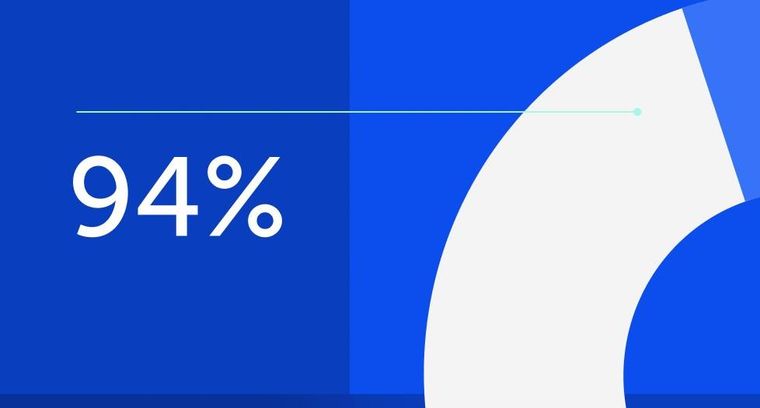
94% of researchers rate our articles as excellent or good
Learn more about the work of our research integrity team to safeguard the quality of each article we publish.
Find out more
REVIEW article
Front. Ecol. Evol., 30 September 2021
Sec. Evolutionary Developmental Biology
Volume 9 - 2021 | https://doi.org/10.3389/fevo.2021.735924
This article is part of the Research TopicHormones and Life History StrategiesView all 10 articles
Diapause is a state of developmental arrest adopted in response to or in anticipation of environmental conditions that are unfavorable for growth. In many cases, diapause is facultative, such that animals may undergo either a diapause or a non-diapause developmental trajectory, depending on environmental cues. Diapause is characterized by enhanced stress resistance, reduced metabolism, and increased longevity. The ability to postpone reproduction until suitable conditions are found is important to the survival of many animals, and both vertebrate and invertebrate species can undergo diapause. The decision to enter diapause occurs at the level of the whole animal, and thus hormonal signaling pathways are common regulators of the diapause decision. Unlike other types of developmental arrest, diapause is programmed, such that the diapause developmental trajectory includes a pre-diapause preparatory phase, diapause itself, recovery from diapause, and post-diapause development. Therefore, developmental pathways are profoundly affected by diapause. Here, I review two conserved hormonal pathways, insulin/IGF signaling (IIS) and nuclear hormone receptor signaling (NHR), and their role in regulating diapause across three animal phyla. Specifically, the species reviewed are Austrofundulus limnaeus and Nothobranchius furzeri annual killifishes, Caenorhabditis elegans nematodes, and insect species including Drosophila melanogaster, Culex pipiens, and Bombyx mori. In addition, the developmental changes that occur as a result of diapause are discussed, with a focus on how IIS and NHR pathways interact with core developmental pathways in C. elegans larvae that undergo diapause.
Diapause is a stress-resistant and developmentally arrested stage that can be adopted in order to increase the chance of survival in adverse environmental conditions (Hand et al., 2016). Diapause can occur as an obligate part of development, but in many species, diapause is facultative, occurring in response to environmental cues that signal that an unfavorable environment either exists or is likely to exist in the near future. For example, temperature, nutrient availability, and photoperiod are common cues that influence the decision to enter diapause (Hand et al., 2016). This type of facultative diapause exists in many animal species, both vertebrate and invertebrate. In these species, there are two separate life histories or developmental trajectories available: one that includes diapause and one that does not.
The diapause developmental trajectory differs in several respects from the non-diapause trajectory. First, there is a pre-diapause stage where the animal prepares to enter diapause. Then there is diapause itself which can last a variable amount of time. Next there is a period of recovery, and finally there is post-diapause development. Therefore, developmental pathways must accommodate all of these aspects of the diapause developmental trajectory to allow development to occur normally. This review describes hormonal regulation of diapause and the effects of diapause on development.
Diapause occurs in a wide range of animal species including nematodes, insects and other arthropods, fishes, and mammals (Hand et al., 2016). This review will focus on a few well-studied examples of these systems that undergo facultative diapause. A brief description of how diapause occurs in each system is provided in this section, along with the ecological context which diapause serves in this organism.
Nematodes are highly abundant and widespread animals. Nematode species generally have two sexes, which can either be male and female or male and self-fertilizing hermaphrodite (Ellis and Lin, 2014). Each female or hermaphrodite lays hundreds of embryos, contributing to a boom-and-bust life cycle (Frézal and Félix, 2015). Many species within the order Rhabditida have a facultative diapause stage called dauer that can be used to survive the bust periods (Ley, 2006). Dauer occurs midway through larval development and is thought to be analogous to the infective stage adopted by parasitic nematodes (Crook, 2013). While diapause affects many rhabditids, the Caenorhabditis elegans (C. elegans) model system provides the most detailed dissection of both dauer formation and the developmental pathways that are affected, and this review will focus on C. elegans to represent the nematodes.
After embryogenesis, C. elegans develops through four larval stages (L1-L4) separated by molts before becoming a reproductively mature adult (Byerly et al., 1976). C. elegans can undergo environmentally induced developmental arrest at a number of stages, but dauer is the best example of a diapause stage, with a preparatory phase, long-term developmental arrest, recovery, and post-diapause stages (Baugh and Hu, 2020). Adverse environmental conditions sensed in the L1 stage will trigger entry into the pre-diapause L2d stage (Golden and Riddle, 1984b; Schaedel et al., 2012). During L2d, larvae prepare for diapause, for example by fat storage, and continue to sense their environment. If adverse conditions persist, L2d larvae will molt into the dauer stage (Golden and Riddle, 1984b; Schaedel et al., 2012). Dauer larvae are highly stress-resistant and can survive for months, longer than the lifespan of a worm that developed through the non-dauer trajectory (Cassada and Russell, 1975; Klass and Hirsh, 1976). If favorable conditions are encountered, dauer larvae undergo a recovery process and then proceed with development normally (Cassada and Russell, 1975; Klass and Hirsh, 1976; Liu and Ambros, 1991; Euling and Ambros, 1996; Hall et al., 2010).
In the wild, C. elegans can be found across the globe in temperate regions, where it is located in rich soil, compost, and decomposing vegetation (Frézal and Félix, 2015). C. elegans eats the microorganisms that are abundant in environments that include rotting fruit and stems. A worm born in the presence of a rich food source will develop quickly to adulthood (Félix and Duveau, 2012). Because C. elegans populations exist largely as self-fertilizing hermaphrodites, each worm in these circumstances will produce approximately 300 offspring within about 3–5 days. Thus, in a short time the food will become exhausted.
Young larvae that hatch when food is scarce and the worm population is high will be induced to enter dauer. Dauer larvae can not only survive in the absence of food, but they have dispersal behaviors that increase the chance that they will find another source of food. One dauer-specific dispersal behavior is nictation, where dauer larvae stand on their tails and wave their heads. This behavior increases the chances of associating with a passing invertebrate that can carry the worm to a new location (Félix and Duveau, 2012; Lee et al., 2012). Parasitic larvae in the infective stage also exhibit nictation behavior, reinforcing the similarities between dauer and infective stages (Reed and Wallace, 1965). If dauer larvae successfully move to a new, more favorable location, they will recover, complete development, and produce progeny that will begin a new cycle (Frézal and Félix, 2015). Therefore, the dauer formation decision must be made accurately to allow the population to survive. Failure to enter diapause when food is scarce is likely to lead to death, whereas adoption of diapause when food is plentiful allows time for other animals to consume the available food (Avery, 2014).
The primary sensory cue that induces dauer is a pheromone that is secreted by all non-dauer members of the population (Golden and Riddle, 1982; Kaplan et al., 2011). The pheromone is comprised of a mix of ascarosides (Jeong et al., 2005; Butcher et al., 2007). Ascarosides are derived from the sugar disaccharide ascarylose and then modified with lipid side chains (Ludewig and Schroeder, 2013). The abundance of pheromone is compared to signals from the food source, and it is the pheromone-to-food ratio that dictates dauer formation (Golden and Riddle, 1984a). Finally, these signals are further modulated by temperature, where higher temperatures are more likely to induce dauer formation (Golden and Riddle, 1984a). Nematodes thrive in cooler temperatures; in the lab 15–25°C is the typical range (Stiernagle, 2006).
Like nematodes, insects are a highly abundant and widespread group of animal species. Unlike nematodes, the need for diapause in insects is typically seasonal. In temperate climates insects may spend the winter in diapause, whereas in tropical climates seasonal variations in rainfall may create conditions that necessitate diapause. Therefore, photoperiod is the most important cue that induces diapause, with temperature being the second most important. The availability of food, maternal cues, and other factors can also play a role (Denlinger et al., 2012; Hand et al., 2016).
Unlike in nematodes where diapause occurs midway through larval development, each species of insect has a different stage at which it may undergo diapause, including embryonic, larval, pupal, or adult reproductive diapause. Because there is extensive literature on many insect species and existing excellent comprehensive reviews available (Denlinger et al., 2012; Schiesari and O’Connor, 2013), this paper focuses on three species. The three species chosen are the fruit fly Drosophila melanogaster (D. melanogaster) for its importance as a model organism, the mosquito Culex pipiens (C. pipiens), and the silkmoth Bombyx mori (B. mori). The latter two were chosen for the literature dissecting at least one of the two major hormonal pathways covered in this review.
Drosophila melanogaster is arguably the most intensely studied insect model organism. This species arose in tropical regions in Africa, but has spread widely and is now present in most tropical and temperate regions across the globe (David and Capy, 1988). In response to cold temperatures and reduced photoperiod, young adult females can undergo a reproductive diapause, where ovarian development is arrested at the previtellogenic stage (Saunders et al., 1989; Saunders and Gilbert, 1990). A reproductive dormancy has also been described in males, but less is known about this system (Kubrak et al., 2016).
In contrast to many insects, diapause in D. melanogaster is more strongly dependent on temperature than photoperiod (Saunders et al., 1989; Emerson et al., 2009; Anduaga et al., 2018; Nagy et al., 2018). Because the response to temperature can be thought of as an immediate stress-response, rather than a programmed developmental arrest, the term “diapause” is sometimes avoided in favor of quiescence or dormancy. However, there are some features of diapause present in this reproductive arrest in D. melanogaster, including stress-resistance, an increase in lipid stores, and lifespan extension (Tatar et al., 2001; Schmidt et al., 2005a, b; Schmidt and Paaby, 2008). The term reproductive diapause will be used for D. melanogaster in this review.
The mosquito C. pipiens lives in temperate regions and adult females undergo a reproductive diapause in response to short photoperiod and cool temperatures sensed during late larval development and early pupal development (Eldridge, 1966; Sanburg and Larsen, 1973; Spiebnan and Wong, 1973). This diapause is similar to that described for D. melanogaster, but photoperiod plays a larger role in inducing diapause, and there is no disagreement that this reproductive arrest is a genuine diapause (Denlinger and Armbruster, 2013). Diapause induces changes in behavior and metabolism, including hypertrophy of the fat body, movement to overwintering sites, and a shift from blood meals to sugar sources such as nectar, with accompanying changes in the production of enzymes involved in digestion (Robich and Denlinger, 2005; Denlinger and Armbruster, 2013; Diniz et al., 2017).
Bombyx mori is a silkmoth that apparently originated from a Chinese strain of B. mandarina but has been domesticated for thousands of years. This species is important economically and also serves as a model organism (Arunkumar et al., 2006; Furdui et al., 2014). Diapause in B. mori occurs during mid-embryogenesis and is maternally programmed. Environmental conditions sensed by the mother during embryogenesis are translated into diapause-regulating signals for the next generation. Diapause-inducing cues include warmer temperatures of 25°C and longer photoperiods, whereas temperatures of 15°C and below and short photoperiods stimulate non-diapause development (Xu et al., 1995b).
Annual killifishes live in Africa and South America in small ponds that can be temporary and present only in the rainy season. Diapause is therefore a mechanism to survive when the water evaporates. Diapausing embryos are packed into the pond sediment and can survive until the water returns.
Two species of annual fishes that have emerged as model systems are Austrofundulus limnaeus from Venezuela and Nothobranchius furzeri from Zimbabwe and Mozambique (Podrabsky et al., 2017; Reichard and Polačik, 2019). Annual killifishes undergo embryonic diapause at three different stages (DI-DIII) (Wourms, 1972). DI occurs early in development, before the embryonic axis is established. DI-promoting environmental cues include anaerobic conditions, low ambient temperatures, and the presence of adult fishes (Wourms, 1972; Levels et al., 1986; Arezo et al., 2017). DII occurs midway through development before the onset of organogenesis. Lower temperatures of approximately 20°C induce DII whereas at 30°C most embryos bypass DII, termed the “escape” developmental trajectory. DIII occurs at the end of embryogenesis, just before hatching, and this diapause seems to occur in nearly every embryo (Wourms, 1972; Podrabsky et al., 2010). DIII may be related to the delayed hatching that can occur in other species without a clear diapause stage (Wourms, 1972; Martin and Podrabsky, 2017).
Under uniform laboratory conditions, embryos in a population are arrested in different diapauses and for different amounts of time, resulting in a mixture of embryos at a range of developmental stages. This range has been thought to represent a bet-hedging strategy to maximize the chance that some embryos will survive despite unpredictable environments. The mechanism behind these differences in growth rate is still unclear (Wourms, 1972; Rowiński et al., 2021). However, a recent study that sampled natural populations of embryos found much tighter developmental synchrony within populations and provided evidence that environmental cues play a much greater role in controlling developmental progression than previously seen in laboratory studies (Polačik et al., 2021).
This review will focus on DII, as this diapause is well-studied and shares the most features with diapause in the invertebrates featured here, including the highest level of stress-resistance and the most facultative (Podrabsky et al., 2016a, b).
The decision to enter diapause occurs in response to sensory cues, and diapause must be adopted across the entire organism. Hormones are thus key to regulating diapause. The details of hormonal regulation of diapause are different across species, and there are many excellent reviews that focus on individual phyla or two-way comparisons (Fielenbach and Antebi, 2008; Denlinger et al., 2012; Schiesari and O’Connor, 2013; Sim and Denlinger, 2013a; Podrabsky et al., 2016b; Baugh and Hu, 2020). An additional important review describes all three phyla, but without the focus on hormonal pathways (Hand et al., 2016). Here, I describe two of the hormonal pathways that play an important role in regulating diapause across nematodes, insects, and fishes: insulin/IGF signaling and nuclear hormone receptor signaling.
Insulin and insulin-like growth factor (IGF) signaling (IIS) is an evolutionarily conserved hormonal pathway that regulates growth, metabolism, and stress-resistance (Figure 1; Oldham and Hafen, 2003; Tothova and Gilliland, 2007; Murphy and Hu, 2013). Insulin, IGF, or insulin-like peptides (ILPs) are expressed in response to environmental signals. When insulin/IGF agonists are plentiful, they bind to insulin or IGF receptors (IR or IGFR) on the surface of target cells. IR/IGFRs are receptor tyrosine kinases that dimerize upon agonist binding, thereby activating phosphoinositide 3-kinase (PI3K). Activated PI3K catalyzes the addition of a phosphate group to phosphatidylinositol-3,4-bisphosphate (PIP2), forming phosphatidylinositol-3,4,5-trisphosphate (PIP3). The phosphatase and tensin homolog (PTEN) catalyzes the reverse reaction, removing a phosphate group from PIP3. When levels of PIP3 are high, it acts as a second messenger and leads to activation of downstream kinases, including the serine-threonine kinase AKT. AKT can regulate a number of targets, including Forkhead box O (FOXO) transcription factors. When phosphorylated by AKT, FOXO proteins are cytosolic and therefore inactive. FOXO transcription factors regulate expression of genes involved in stress resistance, metabolism, and cell-cycle arrest. Perhaps for these reasons, downregulation of IIS and/or activation of FOXO are common themes in diapausing animals across species (Murphy and Hu, 2013; Schiesari and O’Connor, 2013; Sim and Denlinger, 2013a).
Figure 1. The regulation of diapause by insulin/IGF signaling (IIS). Major, conserved components of IIS signaling are shown in favorable environments (left) and unfavorable environments (right). Orange components promote diapause and green components promote non-diapause development. Favorable environments stimulate the production of IGF-I in annual fishes or insulin-like peptides (ILPs) in C. elegans and insects. These ligands activate IIS, leading to the phosphorylation and nuclear exclusion of FOXO transcription factors by AKT. When active FOXO proteins regulate downstream genes involved in diapause. See text for additional details.
The core components of IIS signaling are conserved in animal species (Figure 1). Insulin, IGFs, and/or ILPs are typically expressed from particular cells in response to environmental signals. Secreted insulins/IGFs then travel throughout the body and bind to their cognate receptors. The number of insulins/IGFs and the receptors they bind to differs across species. Vertebrates have seven related ligands: insulin, IGF-I, IGF-II, and four relaxins, though relaxins appear to function via G-protein coupled receptors rather than receptor tyrosine kinases related to IR and IGFR (Hsu et al., 2002). Insulin binds primarily to the insulin receptor. IGFR1 is responsible for the signaling activity of IGFs, while IGFR2 functions as a negative regulator of IIS (Czech, 1989; Lau et al., 1994; Jones and Clemmons, 1995; Vincent and Feldman, 2002). Insects have seven ligands, ILP1-7 and only one receptor (Oldham and Hafen, 2003). In C. elegans, the ligands have expanded to include 40 ILPs and a single receptor, DAF-2/IR. The downstream components are also conserved across phyla, including PI3K, PTEN, AKT, and FOXO. Mammals have four FOXO proteins, while several species of fishes that have been examined so far possess seven FOXO proteins, likely due to the teleost-specific genome duplication event (Brunet et al., 1999; Kops et al., 1999; Oldham and Hafen, 2003; Tothova and Gilliland, 2007; Murphy and Hu, 2013; Gao et al., 2019). The IIS components with known connections to diapause are listed in Table 1 and described in more detail below.
All animal species possess multiple insulin-related genes, and these genes appear to work in combination to regulate diapause. In contrast, in the species where IIS signaling has been shown to regulate diapause, these ligands appear to signal through a single receptor. Worms and insects have only a single insulin/IGF receptor, and in fishes IGFR1 is the key receptor that regulates diapause, as described below.
The combinatorial nature of the ligands is most apparent in C. elegans, whose genome encodes 40 ILPs. C. elegans ILPs have been studied singly and in combination to reveal a complex network of IIS agonists and antagonists that regulate diapause entry, and a slightly different set that regulates diapause exit (Pierce et al., 2001; Cornils et al., 2011; Fernandes-de-Abreu et al., 2014). ILPs that regulate diapause are expressed in sensory neurons, and their expression changes in response to environmental cues (Pierce et al., 2001; Li et al., 2003; Cornils et al., 2011). The best-studied regulators of dauer diapause include daf-28, ins-6, and ins-1. daf-28 and ins-6 both promote IIS to oppose dauer entry and promote dauer exit; however, daf-28 plays a more prominent role in dauer entry and ins-6 plays a more prominent role in dauer exit (Malone et al., 1996; Li et al., 2003; Cornils et al., 2011). By contrast, ins-1 antagonizes IIS to promote dauer entry and oppose dauer exit (Pierce et al., 2001; Cornils et al., 2011). If the human insulin protein is introduced into C. elegans it also antagonizes IIS, similar to ins-1, demonstrating the conservation between ILPs across species (Pierce et al., 2001). In addition to these three ILPs that have been studied in detail, systematic studies have shown that many other ILPs also play more subtle roles in regulating dauer entry and/or exit (Pierce et al., 2001; Fernandes-de-Abreu et al., 2014). ILP signaling converges on the sole C. elegans IR, DAF-2, which was discovered on the basis of its effect on entry to dauer diapause. Reduction-of-function mutations in daf-2 cause a dauer formation phenotype whereby larvae enter dauer diapause even in conditions that normally promote non-dauer development (Riddle, 1977). Therefore, active DAF-2/IR signaling promotes non-diapause development.
In insects, the role of IIS in regulating diapause has been studied extensively with respect to the reproductive diapause that occurs in adult mosquitos (C. pipiens) and fruit flies (D. melanogaster). In C. pipiens, levels of two ILPs, ILP-1, and ILP-5, are reduced during diapause compared with non-diapausing animals. RNAi of ILP-1 but not ILP-5 is sufficient to induce adult diapause as measured by ovarian maturation and follicle length (Sim and Denlinger, 2009). Similarly, RNAi of IR induces adult diapause by the same measures (Sim and Denlinger, 2008). Therefore, IIS signaling opposes diapause in C. pipiens.
Similarly, in D. melanogaster, loss of dilps 1-5 strongly induces reproductive diapause. Single dilp2 and dilp5, but not dilp1, mutations are sufficient to induce reproductive diapause at low penetrance, whereas overexpression of dilp2 and dilp5 from any tissue strongly suppresses reproductive diapause (Kubrak et al., 2014; Liu et al., 2016; Schiesari et al., 2016). Furthermore, hypomorphic mutations in InR or the insulin receptor substrate protein Chico cause reproductive diapause entry (Schiesari et al., 2016). Surprisingly, although dILPs functionally oppose reproductive diapause, mRNA levels of the five ILPs tested (dilp1, dilp2, dilp3, dilp5, dilp6) are all upregulated during reproductive diapause and then return to non-diapause levels after recovery from reproductive diapause (Kubrak et al., 2014; Liu et al., 2016). This upregulation may be a compensatory response to reduced IIS signaling under diapause-inducing conditions (Schiesari et al., 2016).
Another insect that undergoes reproductive diapause is the bumblebee Bombus terrestris. In this species, genes within the IIS pathway were upregulated in post-diapause animals compared to diapause. Furthermore, injection of ILPs stimulated metabolic changes consistent with non-diapause development and increased the numbers of offspring produced (Chen et al., 2021). Finally, there are many insect species where reduced expression of ILPs or IR has been reported to correlate with diapause at different stages of development, consistent with the notion that downregulation of IIS is a broadly conserved phenomenon. Some examples include egg diapause in the aphid Acyrthosiphon pisum and in Locusta migratoria (Barberà et al., 2019; Hao et al., 2019), embryonic diapause in B. mori (Gong et al., 2020), and pre-pupal diapause in leafcutting bees, Megachile rotundata (Cambron et al., 2021).
In the annual fish A. limnaeus, levels of IGF-I are reduced before and during DII compared to escape embryos at the same stages. After recovery, IGF-I levels climb, eventually surpassing those of escape embryos (Woll and Podrabsky, 2017). IGF-II levels are similar in escape and diapause embryos before and during DII, but are higher post-DII than in escape embryos. Higher levels of IGF after diapause may be related to the catch-up growth that is seen in post-diapause embryos. In addition to the correlation of IGF levels, treatment of embryos with an IGF1R inhibitor induces diapause in a dose-dependent manner. Pre-DII and DII embryos induced by this treatment show similarities to naturally induced DII, including slowed heart rate and slowed somitogenesis. However, there were a few differences, including the presence of melanocytes and hemoglobin in circulating blood, indicating that IGF signaling is not the sole regulator of diapause (Woll and Podrabsky, 2017).
The role of downstream components of IIS in the regulation of diapause has been studied primarily in C. elegans, where the dauer diapause-regulating pathways have been worked out in detail (Fielenbach and Antebi, 2008; Murphy and Hu, 2013). Each component functions as expected given its role in insulin/IGF signaling. Specifically, when active, the orthologs of PI3K and AKT oppose dauer formation, whereas the ortholog of PTEN promotes dauer formation. The single PI3K in C. elegans is encoded by age-1, also called daf-23 (Morris et al., 1996). age-1 was first identified based on the long-lived phenotype conferred by mutants (Klass, 1983). Separately, this gene was identified in a screen for mutants that enter dauer in conditions that normally promote non-dauer development (Gottlieb and Ruvkun, 1994). Similarly, the single PTEN ortholog, daf-18 was identified on the basis of the inability of a daf-18(-) mutant to enter dauer under dauer-inducing conditions (Riddle et al., 1981). There are two AKT homologs in C. elegans, akt-1 and akt-2 that function partially redundantly to oppose dauer diapause. Loss of akt-1 produces a weak dauer-constitutive phenotype, whereas loss of both akt-1 and akt-2 produces a very strong constitutive dauer diapause phenotype (Ailion and Thomas, 2003; Oh et al., 2005).
In insects, the PI3K ortholog, Dp110 was identified based on the variation in reproductive diapause rates between two natural strains of D. melanogaster, one that enters reproductive diapause more frequently than the other. Dp110 appears to be one locus responsible for this difference (Williams et al., 2006). Although the molecular changes in Dp110 that lead to this reproductive diapause phenotype were not clear, reducing the dosage of Dp110 by half increases the percentage of flies adopting reproductive diapause, whereas misexpressing Dp110 in neurons decreases the percentage of flies adopting reproductive diapause (Williams et al., 2006).
One common output of IIS is regulation of the FOXO transcription factors. IIS negatively regulates FOXO, such that when IIS is active, FOXO proteins are phosphorylated by AKT, which triggers nuclear exit and therefore inactivity (Manning and Cantley, 2007). Therefore, low levels of IIS are required for FOXO nuclear localization and activity. Across species, FOXO transcription factors promote diapause by directly and indirectly controlling the expression of numerous genes that are involved in diapause. Furthermore, if situations are contrived whereby animals within diapause have reduced FOXO activity, those animals lose key characteristics of diapause, suggesting that FOXO promotes both the decision to enter diapause and the physiological and structural changes that accompany diapause, as explained below.
The specific role of FOXO proteins in diapause has not been studied in fishes, but there is a great deal of information from invertebrate species, which typically have a single FOXO ortholog. In C. elegans, the FOXO protein is encoded by daf-16. Like most of the genes in the IIS pathway, daf-16 was also discovered on the basis of its dauer formation phenotype. Whereas loss-of-function mutations in daf-2 or other IIS components cause dauer formation even in environments favorable for growth, loss-of-function mutations in daf-16 cause the opposite phenotype: inability to enter dauer diapause even in dauer-inducing conditions. Furthermore, when double mutants are created, the daf-16 dauer-defective phenotype is observed (Murphy and Hu, 2013). The complete suppression of the daf-2(-) dauer-constitutive phenotype by loss of daf-16 indicates that the major output of IIS with respect to regulation of diapause is daf-16/FOXO.
While daf-16(-) larvae do not readily enter dauer diapause, it is possible to obtain daf-16(-) dauer larvae by making use of dauer-constitutive mutations outside of the IIS pathway, or by the use of very potent dauer-inducing cues. However, these daf-16(0) dauer larvae display defects in some aspects of the remodeling that occurs as part of dauer morphogenesis, demonstrating that daf-16/FOXO is not only responsible for the decision to enter dauer but for some of the aspects of diapause itself. For this reason, daf-16(-) dauer larvae have been referred to as “partial dauers” (Vowels and Thomas, 1992; Larsen et al., 1995; Ogg et al., 1997). For example, daf-16 mutants lack the particular collagens in their cuticle that are normally enriched in dauer larvae, and perhaps for this reason they are more sensitive to environmental assaults (Gottlieb and Ruvkun, 1994; Nika et al., 2016; Wirick et al., 2021).
The specific gene expression changes that occur in the absence of daf-16/FOXO have been studied primarily in non-diapause contexts: adult worms with activated DAF-16 due to a mutation in IR are compared to IR mutants that lack daf-16. As in other species, daf-16 promotes expression of genes involved in stress resistance and metabolism (Tepper et al., 2013). A recent study examined the changes in gene expression of dauer larvae with and without daf-16. In this context, genes related to collagens and signaling were differentially expressed (Wirick et al., 2021).
In mosquitos, levels of FOXO are increased in the fat body of diapausing females, compared to non-diapause animals (Sim and Denlinger, 2013b). Fat storage is an important component of diapause and appears to be dependent on FOXO because knockdown of FOXO with dsRNA in diapausing females causes a reduction in lipid stores (Sim and Denlinger, 2008). These females also showed reduced survival over time in diapause. Reduced survival may be due to insufficient fat storage, but because addition of an oxidoreductase (Mn(III)TBAP) partially rescues survival, oxidative stress is also implicated (Sim and Denlinger, 2008). FOXO promotes resistance to oxidative stress across species. Genes bound by FOXO during diapause were identified by ChIP-seq. These target genes are involved in stress-resistance, metabolism, longevity, cell-cycle, development, and circadian rhythm (Sim et al., 2015). RNAi knockdown of three of these targets reduced glycogen and lipid levels during diapause, consistent with the idea that FOXO regulation of target genes is important for diapause characteristics (Olademehin et al., 2020). In Drosophila, a FOXO-responsive luciferase reporter is upregulated during reproductive diapause. Abdomens isolated from cold-reared flies in reproductive diapause showed six-fold more activity of this reporter than non-diapausing flies reared under standard lab conditions (Schiesari et al., 2016).
In Locusta migratoria egg diapause, if FOXO is knocked down in the mother by RNAi under diapause-inducing conditions (short photoperiod), the percentage of eggs that enter diapause is reduced, indicating that FOXO promotes entry into diapause (Hao et al., 2019). Consistent with the activity of FOXO being important for diapause, the known FOXO target, MnSod/sod2, was found to be upregulated under diapause-inducing conditions (Hao et al., 2019).
In the cotton bollworm Helicoverpa armigera pupal diapause, levels of total FOXO are high but phospho-FOXO levels are low, indicating that FOXO is in its active state. Interestingly, this regulation occurs by a different mechanism than the typical IIS pathway (Zhang X.-S. et al., 2017). Although ILP levels are low, these ILP levels do not appear to regulate FOXO activity via Akt, because phospho-AKT levels are high in diapause. Instead, high levels of ROS lead to phosphorylation of Akt as well as high levels of protein arginine methyltransferase 1 (PRMT1). PRMT1 methylates FOXO, thus abrogating phosphorylation by Akt. In this way, FOXO remains active to promote diapause at the same time as active Akt can phosphorylate other targets (Zhang X.-S. et al., 2017).
Nuclear hormone receptors (NHRs) are ligand-gated transcription factors comprised of a DNA-binding domain and a ligand-binding domain. The DNA-binding domain directs binding of the NHR to target sequences called hormone response elements (HREs), while ligand binding modulates the regulation of target gene expression. Class I receptors are activated by the binding of steroid ligands but inactive in the absence of ligand (Mangelsdorf et al., 1995). Class II receptors are also activated by ligand binding. However, in the absence of ligand, Class II receptors act with corepressors to repress transcription of target genes. Upon ligand binding, corepressors are released, and subsequent binding of coactivators switches the NHR from a transcriptional repressor to a transcriptional activator (Figure 2). Class II receptors typically function as heterodimers with the retinoid X receptor (RXR) (Mangelsdorf and Evans, 1995; Mangelsdorf et al., 1995).
Figure 2. The regulation of diapause by nuclear hormone receptor signaling (NHR). In favorable environments (left), enzymes that catalyze the production of the lipid ligands are present, and these ligands are produced. Because the ligands can freely pass through the plasma and nuclear membrane, they are able to bind to their target NHRs. Ligand-bound NHRs function as transcriptional activators and turn on expression of genes that promote non-dauer development. In unfavorable environments (right), expression of the required enzymes is reduced and little ligand is present. Unliganded NHRs bind to corepressors and repress transcription of target genes, thus promoting diapause. Orange components promote diapause and green components promote non-diapause development. Note that in some insect species, NHR signaling functions opposite to what is described here, in that NHR signaling promotes diapause. See text for additional details.
As described above, NHR activity is controlled primarily by the presence or absence of ligand. NHR ligands are small, lipophilic molecules that can pass through membranes without the aid of a transport protein (Figure 2). Therefore, signaling is regulated primarily by controlling the production and release of ligand. These ligands are derived from cholesterol or related molecules by a series of biosynthetic steps, as elaborated on in the following subsections. The expression of enzymes that catalyze the biosynthesis of the mature, active form of the ligand is regulated to control NHR activity. The components of NHR signaling known to be associated with diapause within the species focused on in this review are listed in Table 2 and explained in more detail below.
Class II NHRs play important roles in the regulation of diapause across phyla. The NHRs implicated in diapause are phylogenetically related and include the vitamin D receptor in annual fishes, the ecdysone receptor (EcR) in insects, and DAF-12 in C. elegans. In most of these cases, low levels of ligand are associated with diapause whereas ligand-bound NHR leads to non-diapause development (Figure 2 and Table 3).
In C. elegans, daf-12 was discovered on the basis of the dauer formation phenotypes observed in daf-12 mutants. Notably, both dauer-defective and dauer-constitutive alleles of daf-12 were isolated (Antebi et al., 1998). Dauer defective alleles are mutants that failed to enter diapause even in diapause-inducing conditions. Dauer-constitutive alleles enter diapause even in conditions favorable for growth. This complexity stems in part from the two activities of NHRs as activators and repressors. Null alleles remove both activator and repressor functions, whereas other alleles can affect one function more than the other. Null alleles do not enter diapause under any known condition, indicating that daf-12 activity is required for diapause. By contrast, all of the dauer-constitutive alleles disrupt the ligand binding domain, suggesting that it is the unliganded version of DAF-12 that is required for dauer formation (Antebi et al., 2000). Consistent with this idea, the DIN1S co-repressor was found to bind unliganded DAF-12 and to be required for dauer formation (Ludewig et al., 2004).
As mentioned above, most Class II NHRs function as heterodimers with RXR proteins. However, C. elegans lacks a clear RXR ortholog despite the expansion of NHR-encoding genes in C. elegans: 284, compared to 48 NHRs in humans (Antebi, 2015). NHRs without clear orthology to RXR have been suggested to play a similar role in some cases, however, whether DAF-12 functions as a homo- or heterodimer is still unknown (Antebi, 2015). DAF-12 does possess a conserved dimerization domain, and the DNA response element to which DAF-12 binds is consistent with either homo- or heterodimerization (Antebi et al., 2000; Shostak et al., 2004; Hochbaum et al., 2011).
In insects, EcR signaling has been studied in depth in D. melanogaster and other insects, primarily for its role in molting. Indeed, ecdysteroids are often referred to as molting hormones. In insects, the EcR functions as a heterodimer with the RXR ortholog, Ultraspiracle (USP) (Oro et al., 1990; Koelle et al., 1991; Yao et al., 1992; Thomas et al., 1993). There are three isoforms of EcR: A, B1, and B2, that share DNA-binding and ligand-binding domains but differ at the N-terminus. These isoforms differ in expression pattern and biochemical activity; however, they all function to activate transcription of target genes when bound to ligand, and to repress transcription when ligand-free (Talbot et al., 1993). In some contexts, the unliganded form of EcR is specifically required (Mansilla et al., 2016).
With respect to diapause, EcR signaling plays a regulatory role in many insects, a few of which will be highlighted here. These include reproductive diapause in D. melanogaster and embryonic diapause in B. mori. For a more comprehensive view of EcR signaling regulating diapause across insect species (see Denlinger et al., 2012). Unlike in C. elegans, functional consequences of reduced EcR signaling have not been described in the context of insect diapause, and instead more work has been done manipulating hormone levels, as described in section “Nuclear Hormone Receptor Signaling Ligands and Biosynthetic Enzymes and the Regulation of Diapause.”
In the annual fish A. limnaeus, a role for the vitamin D receptor (VDR) in the regulation of diapause II (DII) was recently described (Romney et al., 2018). There are two VDR-encoding genes in A. limnaeus: VDR-A and VDR-B. These receptors also bind to target DNA as homodimers or heterodimers, where heterodimerization with RXR is the predominant mode (Carlberg et al., 1993; Carlberg and Campbell, 2013). In A. limnaeus embryos the expression of VDR-A and RXR does not change between pre-diapause and pre-escape embryos. However, the expression of VDR-B is increased in pre-diapause embryos relative to those on the escape trajectory (Romney et al., 2018).
As described in the prior section, in the examples given, active NHR signaling promotes non-diapause development. The control of this signaling appears to occur primarily at the level of production of the active ligand. In insects, the bulk of the studies on the role of EcR signaling in diapause have focused on the ligand and the enzymes necessary to produce the active form. The most active EcR ligand is 20-hydroxyecdysone (20E). In Drosophila, 20E is synthesized from cholesterol by a series of enzymatic reactions. These reactions begin with cholesterol or plant-derived sterols taken up from the environment, since insects cannot synthesize cholesterol themselves (Niwa and Niwa, 2014). The first step in the pathways is catalyzed by Rieske oxygenase Neverland (Nvd) which converts cholesterol to 7-dehydrocholesterol. Next is a “black box,” between 7-dehydrocholesterol and 5β-ketodiol, where the precise reactions are not known. However, the short-chain dehydrogenase Non-molting glossy/Shroud (Nm-g/Sro) and the cytochrome P450 enzymes Spook and Spookier (Spo, Spok) are thought to catalyze reactions within the black box. After that, the rest of the biosynthetic pathway is known, and each step is catalyzed by a series of cytochrome P450 enzymes, including Phantom (Phm), Disembodied (Dib), Shadow (Sad), and Shade (Shd), in that order (Niwa and Niwa, 2014).
To regulate molting, ecdysone is synthesized in the prothoracic glands and then ecdysone is released into the hemolymph. Ecdysone is then converted into 20E in target tissues around the body (Petryk et al., 2003). However, ecdysone can also be synthesized in the ovaries. In D. melanogaster, the strongest evidence for a role for ecdysone signaling in reproductive diapause comes from experiments where injection of diapausing animals with 20E caused recovery, as assessed by the resumption of vitellogenesis (Richard et al., 2001a). Additionally, levels of 20E are low in reproductive diapause animals compared with non-diapause animals. When flies in reproductive diapause are stimulated to recover, the 20E levels rise rapidly (Richard et al., 1998).
Similarly, in B. mori, high levels of 20E are found in non-diapause embryos, but not diapause embryos. Instead, diapausing embryos contain high levels of 20E precursors or inactive derivatives. Furthermore, treatment of diapausing embryos with 20E can induce the resumption of development and recovery from diapause (Makka et al., 2002; Sonobe and Yamada, 2004). Therefore, regulation of the production of 20E is necessary to control diapause entry. Because the prothoracic gland has not yet developed in these embryos, the regulation of 20E synthesis differs from the canonical pathway. 20E in embryos is derived from two sources. First, inactive, phosphorylated forms of 20E and its precursors are bound to yolk proteins and maternally packaged into the embryo. Second, 20E is synthesized de novo. In both cases, the regulation of enzymes determines whether active 20E is produced. The ecdysteroid-phosphate phosphatase (EPPase) is required to activate the phosphorylated molecules, whereas the enzyme 20-hydroxylase (E20OHase, also called Shade in some species) is required for de novo synthesis. The expression of these enzymes is upregulated over time in non-diapause embryos whereas expression remains low during diapause (Sonobe and Yamada, 2004; Maeda et al., 2008; Gu et al., 2021).
Other species with larval and pupal diapause show similar effects whereby increased levels of 20E have been shown to correlate with and/or actively promote non-diapause development. Since EcR signaling is necessary for progression through larval molts and for metamorphosis, it makes sense that removing 20E would allow for developmental arrest. Somewhat surprisingly, however, in some insect species, the role for EcR signaling is reversed, and high levels of 20E are required to maintain diapause. For example, this mode of diapause regulation was shown for the obligate diapause at the end of embryogenesis in the gypsy moth, Lymantria dispar (Denlinger et al., 2012; Schiesari and O’Connor, 2013).
In C. elegans the first two ligands that activate DAF-12 signaling to be discovered were two 3-Keto-4-Cholestenoic Acids termed Δ4-dafachronic acid and Δ7-dafachronic acid (Δ4-DA and Δ7-DA) (Motola et al., 2006). The term “dafachronic” comes from the two major roles of DAF-12: the regulation of diapause and the regulation of developmental timing (see section “Developmental Changes Accompanying Diapause in C. elegans”). More recent work identified additional DAs, the most prominent being Δ1,7-DA. This study failed to find Δ4-DA, suggesting that ligand may be less relevant in endogenous contexts (Mahanti et al., 2014).
Fewer steps in the synthesis of DAs are known in detail in comparison to the synthesis of 20E. However, some key enzymes show similar requirements across species. First, the Rieske oxygenase DAF-36 is required for the synthesis of Δ7-DA. Similar to the role of Nvd in ecdysone synthesis, this enzyme catalyzes the first reaction within this biosynthetic pathway: conversion of cholesterol to 7-dehydrocholesterol (Rottiers et al., 2006; Wollam et al., 2011; Yoshiyama-Yanagawa et al., 2011). Indeed, these enzymes are functionally conserved as addition of daf-36 to D. melanogaster with a mutation in nvd rescues the lethal phenotype (Yoshiyama-Yanagawa et al., 2011). The short chain dehydrogenase DHS-16 works further downstream in this pathway. The enzymes involved in the production of other dafachronic acids are unknown. The hydroxysteroid dehydrogenase HSD-1 was proposed to be involved in the production of Δ4-DA, but subsequent work raises doubts about this notion. At the end of the pathway, the cytochrome P450 enzyme DAF-9 is required to catalyze the final step in biosynthesis of both Δ4-DA and Δ7-DA (Antebi, 2015). The expression of daf-9 is highly regulated during dauer formation, where mildly stressful conditions increase daf-9 expression, but more severe, dauer-inducing conditions drastically reduce daf-9 expression, leading to a decrease in DA production and entry into dauer diapause (Gerisch et al., 2001; Gerisch and Antebi, 2004; Schaedel et al., 2012).
In vertebrates, biosynthesis of the active VDR ligand 1,25(OH)2D3 is similar in many respects to the biosynthesis of the invertebrate NHR ligands. Biosynthesis in vertebrates also begins with the conversion of cholesterol to 7-hydroxycholesterol, in this case by the action of the dehydrocholesterol reductase enzyme, DHCR7. Unlike in invertebrates, the next step of the biosynthetic pathway requires UVB light, which enables the conversion of 7-hydroxycholesterol to pre-vitamin D3. Once pre-vitamin D3 is produced, the remainder of the biosynthetic steps are catalyzed by a series of cytochrome P450 enzymes, similar to the invertebrate pathway (Tuckey et al., 2018; Saponaro et al., 2020).
Within the annual fish A. limnaeus, expression of the active VDR ligand 1,25(OH)2D3 is reduced in pre-diapause embryos compared to pre-escape embryos. Consistent with this reduction, the expression of several biosynthetic enzymes necessary to produce 1,25(OH)2D3 are also reduced (Romney et al., 2018). This difference in expression is functionally relevant because incubating embryos reared under diapause-inducing conditions with 1,25(OH)2D3 at picomolar concentrations strongly reduced the number of embryos that underwent diapause (Romney et al., 2018). Remarkably, performing a similar experiment with C. elegans Δ4-dafachronic acid also increased the number of embryos following the non-diapause, escape developmental trajectory. This effect was specific because incubating embryos with ligands for the pregnane X, farnesoid X, and liver X receptors, which are closely related to VDR, was ineffective at preventing diapause (Romney et al., 2018). Furthermore, treating A. limnaeus embryos grown in non-diapause conditions with dafadine A causes embryos to enter DII. Dafadine A is a compound that inhibits the activity of the cytochrome P450 enzyme DAF-9 and its mammalian counterpart CYP27A1 (vitamin D3 25-hydroxylase) (Luciani et al., 2011). The diapause-inducing effect of dafadine A in A. limnaeus can be countered by addition of 1,25(OH)2D3, consistent with the interpretation that dafadine A promotes diapause by interfering with the production of 1,25(OH)2D3 (Romney et al., 2018). Taken together, these results demonstrate the conserved nature of NHR signaling and its effect on diapause in A. limnaeus and C. elegans.
As described in sections “Insulin/Insulin-Like Growth Factor Signaling and Nuclear Hormone Receptor Signaling,” both IIS and NHR pathways regulate development across species. Therefore, there must be interactions between these pathways. Furthermore, additional hormonal pathways contribute to the regulation of diapause. This section provides a brief overview of how those pathways fit together, focusing on the invertebrate systems which lend themselves to in-depth genetic analysis.
Caenorhabditis elegans geneticists have worked out the major regulators of dauer formation in detail. In addition to the IIS and NHR/DAF-12 pathways described in the previous sections, TGFβ signaling is also involved. In brief, TGFβ and IIS pathways operate largely in parallel and both pathways converge to regulate DAF-12 (Figure 3A; Baugh and Hu, 2020; Fielenbach and Antebi, 2008).
Figure 3. Crosstalk between diapause-regulating pathways in C. elegans (A) and C. pipiens (B). Genetic models outlining the interactions between signaling pathways known to regulate diapause in these two species. Orange components promote diapause and green components promote non-diapause development. In C. pipiens, gray arrows indicate interactions that were inferred from a different mosquito species. See text for details.
Favorable environmental cues lead to the production of both DAF-7/TGFβ and multiple insulin-like peptides in sensory neurons (Ren et al., 1996; Schackwitz et al., 1996; Cornils et al., 2011; Murphy and Hu, 2013; Ritter et al., 2013; Fernandes-de-Abreu et al., 2014; Zheng et al., 2018). These signals are released and then received in multiple tissues where DAF-7/TGFβ blocks activity of the downstream DAF-3/SMAD-DAF-5/Ski complex, and insulin signaling blocks the activity of the downstream DAF-16/FOXO transcription factor (Kimura et al., 1997; Lin et al., 1997; Ogg et al., 1997; Patterson et al., 1997; Pierce et al., 2001; da Graca et al., 2004). Both IIS and TGFβ pathways regulate the expression of daf-9/CYP, which encodes the last enzyme required to produce dafachronic acid, the ligand for the DAF-12 nuclear hormone receptor (Gerisch et al., 2001; Gerisch and Antebi, 2004; Mak and Ruvkun, 2004; Motola et al., 2006). As described above, ligand-bound DAF-12 promotes continuous (non-dauer diapause) development whereas ligand-free DAF-12 is required for dauer formation (Antebi et al., 1998, 2000; Ludewig et al., 2004). IIS appears to also act in parallel to daf-12 because when both daf-2/IR and daf-12/NHR signaling are compromised, larvae arrest development but do not enter dauer. Overexpression of daf-9 in a daf-2 mutant background produces a similar arrested phenotype that is different from dauer diapause (Larsen et al., 1995; Gems et al., 1998; Gerisch et al., 2001; Gerisch and Antebi, 2004).
In insects, another major regulator of diapause is juvenile hormone (JH). JH is a sesquiterpenoid that is produced in a group of endocrine organs called the corpora allata (CA) in response to the hormone allatotropin (Flatt et al., 2005; Bendena et al., 2020). JH is then secreted and travels through the hemolymph to act on the ovaries, stimulating ovarian development and vitellogenesis. Since reproductive diapause occurs prior to vitellogenesis, it is not surprising that JH is a central regulator of reproductive diapause across insect species (Denlinger et al., 2012).
Juvenile hormone and Insulin/IGF signaling exhibit an interesting and complex relationship in C. pipiens (Figure 3B). During diapause in C. pipiens, JH levels are low, and addition of synthetic JH can induce exit from diapause (Spielman, 1974). Addition of a JH analog is effective at stimulating diapause exit even in IIS mutants, indicating that IIS regulates diapause formation upstream of JH (Sim and Denlinger, 2008, 2009). However, both IIS and JH signaling appear to converge on the same target: negative regulation of FOXO. Negative regulation of FOXO by IIS is expected, but the role of JH is novel. Addition of JH to diapausing animals reduces FOXO levels in the fat body (Sim and Denlinger, 2013b). FOXO appears to be the most direct regulator of diapause genes, including genes that regulate stress-resistance, metabolism, longevity, and other key characteristics of diapause (Sim et al., 2015).
Finally, there is a potential connection to EcR signaling in C. pipiens as well. This connection was proposed by Sim and Denlinger and is based on work done in another mosquito species, Aedes aegypti (A. aegypti) (Sim and Denlinger, 2013b). In this species, a blood meal enables reproduction by stimulating vitellogenesis. Vitellogenesis requires EcR signaling and the EcR/USP heterodimer directly binds to the promoter of genes encoding yolk proteins in the fat body. JH expression promotes expression of the NHR bFTZ-F1, which in turn recruits the FISC p160/SRC coactivator. These proteins form a complex with EcR and USP at the promoters of target genes and are required for full EcR activation (Zhu et al., 2006). If the same process occurs in C. pipiens in the context of diapause, that would forge a connection between the signaling pathways described here.
Similar to the situation in C. pipiens, JH levels are reduced in D. melanogaster in reproductive diapause. Furthermore, addition of JH to diapausing animals induces recovery from reproductive diapause (Saunders et al., 1990). However, EcR was later proposed to be more directly involved in the regulation of reproductive diapause than JH because levels of ecdysteroids rose more rapidly after stimulation to recover from reproductive diapause, and addition of ecdysteroids to diapausing animals was more effective at terminating reproductive diapause than addition of JH (Richard et al., 1998, 2001b). Unlike in C. pipiens, the IIS pathway has been proposed to act downstream of JH and EcR signaling because loss of the insulin receptor substrate protein Chico induces reproductive diapause in an ovary-autonomous manner, suggesting that external signals such as JH and 20E do not affect reproductive diapause in this mutant context. chico mutant ovaries did not develop upon transplantation to a wild-type host, whereas wild-type ovaries did develop when transplanted to a chico mutant host. Furthermore, chico mutants expressed normal levels of JH and ecdysteroids (Richard et al., 2005). Consistent with the notion that IIS is downstream of JH, the addition of a JH inhibitor did affect the expression of dilp1, however, the functional consequences of this observation are unclear (Liu et al., 2016).
Diapause in B. mori is controlled by a neuropeptide called diapause hormone (DH). This mode of regulation is well studied, though uncommon in other insect species (Denlinger et al., 2012). B. mori diapause is transgenerationally programmed (see section “Diapause in Insects: Drosophila melanogaster, Culex pipiens, and Bombyx mori”). Mothers reared in diapause-inducing conditions during their own embryonic development will express DH in the subesophageal ganglion during the pupal stage. DH is released and then received in the ovary via the DH receptor, a G-protein coupled receptor. This stimulus prompts the developing ovaries to induce diapause (Xu et al., 1995b; Yamashita et al., 2001; Homma et al., 2006; Denlinger et al., 2012; Sato et al., 2014). A connection between DH and EcR signaling has been proposed, but experiments testing this connection have yielded few conclusions, such that the relationship between these pathways is still unclear (Xu et al., 1995a; Shiomi et al., 2015). However, MEK/ERK signaling is needed to terminate diapause, and this process appears to occur upstream of EcR signaling (Fujiwara et al., 2006a, b; Schiesari and O’Connor, 2013). Additionally, the IIS pathway has been implicated in diapause in B. mori, but it is unclear how this function fits with DH or EcR pathways (Zheng et al., 2016; Gu et al., 2019).
Unlike an immediate response to stress, diapause involves an alternate developmental trajectory, including a preparatory stage prior to diapause, the diapause itself, recovery, and post-diapause development. Each stage can differ from its non-diapause equivalent in terms of morphology, metabolism, and rate of development. Not surprisingly, differences in gene expression often exist between diapause and non-diapause animals at equivalent developmental stages. Developmental pathways must accommodate these changes to allow the normal completion of development as a reproductively mature adult. Across species, the pre-diapause preparatory stage is typically marked by a slower rate of development, metabolic changes and accumulation of lipid stores, and morphological changes. During diapause, development is halted. After diapause, animals must quickly resume growth and development. In C. elegans, the same hormonal pathways that regulate diapause appear to also modulate developmental pathways to accommodate diapause, as described below.
In A. limnaeus, N. furzeri, and other annual fishes that undergo facultative DII, visible differences between embryos in the diapause and escape trajectories become apparent around the 18-somite stage. In particular, growth of the head continues more rapidly in escape embryos, and both the length and the width of the pre-DII embryos increase very little until diapause is reached at approximately the 38-somite stage (Podrabsky et al., 2010, 2017; Furness et al., 2015). Other pre-diapause developmental changes include a lack of melanocyte production and reduced heart rate in pre-DII embryos relative to escape embryos (Podrabsky et al., 2010, 2017; Furness et al., 2015).
The developmental changes that occur prior to DII are accompanied by changes in chromatin structure and gene expression (Toni and Padilla, 2016; Romney and Podrabsky, 2018; Romney et al., 2018; Hu et al., 2020). With respect to development-related gene expression, in N. furzeri, genes involved in the Notch, Wnt, FGF, SHH & IHH, TGF, and retinoic acid developmental pathways were all downregulated in pre-DII embryos compared to escape embryos (Hu et al., 2020). In A. limnaeus, the expression of microRNAs was examined in pre-DII and escape embryos. Some variants in the miR-10 and miR-430 families were expressed more highly in escape embryos. miR-10 targets Hox genes, whereas miR-430 targets genes involved in the maternal-to-zygotic transition (Romney and Podrabsky, 2018).
Developmental changes during DII have not been described, other than the expected developmental arrest. Upon recovery from DII, cells throughout the embryo re-enter the cell cycle and resume development. Post-DII embryos develop more rapidly than escape embryos in order to catch up in size. By the stage where the embryo covers approximately one half the surface of the yolk, morphological differences between DII and escape embryos are no longer apparent (Podrabsky et al., 2010, 2017; Dolfi et al., 2019). Levels of IGF-I and IGF-II are both increased after DII relative to escape embryos, suggesting that IIS could promote catch-up growth (Woll and Podrabsky, 2017).
In C. pipiens, egg follicle length at the onset of diapause is slightly longer than that of non-diapausing animals. However, this length increases rapidly in non-diapause development, whereas during diapause there is no increase for the first 12 days and a very slight increase thereafter (Meuti et al., 2018). mRNA and small RNA sequencing of diapausing and non-diapausing females identified differentially expressed genes encoding proteins and microRNAs. These changes primarily affected genes involved in metabolic processes (Kang et al., 2016; Meuti et al., 2018). These effects are expected given the metabolic changes that occur during diapause. With respect to development, some microRNAs that promote ovarian development were differentially expressed. miR-8-3p and miR-275-3p were downregulated in young, pre-diapause adults, and miR-8-3p, miR-275-3p, and miR-375-3p were all downregulated during diapause compared to non-diapause females (Meuti et al., 2018). In B. mori, embryos enter diapause beginning approximately 48 h after oviposition. During the day leading up to diapause, the color of the embryos shifts from pale yellow to reddish (Zhang H. et al., 2017; Gong et al., 2020). mRNA sequencing experiments performed during the pre-diapause pupal stage identified genes involved in the Hippo pathway that regulates organ size. The upstream regulator lft was downregulated and the downstream regulator hth was upregulated in pre-diapause animals. Genes involved in regulating metabolism were also differentially regulated (Chen et al., 2017).
Caenorhabditis elegans development is understood in detail, in part due to the invariant cell lineage that has been mapped, so that individual cells can be identified and followed throughout development (Sulston and Horvitz, 1977; Kimble and Hirsh, 1979; Sulston et al., 1983). The ability to study development at the single-cell level coupled with the powerful genetic tools available in this model organism has resulted in some of the most detailed understanding currently available of the effect of diapause on development. In many cases, these changes are induced by the same hormonal pathways that regulate the diapause decision. In other words, these pathways coordinate the decision to enter diapause with the developmental consequences of diapause. These changes are outlined in this subsection and summarized in Figure 4.
Figure 4. Hormonal pathways regulate development during diapause in C. elegans. Graphical summary of the hormonal pathways that interface with developmental pathways to accommodate changes that occur during the diapause developmental trajectory. A diagram of a worm is shown, with the cell types discussed in the text featured. Arrows pointing at cells indicate processes that promote developmental progression, whereas blunt arrows pointing at cells indicate processes that inhibit developmental progression. See text for details.
As described in section “Diapause in Nematodes: Caenorhabditis elegans,” C. elegans development consists of embryogenesis followed by four larval stages (L1-L4). Dauer diapause occurs immediately after the second larval stage (Cassada and Russell, 1975). Adverse environmental conditions sensed during the first larval stage will induce entry into the alternative, pre-dauer stage called L2d. During L2d, larvae prepare for dauer by increasing lipid stores as they continue to sense their environment. After L2d, larvae can either continue development as L3 stage larvae or molt into dauer diapause, depending on environmental conditions. The L2d stage lasts at least 50% longer than the non-dauer L2 stage (Golden and Riddle, 1984b). There are several developmental events that occur during the second larval stage, and therefore these must occur at a slower pace in L2d larvae, as described below. During dauer, development is paused at the equivalent of an early L3-staged larva (Cassada and Russell, 1975). Progenitor cells that have not yet completed development must retain the capacity to take on normal cell fate in the event of recovery. As described below, this seems to be an active process. Finally, if favorable conditions are found, C. elegans dauer larvae undergo a process of recovery and resume development. Post-dauer larvae must complete the developmental events that normally occur in the L3 stage before molting into an L4-staged larva (Cassada and Russell, 1975).
As in insects and annual fishes, chromatin modification and gene expression changes have been identified when comparing pre-dauer, dauer, or post-dauer animals to their developmental equivalents. Differentially expressed genes include protein-coding genes and small RNAs, and in at least some cases effects on developmental pathways have been observed (Liu et al., 2004; Harvey et al., 2009; Hall et al., 2010, 2013; Karp et al., 2011). However, the remainder of this subsection will focus on three developmental contexts and how hormone pathways affect their development in the diapause life history.
When L1 larvae hatch from embryos, their germline consists of two cells. In response to Notch signaling, these cells proliferate and expand the germline stem cell population over the first several larval stages. Meiosis does not begin until after dauer would have occurred, during the mid-L3 stage (Hubbard and Schedl, 2019).
During L2d, germ cell proliferation slows as larvae prepare to enter dauer, and proliferation ceases during dauer diapause (Narbonne and Roy, 2006). Reducing the rate of germ cell proliferation in L2d requires several genes within the AMPK and IIS pathways, including genes encoding both subunits of the energy-sensing AMP-activated Protein Kinase (AMPK), called aak-1 and aak-2 in worms, the gene encoding the AMPK-activating kinase PAR-4/LKB1, and the gene encoding the PTEN phosphatase DAF-18 (Figure 4). Although these genes all affect related pathways, they are each required independently. Furthermore, these pathways function downstream of or in parallel to the Notch pathway that promotes germ cell division in favorable environments (Narbonne and Roy, 2006, 2009; Tenen and Greenwald, 2019).
In addition to its importance in slowing germ cell proliferation in L2d larvae, AMPK is required during and after dauer to regulate small RNA pathways that in turn maintain proper chromatin marks and therefore affect gene expression. Dauer and post-dauer larvae that lack aak-1 display aberrant gene expression and post-dauer adults are sterile. Remarkably, AMPK activity is required in somatic cells for these functions in germ cells (Kadekar and Roy, 2019).
daf-18/PTEN is unique among the genes listed above in that it is required in the somatic gonad for quiescence in both the germ line and the somatic gonad (Tenen and Greenwald, 2019). Furthermore, daf-18 is required to maintain developmental arrest during dauer in the somatic gonad progenitor cells, because cell fate markers that indicate developmental decisions that normally occur in the L3 or L4 stage are observed in dauer larvae that lack daf-18 (Tenen and Greenwald, 2019).
In parallel to AMPK and IIS signaling, the DAF-12 NHR and its co-repressor DIN-1S also inhibit proliferation of germline and somatic gonad cells in the L2d stage (Figure 4). In contrast to aak-1 and daf-18 that function non-cell-autonomously in somatic cells, din-1s is required autonomously in germ cells to prevent germ cell proliferation and in somatic gonad cells to prevent their proliferation (Colella et al., 2016).
Developmental pathways appear to be not only inactivated but actively re-set during dauer diapause. Some of the best evidence for active re-setting of developmental pathways comes from work examining the vulval precursor cells (VPCs). VPCs are born during the L1 stage and remain multipotent and unspecified until the L3 stage. During the L3 stage these cells respond to a combination of EGFR/Ras and Notch signaling pathways to specify VPCs to one of two vulval cell fates (1° and 2°). The absence of signaling leads to a default non-vulval cell fate (3°) (Sternberg, 2005). In wild-type larvae, 1° cell fate markers are expressed in the presumptive 1° VPC during the molt into dauer diapause, but this specification is erased during dauer. After recovery from dauer, larvae enter the post-dauer L3 (PDL3) stage that is the developmental equivalent of the L3 stage that occurs during the non-diapause developmental trajectory. VPC specification is again initiated in PDL3 (Karp and Greenwald, 2013).
The re-setting of VPC specification during dauer is more apparent in mutants that cause VPC specification prior to dauer. There are two categories of these mutants. First, loss of the heterochronic gene lin-28 causes precocious VPC specification during the L2 stage (Ambros and Horvitz, 1984). Larvae lacking lin-28 that develop through the dauer trajectory show VPC specification during the pre-dauer L2d stage. This specification is erased during dauer, and then re-initiates in VPCs or their descendants in post-dauer larvae (Euling and Ambros, 1996; Karp and Greenwald, 2013). Second, mutants in which EGFR/Ras or LIN-12/Notch signaling have been activated also show VPC specification during the pre-dauer L2d stage. Again, this specification is lost during dauer (Karp and Greenwald, 2013). Therefore, there is an active mechanism that erases any VPC specification that has occurred prior to dauer formation and re-establishes multipotent VPC fate.
Both DAF-12/NHR signaling and DAF-16/FOXO have been implicated in VPC development during dauer (Figure 4). Mutations in daf-12/NHR, din-1s, which encodes a corepressor that binds unliganded DAF-12, or daf-9, which encodes an enzyme required for synthesis of the DAF-12 ligand, all result in VPC division and vulval development during dauer (Karp and Greenwald, 2013; Colella et al., 2016). However, this phenotype is suppressed when daf-12 or daf-9 mutants are exposed to dauer-inducing conditions (dauer pheromone), suggesting that these genes are not the most proximal regulators of VPC quiescence. By contrast, loss of daf-16 results in VPC division as well as adoption of 1° and 2° fate markers, even in the presence of dauer pheromone (Karp and Greenwald, 2013). Therefore, during dauer, daf-16/FOXO blocks EGFR/Ras and LIN-12/Notch signaling to prevent precocious specification.
One of the developmental pathways most profoundly affected by diapause is the “heterochronic” pathway that controls stage-specific cell fate, particularly in the stem-cell-like seam cells within the lateral hypodermis. Seam cells divide in a particular pattern and sequence at each larval stage before terminally differentiating at adulthood. The gene network that controls this pattern of stage-specific cell divisions and differentiation is termed the “heterochronic” pathway (Ambros and Horvitz, 1984). These heterochronic genes function as a molecular timer whereby transcription factors and RNA-binding proteins that specify early cell fate are expressed at each larval stage. These early-promoting factors are then downregulated by microRNAs in order to allow progression to the next cell fate (Rougvie and Moss, 2013). A molecular timer would seem to be less useful in an interrupted developmental trajectory, and perhaps for that reason the heterochronic pathway is altered in larvae whose development is interrupted by dauer diapause (Liu and Ambros, 1991). For example, microRNA activity is modulated in L2d and dauer larvae, so that a different set of microRNAs becomes more important in the dauer diapause context (Karp and Ambros, 2012; Ilbay and Ambros, 2019).
DAF-12/NHR plays an important role in coordinating the decision to enter dauer with seam cell fate (Figure 4). In diapause-inducing conditions, there is little of the DAF-12 ligand produced and DAF-12 binds to its co-repressor, DIN-1S to promote dauer formation. DAF-12 also directly regulates the transcription of heterochronic microRNAs within the let-7 family (Bethke et al., 2009). These microRNAs are upregulated in order to promote L3 cell fate. In the lengthened L2d stage, the repressor form of DAF-12 keeps levels of these microRNAs low and L3 fate is not adopted prematurely (Bethke et al., 2009; Hammell et al., 2009). The let-7 family microRNAs also directly target daf-12, forming a feedback loop that helps to coordinate the dauer formation decision with the regulation of seam cell development (Hammell et al., 2009).
As described in section “Effect of Diapause on Vulval Precursor Development in C. elegans,” VPC fate appears to be re-set during dauer. A similar type of re-setting appears to occur in lateral hypodermal cells, including the seam cells. This type of re-setting was initially inferred based on the observation that the seam cell lineage defects that occur in certain heterochronic mutants were corrected in larvae that developed through the diapause trajectory (Liu and Ambros, 1991). More recently, a role for daf-16/FOXO was discovered in these cells. daf-16/FOXO is required in dauer larvae to prevent the precocious expression of collagens that are normally enriched in adults. daf-16/FOXO appears to act partially via known heterochronic genes and partially via a novel mechanism. Specifically, daf-16/FOXO opposes expression of the lin-41/TRIM71 RNA-binding protein during dauer to block adult cell fate. However, lin-41/TRIM71 does not act via its canonical target, the LIN-29 transcription factor, indicating a novel mechanism at play during dauer (Wirick et al., 2021). Thus, daf-16/FOXO coordinates the diapause developmental trajectory with multiple developmental events (Figure 4).
Diapause is a commonly used mechanism to help animals in the wild increase their chances of survival and reproduction in changing environmental conditions. Since diapause occurs at the level of the organism, individual cells and tissues must respond to diapause-inducing cues in a consistent manner. Hormonal pathways function to relay these cues across the organism, coordinating the developmental trajectory according to current or predicted environmental conditions. Many of the pathways that regulate diapause also regulate growth and development in non-diapause contexts. One possibility is that negative regulation of these growth-promoting pathways is a common molecular mechanism that enabled the evolution of diapause in different species.
The IIS and NHR hormonal pathways are involved in the diapause decision across animal phyla. IIS appears to function universally as an anti-diapause pathway. The IIS pathway has two evolutionarily conserved features that would seem to make this pathway particularly well-suited to this role. First, IIS positively regulates growth and metabolism in a variety of contexts. Second, production of insulin and related ligands is regulated by environmental cues, including nutritional status (Oldham and Hafen, 2003; Tothova and Gilliland, 2007; Murphy and Hu, 2013).
Nuclear hormone receptor signaling also commonly opposes diapause, but this direction is not universal among insect species. Class II NHRs are also well-positioned to serve as environmentally responsive regulators of diapause, given their function as molecular switches between transcriptional repressors and activators (Mangelsdorf et al., 1995). This switch function allows them to promote growth when ligand is present and inhibit growth when ligand is absent. In insects EcR signaling is required for each molt, making negative regulation of EcR signaling a straightforward way to pause developmental progression. It is interesting that among the large and diverse family of NHRs, the NHRs involved in regulating diapause across phyla are closely related, such that addition of C. elegans ligands or inhibitors can control diapause in the annual fish A. limnaeus (Romney et al., 2018). These observations indicate a high degree of conservation at the molecular level between these two species.
In addition to IIS and NHR pathways, other hormonal and non-hormonal pathways that regulate growth and development are also involved in the diapause decision in each species. Some examples of these pathways are juvenile hormone in insects and growth-factor-mediated signaling in C. elegans and insects (Fielenbach and Antebi, 2008; Denlinger et al., 2012; Schiesari and O’Connor, 2013). It makes sense that the use of juvenile hormone to regulate diapause occurs only in insects, since juvenile hormone does not regulate growth and development in nematodes or fishes. However, in other cases it is unclear why some pathways regulate diapause only in particular species. The differences may relate to the specific role of such pathways in growth and development in that species, or how readily those pathways could integrate with diapause-inducing machinery.
In C. elegans, the same hormonal pathways that regulate the decision to enter diapause are also critical regulators of the alterations to developmental programs that occur to accommodate the pre-diapause preparatory phase, the interruption to development that occurs during diapause, and the post-diapause phase where development is rejoined. In the future it will be interesting to discover the extent to which these pathways also regulate development in insects and annual fishes that undergo diapause.
XK wrote this manuscript in its entirety.
XK was supported by NSF CAREER award 1652283.
The author declares that the research was conducted in the absence of any commercial or financial relationships that could be construed as a potential conflict of interest.
All claims expressed in this article are solely those of the authors and do not necessarily represent those of their affiliated organizations, or those of the publisher, the editors and the reviewers. Any product that may be evaluated in this article, or claim that may be made by its manufacturer, is not guaranteed or endorsed by the publisher.
I would like to thank members of my lab for helpful discussions.
Ailion, M., and Thomas, J. H. (2003). Isolation and characterization of high-temperature-induced Dauer formation mutants in Caenorhabditis elegans. Genetics 165, 127–144.
Ambros, V., and Horvitz, H. R. (1984). Heterochronic mutants of the nematode Caenorhabditis elegans. Science 226, 409–416.
Anduaga, A. M., Nagy, D., Costa, R., and Kyriacou, C. P. (2018). Diapause in Drosophila melanogaster – Photoperiodicity, cold tolerance and metabolites. J. Insect. Physiol. 105, 46–53. doi: 10.1016/j.jinsphys.2018.01.003
Antebi, A. (2015). Nuclear receptor signal transduction in C. elegans. WormBook 1–49. doi: 10.1895/wormbook.1.64.2
Antebi, A., Culotti, J. G., and Hedgecock, E. M. (1998). daf-12 regulates developmental age and the dauer alternative in Caenorhabditis elegans. Development 125, 1191–1205.
Antebi, A., Yeh, W. H., Tait, D., Hedgecock, E. M., and Riddle, D. L. (2000). daf-12 encodes a nuclear receptor that regulates the Dauer diapause and developmental age in C. elegans. Genes Dev. 14, 1512–1527. doi: 10.1101/gad.14.12.1512
Arezo, M. J., Papa, N. G., Berois, N., Clivio, G., Montagne, J., and De la Piedra, S. (2017). Annual killifish adaptations to ephemeral environments: diapause I in two austrolebias species. Dev. Dyn. 246, 848–857. doi: 10.1002/dvdy.24580
Arunkumar, K. P., Metta, M., and Nagaraju, J. (2006). Molecular phylogeny of silkmoths reveals the origin of domesticated silkmoth, Bombyx mori from Chinese Bombyx mandarina and paternal inheritance of Antheraea proylei mitochondrial DNA. Mol. Phylogenet. Evol. 40, 419–427. doi: 10.1016/j.ympev.2006.02.023
Avery, L. (2014). A model of the effect of uncertainty on the C. elegans L2/L2d decision. PLoS One 9:e100580. doi: 10.1371/journal.pone.0100580
Barberà, M., Cañas-Cañas, R., and Martínez-Torres, D. (2019). Insulin-like peptides involved in photoperiodism in the aphid Acyrthosiphon pisum. Insect. Biochem. Mol. 112:103185. doi: 10.1016/j.ibmb.2019.103185
Baugh, L. R., and Hu, P. J. (2020). Starvation responses throughout the Caenorhabditis elegans life cycle. Genetics 216, 837–878. doi: 10.1534/genetics.120.303565
Bendena, W. G., Hui, J. H. L., Chin-Sang, I., and Tobe, S. S. (2020). Neuropeptide and microRNA regulators of juvenile hormone production. Gen. Comp. Endocr. 295:113507. doi: 10.1016/j.ygcen.2020.113507
Bethke, A., Fielenbach, N., Wang, Z., Mangelsdorf, D. J., and Antebi, A. (2009). Nuclear hormone receptor regulation of microRNAs controls developmental progression. Science 324, 95–98. doi: 10.1126/science.1164899
Brunet, A., Bonni, A., Zigmond, M. J., Lin, M. Z., Juo, P., Hu, L. S., et al. (1999). Akt promotes cell survival by phosphorylating and inhibiting a Forkhead transcription factor. Cell 96, 857–868. doi: 10.1016/s0092-8674(00)80595-4
Butcher, R. A., Fujita, M., Schroeder, F. C., and Clardy, J. (2007). Small-molecule pheromones that control dauer development in Caenorhabditis elegans. Nat. Chem. Biol. 3, 420–422. doi: 10.1038/nchembio.2007.3
Byerly, L., Cassada, R. C., and Russell, R. L. (1976). The life cycle of the nematode Caenorhabditis elegans. I. Wild-type growth and reproduction. Dev. Biol. 51, 23–33.
Cambron, L. D., Yocum, G. D., Yeater, K. M., and Greenlee, K. J. (2021). Overwintering conditions impact insulin pathway gene expression in diapausing Megachile rotundata. Comp. Biochem. Physiol. Mol. Integr. Physiol. 256:110937. doi: 10.1016/j.cbpa.2021.110937
Carlberg, C., Bendik, I., Wyss, A., Meier, E., Sturzenbecker, L. J., Grippo, J. F., et al. (1993). Two nuclear signalling pathways for vitamin D. Nature 361, 657–660. doi: 10.1038/361657a0
Carlberg, C., and Campbell, M. J. (2013). Vitamin D receptor signaling mechanisms: integrated actions of a well-defined transcription factor. Steroids 78, 127–136. doi: 10.1016/j.steroids.2012.10.019
Cassada, R. C., and Russell, R. L. (1975). The dauerlarva, a post-embryonic developmental variant of the nematode Caenorhabditis elegans. Dev. Biol. 46, 326–342.
Chen, H., Wu, G., Zhou, H., Dai, X., Steeghs, N. W. F., Dong, X., et al. (2021). Hormonal regulation of reproductive diapause that occurs in the year-round mass rearing of Bombus terrestris queens. J. Proteome Res. 20, 2240–2250. doi: 10.1021/acs.jproteome.0c00776
Chen, Y., Jiang, T., Zhu, J., Xie, Y., Tan, Z., Chen, Y., et al. (2017). Transcriptome sequencing reveals potential mechanisms of diapause preparation in bivoltine silkworm Bombyx mori (Lepidoptera: Bombycidae). Comp. Biochem. Physiol. D Genomics Proteomics 24, 68–78. doi: 10.1016/j.cbd.2017.07.003
Colella, E., Li, S., and Roy, R. (2016). Developmental and cell cycle quiescence is mediated by the nuclear hormone receptor coregulator DIN-1S in the Caenorhabditis elegans dauer larva. Genetics 203, 1763–1776. doi: 10.1534/genetics.116.191858
Cornils, A., Gloeck, M., Chen, Z., Zhang, Y., and Alcedo, J. (2011). Specific insulin-like peptides encode sensory information to regulate distinct developmental processes. Development 138, 1183–1193. doi: 10.1242/dev.060905
Crook, M. (2013). The dauer hypothesis and the evolution of parasitism: 20 years on and still going strong. Int. J. Parasitol. 44, 1–8. doi: 10.1016/j.ijpara.2013.08.004
Czech, M. P. (1989). Signal transmission by the insulin-like growth factors. Cell 59, 235–238. doi: 10.1016/0092-8674(89)90281-x
da Graca, L. S., Zimmerman, K. K., Mitchell, M. C., Kozhan-Gorodetska, M., Sekiewicz, K., Morales, Y., et al. (2004). DAF-5 is a Ski oncoprotein homolog that functions in a neuronal TGF beta pathway to regulate C. elegans dauer development. Development 131, 435–446. doi: 10.1242/dev.00922
David, J. R., and Capy, P. (1988). Genetic variation of Drosophila melanogaster natural populations. Trends Genet. 4, 106–111. doi: 10.1016/0168-9525(88)90098-4
Denlinger, D. L., and Armbruster, P. A. (2013). Mosquito diapause. Annu. Rev. Entomol. 59, 73–93. doi: 10.1146/annurev-ento-011613-162023
Denlinger, D. L., Yocum, G. D., and Rinehart, J. P. (2012). “Hormonal control of diapause,” in Insect Endocrinology, ed. L. I. Gilbert (Amsterdam: Elsevier), 430–463. doi: 10.1016/b978-0-12-384749-2.10010-x
Diniz, D. F. A., de Albuquerque, C. M. R., Oliva, L. O., Melo-Santos, M. A. V., and de, and Ayres, C. F. J. (2017). Diapause and quiescence: dormancy mechanisms that contribute to the geographical expansion of mosquitoes and their evolutionary success. Parasit. Vectors 10:310. doi: 10.1186/s13071-017-2235-0
Dolfi, L., Ripa, R., Antebi, A., Valenzano, D. R., and Cellerino, A. (2019). Cell cycle dynamics during diapause entry and exit in an annual killifish revealed by FUCCI technology. Evodevo 10:29. doi: 10.1186/s13227-019-0142-5
Eldridge, B. F. (1966). Environmental control of ovarian development in mosquitoes of the Culex pipiens complex. Science 151, 826–828. doi: 10.1126/science.151.3712.826
Ellis, R. E., and Lin, S.-Y. (2014). The evolutionary origins and consequences of self-fertility in nematodes. F1000Prime Rep. 6:62. doi: 10.12703/p6-62
Emerson, K. J., Uyemura, A. M., McDaniel, K. L., Schmidt, P. S., Bradshaw, W. E., and Holzapfel, C. M. (2009). Environmental control of ovarian dormancy in natural populations of Drosophila melanogaster. J. Comp. Physiol. 195, 825–829. doi: 10.1007/s00359-009-0460-5
Euling, S., and Ambros, V. (1996). Reversal of cell fate determination in Caenorhabditis elegans vulval development. Development 122, 2507–2515.
Félix, M.-A., and Duveau, F. (2012). Population dynamics and habitat sharing of natural populations of Caenorhabditis elegans and C. briggsae. BMC Biol. 10:59. doi: 10.1186/1741-7007-10-59
Fernandes-de-Abreu, D. A., Caballero, A., Fardel, P., Stroustrup, N., Chen, Z., Lee, K., et al. (2014). An insulin-to-insulin regulatory network orchestrates phenotypic specificity in development and physiology. PLoS Genet. 10:e1004225. doi: 10.1371/journal.pgen.1004225
Fielenbach, N., and Antebi, A. (2008). C. elegans dauer formation and the molecular basis of plasticity. Genes Dev. 22, 2149–2165. doi: 10.1101/gad.1701508
Flatt, T., Tu, M., and Tatar, M. (2005). Hormonal pleiotropy and the juvenile hormone regulation of Drosophila development and life history. Bioessays 27, 999–1010. doi: 10.1002/bies.20290
Frézal, L., and Félix, M.-A. (2015). C. elegans outside the Petri dish. eLife 4:e05849. doi: 10.7554/elife.05849
Fujiwara, Y., Shindome, C., Takeda, M., and Shiomi, K. (2006a). The roles of ERK and P38 MAPK signaling cascades on embryonic diapause initiation and termination of the silkworm, Bombyx mori. Insect Biochem. Mol. 36, 47–53. doi: 10.1016/j.ibmb.2005.10.005
Fujiwara, Y., Tanaka, Y., Iwata, K., Rubio, R. O., Yaginuma, T., Yamashita, O., et al. (2006b). ERK/MAPK regulates ecdysteroid and sorbitol metabolism for embryonic diapause termination in the silkworm. Bombyx mori. J. Insect. Physiol. 52, 569–575. doi: 10.1016/j.jinsphys.2006.02.004
Furdui, E. M., Mărghitaş, L. A., Dezmirean, D. S., Paşca, I., Pop, I. F., Erler, S., et al. (2014). Genetic characterization of Bombyx mori (Lepidoptera: Bombycidae) breeding and hybrid lines with different geographic origins. J. Insect. Sci. 14:211. doi: 10.1093/jisesa/ieu073
Furness, A. I., Reznick, D. N., Springer, M. S., and Meredith, R. W. (2015). Convergent evolution of alternative developmental trajectories associated with diapause in African and South American killifish. Proc. R. Soc. B Biol. Sci. 282:20142189. doi: 10.1098/rspb.2014.2189
Gao, L., Yuan, Z., Zhou, T., Yang, Y., Gao, D., Dunham, R., et al. (2019). FOXO genes in channel catfish and their response after bacterial infection. Dev. Comp. Immunol. 97, 38–44. doi: 10.1016/j.dci.2019.03.010
Gems, D., Sutton, A. J., Sundermeyer, M. L., Albert, P. S., King, K. V., Edgley, M. L., et al. (1998). Two pleiotropic classes of daf-2 mutation affect larval arrest, adult behavior, reproduction and longevity in Caenorhabditis elegans. Genetics 150, 129–155.
Gerisch, B., and Antebi, A. (2004). Hormonal signals produced by DAF-9/cytochrome P450 regulate C. elegans dauer diapause in response to environmental cues. Development 131, 1765–1776. doi: 10.1242/dev.01068
Gerisch, B., Weitzel, C., Kober-Eisermann, C., Rottiers, V., and Antebi, A. (2001). A hormonal signaling pathway influencing C. elegans metabolism, reproductive development, and life span. Dev. Cell 1, 841–851.
Golden, J. W., and Riddle, D. L. (1982). A pheromone influences larval development in the nematode Caenorhabditis elegans. Science 218, 578–580.
Golden, J. W., and Riddle, D. L. (1984a). A Caenorhabditis elegans dauer-inducing pheromone and an antagonistic component of the food supply. J. Chem. Ecol. 10, 1265–1280. doi: 10.1007/bf00988553
Golden, J. W., and Riddle, D. L. (1984b). The Caenorhabditis elegans dauer larva: developmental effects of pheromone, food, and temperature. Dev. Biol. 102, 368–378.
Gong, J., Zheng, X., Zhao, S., Yang, L., Xue, Z., Fan, Z., et al. (2020). Early molecular events during onset of diapause in silkworm eggs revealed by transcriptome analysis. Int. J. Mol. Sci. 21:6180. doi: 10.3390/ijms21176180
Gottlieb, S., and Ruvkun, G. (1994). daf-2, daf-16 and daf-23: genetically interacting genes controlling dauer formation in Caenorhabditis elegans. Genetics 137, 107–120.
Gu, S., Chen, C., and Lin, P. (2021). Changes in expressions of ecdysteroidogenic enzyme and ecdysteroid signaling genes in relation to Bombyx embryonic development. J. Exp Zool. Ecol. Integr. Physiol. 335, 477–488. doi: 10.1002/jez.2466
Gu, S.-H., Lin, P.-L., and Hsieh, H.-Y. (2019). Bombyxin/Akt signaling in relation to the embryonic diapause process of the silkworm, Bombyx mori. J. Insect Physiol. 116, 32–40. doi: 10.1016/j.jinsphys.2019.04.007
Hall, S. E., Beverly, M., Russ, C., Nusbaum, C., and Sengupta, P. (2010). A cellular memory of developmental history generates phenotypic diversity in C. elegans. Curr. Biol. CB 20, 149–155. doi: 10.1016/j.cub.2009.11.035
Hall, S. E., Chirn, G. W., Lau, N. C., and Sengupta, P. (2013). RNAi pathways contribute to developmental history-dependent phenotypic plasticity in C. elegans. RNA 19, 306–319. doi: 10.1261/rna.036418.112
Hammell, C. M., Karp, X., and Ambros, V. (2009). A feedback circuit involving let-7-family miRNAs and DAF-12 integrates environmental signals and developmental timing in Caenorhabditis elegans. Proc. Natl. Acad. Sci. U.S.A. 106, 18668–18673. doi: 10.1073/pnas.0908131106
Hand, S. C., Denlinger, D. L., Podrabsky, J. E., and Roy, R. (2016). Mechanisms of animal diapause: recent developments from nematodes, crustaceans, insects, and fish. Am. J. Physiol. Regul. Integr. Comp. Physiol. 310, R1193–R1211. doi: 10.1152/ajpregu.00250.2015
Hao, K., Jarwar, A. R., Ullah, H., Tu, X., Nong, X., and Zhang, Z. (2019). Transcriptome sequencing reveals potential mechanisms of the maternal effect on egg diapause induction of Locusta migratoria. Int. J. Mol. Sci. 20:1974. doi: 10.3390/ijms20081974
Harvey, S. C., Barker, G. L., Shorto, A., and Viney, M. E. (2009). Natural variation in gene expression in the early development of dauer larvae of Caenorhabditis elegans. BMC Genomics 10:325. doi: 10.1186/1471-2164-10-325
Hochbaum, D., Zhang, Y., Stuckenholz, C., Labhart, P., Alexiadis, V., Martin, R., et al. (2011). DAF-12 regulates a connected network of genes to ensure robust developmental decisions. PLoS Genet. 7:e1002179. doi: 10.1371/journal.pgen.1002179.g009
Homma, T., Watanabe, K., Tsurumaru, S., Kataoka, H., Imai, K., Kamba, M., et al. (2006). G protein-coupled receptor for diapause hormone, an inducer of Bombyx embryonic diapause. Biochem. Biophys. Res. Co 344, 386–393. doi: 10.1016/j.bbrc.2006.03.085
Hsu, S. Y., Nakabayashi, K., Nishi, S., Kumagai, J., Kudo, M., Sherwood, O. D., et al. (2002). Activation of orphan receptors by the hormone relaxin. Science 295, 671–674. doi: 10.1126/science.1065654
Hu, C.-K., Wang, W., Brind’Amour, J., Singh, P. P., Reeves, G. A., Lorincz, M. C., et al. (2020). Vertebrate diapause preserves organisms long term through Polycomb complex members. Science 367, 870–874. doi: 10.1126/science.aaw2601
Hubbard, E. J. A., and Schedl, T. (2019). Biology of the Caenorhabditis elegans germline stem cell system. Genetics 213, 1145–1188. doi: 10.1534/genetics.119.300238
Ilbay, O., and Ambros, V. (2019). Pheromones and nutritional signals regulate the developmental reliance on let-7 family microRNAs in C. elegans. Curr. Biol. 29, 1735.e–1745.e. doi: 10.1016/j.cub.2019.04.034
Jeong, P.-Y., Jung, M., Yim, Y.-H., Kim, H., Park, M., Hong, E., et al. (2005). Chemical structure and biological activity of the Caenorhabditis elegans dauer-inducing pheromone. Nature 433, 541–545. doi: 10.1038/nature03201
Jones, J. I., and Clemmons, D. R. (1995). Insulin-like growth factors and their binding proteins: biological actions. Endocr. Rev. 16, 3–34. doi: 10.1210/edrv-16-1-3
Kadekar, P., and Roy, R. (2019). AMPK regulates germline stem cell quiescence and integrity through an endogenous small RNA pathway. PLoS Biol. 17:e3000309. doi: 10.1371/journal.pbio.3000309
Kang, D. S., Cotten, M. A., Denlinger, D. L., and Sim, C. (2016). Comparative transcriptomics reveals key gene expression differences between diapausing and non-diapausing adults of Culex pipiens. PLoS One 11:e0154892. doi: 10.1371/journal.pone.0154892
Kaplan, F., Srinivasan, J., Mahanti, P., Ajredini, R., Durak, O., Nimalendran, R., et al. (2011). Ascaroside expression in Caenorhabditis elegans is strongly dependent on diet and developmental stage. PLoS One 6:e17804. doi: 10.1371/journal.pone.0017804
Karp, X., and Ambros, V. (2012). Dauer larva quiescence alters the circuitry of microRNA pathways regulating cell fate progression in C. elegans. Development 139, 2177–2186. doi: 10.1242/dev.075986
Karp, X., and Greenwald, I. (2013). Control of cell-fate plasticity and maintenance of multipotency by DAF-16/FoxO in quiescent Caenorhabditis elegans. Proc. Natl. Acad. Sci. U.S.A. 110, 2181–2186. doi: 10.1073/pnas.1222377110
Karp, X., Hammell, M., Ow, M. C., and Ambros, V. (2011). Effect of life history on microRNA expression during C. elegans development. RNA 17, 639–651. doi: 10.1261/rna.2310111
Kimble, J., and Hirsh, D. (1979). The postembryonic cell lineages of the hermaphrodite and male gonads in Caenorhabditis elegans. Dev. Biol. 70, 396–417.
Kimura, K. D., Riddle, D. L., and Ruvkun, G. (2011). The C. elegans DAF-2 insulin-like receptor is abundantly expressed in the nervous system and regulated by nutritional status. Cold Spring Harb. Symp. Quant. Biol. 76, 113–120. doi: 10.1101/sqb.2011.76.010660
Kimura, K. D., Tissenbaum, H. A., Liu, Y., and Ruvkun, G. (1997). daf-2, an insulin receptor-like gene that regulates longevity and diapause in Caenorhabditis elegans. Science 277, 942–946.
Klass, M., and Hirsh, D. (1976). Non-ageing developmental variant of Caenorhabditis elegans. Nature 260, 523–525.
Klass, M. R. (1983). A method for the isolation of longevity mutants in the nematode Caenorhabditis elegans and initial results. Mech. Ageing Dev. 22, 279–286.
Koelle, M. R., Talbot, W. S., Segraves, W. A., Bender, M. T., Cherbas, P., and Hogness, D. S. (1991). The Drosophila EcR gene encodes an ecdysone receptor, a new member of the steroid receptor superfamily. Cell 67, 59–77. doi: 10.1016/0092-8674(91)90572-g
Kops, G. J. P. L., de Ruiter, N. D., Vries-Smits, A. M. M. D., Powell, D. R., Bos, J. L., Burgering, B. M., et al. (1999). Direct control of the Forkhead transcription factor AFX by protein kinase B. Nature 398, 630–634. doi: 10.1038/19328
Kubrak, O. I., Kuèerová, L., Theopold, U., and Nässel, D. R. (2014). The sleeping beauty: how reproductive diapause affects hormone signaling, metabolism, immune response and somatic maintenance in Drosophila melanogaster. PloS One 9:e113051. doi: 10.1371/journal.pone.0113051
Kubrak, O. I., Kučerová, L., Theopold, U., Nylin, S., and Nässel, D. R. (2016). Characterization of reproductive dormancy in male Drosophila melanogaster. Front. Physiol. 7:572. doi: 10.3389/fphys.2016.00572
Larsen, P. L., Albert, P. S., and Riddle, D. L. (1995). Genes that regulate both development and longevity in Caenorhabditis elegans. Genetics 139, 1567–1583. doi: 10.1093/genetics/139.4.1567
Lau, M. M., Stewart, C. E., Liu, Z., Bhatt, H., Rotwein, P., and Stewart, C. L. (1994). Loss of the imprinted IGF2/cation-independent mannose 6-phosphate receptor results in fetal overgrowth and perinatal lethality. Gene Dev. 8, 2953–2963. doi: 10.1101/gad.8.24.2953
Lee, H., Choi, M., Lee, D., Kim, H., Hwang, H., Kim, H., et al. (2012). Nictation, a dispersal behavior of the nematode Caenorhabditis elegans, is regulated by IL2 neurons. Nat. Neurosci. 15, 107–112. doi: 10.1038/nn.2975
Levels, P. J., van Tits, L. J. H., and Denucé, J. M. (1986). The effect of the presence of adult fishes, gonad homogenates, and embryo homogenates on the dispersion-reaggregation phase during early embryonic development of the annual fish Nothobranchius korthausae. J. Exp. Zool. 240, 259–264. doi: 10.1002/jez.1402400212
Ley, P. D. (2006). A quick tour of nematode diversity and the backbone of nematode phylogeny. Wormbook 1–8. doi: 10.1895/wormbook.1.41.1
Li, W., Kennedy, S. G., and Ruvkun, G. (2003). daf-28 encodes a C. elegans insulin superfamily member that is regulated by environmental cues and acts in the DAF-2 signaling pathway. Genes Dev. 17, 844–858. doi: 10.1101/gad.1066503
Lin, K., Dorman, J. B., Rodan, A., and Kenyon, C. (1997). daf-16: an HNF-3/forkhead family member that can function to double the life-span of Caenorhabditis elegans. Science 278, 1319–1322. doi: 10.1126/science.278.5341.1319
Lin, K., Hsin, H., Libina, N., and Kenyon, C. (2001). Regulation of the Caenorhabditis elegans longevity protein DAF-16 by insulin/IGF-1 and germline signaling. Nat. Genet. 28, 139–145. doi: 10.1038/88850
Liu, T., Zimmerman, K. K., and Patterson, G. I. (2004). Regulation of signaling genes by TGFbeta during entry into dauer diapause in C. elegans. BMC Dev. Biol. 4:11. doi: 10.1186/1471-213x-4-11
Liu, Y., Liao, S., Veenstra, J. A., and Nässel, D. R. (2016). Drosophila insulin-like peptide 1 (DILP1) is transiently expressed during non-feeding stages and reproductive dormancy. Sci. Rep. UK 6:26620. doi: 10.1038/srep26620
Liu, Z., and Ambros, V. (1991). Alternative temporal control systems for hypodermal cell differentiation in Caenorhabditis elegans. Nature 350, 162–165. doi: 10.1038/350162a0
Luciani, G. M., Magomedova, L., Puckrin, R., Urbanus, M. L., Wallace, I. M., Giaever, G., et al. (2011). Dafadine inhibits DAF-9 to promote dauer formation and longevity of Caenorhabditis elegans. Nat. Chem. Biol. 7, 891–893. doi: 10.1038/nchembio.698
Ludewig, A. H., Kober-Eisermann, C., Weitzel, C., Bethke, A., Neubert, K., Gerisch, B., et al. (2004). A novel nuclear receptor/coregulator complex controls C. elegans lipid metabolism, larval development, and aging. Genes Dev. 18, 2120–2133. doi: 10.1101/gad.312604
Ludewig, A. H., and Schroeder, F. C. (2013). Ascaroside signaling in C. elegans. WormBook 1–22. doi: 10.1895/wormbook.1.155.1
Maeda, S., Nakashima, A., Yamada, R., Hara, N., Fujimoto, Y., Ito, Y., et al. (2008). Molecular cloning of ecdysone 20-hydroxylase and expression pattern of the enzyme during embryonic development of silkworm Bombyx mori. Comp. Biochem. Physiol. B Biochem. Mol. Biol. 149, 507–516. doi: 10.1016/j.cbpb.2007.11.015
Mahanti, P., Bose, N., Bethke, A., Judkins, J. C., Wollam, J., Dumas, K. J., et al. (2014). Comparative metabolomics reveals endogenous ligands of DAF-12, a nuclear hormone receptor, regulating C. elegans development and lifespan. Cell Metab. 19, 73–83. doi: 10.1016/j.cmet.2013.11.024
Mak, H. Y., and Ruvkun, G. (2004). Intercellular signaling of reproductive development by the C. elegans DAF-9 cytochrome P450. Development 131, 1777–1786. doi: 10.1242/dev.01069
Makka, T., Seino, A., Tomita, S., Fujiwara, H., and Sonobe, H. (2002). A possible role of 20-hydroxyecdysone in embryonic development of the silkworm Bombyx mori. Arch. Insect Biochem. 51, 111–120. doi: 10.1002/arch.10055
Malone, E. A., Inoue, T., and Thomas, J. H. (1996). Genetic analysis of the roles of daf-28 and age-1 in regulating Caenorhabditis elegans dauer formation. Genetics 143, 1193–1205.
Mangelsdorf, D. J., and Evans, R. M. (1995). The RXR heterodimers and orphan receptors. Cell 83, 841–850. doi: 10.1016/0092-8674(95)90200-7
Mangelsdorf, D. J., Thummel, C., Beato, M., Herrlich, P., Schütz, G., Umesono, K., et al. (1995). The nuclear receptor superfamily: the second decade. Cell 83, 835–839. doi: 10.1016/0092-8674(95)90199-x
Manning, B. D., and Cantley, L. C. (2007). AKT/PKB signaling: navigating downstream. Cell 129, 1261–1274. doi: 10.1016/j.cell.2007.06.009
Mansilla, A., Martín, F. A., Martín, D., and Ferrús, A. (2016). Ligand-independent requirements of steroid receptors EcR and USP for cell survival. Cell Death Differ. 23, 405–416. doi: 10.1038/cdd.2015.108
Martin, K., and Podrabsky, J. E. (2017). Hit pause: developmental arrest in annual killifishes and their close relatives. Dev. Dyn. 246, 858–866. doi: 10.1002/dvdy.24507
Meuti, M. E., Bautista-Jimenez, R., and Reynolds, J. A. (2018). Evidence that microRNAs are part of the molecular toolkit regulating adult reproductive diapause in the mosquito, Culex pipiens. PLoS One 13:e0203015. doi: 10.1371/journal.pone.0203015
Morris, J. Z., Tissenbaum, H. A., and Ruvkun, G. (1996). A phosphatidylinositol-3-OH kinase family member regulating longevity and diapause in Caenorhabditis elegans. Nature 382, 536–539. doi: 10.1038/382536a0
Motola, D. L., Cummins, C. L., Rottiers, V., Sharma, K. K., Li, T., Li, Y., et al. (2006). Identification of ligands for DAF-12 that govern dauer formation and reproduction in C. elegans. Cell 124, 1209–1223. doi: 10.1016/j.cell.2006.01.037
Murphy, C. T., and Hu, P. J. (2013). Insulin/insulin-like growth factor signaling in C. elegans. WormBook 1–43.
Nagy, D., Andreatta, G., Bastianello, S., Anduaga, A. M., Mazzotta, G., Kyriacou, C. P., et al. (2018). A semi-natural approach for studying seasonal diapause in Drosophila melanogaster reveals robust photoperiodicity. J. Biol. Rhythm 33, 117–125. doi: 10.1177/0748730417754116
Narbonne, P., and Roy, R. (2006). Inhibition of germline proliferation during C. elegans dauer development requires PTEN. LKB1 and AMPK signalling. Development 133, 611–619. doi: 10.1242/dev.02232
Narbonne, P., and Roy, R. (2009). Caenorhabditis elegans dauers need LKB1/AMPK to ration lipid reserves and ensure long-term survival. Nature 457, 210–214. doi: 10.1038/nature07536
Nika, L., Gibson, T., Konkus, R., and Karp, X. (2016). Fluorescent beads are a versatile tool for staging Caenorhabditis elegans in different life histories. G3 6, 1923–1933. doi: 10.1534/g3.116.030163
Niwa, R., and Niwa, Y. S. (2014). Enzymes for ecdysteroid biosynthesis: their biological functions in insects and beyond. Biosci. Biotechnol. Biochem. 78, 1283–1292. doi: 10.1080/09168451.2014.942250
Ogg, S., Paradis, S., Gottlieb, S., Patterson, G. I., Lee, L., Tissenbaum, H. A., et al. (1997). The Fork head transcription factor DAF-16 transduces insulin-like metabolic and longevity signals in C. elegans. Nature 389, 994–999. doi: 10.1038/40194
Oh, S. W., Mukhopadhyay, A., Svrzikapa, N., Jiang, F., Davis, R. J., and Tissenbaum, H. A. (2005). JNK regulates lifespan in Caenorhabditis elegans by modulating nuclear translocation of forkhead transcription factor/DAF-16. Proc. Natl. Acad. Sci. U.S.A. 102, 4494–4499. doi: 10.1073/pnas.0500749102
Olademehin, O. P., Liu, C., Rimal, B., Adegboyega, N. F., Chen, F., Sim, C., et al. (2020). Dsi-RNA knockdown of genes regulated by Foxo reduces glycogen and lipid accumulations in diapausing Culex pipiens. Sci. Rep. UK 10:17201. doi: 10.1038/s41598-020-74292-6
Oldham, S., and Hafen, E. (2003). Insulin/IGF and target of rapamycin signaling: a TOR de force in growth control. Trends Cell Biol. 13, 79–85. doi: 10.1016/s0962-8924(02)00042-9
Oro, A. E., McKeown, M., and Evans, R. M. (1990). Relationship between the product of the Drosophila ultraspiracle locus and the vertebrate retinoid X receptor. Nature 347, 298–301. doi: 10.1038/347298a0
Patterson, G. I., Koweek, A., Wong, A., Liu, Y., and Ruvkun, G. (1997). The DAF-3 Smad protein antagonizes TGF-beta -related receptor signaling in the Caenorhabditis elegans dauer pathway. Genes Dev. 11, 2679–2690. doi: 10.1101/gad.11.20.2679
Petryk, A., Warren, J. T., Marqués, G., Jarcho, M. P., Gilbert, L. I., Kahler, J., et al. (2003). Shade is the Drosophila P450 enzyme that mediates the hydroxylation of ecdysone to the steroid insect molting hormone 20-hydroxyecdysone. Proc. Natl. Acad. Sci. U.S.A. 100, 13773–13778. doi: 10.1073/pnas.2336088100
Pierce, S. B., Costa, M., Wisotzkey, R., Devadhar, S., Homburger, S. A., Buchman, A. R., et al. (2001). Regulation of DAF-2 receptor signaling by human insulin and ins-1, a member of the unusually large and diverse C. elegans insulin gene family. Genes Dev. 15, 672–686. doi: 10.1101/gad.867301
Podrabsky, J., Riggs, C., and Wagner, J. (2016a). “Tolerance of environmental stress,” in Annual Fishes Life History Strategy, Diversity, and Evolution, eds N. Berois, G. Garcia, and R. D. Sá (Boca Raton, FL: CRC Press, Taylor & Francis), 159–184. doi: 10.1201/b19016-13
Podrabsky, J., Romney, A., and Culpepper, K. (2016b). “Alternative developmental pathways,” in Annual Fishes Life History Strategy, Diversity, and Evolution, eds N. Berois, G. Garcia, and R. D. Sá (Boca Raton, FL: CRC Press, Taylor and Francis), 63–74. doi: 10.1201/b19016-6
Podrabsky, J. E., Garrett, I. D. F., and Kohl, Z. F. (2010). Alternative developmental pathways associated with diapause regulated by temperature and maternal influences in embryos of the annual killifish Austrofundulus limnaeus. J. Exp. Biol. 213, 3280–3288. doi: 10.1242/jeb.045906
Podrabsky, J. E., Riggs, C. L., Romney, A. L., Woll, S. C., Wagner, J. T., Culpepper, K. M., et al. (2017). Embryonic development of the annual killifish Austrofundulus limnaeus: an emerging model for ecological and evolutionary developmental biology research and instruction. Dev. Dyn. 246, 779–801. doi: 10.1002/dvdy.24513
Polačik, M., Vrtílek, M., Reichard, M., Žák, J., Blažek, R., and Podrabsky, J. (2021). Embryo ecology: developmental synchrony and asynchrony in the embryonic development of wild annual fish populations. Ecol. Evol. 11, 4945–4956. doi: 10.1002/ece3.7402
Reed, E., and Wallace, H. (1965). Leaping locomotion by an insect-parasitic nematode. Nature 206, 210–211. doi: 10.1038/206210a0
Reichard, M., and Polačik, M. (2019). Nothobranchius furzeri, an “instant” fish from an ephemeral habitat. Elife 8:e41548. doi: 10.7554/elife.41548
Ren, P., Lim, C.-S., Johnsen, R., Albert, P. S., Pilgrim, D., and Riddle, D. L. (1996). Control of C. elegans larval development by neuronal expression of a TGF-β homolog. Science 274, 1389–1391. doi: 10.1126/science.274.5291.1389
Richard, D. S., Gilbert, M., Crum, B., Hollinshead, D. M., Schelble, S., and Scheswohl, D. (2001a). Yolk protein endocytosis by oocytes in Drosophila melanogaster: immunofluorescent localization of clathrin, adaptin and the yolk protein receptor. J. Insect Physiol. 47, 715–723. doi: 10.1016/s0022-1910(00)00165-7
Richard, D. S., Jones, J. M., Barbarito, M. R., Cerula, S., Detweiler, J. P., Fisher, S. J., et al. (2001b). Vitellogenesis in diapausing and mutant Drosophila melanogaster: further evidence for the relative roles of ecdysteroids and juvenile hormones. J. Insect. Physiol. 47, 905–913. doi: 10.1016/s0022-1910(01)00063-4
Richard, D. S., Rybczynski, R., Wilson, T. G., Wang, Y., Wayne, M. L., Zhou, Y., et al. (2005). Insulin signaling is necessary for vitellogenesis in Drosophila melanogaster independent of the roles of juvenile hormone and ecdysteroids: female sterility of the chico1 insulin signaling mutation is autonomous to the ovary. J. Insect Physiol. 51, 455–464. doi: 10.1016/j.jinsphys.2004.12.013
Richard, D. S., Watkins, N. L., Serafin, R. B., and Gilbert, L. I. (1998). Ecdysteroids regulate yolk protein uptake by Drosophila melanogaster oocytes. J. Insect Physiol. 44, 637–644. doi: 10.1016/s0022-1910(98)00020-1
Riddle, D. L. (1977). A genetic pathway for dauer larva formation in Caenorhabditis elegans. Stad. Genet. Symp. 9, 101–120.
Riddle, D. L., Swanson, M. M., and Albert, P. S. (1981). Interacting genes in nematode dauer larva formation. Nature 290, 668–671.
Ritter, A. D., Shen, Y., Bass, J. F., Jeyaraj, S., Deplancke, B., Mukhopadhyay, A., et al. (2013). Complex expression dynamics and robustness in C. elegans insulin networks. Genome Res. 23, 954–965. doi: 10.1101/gr.150466.112
Robich, R. M., and Denlinger, D. L. (2005). Diapause in the mosquito Culex pipiens evokes a metabolic switch from blood feeding to sugar gluttony. Proc. Natl. Acad. Sci. U.S.A. 102, 15912–15917. doi: 10.1073/pnas.0507958102
Romney, A. L. T., Davis, E. M., Corona, M. M., Wagner, J. T., and Podrabsky, J. E. (2018). Temperature-dependent vitamin D signaling regulates developmental trajectory associated with diapause in an annual killifish. Proc. Natl. Acad. Sci. U.S.A. 115, 12763–12768. doi: 10.1073/pnas.1804590115
Romney, A. L. T., and Podrabsky, J. E. (2018). Small noncoding RNA profiles along alternative developmental trajectories in an annual killifish. Sci. Rep. 8:13364. doi: 10.1038/s41598-018-31466-7
Rottiers, V., Motola, D. L., Gerisch, B., Cummins, C. L., Nishiwaki, K., Mangelsdorf, D. J., et al. (2006). Hormonal control of C. elegans dauer formation and life Span by a Rieske-like oxygenase. Dev. Cell 10, 473–482. doi: 10.1016/j.devcel.2006.02.008
Rougvie, A. E., and Moss, E. G. (2013). Developmental transitions in C. elegans larval stages. Curr. Top. Dev. Biol. 105, 153–180. doi: 10.1016/b978-0-12-396968-2.00006-3
Rowiński, P. K., Sowersby, W., Näslund, J., Eckerström-Liedholm, S., Gotthard, K., and Rogell, B. (2021). Variation in developmental rates is not linked to environmental unpredictability in annual killifishes. Ecol. Evol. 11, 8027–8037. doi: 10.1002/ece3.7632
Sanburg, L. L., and Larsen, J. R. (1973). Effect of photoperiod and temperature on ovarian development in Culex pipiens pipiens. J. Insect Physiol. 19, 1173–1190. doi: 10.1016/0022-1910(73)90202-3
Saponaro, F., Saba, A., and Zucchi, R. (2020). An update on vitamin D metabolism. Int. J. Mol. Sci. 21:6573. doi: 10.3390/ijms21186573
Sato, A., Sokabe, T., Kashio, M., Yasukochi, Y., Tominaga, M., and Shiomi, K. (2014). Embryonic thermosensitive TRPA1 determines transgenerational diapause phenotype of the silkworm, Bombyx mori. Proc. Natl. Acad. Sci. U.S.A. 111, E1249–E1255. doi: 10.1073/pnas.1322134111
Saunders, D. S., and Gilbert, L. I. (1990). Regulation of ovarian diapause in Drosophila melanogaster by photoperiod and moderately low temperature. J. Insect Physiol. 36, 195–200. doi: 10.1016/0022-1910(90)90122-v
Saunders, D. S., Henrich, V. C., and Gilbert, L. I. (1989). Induction of diapause in Drosophila melanogaster: photoperiodic regulation and the impact of arrhythmic clock mutations on time measurement. Proc. Natl. Acad. Sci. U.S.A. 86, 3748–3752. doi: 10.1073/pnas.86.10.3748
Saunders, D. S., Richard, D. S., Applebaum, S. W., Ma, M., and Gilbert, L. I. (1990). Photoperiodic diapause in Drosophila melanogaster involves a block to the juvenile hormone regulation of ovarian maturation. Gen. Comp. Endocr. 79, 174–184. doi: 10.1016/0016-6480(90)90102-r
Schackwitz, W. S., Inoue, T., and Thomas, J. H. (1996). Chemosensory neurons function in parallel to mediate a pheromone response in C. elegans. Neuron 17, 719–728. doi: 10.1016/s0896-6273(00)80203-2
Schaedel, O. N., Gerisch, B., Antebi, A., and Sternberg, P. W. (2012). Hormonal signal amplification mediates environmental conditions during development and controls an irreversible commitment to adulthood. PLoS Biol. 10:e1001306. doi: 10.1371/journal.pbio.1001306
Schiesari, L., Andreatta, G., Kyriacou, C. P., O’Connor, M. B., and Costa, R. (2016). The insulin-like proteins dILPs-2/5 determine diapause inducibility in Drosophila. PLoS One 11:e0163680. doi: 10.1371/journal.pone.0163680
Schiesari, L., and O’Connor, M. B. (2013). Diapause: delaying the developmental clock in response to a changing environment. Curr. Top. Dev. Biol. 105, 213–246. doi: 10.1016/b978-0-12-396968-2.00008-7
Schmidt, P. S., Matzkin, L., Ippolito, M., and Eanes, W. F. (2005a). Geographic variation in diapause incidence, life-history traits, and climactic adaptation in Drosophila melanogaster. Evolution 59, 1721–1732. doi: 10.1111/j.0014-3820.2005.tb01821.x
Schmidt, P. S., Paaby, A. B., and Heschel, M. S. (2005b). Genetic variance for diapause expression and associated life histories in Drosophila melanogaster. Evolution 59, 2616–2625. doi: 10.1111/j.0014-3820.2005.tb00974.x
Schmidt, P. S., and Paaby, A. B. (2008). Reproductive diapause and life-history clines in North American populations of Drosophila melanogaster. Evolution 62, 1204–1215. doi: 10.1111/j.1558-5646.2008.00351.x
Shiomi, K., Takasu, Y., Kunii, M., Tsuchiya, R., Mukaida, M., Kobayashi, M., et al. (2015). Disruption of diapause induction by TALEN-based gene mutagenesis in relation to a unique neuropeptide signaling pathway in Bombyx. Sci. Rep. 5:15566. doi: 10.1038/srep15566
Shostak, Y., Gilst, M. R. V., Antebi, A., and Yamamoto, K. R. (2004). Identification of C. elegans DAF-12-binding sites, response elements, and target genes. Genes Dev. 18, 2529–2544. doi: 10.1101/gad.1218504
Sim, C., and Denlinger, D. L. (2008). Insulin signaling and FOXO regulate the overwintering diapause of the mosquito Culex pipiens. Proc. Natl. Acad. Sci. U.S.A. 105, 6777–6781. doi: 10.1073/pnas.0802067105
Sim, C., and Denlinger, D. L. (2009). A shut-down in expression of an insulin-like peptide, ILP-1, halts ovarian maturation during the overwintering diapause of the mosquito Culex pipiens. Insect Mol. Biol. 18, 325–332. doi: 10.1111/j.1365-2583.2009.00872.x
Sim, C., and Denlinger, D. L. (2013a). Insulin signaling and the regulation of insect diapause. Front. Physiol. 4:189. doi: 10.3389/fphys.2013.00189
Sim, C., and Denlinger, D. L. (2013b). Juvenile hormone III suppresses forkhead of transcription factor in the fat body and reduces fat accumulation in the diapausing mosquito, Culex pipiens. Insect Mol. Biol. 22, 1–11. doi: 10.1111/j.1365-2583.2012.01166.x
Sim, C., Kang, D. S., Kim, S., Bai, X., and Denlinger, D. L. (2015). Identification of FOXO targets that generate diverse features of the diapause phenotype in the mosquito Culex pipiens. Proc. Natl. Acad. Sci. U.S.A. 112, 3811–3816. doi: 10.1073/pnas.1502751112
Sonobe, H., and Yamada, R. (2004). Ecdysteroids during early embryonic development in silkworm Bombyx mori: metabolism and functions. Zool. Sci. 21, 503–516. doi: 10.2108/zsj.21.503
Spiebnan, A., and Wong, J. (1973). Studies on autogeny in natural populations of Culex pipiens. III. Midsummer preparation for hibernation in anautogenous populations. J. Med. Entomol. 10, 319–324. doi: 10.1093/jmedent/10.4.319
Spielman, A. (1974). Effect of synthetic juvenile hormone on ovarian diapause of Culex pipiens mosquitoes. J. Med. Entomol. 11, 223–225. doi: 10.1093/jmedent/11.2.223
Sulston, J. E., and Horvitz, H. R. (1977). Post-embryonic cell lineages of the nematode, Caenorhabditis elegans. Dev. Biol. 56, 110–156.
Sulston, J. E., Schierenberg, E., White, J. G., and Thomson, J. N. (1983). The embryonic cell lineage of the nematode Caenorhabditis elegans. Dev. Biol. 100, 64–119.
Talbot, W. S., Swyryd, E. A., and Hogness, D. S. (1993). Drosophila tissues with different metamorphic responses to ecdysone express different ecdysone receptor isoforms. Cell 73, 1323–1337. doi: 10.1016/0092-8674(93)90359-x
Tatar, M., Chien, S. A., and Priest, N. K. (2001). Negligible senescence during reproductive dormancy in Drosophila melanogaster. Am. Nat. 158, 248–258. doi: 10.1086/321320
Tenen, C. C., and Greenwald, I. (2019). Cell non-autonomous function of daf-18/PTEN in the somatic gonad coordinates somatic gonad and germline development in C. elegans dauer larvae. Curr. Biol. 29, 1064.e–1072.e. doi: 10.1016/j.cub.2019.01.076
Tepper, R. G., Ashraf, J., Kaletsky, R., Kleemann, G., Murphy, C. T., and Bussemaker, H. J. (2013). PQM-1 complements DAF-16 as a key transcriptional regulator of DAF-2-mediated development and longevity. Cell 154, 676–690. doi: 10.1016/j.cell.2013.07.006
Thomas, H. E., Stunnenberg, H. G., and Stewart, A. F. (1993). Heterodimerization of the Drosophila ecdysone receptor with retinoid X receptor and ultraspiracle. Nature 362, 471–475. doi: 10.1038/362471a0
Toni, L. S., and Padilla, P. A. (2016). Developmentally arrested Austrofundulus limnaeus embryos have changes in post-translational modifications of histone H3. J. Exp. Biol. 219, 544–552. doi: 10.1242/jeb.131862
Tothova, Z., and Gilliland, D. G. (2007). FoxO transcription factors and stem cell homeostasis: insights from the hematopoietic system. Cell Stem Cell 1, 140–152. doi: 10.1016/j.stem.2007.07.017
Tuckey, R. C., Cheng, C. Y. S., and Slominski, A. T. (2018). The serum vitamin D metabolome: what we know and what is still to discover. J. Steroid Biochem. Mol. Biol. 186, 4–21. doi: 10.1016/j.jsbmb.2018.09.003
Vincent, A. M., and Feldman, E. L. (2002). Control of cell survival by IGF signaling pathways. Growth Horm. IGF Res. 12, 193–197. doi: 10.1016/s1096-6374(02)00017-5
Vowels, J. J., and Thomas, J. H. (1992). Genetic analysis of chemosensory control of dauer formation in Caenorhabditis elegans. Genetics 130, 105–123.
Williams, K. D., Busto, M., Suster, M. L., So, A. K.-C., Ben-Shahar, Y., Leevers, S. J., et al. (2006). Natural variation in Drosophila melanogaster diapause due to the insulin-regulated PI3-kinase. Proc. Natl. Acad. Sci. U.S.A. 103, 15911–15915. doi: 10.1073/pnas.0604592103
Wirick, M. J., Cale, A. R., Smith, I. T., Alessi, A. F., Starostik, M. R., Cuko, L., et al. (2021). daf-16/FOXO blocks adult cell fate in Caenorhabditis elegans dauer larvae via lin-41/TRIM71. bioRxiv [Preprint] doi: 10.1101/2021.06.30.450637
Woll, S. C., and Podrabsky, J. E. (2017). Insulin-like growth factor signaling regulates developmental trajectory associated with diapause in embryos of the annual killifish Austrofundulus limnaeus. J. Exp. Biol. 220:jeb.151373. doi: 10.1242/jeb.151373
Wollam, J., Magomedova, L., Magner, D. B., Shen, Y., Rottiers, V., Motola, D. L., et al. (2011). The Rieske oxygenase DAF-36 functions as a cholesterol 7-desaturase in steroidogenic pathways governing longevity. Aging Cell 10, 879–884. doi: 10.1111/j.1474-9726.2011.00733.x
Wourms, J. P. (1972). The developmental biology of annual fishes. III. Pre-embryonic and embryonic diapause of in the eggs of annual fishes. J. Exp. Zool. 182, 389–414. doi: 10.1002/jez.1401820310
Xu, W.-H., Sato, Y., Ikeda, M., and Yamashita, O. (1995a). Molecular characterization of the gene encoding the precursor protein of diapause hormone and pheromone biosynthesis activating neuropeptide (DH-PBAN) of the silkworm, Bombyx mori and its distribution in some insects. Biochim. Biophys. Acta BBA Gene Struct. Expr. 1261, 83–89. doi: 10.1016/0167-4781(94)00238-x
Xu, W.-H., Sato, Y., Ikeda, M., and Yamashita, O. (1995b). Stage-dependent and temperature-controlled expression of the gene encoding the precursor protein of diapause hormone and pheromone biosynthesis activating neuropeptide in the silkworm, Bombyx mori. J. Biol. Chem. 270, 3804–3808. doi: 10.1074/jbc.270.8.3804
Yamashita, O., Shiomi, K., Ishida, Y., Katagiri, N., and Niimi, T. (2001). “Insights for future studies on embryonic diapause promoted by molecular analyses of diapause hormone and its action in Bombyx mori,” in Insect Timing: Circadian Rhythmicity to Seasonality, eds D. L. Denlinger, J. Giebultowicz, and D. S. Saunders (Amsterdam: Elsevier Science BV), 145–153. doi: 10.1016/b978-044450608-5/50044-1
Yao, T.-P., Segraves, W. A., Oro, A. E., McKeown, M., and Evans, R. M. (1992). Drosophila ultraspiracle modulates ecdysone receptor function via heterodimer formation. Cell 71, 63–72. doi: 10.1016/0092-8674(92)90266-f
Yoshiyama-Yanagawa, T., Enya, S., Shimada-Niwa, Y., Yaguchi, S., Haramoto, Y., Matsuya, T., et al. (2011). The conserved Rieske oxygenase DAF-36/Neverland Is a novel cholesterol-metabolizing enzyme. J. Biol. Chem. 286, 25756–25762. doi: 10.1074/jbc.m111.244384
Zhang, H., Lin, Y., Shen, G., Tan, X., Lei, C., Long, W., et al. (2017). Pigmentary analysis of eggs of the silkworm Bombyx mori. J. Insect. Physiol. 101, 142–150. doi: 10.1016/j.jinsphys.2017.07.013
Zhang, X.-S., Wang, T., Lin, X.-W., Denlinger, D. L., and Xu, W.-H. (2017). Reactive oxygen species extend insect life span using components of the insulin-signaling pathway. Proc. Natl. Acad. Sci. U.S.A. 114, E7832–E7840. doi: 10.1073/pnas.1711042114
Zheng, S., Chiu, H., Boudreau, J., Papanicolaou, T., Bendena, W., and Chin-Sang, I. (2018). A functional study of all 40 C. elegans insulin-like peptides. J. Biol. Chem. 293, 16912–16922. doi: 10.1074/jbc.ra118.004542
Zheng, X., Gong, Y., Kumar, D., Chen, F., Kuan, S., Liang, Z., et al. (2016). Effect of BBX-B8 overexpression on development, body weight, silk protein synthesis and egg diapause of Bombyx mori. Transgenic Res. 25, 507–516. doi: 10.1007/s11248-016-9947-3
Keywords: diapause, insulin/IGF, nuclear hormone receptor, hormone, developmental trajectory, life histories
Citation: Karp X (2021) Hormonal Regulation of Diapause and Development in Nematodes, Insects, and Fishes. Front. Ecol. Evol. 9:735924. doi: 10.3389/fevo.2021.735924
Received: 03 July 2021; Accepted: 08 September 2021;
Published: 30 September 2021.
Edited by:
Sarah Kelly McMenamin, Boston College, United StatesReviewed by:
Jason E. Podrabsky, Portland State University, United StatesCopyright © 2021 Karp. This is an open-access article distributed under the terms of the Creative Commons Attribution License (CC BY). The use, distribution or reproduction in other forums is permitted, provided the original author(s) and the copyright owner(s) are credited and that the original publication in this journal is cited, in accordance with accepted academic practice. No use, distribution or reproduction is permitted which does not comply with these terms.
*Correspondence: Xantha Karp, a2FycDF4QGNtaWNoLmVkdQ==
Disclaimer: All claims expressed in this article are solely those of the authors and do not necessarily represent those of their affiliated organizations, or those of the publisher, the editors and the reviewers. Any product that may be evaluated in this article or claim that may be made by its manufacturer is not guaranteed or endorsed by the publisher.
Research integrity at Frontiers
Learn more about the work of our research integrity team to safeguard the quality of each article we publish.