- 1Department of Anthropology, University of Massachusetts, Amherst, MA, United States
- 2Department of Geology, University of Cincinnati, Cincinnati, OH, United States
- 3Department of Anthropology, University of Cincinnati, Cincinnati, OH, United States
- 4Department of Anatomy, College of Graduate Studies, Midwestern University, Glendale, AZ, United States
- 5Department of Geosciences, University of Massachusetts, Amherst, MA, United States
- 6School of Earth Sciences, University College Dublin, Dublin, Ireland
- 7Department of Foundational Sciences, College of Medicine, Central Michigan University, Mount Pleasant, MI, United States
- 8Mention Bassins Sédimentaires, Evolution, Conservation, Université d’Antananarivo, Antananarivo, Madagascar
- 9Department of Geography, University of Tennessee, Knoxville, Knoxville, TN, United States
- 10Duke Lemur Center Museum of Natural History, Duke University, Durham, NC, United States
- 11Madagascar Cave Diving Association, Antananarivo, Madagascar
- 12Field Museum of Natural History, Chicago, IL, United States
- 13Association Vahatra, Antananarivo, Madagascar
- 14Institute of Zoology, Zoological Society of London, London, United Kingdom
- 15Department of Biological Sciences, Northern Illinois University, DeKalb, IL, United States
- 16Department of Biological Sciences, Fordham University, New York, NY, United States
- 17Division of Vertebrate Zoology, American Museum of Natural History, New York, NY, United States
- 18Department of Earth, Atmospheric and Planetary Sciences, Massachusetts Institute of Technology, Cambridge, MA, United States
- 19Dominican Republic Speleological Society, Cabarete, Dominican Republic
- 20Biology Program, Stockton University, Galloway, NJ, United States
- 21Madagascar National Parks, Antananarivo, Madagascar
- 22Department of Anthropology, Portland State University, Portland, OR, United States
- 23Protec Dive Centers, Tulum, Mexico
Madagascar experienced a major faunal turnover near the end of the first millenium CE that particularly affected terrestrial, large-bodied vertebrate species. Teasing apart the relative impacts of people and climate on this event requires a focus on regional records with good chronological control. These records may document coeval changes in rainfall, faunal composition, and human activities. Here we present new paleontological and paleoclimatological data from southwestern Madagascar, the driest part of the island today. We collected over 1500 subfossil bones from deposits at a coastal site called Antsirafaly and from both flooded and dry cave deposits at Tsimanampesotse National Park. We built a chronology of Late Holocene changes in faunal assemblages based on 65 radiocarbon-dated specimens and subfossil associations. We collected stalagmites primarily within Tsimanampesotse but also at two additional locations in southern Madagascar. These provided information regarding hydroclimate variability over the past 120,000 years. Prior research has supported a primary role for drought (rather than humans) in triggering faunal turnover at Tsimanampesotse. This is based on evidence of: (1) a large freshwater ecosystem west of what is now the hypersaline Lake Tsimanampesotse, which supported freshwater mollusks and waterfowl (including animals that could not survive on resources offered by the hypersaline lake today); (2) abundant now-extinct terrestrial vertebrates; (3) regional decline or disappearance of certain tree species; and (4) scant local human presence. Our new data allow us to document the hydroclimate of the subarid southwest during the Holocene, as well as shifts in faunal composition (including local extirpations, large-vertebrate population collapse, and the appearance of introduced species). These records affirm that climate alone cannot have produced the observed vertebrate turnover in the southwest. Human activity, including the introduction of cattle, as well as associated changes in habitat exploitation, also played an important role.
Introduction
The literature on humans versus climate shifts as triggers for extinction of Madagascar’s megafauna has grown enormously over the past few years, but no consensus has emerged. There are cultural sites where humans have been present over extended periods of time with little impact on megafauna (e.g., Douglass et al., 2018). Likewise, climate fluctuations in the Late Quaternary have been documented from northern to southern Madagascar (Scroxton et al., 2017, 2019; Voarintsoa et al., 2017; Wang et al., 2019; Railsback et al., 2020) with little apparent impact on the fauna; large-vertebrate extinctions are well represented at a range of sites from the very late Holocene (Crowley, 2010; Godfrey et al., 2019; Hansford et al., 2021). The notion that neither climate nor humans alone, but the combination of the two, was responsible for the megafaunal crash of the Late Holocene has gained broad acceptance in recent years (e.g., Burney et al., 2003, 2004; Goodman and Jungers, 2014), but no single hypothesis appears to apply across the island.
The problem of understanding endemic vertebrate decline is perhaps most vexing for the subarid region of Tsimanampesotse National Park (hereafter TNP) in southwestern Madagascar, where very different interpretations of past events have been proposed. MacPhee (1986), for example, was reluctant to attribute the disappearance of large vertebrates from this part of Madagascar to climate change. He argued on the basis of the xerophytic plants and microfauna endemic to the region that conditions in southwestern Madagascar have been dry over a long period of time, and that we cannot assume the hydroclimate of the subarid south prior to large vertebrate extinctions was markedly different than it is today. He further maintained that even in dry regions, wet microhabitats may be sufficient to support species dependent on moist conditions. In contrast, Goodman and Jungers (2014) defended a primary role for aridification in triggering local species extirpation and extinction. They hypothesized that the climate of the southwest was wetter and less seasonal when now-extinct vertebrates were present, and that wetter habitats likely persisted up to around 1000 years ago when conditions became too dry to sustain now-extinct species, both large and small, including certain freshwater birds.
Developing an understanding of extinction across Madagascar demands that we first focus on changes with good chronological resolution at the local area or ecological levels, and then gradually build broader regional chronologies. Here we present paleontological and paleoclimatological data from a multiyear, collaborative project focusing on a part of Madagascar that is exceptionally dry today – the southwestern coastal region south of the Onilahy River. Our team is the first to document this region’s changes in hydroclimate through the Holocene based on stalagmites collected from several caves at TNP as well as caves to the north and northeast of the park. Our team is also the first to explore subfossils preserved in the underwater deposits of the flooded caves of TNP. Our paleontological mission focused mainly on retrieving bones from flooded as well as dry caves within the park, but we also collected bones from lacustrine deposits at Antsirafaly, ∼45 km north of TNP.
Our goal in this paper is to provide an overview of our work and place our results within a broader regional context. We describe: (1) changes in hydroclimate or rainfall of southern Madagascar over the past 25,000 years; (2) vertebrate taxa found in subfossil cave deposits at TNP and lacustrine deposits at Antsirafaly; and (3) the chronology of local decline of endemic vertebrate taxa. We also document evidence, direct and indirect, of past human presence at TNP. We then address the following four questions: (1) Is today’s hydroclimate in southwestern Madagascar significantly drier than the hydroclimate several thousand years ago, when there were numerous large-bodied endemic vertebrate species in this region? (2) What triggered local extirpation of vertebrate species at TNP and Antsirafaly? (3) How does the local extirpation of vertebrate species at TNP and Antsirafaly relate to their regional decline at other sites in southern Madagascar? (4) What, ultimately, caused the extinction of the large-bodied vertebrates in Madagascar’s southwest?
Materials and Methods
Collection Sites
Geographic Locations of Collection Sites
We collected stalagmites and subfossils in southern Madagascar over the course of four field seasons. In Figure 1 we show the locations of the limestone caves that yielded stalagmites covering the period covering the Last Glacial Maximum (LGM, 22.9-19.4 kyr BP) and onward, and the sites at which we obtained subfossils. All collection sites are in the subarid “spiny thicket” terrestrial ecoregion, with the exception of Andranomilitsy Cave, which is located in the southern part of the subhumid “central highland” terrestrial ecoregion. Ecoregions are geographic units that share environmental features and characteristic species; the major ecoregions of Madagascar have been described by Burgess et al. (2004).
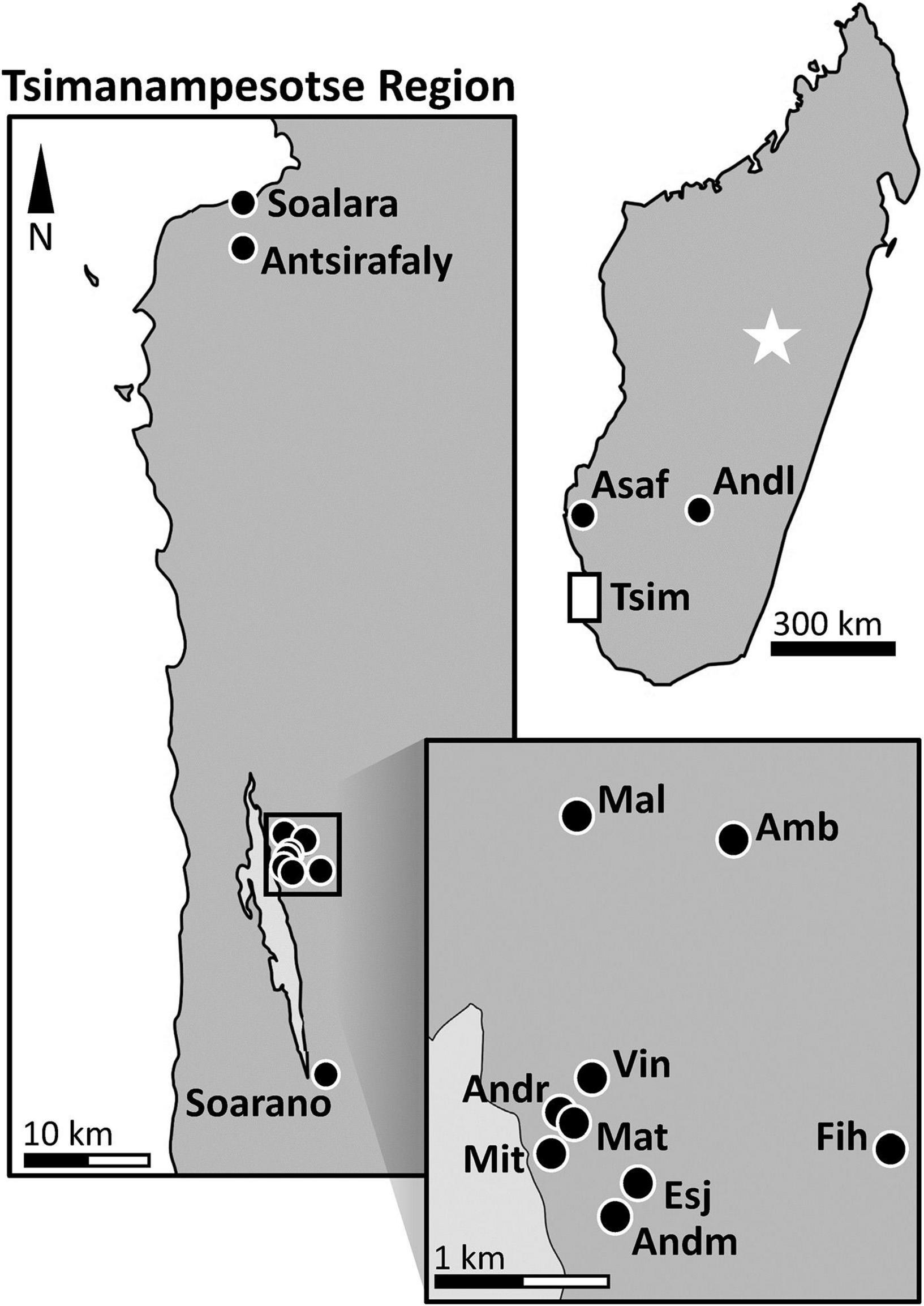
Figure 1. Fossil and stalagmite site names and abbreviations. Map of Madagascar: Asaf, Asafora Cave; Andl, Andranomilitsy Cave; Tsim, region of the Tsimanampesotse National Park. The star represents the capital of Madagascar, Antananarivo. Vertical rectangular blowout box: Town of Soalara and nearby lacustrine site Antsirafaly; Soarano Cave is indicated near the southernmost end of Lake Tsimanampesotse. Square blowout box: Amb, Ambolely Sinkhole; Andm, Andriamaniloke Cave; Andr, Andranohilova Cave; Esj, Esajo Cave; Fih, Fihamy Cave; Mal, Malazamanga Cave; Mat, Maty Cave, or Anjohimpaty Rock Shelter; Mit, Mitoho Cave; Vin, Vintany Cave.
Stalagmites were collected at two of the three major caves of the TNP karst system that are now phreatic (i.e., largely below the water table): Mitoho and Vintany. We also collected stalagmites at four dry caves within the park (Soarano, Andriamaniloke, Esajo, and Fihamy), as well as two dry caves well outside the park (Andranomilitsy and Asafora). Andranomilitsy Cave is located 300 km northeast of Tsimanampesotse, off Route National 7 in the southcentral portion of the subhumid Central Plateau and near the town of Ihosy and the Isalo National Park. Given its position in the interior of the island and given the dual east-west and north-south precipitation gradients in Madagascar, this site can be expected to have been consistently wetter than sites south of Toliara on or near the west coast. Asafora Cave is in the Morombe District, 130 km north of Toliara, 200 km north of Tsimanampesotse, and ∼10 km from the coast. It also can be expected to have been slightly wetter than the region of Tsimanampesotse (including Antsirafaly) due to the north-south precipitation gradient.
We collected subfossils at all three phreatic caves of the karst system at TNP: Vintany, Mitoho, and a cave called Malazamanga (literally “famous blue”). Subfossils were also collected at four dry sites within TNP, two of which (Anjohimpaty and Andranohilova) are part of the Mitoho Cave system. Anjohimpaty (or Maty Cave, literally “cave of the dead”) is a rock shelter with cave art (anthropomorphic images and graffiti). Andranohilova (literally “at the water our descendants will inherit, where the drongo drinks”) is a small, shaft-like dry cave that opens into the main portion of Mitoho Cave. The other two dry subfossil collection sites at TNP were Ambolely sinkhole and Soarano Cave. Finally, we also collected subfossils at Antsirafaly (literally “place of the sacred salt”), a lacustrine site near the coastal town of Soalara, ∼45 km north of TNP. We collected plant material (seeds, bark, twigs) in association with subfossils from sediments in two caves at TNP (five locations from Vintany and one from Malazamanga).
Exploration History
Exploratory paleontological missions to Tsimanampesotse began in the 1930s when Perrier de la Bâthie (1934) and Petit (1935) reported fragmentary subfossil remains of crocodiles (Voay robustus) and giant tortoises (Aldabrachelys grandidieri) at the main entrance to Mitoho Cave. Three decades later, Ravelonanosy and Duflos (1964) explored Andranohilova Cave, which was much later discovered by us to be one of several entrances into the Mitoho flooded cave system. MacPhee (1986) conducted a survey of the dry deposits at the entrances to TNP caves, including Mitoho as well as Anjohimpaty where he reported seeing human bones. He also compared the subfossils he found at the various caves to those reported by Lamberton (1937) at Ankazoabo Cave, almost 60 km south of Mitoho Cave. In 2001, a team led by Karen Samonds visited Andranohilova Cave while prospecting for Cenozoic fossil-bearing sediments, and collected some reference specimens from the floor of this cave.
Our paleontological mission began in 2014 with an initial exploration and survey of both TNP (Rosenberger et al., 2015) and Antsirafaly. Limited collection of subfossil bones from the surface of the submerged floor of Vintany Cave to determine underwater preservation of organic material began in 2015.
Our first large-scale effort to collect subfossils was launched in 2016, when team divers collected bones from the floors of all three flooded caves. We did no underwater excavation of sediments at that time so we did not know the degree to which the sediment might be fossiliferous. In addition to doing surface collection under water, we collected bones from three dry sites within the park: Soarano, Anjohimpaty, and Ambolely (Figure 1).
In 2018 and 2019, we followed different collection methodologies for each TNP locality, and, for the first time, targeted selected locations within Vintany Cave for careful manual excavation by divers, down to a maximum of one foot below the sediment surface. We found that Vintany Cave sediments preserved abundant subfossils, and we mapped and recorded all collection localities within the cave. We further noted whether each specimen came from excavated sediment or from the surface of the submerged cave floor (i.e., on top of rocks or sediment).
In the Mitoho Cave system, we collected microfaunal bones (rodents, bats, and birds) from eolian deposits on the floor of Anjohimpaty Rock Shelter. In the flooded cave passages of the Mitoho system, divers collected bones underwater from: (1) the surface of the cave floor, (2) between large rocks, (3) on the surface of a massive bat guano mound, (4) in the crevices and on the shelves that lined the walls of a vertical “chimney,” and (5) in a deep horizontal passage at the chimney floor. The top of the vertical chimney is close to Andranohilova. Given the proximity of this entrance to the underwater chimney, it is clear that Andranohilova was the original source of the subfossil remains that accumulated within, and below, the vertical chimney passage. The narrow, open-air entrance of Andranohilova leads to a wide chamber that we called the “bat cave room”; this room has a pool of water at its floor and a low ceiling where bats roost today. Beneath the water is the flooded portion of the Mitoho Cave system. Directly below the bat cave room there is a very large, now entirely submerged, guano mound which spans the floor of this chamber. The periphery of the southwestern chamber floor is marked by a series of boulders, a gap among which forms the entrance to the vertical chimney passage to the deeper sections of the cave.
We spent limited time exploring Malazamanga Cave. Access to this flooded cave is precarious because it is situated at the bottom of a dry and deep, collapsed sinkhole, and navigation of the underwater passages requires technical skills. On the floor of the sinkhole there is a wide rock shelter visible in the northern wall that narrows to a dark passage about 5–6 m wide and 2.5 m tall and ends at a small pool only about 1 m deep. Below the water’s surface, a small “duck-under” opening leads to a circuitous underwater passage, marked by small (garage-sized) rooms that ultimately lead to a series of chambers more comparable in size to basketball courts. Divers found partially buried subfossils in only one of the small rooms, approximately 400 m from the entrance at the shallow pool, shortly before reaching the larger chambers. The bones likely washed in from an overhead fissure that was open for a limited period but is now closed, and invisible to divers from the inside.
In 2019, our team returned to Antsirafaly where we extracted subfossils from an isolated dried pond. In the region there are multiple such features, which appear to have been part of an extensive fluvial-lacustrine system that may have produced isolated oxbow lakes and ponds as it dried. Subfossils had been retrieved at this site previously by local villagers (which we designated “Soalara” to distinguish them from bones we collected ourselves). Some of these bones articulated with the bones that we collected, suggesting that they belonged to the same individuals.
Within the perimeter of the former pond, we dug pits at 14 locations, searching for subfossil bones. We also dug two pits outside its perimeter (one to a depth of 113 cm and the other to a depth of 80 cm) to build a stratigraphic profile of the site. Our profile-pit sediments transitioned gradually from sand at the top to clay at a depth of 30 cm from the top. The color of the clay transitioned from red to gray from 50 to 73 cm below the surface. There was a light gray indurated layer between 73 and 80 cm, possibly indicative of drying, below which the sediment was composed entirely of gray clay. This clay also became wetter with depth, and we reached the current water table at 113 cm depth. These profile pits contained gastropod shells but no vertebrate bones.
In contrast, the 14 pits we dug within the perimeter of the pond yielded abundant bones of now-extinct species, beginning at slightly different depths (from 65 to 76 cm, depending on the pit) and continuing to below the water table in at least one pit (Pit H, GPS coordinates –23.62959 S, 43.71833 E). Most subfossils were found in moist clay, below the indurated layer. Because of the disturbed nature of this site, we selected many specimens for radiocarbon dating to establish a chronology, taking precautions not to sample different bone elements that could belong to the same individual.
Analytical Methods
Stalagmite Age Determinations
Of the stalagmites that we collected, 27 were amenable to U-Th disequilibrium dating, producing stratigraphically sequential dates. Twenty-three stalagmites came from six caves within the boundaries of TNP, one from Andranomilitsy Cave, and three from Asafora Cave. From these 27 stalagmites we conducted 131 U-Th individual age determinations; 120 came from the Tsimanampesotse stalagmites, 10 from Asafora stalagmites, and one from the single Andranomilitsy stalagmite. Our U-Th disequilibrium dating methods follow Hellstrom (2003) and Cheng et al. (2013), with half-lives from Jaffey et al. (1971) and Cheng et al. (2013). All dates were generated at the Department of Earth, Atmospheric and Planetary Sciences, Massachusetts Institute of Technology, Cambridge, MA, United States. Seventeen U-Th disequilibrium dates have not been previously published; 33 are in Scroxton et al. (2019), two are in Faina et al. (in press), and 791. All use the same chronological scale as radiocarbon (14C), where 1950 CE = 0 calendar years before present (yr BP) and subsequent years are given negative numbers. Additionally, Faina et al. (in press) used 10 radiocarbon dates in the age model of stalagmite AF2. These extend the growth period of AF2, and at Morombe in general, to 3.5 kyr BP.
Identifying Taxa and Documenting Traces of Human Activity
Genus and species identifications of subfossils were made by team members with appropriate expertise for particular orders or families. When needed, we used comparative collections housed at the Université d’Antananarivo, Madagascar; the University of Massachusetts, Amherst, MA, United States; Midwestern University, Glendale, AZ, United States; the Field Museum of Natural History, Chicago, IL, United States; the Duke University Division of Fossil Primates, Durham, NC, United States; and the American Museum of Natural History, New York, NY, United States. We examined bone specimens for any traces of human butchery (following the methods of Hansford et al., 2020) and we documented additional signs of past human presence at bone collection sites.
Radiocarbon Dating and Date Calibration for Vertebrate and Plant Remains
Subfossil specimens selected for dating were processed at the University of Cincinnati, OH, United States. From each specimen, we removed approximately 250 mg of bone with tin snips or a rotary Dremel tool equipped with a saw blade. Samples were coarsely chopped or crushed into ∼1–3 mm fragments and demineralized in 0.5 N hydrochloric acid (HCl) under refrigeration for 5–10 days. HCl was refreshed every 3–5 days until samples were completely demineralized. Samples were rinsed five times in ultrapure water, with agitation (vortexing) and centrifugation steps occurring with each rinse. Samples were then soaked in 0.01 N sodium hydroxide (NaOH) for 4 h at room temperature to remove humic acids, rinsed with ultrapure water five times (as described above), and freeze dried. Lastly, samples were gelatinized in 0.01 N HCl at ca. 75°C overnight and passed through 1.5 μm glass fiber filters under vacuum. Samples were again dried down via lyophilization.
After we confirmed sufficient preservation of organic content using collagen yield, atomic C:N, and stable carbon and nitrogen isotope values (e.g., Ambrose, 1990; van Klinken, 1999), we sent aliquots of dried collagenous residue to the Center for Accelerator Mass Spectrometry at Lawrence Livermore National Laboratory (Livermore, CA, United States) for radiocarbon dating. Plant samples from sediment in flooded caves were dried, packaged in plastic bags and sent to the Beta Analytic Testing Laboratory (Miami, FL, United States).
We calibrated individual conventional 14C ages to 2σ calendar years before present (Cal yr BP) using the Southern Hemisphere calibration curve SHCal20, in Calib 8.2 (Hogg et al., 2020; Stuiver et al., 2021). Calibrated age ranges (min and max) were rounded to the nearest 5-year intervals. All radiocarbon dates (vertebrate and plant) reported herein are calibrated ages (with the exception of conventional 14C dates also provided in Tables 3, 4). We report them as mean values in yr BP ± 1σ.
In total, we obtained 14C dates from 65 bones (32 from TNP and 33 from Antsirafaly) and six plant remains. All samples from Antsirafaly yielded sufficient organic material for direct dating but the same was not true for TNP, where we needed to process many more than 32 samples to obtain 32 dates. While all TNP botanic samples were datable, many vertebrate specimens from the flooded caves could not be directly dated. This is not peculiar to the flooded caves of Tsimanampesotse; other researchers have noted that bones that are continually immersed in water tend to have low protein content (e.g., Nielsen-Marsh and Hedges, 2000; Bailey and Flemming, 2008; Stinnesbeck et al., 2017).
For Vintany Cave, our overall failure rate for obtaining sufficient organic material was 68.4% for bones of extinct animals and 51.7% for bones of extant animals. We failed to extract sufficient collagen for radiocarbon dating from any of the sampled extinct animal specimens that we collected from the top of the cave floor. However, our failure rate was much lower (25%) for bones of extinct animals excavated from within sediment. For much smaller-bodied extant species at Vintany, a different pattern held, probably because many individuals died so recently that their bones had not lost much organic content. In contrast to the extinct animals sampled, over 80% of the bones of extant animals sampled from the cave floor yielded good collagen, while our success rate for buried bones was much lower (presumably because many are considerably older). These discrete trends in collagen preservation for buried bones are unsurprising given expected differences in protein loss due to microbial activity in buried bones of small versus large diameter (Pfretzschner, 2004).
Reconstructing Hydroclimate
To develop a long-term record of pluvial versus interpluvial conditions in southwestern and southcentral Madagascar, we used U-Th disequilibrium dating of growth phases of speleothems. In semiarid and arid environments, this tool can be used as a paleoclimate indicator of pluvial conditions (Ayliffe et al., 1998; Vaks et al., 2010). To grow, speleothems require calcium ion supersaturation in percolating drip waters (White, 1976). To achieve supersaturation, sufficient precipitation must fall to allow for soil development, soil respiration and high soil CO2 concentrations. This results in sufficiently strong percolating carbonic acid to dissolve the carbonate host rock and redeposit the calcium carbonate upon degassing of CO2, which occurs in the lower-CO2 concentration atmospheres of cave environments. The amount of rainfall necessary for speleothem growth to occur is estimated to be approximately 250–300 mm per year, but this is also highly dependent on evaporation and the seasonality of rainfall (Vaks et al., 2010). For southwest Madagascar, with annual rainfall near the minimum required for speleothem growth, periods of stalagmite deposition and non-deposition can be used to infer pluvial versus arid climate. The difference in rainfall amount between pluvial and interpluvial periods need not be large.
One hundred and seventeen of the 120 TNP stalagmite dates were used to generate a summary of stalagmite growth periods in southern Madagascar between 125,000 and 0 years BP (one U-Th date that was older than 125,000 years and two that were younger than 0 were not used). Seventy-two dates from stalagmites from all sites (TNP, Asafora and Andranomilitsy) provided a detailed record of the past 25,000 years (i.e., LGM onward), the period of primary interest to us.
We plotted date “density” curves to determine the probability of wet versus dry hydroclimate at any point in time. Probability density plots are created by summing assumed Gaussian distributions for each individual age with attendant error at regular intervals and normalizing the distribution so that the integral equals 1. Kernel density estimates are like probability density plots except that a constant Gaussian width is used for all ages. This prevents individual precise ages from dominating the plot, but also underplays the spread of low-precision ages. Because some stalagmites were sampled more than others, the dates cannot be considered independent of one another, and therefore the relative heights of the peaks are as much a function of sampling density as a natural indicator of relative wetness.
Reconstructing the History of Cave Flooding
In addition to the 117 dates from stalagmites mentioned above, a small number of stalagmites (notably stalagmite AV2 from Vintany Cave) revealed significant age reversals. We excluded these ages from our reconstruction of past hydroclimate, but as these stalagmites still provided an approximate indication of growth timing, they were nevertheless capable of providing geochronological constraints on, for example, the timing of cave flooding.
Stalagmites from coastal caves can be used, along with global records of the rise in sea level, to reconstruct the history of cave flooding (Dumitru et al., 2021). This history can in turn be used to determine a minimum age for bones that must have accumulated in certain locations prior to when the water table reached their top depths within the caves. This method for inferring minimum age for extinct animals is particularly useful when bones lack collagen and cannot be dated directly.
The Tsimanampesotse flooded cave network is low lying and only a few kilometers from the sea; thus, the local water table is closely tied to sea level. The lower parts of these caves have been alternatively phreatic (when sea level is high, as it is now) or vadose (when sea level was lower). Because speleothem growth is almost always subaerial, the fact that stalagmites exist in now-flooded portions of the caves is evidence of a much lower water table at times in the past. As sea level rose during the last deglaciation and into the Early Holocene, the freshwater lens would have been pushed up until it intersected with the caves. Cave flooding would have been gradual, progressing from bottom to top. Stalagmites that were actively growing when the water level reached their tops would have ceased growing at that time; their top dates would thus provide a record of when they became submerged. It is therefore possible to extract a maximum age for the timing of the last rise of the water table from the top dates of submerged stalagmites, combined with information on submerged stalagmite distribution and depths. This is a maximum age for flooding because stalagmites could have stopped growing earlier due to arid conditions.
Results
Stalagmites
Glacial and Post-glacial Climate Inferred From Growth Periods of Stalagmites
Eleven of the 23 stalagmites that we collected at TNP cover portions of the LGM and/or the last deglaciation (Table 1) and allow us to affirm pluvial conditions at TNP between 25.8 and 11.7 thousand years before present (kyr BP). The wettest interval was the LGM itself, judging from the large number of stalagmites showing growth at that time and their widespread distribution within the park (Table 1); however, this pluvial period can be described broadly as having lasted until stalagmite MT1 stopped growing at 11.7 kyr BP. This was synchronous with the end of the Younger Dryas, the terminal cold event of both the last deglaciation period and the Late Pleistocene.
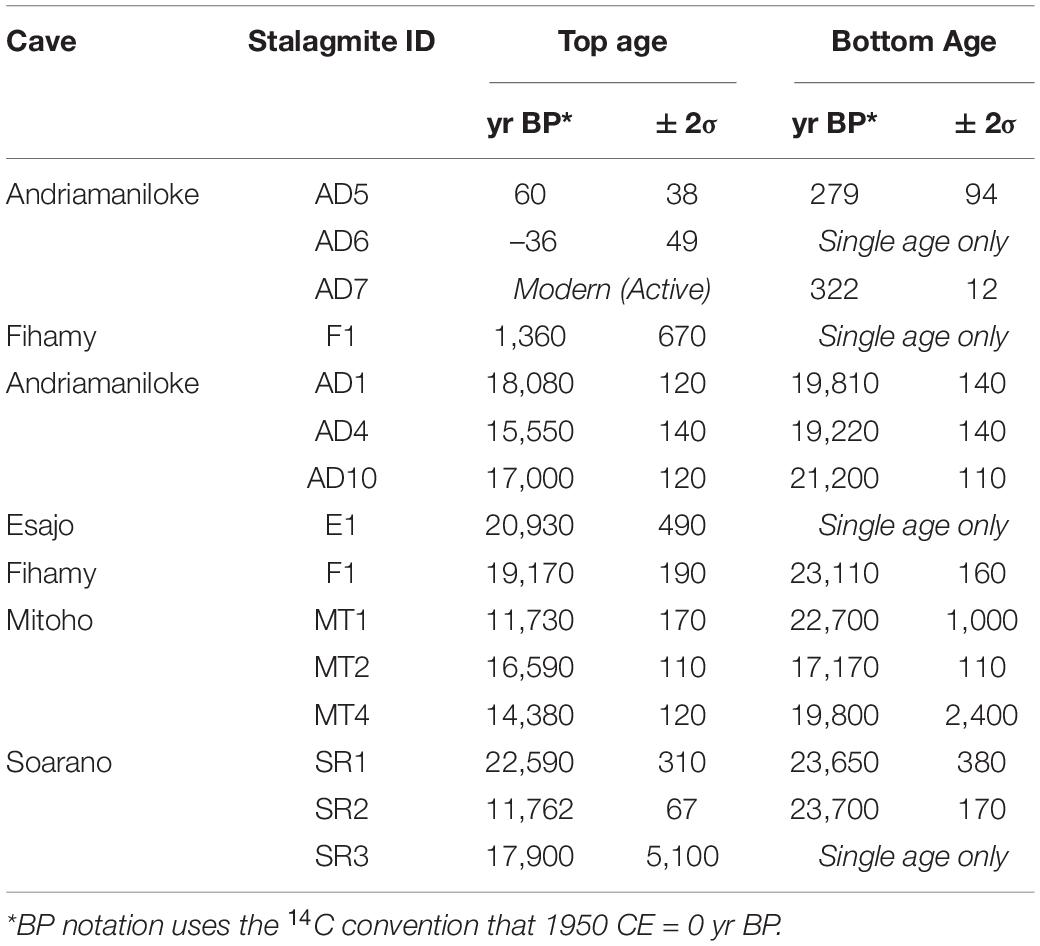
Table 1. Youngest and oldest age determinations for Tsimanampesotse stalagmites that cover the Last Glacial Maximum (which peaked between 22,900 and 19,400 yr BP), the last deglaciation, and the Holocene (beginning 11,700 yr BP).
Other stalagmites collected at Tsimanampesotse covered much earlier time intervals. Together, stalagmites from southwest Madagascar document an alternating wet/dry pattern. The pattern holds for at least the last six summer insolation peaks extending back to 120,000 yr BP and follows the ∼19–23 kyr precession cycle (Figures 2, 3; see text footnote 1). In general, periods of stalagmite growth coincide with local summer insolation above the mean.
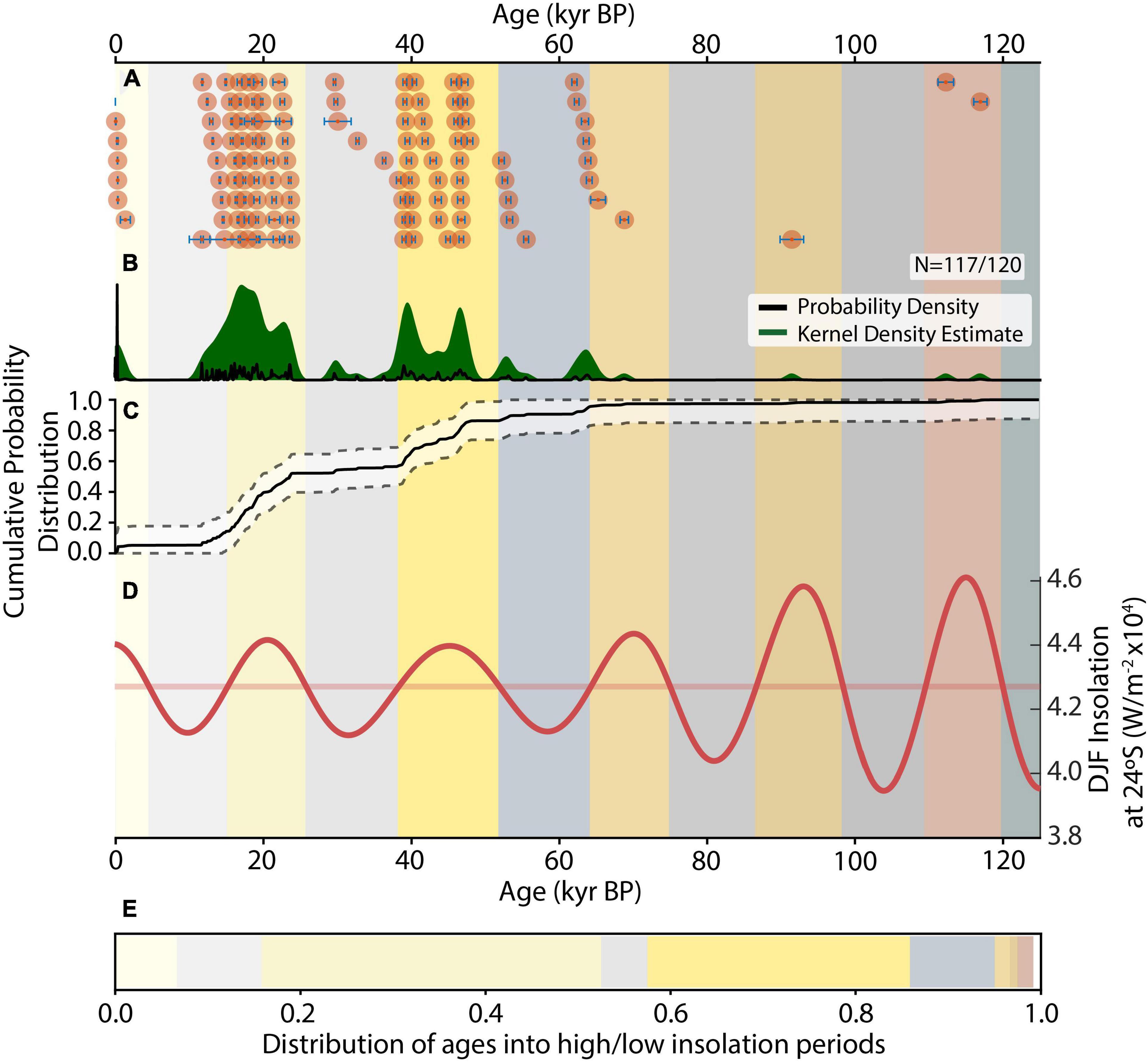
Figure 2. Age distribution of stalagmites from TNP through time. (A) Individual U-Th ages (red dots) and 2σ uncertainty (blue bars) based on 117 of 120 dated samples, (B) Probability density function of age distributions (black line) and kernel density estimate (green shading). (C) Cumulative probability distribution of U-Th age distributions with error. The fluctuating gradient highlights relative periods of excess and deficient speleothem growth relative to the idealized smooth exponential distribution of stalagmite age distributions with time (Scroxton et al., 2016). (D) Integrated summer (December–January–February, or DJF) insolation at 24°S (bold red line), and mean value (pale red line). Vertical color bars indicate periods with higher than mean insolation (yellows, darkening with time), and lower than mean insolation (grays, darkening with time). (E) Distribution of ages into high/low insolation periods following the color scheme above. Probability Density and Kernel Density Estimate curves determined using the detritalPy Python package (Sharman et al., 2018), with a fixed optimized kernel bandwidth and Dvoretsky-Kiefer-Wolfowitz inequality error estimates on the cumulative probability function. Insolation curves from The Climate Data Toolbox for MATLAB (Greene et al., 2019) using the daily insolation function originally by Eisenman and Huybers (Huybers, 2006).
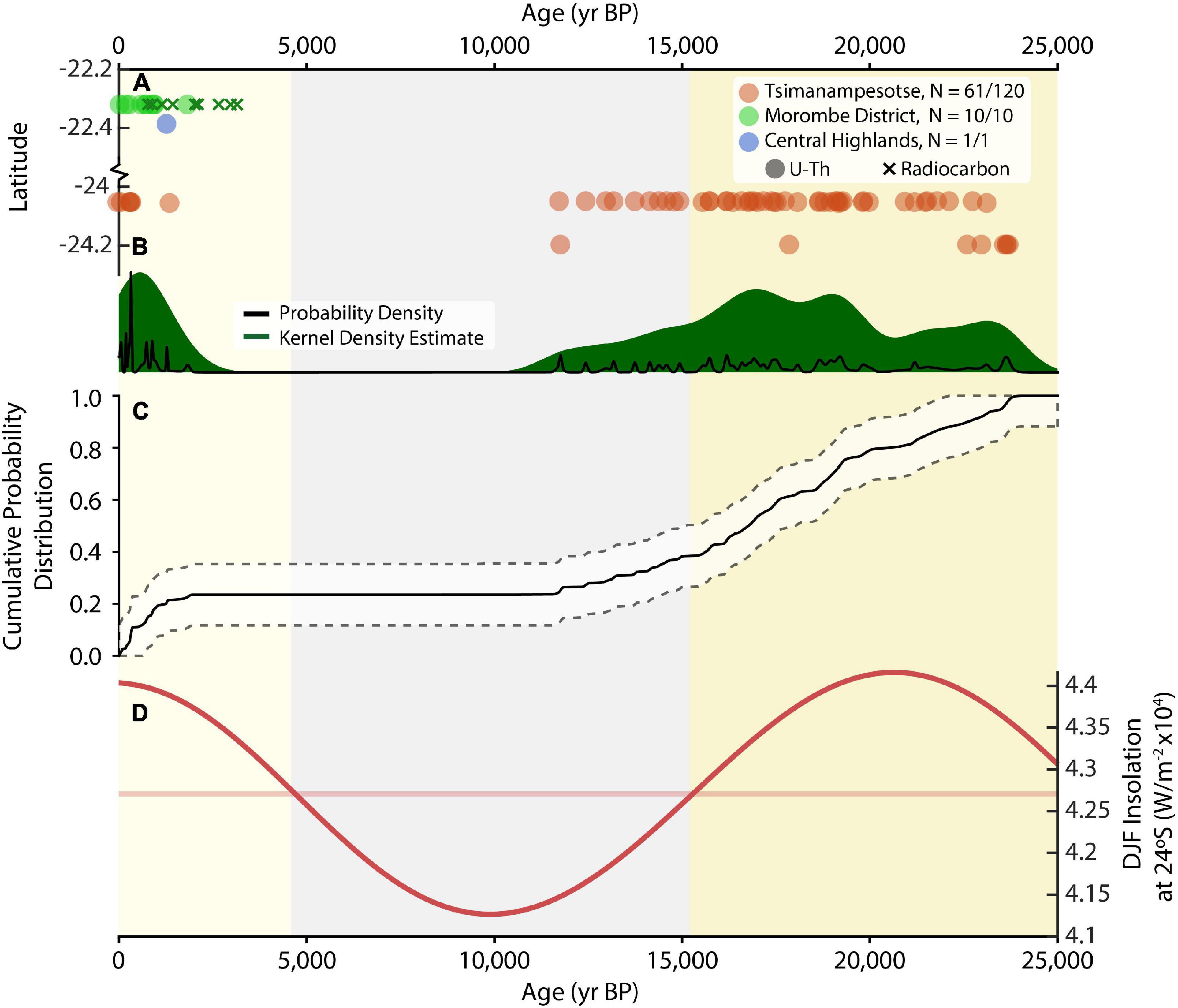
Figure 3. Age distribution of stalagmites from southern Madagascar caves through time (see Figure 1 for map and Table 1 for full cave list). (A) Individual age determinations against latitude from caves within the TNP (red dots, based on 61 of 120 U-Th dated samples), Asafora Cave, Morombe District (green dots, based on all 10 U-Th dated samples, and green crosses based on 9 radiocarbon ages), and Andranomilitsy in the southernmost part of the Central Plateau, near Isalo (blue dot, based on a single U-Th dated sample). (B) Probability density function of age distributions (black line) and kernel density estimate (green shading). (C) Cumulative probability distribution of U-Th age distributions with error. The fluctuating gradient highlights relative periods of excess and deficient speleothem growth relative to the idealized smooth exponential distribution of stalagmite age distributions with time (Scroxton et al., 2016). (D) Integrated summer (DJF) insolation at 24°S (bold red line), and mean value (pale red line). Vertical color bars indicate periods with higher than mean insolation (yellows), and lower than mean insolation (gray).
Our data show that the Holocene falls largely within an interpluvial. From the beginning of the Holocene until only several hundred years ago, we have almost no record of speleothem growth at TNP. The sole exception is a single short record of growth around a millenium ago. This implies that, despite almost-certain fluctuations in the hydroclimate of TNP over the Holocene, annual rainfall in this region was never sufficient to trigger stalagmite growth, likely remaining below ∼300 mm/yr. Southern Madagascar is approaching peak summer insolation once again. Thus, taking sufficient rainfall for stalagmite growth as the measure of pluvial conditions, we infer that southern Madagascar is currently at the beginning of a pluvial period. This is consistent with current average annual rainfall at TNP of 389 mm/year (1981–2017; Goodman et al., 2018), which is above the minimum necessary for stalagmite growth. The current pluvial period should last until summer insolation declines sufficiently, depending on current and future anthropogenic forced changes.
Four stalagmites from TNP (stalagmites F1, AD7, AD6, and AD5) record the beginning of the current pluvial period in this region (Table 1). The first (F1) is from Fihamy Cave. This stalagmite grew through much of the LGM, and stopped growing at 19,172 yr BP. After a long hiatus, growth resumed. Regrowth is represented by a small segment (30 mm) at the top of this stalagmite; a sample from the middle of this small segment is dated to 1360 ± 670 yr BP. The large confidence limit on this date allows us to infer only that this date fell sometime between 2,000 and 700 years ago. More precisely dated speleothem growth occurred during the past millennium at Andriamaniloke Cave: AD5 (279 to 60 yr BP), AD7 (322 yr BP to the present day), and AD6 (modern) (Table 1).
Late Holocene stalagmite growth, and the current pluvial period, likely began earlier to the north and east of TNP and Antsirafaly. At Asafora Cave, speleothem growth began as early as 3500 yr BP (Figure 3; Faina et al., in press). At Andranomilitsy Cave, speleothem growth began by at least 1274 ± 35 yr BP. These dates indicate that the modern north-south rainfall gradient was manifested in the past, with the isohyet that triggers stalagmite growth tracking north to south following the precession cycle.
Age of Cave Flooding Inferred From Top Dates of Submerged Stalagmites, Combined With Submerged Stalagmite Distributions and Depths
At present the cave network at Tsimanampesotse is flooded by a lens of fresh to slightly saline water (typically less than 1500 mg/l total dissolved solids; Rasoloariniaina et al., 2015), with the current water table approximately 10 m above sea level. The freshwater lens extends to at least the deepest explored cave section (>60 m maximum depth at Malazamanga Cave, i.e., over 50 m below sea level). During the last glacial, sea level off southwestern Madagascar would have been approximately 120 m below present day and the caves were subaerially exposed, as evidenced by the abundant speleothem growth in currently flooded sections. As sea level rose during the last deglaciation, the caves would have gradually flooded as the top of the water table intersected them. The timing of flooding may not have perfectly followed global, or local, sea-level curves as the freshwater lens can vary in thickness with the attendant climatic conditions. Nevertheless, stalagmite growth phases, which must be subaerial, can be used to date cave flooding.
Owing to logistical challenges, stalagmites were not recovered from underwater depths greater than 20 m, and the ages and depths of most stalagmites that we did recover from flooded caves do not meet the conditions required to test reasonable last deglaciation flooding hypotheses. However, the analysis of one stalagmite from Vintany, AV2, reveals that, at ∼8.3 kyr BP, the caves were still subaerial at 14 m below the current water table. This depth is important because it is also the depth of the entrance to the fossiliferous vertical chimney at Mitoho that we described above. Prior to its filling with water during the last deglaciation, this chimney would have functioned like a water chute during storms, carrying sediment, bones and carcasses into it from the guano mound, which would have been at that time subaerial. This would explain the concentration of fossils in the horizontal passage at the floor of the vertical chimney and in bends or ledges within the vertical passage, all of which likely predate the flooding of the chimney.
We consider the 8.3 kyr date uncertain due to age reversal of two dates in this stalagmite (hence its exclusion from our overall date compilation), but the date is consistent with inferences drawn from data on global sea-levels, which would have reached –22 masl at 9,000 years ago, –13 masl about 8,000 years ago and –5 masl around 7,000 years ago (Fleming et al., 1998). Therefore, the freshwater lens cannot have been substantially thicker than present in the Early Holocene and the water table in the karst system, and presumably local sea-level, could only have reached present levels at 8.3 kyr BP or afterward.
Subfossils
Taxonomic Identifications
Although separated by only ∼45 km direct distance, the deposits of the Antsirafaly pond and TNP caves show striking differences in faunal composition (Table 2). In total, we found 16 extinct species, eight at Antsirafaly and 13 at Tsimanampesotse, with five species occurring at both sites. At Antsirafaly, we only recovered extinct species. These included three primates (Megaladapis edwardsi, M. madagascariensis, and Archaeolemur majori), two elephant birds (Mullerornis modestus and the largest-bodied species, Vorombe titan), two reptiles (Voay robustus and Aldabrachelys sp.), and a hippo (Hippopotamus lemerlei). Delicate bones of small vertebrates were either not preserved or may have been missed because we did no fine sieving of sediments.
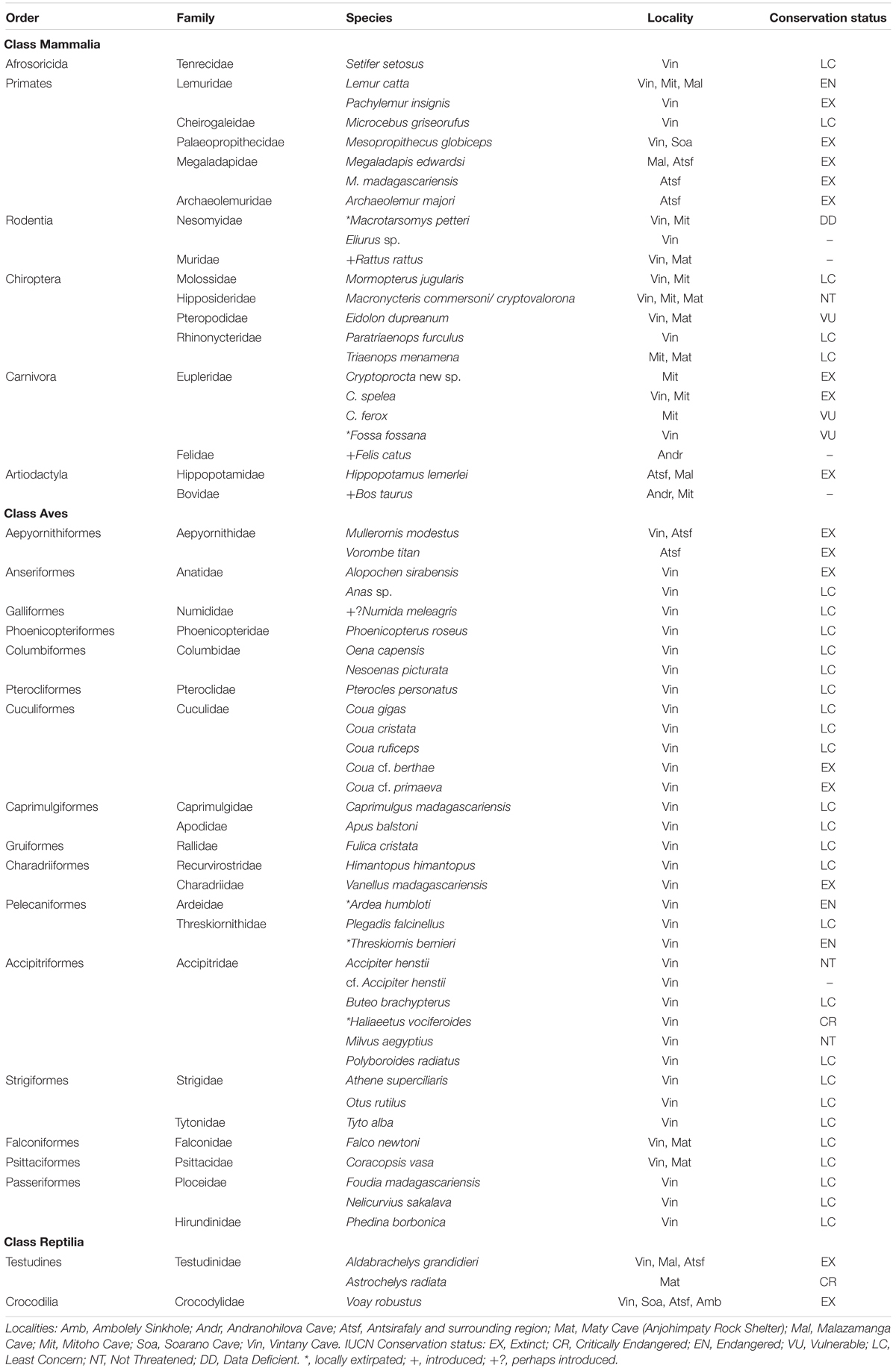
Table 2. Vertebrate taxa found in subfossil deposits at Tsimanampesotse and/or region of Antsirafaly.
At TNP, the extinct species included three primates (Pachylemur insignis, Mesopropithecus globiceps, and Megaladapis edwardsi), two euplerid carnivorans (Cryptoprocta new sp. and C. spelea), an artiodactyl (Hippopotamus lemerlei), an elephant bird (Mullerornis modestus), four volant birds (Alopochen sirabensis, Coua cf. berthae, Coua cf. primaeva, and Vanellus madagascariensis), a tortoise (Aldabrachelys grandidieri), and the horned crocodile (Voay robustus).
Comparing the extinct taxa at Antsirafaly and TNP, the two extinct primate species most common at TNP (Pachylemur insignis and Mesopropithecus globiceps) are notably absent at Antsirafaly. Crocodiles are much more common at the TNP sites than at Antsirafaly. In contrast, the extinct Megaladapis, which is relatively common at Antsirafaly, is extremely rare at TNP. Megaladapis edwardsi is represented by a single skull at Malazamanga Cave and two specimens found at the entrance to Mitoho Cave by MacPhee (1986); M. madagascariensis is absent entirely at TNP. Similarly, Hippopotamus is extremely rare at TNP, being represented by just two (likely associated) specimens from Malazamanga Cave, and found under the Megaladapis skull. Elephant birds are far more common at Antsirafaly than at TNP.
We recovered bones from over 40 endemic extant taxa at TNP (Table 2). Many of these still live within the park, but five are locally extirpated. The latter include a rare and poorly known nesomyid rodent (Macrotarsomys petteri, Petter’s big-footed mouse), a Vulnerable euplerid (Fossa fossana, the fanaloka), and three Endangered or Critically Endangered birds (Ardea humbloti, Humblot’s heron, Threskiornis bernieri, the Madagascar sacred ibis, and Haliaeetus vociferoides, the Madagascar fish-eagle). Fossa fossana subfossils have never previously been collected in the dry west or subarid southwest; this euplerid is restricted today to the humid eastern rainforest and parts of the Central Plateau. Haliaeetus vociferoides is of interest because it would have presumably exploited freshwater fish from Lake Tsimanampesotse, which no longer exist due to the lake’s current salinization (Goodman and Jungers, 2014).
Lastly, we found bones of introduced cats, rats, and cows (Felis catus, Rattus rattus, and Bos taurus) at TNP. MacPhee (1986) reported finding multiple suid specimens (probably Potamochoerus larvatus, the bushpig) at the main entrance to Mitoho Cave and remains of mice (Mus), as well as rats, at Anjohimpaty Rock Shelter.
The Chronology of Change in Faunal Assemblages at Tsimanampesotse and Antsirafaly
Calibrated dates for our animal specimens from TNP range from 2985 ± 285 yr BP to modern (<0 Cal yr BP); those from Antsirafaly range from 1823 ± 73 to 933 ± 133 yr BP. This age range extends back to 4443 ± 208 yr BP if we include an additional single date reported by MacPhee (1986) for elephant bird eggshell. Calibrated dates for plant specimens from Vintany Cave range from 2245 ± 100 to 225 ± 85 yr BP. The more recent plant dates derive from underwater sediments in a “shallow” portion of Vintany with continuing heavy sedimentation today (Figure 4, Localities 1 and 2). The older plant dates come from a “deeper” portion of the cave that is largely (but not entirely) blocked from deposition today, and that currently experiences much slower sedimentation2 (Figure 4, Localities 4 and B, the latter locality also called “Bone Soup” because of the thick, gelatinous quality of the sediment). Because the taxonomic composition of the fauna changes dramatically (described below) from before to after 900 yr BP, we present the dates for individual specimens in two tables: Table 3 for dates earlier than 900 yr BP and Table 4 for more recent specimens. Table 5 presents all Vintany plant dates and one from Malazamanga.
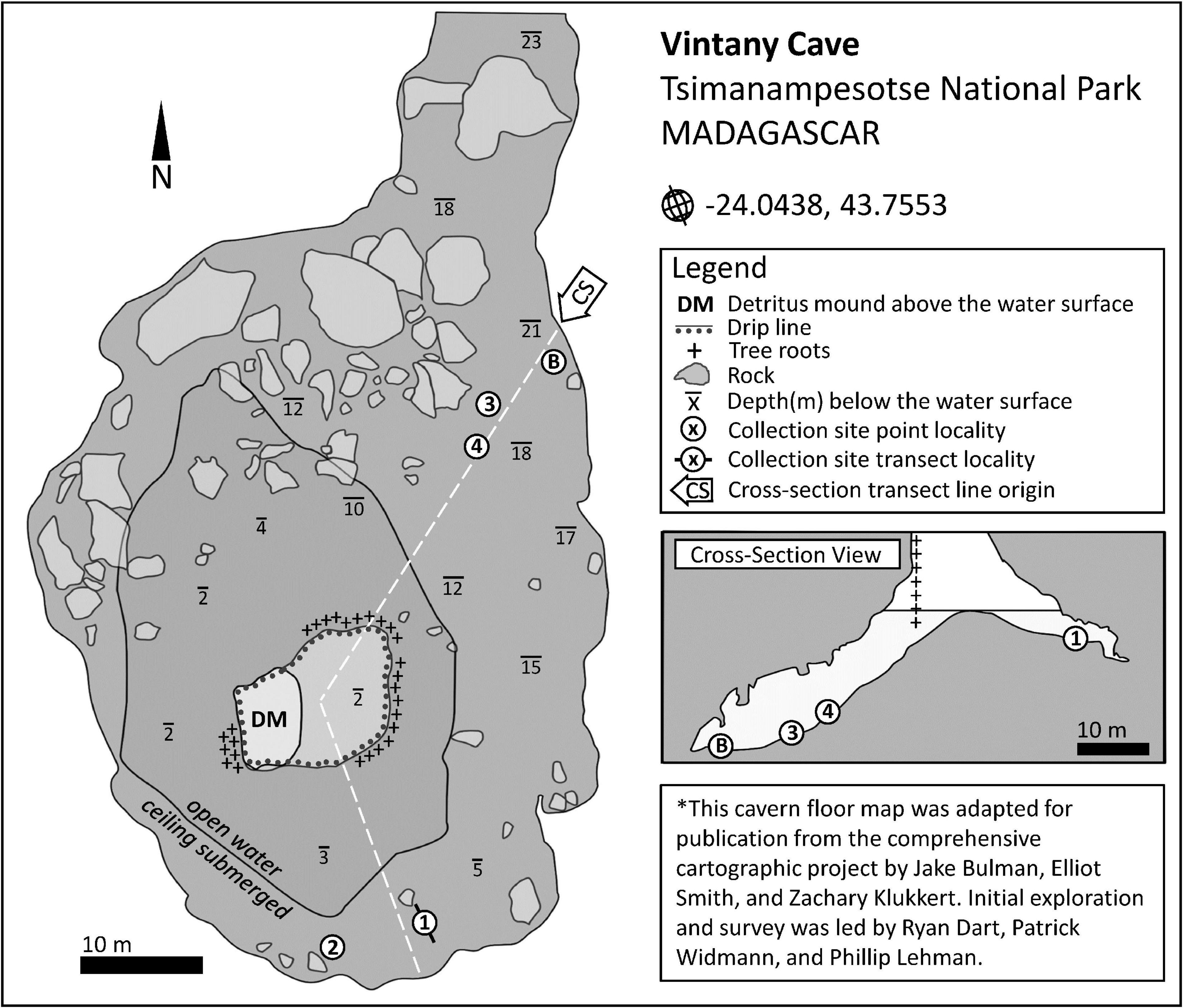
Figure 4. Vintany Cave, showing excavation sites in shallow (Localities 1 and 2) and deep (Localities 3, 4, and B = Bone soup) sections of the cave.
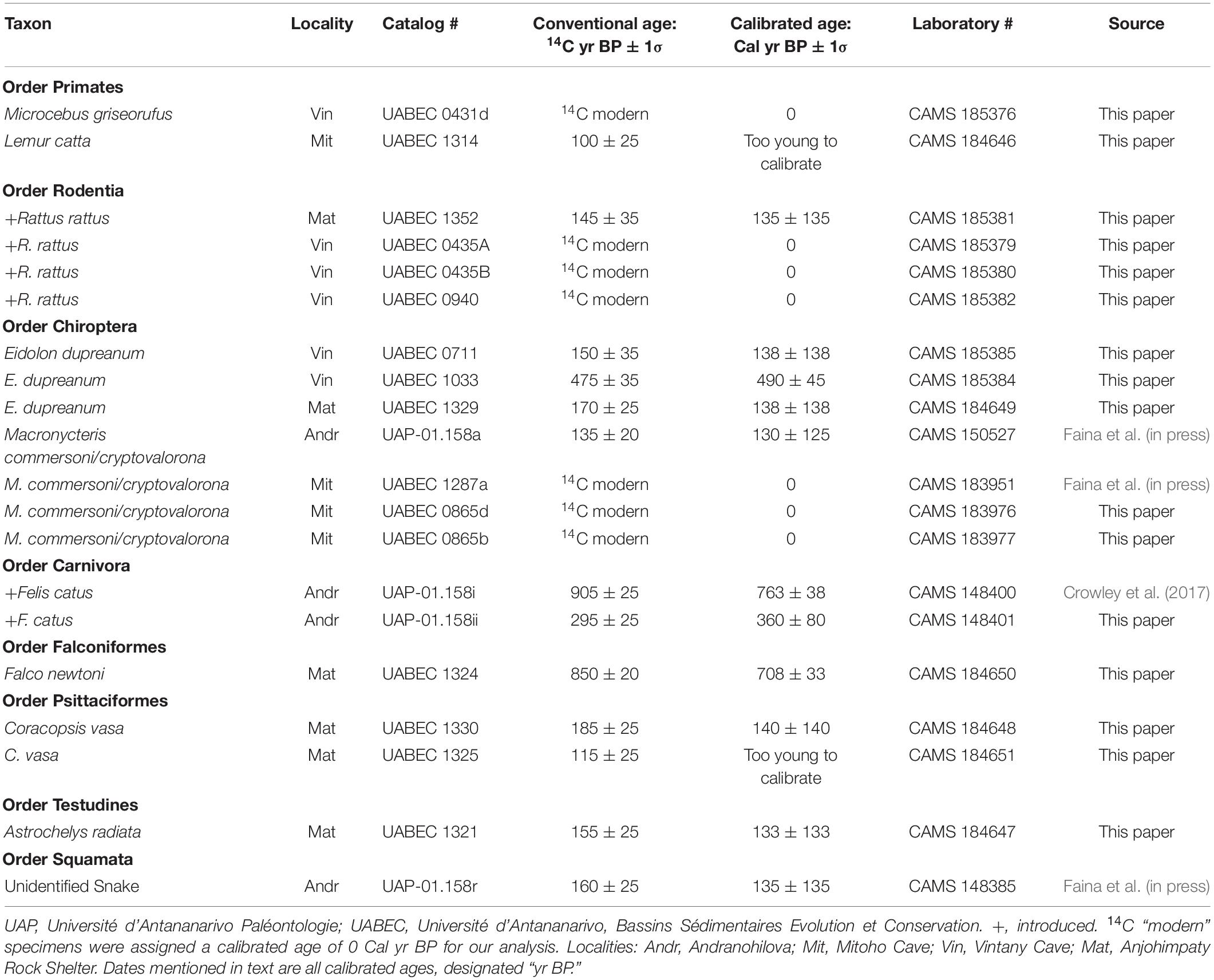
Table 4. Tsimanampesotse/Antsirafaly specimens with conventional and calibrated 14C dates younger than 900 yr BP.
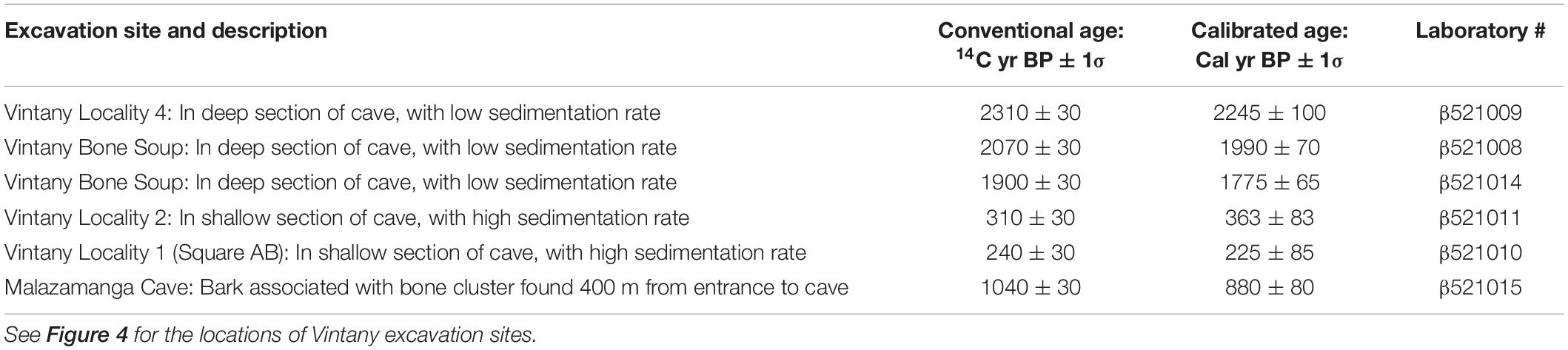
Table 5. Plant material from sediments collected from underwater deposits at Vintany and Malazamanga Caves (Tsimanampesotse National Park), AMS-dated at Beta Analytic Laboratories, FL, United States.
When dated individuals are placed on a timeline displaying their current distributional status (extinct, locally extirpated, local extant, or introduced), the profile of faunal change becomes evident (Figure 5). The TNP vertebrate assemblage shows a dramatic shift in taxonomic composition ∼2,000 years ago and another at ∼900 years ago (Tables 3, 4). Prior to ∼2000 years ago, the assemblage is comprised of species that are today extinct, locally extirpated, and extant in the park. With one possible exception (Numida meleagris; see below), there are no introduced species.
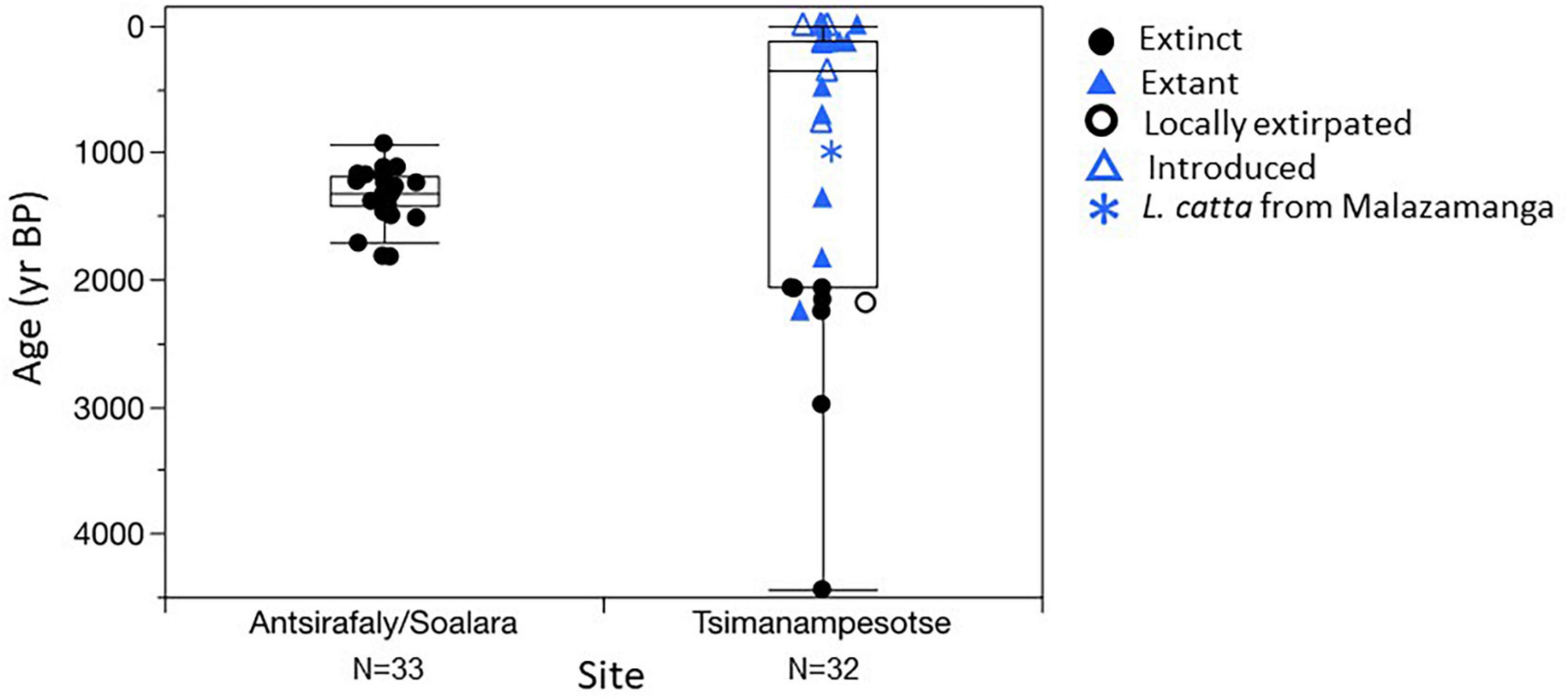
Figure 5. Distribution of calibrated dates for extinct, extant, locally extirpated, and introduced taxa at Antsirafaly and Tsimanampesotse. The date for a humerus of the extant Lemur catta at Malazamanga is highlighted (blue asterisk) because of its possible temporal association with several bones of extinct species (Megaladapis edwardsi, Hippopotamus lemerlei, and Cryptoprocta spelea). See text.
The oldest subfossils we collected at TNP are probably euplerids (Cryptoprocta spelea and a new, much larger species of extinct Cryptoprocta)3 that we found in the vertical chimney at Mitoho Cave. These are most likely individuals that sheltered or made birthing dens within Andranohilova. We have no way of knowing exactly how old these bones may be, or whether they date to the LGM pluvial period. None of the fossils from this location (including bats, Macronycteris commersoni, M. cryptovalorona, and Triaenops menamena) could be directly dated; they were all brittle and lacked organic material. As the fossils in the chimney of Mitoho Cave were found between 20 and 32 m below the present water table, we conclude that they were deposited prior to cave flooding (i.e., when the subaerial chimney would have functioned like a water chute during storms). They are likely older than 8,300 yr BP and almost certainly older than 7,000 yr BP.
The next oldest date is from a piece of elephant bird eggshell (likely Mullerornis) found by MacPhee (1986) at the main entrance to Mitoho Cave (Table 3). Only slightly younger are a series of radiocarbon dates for other TNP extinct species (Pachylemur insignis, Coua cf. primaeva, and Voay robustus), as well as the locally extirpated Macrotarsomys petteri, all falling between 3,000 and 2,000 years ago. We can infer similar ages for additional extinct species (Mesopropithecus globiceps, Coua cf. berthae, and Mullerornis modestus) on the basis of depositional association. Specimens belonging to these three species were retrieved from the locality in the deep portion of Vintany Cave known as “Bone Soup” (Figure 4, site B). Present in the same deposit are specimens of Voay robustus and Pachylemur insignis, both of which are known to have survived at TNP until ∼2,000 years ago or later based on dated specimens at other Vintany localities and at another cave within the park. At Bone Soup we found associated bones of a single Pachylemur along with mixed remains of individuals belonging to other extinct species, as well as plant material. None of the bones from Bone Soup were directly datable, but plants from this deposit yielded calibrated dates of 1990 ± 70 and 1775 ± 65 yr BP. Given that these sediments appeared to be little disturbed, one might infer that the entire Bone Soup assemblage could have been slightly under 2,000 years old.
All TNP vertebrate dates between 2000 and 900 yr BP belong to smaller-bodied, endemic species, such as extant lemurs or bats. However, there is some indirect evidence that Megaladapis edwardsi, Hippopotamus lemerlei, and Cryptoprocta spelea were still present at TNP around 1,000 years ago. As mentioned above, divers found these bones in a cluster far from the entrance to Malazamanga Cave. Most were buried in sediment but an undamaged skull of a Megaladapis edwardsi was exposed on the cave floor. We did not sample these bones for dating, but we did obtain radiocarbon dates for a piece of bark (880 ± 80 yr BP) (Table 5) and a Lemur catta humerus (998 ± 68 yr BP) (Table 3), both of which were found directly below the Megaladapis skull. The overlapping confidence limits of these dates suggest that subfossils and other debris accumulated at this location over a short window of time, most likely through a fissure in the ceiling that was only open for a limited period. This bone cluster is unique in that this was the only place at Malazamanga where bones and other debris from outside were found. Furthermore, the bones showed no evidence of abrasion through water transport. The taxonomic composition of this cluster of bones is strikingly different from that of all other subfossil assemblages at TNP. Pachylemur and Mesopropithecus, common at nearby Vintany Cave, are both notably absent at Malazamanga. With the exception of C. spelea, the extinct species found in the bone cluster at Malazamanga are all also present at Antsirafaly until nearly 1,000 years ago, so survival of these particular species in the region until the beginning of the second millenium is not unexpected.
Finally, after 900 yr BP at TNP, we begin to see introduced taxa (e.g., cats, rats, and cows) alongside endemic extant species (Table 4). The date range for bones of introduced taxa at TNP is 763 ± 38 yr BP to modern. The oldest are cat bones from Andranohilova. Cow bone was also present at Andranohilova, but we failed to recover collagen from a specimen that we treated for dating, and thus cannot exclude the possibility that cattle were present prior to 900 yr BP. We found many rat bones at the Anjohimpaty Rock Shelter and in both shallow and deep sections of Vintany Cave, but always exposed on the floor of the flooded cave rather than within the sediments. Most of the Rattus specimens we dated are radiocarbon-modern, and none is older than ∼200 years, suggesting recent colonization of natural environments of the southwest.
At Antsirafaly, we see a very different profile of faunal change (Figure 5). There was no early first millenium CE crash; instead, the subfossil record ends abruptly ∼1,000 years later, near the end of the first millenium but before the appearance of introduced taxa at TNP (Table 3). Only one dated specimen (an Archaeolemur majori) was younger than 1118 yr BP (933 ± 133 yr BP), suggesting that, not long after 1100 yr BP, the Antsirafaly pond dried up or became too muddy to support local animals seeking fresh water. We found no bones at this site older than 1823 yr BP; this may simply mean that we did not dig sufficiently deep below the current water table to expose older specimens. However, sites such as Ampoza with similar depositional profiles, have never yielded additional fossils from below the fossiliferous layer despite continued excavation. Hippopotamus lemerlei, Mullerornis modestus, Megaladapis edwardsi, and M. madagascariensis were present at Antsirafaly through much of the first millenium CE. We have few dates for Vorombe titan and Archaeolemur majori, but the dates we have, with the exception of the single dated A. majori mentioned above, fall within the temporal range of the other species. Giant tortoises (Aldabrachelys sp.) and horned crocodile (Voay robustus) bones from the same deposits were not dated. We found no bones of introduced domesticated species at Antsirafaly, suggesting that these animals may have arrived at this locality after the pond dried up.
Evidence of Human Presence in the Region of TNP
Neither TNP nor Antsirafaly was an early human settlement site, nor did we find any evidence at either of large-vertebrate butchery. MacPhee (1986) reported a scattering of elephant bird eggshell on the talus below Anjohimpaty, which may suggest that people used elephant bird eggs to carry water to this site, but this could have happened long after elephant birds were locally extirpated. However, there are clear signs of human presence, minimally over the past ∼800 years, derived from introduced taxa. Our two calibrated radiocarbon dates for introduced Felis catus from Andranohilova (763 ± 38 and 360 ± 80 yr BP; Table 4) span much of the past millenium. They suggest that feral cats at least occasionally used Andranohilova. This in turn may suggest that Cryptoprocta spelea were rarely sheltering in the cave or absent entirely during this period. Modern introduced carnivorans often displace native carnivores (Farris et al., 2015), but cats are much smaller in body size than C. spelea and likely would have been displaced by them. The still-extant C. ferox is smaller than C. spelea and still lives in the park.
We found specimens of Numida meleagris (helmeted guineafowl, long assumed to be introduced to Madagascar) in association with nearly 2,000-year-old plant remains and over 2,000-year-old subfossil Pachylemur within the sediments of the deep-section of Vintany Cave (Localities Bone Soup and 4). Goodman et al. (2013) reported guineafowl at Ankilitelo in southwest Madagascar over 13,000 years ago, raising the possibility that this species colonized Madagascar from Africa prior to, and independently of, the initial colonization of the island by humans (but see Hansford et al., 2018; Godfrey et al., 2019). Our discovery of Numida in 2,000-year-old deposits at Vintany provides indirect support for the prolonged presence of guineafowl in southwestern Madagascar, but not necessarily for human presence at Tsimanampesotse 2,000 years ago. This is because helmeted guineafowl may have colonized Madagascar independently of humans (Goodman et al., 2013), and because, even if they were introduced by humans over 13,000 years ago, we cannot assume that the presence of feral Numida at any one locality thousands of years later indicates the local presence of humans.
Cave art (including two graffiti messages using the Roman alphabet and two anthropomorphic figures) at Anjohimpaty Rock Shelter covers much of the northern wall of the shelter (Figures 6–8). Both anthropomorphic figures (a possible solitary sorcerer figure, and a very faint combination of seven figures in ‘human-pyramid’ formation, four in a bottom layer, two in a middle layer, and one on top) show images with down-curved arms. The solitary figure has a pointed head and bowed legs. Finally, at the eastern end of the wall, there is a second graffiti message located above a small cavity where the wall meets the floor (Figure 8).
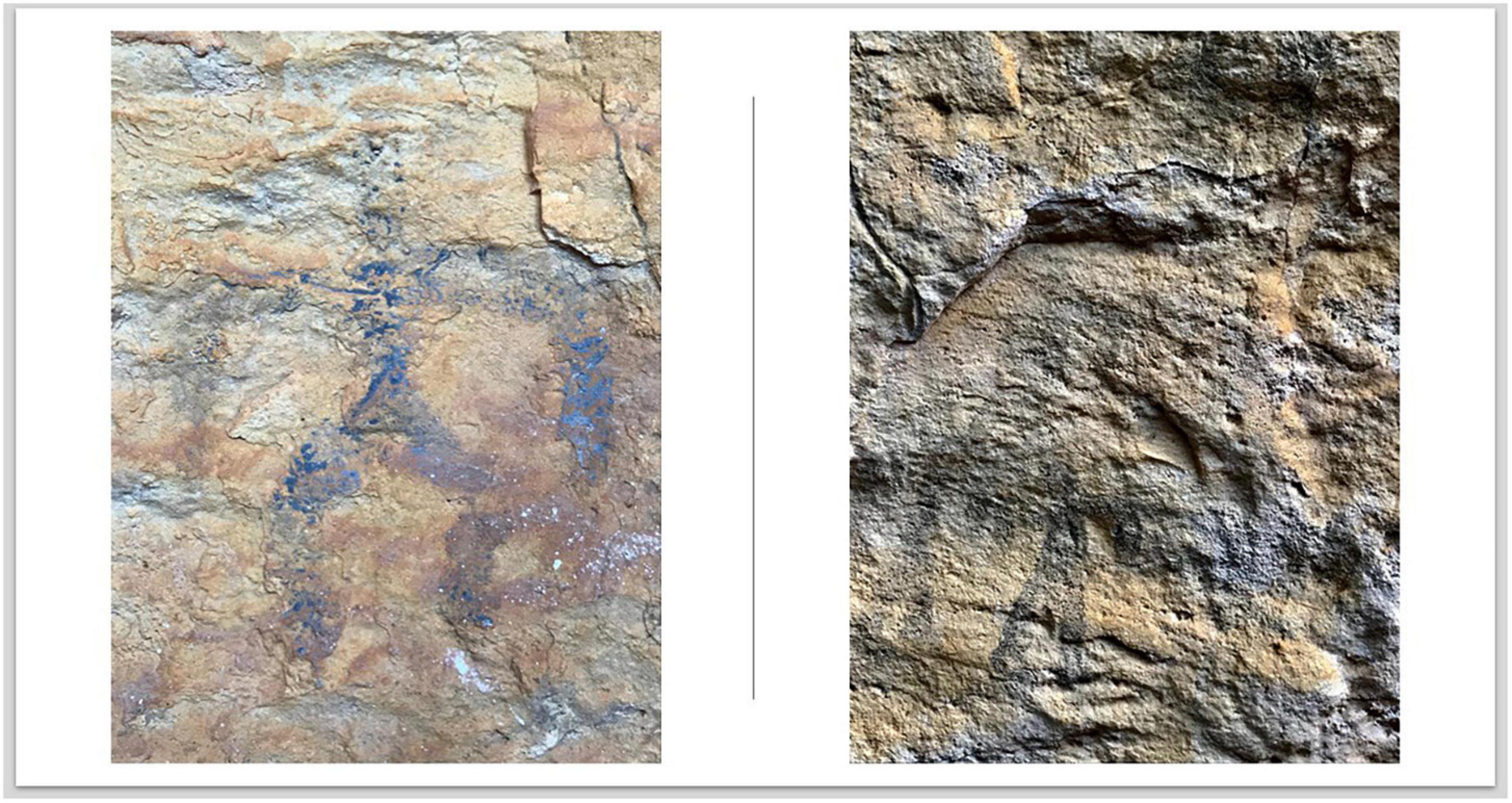
Figure 7. Possible anthropomorph (left) and human pyramid (right) figures on northern wall at Anjohimpaty Rock Shelter.
The graffiti depicted in Figure 6 dates from 1934. It was written by a Malagasy person using the local Mahafale (primarily) and Central Highlands Merina dialects. Although some of the writing is faded, and although characters may be missing, the general message can be deciphered. The first line, TZI∗NBANA (where the asterisk represents a likely missing letter), can be read as ‘tsy ombàna.’ This is a combination of the Mahafale expression ‘tsy ombà’ (no trespassing) and the Merina suffix ‘-na.’ Mahafale people would not use the suffix ‘-na’ unless they are trying to communicate with Merina people. This suggests that the person who wrote this inscription was a local Mahafale person (hence the use of ‘tsy ombà’) who interacted with either a Merina person (from the Central Highlands) or a foreigner (vazaha) who spoke the Merina language (hence the use of the suffix ‘na’). The next line appears to read ‘10 MINTOHO.’ If, instead of the number 10, the inscriber intended to write ‘IO,’ the first word would mean ‘here’ in the Mahafale dialect. MINTOHO is an alternative spelling of the name of the cave. Taken together, this becomes ‘this cave, Mitoho.’ The next line reads 1934 TANITIVAZA. 1934 can be easily interpreted as the date the graffiti was written. In the Mahafale dialect, ‘TANITIVAZA’ should read ‘tani’ty vazaha’ or ‘tane’ty vazaha’ which means ‘vazaha’s land’ (foreigner’s land). The next portion of this inscription (1934 FA TZIVAZA) could have been written by a second person as a reply to the first person (which might explain the repetition of the date, 1934), or by the first person replying to his or her rhetorical question. ‘FA’ means ‘but’ in the Merina dialect (‘fe’ in Mahafale), but ‘fa’ means ‘already’ in Mahafale, and together with the negation ‘tsy,’ it means ‘no longer.’ Thus, ‘Fa tsy vazaha’ means ‘it is no longer vazaha’s’ in Mahafale.
The second graffiti on the eastern end of the northern wall of the shelter appears to be a solitary word, I?)BERTEM(T?Y?I?)OK. It was written above a small arched inner depression, 1 m from the floor of the main shelter (Figure 8). This inscription does not resemble Mahafale or any other dialect of Malagasy. We cannot exclude the possibility that it is misspelled Mahafale, or the name of a person – perhaps of someone buried there. There is no reason to believe that the anthropomorphic images or the two graffiti messages are related to one another. Whereas both messages appear to be recent, the anthropomorphic images are faint and may be older. There is evidence of additional, very faint, writing. The Anjohimpaty Rock Shelter wall may have been used by people over an extended period, but at present, we have no definitive indication of great antiquity for any particular image or inscription.
Discussion
Is Today’s Hydroclimate in Southwestern Madagascar Significantly Drier Than the Hydroclimate of Several Thousand Years Ago, When There Were Numerous Large-Bodied Endemic Vertebrate Species at TNP?
On the basis of evidence presented herein, we conclude that today’s hydroclimate in southwest Madagascar is not significantly drier than the hydroclimate of several thousand years ago, when there were numerous large-bodied endemic vertebrate species in this region. To the contrary, our analysis of 23 stalagmites from TNP shows that modern mean annual rainfall at this locality is higher than it was throughout most of the Holocene. Low rainfall would have characterized extended periods during which large-bodied vertebrates thrived in the region, including between 3,000 and 2,000 years ago at TNP and between 2000 and ∼1000 yr BP at Antsirafaly. This suggests that the large-bodied vertebrates at TNP and Antsirafaly, like so many plants of the dry southwest (spiny thicket and succulent woodland ecoregions), were likely drought-tolerant.
The hydroclimate of southern Madagascar was characterized by alternating wetter and drier periods. On a coarse scale there were pluvials and interpluvials, each lasting around 10,000 years, but each in turn was characterized by climate fluctuations on a finer, centennial scale. One such drought occurred within the current pluvial, as revealed by a study of stable isotopes in one of our Asafora stalagmites, AF2 (Faina et al., in press). An analysis of stalagmite AF2 indicates a relatively wet period at its base (∼3,500 yr BP) with dominant C3 vegetation. This corresponds to the beginning of the current pluvial at this location. At 3350 yr BP, rainfall began to decline slowly until 1680 yr BP, at which point the decline accelerated markedly. Full drought conditions began ∼1560 yr BP and continued until ∼880 yr BP, after which wetter conditions resumed. Within this 700-year drought, there were fluctuations in both rainfall and vegetation reflected in the δ18O isotope records and the δ13C records respectively. These correlated trends suggest a coupling of changes in rainfall amount and ground vegetation from dominant C3 to higher representation of CAM and C4 vegetation (i.e., succulent plants and grasses) and back. Drought conditions at Asafora were most severe between 1100 and 960 yr BP. At TNP, after 880 yr BP, there was likely a gradual increase in rainfall until the threshold of speleothem growth was reached (perhaps as recently as several hundred years ago).
What Triggered Local Extirpation of Species at Tsimanampesotse and Antsirafaly?
We have identified two episodes of local extirpation of a wide variety of vertebrate species that are now extinct: one at TNP (near the beginning of the first millenium CE, i.e., 2,000 years ago) and one at Antsirafaly (near the end of the first millenium CE, i.e., 1,000 years ago). The two were not synchronous, and neither can be linked to human activities. Instead, they appear to be related to climate change. However, a third event clearly related to human activities, i.e., the local arrival of introduced vertebrates in association with the spread across Madagascar of agropastoralism, impacted the southwest not long after the second extirpation event. We will return to this third event later.
At Antsirafaly, a temporal link between peak drought conditions and local vertebrate extirpation can be defended on the grounds that aridification likely caused the small pond at this site to shrink and then disappear entirely. The mean for last-known occurrences for the six dated extinct species at Antsirafaly is 1146 yr BP (range from 1368 ± 53 to 933 ± 133 yr BP; Table 3); this mean closely matches the beginning of peak drought at Asafora. Large vertebrates such as hippopotamuses and elephant birds likely simply shifted to nearby bodies of water as smaller ones dried. We do not know the extent to which the drought affected water bodies across the region. Hansford et al. (2021) estimate the time of disappearance of hippopotamuses and elephant birds at other sites in the spiny thicket at between 957 and 911 yr BP.
At TNP, the link between vertebrate species extirpation and arid climate is more tenuous. A lot depends on the precise timing of extirpation at this site: did it begin around 2000 yr BP or several centuries later? Given that the vertebrates living in southwestern Madagascar through the Holocene were drought-tolerant, one might expect that only extreme climate perturbations or large-scale changes in underground freshwater sources would have forced local extirpation. As we have discussed above, radiocarbon dates suggest that local extirpation of Pachylemur, Mesopropithecus, and other large vertebrate species at TNP occurred shortly after 2,000 years ago, but dates for plants in apparent association with bones of these same vertebrate species suggest that they may have persisted a bit longer. We know from stalagmite records at both TNP and Asafora Cave that the early first millenium was dry at TNP, but it is not clear that it would have been exceptionally dry at around 2,000 yr BP.
One could make a stronger case for aridity being the trigger for vertebrate decline at TNP if vertebrate decline did not commence until around 1700 yr BP or later. As described above, Asafora stalagmite AF2 recorded a sharp decrease in rainfall beginning 1680 yr BP, with drought conditions manifested by 1560 yr BP (Faina et al., in press). Simultaneously, Lake Ihotry, which is located ∼55 km NE of Asafora Cave, underwent salinization beginning around 1600 years ago (Vallet-Coulomb et al., 2006). We know, on the basis of old strand lines and deltaic sediments, that Lake Tsimanampesotse was once of much greater size and depth than it is today (Loewen et al., 2001), and we know on the basis of freshwater mollusks along the old strand lines and waterfowl bones in subfossil deposits that this was once a freshwater lake (Perrier de la Bâthie, 1934; Petit, 1935; Goodman and Jungers, 2014; Rasolonjatovo et al., in press). Assuming that change in local hydroclimate was the primary driver of lake salinization at Tsimanampesotse, Faina et al. (in press) hypothesized that lake salinization may have begun around 1600 years ago at this site (as at Lake Ihotry), coeval with the beginning of the late first millenium CE drought. Lake salinization has been suggested as a proximate cause of local extirpation of freshwater birds at TNP (Goodman and Jungers, 2014; Rasolonjatovo et al., in press).
There are three potential controls on lake size, salinity, and water availability, however, in addition to local rainfall. These are: (1) Changes in rainfall patterns inland impacting groundwater flow through the resurgences and into the lake (Guyot, 2002; Goodman and Jungers, 2014); (2) Geomorphological changes in the capture of overland streams draining to the lake (Loewen et al., 2001); and (3) Changes in evaporation from the lake surface. Only with new coring and detailed study of lake sediments will we be able to piece this all together for TNP.
Guyot (2002) found that inland water sources have direct underground links to the resurgent-artesian water sources at the western foot of the Mahafaly Plateau. These changes may have affected the gallery forest along the resurgent stream margins and the sources of freshwater that fed into Lake Tsimanampesotse and sites such as the ponds and marshes in the region of Antsirafaly. For variation in lake level during the Middle and Late Holocene (once sea levels were high and stable), groundwater flow from inland rainfall is a plausible control on lake level fluctuations. However, the magnitude of such change would likely have been small compared to the influence of sea-level change between glacial and interglacial periods. We consider it unlikely that Lake Tsimanampesotse could have been fed by groundwater resurgences during the last glacial period while the nearby caves remained subaerial, as evidenced by speleothem growth. Local and regional geomorphological changes remain unstudied in the area, preventing greater assessment of their potential impact. Finally, any significant changes in evaporation would have likely followed glacial/interglacial changes in temperature (and therefore have been correlated with sea-level via global climatic change) and/or orbital scale changes in local precipitation.
We emphasize that the driver of early vertebrate extirpation at Tsimanampesotse, excluding freshwater birds, could not have been lake salinization alone. Salinization at Lake Tsimanampesotse would have had minimal effect on species that could easily access the fresh water at sinkholes on the karst, including primates. Lemur catta drinks fresh water from sinkholes at Tsimanampesotse today (Gould et al., 1999); its close relative, Pachylemur, probably did the same. Others could have avoided drinking water entirely. Mesopropithecus globiceps was a palaeopropithecid that, like its close extant relatives, the indriids, likely obtained all the water they required from the foods it consumed (see, for example, Semel et al., 2019, on Propithecus from the eastern rainforest; similar behaviors are observed for Propithecus in much drier habitats). For primates at TNP, the more critical resources may have been large trees offering preferred foods, nesting sites or protection from large predatory euplerids. At Tsimanampesotse today, big trees and cliff faces serve to protect the extant lemurid, Lemur catta, from the extant fosa, Cryptoprocta ferox (Sauther et al., 2013). Morphological evidence supports the inference that C. spelea was, like its living congener (e.g., Lührs and Dammhahn, 2010; Lührs and Kappeler, 2014), quite capable of climbing trees and hunting on high branches (see text footnote 3), but lemurs may nevertheless use the highest canopy branches of the tallest trees for sleeping or nesting in an attempt to avoid euplerid predation (Sauther et al., 2013; Vasey and Godfrey, in press). Virah-Sawmy et al. (2016) reported an abrupt decline of Pandanus trees beginning at 1574 yr BP at Ambolisatra, followed by gradual disappearance of Pandanus and other large trees, including palms, over the next ∼600 years, and ending with the local extirpation of these plants. Regardless of whether vertebrate extirpation at TNP began 2,000 years ago or several centuries later, and regardless of specific proximate triggers, we can assert with some confidence that none of the species that disappeared from TNP early in the first millenium CE were threatened with broader regional extinction at that time. Pachylemur insignis, for example, is known to have survived in the southwest for another thousand years. There is no reason to believe that it would have disappeared were it not for events that occurred 1,000 years later. We will return to this question below.
There is also no evidence that the large vertebrates at Antsirafaly were adversely affected by dry conditions of the early- or mid- first millenium CE. Hippopotamuses, elephant birds, large-bodied folivorous primates such as Megaladapis, and semiterrestrial generalists such as Archaeolemur, all larger than 15 kg, were apparently more drought-tolerant than Pachylemur, Mesopropithecus, and other species that disappeared early from TNP. Drought tolerance in Megaladapis edwardsi and M. madagascariensis may seem surprising as these animals have clear tree climbing adaptations and have been considered by some to have shunned more open canopy forests. However, we know that Megaladapis, like Palaeopropithecus and other highly arboreal giant lemurs, were victims of crocodiles (Meador et al., 2019). They must have regularly descended to the ground to cross open spaces or to visit waterholes. Megaladapis often occurred at subfossil sites in the southwest in association with Archaeolemur, and to the near exclusion of Pachylemur (Vuillaume-Randriamanantena, 1982). This holds for coastal dune sites with interdunal pools, lakes, or marshes (e.g., Beloha Anavoha, Beavoha, Ambolisatra, and Manombo-Toliara).
Neither Antsirafaly nor TNP shows evidence that humans triggered local vertebrate extirpation. Indeed, it is possible that no people arrived in the region until after the beginning of the second millenium CE, when we know of their proximity through the presence of introduced taxa at TNP. We found no evidence of megafaunal butchery at TNP or Antsirafaly, despite the fact that megafaunal butchery has been documented elsewhere on the southwest coast during the first millenium CE (Hansford et al., 2018).4
Some of the art at Anjohimpaty Rock Shelter may bear testimony to early human presence at TNP, but the graffiti that we documented above is almost certainly recent. The age of the single word on the eastern end of the northern wall of the cave is uncertain, but the inscription regarding foreign versus Malagasy ownership of Mitoho Cave includes a date (1934), and indeed can be understood within the context of events of the 1920s and 1930s. The reserve at Tsimanampesotse was initially established in 1927 under the control of the Muséum National d’Histoire Naturelle in Paris. Henri Perrier de la Bâthie and Georges Petit were two French scientists who surveyed the local wildlife in the early 1930s; both were also complicit in the colonial French campaign to eradicate the prickly pear cactus (Opuntia) from the region of Toliara using cochineal insects that parasitize the plant. Opuntia was and continues to be an important source of food for both people and cattle (Kaufmann, 2004). The eradication policy had disastrous (both intended and unintended) consequences, affecting the human population of most of southern Madagascar (Middleton, 1999; Kaufmann, 2008). That population had increased to significant size by the early 1900s, but the great famine, precipitated by the eradication of Opuntia, resulted in mass starvation and death. For example, in the year 1931 alone, a single district (Tsihombe) of the extreme south was estimated to have lost half of its human population (around 30,000 people; Middleton, 1999). The toll on cattle was said to be much worse. Local anger at Tsimanampesotse over actions of foreigners is easy to understand.
People today do hunt wild animals and similar practices in the past may not have left clear traces. For example, perishable artifacts for trapping bats are sometimes left inside Andranohilova Cave, where the highly sexually dimorphic modern Macronycteris bats are hunted during the season of scarce resources. Bat skulls collected from the submerged guano mound under the active bat roost at Mitoho Cave (dating to as old as 1360 ± 50 yr BP) differ cranially from modern individuals roosting at the same cave. Variation in body size and cranial shape in Macronycteris spp. is correlated with variation in rainfall across western Madagascar (Ranivo and Goodman, 2007; Alumbaugh et al., 2021). The degree to which variation in the skulls of the guano mound Macronycteris bats reflects recent changes in local habitat or recent human activities, such as predation, is currently under study. At present, it appears highly likely that such changes occurred during the second millenium CE.
In summary, aridification remains the most likely explanation for local vertebrate extirpation at TNP and Antsirafaly, but the timing of the two and the proximate causes of extirpation at each differed. In the case of TNP, early local extirpation appears to have resulted from a combination of lake salinization and the loss or decline of tall (likely open canopy) tree species. Much later, at Antsirafaly, aridification appears to have resulted in the drying of the pond we sampled, although we do not know the extent to which other bodies of water in the surrounding wetlands were affected. Habitat changes in the southwest were likely influenced by regional variation in hydroclimate, but perhaps also by shifts in rainfall patterns in the interior to the east of the Mahafaly Plateau that impacted the underground water system and resurged in the immediate vicinity. Two questions remain: first, how do these local extirpation events relate to regional vertebrate decline, and second, what caused so many vertebrate species to go extinct?
How Does the Local Extirpation of Vertebrate Species at Tsimanampesotse and Antsirafaly Relate to Their Regional Decline at Other Sites in Southern Madagascar?
To place local extirpation events at TNP and Antsirafaly within a broader geographic context, we can examine patterns of species decline for now-extinct vertebrate taxa with rich regional radiocarbon records. Of the 16 extinct species we identified from these sites, seven (Hippopotamus lemerlei, Pachylemur insignis, Archaeolemur majori, Megaladapis edwardsi, M. madagascariensis, Mullerornis modestus, and Aldabrachelys sp.) are represented by more than 10 dated specimens from the spiny thicket and succulent woodland ecoregions (Table 6). An eighth species (Voay robustus) has a poor regional radiocarbon record but is richly represented in the recent lore and ethnohistorical records. There is strong evidence (either directly from radiocarbon dates or indirectly from local traditional knowledge and ethnohistoric records) that all eight of these species survived in spiny thicket or succulent woodland ecoregions well into the first millenium CE or beyond (Table 6).
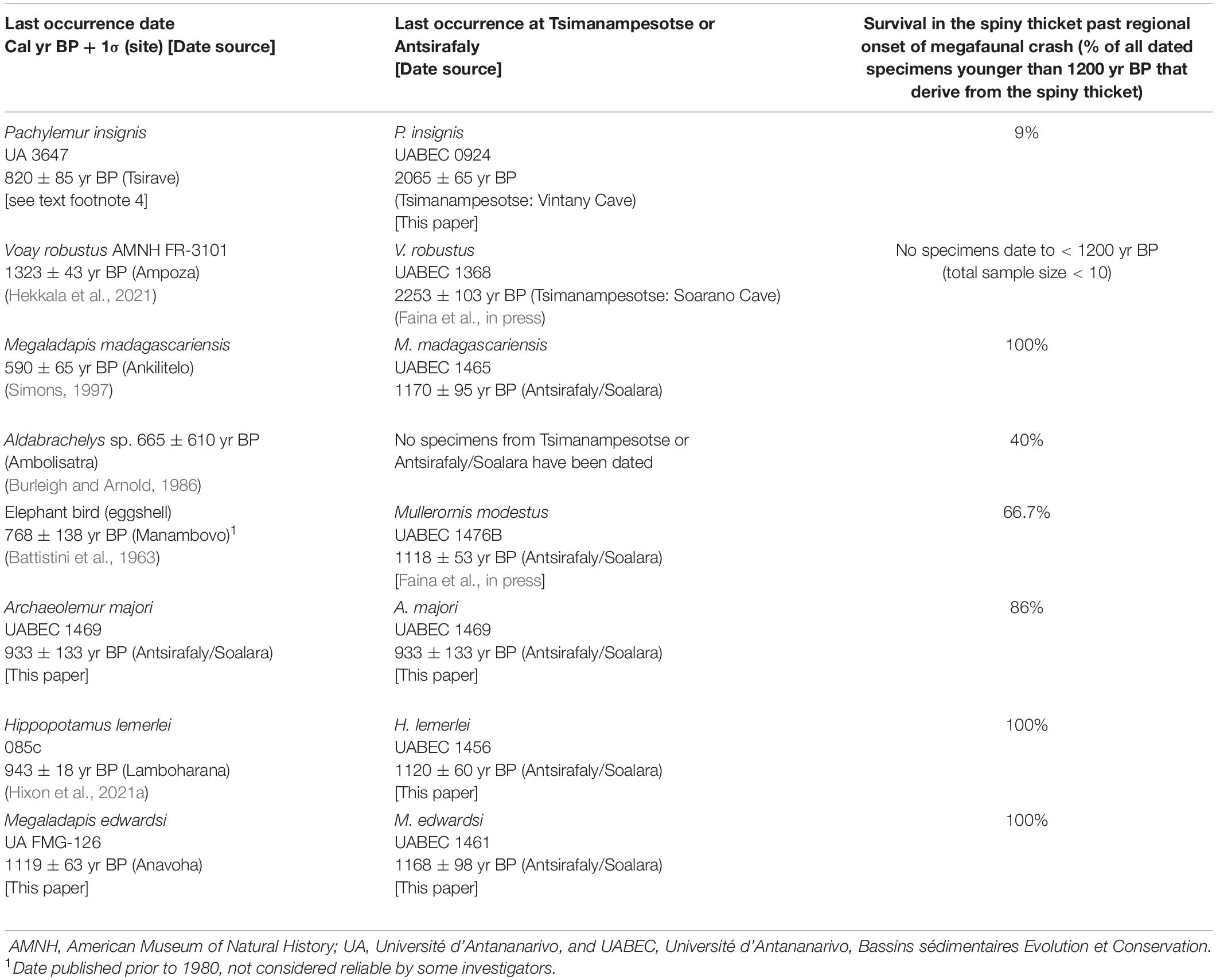
Table 6. Endemic vertebrates of the Tsimanampesotse region (now extinct) that likely survived into the last millenium, with last-known radiocarbon date in the spiny thicket or succulent woodland ecoregions, last-known date at Tsimanampesotse/Antsirafaly, survival past regional onset of large-vertebrate population crash.
It is instructive to examine the decline of each species relative to the time of recent large-bodied vertebrate collapse in southwest Madagascar (i.e., 1200 yr BP; Faina et al., in press). Five of the eight species (Hippopotamus lemerlei, Archaeolemur majori, Megaladapis edwardsi, M. madagascariensis, and Mullerornis modestus) can be characterized as having: (1) A relatively high percentage (on average, just under 30%) of all dated specimens from the southwest postdating 1200 yr BP; (2) A much higher percentage (on average, 95%) of the post-1200 yr BP dates for individuals from the spiny thicket rather than the succulent woodland; and (3) Last-known radiocarbon occurrences in the spiny thicket (Table 6). Traditional ecological knowledge of the survival well into the last millenium exists for some of these species (Supplementary Data File).
Pachylemur insignis shows a distinctly different pattern of regional decline (Table 6). This difference is exemplified by comparing P. insignis to the other large-bodied lemurs from the region: Archaeolemur majori, Megaladapis edwardsi, and M. madagascariensis (Figure 9). Unlike the other three lemur species, P. insignis is rare in the spiny thicket after the beginning of the megafaunal crash in the southwest (i.e., 1200 yr BP), and all dated specimens from the coast predate this period. This bears testimony to a possible decline of Pachylemur along the southern and southwestern coasts during the early part of the first millenium CE, with retreat to (or survival in) parts of the spiny thicket and succulent woodland to the east (inland) and north of TNP and Antsirafaly. The most recent dated Pachylemur insignis specimen from TNP is 1245 years older than the most recent specimen from elsewhere in the southwest. This species survived at Tsirave in the succulent woodland until at least 820 yr BP (Table 6). Similarly, Voay robustus survived at Ampoza in the succulent woodland for at least another 930 years past its last occurrence date at TNP (Table 6).
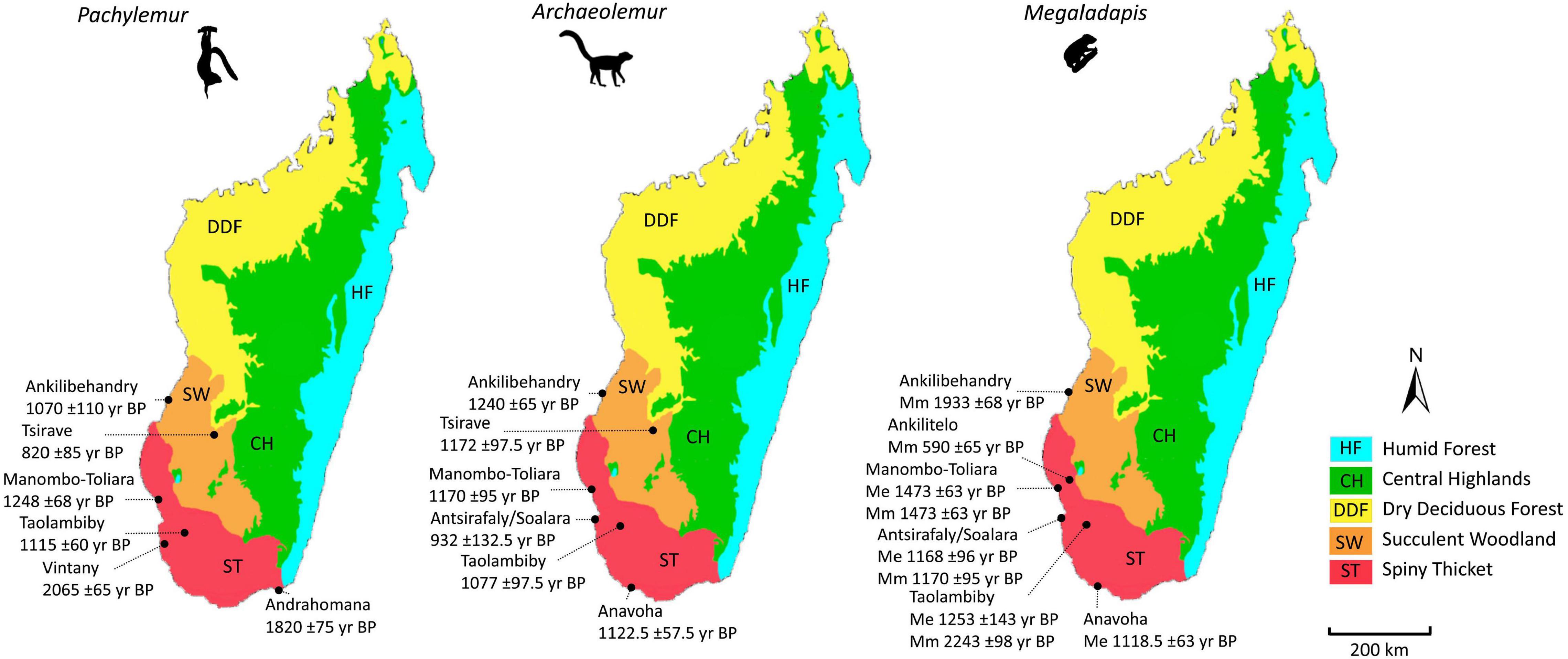
Figure 9. Last occurrence dates for Pachylemur, Archaeolemur, and Megaladapis at sites in the spiny thicket and succulent woodland ecoregions of the dry southwest. For Megaladapis, Mm, M. madagascariensis, and Me, M. edwardsi. All dates are calibrated, and all major terrestrial ecoregions of Madagascar (Burgess et al., 2004) are shown.
Regional chronological records are sparse for the other now-extinct species that we found at Tsimanampesotse, such as Mesopropithecus globiceps, the larger-bodied Coua spp., and Alopochen sirabensis; thus, we cannot be confident in reconstructions of their decline in geographic distribution. Published dates for these taxa at other spiny thicket sites all predate 1200 yr BP. This is true, for example, of M. globiceps from Tsiandroina at 1520 ± 95 yr BP (Burney et al., 2004); C. primaeva from Tsiandroina at 1870 ± 140 yr BP (Goodman and Rakotozafy, 1997); and A. sirabensis from Beloha Anavoha at 1233 ± 178 yr BP (Goodman and Rakotozafy, 1997). There are very few dates for species that are locally extirpated at TNP from other sites in the southwest, and all that exist predate 1200 yr BP. Macrotarsomys petteri has been dated at Andrahomana in the spiny thicket ecoregion (1620 ± 85 yr BP; Burney et al., 2008). It is possible that these extinct and locally extirpated taxa began declining on the southwest coast early in the first millenium CE, as did P. insignis.
Most of the well-dated species that we recovered at Antsirafaly are known to have survived at other sites in the subarid south for centuries past their ages of last-known occurrence at Antsirafaly (see Figure 9 for examples). This difference ranges from 0 years for Archaeolemur majori (Antsirafaly is its most recent last-known occurrence) to 580 years for Megaladapis madagascariensis. The average difference for five species is 231 years (Table 6 and Figure 9).
Aldabrachelys sp. and Voay robustus may also have survived well into the last millenium in the spiny thicket, but species identifications are often difficult for fragmentary giant tortoise remains, and Voay has its last-known radiocarbon occurrence in the succulent woodlands. Only four Voay specimens have been dated and its geographic pattern of decline is not well documented.
In summary, despite their geographical proximity, the extirpation episodes we documented at TNP and Antsirafaly differ not merely in timing and apparent proximate causation, but also in how they relate to the regional decline or extinction of the affected species. Local extirpation does not necessarily portend impending extinction. Along the coast at TNP, we observe early first millenium decline of a suite of species, some of which may have simply migrated to more mesic environments. At Antsirafaly, we observe the local extirpation of a suite of species that appears to have been more drought resistant. There is little evidence that these species migrated to more mesic environments either inland or to the north, but they likely sought nearby freshwater bodies that had not yet dried. Their disappearance at Antsirafaly is closer to the time of their eventual extinction.
What, Ultimately, Caused the Extinction of the Large-Bodied Vertebrates in Madagascar’s Southwest?
It was not long after vertebrate extirpation at Antsirafaly that island-wide extinction of Madagascar’s large-bodied endemic species occurred. Between 1200 and 900 yr BP, populations of the island’s largest endemic terrestrial vertebrates collapsed, not merely throughout the subarid southwest but across the island (Godfrey et al., 2019; Faina et al., in press). Large vertebrates disappeared from the wettest as well as the driest ecoregions of Madagascar; wherever subfossil sites exist, their decline can be documented. Species that were not yet extinct by the end of this period were presumably reduced to such small populations that they became paleontologically invisible. The hydroclimate of Madagascar at the time of the crash of megafaunal populations was neither uniformly wet nor dry. At ∼1,100 years ago when the local crash at Antsirafaly began, the northwest was wet (Scroxton et al., 2017) and the southwest was dry (Faina et al., in press). As we have shown above, a new pluvial period had swept into portions of the south thousands of years earlier, but it only arrived in the driest parts of the southwest as the regional drought of the late first millenium CE subsided.
It is worth emphasizing that the differences in mean annual rainfall between ‘pluvial’ and ‘interpluvial’ periods in the southwest were not necessarily very large, and certainly were smaller than the differences across ecoregions at any single point in time. That said, we can assume that if there is any place in Madagascar where dry climate would have been most likely to trigger the extinction of vertebrate species, it would be the subarid southwest. We have marshaled evidence that, at the end of a long, dry interpluvial, arid conditions did, indeed, impact vertebrate populations. At both TNP and Antsirafaly, local extirpation occurred without evidence for a human trigger. However, our data also suggest that climate alone cannot have caused their extinction. Our profiles of local decline, coupled with regional data, present a picture that is more complex.
We contend that it was the introduction and spread of agropastoralism that transformed what had been a series of local biogeographic shifts into wholesale population collapse and species extinction. Agropastoralism spread to Madagascar during the late first millenium CE with the introduction of domesticated animals and cultivated plants from Asia and Africa (Dewar and Wright, 1993; Boivin et al., 2013; Crowther et al., 2016). Between 1200 and 900 yr BP, it spread across Madagascar. This was long after people had settled on Madagascar; indeed, there is strong evidence that people were present at least intermittently in the southwest during the Early and Middle Holocene and certainly prior to 2,000 years ago (Hansford et al., 2018, 2020; Douglass et al., 2019; Davis et al., 2020). Hunter/forager and fisher/forager impacts on endemic large-bodied vertebrate populations were minimal, however (Douglass et al., 2018; Hansford et al., 2021). Large vertebrates were hunted, but there is little evidence of early megaherbivore population collapse (Godfrey et al., 2019; Faina et al., in press).
Not long after introduced animals appeared in the north, they spread southward. An influx of cattle, dogs, cats and bushpigs to the southwest is documented by a plethora of specimens dating between 900 and 700 yr BP (Hixon et al., 2021a,b). Temporal overlap between large-bodied endemic vertebrates and introduced species can be inferred (Hixon et al., 2021a,b), but what is abundantly clear is that, as populations of introduced species expanded, those of large-bodied endemic species dramatically declined. Bones of introduced species are found in the south and southwest at archeological settlement sites such as Andranosoa and Rezoky and at paleontological sites (lacking human artifacts) such as Lamboharana, Ambolisatra, and Beloha Anavoha as well as Tsimanampesotse. They are found at sites in the spiny thicket and succulent woodland, along the coast and much further inland. And importantly, the rapid increase in the populations of domesticated animals occurred in the southwest precisely when we have documented a shift in hydroclimate to more mesic conditions. We see this sequence of events in the region of Tsimanampesotse and Antsirafaly combined – i.e., with 900 yr BP representing the cut-point for a dramatic change in faunal composition. Now-extinct endemic large vertebrates occur prior to 900 yr BP, and introduced vertebrates appear afterward.
The extinction dynamic in the spiny thicket differed from that in the dry deciduous forest in northwestern Madagascar and likely in other parts of Madagascar. Faina et al. (in press) compared the trajectories of large-vertebrate decline in these two ecoregions; they found that the decline began earlier in the spiny thicket, in concert with drought conditions, whereas it began a century later in the dry deciduous forest, in concert with anthropogenic habitat transformation. In effect, the large-bodied vertebrate species of the southwest were already vulnerable to extinction by the time agropastoralism spread into the region.
There is a growing literature that addresses the reasons why agropastoralists can pose greater danger to endemic species than hunter/foragers. Perhaps counterintuitively, wild-animal hunting is often less sustainable in herder/farmer communities than in hunter/forager communities (Robinson and Bennett, 2000). Extinction of slow-breeding, large-bodied endemic species is more likely in the former because they rely on alternative food sources including cultivated plants and fast-breeding domesticated animals (Belovsky, 1988; Winterhalder, 2001). People are not likely to drive to extinction species on which they depend for sustenance; supplemental sources of food are more expendable.
Modern vertebrate populations may provide some clues regarding the impacts of severe climate events and mechanisms of local vertebrate population recovery. When critical resources decline or disappear at any single locality, populations suffer, but they usually recover (Gould et al., 1999; Ratsimbazafy et al., 2002). The initial stimulus may involve a climate event, but ultimately, extinction occurs because normal population recovery mechanisms are disrupted, particularly for species that have dietary or locomotor specializations. Agropastoralists can disrupt normal population recovery mechanisms by cutting typical dispersal pathways, modifying habitats, competing directly with vulnerable endemic species for resources such as fresh water, competing indirectly by introducing other competitors, and indeed killing endemic wild animals for food or because the latter may pose threats to domesticated animals or crops (e.g., Borgerson, 2016; Borgerson et al., 2019). When humans are present, waterholes that formerly provided sanctuary can pose new risks (Janzen, 1983). Finally, the decline of endemic keystone large-bodied herbivore species (whether from climate change or human impacts) can affect ecosystem functionality, further exacerbating environmental degradation and contributing to the simultaneous disappearance of different vertebrate species. In Madagascar, this happened within a narrow temporal band, regardless of climate conditions (Burney et al., 2003; Hansford et al., 2021).
Conclusion
Returning to the prior analyses of MacPhee (1986) and Goodman and Jungers (2014), we see elements of each that can be supported. Goodman and Jungers (2014) were likely correct in highlighting a role for aridification in driving periodic local extirpations, independent of human activities, in Madagascar’s southwest. Our data do not support their hypothesis, however, that the climate of the southwest was wetter and less seasonal than it is now until around 1,000 years ago. MacPhee (1986) was correct in asserting that southwestern Madagascar may have been as dry as it is today when now-extinct megaherbivores thrived there. Indeed, our stalagmite growth data demonstrate that, between 3,000 and 2,000 years ago, Tsimanampesotse was drier than it is today. MacPhee was also likely correct that wet microhabitats were key to the local survival of species dependent on wetter conditions in the past. Long-term survival would have depended on the existence of dispersal pathways that allowed populations to track these critical resources.
The late first millenium CE drought resulted in a regional decline in the populations of large-bodied, drought-resistant vertebrates in the southwest but likely did not drive these species to extinction. Indeed, it may have temporarily helped to isolate them from expanding agropastoralist populations. With increasing rainfall, albeit limited, agropastoralists spread into localities that they previously considered too dry for habitation. Thus, ironically, one can make the argument that the amelioration of climate contributed to the rapid demise of the large-bodied endemic vertebrates in the southwest. The return to mesic conditions undoubtedly expedited the spread of agropastoralists into the region and prevented the recovery of Madagascar’s large endemic vertebrate species.
Data Availability Statement
The original contributions presented in the study are included in the article/Supplementary Material, further inquiries can be directed to the corresponding author/s.
Author Contributions
LG, BC, KM, SB, NS, and ZK designed the research. LG, BC, KM, SB, NS, ZK, LR, JA, PF, SG, IG, JH, EH, CK, ML, DM, VP, NR, HR, ST, and NV performed the analyses. LG, BC, KM, ZK, LR, JA, MB, RD, PF, SG, IG, JH, EH, PL, ML, NR, HR, KS, ST, and PW aided fossil collection and/or identification. LG, BC, SB, NS, and ZK wrote the manuscript. All the authors edited the manuscript.
Funding
This project was supported by the National Science Foundation (BCS-1750598 to LG, AGS-1702891 to SB, BCS-1749676 to BC, BCS-1749211 to KM, AGS-1702691 to DM, and RAPID DEB-1931213 to EH), a Marie Skłodowska-Curie Actions & Irish Research Council CAROLINE Fellowship (CLNE/2019/290) to NS, and a University of Massachusetts Natural History Collections grant to IG.
Conflict of Interest
The authors declare that the research was conducted in the absence of any commercial or financial relationships that could be construed as a potential conflict of interest.
Publisher’s Note
All claims expressed in this article are solely those of the authors and do not necessarily represent those of their affiliated organizations, or those of the publisher, the editors and the reviewers. Any product that may be evaluated in this article, or claim that may be made by its manufacturer, is not guaranteed or endorsed by the publisher.
Acknowledgments
This research was conducted under a collaborative accord between the University of Massachusetts Amherst (Department of Geosciences, Department of Anthropology) and Université d’Antananarivo, Madagascar (Bassins sédimentaires, Evolution, Conservation), as well as a convention with Madagascar National Parks. We thank the Ministry of Higher Educational and Research, Ministry of Mines and Strategic Resources, Ministry of Communication and Culture. Permission was obtained from the Madagascar National Parks to conduct field research within the boundaries of the Tsimanampesotse National Park and to collect a prescribed number of fossils and a prescribed total weight for stalagmites. We gratefully acknowledge the assistance of the people of the regions of Tampolove near Asafora Cave, Soalara near Antsirafaly, Ihosy near Andranomilitsy, and TNP in making this research possible. This manuscript benefited from discussions with colleagues, to whom we are grateful. These include (among others) Philippe Beaujard, Cortni Borgerson, David Burney, Kristina Douglass, Sean Hixon, Tanambelo Rasolondrainy, and Christopher Raxworthy. This is Duke Lemur Center publication number 1485.
Supplementary Material
The Supplementary Material for this article can be found online at: https://www.frontiersin.org/articles/10.3389/fevo.2021.742203/full#supplementary-material
Footnotes
- ^ Burns, S. J., McGee, D., Scroxton, N., Kinsley, C. W., Godfrey, L. R., Faina, P., et al. (submitted). Southern Hemisphere controls on ITCZ variability in southwest Madagascar over the past 117,000 years.
- ^ Klukkert, Z. S., Godfrey, L. R., Crowley, B. E., Muldoon, K. M., Burns, S. J., Ranivoharimanana, L., et al. (in prep.). Flooding Vintany; Formation and faunal deposition in an ecologically important sinkhole in southwest Madagascar.
- ^ Lewis, M. E., Godfrey, L. R., Rakotondramavo, J.-C., Klukkert, Z., Scroxton, N., Burns, S. J., et al. (Submitted). A new large, extinct fosa (Carnivora: Eupleridae) and other Cryptoprocta from Tsimanampesotse National Park, Madagascar.
- ^ Godfrey, L. R., Pérez, V. R., Crowley, B. E., Borgerson, C., Bankoff, R. J., Perry, G. H., et al. (Submitted). Giant lemur butchery was widespread in southwestern Madagascar prior to 1000 CE.
References
Alumbaugh, J. L., Goodman, S. M., and Samonds, K. E. (2021). Morphometric analyses of modern and subfossil Macronycteris (family Hipposideridae) refine groups from Anjohibe Cave, northwestern Madagascar. Acta Chiropterol. 23, 33–43.
Ambrose, S. H. (1990). Preparation and characterization of bone and tooth collagen for isotopic analysis. J. Archaeol. Sci. 17, 431–451.
Ayliffe, L. K., Pyramo, C. M., Moriarty, K. C., Wells, R. T., McCulloch, M. T., Graham, E. M., et al. (1998). 500 ka precipitation record from southeastern Australia: evidence for interglacial relative aridity. Geology 26, 147–150. doi: 10.1130/0091-7613(1998)026<0147:kprfsa>2.3.co;2
Bailey, G. N., and Flemming, N. C. (2008). Archaeology of the continental shelf: marine resources, submerged landscapes and underwater archaeology. Q. Sci. Rev. 27, 2153–2165. doi: 10.1016/j.quascirev.2008.08.012
Battistini, R., Vérin, P., and Raison, R. (1963). La site archéologique de Talaky: cadre géographique et géologique, premiers travaux de fouilles, notes ethnographiques sur le village actuel proche du site. Ann. de l’Univ. Madagascar 1, 112–134.
Belovsky, G. E. (1988). An optimal foraging-based model of hunter-gatherer population dynamics. J. Anthropol. Archaeol. 7, 329–372. doi: 10.1016/0278-4165(88)90002-5
Boivin, N., Crowther, A., Helm, R., and Fuller, D. Q. (2013). East Africa and madagascar in the Indian Ocean World. J. World Prehist. 26, 213–281. doi: 10.1007/s10963-013-9067-4
Borgerson, C. (2016). Optimizing conservation policy: the importance of seasonal variation in hunting and meat consumption on the Masoala peninsula of Madagascar. Oryx 50, 405–418. doi: 10.1017/s0030605315000307
Borgerson, C., Razafindrapaoly, B., Rajaona, D., Rasolofoniaina, B. J. R., and Golden, C. D. (2019). Food insecurity and the unsustainable hunting of wildlife in a UNESCO World Heritage Site. Front. Sustain. Food Syst. 3:99. doi: 10.3389/fsufs.2019.00099
Burgess, N., D’Amico Hales, J., Underwood, E., Dinerstein, E., Olson, D., Itoua, I., et al. (2004). Terrestrial Ecoregions of Africa and Madagascar: A Conservation Assessment. Washington, D.C: Island Press.
Burleigh, R., and Arnold, E. N. (1986). Age and dietary differences of recently extinct Indian Ocean tortoises (Geochelone s. lat.) revealed by carbon isotope analysis. Proc. R. Soc. Ser. B Biol. Sci. 227, 137–144. doi: 10.1098/rspb.1986.0014
Burney, D. A., Burney, L. P., Godfrey, L. R., Jungers, W. L., Goodman, S. M., Wright, H. T., et al. (2004). A chronology for late prehistoric Madagascar. J. Hum. Evol. 47, 25–63. doi: 10.1016/j.jhevol.2004.05.005
Burney, D. A., Robison, G. S., and Burney, L. P. (2003). Sporormiella and the late Holocene extinctions in Madagascar. Proc. Natl. Acad. Sci. U.S.A. 100, 10800–10805. doi: 10.1073/pnas.1534700100
Burney, D. A., Vasey, N., Godfrey, L. R., Ramilisonina, Jungers, W. L., Ramarolahy, M., et al. (2008). New findings at Andrahomana Cave, southeastern Madagascar. J. Cave Karst Stud. 70, 13–24.
Cheng, H., Edwards, R. L., Shen, C.-C., Polyak, V. J., Asmerom, Y., Woodhead, J., et al. (2013). Improvements in 230Th dating, 230Th and 234U half-life values, and U-Th isotopic measurements by multi-collector inductively coupled plasma mass spectrometry. Earth Planet. Sci. Lett. 37, 82–91. doi: 10.1016/j.epsl.2013.04.006
Crowley, B. E. (2010). A refined chronology of prehistoric Madagascar and the demise of the megafauna. Q. Sci. Rev. 29, 2591–2603. doi: 10.1016/j.quascirev.2010.06.030
Crowley, B. E., Godfrey, L. R., Bankoff, R. J., Perry, G. H., Culleton, B. J., Kennett, D. J., et al. (2017). Island-wide aridity did not trigger recent megafaunal extinctions in Madagascar. Ecography 40, 901–912. doi: 10.1111/ecog.02376
Crowther, A., Lucas, L., Helm, R., Horton, M., Shipton, C., Wright, H. T., et al. (2016). Ancient crops provide first archaeological signature of the westward Austronesian expansion. Proc. Natl. Acad. Sci. U.S.A. 113, 6635–6640. doi: 10.1073/pnas.1522714113
Davis, D. S., DiNapoli, R. J., and Douglass, K. (2020). Integrating point process models, evolutionary ecology and traditional knowledge improves landscape archaeology—A case from Southwest Madagascar. Geosciences 10:287. doi: 10.3390/geosciences10080287
Dewar, R. E., and Wright, H. T. (1993). The culture history of Madagascar. J. World Prehist. 7, 417–466. doi: 10.1007/bf00997802
Douglass, K., Antonites, A. R., Morales, E. M. Q., Grealy, A., Bunce, M., Bruwer, C., et al. (2018). Multi-analytical approach to zooarchaeological assemblages elucidates Late Holocene coastal lifeways in southwest Madagascar. Q. Int. 471, 111–131. doi: 10.1016/j.quaint.2017.09.019
Douglass, K., Hixon, S., Wright, H. T., Godfrey, L. R., Crowley, B. E., Manjakahery, B., et al. (2019). A critical review of radiocarbon dates clarifies the human settlement of Madagascar. Q. Sci. Rev. 221:105878. doi: 10.1016/j.quascirev.2019.105878
Dumitru, O. A., Polyak, V. J., Asmerom, Y., and Onac, B. P. (2021). Last interglacial sea-level history from speleothems: a global standardized database. Earth Syst. Sci. Data 13, 2077–2094. doi: 10.5194/essd-13-2077-2021
Faina, F., Burns, S. J., Godfrey, L. R., Crowley, B. E., Scroxton, N., McGee, D., et al. (in press). Comparing the paleoclimates of northwestern and southwestern Madagascar during the late Holocene: implications for the role of climate in megafaunal extinction. Malagasy Nat. 15.
Farris, Z. J., Golden, C. D., Karpanty, S., Murphy, A., Stauffer, D., Ratelolahy, F., et al. (2015). Hunting, exotic carnivores, and habitat loss: anthropogenic effects on a native carnivore community, Madagascar. PLoS One 10:e0136456. doi: 10.1371/journal.pone.0136456
Fleming, K., Johnston, P., Zwartz, D., Yokoyama, Y., Lambeck, K., and Chappell, J. (1998). Refining the eustatic sea-level curve since the Last Glacial Maximum using far- and intermediate-field sites. Earth Planet. Sci. Lett. 163, 327–342. doi: 10.1016/s0012-821x(98)00198-8
Godfrey, L. R., Scroxton, N., Crowley, B. E., Burns, S. J., Sutherland, M. R., Pérez, V. R., et al. (2019). A new interpretation of Madagascar’s megafaunal decline: the “Subsistence Shift Hypothesis”. J. Hum. Evol. 130, 126–140. doi: 10.1016/j.jhevol.2019.03.002
Goodman, S. M., and Jungers, W. L. (2014). Extinct Madagascar - Picturing the Island’s Past. Chicago: The University of Chicago Press.
Goodman, S. M., Raherilalao, M. J., and Muldoon, K. (2013). Bird fossils from Ankilitelo Cave: inference about Holocene environmental changes in southwestern Madagascar. Zootaxa 3750, 534–548. doi: 10.11646/zootaxa.3750.5.6
Goodman, S. M., Raherilalao, M. J., and Wohlauser, S. (eds) (2018). The Terrestrial Protected Areas of Madagascar: Their History, Description, and Biota, Volume III: Western and Southwestern Madagascar - Synthesis. Antananarivo: Association Vahatra.
Goodman, S. M., and Rakotozafy, L. M. A. (1997). “Subfossil birds from coastal sites in western and southwestern Madagascar: a paleoenvironmental reconstruction,” in Natural Change and Human Impact in Madagascar, eds S. M. Goodman and B. D. Patterson (Washington D.C: Smithsonian Institution Press), 257–279.
Gould, L., Sussman, R. W., and Sauther, M. L. (1999). Natural disasters and primate populations: the effects of a 2-year drought on a naturally occurring population of ring-tailed lemurs (Lemur catta) in southwestern Madagascar. Int. J. Primatol. 20, 69–84.
Greene, C. A., Thirumalai, K., Kearney, K. A., Delgado, J. M., Schwanghart, W., Wolfenbarger, N. S., et al. (2019). The climate data toolbox for MATLAB. Geochem. Geophys. Geosyst. 20, 3774-3781. doi: 10.1029/2019GC008392
Guyot, L. (2002). Reconnaissance Hydrogéologique Pour L’alimentation en Eau D’une Plaine Littorale En Milieu Semi-Aride: Sud-Ouest de Madagascar. Thèse de Doctorat. Nantes: Université de Nantes.
Hansford, J., Wright, P. C., Rasoamiaramanana, A., Pérez, V. R., Godfrey, L. R., Errickson, D., et al. (2018). Early Holocene human presence in Madagascar evidenced by exploitation of avian megafauna. Sci. Adv. 4:eaat6925. doi: 10.1126/sciadv.aat6925
Hansford, J. P., Lister, A. M., Weston, E. M., and Turvey, S. T. (2021). Simultaneous extinction of Madagascar’s megaherbivores correlates with late Holocene human-caused landscape transformation. Q. Sci. Rev. 263:106996. doi: 10.1016/j.quascirev.2021.106996
Hansford, J. P., Wright, P. C., Rasoamiaramanana, A., Pérez, V. R., Muldoon, K. M., Turvey, S. T., et al. (2020). Evidence for early human arrival in Madagascar is robust: a response to Mitchell. J. Island Coast. Archaeol. 15, 596–602. doi: 10.1080/15564894.2020.1771482
Hekkala, E., Gatesy, J., Narechania, A., Meredith, R., Russello, M., Aardema, M. L., et al. (2021). Paleogenomics illuminates the evolutionary history of the extinct Holocene “horned” crocodile of Madagascar, Voay robustus. Commun. Biol. 4:505. doi: 10.1038/s42003-021-02017-0
Hellstrom, J. (2003). Rapid and accurate U/Th dating using parallel ion-counting multi-collector ICP-MS. J. Anal. Atom. Spectr. 18, 1346–1351. doi: 10.1039/b308781f
Hixon, S. W., Douglass, K. G., Crowley, B. E., Rakotozafy, L., Clark, G., Anderson, A., et al. (2021a). Late Holocene spread of pastoralism coincides with endemic megafaunal extinction on Madagascar. Proc. R. Soc. B Biol. Sci. 288:20211204. doi: 10.1098/rspb.2021.1204
Hixon, S. W., Douglass, K. G., Godfrey, L. R., Eccles, L., Crowley, B. E., Rakotozafy, L., et al. (2021b). Ecological consequences of a millennium of introduced dogs on Madagascar. Front. Ecol. Evol. 9:689599. doi: 10.3389/fevo.2021.689559
Hogg, A. G., Heaton, T. J., Hua, Q., Palmer, J. G., Turney, C. S. M., Southon, J., et al. (2020). SHCal20 Southern Hemisphere calibration, 0-55,000 years cal BP. Radiocarbon 62, 759–778. doi: 10.1017/rdc.2020.59
Huybers, P. (2006). Early Pleistocene glacial cycles and the integrated summer insolation forcing. Science 313, 508–511. doi: 10.1126/science.1125249
Jaffey, A. H., Flynn, K. F., Glendenin, L. E., Bentley, W. C., and Essling, A. M. (1971). Precision measurement of half-lives and specific activities of 235U and 238U. Phys. Rev. C 4, 1889–1906. doi: 10.1103/physrevc.4.1889
Kaufmann, J. C. (2004). Prickly pear cactus and pastoralism in Southwest Madagascar. Ethnology 43, 345–361. doi: 10.2307/3774032
Kaufmann, J. C. (2008). The non-modern constitution of famines in Madagascar’s spiny forests: “Water-food” plants, cattle and Mahafale landscape praxis. Environ. Sci. 5, 73–89. doi: 10.1080/15693430801912162
Lamberton, C. (1937). Fouilles paléontologiques faites en 1936. Bull. de l’Académie Malgache 19, 127–170.
Loewen, M. A., Samonds, K. E., and Ramarolahy, M. F. (2001). Lake Tsimanampetsotsa, a modern alkaline playa lake in Madagascar. Geol. Soc. Am. 33:443.
Lührs, M.-L., and Dammhahn, M. (2010). An unusual case of cooperative hunting in a solitary carnivore. J. Ethol. 28, 379–383. doi: 10.1007/s10164-009-0190-8
Lührs, M.-L., and Kappeler, P. M. (2014). Polyandrous mating in treetops: how male competition and female choice interact to determine an unusual carnivore mating system. Behav. Ecol. Sociobiol.’68, 879–889. doi: 10.1007/s00265-014-1701-3
MacPhee, R. D. E. (1986). Environment, extinction, and Holocene vertebrate localities in Southern Madagascar. Natl. Geogr. Res. 2, 441–455.
Meador, L. R., Godfrey, L. R., Rakotondramavo, J. C., and Ranivoharimanana, L. (2019). Cryptoprocta spelea (Carnivora: Eupleridae): what did it eat and how do we know? J. Mammal. Evol. 26, 237–251. doi: 10.1007/s10914-017-9391-z
Middleton, K. (1999). Who killed ‘Malagasy cactus’? Science, environment and colonialism in southern Madagascar (1924-1930). J. South. Afr. Stud. 25, 215–248. doi: 10.1080/030570799108678
Nielsen-Marsh, C. M., and Hedges, R. E. (2000). Patterns of diagenesis in bone I: the effects of site environments. J. Archaeol. Sci. 27, 1139–1150. doi: 10.1006/jasc.1999.0537
Perrier de la Bâthie, H. (1934). Au sujet de l’âge de la faune à Aepyornis et hippopotames. Mém. de l’Académie Malgache 17, 162–168.
Petit, G. (1935). Contribution à l’étude faunistique de la Réserve Naturelle du Manampetsa (Madagascar). Le Lac Manampetsa et la réserve dite du Manampetsa. Ann. Sci. Nat. 10, 423–437.
Pfretzschner, H. U. (2004). Fossilization of Haversian bone in aquatic environments. Comptes Rendus Palevol. 3, 605–616. doi: 10.1016/j.crpv.2004.07.006
Railsback, L. B., Dupont, L. A., Liang, F. Y., Brook, G. A., Burney, D. A., Cheng, H., et al. (2020). Relationships between climate change, human environmental impact, and megafaunal extinction inferred from a 4000-year multi-proxy record from a stalagmite from northwestern Madagascar. Q. Sci. Rev. 234:106244. doi: 10.1016/j.quascirev.2020.106244
Ranivo, J., and Goodman, S. M. (2007). Variation géographique de Hipposideros commersoni de la zone sèche de Madagascar (Mammalia, Chiroptera, Hipposideridae). Verhandlungen Naturwissenschaftlichen Vereins Hamburg 43, 33–56.
Rasoloariniaina, J. R., Ganzhorn, J. U., and Raminosoa, N. (2015). Physicochemical and bacteriological water quality across different forms of land use on the Mahafaly Plateau, Madagascar. Water Qual. Exposure Health 7, 111–124. doi: 10.1007/s12403-014-0129-3
Rasolonjatovo, H. A. M., Muldoon, K. M., Ranivoharimanana, L., Rakotoarijaona, M., and Goodman, S. M. (in press). Subfossil birds from a submerged cave in southwestern Madagascar. Malagasy Nat. 15.
Ratsimbazafy, J. H., Ramarosandratana, H. V., and Zaonarivelo, R. J. (2002). How do black-and-white ruffed lemurs still survive in a highly disturbed habitat? Lemur News 7, 7–10.
Ravelonanosy, C., and Duflos, J. (1964). Bilan des explorations spéléologiques à Madagascar pour l’année 1964. Madagascar Revue Géogr. 6, 117–132.
Robinson, J. G., and Bennett, E. L. (2000). “Carrying capacity limits to sustainable hunting in tropical forests,” in Hunting for Sustainability in Tropical Forests, eds J. G. Robinson and E. L. Bennett (New York: Columbia University Press), 13–30.
Rosenberger, A. L., Godfrey, L. R., Muldoon, K. M., Gunnell, G. F., Andriamialison, H., Ranivoharimanana, L., et al. (2015). Giant subfossil lemur graveyard discovered, submerged, in Madagascar. J. Hum. Evol. 81, 83–87. doi: 10.1016/j.jhevol.2015.01.004
Sauther, M. L., Cuozzo, F. P., Youssouf Jacky, I. A., Fish, K. D., LaFleur, M., Ravelohasindrazana, L. A. L., et al. (2013). Limestone cliff-face and cave use by wild ring-tailed lemurs (Lemur catta) in southwestern Madagascar. Madagascar Conserv. Dev. 8, 73–80.
Scroxton, N., Burns, S. J., McGee, D., Hardt, B., Godfrey, L. R., Ranivoharimanana, L., et al. (2017). Hemispherically in-phase precipitation variability over the last 1700 years in a Madagascar speleothem record. Q. Sci. Rev. 164, 25–36. doi: 10.1016/j.quascirev.2017.03.017
Scroxton, N., Burns, S. J., McGee, D., Hardt, B., Godfrey, L. R., Ranivoharimanana, L., et al. (2019). Competing temperature and atmospheric circulation effects on southwest Madagascan rainfall during the last deglaciation. Paleoceanogr. Paleoclimatol. 34, 275–286. doi: 10.1029/2018PA003466
Scroxton, N., Gagan, M. K., Dunbar, G. B., Ayliffe, L. K., Hantoro, W. S., Shen, C. C., et al. (2016). Natural attrition and growth frequency variations of stalagmites in southwest Sulawesi over the past 530,000 years. Paleoceanogr. Paleoclimatol. 441, 823–833. doi: 10.1016/j.palaeo.2015.10.030
Semel, B. P., Baden, A. L., Salisbury, R. L., McGee, E. M., Wright, P. C., and Arrigo-Nelson, S. J. (2019). Assessing the function of geophagy in a Malagasy rainforest lemur. Biotropica 51, 769–780. doi: 10.1111/btp.12699
Sharman, G. R., Sharman, J. P., and Sylvester, Z. (2018). detritalPy: a Python-based toolset for visualizing and analysing detrital geo-thermochronologic data. Deposit. Rec. 4, 202–215. doi: 10.1002/dep2.45
Simons, E. L. (1997). “Lemurs old and new,” in Natural Change and Human Impact in Madagascar, eds S. M. Goodman and B. D. Patterson (Washington, D.C: Smithsonian Institution Press), 142–166.
Stinnesbeck, W., Becker, J., Hering, F., Frey, E., González, A. G., Fohlmeister, J., et al. (2017). The earliest settlers of Mesoamerica date back to the late Pleistocene. PLoS One 12:e0183345. doi: 10.1371/journal.pone.0183345
Stuiver, M., Reimer, P. J., and Reimer, R. W. (2021). CALIB 8.2 [WWW program]. Available online at: http://calib.org (accessed March 14, 2021).
Vaks, A., Bar-Matthews, M., Matthews, A., Ayalon, A., and Frumkind, A. (2010). Middle-late quaternary paleoclimate of northern margins of the saharan-arabian desert: reconstruction from speleothems of Negev Desert, Israel. Q. Sci. Rev. 29, 2647–2662. doi: 10.1016/j.quascirev.2010.06.014
Vallet-Coulomb, C., Gasse, F., Robison, L., Ferry, L., Van Campo, E., and Chalie, F. (2006). Hydrological modeling of tropical closed Lake Ihotry (SW Madagascar): sensitivity analysis and implications for paleohydrological reconstructions over the past 4000 years. J. Hydrol. 331, 257–271. doi: 10.1016/j.jhydrol.2006.05.026
van Klinken, G. J. (1999). Bone collagen quality indicators for paleodietary and radiocarbon measurements. J. Archaeol. Sci. 26, 687–695. doi: 10.1006/jasc.1998.0385
Vasey, N., and Godfrey, L. R. (in press). “Lemur hunting in Madagascar’s present and past: the case of Pachylemur,” in World Archeoprimatology: Interconnections of Humans and Nonhuman Primates in the Past, eds B. Urbani, D. Youlatos, and A. Antczak (Cambridge, UK: Cambridge University Press).
Virah-Sawmy, M., Gillson, L., Gardner, C. J., Anderson, A., Clark, G., and Haberle, S. (2016). A landscape vulnerability framework for identifying integrated conservation and adaptation pathways to climate change: the case of Madagascar’s spiny forest. Landsc. Ecol. 31, 637–654. doi: 10.1007/s10980-015-0269-2
Voarintsoa, N. R. G., Railsback, L. B., Brook, G. A., Wang, L. X., Kathayat, G., Cheng, H., et al. (2017). Three distinct Holocene intervals of stalagmite deposition and nondeposition revealed in NW Madagascar, and their paleoclimate implications. Clim. Past 13, 1771–1817. doi: 10.5194/cp-13-1771-2017
Vuillaume-Randriamanantena, M. (1982). Contribution À l’étude Des os Longs Des Lémuriens Subfossiles Malgaches : Leurs Particularités au Niveau des Proportions. Dissertation de 3e Cycle. Antananarivo: Université d’Antananarivo.
Wang, L. X., Brook, G. A., Burney, D. A., Voarintsoa, N. R. G., Liang, F. Y., Cheng, H., et al. (2019). The African Humid Period, rapid climate change events, the timing of human colonization, and megafaunal extinctions in Madagascar during the Holocene: evidence from a 2m Anjohibe Cave stalagmite. Q. Sci. Rev. 210, 136–153. doi: 10.1016/j.quascirev.2019.02.004
White, W. B. (1976). “Cave minerals and speleothems,” in The Science of Speleology, eds T. D. Ford and C. H. D. Cullingford (London: Academic Press), 267–327.
Keywords: megafaunal collapse, climate change, human impacts, southwest Madagascar, Late Holocene
Citation: Godfrey LR, Crowley BE, Muldoon KM, Burns SJ, Scroxton N, Klukkert ZS, Ranivoharimanana L, Alumbaugh J, Borths M, Dart R, Faina P, Goodman SM, Gutierrez IJ, Hansford JP, Hekkala ER, Kinsley CW, Lehman P, Lewis ME, McGee D, Pérez VR, Rahantaharivao NJ, Rakotoarijaona M, Rasolonjatovo HAM, Samonds KE, Turvey ST, Vasey N and Widmann P (2021) Teasing Apart Impacts of Human Activity and Regional Drought on Madagascar’s Large Vertebrate Fauna: Insights From New Excavations at Tsimanampesotse and Antsirafaly. Front. Ecol. Evol. 9:742203. doi: 10.3389/fevo.2021.742203
Received: 15 July 2021; Accepted: 23 August 2021;
Published: 23 September 2021.
Edited by:
Geoffrey Clark, Australian National University, AustraliaReviewed by:
Eduardo Jiménez-Hidalgo, University of the Sea, MexicoMaciej Tomasz Krajcarz, Institute of Geological Sciences (PAN), Poland
Copyright © 2021 Godfrey, Crowley, Muldoon, Burns, Scroxton, Klukkert, Ranivoharimanana, Alumbaugh, Borths, Dart, Faina, Goodman, Gutierrez, Hansford, Hekkala, Kinsley, Lehman, Lewis, McGee, Pérez, Rahantaharivao, Rakotoarijaona, Rasolonjatovo, Samonds, Turvey, Vasey and Widmann. This is an open-access article distributed under the terms of the Creative Commons Attribution License (CC BY). The use, distribution or reproduction in other forums is permitted, provided the original author(s) and the copyright owner(s) are credited and that the original publication in this journal is cited, in accordance with accepted academic practice. No use, distribution or reproduction is permitted which does not comply with these terms.
*Correspondence: Laurie R. Godfrey, lgodfrey@umass.edu