- 1State Key Laboratory of Vegetation and Environmental Change, Institute of Botany, Chinese Academy of Sciences, Beijing, China
- 2Northeast Institute of Geography and Agroecology, Chinese Academy of Sciences, Changchun, China
- 3University of Chinese Academy of Sciences, Beijing, China
- 4Key Laboratory of Ecosystem Network Observation and Modeling, Institute of Geographic Sciences and Natural Resources Research, Chinese Academy of Sciences, Beijing, China
- 5School of Life Sciences, Northeast Normal University, Changchun, China
Knowing the determinants of seed germination helps us understand plant adaptive strategies to the environment and predict population and community regeneration under climate change. However, multiple factors, including plant and seed traits that influence germination and their relative importance, have received little attention. Here, seed germination experiments were conducted on newly collected seeds for 89 herbaceous species from salinized Songnen grassland. We tested the effects of multiple phylogeny-related plant traits and seed morphological and physiological traits on germination percentage and initial germination time and their relative contribution to shaping germination variation. We found that biennials had higher germination percentages and rates than annuals and perennials. Species with brown seeds had higher germination percentages than those with yellow and black seeds. Eudicots germinated faster than monocots, and seeds with morphophysiological dormancy required more time to initiate germination than those with other kinds of dormancy. Phylogeny-related factors explained more of the variation in germination than seed traits. Seed mass and volume of the large-seeded, but not small-seeded group species were positively correlated with germination percentage. Our findings provide important information for understanding germination variation across species and local adaptation for species in the salinized Songnen grassland.
Introduction
Seed germination is a critical life history stage that affects population establishment and regeneration (Nonogaki et al., 2010). Germination is not only influenced by environmental factors such as temperature (Galíndez et al., 2017), light (Lee et al., 2015), soil moisture (Essery et al., 1954), but also closely related to the characteristics of parent plant (Liu et al., 2018) and seed traits (Mamo et al., 2006). However, the effects of plant and seed traits on germination have received less attention than environmental factors. The relationships between germination and plant and seed traits will help us understand the plant life history strategies and their adaptation to environments.
Phylogeny is a relatively stable characteristic of plant species and does not change with the environment (Zhang et al., 2011). Phylogeny can be used to help interpret inter-specific variations in plant growth and reproductive characteristics, e.g., germination strategy (Figueroa and Armesto, 2001). Previous studies have shown that phylogeny had a significant influence on germination time and germination percentage of seeds (Bu et al., 2008; Wang et al., 2016). Monocots and eudicots are two important taxonomic group (Willey and Wilkins, 2008), which may also influence germination. Plant functional groups, such as grass, legumes, and forbs, have been widely used to test variable traits and responses under different environments at the community and ecosystem levels (Zhang et al., 2014; Zhang et al., 2017). Functional groups also have been suggested for use in studies of seed dispersal (Aslan et al., 2019). Therefore, species in different functional groups may differ in germination responses, but this has rarely been investigated. Plant life form is a crucial part of life history strategy, and this may affect germination. However, previous studies have shown mixed results. Plant life form did not show significant effects on germination percentage of 134 common plant species on the eastern Tibetan Plateau (Xu et al., 2014). However, Bu et al. (2008) found that the perennials had a higher germination percentage than the annuals for 633 species in the same Tibetan site.
Dormancy level and germination of seeds may be affected by the environmental conditions of the mother plant during seed maturation (El-Keblawy and Al-Rawai, 2006). Therefore, seed maturation time may indirectly affect germination. For example, seeds had higher germination percentage (low dormancy) when the mother plant experienced high temperature or short days during seed maturation (Evenari et al., 1966; Galloway, 2002; Baskin and Baskin, 2014).
Seed mass is an important seed morphological trait, reflecting the investment of mother plants to offspring (Beaulieu et al., 2007) and is intimately related to germination (Moles and Westoby, 2004). However, seed mass had been shown positive or negative effects on germination percentage of different types or regional species (Galindez et al., 2009; Wang et al., 2016; Wu et al., 2018). Seed volume is significantly and linearly related with seed mass (Casco and Dias, 2008) and also has a significant effect on germination (Tekrony et al., 2005). However, the previous results are also mixed (Mian et al., 1994). Other seed traits such as seed color have been shown to affect germination at within species level (Bhatt et al., 2019). It is not clear whether seed color affects germination between species. Seed dispersal mode also affects germination (Bu et al., 2008). Most studies have indicated that germination percentage of anemochorous species is higher than that of barochorous and zoochorous species (Augspurger, 1983; Wang et al., 2012). However, a few studies found that ombrohydrochorous species had higher germination percentages than other dispersal modes (Liu et al., 2014). Seed dormancy is widely studied seed traits (Baskin and Baskin, 2014). We do not know if the different kinds of seed dormancy correlate with germination across species from the same habitat.
Although the relationship between seed traits and germination has received some attention lately, most previous studies have focused on only a few seed traits and/or a few species. Few people have tested the effects of multiple seed traits, phylogeny, and plant traits such as plant life form on seed germination for a large number of species regionally and their relative importance (Norden et al., 2009). Several cross-species studies have obtained different conclusions in different geographical regions.
In the present study, we investigated the effects of phylogeny, plant functional type, life form and seed traits, including seed color, mass, volume, dispersal mode, kind of dormancy and seed maturation time on initial germination of newly-collected seeds for 89 common herbaceous species from the salinized Songnen grassland in northeast China. Two hypotheses were tested: (1) The factors that influence germination of species from salinized grassland are different from other regions in some aspects due to the salinized habitats; (2) Taxonomic group, plant functional group and/or plant life form may explained larger proportion of the variation in germination than seed traits.
Materials and Methods
Study Region and Seed Collection
Mature seeds of 89 species were collected in the Songnen grassland in northeast China (123° 44′–47′E, 44° 40′–45′N; Supplementary Figure 1A) during July to September 2016. At the time of natural seed dispersal, seeds were collected from more than 50 plants for each species (Supplementary Figures 2A,B). Seeds of each species were dry-stored in cloth bags at 4°C for 2 to 4 months before they were used in germination tests. Seeds of some species were actually fruits (e.g., Asteraceae) or diaspores (e.g., Poaceae), but they are called seeds hereafter.
The Songnen grassland distributes in the semi-humid climate area of temperate zone. The mean annual precipitation is 400 mm, of which most is received between June and September. Mean annual temperature is 5°C with a mean of 2882 sunshine hours per year. The annual temperature and precipitation in 2016 was shown in Supplementary Figure 1B. The main soil type is chernozem, with 2.0% of soil organic carbon content and 0.15% of total nitrogen content. The saline-alkaline soil accounts for 59% of the total area 24220 km2 of Songnen grassland. Electrical conductivity and pH of the soil are 6 mS cm–1 and 10.7, respectively (Wang L. et al., 2009). The main salts in the salinized soil are NaCl, Na2SO4, NaHCO3 and Na2CO3 (Qu and Guo, 2003).
Germination Experiment
The experiment was conducted in December 2016 to test initial germination (Baskin and Baskin, 2014) in programmed incubators (HPG-400HX, Haerbin China) at an alternate temperature regime of 18/25°C, with a 12-h photoperiod (Sylvania cool white fluorescent lamps, 25 μmol photons m–2 s–1, PAR) and a 12-h dark period, which is an appropriate temperature for local species to germinate. Seeds were incubated on two layers of filter paper placed in 7 cm diameter Petri dishes with distilled water. There were four replicates with 25 seeds in each replicate. Petri dishes were sealed with parafilm to prevent the loss of water evaporation. Germination was recorded every day and seeds were considered to have germinated when the radicle emerged. The experiment lasted for 30 days when there were no more seeds germinated. The initial germination percentage (number of germinated seeds/total seeds) and the initial germination time (average of the time when first germinated seed was observed in each Petri dish) of each species were calculated.
Plant and Seed Traits Measurement and Classification
Taxonomic group: The 89 species were recorded as monocot or eudicot according to their families (Supplementary Table 1).
Plant functional group: All the species were divided into four functional groups, which were grass, legume, Asteraceae and forb (Basto et al., 2015).
Plant life form: The longevity of species was divided into annual, biennials or perennials according to Flora of China Online1.
Seed maturation time: Seed maturation time were calculated as days that passed through from 1 January to the collection date of each species. For example, seed maturation time of species with seeds collected on 1 July was 181 days.
Seed mass and volume: One hundred seeds were selected randomly and weighed with an electronic balance (0.0001 g, METTLER TOLEDO ME204), with five replicates for each species. Individual seed weight was then calculated. Seed length (L) and width (W) of five randomly selected seeds were measured with vernier caliper for each species. Seed volume was calculated as: πLW2/6 (Casco and Dias, 2008).
Seed coat color: Species were classified into four color categories based on the observations and relevant literature (Wang et al., 2016): (1) pale yellow, yellow or reddish yellow (referred as yellow); (2) light yellowish brown or yellowish brown (referred as light brown); (3) brown or dark brown (referred as dark brown); (4) black (referred as black).
Seed dispersal mode: The dispersal mode of the species was classified into four groups according to the morphological features and the appendage of the diaspores: (1) zoochorous species: the diaspores have awns, spines, or hooks that can adhere to fur of animals, or fleshly or arillate fruits that can be eaten by animals; (2) anemochorous species, the diaspores have membranous wings, hairs, bracts, a persistent or inflated perianth, or a pappus that is easily dispersed by wind; (3) ombrohydrochorous species, the seeds can produce mucilage when wetted; (4) autochorous species, the diaspores are explosively dehiscing capsules or pods that can throw the seeds to some distance from the parent plant and the diaspores have no apparent morphological structure and disperse via gravity (Navarro et al., 2009).
Kind of seed dormancy: Seed dormancy was classified into four kinds according to the physiology and physical features of seeds, germination experiment and relevant literatures (de Souza et al., 2015): (1) physiological dormancy (referred to as PD); (2) morpho-physiological dormancy (referred as MPD); (3) physical dormancy (referred as PY) and (4) non-dormancy (referred as ND) that were defined as species with germination percentage more than 80% (Baskin and Baskin, 2014).
Seed trait variation among the plant species used in our study was shown in Supplementary Table 2.
Statistical Analysis
We constructed phylogenetic trees using PHYLOCOM1 followed the APG III phylogenetic system (Supplementary Figure 3; Webb et al., 2008; Bremer et al., 2009). To meet normality and homoscedasticity of errors, germination percentage was arcsine transformed and initial germination time were log-transformed. GLMM were carried out using R-package glmmTMB (Bates et al., 2015). Germination percentage and initial germination time were the response variables in the models. We included taxonomic group, plant life form, plant functional group, seed mature time, kind of seed dormancy, dispersal mode, seed coat color, seed mass, and volume as fixed effects and species and replicate of germination Petri dish as the random effects. The models assumed binomial error distributions and Poisson error distributions for germination percentage and initial germination time. We also used variance partitioning (vegan package in R) and venn diagrams (Ding et al., 2020) to determine the total variance in germination percentage that was explained by a predictor matrix of plant life form, seed volume, seed coat color, and kind of seed dormancy and in initial germination time that was explained by a predictor matrix of taxonomic group, plant functional group, plant life form and kind of seed dormancy (uniquely and jointly). We used the factors that had significant effects on the dependent variable in this analysis. The relationships between seed mass, seed volume, seed maturation time and germination percentage, and initial germination time were analyzed using linear regression analysis. In regression analysis of seed mass and volume, seeds were then divided into two classes according to seed mass or volume: >1 mg, <1 mg or >1 mm3, <1 mm3 (Galindez et al., 2009). All analyses were carried out by vegan package of (version 3.5.1, R Core Team, 2015).
Results
The Effects of Plant and Seed Traits on Germination Percentage
Plant life form, seed volume, seed coat color and kind of seed dormancy had significant effects on germination percentage (P < 0.05, Table 1). Germination percentages of biennials (77%) and perennials (36%) were significantly higher than those of annuals (18%, Figure 1C). Brown seeds germinated in a higher percentage than yellow and black seeds (Figure 1D). The significant effect of kind of seed dormancy on germination percentage was due to non-dormant species. There were no significant differences in germination percentage between PD, MPD, and PY (Table 1 and Figure 1F). The effect of seed maturation time on germination percentage was marginally negatively significant (P = 0.0542) with no significant linear regression relationships (Supplementary Figure 4). Taxonomic group, plant functional group, seed mass and seed dispersal mode had no significant effects on germination percentage (Figures 1A,B,E and Table 1).
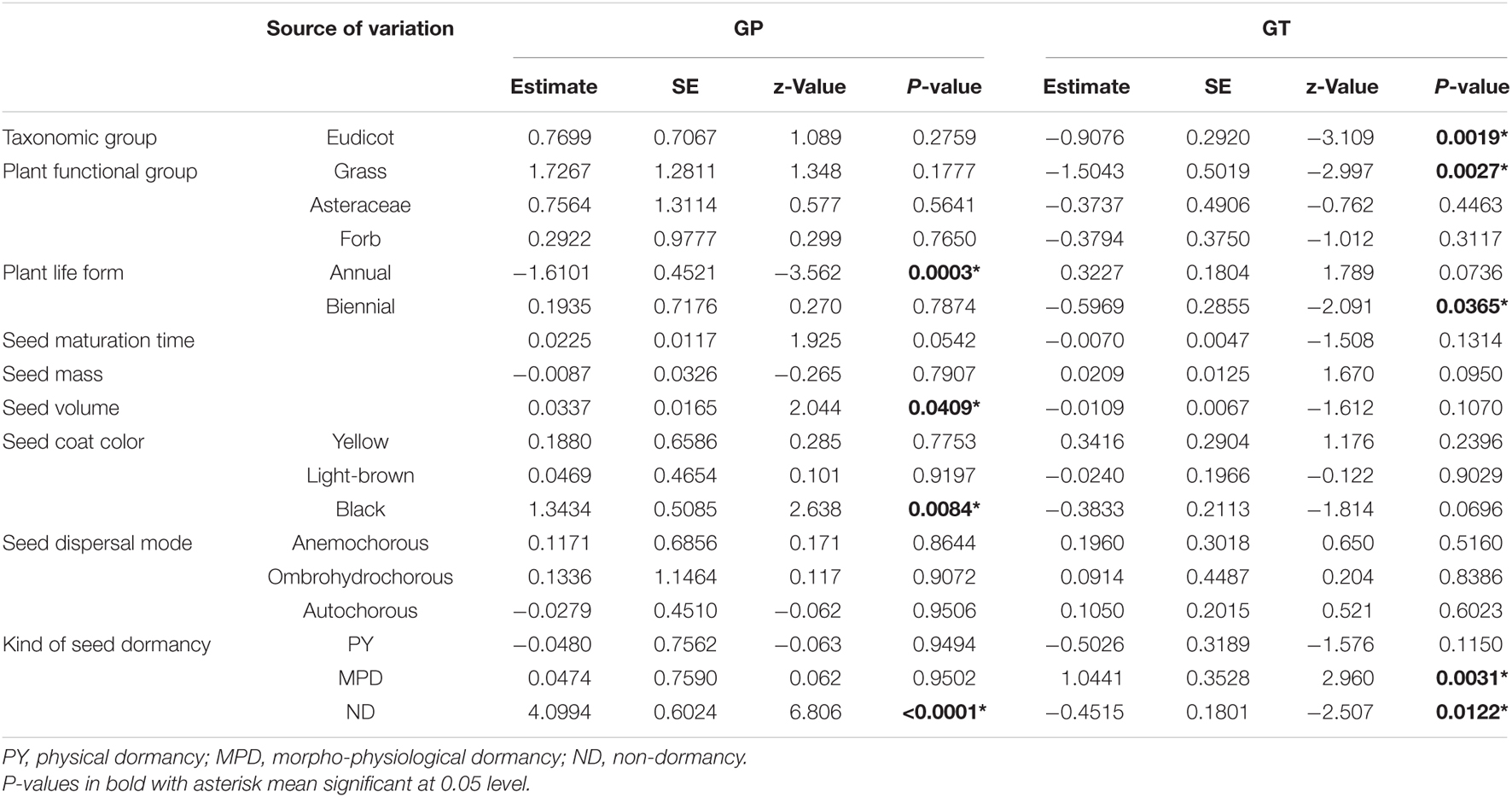
Table 1. Results of generalized linear mixed model testing the effects of taxonomic group, plant functional group, plant life form, seed maturation time, mass, volume, coat color, dispersal mode and kind of dormancy on germination percentage (GP) and initial germination time (GT).
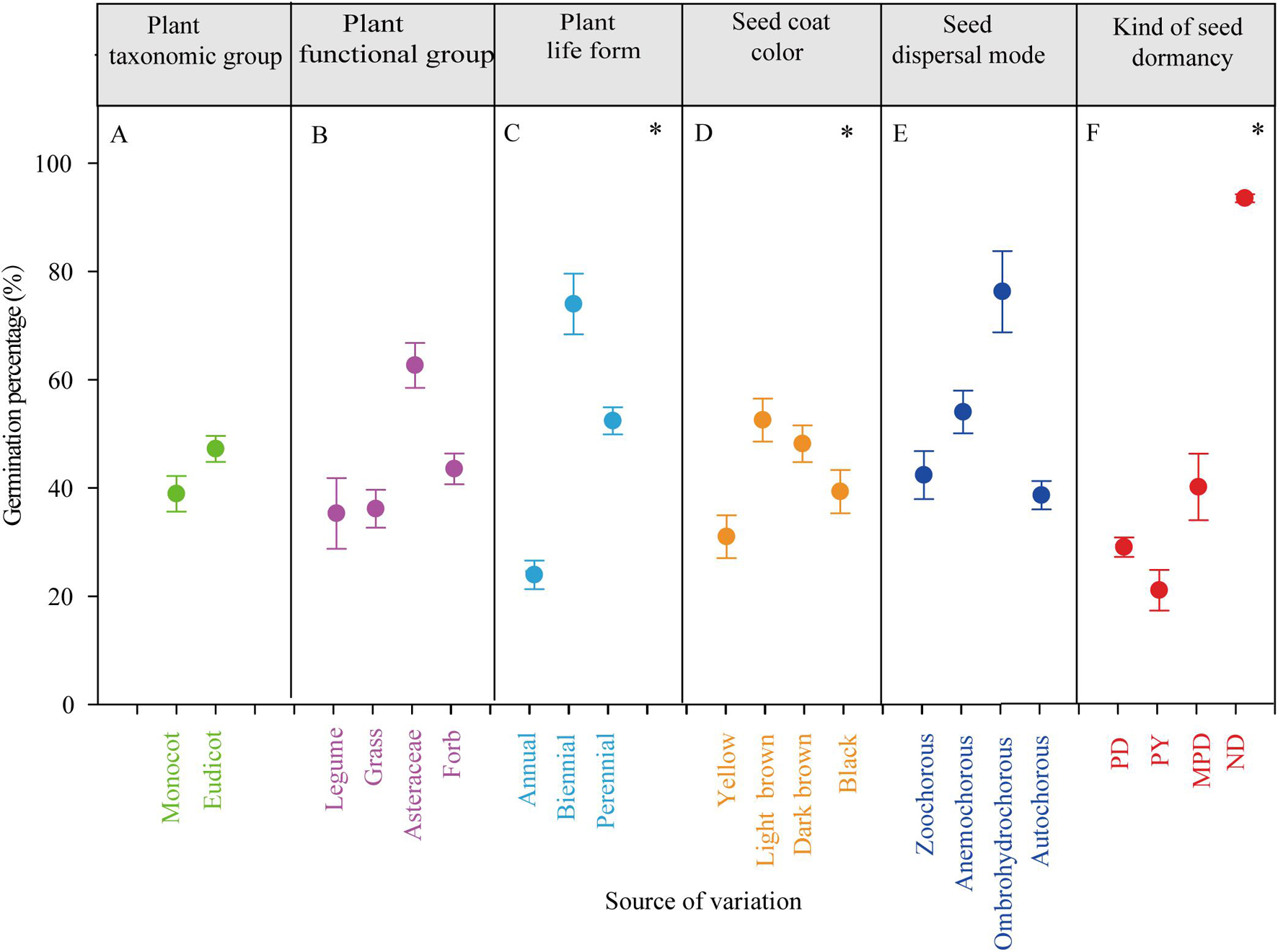
Figure 1. The effects of plant taxonomic group (A), plant functional group (B), plant life form (C), seed coat color (D), seed dispersal mode (E), and kind of seed dormancy (F) on germination percentage. PD, physiological dormancy; PY, physical dormancy; MPD, morpho-physiological dormancy; ND, non-dormancy. ∗P < 0.05.
Although seed volume significantly influenced germination percentage, germination percentage was not significantly linearly correlated with seed volume and seed mass (Supplementary Figure 5). There was no significant correlation between seed mass and volume of the small-seeded group of species and germination percentage (P > 0.05, Supplementary Figure 5). However, seed mass and volume of large-seeded group of species were significantly positively correlated with germination percentage (P < 0.05). When dividing the species based on seed volume (<1 mm3 and >1 mm3), the same results were found (Supplementary Figure 5).
The Effects of Plant and Seed Traits on Time to Initial Germination
Initial germination time was significantly affected by taxonomic group, plant functional group, plant life form and kind of seed dormancy (P < 0.05, Table 1 and Figures 2A–C,F). Specifically, monocot had a higher initial germination time (7.7 d, lower germination rate) than eudicot (5.2 d, Figure 2A). Initial germination time of biennials was significantly lower (2.2 d) than annuals (6.3 d) and perennials (6.4 d, Figure 2C). Species with MPD (14.3 d) required more time to initiate germination than species with other kinds of dormancy (PD, 6.4 d; PY, 4.9 d) and non-dormancy (3.9 d, Figure 2F). Seed mass, seed volume, seed coat color and seed dispersal mode had no significant effects on initial germination time (P > 0.05; Table 1, Figures 2D,E, and Supplementary Figure 6). Initial germination time was not correlated with seed mass and seed volume of small seeded or large-seeded group species (Supplementary Figure 6).
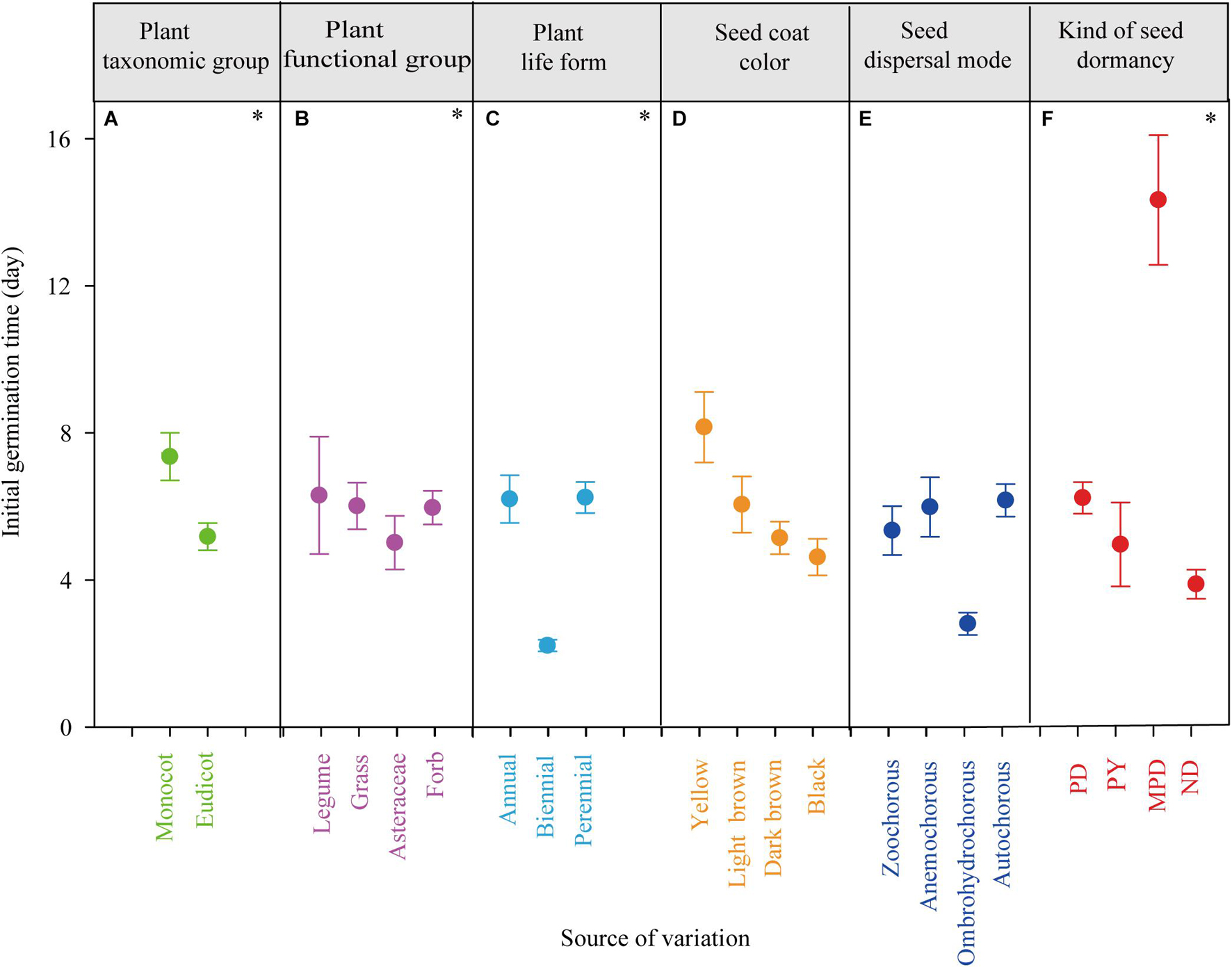
Figure 2. The effects of plant taxonomic group (A), plant functional group (B), plant life form (C), seed coat color (D), seed dispersal mode (E), and kind of seed dormancy (F) on initial germination time. PD, physiological dormancy; PY, physical dormancy; MPD, morpho-physiological dormancy; ND, non-dormancy. ∗P < 0.05.
The Relative Importance of Plant and Seed Traits in Germination Variation
Redundancy analysis revealed that plant life form, seed volume, seed coat color and kind of seed dormancy independently explained 5, 2, 2 and 49% of the variance in germination percentage, respectively (Figure 3A). When other factors were not removed from the analysis, the variance in germination percentage explained by plant life form, seed volume, seed coat color and kind of seed dormancy increased to 22, 5, 6 and 64%, respectively. Therefore, kind of seed dormancy can explain the largest variance of germination percentage among these traits. The joint effects of any two or three factors were low, except that of plant life form and kind of seed dormancy (11%). Overall, the four factors together could explain 75% of the variation in germination percentage.
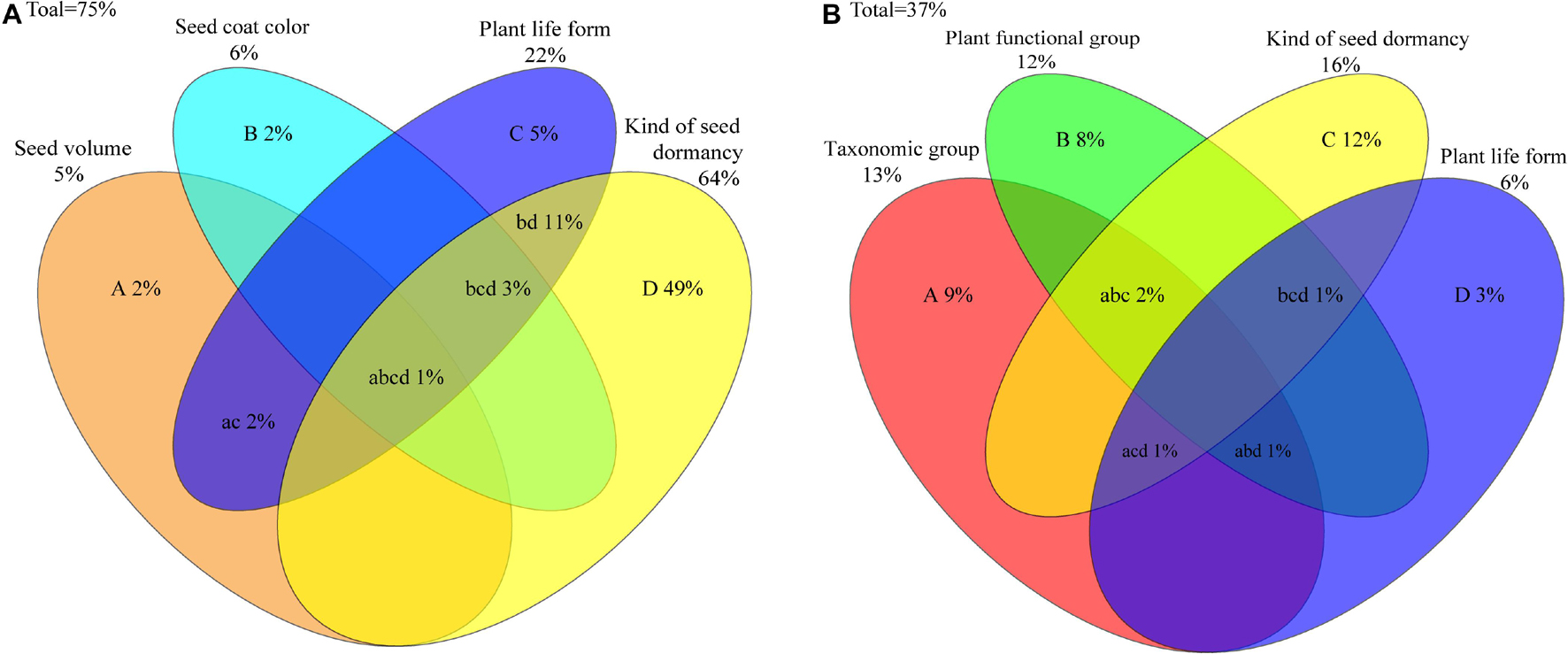
Figure 3. Variation partitioning (%) of plant life form, seed volume, seed coat color, and seed dormancy type accounting for germination percentage (A) and taxonomic group, plant functional group, plant life form and kind of seed dormancy accounting for initial germination time of the studied species (B). The uppercase letters denote the independent effects and the lowercase letters denote the joint effects of the overlapped two, three and four factors.
For initial germination time, taxonomic group, plant function group, plant life form and kind of seed dormancy independently explained 9, 8, 3 and 12% of the variance, respectively (Figure 3B). When the other traits were not removed from the RDA analysis, the variance of initial germination time explained by taxonomic group, plant function group, plant life form and kind of seed dormancy increased to 13, 12, 6 and 16%, respectively. Therefore, kind of seed dormancy explained more of the variance in initial germination time than other factors. The joint effects of any three factors were very low and the four factors together could explain 37% variation of initial germination time.
Discussion
The Relationships Between Plant Traits and Seed Germination
In our study, plant life form had a significant effect on germination percentage, and taxonomic group, plant functional group and life form had significant effects on initial germination time (Table 1). RDA analysis also showed that these factors explained a larger proportion of the variation in germination than seed traits, except kind of seed dormancy (Figure 3). Our results are consistent with those from other studies on the relationship between phylogeny and germination percentage in alpine meadows (Bu et al., 2008), temperate rain forests (Xu et al., 2014), arid and semiarid zones and degraded sandy grassland (Wang J.H. et al., 2009; Wang et al., 2016). Species within the same family or functional group probably have similar germination behavior (Wang et al., 2016). This means that germination percentage is constrained by phylogeny, regardless of the habitats or ecosystems, although germination percentage has large inter-specific and site-specific variations.
Specifically, biennials had a significantly higher germination percentage and rate (less initial germination time) than annuals and perennials. This is a special result in plant life form-germination relationships. Previous studies, which have usually divided plant life form into annuals and perennials, showed contradictory results. Insignificant results have been found in degraded sandy grassland (Wang et al., 2016) and arid/semi-arid zone (Wang J.H. et al., 2009). For 134 common species on the eastern Tibetan Plateau, annuals germinated significantly earlier than perennials (Xu et al., 2014), but perennials of 633 species from the same region had higher germination percentages than annuals (Bu et al., 2008). Schippers et al. (2001) have indicated that non-dormancy (higher germination percentage) is an adaptive strategy of annuals in disturbed environments, but Rees has shown that perennials have less dormancy than annuals in a variable environment (Rees, 1994). Therefore, these results may be due to different habitats or floras. In our study, most of the biennial species were salt-tolerant species (e.g., Artemisia spp.) or with mucilaginous seeds (e.g., Leonurus japonicus). Higher germination percentages or rates may help them adapt to the salinized habitats in Songnen grassland.
Among plant functional groups, Asteraceae had the highest germination percentages and rates. Wang et al. (2016) have also found that Asteraceae species had a high germination percentage. In our study, 15.9% of the species were Asteraceae. Nearly half the Asteraceae species (7/15) had non-dormant seeds (GP >80%, GT <4.5 d), which was 40% of the total number of species with non-dormant seeds. Additionally, we found that eudicots germinated faster than monocots. One possible reason is that most monocot species are Poaceae and no endosperm (for example Asteraceae) vs. endosperm (Poaceae) matter. It takes more time to move the stored food to the growing parts of the embryo in Poaceae than in Asteraceae (Baskin and Baskin, 2014).
The Relationships Between Seed Traits and Germination
Overall, there was no significant linear relationship between seed mass/seed volume and germination percentage, although seed volume had significant effect on it. Previous studies have shown no relationship (Wang et al., 2012), positive relationship (Galindez et al., 2009; Xu et al., 2014) or negative relationship (Jankowska-Blaszczuk and Daws, 2007; Wang et al., 2016) between seed (or diaspore) mass and germination percentage. However, after dividing the species into small-seeded and large-seeded groups, seed mass and seed volume of the large-seeded group (seed mass >1 mg or seed volume >1 mm3) significantly and positively correlated with germination percentage, but seed mass and volume of the small-seeded group were not significantly correlated with germination percentage. This may be the result of local environmental adaptation. Large seeds contain more nutrition substances (Leishman et al., 2000), which helps to improve germination percentage, seedling growth and survival ability in saline conditions (Leishman et al., 2000). Our previous study showed that large-seeded species were more salt tolerant than small-seeded species in high salinity (Zhang et al., 2015). Small seeds have fewer reserves, and they might encounter serious selection pressure and have more complex germination response (Leishman et al., 2000). For example, previous studies reported that germination of small seeds was more sensitive to light (Pearson et al., 2002; Bu et al., 2017) and temperature (Galíndez et al., 2017) than large seeds. Therefore, the germination response of small seeds may be more dependent on the ambient environmental factors than those of large seeds.
Kind of seed dormancy significantly influenced germination percentage and rate and explained the largest variation among the factors. The results were mainly due to species with non-dormant seeds. Germination percentages of species with different kinds of seed dormancy were similar, all with wide variation range, 0–59% for PY, 0–71% for PD, 0–66% for MPD. Therefore, kind of seed dormancy had an insignificant effect on germination percentage in our study. However, the initial germination time of MPD was higher than that for seeds with other kinds of dormancy. MPD is a combination of MD and PD, and the embryo of seeds must grow to a specific size before the seed can germinate (Baskin and Baskin, 2014). Therefore, it is reasonable that seeds with MPD require a long time to germinate.
The dispersal mode of seeds is closely related to germination and establishment of seedling (Grime et al., 1981). However, dispersal mode of seeds had no significant effect on germination percentage and rate when all factors we tested were taken into account in the full model. This result is inconsistent with results from previous studies in other regions (Liu et al., 2014). It has been reported that adhesion-dispersed species (Xu et al., 2014) or wind-dispersed species (Bu et al., 2008; Wang et al., 2012) had the highest germination percentage among the dispersal modes. Seeds of anemochorous and zoochorous species have relatively long dispersal distance, and they usually germinate to a higher percentage than those with short dispersal distance (e.g., barochorous species), which may suggest escape from sibling or kin competition and predation (Venable and Brown, 1993; Levin et al., 2003; Venable et al., 2008). From our results, germination percentage and rate of the ombrohydrochorous species was the highest, followed by anemochorous and zoochorous species (Figures 1E and 2E). Similar results were found in species from degraded sandy grassland (Wang et al., 2016) and the desert (Liu et al., 2014). The mucilage that is secreted by outer layer of the seed coat of ombrohydrochorous species can conserve seed moisture, which would be beneficial for germination and seedling survival under drought and salt stress conditions (Yang et al., 2012). Thus, ombrohydrochorous species had relatively high germination percentage and rate in sandy and salinized regions, such as in Songnen grassland.
Inconsistent with the study in a degraded sandy grassland (Wang et al., 2016), seed color had an significant effect on germination in our study. Although color variation of seeds in these arid and semi-arid regions was not large, we found that species with brown seeds germinated in a higher percentage than those with yellow or black seeds. The effect of seed maturation time on germination percentage approached significance with a negative trend. That means species with late-maturing seeds tended to have lower germination percentage and high dormancy than those with early-maturing seeds (Edwards and Elkassaby, 1988).
The Limitations and Advantages of This Study
Our study have some limitations. First, we studied 89 species. Some rare species and/or species with less seeds were not included. Germination characteristics related with species abundance would deserve to be studied. Second, the sites we collected the seeds were close to each other (Supplementary Figure 1). Germination characteristics of seeds from different sites across Songnen grassland and the relationships with environmental factors may explain phenotypic plasticity of species. These needs further studies.
Germination variation across species are the results of heredity, evolution and adaptation to their environments (Gremer and Venable, 2014; Willis et al., 2014). Many factors interactively influence germination. Knowing how multiple factors related to germination and the relative importance were crucial for understanding plant life history strategies and evolution and predicting future scenarios under climate change. Our study indicate that phylogeny related factors such as taxonomic group, plant functional group and plant life form had significant effects on germination, especially initial germination time. Seed traits such as seed volume and seed coat color may mainly influence germination percentage. Species from Songnen grassland have special local adaptation characteristics. For instance, biennial and ombrohydrochorous species had higher germination percentage and rate, which better adapted to the salinized conditions.
Conclusion
Our study revealed the comprehensive influences and relative importance of phylogeny-related factors and seed traits on germination of species in the salinized grassland. Plant life form, seed volume, and seed coat color significantly influenced germination percentage, and taxonomic group, plant functional group, plant life form and kind of seed dormancy significantly influenced initial germination time. Overall, phylogeny-related factors can explain a larger proportion of the variation in germination than seed traits. We found that biennial and ombrohydrochorous species had higher germination percentages and rates than the other species in salinized Songnen grassland. This study increases our knowledge on the relationships between plant attributes, seed traits and germination and provides important information for plant adaptation under different environmental conditions.
Data Availability Statement
The raw data supporting the conclusions of this article will be made available by the authors, without undue reservation.
Ethics Statement
This study followed the IUCN Policy Statement on Research Involving Species at Risk of Extinction. The plant species involved in this study are not threatened species. The experimental research was carried out under the principle of less collection, less damage and maintaining the population in the natural environment. The processes that research specimens were acquired (including transportation) conformed scrupulously to procedures and regulations adopted under international legal agreements. The plant materials in this study are mainly collected from private land and permission was obtained from the land owner. We also guided local land owners to make rational use of grassland resources to protect the original wild plant species. To support reproducibility, voucher specimens for all wild plants described in the manuscript had deposited in the Germplasm Bank of Wild Species that provides access to deposited material. Information on the voucher specimen via the following link: http://www.genobank.org/. We guarantee that the above statements base on facts. We shall take the corresponding economical and legal responsibilities.
Author Contributions
MZ and HZ: conceptualization. MZ, ZL, and YW: methodology and investigation. MZ, HZ, and HY: writing. All authors contributed to the article and approved the submitted version.
Funding
This work was supported by the National Natural Science Foundation of China (41971069 and 41571055) and the Strategic Priority Research Program of the Chinese Academy of Sciences (No. XDA23080401).
Conflict of Interest
The authors declare that the research was conducted in the absence of any commercial or financial relationships that could be construed as a potential conflict of interest.
Publisher’s Note
All claims expressed in this article are solely those of the authors and do not necessarily represent those of their affiliated organizations, or those of the publisher, the editors and the reviewers. Any product that may be evaluated in this article, or claim that may be made by its manufacturer, is not guaranteed or endorsed by the publisher.
Acknowledgments
We thank Carol Baskin from University of Kentucky for confirming the kind of dormancy of the species and the improvement of English in this study.
Supplementary Material
The Supplementary Material for this article can be found online at: https://www.frontiersin.org/articles/10.3389/fevo.2021.748038/full#supplementary-material
Footnotes
References
Aslan, C., Beckman, N. G., Rogers, H. S., Bronstein, J., Zurell, D., Hartig, F., et al. (2019). Employing plant functional groups to advance seed dispersal ecology and conservation. AoB Plants 11:14. doi: 10.1093/aobpla/plz006
Augspurger, C. K. (1983). Seed dispersal of the tropical tree, platypodium-elegans, and the escape of its seedlings from fungal pathogens. J. Ecol. 71, 759–771. doi: 10.2307/2259591
Baskin, C. C., and Baskin, J. M. (2014). “Chapter 3 – types of seeds and kinds of seed dormancy,” in Seeds, 2nd Edn, eds C. C. Baskin and J. M. Baskin (San Diego, CA: Academic Press), 37–77.
Basto, S., Thompson, K., Phoenix, G., Sloan, V., Leake, J., and Rees, M. (2015). Long-term nitrogen deposition depletes grassland seed banks. Nat. Commun. 6:6185. doi: 10.1038/ncomms7185
Bates, D., Machler, M., Bolker, B. M., and Walker, S. C. (2015). Fitting linear mixed-effects models using lme4. J. Stat. Softw. 67, 1–48. doi: 10.18637/jss.v067.i01
Beaulieu, J. M., Moles, A. T., Leitch, I. J., Bennett, M. D., Dickie, J. B., and Knight, C. A. (2007). Correlated evolution of genome size and seed mass. New Phytol. 173, 422–437. doi: 10.1111/j.1469-8137.2006.01919.x
Bhatt, A., Bhat, N. R., Caron, M. M., and Gallacher, D. (2019). Dimorphic fruit colour is associated with differences in germination of Calligonum comosum. Botany 97, 263–268. doi: 10.1139/cjb-2018-0167
Bremer, B., Bremer, K., Chase, M. W., Fay, M. F., Reveal, J. L., Soltis, D. E., et al. (2009). An update of the Angiosperm Phylogeny Group classification for the orders and families of flowering plants: APG III. Bot. J. Linn. Soc. 161, 105–121. doi: 10.1111/j.1095-8339.2009.00996.x
Bu, H. Y., Du, G. Z., Chen, X. L., Xu, X. L., Liu, K., and Wen, S. J. (2008). Community-wide germination strategies in an alpine meadow on the eastern Qinghai-Tibet plateau: phylogenetic and life-history correlates. Plant Ecol. 195, 87–98. doi: 10.1007/s11258-007-9301-1
Bu, H. Y., Ge, W. J., Zhou, X. H., Qi, W., Liu, K., Xu, D. H., et al. (2017). The effect of light and seed mass on seed germination of common herbaceous species from the eastern Qinghai-Tibet Plateau. Plant Species Biol. 32, 263–269. doi: 10.1111/1442-1984.12147
Casco, H., and Dias, L. S. (2008). Estimating seed mass and volume from linear dimensions of seeds. Seed Sci. Technol. 36, 230–236. doi: 10.15258/sst.2008.36.1.28
de Souza, T. V., Torres, I. C., Steiner, N., and Silveira Paulilo, M. T. (2015). Seed dormancy in tree species of the Tropical Brazilian Atlantic Forest and its relationships with seed traits and environmental conditions. Braz. J. Bot. 38, 243–264. doi: 10.1007/s40415-014-0129-3
Ding, J. X., Kong, D. L., Zhang, Z. L., Cai, Q., Xiao, J., Liu, Q., et al. (2020). Climate and soil nutrients differentially drive multidimensional fine root traits in ectomycorrhizal-dominated alpine coniferous forests. J. Ecol. 108, 2544–2556. doi: 10.1111/1365-2745.13407
Edwards, D. G. W., and Elkassaby, Y. A. (1988). Effect of flowering phenology, date of cone collection, cone-storage treatment and seed pretreatment on yield and germination of seeds from a douglas-fir seed orchard. For. Ecol. Manag. 25, 17–29. doi: 10.1016/0378-1127(88)90131-4
El-Keblawy, A., and Al-Rawai, A. (2006). Effects of seed maturation time and dry storage on light and temperature requirements during germination in invasive Prosopis juliflora. Flora 201, 135–143. doi: 10.1016/j.flora.2005.04.009
Essery, R. E., Kirsop, B. H., and Pollock, J. R. A. (1954). Studies in barley and malt: I. effects of water on germination tests. J. Inst. Brew. 60, 473–481. doi: 10.1002/j.2050-0416.1954.tb06239.x
Evenari, M., Koller, D., and Gutterman, Y. (1966). Effects of evironment of mother plant on germination by control of seed-coat permeability to water in ononis sicula guss. Aust. J. Biol. Sci. 19, 1007–1016. doi: 10.1071/bi9661007
Figueroa, J. A., and Armesto, J. J. (2001). Community-wide germination strategies in a temperate rainforest of Southern Chile: ecological and evolutionary correlates. Aust. J. Bot. 49, 411–425. doi: 10.1071/BT00013
Galindez, G., Ortega-Baes, P., Daws, M. I., Suehring, S., Scopel, A. L., and Pritchard, H. W. (2009). Seed mass and germination in Asteraceae species of Argentina. Seed Sci. Technol. 37, 786–790. doi: 10.15258/sst.2009.37.3.27
Galíndez, G., Seal, C. E., Daws, M. I., Lindow, L., Ortega-Baes, P., and Pritchard, H. W. (2017). Alternating temperature combined with darkness resets base temperature for germination (Tb) in photoblastic seeds of Lippia and Aloysia (Verbenaceae). Plant Biol. 19, 41–45. doi: 10.1111/plb.12449
Galloway, L. F. (2002). The effect of maternal phenology on offspring characters in the herbaceous plant Campanula americana. J. Ecol. 90, 851–858. doi: 10.1046/j.1365-2745.2002.00714.x
Gremer, J. R., and Venable, D. L. (2014). Bet hedging in desert winter annual plants: optimal germination strategies in a variable environment. Ecol. Lett. 17, 380–387. doi: 10.1111/ele.12241
Grime, J. P., Mason, G., Curtis, A. V., Rodman, J., Band, S. R., Mowforth, M. A. G., et al. (1981). A comparative-study of germination characteristics in a local flora. J. Ecol. 69, 1017–1059. doi: 10.2307/2259651
Jankowska-Blaszczuk, M., and Daws, M. I. (2007). Impact of red: far red ratios on germination of temperate forest herbs in relation to shade tolerance, seed mass and persistence in the soil. Funct. Ecol. 21, 1055–1062. doi: 10.1111/j.1365-2435.2007.01328.x
Lee, N., Park, J., Kim, K., and Choi, G. (2015). The transcriptional coregulator LEUNIG_HOMOLOG inhibits light-dependent seed germination in Arabidopsis. Plant Cell 27, 2301–2313. doi: 10.1105/tpc.15.00444
Leishman, M. R., Wright, I. J., Moles, A. T., and Westoby, M. (2000). “The evolutionary ecology of seed size,” in Seeds: The Ecology of Regeneration in Plant Communities, 2nd Edn, ed. M. Fenner (Wallingford, UK: CABI Publishing), 31–57.
Levin, S. A., Muller-Landau, H. C., Nathan, R., and Chave, J. (2003). The ecology and evolution of seed dispersal: a theoretical perspective. Ann. Rev. Ecol. Evol. Syst. 34, 575–604. doi: 10.1146/annurev.ecolsys.34.011802.132428
Liu, H., Zhang, D., Yang, X., Huang, Z., Duan, S., and Wang, X. (2014). Seed dispersal and germination traits of 70 plant species inhabiting the Gurbantunggut Desert in northwest China. Sci. World J. 2014:346405–346405. doi: 10.1155/2014/346405
Liu, K., Cao, S., Du, G., Baskin, J. M., Baskin, C. C., Bu, H., et al. (2018). Linking seed germination and plant height: a case study of a wetland community on the eastern Tibet Plateau. Plant Biol. 20, 886–893. doi: 10.1111/plb.12845
Mamo, N., Mihretu, M., Fekadu, M., Tigabu, M., and Teketay, D. (2006). Variation in seed and germination characteristics among Juniperus procera populations in Ethiopia. For. Ecol. Manag. 225, 320–327. doi: 10.1016/j.foreco.2006.01.026
Mian, M. A. R., Nafziger, E. D., Kolb, F. L., and Teyker, R. H. (1994). Root Size and distribution of field-grown wheat genotypes. Crop Sci. 34, 810–812. doi: 10.2135/cropsci1994.0011183X003400030037x
Moles, A. T., and Westoby, M. (2004). Seedling survival and seed size: a synthesis of the literature. J. Ecol. 92, 372–383. doi: 10.1111/j.0022-0477.2004.00884.x
Navarro, T., El Oualidi, J., Taleb, M. S., Pascual, V., and Cabezudo, B. (2009). Dispersal traits and dispersal patterns in an oro-Mediterranean thorn cushion plant formation of the eastern High Atlas, Morocco. Flora 204, 658–672. doi: 10.1016/j.flora.2008.08.005
Nonogaki, H., Bassel, G. W., and Bewley, J. D. (2010). Germination—still a mystery. Plant Sci. 179, 574–581. doi: 10.1016/j.plantsci.2010.02.010
Norden, N., Daws, M. I., Antoine, C., Gonzalez, M. A., Garwood, N. C., and Chave, J. (2009). The relationship between seed mass and mean time to germination for 1037 tree species across five tropical forests. Funct. Ecol. 23, 203–210. doi: 10.1111/j.1365-2435.2008.01477.x
Pearson, T. R. H., Burslem, D., Mullins, C. E., and Dalling, J. W. (2002). Germination ecology of neotropical pioneers: interacting effects of environmental conditions and seed size. Ecology 83, 2798–2807.
Qu, G., and Guo, J. (2003). The relationship between different plant communities and soil characteristics in Songnen grassland. Acta Prataculturae Sin. 12, 18–22.
R Core Team. (2015). R: A Language and Environment for Statistical Computing. Vienna: R Foundation for Statistical Computing.
Rees, M. (1994). Delayed germination of seeds- a look at the effects of adult longevity, the timing of reproduction, and population age/stage structure. Am. Nat. 144, 43–64. doi: 10.1086/285660
Schippers, P., Van Groenendael, J. M., Vleeshouwers, L. M., and Hunt, R. (2001). Herbaceous plant strategies in disturbed habitats. Oikos 95, 198–210. doi: 10.1034/j.1600-0706.2001.950202.x
Tekrony, D. M., Shande, T., Rucker, M., and Egli, D. B. (2005). Effect of seed shape on corn germination and vigour during warehouse and controlled environmental storage. Seed Sci. Technol. 33, 185–197. doi: 10.15258/sst.2005.33.1.19
Venable, D., and Brown, J. (1993). The population-dynamic function of seed dispersal. Plant Ecol. 10, 31–55. doi: 10.1007/BF00052210
Venable, D. L., Flores-Martinez, A., Muller-Landau, H. C., Barron-Gafford, G., and Becerra, J. X. (2008). Seed dispersal of desert annuals. Ecology 89, 2218–2227. doi: 10.1890/07-0386.1
Wang, J., Chen, W., Baskin, C. C., Baskin, J. M., Cui, X., Zhang, Y., et al. (2012). Variation in seed germination of 86 subalpine forest species from the eastern Tibetan Plateau: phylogeny and life-history correlates. Ecol. Res. 27, 453–465. doi: 10.1007/s11284-011-0916-2
Wang, J. H., Baskin, C. C., Cui, X. L., and Du, G. Z. (2009). Effect of phylogeny, life history and habitat correlates on seed germination of 69 arid and semi-arid zone species from northwest China. Evol. Ecol. 23, 827–846. doi: 10.1007/s10682-008-9273-1
Wang, L., Seki, K., Miyazaki, T., and Ishihama, Y. (2009). The causes of soil alkalinization in the Songnen Plain of Northeast China. Paddy Water Environ. 7, 259–270. doi: 10.1007/s10333-009-0166-x
Wang, Z., Wang, L., Liu, Z., Li, Y., Liu, Q., and Liu, B. (2016). Phylogeny, seed trait, and ecological correlates of seed germination at the community level in a degraded Sandy Grassland. Front. Plant Sci. 7:1532. doi: 10.3389/fpls.2016.01532
Webb, C. O., Ackerly, D. D., and Kembel, S. W. (2008). Phylocom: software for the analysis of phylogenetic community structure and trait evolution. Bioinformatics 24, 2098–2100. doi: 10.1093/bioinformatics/btn358
Willey, N. J., and Wilkins, J. (2008). Phylogeny and growth strategy as predictors of differences in cobalt concentrations between plant species. Environ. Sci. Technol. 42, 2162–2167. doi: 10.1021/es071531r
Willis, C. G., Baskin, C. C., Baskin, J. M., Auld, J. R., Venable, D. L., Cavender-Bares, J., et al. (2014). The evolution of seed dormancy: environmental cues, evolutionary hubs, and diversification of the seed plants. New Phytol. 203, 300–309. doi: 10.1111/nph.12782
Wu, H., Meng, H., Wang, S., Wei, X., and Jiang, M. (2018). Geographic patterns and environmental drivers of seed traits of a relict tree species. For. Ecol. Manag. 422, 59–68. doi: 10.1016/j.foreco.2018.04.003
Xu, J., Li, W. L., Zhang, C. H., Liu, W., and Du, G. Z. (2014). Variation in seed germination of 134 common species on the Eastern Tibetan Plateau: phylogenetic, life history and environmental correlates. PLoS One 9:13. doi: 10.1371/journal.pone.0098601
Yang, X. J., Baskin, J. M., Baskin, C. C., and Huang, Z. Y. (2012). More than just a coating: ecological importance, taxonomic occurrence and phylogenetic relationships of seed coat mucilage. Perspect. Plant Ecol. Evol. Syst. 14, 434–442. doi: 10.1016/j.ppees.2012.09.002
Zhang, H., Zhang, G., Lü, X., Zhou, D., and Han, X. (2015). Salt tolerance during seed germination and early seedling stages of 12 halophytes. Plant Soil 388, 229–241. doi: 10.1107/s11104-2322-3
Zhang, S.-B., Slik, J. W. F., Zhang, J.-L., and Cao, K.-F. (2011). Spatial patterns of wood traits in China are controlled by phylogeny and the environment. Glob. Ecol. Biogeogr. 20, 241–250. doi: 10.1111/j.1466-8238.2010.00582.x
Zhang, X., Johnston, E. R., Barberán, A., Ren, Y., Lü, X., and Han, X. (2017). Decreased plant productivity resulting from plant group removal experiment constrains soil microbial functional diversity. Glob. Change Biol. 23, 4318–4332. doi: 10.1111/gcb.13783
Keywords: dispersal mode, kind of dormancy, germination rate, herbaceous plant, phylogeny, seed mass
Citation: Zhao M, Liu Z, Zhang H, Wang Y and Yan H (2021) Germination Characteristics Is More Associated With Phylogeny-Related Traits of Species in a Salinized Grassland of Northeastern China. Front. Ecol. Evol. 9:748038. doi: 10.3389/fevo.2021.748038
Received: 27 July 2021; Accepted: 08 September 2021;
Published: 06 October 2021.
Edited by:
Jian Sun, Institute of Tibetan Plateau Research, Chinese Academy of Sciences (CAS), ChinaReviewed by:
Zhanhuan Shang, Lanzhou University, ChinaJinniu Wang, Chengdu Institute of Biology, Chinese Academy of Sciences (CAS), China
Copyright © 2021 Zhao, Liu, Zhang, Wang and Yan. This is an open-access article distributed under the terms of the Creative Commons Attribution License (CC BY). The use, distribution or reproduction in other forums is permitted, provided the original author(s) and the copyright owner(s) are credited and that the original publication in this journal is cited, in accordance with accepted academic practice. No use, distribution or reproduction is permitted which does not comply with these terms.
*Correspondence: Hongxiang Zhang, emhhbmdob25neGlhbmdAaWdhLmFjLmNu