- Department of Biological Sciences, University of Manitoba, Winnipeg, MB, Canada
Many different aspects of an animal’s lifecycle such as its behavior, patterns of hormone activity, and internal clock time, can be affected by anthropogenic light at night (ALAN). Exposing an organism to ALAN during its early life could also have an impact on its development. Since photoperiod can trigger or schedule the migration timing of long-distance migratory birds, there is great potential for anthropogenic light to interact with photoperiod to affect timing. However, very little has been investigated regarding the impacts of ALAN on post-hatching development and migration timing. We investigated the impact of ALAN during nestling development in a long-distance migratory songbird to determine the potential impact on the timing of post-breeding movements in the wild. We experimentally manipulated the light by using programmable lighting, in the nest boxes of free-living nestlings of purple martin (Progne subis) in Manitoba, Canada. We exposed two groups of developing nestlings, from hatch to fledge date, to green or white LED lights (5 lux) during the night. We also included a control group that experienced natural, ambient light at night. We found that some adults abandoned their nests shortly after starting the experiment (4 of 15 nests in the white light treatment). For the nests that remained active, nestlings exposed to the white light treatment had higher weights (at day 20 or 22), later fledge dates (1.54 ± 0.37, 95% CI 0.80–2.28), and later colony departure date (2.84 ± 1.00, 95% CI 0.88–4.81), than young of the control group. Moreover, nestlings of both white and green light groups had longer nesting duration than nestlings of the control group. This study demonstrates the impact of ALAN on the development of post-breeding movement timing in nestlings of wild migratory birds. However, our results also indicate that green light may have less of an impact as compared to white light.
Introduction
With more than 50% of the global human population inhabiting cities (Nations, 2012), anthropogenic light at night (ALAN), or light pollution, has become one of the problems of urban sprawl for its impact on local environments (Rich and Longcore, 2005; Chepesiuk, 2009; Dominoni D. M., 2015). It is hypothesized that artificial light can be perceived as an extension of photoperiod in birds (Farner, 1964), where photoperiod can have a strong role in synchronizing internal clock time with seasonal rhythm (Berson et al., 2002). A study by Dominoni and Partecke (2015) on European blackbird (Turdus merula) showed that the impacts of light pollution on a bird’s physiology and seasonal activities are comparable to the influence of longer photoperiods. Therefore, light pollution through its impact on internal clock time which controls many physiological processes (Foster and Kreitzmann, 2004), could impact different aspects of many animal’s lifecycles, such as their natural behavior, and patterns of hormone activity (Rich and Longcore, 2005). Previous studies have revealed an impact of ALAN on the timing of activities such as the timing of singing of songbirds (e.g., Miller, 2006; Kempenaers et al., 2010; Da Silva et al., 2015), the timing of reproductive maturity (e.g, Dominoni and Partecke, 2015), and molt (Dominoni D. et al., 2013). For example, in European blackbird, urban light pollution (0.3 lux) resulted in an advance in their physiological phenotypes, where ALAN contributed to advances in the onset of reproductive development by 26 days (Dominoni D. et al., 2013). In general, and across the annual cycle, changes in timing as a result of exposure to ALAN may have negative fitness consequences, particularly if birds become mismatched with the timing of key resources needed for migration or breeding (Visser and Gienapp, 2019).
There is great potential for anthropogenic light to interact with natural photoperiod to influence perceived photoperiod and affect migration timing which could have fitness consequences (De Jong et al., 2015). However, there have been few studies on the impact of ALAN on the migration timing of animals, particularly birds. For example, Riley et al. (2013) found delays in the dispersal of Atlantic salmon (Salmo salar) fry who were exposed to ALAN. Also, Smith et al. (2021) showed that adult purple martins (Progne subis) who experienced ALAN for more than 10 nights, initiated spring migration 8 days earlier than others who experienced natural darkness. This advance in timing was not compensated for during migration and birds experiencing ALAN that had left earlier also arrived at their breeding grounds 8 days earlier, suggesting the potential for mismatch between bird timing and the availability of resources in early spring. Further studies with other species and at different times of year are now required to further improve our understanding of the impact of ALAN on migration timing. Further, to our knowledge, there has been no study that has investigated the ontogenetic effects of light pollution on movement timing of long-distance migratory songbirds.
In this study, we used purple martin which is a gregarious long-distance Nearctic-Neotropical migratory songbird that journeys 10–20,000 km annually between breeding sites across eastern North American and overwintering locations in South America (Fraser et al., 2012; Neufeld et al., 2021). Considering the potential interaction between light pollution and photoperiod that may impact timing, the objectives of this study were to determine the ontogenetic effects of ALAN during nesting on the subsequent development of post-breeding movement timing in young birds. For this purpose, we experimentally exposed free-living nestlings to artificial light. To also examine whether different spectra of light have different impacts, we used both white and green lights (long and near short wavelength) in our experiment. The different impacts of green versus white light in a lab study on daily rhythms of blue tit (Cyanistes caeruleus) (De Jong et al., 2017), provided context for our investigation of the impacts of different wavelengths on the timing of post-breeding movements in the wild.
We subsequently tracked individuals as they fledged using an automated telemetry system (Taylor et al., 2017) to allow us to determine the responses of their timing to the simulated light pollution. We predicted that the light treatments would have some impacts on physiology as well as on timing, which could be measured through their weight and departure timing. We predicted that nestlings of the experimental groups would have later fledge dates and post-fledge movement timing in comparison with the control group. As songbirds may perceive light pollution as similar to longer day length (Dominoni and Partecke, 2015), we expected that birds in the experimental groups would have later timing of post-breeding movements. This is because a longer day length at the study latitude would simulate an earlier calendar date, as days get shorter through the nesting period. It was also expected that development and weight gain in birds experiencing the light treatments would be slower than those in the control group, as they may be more active under constant light at night. Regarding the study results of De Jong et al. (2017) that both white and green light at an intensity of 5 lux had the same influence on daily rhythms of blue tit (C. caeruleus), we predicted the influence of both spectra of lights would be the same.
Materials and Methods
This study was conducted at two purple martin colonies in southern Manitoba, located just south of Winnipeg (49.7348° N, 97.1301° W) and at Altona (49.126748°, –97.570463°). Purple martins are dependent upon human-supplied houses for nesting, which have multiple nest boxes per housing unit (Brown et al., 2021). We used five purple martin houses for our study. Four of these houses had a total of 14 nest boxes each and the fifth house had 32 nest boxes. We used 33 nest boxes from the location south of Winnipeg and 14 nest boxes from the location at Altona in our experiment. To investigate the impact of ALAN on the timing of post-breeding movements of juveniles, the light within purple martin nest boxes was manipulated during dark hours. Light-emitting diodes (LED) in two colors (white and green) were attached to the ceiling of each nest box before nestlings hatched. Light emitted by the LEDs was directed downward toward the nests (Raap et al., 2016b, a). To control the time that lights turned on and off, LEDs were connected to an Arduino unit (a circuit board that can be programmed with associated software to set the light schedules) and a real-time clock mounted on a circuitboard. The LEDs were programmed to turn on at sunset, stay on during the entire night, and turn off at sunrise of the next day (Supplementary Figure 1). The experimental period began 3–4 days before the hatch date of each nest and continued until the fledge date. The nest boxes were divided into three different experimental groups: controls (no light, dark), treatment group 1 (white light), and treatment group 2 (green light). Light intensity for both treatment groups was set at 5 lux. The dim, 5 lux was chosen to align with De Jong et al. (2017), where in the lab there was no measured difference in the impact between green and white light at this intensity. Therefore, this provided us with the opportunity to compare results and investigate impacts on the timing of post-breeding movements on a migratory species in the wild. In total, each of the control and green light groups included 16 nest boxes each, and the white light group included 15 nest boxes. To determine the fledge date and colony departure date of young, we used the Motus Wildlife Tracking System,1 which is a continent-wide automated radio-telemetry array of receiver stations (Taylor et al., 2017). At each of our research colonies, we installed a Motus receiver, within 8–70 m of the cavities. We randomly selected individuals (3–5 where available and of adequate weight for tagging) from each nest box and equipped them with individually coded radio nanotags (NTQB2-3-2 Lotek Inc.) (0.62 g, 12 × 6 × 5 mm in length, width, and height, respectively) using a leg-loop harness design (Rappole and Tipton, 1991; Streby et al., 2015) made of black elastic sewing thread (∼0.5 mm). Tag deployment was conducted when nestlings were near fledging at the age of 20–22 days (post-hatch). At the time of tagging, the weight of each nestling was recorded by using a digital scale with a resolution of 0.01 g. The weight of a tag and harness was less than 3% of the weight of the juveniles (∼54.27 grams) (the average weight of nestlings). Each nanotag emitted a signal every 29 s and had a battery life of approximately 367 days.2 After turning on the lights, 4 of the nests in the white light group were abandoned. In total, 61, 55, and 49 tags were deployed on nestlings from nests that remained active in each of the control, green and white light groups, respectively.
The fledge date and colony departure date were determined by using a combination of variation in signal strength of each nanotag and complementary nest checks every other day. The date of fledging was determined when after a constant signal fluctuation (indicating the tagged bird is in the nest at a constant distance from the receiver), we observed a great fluctuation in signal strength which indicates fledging from the cavity. After this great fluctuation of signal strength, we observed repetition of this pattern which shows the fledged bird was rapidly changing position in relation to the receiver. Colony departure date was determined according to the fading signals of the tagged birds and the last detections of the tagged bird at the colony site (Supplementary Figure 2). Biologically unrealistic false positive detections were omitted (e.g., from distant receivers pre-fledge). Where tag signals ceased (e.g., owing to tag malfunction, predation, or another unknown cause) or in cases where a constant signal indicated a tag had fallen off or a bird had died, data were removed from further analysis.
All data collection procedures and experiments were conducted in accordance with the guidelines of the University of Manitoba’s Animal Care Committee who have approved this project [Animal Care Protocol Number F18-031/1(AC11388)].
Data Analysis
For examining the effect of ALAN on the timing of fledge date of juveniles and their nesting duration linear mixed-effects models (LMMs) were fit by REML using the “lme4” package (Bates et al., 2014). The variables of weight (gram), first egg date, treatment (green or white light), and the number of nestmates were assigned as fixed effects and cavity ID and colony as the random effects. As only three nests had a second-year parent and all other adults were after-second year, the age of parents was not included in the analyses. To investigate the impact of ALAN on the duration (days) at the colony (hatch to departure) and colony departure date, we used LMMs with the same variables of first egg date, treatment (green or white light), and the number of nestmates as fixed and cavity ID and colony as random effects, except for the weight of the young as this was not possible to measure after their fledging. Preliminary investigation using likelihood ratio tests revealed that the random effect of the colony was not significant in the models (fledge date: χ2 = 0, P = 1, duration in the nest: χ2 = 0, P = 1; colony departure date: χ2 = 0, P = 0.99; duration at the colony: χ2 = 0, P = 1), and the models only converged with random effect of cavity ID. Therefore, to meet model parsimony, the factor of colony was omitted from further analysis. The distribution of residuals of each model was assessed to meet the assumption of normality and equality of variance (Zuur et al., 2010). The collinearity of variables in each model was assessed, which was less than 2 for all variables. To run the possible candidate models from the full model, Akaike Information Criteria corrected for small sample size (AICc) was used (Burnham and Anderson, 2002) through the package “MuMIn” (Barton, 2019). The best model among the competitive models with ΔAICc < 2 was selected (Supplementary Tables 1–3) according to the highest value of the marginal R2 (variance explained by the fixed effects) and the conditional R2 (variance explained by the whole model) (Barton, 2019). ANCOVA was used to test the interaction of treatments (categorical variable) with first egg date (continuous variable) for both dependent factors, fledge date and colony departure date (McDonald, 2014). One-way ANOVA was used to investigate whether the mean weight of juveniles is different among different groups (white light, green light, and control), and where applicable, to explore the differences among weight means of three groups, the package “lmerTest” (Kuznetsova et al., 2017) was used to run Tukey HSD tests for post hoc analyses.
The survival rate of young of each nest box was calculated by dividing the number of fledged young by the number of hatched nestlings of each nest box. Due to the non-normal distribution of data, a Kruskal-Wallis test was used to compare the survivability rate among three groups (white light, green light, and control groups). All analyses were conducted in R version 3.6.3 (R Core Team, 2020).
Results
We tracked the fledge date of 61, 47, and 46 individuals of the control, green light and white light groups, respectively. Among these tagged nestlings, we were able to track the colony departure dates of 45 individuals from the control group, and 20 and 33 individuals of the green and white light groups, respectively.
Nesting Duration and Fledge Date
The average nesting duration of the control, green light and white light groups were (mean ± SEM) 28.16 ± 0.15, 29 ± 0.15, and 30.11 ± 0.23 days, respectively. Nestlings exposed to green light and white light spent (estimate ± SE) 0.78 ± 0.32 (95% CI 0.14–1.41) and (estimate ± SE) 1.67 ± 0.34 (95% CI 1.00–2.34) days, respectively, longer in the nest than those nestlings who experienced natural darkness during the night (Table 1). Moreover, one additional nestmate and a 1-gram increase in weight resulted in nesting duration that was longer by (estimate ± SE) 0.30 ± 0.13 (95% CI 0.03–0.57) and (estimate ± SE) 0.04 ± 0.02 (95% CI 0.00–0.08) days, respectively (Table 1).
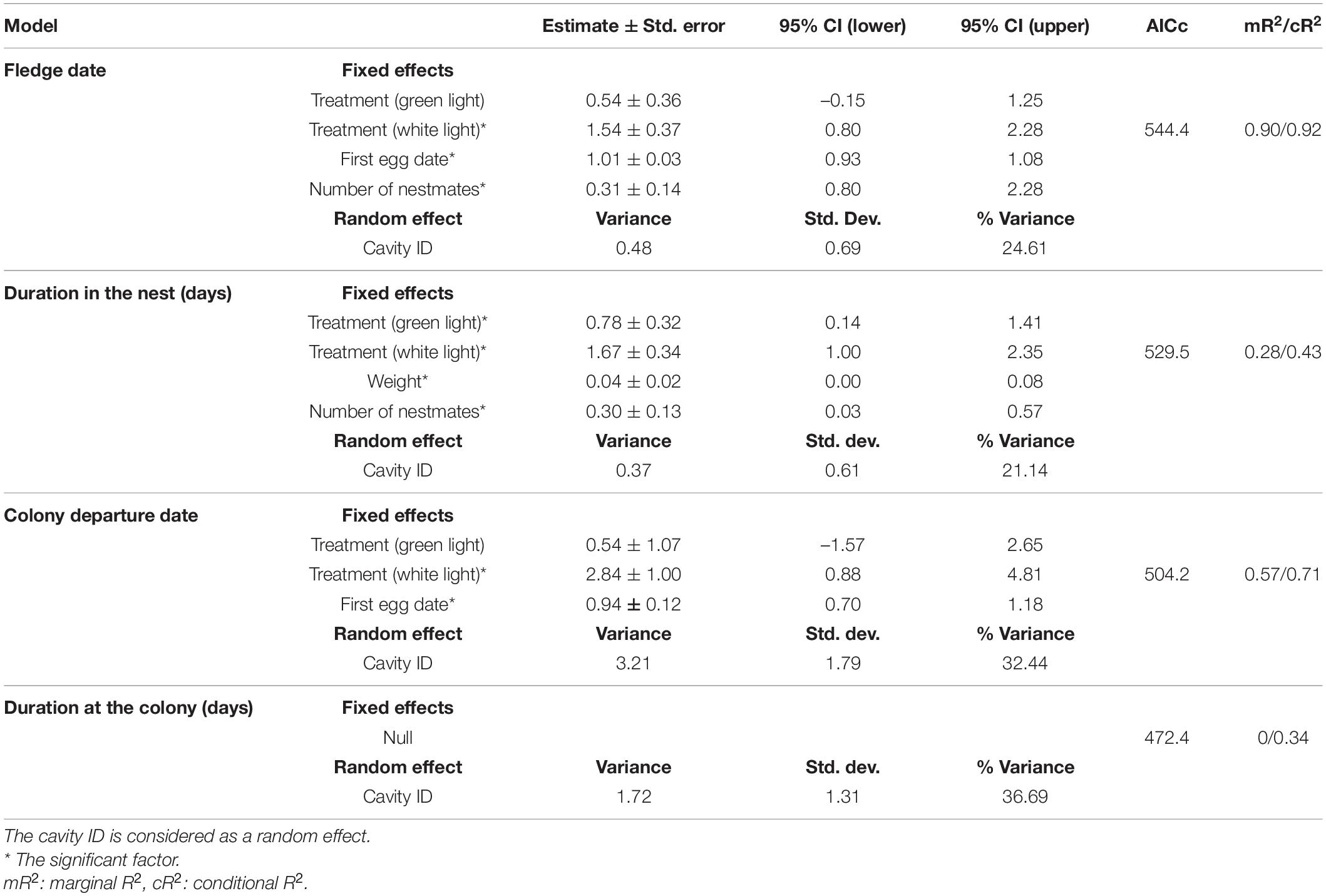
Table 1. The best models of the linear mixed-effects analysis of effects of ALAN, weight, first egg date, number of nestmates on nesting period, fledge date, and effects of ALAN, first egg date, nestmate numbers on duration of staying at the colony and colony departure date.
Overall, fledge dates ranged from 12 July to 3 August. Average fledge dates of control, green light and white light groups were dates 21 July (mean ± SEM) (203 ± 0. 59), 24 July (206 ± 0.65), and 25 July (207.39 ± 0.52), respectively. Nestlings exposed to white light fledged (estimate ± SE) 1.54 ± 0.37 (95% CI 0.80–2.28) days later than nestlings of the control group (Table 1). There was not a significant difference between the fledge dates of nestlings of the green light group and the control group. Moreover, first egg dates that were one day later resulted in nestling fledge dates that were (estimate ± SE) 1.01 ± 0.03 (95% CI 0.93–1.08) days (Figure 1A and Table 1) later. One more nestmates in a cavity delayed fledge date about (estimate ± SE) 0.31 ± 0.14 (95% CI 0.02–0.60) days (Table 1). The results of ANCOVA showed the effect of first egg date on the fledge date is independent of the treatments or different colors of ALAN and it is assumed the slopes are similar (Figure 1A and Supplementary Table 4).
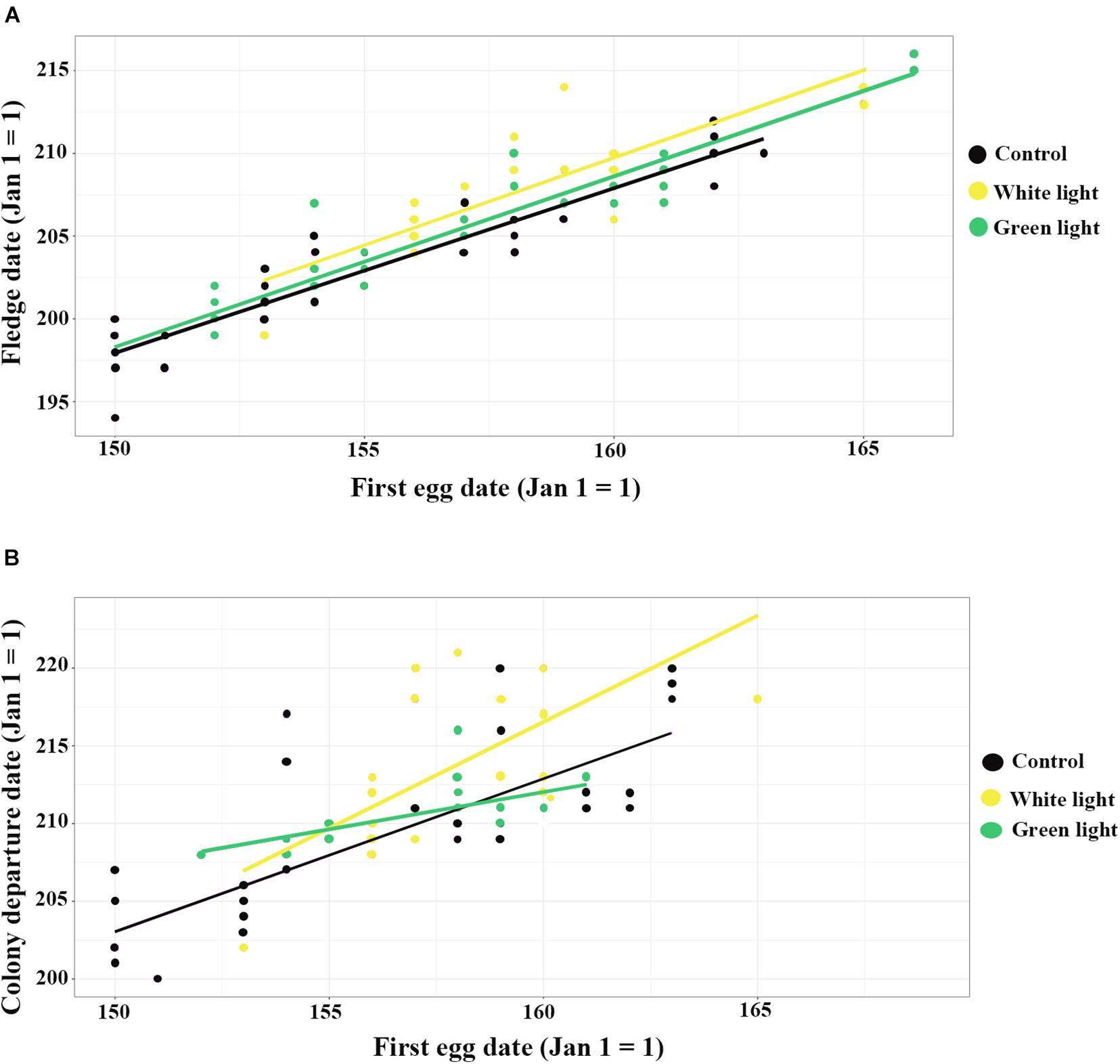
Figure 1. The influence of ALAN (white and green lights) on fledge date and colony departure date, (A) shows the correlation between first egg date and fledge date; (B) shows the correlation between first egg date and colony departure date. Yellow = white light, Green: green light, and Black = control.
Duration at the Colony and Colony Departure Date
The best model of duration at the colony did not include any of the fixed effects as influential factors (Table 1). Nestlings who experienced white light at night departed the colony (estimate ± SE) 2.84 ± 1.00 (95% CI 0.88–4.81) days later than those of the non-treatment group (Table 1). The results of ANCOVA showed the effect of first egg date on the colony departure date was similar among the different treatments of ALAN (Figure 1B and Supplementary Table 4). Moreover, one day delay in first egg date resulted in nestlings departing the colony (estimate ± SE) 0.94 ± 0.12 (95% CI 0.71–1.18) days later (Figure 1B and Table 1).
Weight
The average weight of nestlings of the control, green light and white light groups at day 20–22 were (mean ± SEM) 53.50 ± 0.63, 53.85 ± 0.65, and 56.10 ± 0.92, respectively (Figure 2). The differences of the mean weight of nestlings among groups were significant (DF = 2, F = 3.27, p = 0.04). The Tukey post hoc test showed the mean weight of nestlings of the white light group was significantly more than those in the control group (estimate ± SE) (2.6 ± 1.06, p = 0.03) when they were 20–22 days old. There was no significant difference between the weight of nestlings of the green light group and the weight of nestlings of both the control and white light groups.
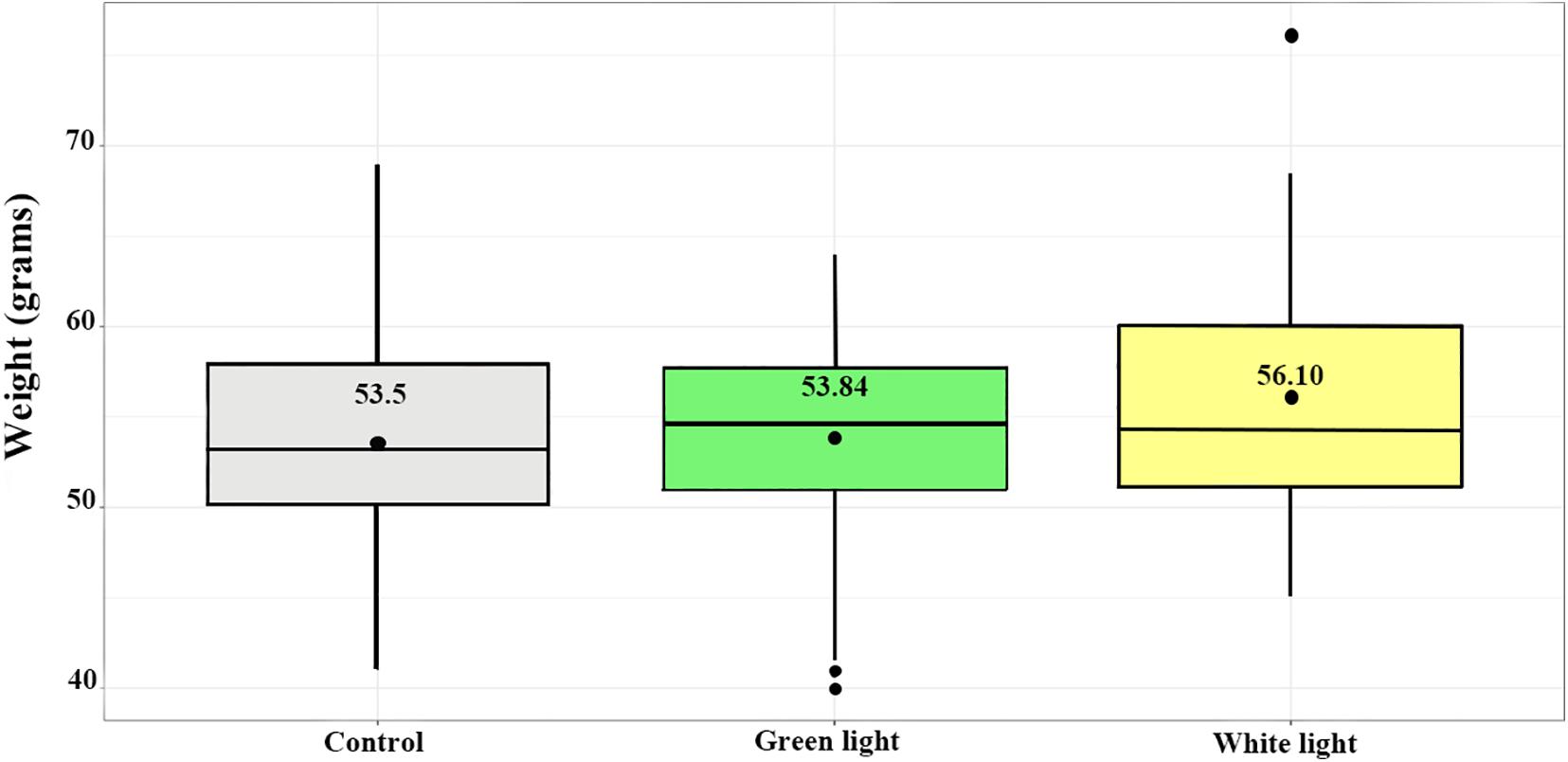
Figure 2. Weights of young in the treatment groups (white and green lights) and control group. Boxes extend to upper and lower quartiles; the line indicates the median and the black point at the middle of the boxes indicates the mean. Whiskers extend to maximum and minimum values; outliers are indicated by filled points.
Survivability Rate
Three of 16 control group nests and 4/16 green light group nests did not fledge completely with 6 and 9 nestlings lost in each group, respectively. In the white light group, 4 out of 15 nests were abandoned at the beginning of the experiment and 2 out of the remaining 11 nests did not fledge completely. This resulted in a total of 10 nestlings lost from the white light group. There was not a significant difference in the nest survivability rate between the treatment groups, (white and green light groups) and the control group, nor between the two treatment groups (χ2 = 0.17, p = 0.91). The actual numbers of fledged young were 79, 74 and 49 from the control, green light, and white light groups, respectively. Nestlings that disappeared between nest checks were not found and may have been taken by avian predators (Brown et al., 2021), as predator guards would prevent terrestrial predators from accessing the nest boxes.
Discussion
In this study, we demonstrate for the first time that ALAN impacts the timing of the post-breeding movements of juveniles of a long-distance migratory songbird. We examined the ALAN impacts of different spectra of light (white and green lights at 5 lux) on the duration of nesting and timing of fledge and post-breeding movements. We found that the effects of different spectra differed for the post-breeding movement timing of juvenile purple martins. Our results reveal that exposing nestlings to white light with an intensity of 5 lux during the night, resulted in later fledging and colony departure as compared to nestlings who experienced either green light or natural darkness. Thus, our data reveal important effects of ALAN on timing but that these differ by spectra of light. We found that green light with an intensity of 5 lux did not influence the timing of the post-breeding movement of young purple martins. However, nestlings exposed to either white or green light had a longer nesting duration than nestlings of the control group (that experienced natural darkness).
Evidence to date suggests that ALAN can influence the perception of photoperiod by birds (De Jong et al., 2015), influencing their internal clock time and consequently impacting biological functions and fitness (Farner, 1964; Dominoni D. M., 2015). Previous studies which investigated the impact of light pollution on circadian rhythms of songbirds revealed that it can cause a phase shift in their circadian rhythm (Gaston et al., 2013) and advance or delay the onset and offset of their daily activities such as singing (e.g., Kempenaers et al., 2010; Da Silva et al., 2014), foraging activity (Russ et al., 2015), and timing of reproduction (Kempenaers et al., 2010; De Jong et al., 2015). Moreover, egg-laying date is influenced by day length as one of the important zeitgebers (Lambrechts et al., 1997; Da Silva et al., 2015). De Jong et al. (2015) found when the temperature was low in spring in comparison with a warmer spring, light pollution at night was perceived as a longer photoperiod by great tit (Parus major) which changed the onset of egg-laying.
In addition to changes in the timing of nesting activities due to ALAN, a study by Smith et al. (2021), demonstrated the advancement of spring migration departure of adult purple martins who experienced light pollution during their overwintering period. One of the reasons posed for this advance was that ALAN led to a perception of a longer day length which mimicked a later calendar date, causing earlier development of reproductive organs (Smith et al., 2021). In this study, we showed an ontogenetic effect of ALAN at breeding sites on nestlings, which resulted in later post-breeding movements. This delay could be due to the impact of ALAN in the nest on the growth rate of nestlings, where birds exposed to ALAN are heavier leading to a delay in timing. An earlier study that compared the weights of nestlings exposed to white light (3 lux) with controls found similar results where ALAN-exposed nestlings gained more weight than those in the control group (Gagné, 2019). Previous studies revealed that a typical pattern of weight gain and loss in nestling purple martins is for them to lose weight as they approach fledge date, possibly to achieve a weight more suited to fledging and first flights (Allen and Nice, 1952; Dellinger and Rogillio, 1991; Gagné, 2019). White light may influence this natural pattern, changing the metabolism or the proportion of rest and active periods of the nestlings and increasing begging for food (Raap et al., 2016c) which could consequently prolong the duration of feeding by adults. This aligns with the results of a study by Titulaer et al. (2012) that showed an increase in the feeding rate of great tit females when nestlings were exposed to ALAN (10 lux) while they were between 9 and 16 days old. However, exposing free-living great tit nestlings to artificial light at night (3 lux), even for two nights, during their development led to substantial impacts on their physiological condition (Raap et al., 2016b) and increased their activity levels which resulted in the nestlings having no weight gain for the two nights of the experimental treatment (Raap et al., 2016a). Our results align with these earlier studies as we found that the weight of nestlings of the white light group was significantly more than the nestlings of the control group. We infer that given that nestling martins tend to lose weight just before fledging, that the higher weight of white light exposed nestlings may have led to the later fledge dates that we observed. However, we found no significant difference in weight between individuals in the green group and the other two groups.
While the ontogenetic effect of photoperiod on circadian rhythms of mammals has been demonstrated (e.g., Ciarleglio et al., 2011), there is scant information about the ontogenetic effects of daylength on avian migration timing (Knudsen et al., 2011). This is particularly so for the potential ontogenetic effects of light pollution. The ontogenetic effect of hatch date on spring migration timing of pied flycatcher and spring arrival time of Arctic terns (Sterna paradisea) has been suggested by Møller et al. (2009) and Both (2010), respectively. Moreover, in a previous study, we found phenotypic plasticity of post-breeding movement timing of young purple martin to an experimentally extended day length during their nesting (Bani Assadi and Fraser, 2021). In our study, the longer nesting period and later fledge date of nestlings exposed to the white light treatment, could potentially indicate a plasticity of post-breeding movements timing of nestlings to the ontogenetic effects of light during the nesting period, with carry-over effects on the timing of their colony departure date. How long these timing effects may last across the rest of the annual cycle, and how they trade-off these delays in timing in the next stage of their life cycle, are important areas of investigation for future research. Delays in timing may be compensated for during fall migration, or during the winter. In wood thrushes, fall migration may mitigate the carry-over effect of late-breeding timing (Stutchbury et al., 2011; Catry et al., 2013) and Gow et al. (2019) found that stationary periods during the non-breeding season in tree swallows may act as a timing reset period, removing carry-over effects on timing from the breeding season and fall migration. However, some impacts on timing may have longer-lasting effects, particularly on juvenile birds. Ouwehand et al. (2017) showed that the carry-over effect of an experimentally delayed hatch for juvenile pied flycatchers extended to spring arrival date back at breeding sites in the following year. While we did not track the timing of our experimental birds to the subsequent spring, based upon the results of Ouwehand et al. (2017), we would expect to observe a carry-over effect of ALAN on their spring arrival date.
Similar to a study of De Jong et al. (2015), which showed a lack of impact of ALAN on the survivability rate of great tits nestlings, we did not detect a difference in the survivability rate (fledging) of the young among our three groups (white light, green light, and controls). However, previous studies have revealed some non-lethal but negative impacts of ALAN via increases in stress hormones (Ouyang et al., 2015) and decreases in melatonin levels (Dominoni D. M. et al., 2013). For example, a field study by Raap et al. (2016b) showed that exposing great tit nestlings to ALAN (0.3 lux) for two nights when they were 13 days old caused a deterioration of their immunity and health condition via a decrease in melatonin and an increase in oxidative stress and stress hormones. Melatonin is secreted by the pineal gland at night and plays an important role in maintaining the circadian rhythm (Raap et al., 2015). A disruption in circadian rhythm can impact several immune responses (Arjona et al., 2012). In our study, despite the lack of influence of two spectra of lights (white and green) on the survivability rate of young, we cannot rule out more subtle effects on their health condition and how this may influence migration. This would therefore be an important future research avenue, as the impact of ALAN on complex neuroendocrine functions, and how this may differ as they traverse different environments, is unknown (Haldar and Singh, 2001).
How different wavelengths of light may impact the behavior and physiology of birds have yielded mixed results across studies, species and time of year. For example, in a field study, Ouyang et al. (2015) showed that the concentration of stress hormone in great tits was greater when nests were closer to white lights in comparison with individuals with nests near green lights (8.2 ± 0.3 lux). Moreover, wavelengths around the blue spectrum have been demonstrated to be more influential on the reproductive physiology of birds (Dominoni D. M., 2015) and laying date (De Jong et al., 2015) than other spectra of light. In our field study, white light of higher intensity (5 lux) was influential on post-breeding movement timing of wild young purple martin, while green light with the same intensity did not have any impact. In contrast with our findings, a laboratory study by De Jong et al. (2017) revealed that at low intensity (0.5 and 1.5 lux), the daily rhythm of blue tits (C. caeruleus) was more disturbed under white and red lights than green light. However, they found that at a higher range of intensities (5 lux), both white and green lights had the same negative impact on the circadian rhythms of a blue tit. In another study De Jong et al. (2015), found that the lay date of great tit was influenced by white and green lights at night (8.2 ± 0.3 lux out of the nest, but 0.05 lux in the nests), however, pied flycatchers’ lay date was not impacted by ALAN (De Jong et al., 2015). In contrast with this result, a study by Poot et al. (2008), showed an influence of the long-wavelength spectrum (red and white lights) where it caused nocturnal migratory birds to be disoriented during flight. In general, the greater influence of white light at low intensity across studies could be due to its greater penetration of the skull as compared to green light, where it may have a corresponding impact on photoreceptors (Hartwig and van Veen, 1979).
In addition to the impacts of our experimental treatments, we found that other factors also impacted timing as expected. For example, we found that first egg date was an influential factor in the timing of fledge date and colony departure date. The number of nestmates also impacted fledge date, which aligns with the results of Wagner et al. (1996), where an increase in the number of nestlings of purple martins increases competition for food and therefore they require more time to reach the optimal body condition for fledging. Among the zeitgebers that may influence timing, photoperiod is expected to play the largest role in synchronizing internal clock time (Gwinner, 1996; Åkesson and Helm, 2020). Therefore, it was expected that longer day lengths experienced by birds that hatched earlier would induce them to have earlier fledge dates (Coppack and Pulido, 2004). However, our investigation did not show any interactions between the experimental light treatments and first egg date.
Conclusion
This study demonstrated the ontogenetic effects of white ALAN during the nesting period on the timing of post-breeding movements of juvenile birds. However, we did not find any significant difference in the timing of post-breeding movements for birds that were exposed to green ALAN as compared to controls. The potential for negative carryover effects on other stages of the annual cycle (Norevik et al., 2017) or whether the carryover effects of light pollution on migration timing are compensated for during migration or during the overwintering period (Senner et al., 2014; Briedis et al., 2018; Gow et al., 2019) requires further investigation. The negative impact of white light on the circadian or circannual rhythm of young migratory songbirds that we demonstrate, and the lack of influence of green light leads to the recommendation of using a shorter wavelength (green light) for illuminating places that are close to breeding sites.
Data Availability Statement
The raw data supporting the conclusions of this article will be made available by the authors, without undue reservation.
Ethics Statement
The animal study was reviewed and approved by University of Manitoba’s Animal Care Committee [Animal Care Protocol Number F18 031/1(AC11388)].
Author Contributions
KF designed, planned, and supervised the research, provided funding, and edited the manuscript. SB planned and performed the research, analyzed the data, and wrote the manuscript.
Funding
Funding and other supports were provided by John R. Evans Leaders Fund, Canadian Foundation for Innovation, Research Manitoba, the Natural Sciences and Engineering Research Council’s Discovery Grant Program, the Connie Holland Bird Study Fund, and the University of Manitoba.
Conflict of Interest
The authors declare that the research was conducted in the absence of any commercial or financial relationships that could be construed as a potential conflict of interest.
Publisher’s Note
All claims expressed in this article are solely those of the authors and do not necessarily represent those of their affiliated organizations, or those of the publisher, the editors and the reviewers. Any product that may be evaluated in this article, or claim that may be made by its manufacturer, is not guaranteed or endorsed by the publisher.
Acknowledgments
We thank Gail Davoren, Colin Garroway, and Saman Muthukumarana for helpful comments on earlier drafts of this manuscript. We thank Leanne Neufeld for her assistance with fieldwork. We especially thank purple martin colony managers Alan Enns, Paul and Maxine Clifton for their patience and assistance with this research.
Supplementary Material
The Supplementary Material for this article can be found online at: https://www.frontiersin.org/articles/10.3389/fevo.2021.735112/full#supplementary-material
Supplementary Figure 1 | Schematic of the ALAN treatment. 5 lux of artificial white or green light were turned on at sunset and off at sunrise.
Supplementary Figure 2 | The panel shows detection data from a Motus receiver station for an individual bird. The colors represent various antennae at the station. Signal strength shows fledging date and time for hatch-year purple martin (first stage of post-fledging movement), with the initiation of fledging on July 24th, at 21:16 GMT and departure from the colony (second stage of post-fledging movement) on July 28th, at 12:00 GMT. The blank spaces demonstrate the moments that the bird left the vicinity of the tower. The signal strength before fledge date shows the time that the bird spent in the cavity.
Footnotes
References
Åkesson, S., and Helm, B. (2020). Endogenous programs and flexibility in bird migration. Front. Ecol. Evol. 8:78. doi: 10.3389/fevo.2020.00078
Allen, R. W., and Nice, M. M. (1952). A study of the breeding biology of the Purple Martin (Progne subis). Am. Mid. Nat. 47, 606–665. doi: 10.2307/2422034
Arjona, A., Silver, A. C., Walker, W. E., and Fikrig, E. (2012). Immunity’s fourth dimension: approaching the circadian–immune connection. Trends Immunol. 33, 607–612. doi: 10.1016/j.it.2012.08.007
Bani Assadi, S., and Fraser, K. C. (2021). Experimental manipulation of photoperiod influences migration timing in a wild, long-distance migratory songbird. Proc. R. Soc. B. 288:20211474. doi: 10.1098/rspb.2021.1474
Barton, K. (2019). MuMIn: Multi-Model Inference, R Package Version 1.42.1. Available online at: https://CRAN.R-project.org/package=MuMIn
Bates, D., Mächler, M., Bolker, B., and Walker, S. (2014). Fitting linear mixed-effects models using lme4. arXiv [Preprint]. arXiv:1406.5823
Berson, D. M., Dunn, F. A., and Takao, M. (2002). Phototransduction by retinal ganglion cells that set the circadian clock. Science 295, 1070–1073. doi: 10.1126/science.1067262
Both, C. (2010). Flexibility oftiming ofavian migration to climate change masked by environmental constraints en route. Curr. Biol. 20, 243–248. doi: 10.1016/j.cub.2009.11.074
Briedis, M., Krist, M., Král, M., Voigt, C. C., and Adamík, P. (2018). Linking events throughout the annual cycle in a migratory bird-non-breeding period buffers accumulation of carry-over effects. Behav. Ecol. Sociobiol. 72:33.
Brown, C. R., Airola, D. A., and Tarof, S. (2021). Purple Martin (Progne subis), version 2.0. In Birds of the World, ed. P. G. Rodewald (Ithaca, NY: The Cornell Lab of Ornithology).
Burnham, K. P., and Anderson, D. R. (2002). A Practical Information-Theoretic Approach. Model Selection And Multimodel Inference. Vol. 10, New York, NY: Springer.
Catry, P., Dias, M. P., Phillips, R. A., and Granadeiro, J. P. (2013). Carry-over effects from breeding modulate the annual cycle of a long-distance migrant: an experimental demonstration. Ecology 94, 1230–1235. doi: 10.1890/12-2177.1
Chepesiuk, R. (2009). Missing the dark: health effects of light pollution. Environ. Health Perspect. 117, A20–A27.
Ciarleglio, C. M., Axley, J. C., Strauss, B. R., Gamble, K. L., and McMahon, D. G. (2011). Perinatal photoperiod imprints the clock. Nat. Neurosci. 14, 25–27.
Coppack, T., and Pulido, F. (2004). Photoperiodic response and the adaptability of avian life cycles to environmental change. Adv. Ecol. Res. 35, 131–150. doi: 10.1016/s0065-2504(04)35007-5
Da Silva, A., Samplonius, J. M., Schlicht, E., Valcu, M., and Kempenaers, B. (2014). Artificial night lighting rather than traffic noise affects the daily timing of dawn and dusk singing in common European songbirds. Behav. Ecol. 25, 1037–1047. doi: 10.1093/beheco/aru103
Da Silva, A., Valcu, M., and Kempenaers, B. (2015). Light pollution alters the phenology of dawn and dusk singing in common European songbirds. Phil. Trans. R. Soc. B Biol. Sci. 370:20140126. doi: 10.1098/rstb.2014.0126
De Jong, M., Caro, S. P., Gienapp, P., Spoelstra, K., and Visser, M. E. (2017). Early birds by light at night: effects of light color and intensity on daily activity patterns in blue tits. J. Biol. Rhythms 32, 323–333. doi: 10.1177/0748730417719168
De Jong, M., Ouyang, J. Q., Da Silva, A., van Grunsven, R. H., Kempenaers, B., Visser, M. E., et al. (2015). Effects of nocturnal illumination on life-history decisions and fitness in two wild songbird species. Phil. Trans. R. Soc. B Biol. Sci. 370:20140128. doi: 10.1098/rstb.2014.0128
Dellinger, T. B., and Rogillio, C. (1991). The graphic development of baby Purple Martins. Purple Martin Update 3, 6–11.
Dominoni, D. M. (2015). The effects of light pollution on biological rhythms of birds: an integrated, mechanistic perspective. J. Ornithol. 156, 409–418. doi: 10.1007/s10336-015-1196-3
Dominoni, D. M., and Partecke, J. (2015). Does light pollution alter daylength? A test using light loggers on free-ranging European blackbirds (Turdus merula). Phil. Trans. R. Soc. B Biol. Sci. 370:20140118. doi: 10.1098/rstb.2014.0118
Dominoni, D., Quetting, M., and Partecke, J. (2013). Artificial light at night advances avian reproductive physiology. Proc. R. Soc. B Biol. Sci. 280:20123017. doi: 10.1098/rspb.2012.3017
Dominoni, D. M., Goymann, W., Helm, B., and Partecke, J. (2013). Urban-like night illumination reduces melatonin release in European blackbirds (Turdus merula): implications of city life for biological time-keeping of songbirds. Front. Zool. 10:1–11. doi: 10.1186/1742-9994-10-60
Farner, D. S. (1964). The photoperiodic control of reproductive cycles in birds. Am. Sci. 52, 137–156.
Fraser, K. C., Stutchbury, B. J., Silverio, C., Kramer, P. M., Barrow, J., Newstead, D., et al. (2012). Continent-wide tracking to determine migratory connectivity and tropical habitat associations of a declining aerial insectivore. Proc. R. Soc. B Biol. Sci. 279, 4901–4906. doi: 10.1098/rspb.2012.2207
Foster, R. G., and Kreitzmann, L. (2004). Rhythms of Life: The Biological Clocks That Control the Daily Lives of Every Living Thing. New Haven, NH: Yale University Press.
Gagné, M. (2019). The Effect Of Artificial Light At Night (Alan) On The Growth And Migration Timing Of Purple Martins (Progne Subis). Unpublished honours Thesis University of Manitoba, Winnipeg.
Gaston, K. J., Bennie, J., Davies, T. W., and Hopkins, J. (2013). The ecological impacts of nighttime light pollution: a mechanistic appraisal. Biol. Rev. 88, 912–927. doi: 10.1111/brv.12036
Gow, E. A., Burke, L., Winkler, D. W., Knight, S. M., Bradley, D. W., Clark, R. G., et al. (2019). A range-wide domino effect and resetting of the annual cycle in a migratory songbird. Proc. R. Soc. B Biol. Sci. 286:20181916. doi: 10.1098/rspb.2018.1916
Gwinner, E. (1996). Circadian and circannual programmes in avian migration. J. Exp. Biol. 199, 39–48. doi: 10.1242/jeb.199.1.39
Haldar, C., and Singh, S. S. (2001). Seasonal changes in melatonin and immunological adaptations in birds. J. Endocrinol. Reprod. 5, 13–24.
Hartwig, H. G., and van Veen, T. (1979). Spectral characteristics of visible radiation penetrating into the brain and stimulating extraretinal photoreceptors. J. Comp. Physiol. 130, 277–282. doi: 10.1007/bf00614615
Kempenaers, B., Borgström, P., Loës, P., Schlicht, E., and Valcu, M. (2010). Artificial night lighting affects dawn song, extra-pair siring success, and lay date in songbirds. Curr. Biol. 20, 1735–1739. doi: 10.1016/j.cub.2010.08.028
Knudsen, E., Lindén, A., Both, C., Jonzén, N., Pulido, F., Saino, N., et al. (2011). Challenging claims in the study of migratory birds and climate change. Biol. Rev. 86, 928–946.
Kuznetsova, A., Brockhoff, P. B., and Christensen, R. H. B. (2017). lmerTest Package: tests in linear mixed effects models. J. Stat. Softw. 82, 1–26. doi: 10.18637/jss.v082.i13
Lambrechts, M. M., Blondel, J., Maistre, M., and Perret, P. (1997). A single response mechanism is responsible for evolutionary adaptive variation in a bird’s laying date. PNAS 94, 5153–5155. doi: 10.1073/pnas.94.10.5153
McDonald, J. H. (2014). Handbook of Biological Statistics, 3rd Edn. Baltimore, MD: Sparky House Publishing.
Miller, M. W. (2006). Apparent effects of light pollution on singing behavior of American robins. Condor 108, 130–139. doi: 10.1650/0010-5422(2006)108[0130:aeolpo]2.0.co;2
Møller, A. P., Flensted-Jensen, E., and Mardal, W. (2009). Adjustment of the annual cycle to climatic change in a long-lived migratory bird species. Curr. Zool. 55, 92–101. doi: 10.1093/czoolo/55.2.92
Nations, U. (2012). World Urbanization Prospects: The 2014 Revision, CD-ROM Edn. New York, NY: DESA.
Neufeld, L. R., Muthukumarana, S., Fischer, J. D., Ray, J. D., Siegrist, J., and Fraser, K. C. (2021). Breeding latitude is associated with the timing of nesting and migration around the annual calendar among Purple Martin (Progne subis) populations. J. Ornithol. 18, 1–16.
Norevik, G., Åkesson, S., and Hedenström, A. (2017). Migration strategies and annual space-use in an Afro-Palaearctic aerial insectivore–the European nightjar Caprimulgus europaeus. J. Avian Biol. 48, 738–747.
Ouwehand, J., Burger, C., and Both, C. (2017). Shifts in hatch dates do not provide pied flycatchers with a rapid ontogenetic route to adjust offspring time schedules to climate change. Funct. Ecol. 31, 2087–2097. doi: 10.1111/1365-2435.12940
Ouyang, J. Q., de Jong, M., Hau, M., Visser, M. E., van Grunsven, R. H., and Spoelstra, K. (2015). Stressful colours: corticosterone concentrations in a free-living songbird vary with the spectral composition of experimental illumination. Biol. Lett. 11:20150517. doi: 10.1098/rsbl.2015.0517
Poot, H., Ens, B. J., de Vries, H., Donners, M. A., Wernand, M. R., and Marquenie, J. M. (2008). Green light for nocturnally migrating birds. Ecol. Soc. 13:47.
R Core Team (2020). R: A Language And Environment For Statistical Computing. R Foundation for Statistical Computing. Vienna, Austria.
Raap, T., Casasole, G., Costantini, D., AbdElgawad, H., Asard, H., Pinxten, R., et al. (2016a). Artificial light at night affects body mass but not oxidative status in free-living nestling songbirds: an experimental study. Sci. Rep. 6, 1–8.
Raap, T., Casasole, G., Pinxten, R., and Eens, M. (2016b). Early life exposure to artificial light at night affects the physiological condition: an experimental study on the ecophysiology of free-living nestling songbirds. Environ. Pollut. 218, 909–914. doi: 10.1016/j.envpol.2016.08.024
Raap, T., Pinxten, R., and Eens, M. (2016c). Artificial light at night disrupts sleep in female great tits (Parus major) during the nestling period, and is followed by a sleep rebound. Environ. Pollut. 215, 125–134. doi: 10.1016/j.envpol.2016.04.100
Raap, T., Pinxten, R., and Eens, M. (2015). Light pollution disrupts sleep in free-living animals. Sci. Rep. 5, 1–8.
Rappole, J. H., and Tipton, A. R. (1991). New harness design for attachment of radio transmitters to small passerines (Nuevo Diseño de Arnés para Atar Transmisores a Passeriformes Pequeños). J. Field Ornithol. 62, 335–337.
Rich, C., and Longcore, T. (2005). Ecological Consequences of Artificial Night Lighting. Washington, DC: Island Press.
Riley, W. D., Davison, P. I., Maxwell, D. L., and Bendall, B. (2013). Street lighting delays and disrupts the dispersal of Atlantic salmon (Salmo salar) fry. Biol. Conserv. 158, 140–146. doi: 10.1016/j.biocon.2012.09.022
Russ, A., Reitemeier, S., Weissmann, A., Gottschalk, J., Einspanier, A., and Klenke, R. (2015). Seasonal and urban effects on the endocrinology of a wild passerine. Ecol. Evol. 5, 5698–5710. doi: 10.1002/ece3.1820
Senner, N. R., Hochachka, W. M., Fox, J. W., and Afanasyev, V. (2014). An exception to the rule: carry-over effects do not accumulate in a long-distance migratory bird. PLoS One 9:e86588. doi: 10.1371/journal.pone.0086588
Smith, R. A., Gagné, M., and Fraser, K. C. (2021). Pre-migration artificial light at night advances the spring migration timing of a trans-hemispheric migratory songbird. Environ. Pollut. 269:116136. doi: 10.1016/j.envpol.2020.116136
Streby, H. M., McAllister, T. L., Peterson, S. M., Kramer, G. R., Lehman, J. A., and Andersen, D. E. (2015). Minimizing marker mass and handling time when attaching radio-transmitters and geolocators to small songbirds. Condor 117, 249–255. doi: 10.1650/condor-14-182.1
Stutchbury, B. J., Gow, E. A., Done, T., MacPherson, M., Fox, J. W., and Afanasyev, V. (2011). Effects of post-breeding moult and energetic condition on timing of songbird migration into the tropics. Proc. R. Soc. B Biol. Sci. 278, 131–137. doi: 10.1098/rspb.2010.1220
Taylor, P., Crewe, T., Mackenzie, S., Lepage, D., Aubry, Y., Crysler, Z., et al. (2017). The motus wildlife tracking system: a collaborative research network to enhance the understanding of wildlife movement. Avian Conserv. Ecol. 18:8.
Titulaer, M., Spoelstra, K., Lange, C. Y. M. J. G., and Visser, M. E. (2012). Activity patterns during food provisioning are affected by artificial light in free living great tits (Parus Major). PLoS One 7, 5–8. doi: 10.1371/journal.pone.0037377
Visser, M. E., and Gienapp, P. (2019). Evolutionary and demographic consequences of phenological mismatches. Nat. Ecol. Evol. 3, 879–885. doi: 10.1038/s41559-019-0880-8
Wagner, R. H., Schug, M. D., and Morton, E. S. (1996). Condition-dependent control of paternity by female purple martins: implications for coloniality. Behav. Ecol. Sociobiol. 38, 379–389. doi: 10.1007/s002650050255
Keywords: light pollution, artificial light at night, phenotypic plasticity, post-breeding movements, migration timing, ontogenetic effect
Citation: Bani Assadi S and Fraser KC (2021) The Influence of Different Light Wavelengths of Anthropogenic Light at Night on Nestling Development and the Timing of Post-fledge Movements in a Migratory Songbird. Front. Ecol. Evol. 9:735112. doi: 10.3389/fevo.2021.735112
Received: 02 July 2021; Accepted: 01 October 2021;
Published: 21 October 2021.
Edited by:
Alejandro Ariel Rios-Chelen, Universidad Autónoma de Tlaxcala, MexicoReviewed by:
Alfredo G. Nicieza, University of Oviedo, SpainPierre J. Deviche, Arizona State University, United States
Copyright © 2021 Bani Assadi and Fraser. This is an open-access article distributed under the terms of the Creative Commons Attribution License (CC BY). The use, distribution or reproduction in other forums is permitted, provided the original author(s) and the copyright owner(s) are credited and that the original publication in this journal is cited, in accordance with accepted academic practice. No use, distribution or reproduction is permitted which does not comply with these terms.
*Correspondence: Saeedeh Bani Assadi, cy5iYW5pYXNzYWRpQGdtYWlsLmNvbQ==