- 1Key Laboratory of Humid Subtropical Eco-Geographical Process, Ministry of Education, College of Geographical Sciences, Fujian Normal University, Fuzhou, China
- 2Swiss Federal Research Institute WSL, Birmensdorf, Switzerland
- 3College of Life Sciences, Anhui Normal University, Wuhu, China
- 4Key Laboratory of Alpine Ecology, Institute of Tibetan Plateau Research, Chinese Academy of Sciences, Beijing, China
Monitoring cambial activity is important for a better understanding of the mechanisms governing xylem growth responses to climate change, providing a scientific basis for tree-ring-based climate reconstructions and projections about tree growth under future climate scenarios. It plays an even more important role in investigating evergreen tree growth in regions with less distinct seasonal cycles. Subtropical evergreen forests have been studied in recent years for their sensitivity to climate change, but it remains unclear how xylem growth is driven by subtropical climates. To further understand the climate-growth response strategies of subtropical conifers, we micro-cored Cryptomeria fortunei and Cunninghamia lanceolata weekly in 2016 and 2017 at the humid subtropical Gushan Mountain in southeastern China. Our weekly growth monitoring showed that the vegetation periods of these two species were both approximately 2–3 months longer than trees in temperate and boreal forests. The growth of C. fortunei in 2016 and 2017 and C. lanceolata in 2017 showed a bimodal pattern of xylogenesis, which was induced by summer drought. The results also indicated that the earlier end of the xylem formation was related to the yearly drought stress. These findings provide more specific information about tree growth and evidence of how climate influences wood production at the cellular level in subtropical regions.
Introduction
A high portion of the carbon in a forest ecosystem is deposited in the xylem, and its dynamics play a crucial role in the forest carbon cycle (Pan et al., 2011; Cuny et al., 2015). Consequently, a better understanding of xylem growth in various ecosystems, which can be characterized by its onset, growth rate, and anatomical features, has helped improve predictions of forest responses to climate change (De Luis et al., 2011; Deslauriers et al., 2017; Ren et al., 2019).
The process of xylem growth, that is, xylogenesis, describes the phenological phases of various cellular development processes involved in wood formation, as well as the factors driving growth rates (Deslauriers et al., 2017). The key parameters of xylogenesis include cell division, cell enlargement, cell wall thickening, lignification, and programmed cell death (Gričar et al., 2015; Rossi et al., 2016; Deslauriers et al., 2017). Previous studies on cambial phenology (both observations and controlled experiments) in temperate and boreal forests have demonstrated the crucial role of temperature in the onset of cambial growth (Rossi et al., 2008, 2016; Begum et al., 2013; Bryukhanova et al., 2013; Chen et al., 2019). In the Mediterranean forests, the onset of xylogenesis is mainly determined by temperature (Gričar et al., 2006; Camarero et al., 2010; Vieira et al., 2014). Moreover, cambial activity may have a bimodal pattern, with a low growth rate in the summer due to heat and drought stress (Balducci et al., 2013; Garcia-Forner et al., 2019). In semiarid alpine forests, low spring precipitation combined with low temperatures delays the onset of the growing season (Ren et al., 2015; Amoroso et al., 2017). The mechanism of xylogenesis cessation is still relatively unexplored (Dox et al., 2020; Marchand et al., 2020). In boreal forests, low temperature or a short photoperiod plays an important role at the end of xylogenesis (Hamilton et al., 2016; Guada et al., 2020). In contrast, the end of xylogenesis in the dry or semidry regions is more related to drought stress (Delzon et al., 2015; Ren et al., 2015; Novak et al., 2016; Zhang et al., 2018; Fonti et al., 2020).
Among the various ecosystems investigated in most previous studies, subtropical forests are underrepresented. However, it has been suggested that subtropical forests are sensitive to climate change (Zhou et al., 2013), and could suffer more frequent or severe heat and drought stresses in the future (Stocker et al., 2013; Liu Q. et al., 2019). The phenology of xylem growth and how it is affected by climatic drivers remain uncertain in subtropical forests (Dhirendra Singh and Venugopal, 2011; Singh and Venugopal, 2011; Pumijumnong and Buajan, 2012; Huang et al., 2018; Rahman et al., 2019; Zhang et al., 2019). Indeed, the xylem growth of subtropical forests exhibits different dynamic patterns as observed in a limited number of existing studies. For example, Yan et al. (2013) revealed a long growing season, which spanned almost a whole year in the subtropics, while Huang et al. (2018) observed an inactive semidormancy period in January. There are also subtropical species (e.g., Cunninghamia lanceolata), which show bimodal growth due to seasonal drought as visible in stem radial variation (Huang et al., 2019).
Additionally, most current studies in the subtropics have only explored the intra-annual variability of tree growth (Huang et al., 2018; Liu et al., 2018), but comparative studies on the interannual differences in intra-annual wood formation are limited (Zhang et al., 2019). Based on the few climate conditions investigated in the existing studies, only limited generalizations could be made regarding xylem growth in subtropical forests and their responses to environmental changes. Therefore, high-resolution monitoring of cambial phenology is needed, especially with more climatic conditions included. This will improve our understanding of xylem growth dynamics in subtropical forests and subsequently help reduce the uncertainty in predicting growth trends in the context of climate change (Huang et al., 2018).
Cryptomeria fortunei and Cunninghamia lanceolata are fast-growing conifer species inhabiting subtropical China and are widely planted because of their high-economic value. In addition, these two species provide important ecological benefits, including carbon sequestration, soil erosion conservation, and diminishing atmospheric pollutants (Tian et al., 2011; Zhao et al., 2017). We aimed to compare the onset and end of xylogenesis as well as the growth rate between C. fortunei and C. lanceolata in relation to climate variables during the two sampling years. We hypothesized that (i) summer drought triggers bimodal growth, and (ii) drought stress induces the earlier end of xylem formation. The results of the present study provide more specific information about subtropical tree growth and could help improve the modeling strategy for the carbon cycle in subtropical forest ecosystems.
Materials and Methods
Study Site
This study was conducted in a subtropical evergreen forest on the north-facing slopes of the Gushan Mountains at an elevation of 450 m a.s.l. (26.05°N, 119.38°E) (Figure 1). The forest canopy is dense, and the overstory is dominated by conifer species (C. lanceolata, Pinus massoniana, and C. fortunei) mixed with a small number of Castanopsis carlesii and Schima superba. The soil is classified as Humic Acrisols with high moisture content. The climate is subtropical, with an annual mean temperature of 19.9°C and total annual precipitation of 1,366 mm (according to the records of the near Fuzhou meteorological station from 1954 to 2017). The Western Pacific Subtropical High and the Spring Persistent Rains strongly affect the region, and it usually has wet springs and dry summers. Spring precipitation (April to June) accounts for 40.6% of the annual precipitation, while the typhoon season (from July to September) accounts for 31.8% of annual precipitation, but these percentages have high inter-annual variability. July is usually the driest month with an average precipitation of 137 mm and the highest average temperature of 28.72°C. Extreme high-temperature events most often occurred in July and August (Supplementary Figure 1A).
To assess weather conditions during 2016 and 2017, daily meteorological data, including daily mean temperature (°C), total precipitation (mm), and sunshine duration (h), were obtained from the Fuzhou weather station (Figure 1). There was a comparable mean air temperature of 23.8 and 23.9°C during March to November in 2016 and 2017. However, the mean air temperature from March to June in 2016 was 0.7°C higher than in 2017 (21.4 vs. 20.7°C), and the mean air temperature from July to November was 0.7°C higher in 2017 (Figure 2A and Table 1). From March to November, the sum of precipitation was 1,969.9 mm in 2016 and 1,282.3 mm in 2017. However, the total precipitation from March to June in 2016 was 256.8 mm lower than it was in 2017 (659.9 vs. 916.7 mm). Few precipitation events were observed from July to November in 2017, and the mean precipitation during September to November was 41.5 mm, indicating a serious drought (Figure 2B and Table 1). The lower precipitation from July to November 2017 was consistent with the higher vapor-pressure deficit (VPD) (Figures 2C,D and Table 1).
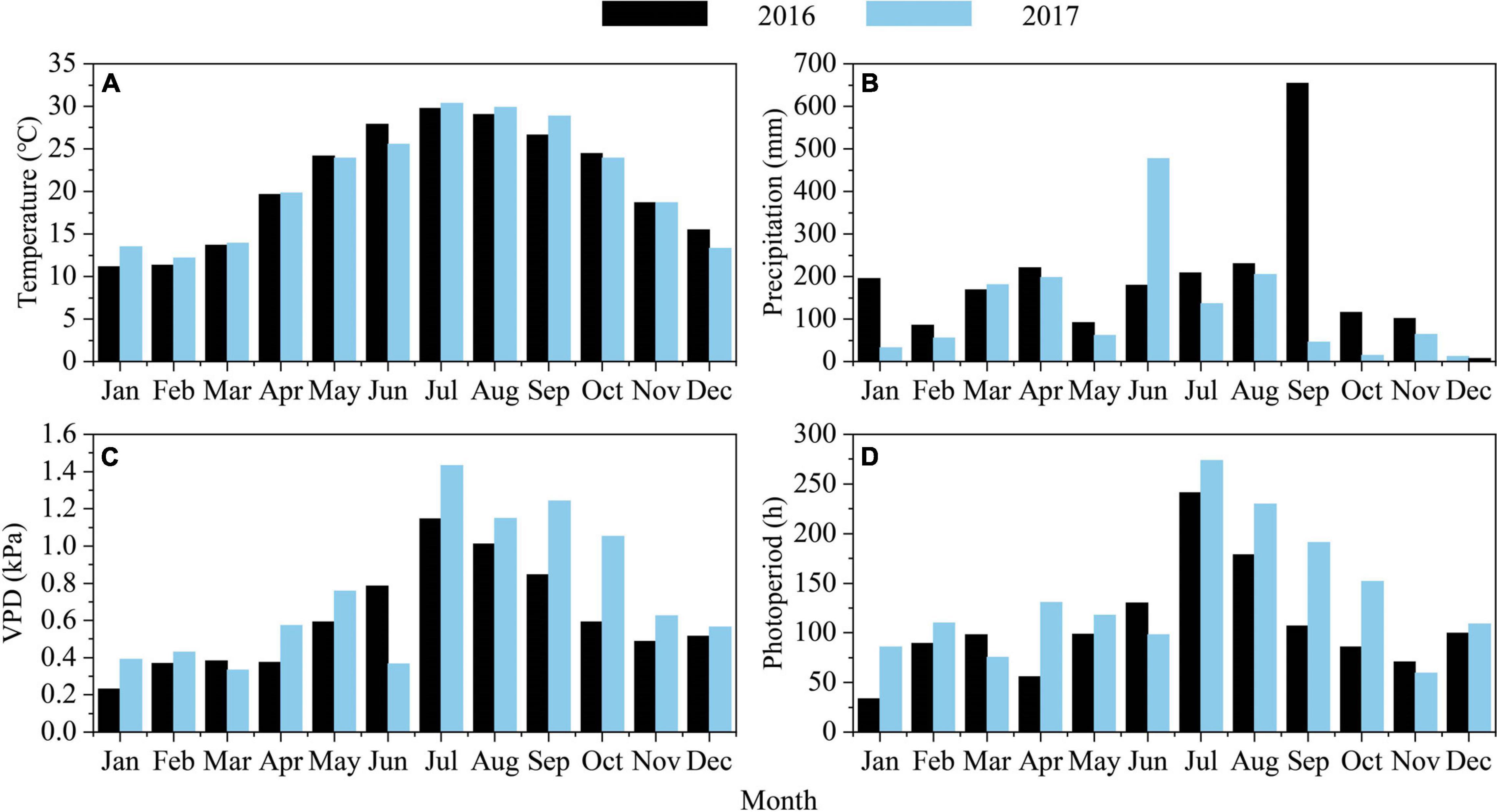
Figure 2. (A) Mean air temperature and (B) precipitation and (C) vapor pressure deficit (VPD) and (D) sunshine duration during 2016 and 2017 in Fuzhou city (10 km away from the site location).
Sample Collection and Preparation
We selected Cryptomeria fortunei and Cunninghamia lanceolata in a subtropical evergreen forest in southeastern China for observation and collected microcore samples for two consecutive years to investigate the intra-annual variability of the xylem growth of these two species. Microcores were collected weekly from C. fortunei and C. lanceolata trees from March to November 2016 and from February 2017 to January 2018. Three healthy and mature trees of each species were randomly selected and micro-cored at breast height (1.3 m) using a Trephor tool (Rossi et al., 2006). The monitored C. fortunei and C. lanceolata trees had an average diameter at breast height of 32.8 ± 1.4 cm and 23.7 ± 1.9 cm, a height of 10.9 ± 1 m and 13.1 ± 0.9 m, and an age of 36 ± 1 years and 24 ± 1 years, respectively. Micro-cores were sampled at least 5 cm apart to avoid wound reactions from nearby sampling points. The cores were 2 mm in diameter and 20–30 mm in length, containing two to five annual rings and a cambium zone with adjacent phloem (Rossi et al., 2006). The microcores were stored in formalin-ethanol-acetic acid solution (mixing ratio: 5:90:5) immediately after sampling in the field (Zhang et al., 2013). In the laboratory, the microcores were dehydrated in an ethanol gradient (70, 90, 95, and 100%), cleaned in a clearing solvent of D-limonene, and embedded in paraffin. Transverse sections (9–12 μm thick) were cut with a rotary microtome (YD315, Jinhua YIDI Medical Appliance Co., Ltd., China) and stained with safranin (3 in 95% ethanol) and Astra blue (0.5 in 95% ethanol).
Microscopic Observations
To assess the dynamics of annual xylem ring formation, we counted the number of tracheids during the stages of enlargement, wall thickening, and maturation on each collected microsection along with three representative radial files (Rossi et al., 2006; Gärtner et al., 2015; Deslauriers et al., 2017). We identified (i) cells in the phase of enlargement when the cell was at least two times larger than the cambial cells, (ii) cells in the phase of wall thickening when the birefringence of the secondary walls appeared under polarized light (Abe et al., 1997), and (iii) cells in the phase of matured when the tracheid walls were lignified and cell protoplasts disappeared (Rossi et al., 2006; Deslauriers et al., 2017) (Supplementary Figure 2). The onset of xylem formation in spring was defined when at least one cell entered the enlargement phase, while the end of the season in autumn was determined when there were no more wall thickening tracheids (Rossi et al., 2006).
Data Analysis
Cambial phenology was computed for days of the year (DOY), corresponding to dates of (i) the onset and (ii) the end of cell enlargement, (iii) the onset, and (iv) the end of wall thickening. Micro-cores were taken from different positions within the tree circumference during the growing season (Rossi et al., 2003). Circumferential variability in cambial activities, including ring width, cell number, cell diameter, and wall thickness, exist at different positions of the stem (Creber and Chaloner, 1984). Thus, standardization of the cell number was required (Oberhuber and Gruber, 2010):
In Equation 1, where nci is the standardized number of xylem cells, ncmi is the measured number; of xylem cells, rwm is the mean ring width of previous rings for all samples, and rws is the ring width of previous rings for each sample. We conducted ANOVA and Tukey’s tests for the timing of the onset of each xylogenesis phase between years and used the results to assess the dynamics of intra-annual xylem ring formation (Queen et al., 2002).
We then fitted the total number of xylem cells (enlargement + wall thickening + mature cells) of each tree and year over the whole growing season with the Generalized Additive Model (GAM). Subsequently, we averaged at the tree and year level to indicate the intra-annual variation in the progress of wood production (Cuny et al., 2013).
In Equation 2, where y is the total number of xylem cells, x1 is the day of the year (DOY), and f represents the smoothing function. The Poisson link function was used, given that the response variable is a count variable. To fit GAMs, the mgcv package was used within the R statistical software environment (R Core Team, 2013). The non-negative value of the derivative of GAM was used to obtain the rate of cell division (Huang et al., 2018; Yu et al., 2019).
We used Pearson coefficients to evaluate the relationship between growth rate, cambium cells, enlargement cells, and climatic variables. The number of cambium and enlargement cells was chosen during the onset and the end of enlargement, while the growth rate was calculated as the mean value between successive sampling dates. Three climatic variables were included: daily mean temperature, precipitation, and VPD. All factors were calculated as averaged values, except for precipitation for which we used summed values between successive sampling dates (7 days). For C. fortunei, the sample sizes of cambium cells and enlargement cells were 87 and 76 in 2016 and 2017, respectively. For C. lanceolata, the sample sizes of cambium cells and enlargement cells were 58 and 67 in 2016 and 2017, respectively. We also used Pearson coefficients to evaluate the relationship between the duration of wood formation and climatic variables (annual mean temperatures, precipitation, and VPD).
Results
Cambial Phenology
In general, Cryptomeria fortunei had a higher growth rate and longer growth duration than Cunninghamia lanceolata (Table 2). For C. fortunei, the onset of xylogenesis occurred on 8 April ± 1.7 days (mean ± SEs; DOY, 99.3 ± 1.7) and 4 April ± 6.2 days (DOY, 94.3 ± 6.2) in 2016 and 2017, respectively. The cambial cells reached the peak of 4–6 cells soon after the onset and then gradually decreased until they were stable within the range of 2–4 cells. Cell wall thickening started on April 15 ± 5 days (DOY, 106 ± 5) in 2016 and April 13 ± 4.7 days (DOY, 103.3 ± 4.7) in 2017. Growth ended on November 13 (DOY, 318) in 2016 and October 26 ± 8.4 days (DOY, 299.7 ± 8.4) in 2017. The duration of xylogenesis for C. fortunei was thus 218.7 ± 1.7 days in 2016 and 205.3 ± 12.3 days in 2017, which was about 13 days shorter than in the previous year (Figures 3A–D and Table 2).
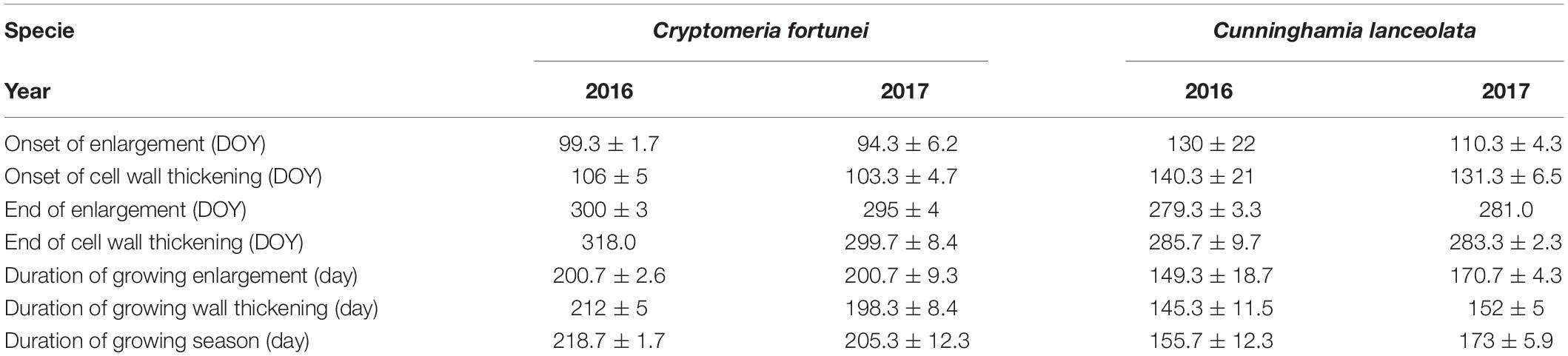
Table 2. Characterization of cambial phenology and seasonal growth patterns (Mean ± 1 SD) of Cryptomeria fortunei and Cunninghamia lanceolata during 2016–2017 in the Gushan Mountain.
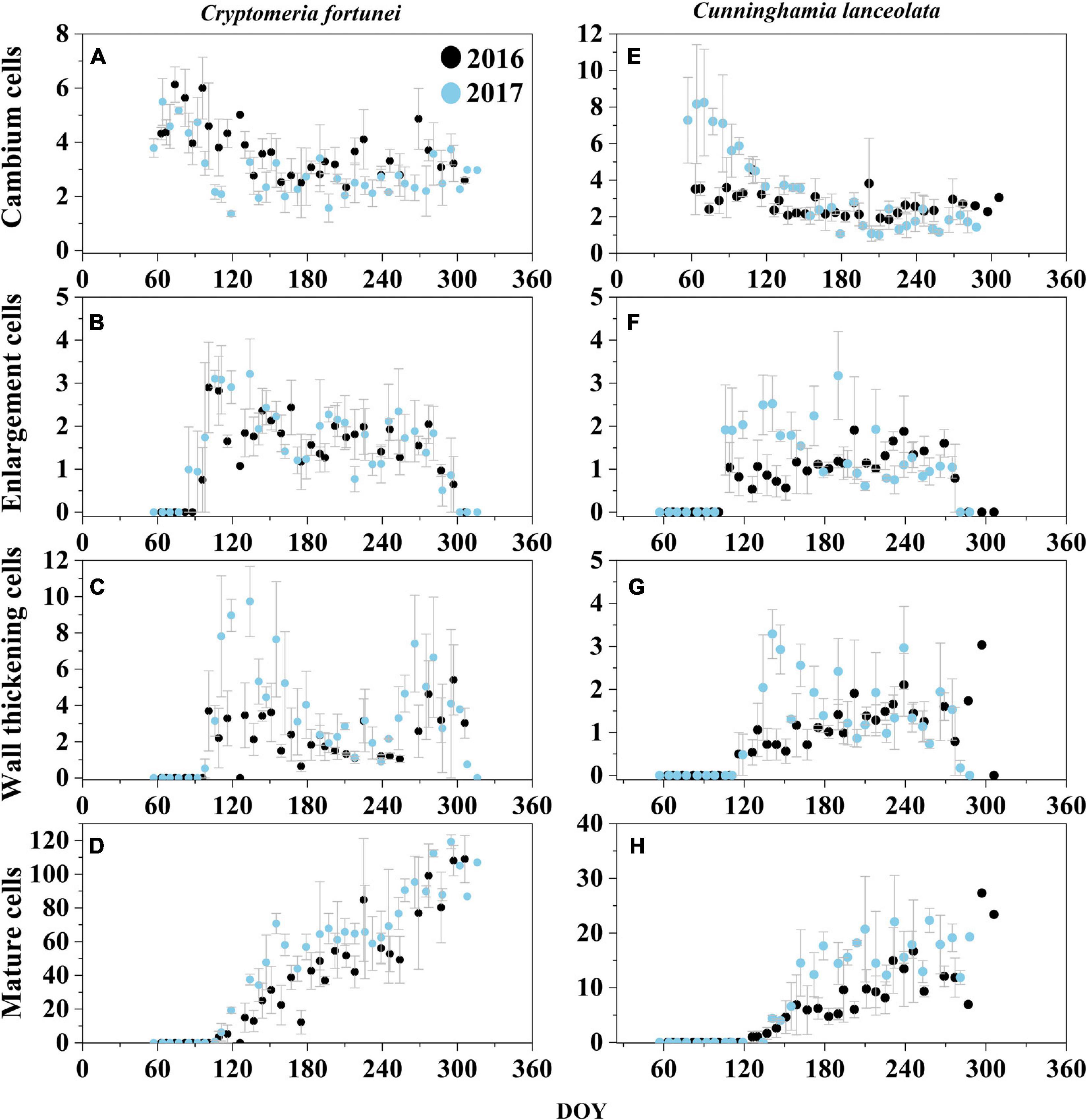
Figure 3. Number of cambial, enlarging, wall thickening, and mature cells of Cryptomeria fortunei (A–D) and Cunninghamia lanceolata (E,F) as observed during 2016 and 2017 at the Gushan Mountain. The dots and the bars represent mean cell number and standard errors among the three selected trees, respectively.
For C. lanceolata, the growing season started later, initiating on May 9 ± 22 days [DOY, 130 ± 22 in 2016 and on April 20 ± 4.3 days (DOY, 110.3 ± 4.3)] in 2017. Cambium cells fluctuated within the same range of 2–4 in 2016, whereas, in 2017, they reached a maximum of seven cells in April and then gradually decreased to 2–3 cells. Cell enlargement showed similar trends for both species during 2016 and 2017, showing 1–3 cell layers. Wall thickening started on May 19 ± 21 days (DOY, 140.3 ± 21) in 2016 and May 11 ± 6.5 days (DOY, 131.3 ± 6.5) in 2017. The cambium started its dormancy on 11 November ± 9.7 days (DOY, 285.7 ± 9.7) and 10 October ± 21 days (DOY, 283.3 ± 2.3), in 2016 and 2017, respectively. For C. lanceolata, the duration of xylogenesis was 155.7 ± 12.3 in 2016 and 173 ± 5.9 days in 2017 (Figures 3E–H and Table 2).
The cell wall thickening showed a bimodal pattern, with two peaks of cell activity in spring (April–June) and late summer to autumn (September–November) in both species across the study period except C. lanceolata in 2016 (Table 2 and Figures 3B,C). The duration of xylogenesis was significantly (p < 0.01) different between species, but not between years (Supplementary Table 1). In 2016, C. fortunei and C. lanceolata showed a relatively higher growth rate and increased cell growth in summer. In 2017, both species showed a bimodal pattern of the growth rate, with a relatively high growth rate in spring and a relatively low growth rate in summer (Figure 4).
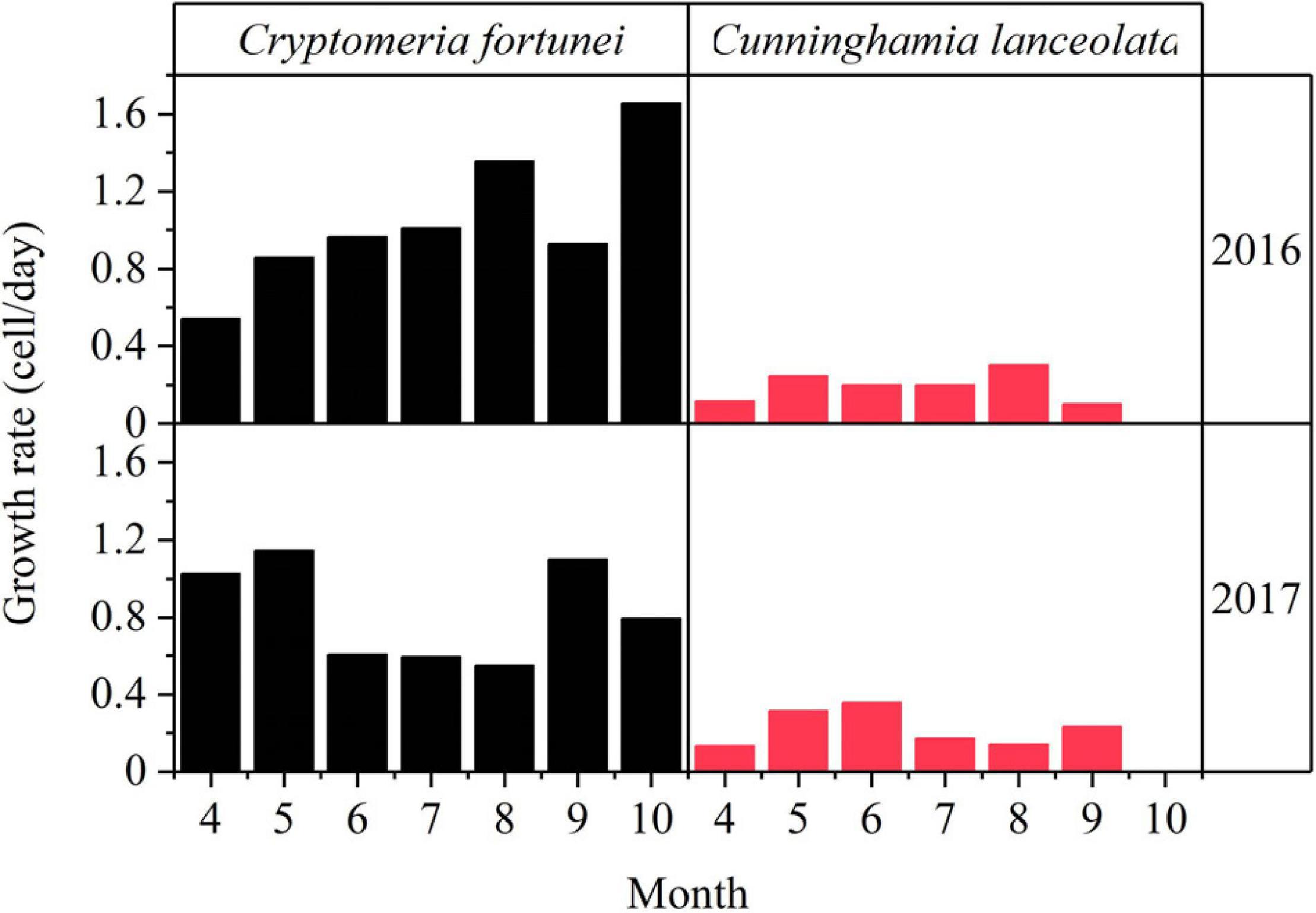
Figure 4. Monthly growth rates of Cryptomeria fortunei (left) and Cunninghamia lanceolata (right) as observed during the growing season of 2016–2017.
Wood Formation and Climate
Both Cryptomeria fortunei and Cunninghamia lanceolata have obvious inter-annual differences in the relationship between intra-annual growth and climate. Due to the large sample sizes, the correlations are significant, although their values are not that high. For C. fortunei, temperature and VPD showed significant negative impacts on the cambium and the enlargement of cells in 2016, and precipitation showed significant positive impacts on the cambium cells. In 2017, precipitation and VPD had no significant effect on the phase of xylogenesis, while temperature had significant negative effects on the enlargement of cells (Figure 5). For C. lanceolata, temperature and VPD showed significant negative impacts on cambium cells in 2016, and there was no significant correlation between the enlargement of cells and climatic factors. In 2017, temperature and VPD had significant negative impacts on the cambium and the enlargement of cells. Precipitation had no significant impact on the cambium and cell enlargement in C. lanceolata from 2016 to 2017 (Figure 6). There was no significant correlation between climate factors, growth rate, and duration of xylogenesis in the two species in 2016–2017 (Supplementary Figures 3, 4).
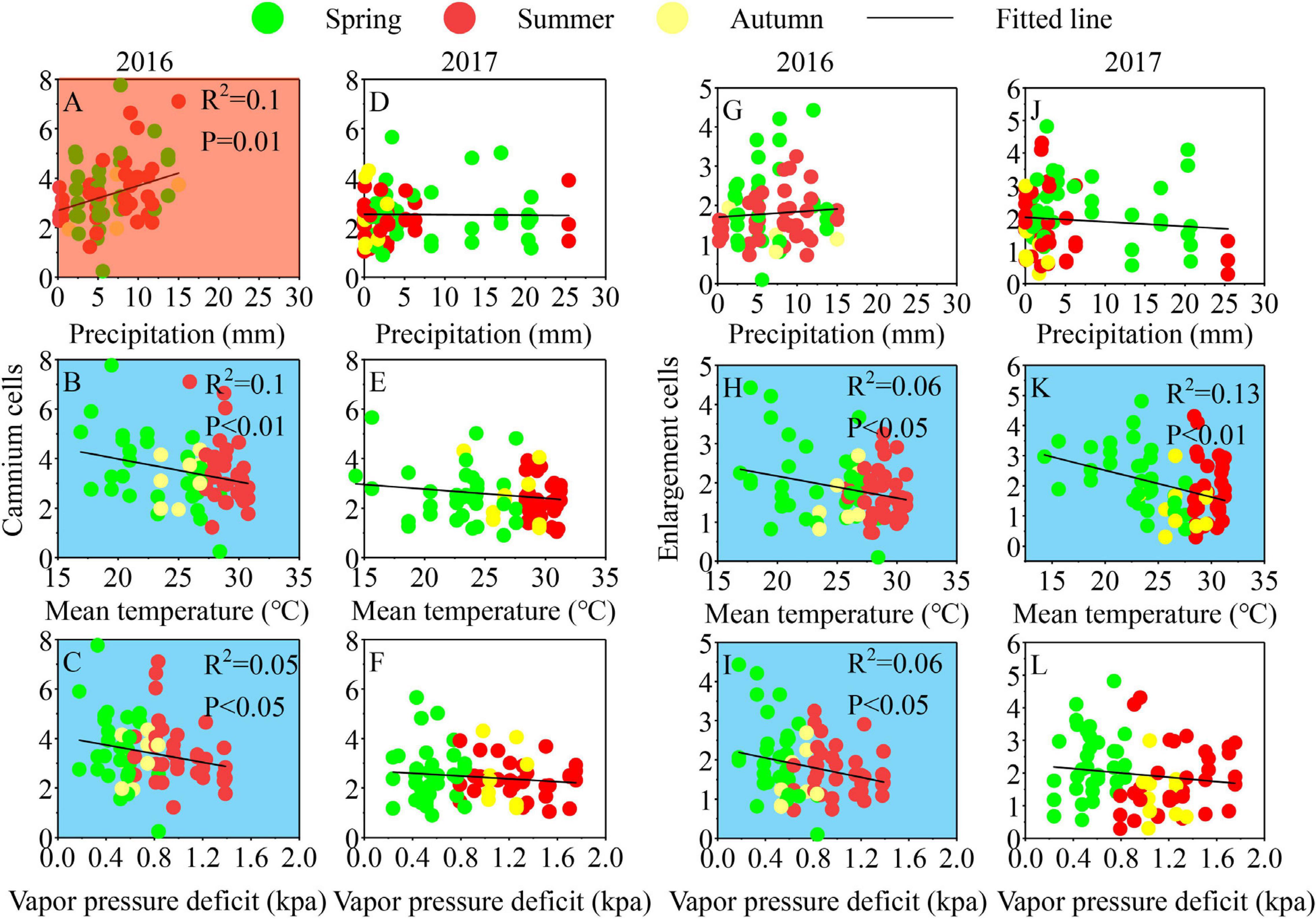
Figure 5. Relationships between climate variables and the number of cells in cambium (A–F) and enlargement (G–L) of Cryptomeria fortunei during 2016–2017. Significant correlations (p < 0.05) were shaded red (positive) and blue (negative).
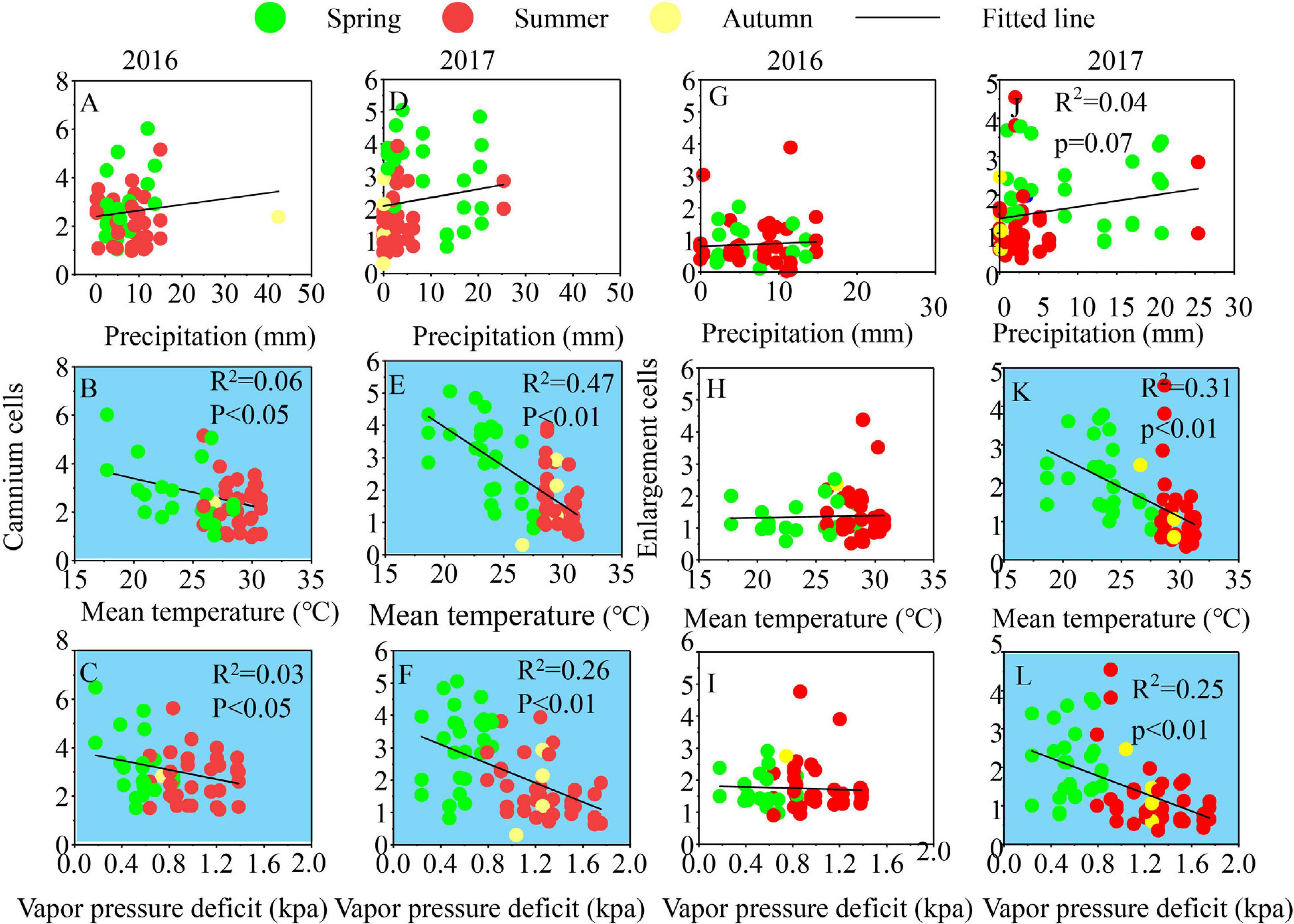
Figure 6. Relationships between climate variables and the number of cells in cambium (A–F) and enlargement (G–L) of Cunninghamia lanceolata 2016–2017. Significant correlations (p < 0.05) were shaded red (positive) and blue (negative).
Discussion
Climatic Control on the Onset and Termination of Cambial Activity
The two species (C. fortunei and C. lanceolata) included in this study started growing between March and April, while temperate and boreal conifer species started in April-May (Cuny et al., 2015; Rossi et al., 2016; Chen et al., 2019). Mediterranean species started between late March and early May (Camarero et al., 2010; Palombo et al., 2018; Antonucci et al., 2019). C. fortunei and C. lanceolata stopped growing from October to November (Camarero et al., 2010; Vieira et al., 2014; Antonucci et al., 2019). In comparison, temperate boreal species (Cuny et al., 2015; Rossi et al., 2016) stopped from September to October. Some Mediterranean conifers stopped in September to mid-November, while some species continued with cell differentiation during the winter (Camarero et al., 2010; Vieira et al., 2014; Prislan et al., 2016; Balzano et al., 2018; Antonucci et al., 2019). Thus, the duration of the growing season of our studied species was approximately 2–3 months longer than that in the temperate and boreal zones, but shorter than that in the Mediterranean zones. Compared to other research in subtropical regions, the later onset and the earlier termination of xylem formation in this study lead to a shorter growing season (Huang et al., 2018), which may be caused by the geographic location and local climate differences.
The onset of cambium activity of the two species in 2017 occurred earlier than in 2016, but this difference was not significant. The temperature has long been recognized as the major trigger for growth initiation in coniferous and broadleaf trees. This is true across temperate and boreal forests, with the physiological threshold daily minimum temperature being more than 4–5°C (Rossi et al., 2008; Li et al., 2016). Higher temperatures in early spring can induce earlier reactivation and differentiation of secondary xylem (Begum et al., 2009, 2017). For example, the higher temperatures in the spring of 2016 compared with 2015 induced an earlier onset cambium of Quercus variabilis on Qinling Mountain (China) (Zhang et al., 2019). Moreover, monitoring results of Taiwan pines at different altitudes in the Lushan Mountains show that trees at low altitude sites will start activity earlier than those grown at high altitudes due to higher air temperatures (Liu X. et al., 2019). In this study, mean air temperatures during the 7 days before the onset of cambium activity of C. fortunei were 18.9°C in 2016 and 16.7°C in 2017. Temperatures for C. lanceolata in the week before the onset of cambium activity were 21.3°C in 2016 and 19.5°C in 2017 (Table 3). However, the increase in temperature in 2016 did not induce the earlier onset of cambium activity. Similar results have been found in other studies in the subtropics (Liu X. et al., 2019; Zhang et al., 2019). Consequently, our results indicate that temperature may not be the only factor influencing wood formation in C. fortunei and C. lanceolata.
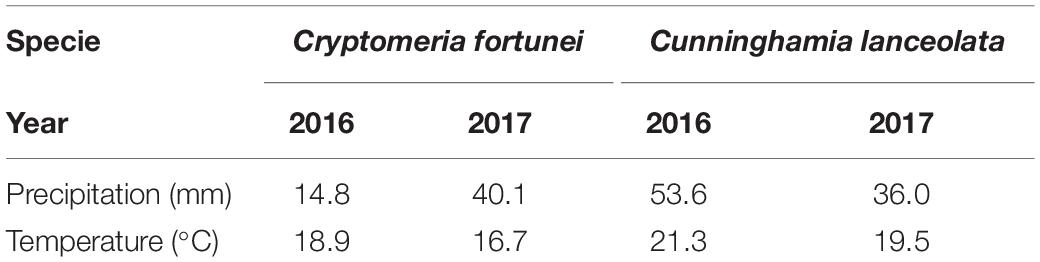
Table 3. Mean air temperatures and precipitation sums during the week prior to the onset of wood formation of Cryptomeria fortunei and Cunninghamia lanceolata in 2016 and 2017.
Trees need water to generate turgor pressure during cell expansion, so precipitation could also drive the onset of cambium activity, and the effect may depend on the geographic location and local climate (Trouet et al., 2012). Previous research has found that, in arid and cold regions, precipitation is a trigger for the onset of xylogenesis in Juniperus przewalskii (Ren et al., 2015, 2018; Zhang et al., 2018). In the present study, total precipitation during the 7 days before the onset of cambium activity in C. fortunei was 14.8 mm in 2016 and 40.1 mm in 2017. In the case of C. lanceolata, the total precipitation measured was 53.6 mm in 2016 and 36 mm in 2017 (Table 3), as cambium activity was not delayed in 2017 despite the lower precipitation before cambium cell division of C. lanceolata. This is consistent with previous studies showing that precipitation has a weak influence on xylem growth resumption for Quercus variabilis in the subtropical region (Zhang et al., 2019). Indeed, the influence of precipitation on the onset of xylogenesis in hot and moist regions remains uncertain. For instance, in tropical regions, the seasonal variability of precipitation regulates cambial activity. Active cambium in several hardwood species has been associated with a rainy season (high rainfall), and dormancy is associated with the dry season (low rainfall) (Rahman et al., 2019). However, Trouet et al. (2012) revealed that the beginning and the end of cambial growth of Brachystegia spiciformis did not coincide with the beginning and the end of the rainy season. It is worth noting that, although both tree species in our study started to onset earlier in 2017, considering the ideal and similar spring hydrothermal conditions in 2016–2017, it is difficult to determine which climatic factors play an important role in initiating cambium activity.
In 2017, the study area experienced severe consecutive droughts in late summer and autumn, and the average monthly precipitation from September to November was only 41.5 mm. Both C. fortunei and C. lanceolata ended wood production earlier in 2017. Worbes (1995) stated that an annual dry season of 2–3-month long and monthly precipitation of less than 60 mm would lead to cambial dormancy in tropical regions, which is consistent with our results. Underwater stress conditions, the extracellular water potential pressure suddenly drops even lower than the osmotic pressure of the enlarged cells. This causes the loss of turgor pressure, and the cell activity in the cambium area is weakened, thereby inhibiting the division of new cells (Abe et al., 2003). During periods of drought, cell wall synthesis and protein synthesis are substantially affected (Choat et al., 2012; Bader et al., 2013). Additionally, irrigation and natural experiments on mature trees found that the unirrigated trees/xericsite finished wood formation earlier than the irrigated trees/dry-mesic site (Gruber et al., 2010; Eilmann et al., 2011), confirming the critical role of water availability in stem growth cessation. Generally, drought-stressed termination of cambium activity has been observed in arid regions such as the Qilian Mountains in northwestern China (Zhang et al., 2021) and the Mediterranean region with summer drought stress (Bader et al., 2013; Garcia-Forner et al., 2019). In 2017, the earlier cessation of the cambium activities of C. fortunei and C. lanceolata indicates that, although in relatively humid subtropical regions, seasonal droughts may also limit tree growth.
Effects of Climate Factors on the Bimodal Pattern of Xylogenesis
Cryptomeria fortunei and Cunninghamia lanceolata showed different intra-annual growth dynamics between 2016 and 2017. We detected a bimodal pattern of xylogenesis and the growth rate for C. fortunei and C. lanceolata in the relatively drier year (2017). It is worth noting that this bimodal phenomenon in the research was more obvious when looking at the number of wall-thickening cells. The process of wall thickening depends mainly on the carbohydrates produced by photosynthesis (Cuny and Rathgeber, 2016). Such bimodal patterns of xylogenesis are in contrast to the studies in temperate and boreal forests where a bell-shaped annual pattern in cambium cell numbers was commonly found (Rossi et al., 2008); Ren et al., 2015; Chen et al., 2019). However, our finding is similar to the studies in the Mediterranean, where a bimodal pattern of cambium activity was reported as a result of distinct seasonal water availability (Camarero et al., 2010; Pacheco et al., 2016).
A warm and humid climate enhances photosynthetic ability, carbon fixation capacity (Kellomäki, 2001), and the activity of enzymes in relation to the photosynthesis of leaves. Consequently, photosynthetic capacity is improved, and the promotion of radial growth of the stem occurs (Strand et al., 2002). This can explain why C. fortunei and C. lanceolata could maintain a relatively high growth rate and cell increment during the summer of 2016, and a higher growth rate during spring in 2017 (Figure 4 and Supplementary Figure 5). In 2017, the high VPD accompanied by high temperatures in the study area from July to August is likely to aggravate the transpiration of the trees, which may lead to a decrease of stomatal conductance and limited photosynthesis (Mathur et al., 2014; Zhou et al., 2015; Ouyang et al., 2018; Grossiord et al., 2020; Song et al., 2020). These conditions may cause a decrease in carbohydrate availability and a decreased growth rate (Michelot et al., 2012; Zhang et al., 2021). Relevant studies based on tree rings and stable carbon isotope have also confirmed the negative effect of summer drought on tree growth on an interannual scale (Li et al., 2019; Bai et al., 2020; Dong et al., 2020).
We found that the percentage of net cell increment to the total xylem cells during 2016–2017 was consistent with the seasonal distribution of precipitation, and the final number of xylem cells was similar even though the precipitation showed such a remarkable difference. This result suggests that xylem growth in the subtropical zone can adjust its physiological activities to deal with environmental changes. Such plastic growth characteristics also offset the influence of climate to a certain extent.
Xylogenesis Difference Between Cryptomeria fortunei and Cunninghamia lanceolata
Marked differences were observed in the responses of xylogenesis to climatic factors between 2016 and 2017, especially in C. lanceolata. The negative effects of temperature (p < 0.01) and VPD (p < 0.01) on the enlargement stages of C. lanceolata were significant in 2017 but not in 2016. Moreover, the positive effect of precipitation on the enlargement stage of C. lanceolata was also enhanced. Both high VPD and low soil moisture are considered characteristics of drought (Liu et al., 2020). When the plant is in a high VPD situation, it increases the absorption of soil water by the root system to cope with the loss of water (Liu et al., 2020). Thus, we can infer that, in the relatively arid 2017, the xylogenesis process of C. lanceolata was affected by high temperature and drought. Lower drought tolerance caused by a smaller root system and less fine roots (Sun et al., 1990) may explain why C. lanceolata was more sensitive to moisture restrictions in 2017 than C. fortunei.
Wood production was determined by the duration of the growing season and the growth rate. The growth rate and duration of the growing season of C. fortunei are much higher than those of C. lanceolata, which explains the much higher number of xylem cells. Previous research found that the growth rate of C. lanceolata will decrease significantly after the mature stage, while C. fortunei can maintain a high growth rate even at the mature stage (Wu et al., 2001). A higher growth rate can increase the competitive ability, survival, and long-term success of a species, especially in severe environmental conditions (Silvertown, 2004; Grotkopp and Rejmánek, 2007). These observations confirmed that C. fortunei had a higher competitive advantage than C. lanceolata in mixed forests (Wu et al., 2001).
Conclusion
This study presented 2 years of weekly monitoring of xylem formation in two subtropical conifers (C. fortunei and C. lanceolata). The growing season in the humid subtropics was observed to last 2–3 months longer than in temperate and boreal forests. Observations also highlighted that drought was the dominant factor ruling the earlier end of xylem formation. Drought during summer induced bimodal xylogenesis in drier years. C. fortunei appeared to be less sensitive to drought and thus performed better than C. lanceolata. These findings provide evidence of how climate influences wood production at the cellular level in subtropical regions.
Data Availability Statement
The raw data supporting the conclusions of this article will be made available by the authors, without undue reservation.
Author Contributions
KF, ZZ, and FZ designed the project. ZZ, FZ, and ZD performed field-based microcores. ZZ performed lab-based experiments, data analysis, and drafted the manuscript. PF, PR, XL, and GM contributed to the final version. All authors reviewed the manuscript.
Funding
This study was funded by the Strategic Priority Research Program of the Chinese Academy of Sciences (XDB26020000), National Science Foundation of China (41822101, 41971022, 41888101, and 41772180), the State Administration of Foreign Experts Affairs of China (GS20190157002), fellowship for the National Youth Talent Support Program of China (Ten Thousand People Plan), the Natural Science Foundation Project of Fujian Province (2017J0101), and fellowship for Youth Talent Support Program of Fujian Province and the innovation team project (IRTL1705).
Conflict of Interest
The authors declare that the research was conducted in the absence of any commercial or financial relationships that could be construed as a potential conflict of interest.
Publisher’s Note
All claims expressed in this article are solely those of the authors and do not necessarily represent those of their affiliated organizations, or those of the publisher, the editors and the reviewers. Any product that may be evaluated in this article, or claim that may be made by its manufacturer, is not guaranteed or endorsed by the publisher.
Acknowledgments
We thank anonymous reviewers for their careful work and thoughtful suggestions that have helped improve this manuscript substantially.
Supplementary Material
The Supplementary Material for this article can be found online at: https://www.frontiersin.org/articles/10.3389/fevo.2021.733974/full#supplementary-material
Supplementary Figure 1 | Distribution of the monthly mean temperature (symbol line) and precipitation (bar) from 1953 to 2017 from the Fuzhou meteorological station (A). Long-term series of annual precipitation (B), temperature (C) and their trends (dashed lines) recorded by Fuzhou meteorological station for the period of 1953–2017.
Supplementary Figure 2 | Phases of cambial phenology in Cryptomeria fortunei (A–D) on DOY 119 (April 29) and Cunninghamia lanceolata (E,F) on DOY 141 (May 21) in 2017. Observed under a bright field (A,B,E) and polarized light (C,D,F), respectively. CZ, cambium zone; EC, enlarging cells; WT, wall thickenings; MC, mature cells.
Supplementary Figure 3 | Relationships between the growth rate and associated climatic factors. Significant correlations of p < 0.05 (∗), p < 0.01 (∗∗) are displayed.
Supplementary Figure 4 | Relationships between duration of xylogenesis and associated climatic factors. Significant correlations of p < 0.05 (∗), p < 0.01 (∗∗) are displayed.
Supplementary Figure 5 | Percentage of net cell increment to the total xylem cells in spring and summer of (A) Cryptomeria fortunei and (B) Cunninghamia lanceolata during the growing season of 2016–2017.
References
Abe, H., Funada, R., Ohtani, J., and Fukazawa, K. (1997). Changes in the arrangement of cellulose microfibrils associated with the cessation of cell expansion in tracheids. Trees 11, 328–332.
Abe, H., Nakai, T., Utsumi, Y., and Kagawa, A. (2003). Temporal water deficit and wood formation in Cryptomeria japonica. Tree Physiol. 23, 859–863.
Amoroso, M. M., Daniels, L. D., Baker, P. J., and Camarero, J. J. (2017). Dendroecology: Tree-ring Analyses Applied to Ecological Studies. Berlin: Springer.
Antonucci, S., Rossi, S., Lombardi, F., Marchetti, M., and Tognetti, R. (2019). Influence of climatic factors on silver fir xylogenesis along the Italian Peninsula. IAWA J. 40, 259–S253. doi: 10.1163/22941932-40190222
Bader, M. K. F., Leuzinger, S., Keel, S. G., Siegwolf, R. T., Hagedorn, F., Schleppi, P., et al. (2013). Central E uropean hardwood trees in a high-CO 2 future: synthesis of an 8-year forest canopy CO 2 enrichment project. J. Ecol. 101, 1509–1519.
Bai, M., Dong, Z., Chen, D., Zheng, H., Zhou, F., Cao, X., et al. (2020). Different responses of the radial growth of the planted and natural forests to climate change in humid subtropical China. Geografiska Annaler: Series A Phys. Geography 102, 235–246. doi: 10.1080/04353676.2020.1769364
Balducci, L., Deslauriers, A., Giovannelli, A., Rossi, S., and Rathgeber, C. B. (2013). Effects of temperature and water deficit on cambial activity and woody ring features in Picea mariana saplings. Tree Physiol. 33, 1006–1017. doi: 10.1093/treephys/tpt073
Balzano, A., Cufar, K., Battipaglia, G., Merela, M., Prislan, P., Aronne, G., et al. (2018). Xylogenesis reveals the genesis and ecological signal of IADFs in Pinus pinea L. and Arbutus unedo L. Ann. Bot. 121, 1231–1242. doi: 10.1093/aob/mcy008
Begum, S., Kudo, K., Rahman, M. H., Nakaba, S., Yamagishi, Y., Nabeshima, E., et al. (2017). Climate change and the regulation of wood formation in trees by temperature. Trees 32, 3–15.
Begum, S., Nakaba, S., Oribe, Y., Kubo, T., and Funada, R. (2009). Cambial sensitivity to rising temperatures by natural condition and artificial heating from late winter to early spring in the evergreen conifer Cryptomeria japonica. Trees 24, 43–52. doi: 10.1007/s00468-009-0377-371
Begum, S., Nakaba, S., Yamagishi, Y., Oribe, Y., and Funada, R. (2013). Regulation of cambial activity in relation to environmental conditions: understanding the role of temperature in wood formation of trees. Physiol. Plant. 147, 46–54. doi: 10.1111/j.1399-3054.2012.01663.x
Bryukhanova, M., Kirdyanov, A., Prokushkin, A., and Silkin, P. (2013). Specific features of xylogenesis in Dahurian larch, Larix gmelinii (Rupr.) Rupr., growing on permafrost soils in Middle Siberia. Russian J. Ecol. 44, 361–366.
Camarero, J. J., Olano, J. M., and Parras, A. (2010). Plastic bimodal xylogenesis in conifers from continental Mediterranean climates. New Phytol. 185, 471–480.
Chen, L., Rossi, S., Deslauriers, A., and Liu, J. (2019). Contrasting strategies of xylem formation between black spruce and balsam fir in Quebec. Canada. Tree Physiol. 39, 747–754.
Choat, B., Jansen, S., Brodribb, T. J., Cochard, H., Delzon, S., Bhaskar, R., et al. (2012). Global convergence in the vulnerability of forests to drought. Nature 491, 752–755. doi: 10.1038/nature11688
Creber, G., and Chaloner, W. G. (1984). Influence of environmental factors on the wood structure of living and fossil trees. Botanical Rev. 50, 357–448.
Cuny, H. E., and Rathgeber, C. B. (2016). Xylogenesis: coniferous trees of temperate forests are listening to the climate tale during the growing season but only remember the last words! Plant Physiol. 171, 306–317. doi: 10.1104/pp.16.00037
Cuny, H. E., Rathgeber, C. B., Frank, D., Fonti, P., Makinen, H., Prislan, P., et al. (2015). Woody biomass production lags stem-girth increase by over one month in coniferous forests. Nat. Plants 1:15160. doi: 10.1038/nplants.2015.160
Cuny, H. E., Rathgeber, C. B., Kiessé, T. S., Hartmann, F. P., Barbeito, I., and Fournier, M. (2013). Generalized additive models reveal the intrinsic complexity of wood formation dynamics. J. Exp. Bot. 64, 1983–1994.
De Luis, M., Novac, K., Raventós, J., Gricar, J., and Cufar, K. (2011). Monitoring seasonal dynamics of wood formation. Dendrochronologia 29, 123–125.
Delzon, S., Vieira, J., Campelo, F., Rossi, S., Carvalho, A., Freitas, H., et al. (2015). Adjustment capacity of maritime pine cambial activity in drought-prone environments. PLoS One 10:e0126223. doi: 10.1371/journal.pone.0126223
Deslauriers, A., Fonti, P., Rossi, S., Rathgeber, C. B. K., and Gričar, J. (2017). “Ecophysiology and plasticity of wood and phloem formation,” in Ecological Studies: Dendroecology: Tree-ring Analyses Applied to Ecological Studies, eds M. M. Amoroso, L. D. Daniels, P. J. Baker, and J. J. Camarero (Switzerland: Springer Nature), 13–33.
Dhirendra Singh, N., and Venugopal, N. (2011). Cambial activity and annual rhythm of xylem production of Pinus kesiya Royle ex. Gordon (Pinaceae) in relation to phenology and climatic factors growing in sub-tropical wet forest of North East India. Flora - Morphol. Distribut. Funct. Ecol. Plants 206, 198–204. doi: 10.1016/j.flora.2010.04.021
Dong, Z., Chen, D., Du, J., Yang, G., Bai, M., Zhou, F., et al. (2020). A 241-Year Cryptomeria fortune tree-ring chronology in humid subtropical China and its linkages with the pacific decadal oscillation. Atmosphere 11:247. doi: 10.3390/atmos11030247
Dox, I., Gričar, J., Marchand, L. J., Leys, S., Zuccarini, P., Geron, C., et al. (2020). Timeline of autumn phenology in temperate deciduous trees. Tree Physiol. 40, 1001–1013. doi: 10.1093/treephys/tpaa058
Eilmann, B., Zweifel, R., Buchmann, N., Graf Pannatier, E., and Rigling, A. (2011). Drought alters timing, quantity, and quality of wood formation in Scots pine. J. Exp. Bot. 62, 2763–2771. doi: 10.1093/jxb/erq443
Fonti, M. V., Babushkina, E. A., Zhirnova, D. F., and Vaganov, E. A. (2020). Xylogenesis of scots pine in an uneven-aged stand of the minusinsk depression (Southern Siberia). J. Sib. Fed. Univ. Biol. 13, 197–207.
Garcia-Forner, N., Vieira, J., Nabais, C., Carvalho, A., Martínez-Vilalta, J., and Campelo, F. (2019). Climatic and physiological regulati on of the bimodal xylem formation pattern in Pinus pinaster saplings. Tree Physiol. 39, 2008–2018.
Gärtner, H., Cherubini, P., Fonti, P., von Arx, G., Schneider, L., Nievergelt, D., et al. (2015). Technical challenges in tree-ring research including wood anatomy and dendroecology. J. Vis. Exp. 97:52337.
Gričar, J., Prislan, P., De Luis, M., Gryc, V., Hacurová, J., Vavrčík, H., et al. (2015). Plasticity in variation of xylem and phloem cell characteristics of Norway spruce under different local conditions. Front. Plant Sci. 6:730. doi: 10.3389/fpls.2015.00730
Gričar, J., Zupančič, M., Čufar, K., Koch, G., Schmitt, U., and Oven, P. (2006). Effect of local heating and cooling on cambial activity and cell differentiation in the stem of Norway spruce (Picea abies). Ann. Bot. 97, 943–951.
Grossiord, C., Buckley, T. N., Cernusak, L. A., Novick, K. A., Poulter, B., Siegwolf, R. T. W., et al. (2020). Plant responses to rising vapor pressure deficit. New Phytol. 226, 1550–1566. doi: 10.1111/nph.16485
Grotkopp, E., and Rejmánek, M. (2007). High seedling relative growth rate and specific leaf area are traits of invasive species: phylogenetically independent contrasts of woody angiosperms. Am. J. Botany 94, 526–532.
Gruber, A., Strobl, S., Veit, B., and Oberhuber, W. (2010). Impact of drought on the temporal dynamics of wood formation in Pinus sylvestris. Tree Physiol. 30, 490–501. doi: 10.1093/treephys/tpq003
Guada, G., Vázquez-Ruiz, R. A., and García-González, I. (2020). Meteorological conditions control the cessation rather than the beginning of wood formation in a sub-Mediterranean ring-porous oak. Agricultural Forest Meteorol. 281:107833. doi: 10.1016/j.agrformet.2019.107833
Hamilton, J. A., El Kayal, W., Hart, A. T., Runcie, D. E., Arango-Velez, A., and Cooke, J. E. (2016). The joint influence of photoperiod and temperature during growth cessation and development of dormancy in white spruce (Picea glauca). Tree Physiol. 36, 1432–1448.
Huang, J. G., Guo, X., Rossi, S., Zhai, L., Yu, B., Zhang, S., et al. (2018). Intra-annual wood formation of subtropical Chinese red pine shows better growth in dry season than wet season. Tree Physiol. 38, 1225–1236. doi: 10.1093/treephys/tpy046
Huang, Y., Deng, X., Zhao, Z., Xiang, W., Yan, W., Ouyang, S., et al. (2019). Monthly radial growth model of Chinese Fir (Cunninghamia lanceolata (Lamb.) Hook.), and the relationships between radial increment and climate factors. Forests 10:757.
Kellomäki, S. (2001). Growth and resource use of birch seedlings under elevated carbon dioxide and temperature. Ann. Bot. 87, 669–682. doi: 10.1006/anbo.2001.1393
Li, X., Rossi, S., Liang, E., and Julio Camarero, J. (2016). Temperature thresholds for the onset of xylogenesis in alpine shrubs on the Tibetan Plateau. Trees 30, 2091–2099. doi: 10.1007/s00468-016-1436-z
Li, Y., Dong, Z., Chen, D., Zhao, S., Zhou, F., Cao, X., et al. (2019). Growth decline of Pinus massoniana in response to warming induced drought and increasing intrinsic water use efficiency in humid subtropical China. Dendrochronologia 57:125609. doi: 10.1016/j.dendro.2019.125609
Liu, L., Gudmundsson, L., Hauser, M., Qin, D., Li, S., and Seneviratne, S. I. (2020). Soil moisture dominates dryness stress on ecosystem production globally. Nat. Commun. 11:4892. doi: 10.1038/s41467-020-18631-1
Liu, Q., Zhou, T., Mao, H., and Fu, C. (2019). Decadal variations in the relationship between the Western Pacific subtropical high and summer heat waves in East China. J. Climate 32, 1627–1640. doi: 10.1175/jcli-d-18-0093.1
Liu, X., Wang, C., and Zhao, J. (2019). Seasonal drought effects on intra-annual stem growth of taiwan pine along an elevational gradient in subtropical China. Forests 10:1128.
Liu, X., Nie, Y., and Wen, F. (2018). Seasonal dynamics of stem radial increment of Pinus taiwanensis hayata and its response to environmental factors in the lushan mountains. Southeastern China. Forests 9:387. doi: 10.3390/f9070387
Marchand, L. J., Dox, I., Gričar, J., Prislan, P., Leys, S., Van den Bulcke, J., et al. (2020). Inter-individual variability in spring phenology of temperate deciduous trees depends on species, tree size and previous year autumn phenology. Agricultural Forest Meteorol. 290:108031. doi: 10.1016/j.agrformet.2020.108031
Mathur, S., Agrawal, D., and Jajoo, A. (2014). Photosynthesis: response to high temperature stress. J. Photochem. Photobiol. B: Biol. 137, 116–126.
Michelot, A., Simard, S., Rathgeber, C., Dufrene, E., and Damesin, C. (2012). Comparing the intra-annual wood formation of three European species (Fagus sylvatica, Quercus petraea and Pinus sylvestris) as related to leaf phenology and non-structural carbohydrate dynamics. Tree Physiol. 32, 1033–1045. doi: 10.1093/treephys/tps052
Novak, K., De Luis, M., Gričar, J., Prislan, P., Merela, M., Smith, K. T., et al. (2016). Missing and dark rings associated with drought in Pinus halepensis. IAWA J. 37, 260–274. doi: 10.1163/22941932-20160133
Oberhuber, W., and Gruber, A. (2010). Climatic influences on intra-annual stem radial increment of Pinus sylvestris (L.) exposed to drought. Trees (Berl West) 24, 887–898. doi: 10.1007/s00468-010-0458-451
Ouyang, S., Xiao, K., Zhao, Z., Xiang, W., Xu, C., Lei, P., et al. (2018). Stand transpiration estimates from recalibrated parameters for the granier equation in a Chinese Fir (Cunninghamia lanceolata) plantation in Southern China. Forests 9:162. doi: 10.3390/f9040162
Pacheco, A., Camarero, J. J., and Carrer, M. (2016). Linking wood anatomy and xylogenesis allows pinpointing of climate and drought influences on growth of coexisting conifers in continental Mediterranean climate. Tree Physiol. 36, 502–512. doi: 10.1093/treephys/tpv125
Palombo, C., Fonti, P., Lasserre, B., Cherubini, P., Marchetti, M., and Tognetti, R. (2018). Xylogenesis of compression and opposite wood in mountain pine at a Mediterranean treeline. Ann. Forest Sci. 75:93. doi: 10.1007/s13595-018-0773-z
Pan, Y., Birdsey, R. A., Fang, J., Houghton, R., Kauppi, P. E., Kurz, W. A., et al. (2011). A large and persistent carbon sink in the world’s forests. Science 333, 988–993.
Prislan, P., Gricar, J., De Luis, M., Novak, K., Martinez Del, Castillo, E., et al. (2016). Annual cambial rhythm in Pinus halepensis and Pinus sylvestris as indicator for climate adaptation. Front. Plant Sci. 7:1923. doi: 10.3389/fpls.2016.01923
Pumijumnong, N., and Buajan, S. (2012). Seasonal cambial activity of five tropical tree species in central Thailand. Trees 27, 409–417. doi: 10.1007/s00468-012-0794-794
Queen, J. P., Quinn, G. P., and Keough, M. J. (2002). Experimental Design and Data Analysis for Biologists. Cambridge: Cambridge University Press.
R Core Team (2013). R: A Language and Environment for Statistical Computing. Vienna: R Foundation for Statistical Computing.
Rahman, M. H., Nugroho, W. D., Nakaba, S., Kitin, P., Kudo, K., Yamagishi, Y., et al. (2019). Changes in cambial activity are related to precipitation patterns in four tropical hardwood species grown in Indonesia. Am. J. Botany 106, 760–771.
Ren, P., Rossi, S., Gricar, J., Liang, E., and Cufar, K. (2015). Is precipitation a trigger for the onset of xylogenesis in Juniperus przewalskii on the north-eastern Tibetan Plateau? Ann. Botany 115, 629–639.
Ren, P., Rossi, S., Camarero, J. J., Ellison, A. M., Liang, E., and Peñuelas, J. (2018). Critical temperature and precipitation thresholds for the onset of xylogenesis of Juniperus przewalskii in a semi-arid area of the north-eastern Tibetan Plateau. Ann. Bot. 121, 617–624. doi: 10.1093/aob/mcx188.
Ren, P., Ziaco, E., Rossi, S., Biondi, F., Prislan, P., and Liang, E. (2019). Growth rate rather than growing season length determines wood biomass in dry environments. Agricultural Forest Meteorol. 271, 46–53. doi: 10.1016/j.agrformet.2019.02.031
Rossi, S., Anfodillo, T., Čufar, K., Cuny, H. E., Deslauriers, A., Fonti, P., et al. (2016). Pattern of xylem phenology in conifers of cold ecosystems at the Northern Hemisphere. Global Change Biol. 22, 3804–3813.
Rossi, S., Anfodillo, T., and Menardi, R. (2006). Trephor: a new tool for sampling microcores from tree stems. IAWA J. 27, 89–97. doi: 10.1163/22941932-90000139
Rossi, S., Deslauriers, A., Griçar, J., Seo, J.-W., Rathgeber, C. B. K., Anfodillo, T., et al. (2008). Critical temperatures for xylogenesis in conifers of cold climates. Global Ecol. Biogeography 17, 696–707. doi: 10.1111/j.1466-8238.2008.00417.x
Rossi, S., Deslauriers, A., and Morin, H. (2003). Application of the Gompertz equation for the study of xylem cell development. Dendrochronologia 21, 33–39.
Singh, N. D., and Venugopal, N. (2011). Cambial activity and annual rhythm of xylem production of Pinus kesiya Royle ex. Gordon (Pinaceae) in relation to phenology and climatic factors growing in sub-tropical wet forest of North East India. Flora-Morphol. Distribut. Funct. Ecol. Plants 206, 198–204.
Song, X., Lyu, S., and Wen, X. (2020). Limitation of soil moisture on the response of transpiration to vapor pressure deficit in a subtropical coniferous plantation subjected to seasonal drought. J. Hydrol. 591:125301.
Stocker, T. F., Qin, D., Plattner, G.-K., Tignor, M., Allen, S. K., Boschung, J., et al. (2013). Climate Change 2013. The Physical Science Basis. Working Group I Contribution to the 5th Assessment Report of the Intergovernmental Panel on Climate Change—Abstract for Decision-Makers.
Strand, M., Lundmark, T., Söderbergh, I., and Mellander, P.-E. (2002). Impacts of seasonal air and soil temperatures on photosynthesis in Scots pine trees. Tree Physiol. 22, 839–847.
Sun, M., Jiang, M., Zhou, Z., and Zheng, L. (1990). Investigation study on mixed forest of Chinese fir and Cryptomeria. J. Zhejiang For. Sci. Technol.
Tian, D., Xiang, W., Chen, X., Yan, W., Fang, X., Kang, W., et al. (2011). A long-term evaluation of biomass production in first and second rotations of Chinese fir plantations at the same site. Forestry 84, 411–418. doi: 10.1093/forestry/cpr029
Trouet, V., Mukelabai, M., Verheyden, A., and Beeckman, H. (2012). Cambial growth season of brevi-deciduous Brachystegia spiciformis trees from south central Africa restricted to less than four months. PLoS One 7:e47364. doi: 10.1371/journal.pone.0047364
Vieira, J., Rossi, S., Campelo, F., Freitas, H., and Nabais, C. (2014). Xylogenesis of Pinus pinaster under a Mediterranean climate. Ann. Forest Sci. 71, 71–80.
Wu, C., Hong, W., Lin, C., and Wei, Z. (2001). A study on the competition of interspecies in Cryptomereu forturei & Cunninghamia lanceolata mixed forest. J. Biomath. 16, 247–251.
Yan, J., Zhang, Y., Yu, G., Zhou, G., Zhang, L., Li, K., et al. (2013). Seasonal and inter-annual variations in net ecosystem exchange of two old-growth forests in southern China. Agricultural Forest Meteorol. 182, 257–265.
Yu, B., Huang, J.-G., Ma, Q., Guo, X., Liang, H., Zhang, S., et al. (2019). Comparison of the effects of canopy and understory nitrogen addition on xylem growth of two dominant species in a warm temperate forest. China. Dendrochronologia 56:125604.
Zhang, J., Gou, X., Alexander, M. R., Xia, J., Wang, F., Zhang, F., et al. (2021). Drought limits wood production of Juniperus przewalskii even as growing seasons lengthens in a cold and arid environment. Catena 196: 104936.
Zhang, J., Gou, X., Pederson, N., Zhang, F., Niu, H., Zhao, S., et al. (2018). Cambial phenology in Juniperus przewalskii along different altitudinal gradients in a cold and arid region. Tree Physiol. 38, 840–852.
Zhang, J., Gou, X., Zhao, Z., Liu, W., Zhang, F., Cao, Z., et al. (2013). Improved method of obtaining micro-core paraffin sections in dendroecological research. Chinese J. Plant Ecol. 37, 972–977.
Zhang, Y., Xu, J., Su, W., Zhao, X., and Xu, X. (2019). Spring precipitation effects on formation of first row of earlywood vessels in quercus variabilis at qinling mountain (China). Trees-Structure Funct. 33, 457–468. doi: 10.1007/s00468-018-1792-y
Zhao, H., Yang, W., Wu, F., and Tan, B. (2017). Mixed forest plantations can efficiently filter rainfall deposits of sulfur and chlorine in Western China. Sci. Rep. 7:41680. doi: 10.1038/srep41680
Zhou, G., Peng, C., Li, Y., Liu, S., Zhang, Q., Tang, X., et al. (2013). A climate change-induced threat to the ecological resilience of a subtropical monsoon evergreen broad-leaved forest in Southern China. Global Change Biol. 19, 1197–1210.
Keywords: cambium, subtropical forest, xylem formation, xylogenesis, conifers
Citation: Zheng Z, Zhou F, Fonti P, Ren P, Li X, Miao G, Dong Z and Fang K (2021) Intra-Annual Wood Formation of Cryptomeria fortunei and Cunninghamia lanceolata in Humid Subtropical China. Front. Ecol. Evol. 9:733974. doi: 10.3389/fevo.2021.733974
Received: 30 June 2021; Accepted: 01 October 2021;
Published: 17 November 2021.
Edited by:
Andrea Seim, University of Freiburg, GermanyReviewed by:
Cristina Nabais, University of Coimbra, PortugalPeter Prislan, Slovenian Forestry Institute, Slovenia
Copyright © 2021 Zheng, Zhou, Fonti, Ren, Li, Miao, Dong and Fang. This is an open-access article distributed under the terms of the Creative Commons Attribution License (CC BY). The use, distribution or reproduction in other forums is permitted, provided the original author(s) and the copyright owner(s) are credited and that the original publication in this journal is cited, in accordance with accepted academic practice. No use, distribution or reproduction is permitted which does not comply with these terms.
*Correspondence: Keyan Fang, a2ZhbmdAZmpudS5lZHUuY24=