on male great tits, which on exposure to 1.6 lux light intensity throughout the night advanced the onset of their morning activity (Raap et al., 2015). Also, in our study, the LAN accelerated a gonadal response in midnight and all night groups, similar to that observed in blackbirds where the LAN advanced the gonadal growth almost a month earlier than birds kept under dark nights (Dominoni et al., 2013c).
Light at night also seems to have a strong effect on song timing in some early singing species such as European robin (Erithacus rubecola), American robin (Turdus migratorius), Common blackbird (Turdus merula), and Great tit (Parus major) (Miller, 2006; Fuller et al., 2007; Kempenaers et al., 2010; Da Silva et al., 2014; Dominoni et al., 2014). The effect ranges from the previous onset of dawn song to singing in the middle of the night (Fuller et al., 2007; Da Silva et al., 2014; Dominoni et al., 2014). Although we have not measured the song behavior in our birds, we have shown a 24 h profile of their locomotor activity.
In our study, we also observed that LAN affected the rhythm of melatonin secretion. In general, the melatonin levels were low during the day and high at night, however, in the groups exposed to 4 h LAN, the level of melatonin was significantly low at the time of LAN, which seemed to be “perceived” as day. This altered the shape of the melatonin profile in different groups. The amplitude of melatonin was the highest in the early night and late night groups but was the lowest in midnight and all night groups (having a maximum gonadal response) that received light throughout the night. Thus, these results may be helpful in understanding the control of seasonal processes in birds experiencing high light intensity at night such as the urban birds. Although a direct role of melatonin in the regulation of seasonal responses in birds is debatable (Dawson et al., 2001), there is a possibility that reduced melatonin levels during the night must have advanced the onset of reproductive functions by changing the perception of day length longer than the actual one. Our speculation is based on a study on European starlings, which showed that the exogenous administration of melatonin reduces the volume of song control nuclei, HVC, and area X (Bentley et al., 1999). High melatonin levels upregulate GnIH in the brain and the gonads, which suppresses the hypothalamo–hypophyseal–gonadal axis and maintains the small gonadal size during the prebreeding stage (Ubuka et al., 2005; McGuire et al., 2011). LAN may also affect the physiology of birds by reducing the ratio between the day and night light intensity, which would not only lower the difference between melatonin levels during the day and nighttime but also advance the onset of Zugunruhe (Roenneberg et al., 2003; Singh et al., 2012). Lower melatonin levels during the night as a consequence of LAN may reduce the degree of self-sustainment of the circadian clock and allows an alternative temporal activity such as Zugunruhe in migratory birds (Fusani and Gwinner, 2005) or the appearance of activity during the night in a resident Indian weaver bird (Singh et al., 2012). Such a prediction is supported by a recent study on urban and rural blackbirds, which showed that possibly due to the night light, urban birds have faster (advanced) but weaker circadian clocks than their rural counterparts (Dominoni et al., 2013b).
To sum up, our results show that even under non-stimulatory short days, the low intensity (∼2 lux) night light could induce migratory and reproductive phenotypes. Also, the effect of LAN was phase dependent. These findings may have ecological implications the birds under short days are still in their recovery phase and, when exposed to LAN, they displayed an activity for ∼12 h in all the light treatments. Thus, 4-h LAN of ∼2 lux light intensity could alter the perception of day length, thereby advancing their migratory and reproductive activities. Our results also suggest that the LAN interruptions of 4 h during midnight have a greater impact on circadian and seasonal responses as compared to other groups where the LAN was given in continuation with the day. Hence, this information may be valuable in adopting a part-night lighting approach to help reduce the physiological burden, such as early migration and reproduction, of artificial lighting on the nocturnal migrants.
The original contributions presented in the study are included in the article/supplementary material, further inquiries can be directed to the corresponding author.
The animal study was reviewed and approved by the Institutional Animal Ethics Committee, University of Lucknow, Lucknow, India (LU/ZOOL/SR/SM/Ph.D.JK/11-2014).
SR conceptualized and designed this study. JK and SM carried out the experiments. JK, SM, SB, and SR participated in the data analysis. JK, SM, and SR were involved in writing the manuscript. All authors gave final approval for publication.
Financial support from the Department of Biotechnology, New Delhi, India (BT/PR4984/MED/30/752/2012) and also from the PROTSAHAN scheme of the University of Lucknow, is gratefully acknowledged. JK is a recipient of the UGC Rajiv Gandhi National Fellowship (201112-RGNF-SC-UTT-11960).
The authors declare that the research was conducted in the absence of any commercial or financial relationships that could be construed as a potential conflict of interest.
All claims expressed in this article are solely those of the authors and do not necessarily represent those of their affiliated organizations, or those of the publisher, the editors and the reviewers. Any product that may be evaluated in this article, or claim that may be made by its manufacturer, is not guaranteed or endorsed by the publisher.
Alerstam, T., and Lindström, A. (1990). “Optimal bird migration: the relative importance of time, energy, and safety,” in Bird Migration: Physiology and Ecophysiology, ed. E. Gwinner (Berlin: Springer), 331–351. doi: 10.1007/978-3-642-74542-3_22
Ali, S., and Ripley, S. D. (1974). Handbook of the Birds of India and Pakistan, Vol. 10. New York, NY: Oxford University Press.
Arendt, J., and Skene, D. J. (2005). Melatonin as a chronobiotic. Sleep Med. Rev. 9, 25–39. doi: 10.1016/j.smrv.2004.05.002
Bentley, G. E., Goldsmith, A. R., Dawson, A., Briggs, C., and Pemberton, M. (1998). Decreased light intensity alters the perception of day length by male European starlings (Sturnus vulgaris). J. Biol. Rhythms 13, 148–158. doi: 10.1177/074873098128999998
Bentley, G. E., Van’t Hof, T. J., and Ball, G. F. (1999). Seasonal neuroplasticity in the songbird telencephalon: a role for melatonin. Proc. Natl. Acad. Sci. U.S.A. 96, 4674–4679. doi: 10.1073/pnas.96.8.4674
Berson, D. M., Dunn, F. A., and Takao, M. (2002). Phototransduction by retinal ganglion cells that set the circadian clock. Science 295, 1070–1073. doi: 10.1126/science.1067262
Bruderer, B., Peter, D., and Steuri, T. (1999). Behaviour of migrating birds exposed to X-band radar and a bright light beam. J. Exp. Biol. 202(Pt 9), 1015–1022. doi: 10.1242/jeb.202.9.1015
Cassone, V. M., Bartell, P. A., Earnest, B. J., and Kumar, V. (2008). Duration of melatonin regulates seasonal changes in song control nuclei of the house sparrow (Passer domesticus): independence from gonads and circadian entrainment. J. Biol. Rhythms 23, 49–58. doi: 10.1177/0748730407311110
Cochran, W. W., and Graber, R. R. (1958). Attraction of nocturnal migrants by lights on a television tower. Wilson. Bull. 70, 378–380.
Cohen, E. B., Barrow, W. C., Buler, J., and Deppe, J. (2017). How do en route events around the Gulf of Mexico influence migratory landbird populations? Condor 119, 327–343. doi: 10.1650/condor-17-20.1
Conklin, J. R., Senner, N. R., Battley, P. F., and Piersma, T. (2017). Extreme migration and the individual quality spectrum. J. Avian. Biol. 48, 19–36. doi: 10.1111/jav.01316
Da Silva, A., Samplonius, J. M., Schlicht, E., Valcu, M., and Kempenaers, B. (2014). Artificial night lighting rather than traffic noise affects the daily timing of dawn and dusk singing in common European songbirds. Behav. Ecol. 25, 1037–1047. doi: 10.1093/beheco/aru103
Dawson, A., King, V. M., Bentley, G. E., and Ball, G. F. (2001). Photoperiodic control of seasonality in birds. J. Biol. Rhythms 16, 365–380.
Day, R. H., Rose, J. R., Prichard, A. K., and Streever, B. (2015). Effects of gas flaring on the behavior of night-migrating birds at an artificial oil-production Island, Arctic Alaska. Arctic 68, 367–379.
de Jong, M., Ouyang, J. Q., van Grunsven, R. H. A., Visser, M. E., and Spoelstra, K. (2016). Do wild great tits avoid exposure to light at night? PLoS One 11:e0157357. doi: 10.1371/journal.pone.0157357
Dominoni, D. M., Carmona-Wagner, E. O., Hofmann, M., Kranstauber, B., and Partecke, J. (2014). Individual-based measurements of light intensity provide new insights into the effects of artificial light at night on daily rhythms of urban-dwelling songbirds. J. Anim. Ecol. 83, 681–692. doi: 10.1111/1365-2656.12150
Dominoni, D. M., and Partecke, J. (2015). Does light pollution alter day length? A test using light loggers on free-ranging European blackbirds (Turdus merula). Philos. T. Roy. Soc. B. 370:20140118. doi: 10.1098/rstb.2014.0118
Dominoni, D. M., Quetting, M., and Partecke, J. (2013c). Artificial light at night advances avian reproductive physiology. Proc. R. Soc. Lond. B 280:20123017. doi: 10.1098/rspb.2012.3017
Dominoni, D. M., Quetting, M., and Partecke, J. (2013d). Long term effects of chronic light pollution on seasonal functions of European blackbirds (Turdus merula). PLoS One 8:e85069. doi: 10.1371/journal.pone.0085069
Dominoni, D. M., Goymann, W., Helm, B., and Partecke, J. (2013a). Urban-like night illumination reduces melatonin release in European blackbirds (Turdus merula): implications of city life for biological time-keeping of songbirds. Front. Zool. 10:60. doi: 10.1186/1742-9994-10-60
Dominoni, D. M., Helm, B., Lehmann, M., Dowse, H. B., and Partecke, J. (2013b). Clocks for the city: circadian differences between forest and city songbirds. Proc. R. Soc. B 280:20130593. doi: 10.1098/rspb.2013.0593
Eisenbeis, G., and Hanel, A. (2009). “Light pollution and the impact of artificial night lighting on insects,” in Ecology of Cities and Towns, eds M. J. McDonnell, A. K. Hahs, and J. H. Breuste (Cambridge: Cambridge University Press), 243–263. doi: 10.1017/cbo9780511609763.016
Follett, B. K., and Maung, S. L. (1978). Rate of testicular maturation, in relation to gonadotrophin and testosterone levels, in quail exposed to various artificial photoperiods and to natural daylengths. J. Endocrinol. 78, 267–280. doi: 10.1677/joe.0.0780267
Fonken, L. K., Workman, J. L., Walton, J. C., Weil, Z. M., Morris, J. S., Haim, A., et al. (2010). Light at night increases body mass by shifting the time of food intake. Proc. Natl. Acad. Sci. U.S.A. 107, 18664–18669. doi: 10.1073/pnas.1008734107
Foster, R. G., and Kreitzmann, L. (2004). Rhythms of Life: The Biological Clocks that Control the Daily Lives of Every Living Thing. New Haven, CT: Yale University Press.
Fuller, R. A., Warren, P. H., and Gaston, K. J. (2007). Daytime noise predicts nocturnal singing in urban robins. Biol. Lett. 3, 368–370. doi: 10.1098/rsbl.2007.0134
Fusani, L., and Gwinner, E. (2005). Melatonin and nocturnal migration. Ann. N. Y. Acad. Sci. 1046, 264–270. doi: 10.1196/annals.1343.024
Gaston, K. J., Bennie, J., Davies, T. W., and Hopkins, J. (2013). The ecological impacts of nighttime light pollution: a mechanistic appraisal. Biol. Rev. Camb. Philos. Soc. 88, 912–927. doi: 10.1111/brv.12036
Gwinner, E. (1990). Bird Migration: Physiology and Ecophysiology. Heidelberg: Springer-Verlag. doi: 10.1007/978-3-642-74542-3
Gwinner, E., and Brandstaetter, R. (2001). Complex bird clocks. Philos. Trans. R. Soc. Lond. B Biol. Sci 356, 1801–1810. doi: 10.1098/rstb.2001.0959
Gwinner, E., Hau, M., and Heigl, S. (1997). Melatonin: generation and modulation of avian circadian systems. Brain Res. Bull. 44, 439–444. doi: 10.1016/S0361-9230(97)00224-4
Hewson, C. M., Thorup, K., Pearce-Higgins, J. W., and Atkinson, P. W. (2016). Population decline is linked to migration route in the common cuckoo. Nat. Commun. 7:12296. doi: 10.1038/ncomms12296
Hiscock, H. G., Worster, S., Kattnig, D. R., Steers, C., Jin, Y., Manolopoulos, D. E., et al. (2016). The quantum needle of the avian magnetic compass. Proc. Natl. Acad. Sci. U.S.A. 113, 4634–4639. doi: 10.1073/pnas.1600341113
Jain, N., and Kumar, V. (1995). Changes in food intake, body weight, gonads and plasma concentrations of thyroxine, luteinizing hormone and testosterone in captive male buntings exposed to natural daylengths at 29o N. J. Biosci. 20, 417–426. doi: 10.1007/bf02703845
Kelly, J. F., Horton, K., Stepanian, P., De Beurs, K., Fagin, T., Bridge, E., et al. (2016). Novel measures of continental-scale avian migration phenology related to proximate environmental cues. Ecosphere 7:e01434.
Kempenaers, B., Borgstrom, P., Loes, P., Schlicht, E., and Valcu, M. (2010). Artificial night lighting affects dawn song, extra-pairing success and lay-date in songbirds. Curr. Biol. 20, 1735–1739. doi: 10.1016/j.cub.2010.08.028
Kishkinev, D., Chernetsov, N., Pakhomov, A., Heyers, D., and Mouritsen, H. (2015). Eurasian reed warblers compensate for virtual magnetic displacement. Curr. Biol. 25, R822–R824.
Kumar, J., Malik, S., Bhardwaj, S. K., and Rani, S. (2018). Bright light at night alters the perception of daylength in Indian weaver bird (Ploceus philippinus). J. Exp. Zool. 329, 488–496. doi: 10.1002/jez.2201
Kumar, V., Kumar, B. S., and Singh, B. P. (1992). Photostimulation of blackheaded bunting: subjective interpretation of day and night depends upon both photophase contrast and light intensity. Physiol. Behav. 51, 1213–1217. doi: 10.1016/0031-9384(92)90311-o
Kumar, V., Rani, S., Malik, S., Trivedi, A. K., Schwabl, I., Helm, B., et al. (2007). Daytime light intensity affects seasonal timing via changes in the nocturnal melatonin levels. Naturwissenschaften 94, 693–696. doi: 10.1007/s00114-007-0243-1
Kumar, V., Singh, S., Misra, M., and Malik, S. (2001). Effects of duration and time of food availability on photoperiodic responses in the migratory male blackheaded bunting (Emberiza melanocephala). J. Exp. Biol. 204, 2843–2848. doi: 10.1242/jeb.204.16.2843
Kumar, V., Singh, S., Misra, M., Malik, S., and Rani, S. (2002). Role of melatonin in photoperiodic time measurement in the migratory redheaded bunting (Emberiza bruniceps) and the nonmigratory Indian weaver bird (Ploceus philippinus). J. Exp. Zool. 292, 277–286. doi: 10.1002/jez.10079
Lahiri, D. K., Ge, Y. W., Sharman, E. H., and Bondy, S. C. (2004). Age-related changes in serum melatonin in mice: higher levels of combined melatonin and 6-hydroxymelatonin sulfate in the cerebral cortex than serum, heart, liver and kidney tissues. J. Pineal Res. 36, 217–223. doi: 10.1111/j.1600-079X.2004.00120.x
Larkin, R. P., and Frase, B. A. (1988). Circular paths of birds flying near a broadcasting tower in cloud. J. Comp. Psychol. 102, 90–93. doi: 10.1037/0735-7036.102.1.90
Liechti, F. (2006). Birds: blowin’ by the wind? J. Ornithol. 147, 202–211. doi: 10.1007/s10336-006-0061-9
Longcore, T., and Rich, C. (2004). Ecological light pollution. Front. Ecol. Environ. 2, 191–198. doi: 10.1890/1540-92952004002[0191:ELP]2.0.CO;2
Malik, S., Rani, S., and Kumar, V. (2004). Wavelength dependency of light induced effects on photoperiodic clock in the migratory blackheaded bunting (Emberiza melanocephala). Chronobiol. Int. 21, 367–384. doi: 10.1081/CBI-120038742
Marra, P. P., Francis, C. M., Mulvihill, R. S., and Moore, F. R. (2005). The influence of climate on the timing and rate of spring bird migration. Oecologia 142, 307–315. doi: 10.1007/s00442-004-1725-x
McGuire, N. L., Kangas, K., and Bentley, G. E. (2011). Effects of melatonin on peripheral reproductive function: regulation of testicular GnIH and testosterone. Endocrinology 152, 3461–3470. doi: 10.1210/en.2011-1053
Miller, M. W. (2006). Apparent effects of light pollution on singing behavior of American robins. Condor 108, 130–139. doi: 10.1650/0010-5422.2006
Misra, M., Rani, S., Singh, S., and Kumar, V. (2004). Regulation of seasonality in the migratory male blackheaded bunting (Emberiza melanocephala). Reprod. Nutr. Dev. 44, 341–352. doi: 10.1051/rnd:2004039
Moore, M. V., Pierce, S. M., Walsh, H. M., Kvalvik, S. K., and Lim, J. D. (2000). Urban light pollution alters the diel vertical migration of Daphnia. Verh. Int. Verein. Limnol. 27, 779–782. doi: 10.1080/03680770.1998.11901341
Mouritsen, H., Heyers, D., and Güntürkün, O. (2016). The neural basis of long-distance navigation in birds. Annu. Rev. Physiol. 78, 133–154. doi: 10.1146/annurev-physiol-021115-105054
Navara, K. J., and Nelson, R. J. (2007). The dark side of light at night: physiological, epidemiological, and ecological consequences. J. Pineal Res. 43, 215–224. doi: 10.1111/j.1600-079X.2007.00473.x
Polak, T., Korine, C., Yair, S., and Holderied, M. W. (2011). Differential effects of artificial lighting on flight and foraging behaviour of two sympatric bat species in a desert. J. Zool. 285, 21–27. doi: 10.1111/j.1469-7998.2011.00808.x
Raap, T., Pinxten, R., and Eens, M. (2015). Light pollution disrupts sleep in free-living animals. Sci. Rep. 5:13557. doi: 10.1038/srep13557
Rani, S., Singh, S., and Kumar, V. (2005a). The pineal clock affects behavioral circadian rhythms but not photoperiodic induction in the Indian weaver bird (Ploceus philippinus). J. Ornithol. 146, 355–364. doi: 10.1007/s10336-007-0236-z
Rani, S., Singh, S., Misra, M., Malik, S., Singh, B. P., and Kumar, V. (2005b). Daily light regulates seasonal responses in the migratory male redheaded bunting (Emberiza bruniceps). J. Exp. Zool. 303A, 541–550. doi: 10.1002/jez.a.187
Rich, C., and Longcore, T. (eds) (2006). Ecological Consequences of Artificial Night Lighting. Washington, DC: Island Press.
Richardson, W. J. (1990). Wind and orientation of migrating birds: a review. Experientia 46, 416–425. doi: 10.1007/BF01952175
Roenneberg, T., Daan, S., and Merrow, M. (2003). The art of entrainment. J. Biol. Rhythms 18, 183–194. doi: 10.1177/0748730403018003001
Santos, C. D., Miranda, A. C., Granadeiro, J. P., Lourenco, P. M., Saraiva, S., and Palmeirim, J. M. (2010). Effects of artificial illumination on the nocturnal foraging of waders. Acta Oecol. 36, 166–172. doi: 10.1016/j.actao.2009.11.008
Singh, J., Rani, S., and Kumar, V. (2012). Functional similarity in relation to the external environment between circadian behavioral and melatonin rhythms in the subtropical Indian weaver bird. Horm. Behav. 61, 527–534. doi: 10.1016/j.yhbeh.2012.01.015
Singh, S., Misra, M., Rani, S., and Kumar, V. (2002). The photoperiodic entrainment and induction of the circadian clock regulating seasonal responses in the migratory blackheaded bunting. Chronobiol. Int. 19, 865–881. doi: 10.1081/CBI-120014570
Terzieva, D. D., Mateva, N. D., and Vladimirova-Kitova, L. G. (2009). Melatonin reference limits at 3:00 AM and 8:00 AM in healthy adults. Clin. Lab. 55, 359–361.
Titulaer, M., Spoelstra, K., Lange, C., and Visser, M. E. (2012). Activity patterns during food provisioning are affected by artificial light in free living great tits (Parus major). PLoS One 7:e37377. doi: 10.1371/journal.pone.0037377
Ubuka, T., Bentley, G. E., Ukena, K., Wingfield, J. C., and Tsutsui, K. (2005). Melatonin induces the expression of gonadotropin-inhibitory hormone in the avian brain. Proc. Natl. Acad. Sci. U.S.A. 102, 3052–3057. doi: 10.1073/pnas.0403840102
Vincze, O., Vágási, C. I., Pap, P. L., Osváth, G., and Møller, A. P. (2015). Brain regions associated with visual cues are important for bird migration. Biol. Lett. 11:20150678. doi: 10.1098/rsbl.2015.0678
Wiltschko, W., Munro, U., Ford, H., and Wiltschko, R. (1993). Red light disrupts magnetic orientation of migratory birds. Nature 364, 525–527. doi: 10.1038/364525a0
Keywords: bunting, light at night, melatonin, migratory, photoperiodic response
Citation: Kumar J, Malik S, Bhardwaj SK and Rani S (2021) Impact of Light at Night Is Phase Dependent: A Study on Migratory Redheaded Bunting (Emberiza bruniceps). Front. Ecol. Evol. 9:751072. doi: 10.3389/fevo.2021.751072
Received: 31 July 2021; Accepted: 06 October 2021;
Published: 08 November 2021.
Edited by:
Davide M. Dominoni, University of Glasgow, United KingdomReviewed by:
René Quispe, Universidad Católica del Norte, Coquimbo, ChileCopyright © 2021 Kumar, Malik, Bhardwaj and Rani. This is an open-access article distributed under the terms of the Creative Commons Attribution License (CC BY). The use, distribution or reproduction in other forums is permitted, provided the original author(s) and the copyright owner(s) are credited and that the original publication in this journal is cited, in accordance with accepted academic practice. No use, distribution or reproduction is permitted which does not comply with these terms.
*Correspondence: Sangeeta Rani, c2FuZ2VldGFyYW5pN0B5YWhvby5jb20=
Disclaimer: All claims expressed in this article are solely those of the authors and do not necessarily represent those of their affiliated organizations, or those of the publisher, the editors and the reviewers. Any product that may be evaluated in this article or claim that may be made by its manufacturer is not guaranteed or endorsed by the publisher.
Research integrity at Frontiers
Learn more about the work of our research integrity team to safeguard the quality of each article we publish.