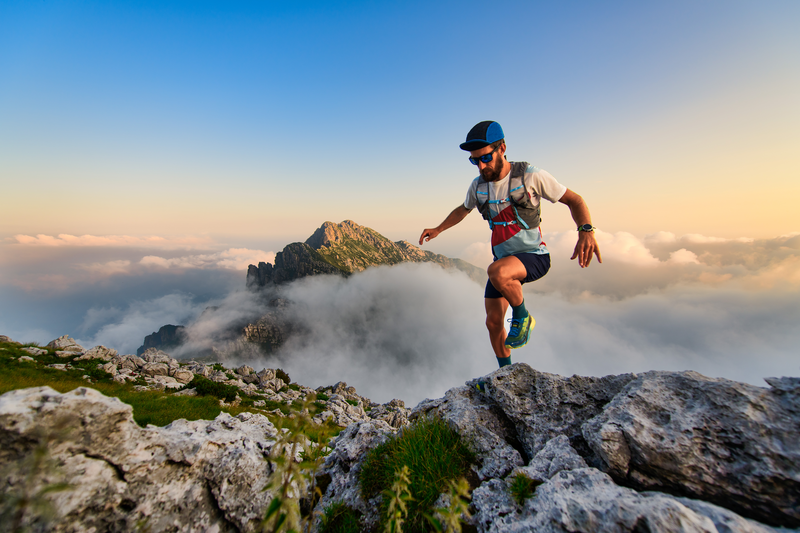
94% of researchers rate our articles as excellent or good
Learn more about the work of our research integrity team to safeguard the quality of each article we publish.
Find out more
PERSPECTIVE article
Front. Ecol. Evol. , 18 November 2021
Sec. Evolutionary Ecology of Social Behaviour
Volume 9 - 2021 | https://doi.org/10.3389/fevo.2021.733228
This article is part of the Research Topic Neuroethology of the Colonial Mind: Ecological and Evolutionary Context of Social Brains View all 14 articles
The evolution of social systems can place novel selective forces on investment in expensive neural tissue by changing cognitive demands. Previous hypotheses about the impact of sociality on neural investment have received equivocal support when tested across diverse taxonomic groups and social structures. We suggest previous models for social behavior-brain relationships have overlooked important variation in social groups. Social groups vary significantly in structure and function, and the specific attributes of a social group may be more relevant to setting cognitive demands than sociality in general. We have identified intragroup competition, relationship differentiation, information sharing, dominance hierarchies, and task specialization and redundancy as attributes of social behavior which may impact selection for neural investment, and outline how variation in these attributes can result in increased or decreased neural investment with transitions to sociality in different taxa. Finally, we test some of the predictions generated using this framework in a phylogenetic comparison of neural tissue investment in Anelosimus social spiders. Social Anelosimus spiders engage in cooperative prey capture and brood care, which allows for individual redundancy in the completion of these tasks. We hypothesized that in social spider species, the presence of redundancy would reduce selection for individual neural investment relative to subsocial species. We found that social species had significantly decreased investment in the arcuate body, the cognitive center of the spider brain, supporting our predictions. Future comparative tests of brain evolution in social species should account for the special behavioral characteristics that accompany social groups in the subject taxa.
Evolutionary transitions of social behavior can introduce novel cognitive demands (Silk, 2007). Metabolic costs of neural tissue are disproportionately high and brain tissue investment should be constrained to meet cognitive demands (Niven and Laughlin, 2008; Iwaniuk, 2017). Origins or modification of sociality may drive changes in brain tissue investment. Several hypotheses have been advanced to explain changes in neural tissue investment associated with transitions in social behavior, generally referred to as “social brain” hypotheses (Dunbar, 1998). Initially, social brain hypotheses assumed there would be a positive effect of sociality on brain investment, but more recent theoretical treatments (Gronenberg and Riveros, 2009; Lihoreau et al., 2012) and empirical findings (vertebrates: Gonzalez-Voyer et al., 2009; Fedorova et al., 2017; Kverková et al., 2018; social insects: O’Donnell et al., 2015) have called this assumption into question.
Studies to date have not adequately identified or accounted for varying behavioral selective pressures that could change cognitive demands as sociality evolves. Analyzing the diversity of social interactions can contribute to more rigorous theory linking social environments with brain evolution. We suggest comparative tests of social brain hypotheses should explicitly consider which aspects of behavior change during transitions from solitary to group living, or during transitions in social complexity, in each study taxon. We identify some key features of social systems that can act as independent selective forces on neural investment. We explore how each of the following attributes of social systems can affect cognitive demands and thus selection for neural investment: intragroup competition, individual recognition, information sharing, dominance hierarchies, and task performance specialization and redundancy. We predict that some of these behavioral attributes will decrease the fitness effects of individual cognitive abilities, such that selection for neural investment will be relaxed. In contrast, other attributes of sociality may place greater cognitive demands on individuals, selecting for increased neural investment.
It is important to note that few, if any, of the behavioral attributes we explore are universally present among social taxa. The relevance of these behavioral attributes vary among animal taxa, depending in part on how social groups form and function. An important complicating factor is the role of individual vs. group/colony selection as the main determinant of fitness in a species (O’Donnell et al., 2018). Variation among animal lineages in the structure of social groups provides opportunities for cross-taxon comparative analyses, which can be used to assess the relative importance of different behavioral factors as determinants of neural investment.
Our paper is divided into two main sections. In the first section we present some attributes of social behavior that could independently impact cognitive demands on animals, either increasing or relaxing selection for brain investment. We discuss potential effects of these demands on the evolution of brain investment. The second section is an empirical test of social brain effects in a taxon with diverse grades of sociality and a relatively simple social system (cobweb spiders: Avilés and Guevara, 2017). Social cobweb spider species exhibit task sharing and redundancy among females; social males and subsocial species do not (Samuk et al., 2011; Harwood and Avilés, 2013, 2018). Furthermore, social spiders do not exhibit some potentially confounding elements of sociality, such as dominance hierarchies and task specialization (Lubin, 1995; Settepani et al., 2013) that could complicate the interpretation of social brain effects comparisons.
The evolution of social behavior potentially increases the frequency or intensity of competition between animals, and social competitive costs must nearly always be compensated by other fitness payoffs to favor sociality (Silk, 2007). Social group participation can drive increased brain tissue investment, either in response to or in anticipation of competitive interactions. Greater neural investment can improve performance on behavioral tasks, such as mate location, and may confer individual benefits in highly competitive social environments (Kotrschal et al., 2015). Potential relationships of heightened competition with brain investment are illustrated by species where social groups involve temporary or facultative aggregations that can increase local competition for food or other resources. In desert locusts, the transition from solitary living to the formation of massive swarms that compete for food and engage in cannibalism (Guttal et al., 2012) is accompanied by increased total brain investment and greater relative investment in the mushroom bodies, which are cognitive integrating regions of the insect brain (Ott and Rogers, 2010). Experimental manipulations that induce sociality in species that are typically solitary have found similar increases in cognitive performance or neural investment in social groups. In Drosophila melanogaster fruit flies, larval crowding resulted in increased food competition and was associated with greater adult neural investment (Heisenberg et al., 1995); juvenile Marpissa muscosa jumping spiders reared in groups had significantly improved performance on learning assays although competition was not directly implicated in this study (Liedtke and Schneider, 2017).
Individual recognition can be cognitively demanding because it requires discriminating and remembering the individual identities of social partners (Shultz and Dunbar, 2006; Bergman and Beehner, 2015). In addition to the number of unique relationships, qualitative differences in interactions between group members can impose different cognitive demands. For example, monogamous pair bonds are common among birds, but species vary in the level of pairs’ cooperation and affiliative behaviors (Emery et al., 2007). Emery et al. (2007) proposed that increased total brain investment in some bird species can be attributed to a higher degree of cooperation during brood care, increased affiliative displays, and social support interactions among mates. Social alliances in particular may be expected to impose higher cognitive demands because of the necessity to remember group member identities, predict the outcome of interactions, and recognize others with similar status (Chapais, 1995).
Dominance hierarchies describe ranks of group members in relation to one another (Drews, 1993). The criteria used to define a “dominant position” vary among species but may include increased access to food resources or reproductive opportunities relative to other members of a group (Drews, 1993). Achieving dominant status and maintaining a hierarchy may increase cognitive demands (and brain investment) by requiring dominant individuals to differentiate group member relationships (Dunbar, 1992). Socially dominant positions in hierarchies are associated with increased neural investment in socially flexible bees relative to both solitary and subordinate individuals (Smith et al., 2010; Rehan et al., 2015). A loss of sociality in a primitively social bee lineage resulted in decreased foundress neural investment, potentially due to the loss of dominance interactions (Pahlke et al., 2019, 2020).
The expected relationship of dominance behavior with brain investment is complicated because dominance hierarchies do not always rely on cognitively demanding, individualized aggressive behaviors. Some hierarchies rely partially or entirely on chemical signaling (Faulkes and Bennett, 2001; Sundström and Boomsma, 2001; Orlova et al., 2020). In hierarchies which do not rely on aggressive interactions, selection for neural investment to navigate social interactions may be minimal (Lihoreau et al., 2012). An additional complication is that dominance status can affect other aspects of behavior such as task performance. For example, reproductively-dominant social insect queens may perform few behaviors other than egg laying (Noirot, 1989; Barchuk et al., 2018), potentially permitting reduced brain investment for dominants compared to subordinates (Barchuk et al., 2018; Gordon et al., 2019; but see O’Donnell et al., 2017). In these systems, neural investment in dominants and subordinates may no longer be driven by social interactions, but instead by caste-specific division of labor (thus falling under the task specialization attribute, described below).
Information sharing allows social animals to respond adaptively to stimuli they have not experienced directly. Information sharing can decrease the fitness costs of inaccurate stimulus detection and may reduce selection for individual cognitive abilities, permitting decreased brain tissue investment. The effect of group information sharing on cognitive demands is well-illustrated by vigilance behaviors. Individual vigilance behavior performance rates decrease in the presence of conspecifics in some species (McBlain et al., 2020; Wang et al., 2021), and predator detection can be faster and more accurate in social groups compared to lone individuals (Ward et al., 2011). However, the need for increased ability to produce, detect and respond to social signals can involve additional cognitive and neural costs. In this case, social and environmental information may be integrated by different parts of the brain, resulting in distinct patterns of mosaic brain evolution: in cichlid fish, environmental complexity is associated with increased telencephalon investment, while increased social complexity is associated with increased hypothalamus investment (Pollen et al., 2007). Thus, for systems in which information sharing occurs, assessing changes in specific brain regions, rather than total brain investment, may better detect the effects of changes in cognitive demands on brain investment.
A common theme in some taxa is for social group members to specialize on a subset of the tasks typically performed by solitary-living individuals (Jeanne, 1986). Individuals may specialize on specific behaviors for all or part of their lifetime; in extreme cases, the evolution of morphologically distinct castes in some social insects limits workers to performing certain behaviors or tasks (Korb and Thorne, 2017; O’Donnell et al., 2018; Gordon et al., 2019). Task specialization is often paired with neural adaptations to meet the cognitive demands of a specific behavioral class (Amador-Vargas et al., 2015; Iwaniuk, 2017), but may result in decreased selection for general cognition (Gronenberg and Riveros, 2009). Task specialization may improve colony-level efficiency (Keller et al., 2011), and decreases in individual neural investment can represent a savings of resource investment by the social group. Reduced individual neural investment may mark a transition to colony-level selection on neural investment (O’Donnell et al., 2015, 2018).
Redundancy, or the availability of multiple individuals to perform or complete a given task, may also reduce individual cognitive demands. Redundancy can facilitate high probabilities of successful task completion even when individual competency is relatively low (Herbers, 1981). Redundancy in colony labor is often present in caste-specialist systems and may be critical for effective task partitioning among group mates (Jeanne, 1999), but redundancy is not restricted to task-specialist systems. Redundancy applies whenever the completion of a shared task is not the responsibility of a single individual, such as in cooperatively breeding social groups (Taborsky et al., 2007; Riehl, 2013). Decreased neural investment has been associated with living in stable groups in woodpeckers (Fedorova et al., 2017), and in cichlid fishes who engage in biparental care (Gonzalez-Voyer et al., 2009). In both of these systems redundancy to complete tasks may reduce individual cognitive demands. In fact, the evolution of redundant cooperation may be more likely to occur when individuals are unaware of group-mates’ social strategies (Pedroso, 2020).
Several evolutionary transitions between subsocial and social behavior have occurred in the cobweb spider genus Anelosimus (Figure 1). In subsocial species, individual females perform extended maternal care but offspring disperse prior to or at adulthood (Yip and Rayor, 2014; Avilés and Guevara, 2017). In social species the offspring do not disperse, leading to the maintenance of permanently communal nests. Social species adult females cooperatively capture prey and share offspring care (Avilés and Tufino, 1998; Yip et al., 2008; Samuk and Avilés, 2013; Avilés and Guevara, 2017). However, social Anelosimus spiders lack task specialization and do not exhibit individual recognition or dominance hierarchies (Jones and Parker, 2000; Aviles and Bukowski, 2006; Settepani et al., 2013). Individual redundancy, resulting from cooperation on maternal care and prey capture, could drive reduced selection for individual competency at these tasks. As predicted, individual females in social Anelosimus species are less competent at maternal care (Samuk et al., 2011) and less effective hunters (Harwood and Avilés, 2018) when compared to females from related subsocial species. We expected brain investment to differ among the sociality levels due to differences in reliance on individual ability to successfully complete maternal care and prey capture tasks. We predicted the arcuate body would be less developed (relatively smaller) in females of the social compared to subsocial species, because the arcuate body is a center of mechanosensory integration (Babu and Barth, 1984; Steinhoff et al., 2018). Unlike females, males of both social and subsocial species die shortly following mating (Avilés and Gelsey, 1998; Avilés and Salazar, 1999; Viera et al., 2007) and have little or no engagement in colony activity, except in nest maintenance (Aviles, 1986). Because male behavior does not differ consistently between the sociality categories, we predicted male brain investment patterns would be similar in subsocial and social species.
Figure 1. (A) Light photomicrographs of stained and sectioned protocerebrum tissue, showing supraesophageal ganglion (SEG) and arcuate body (AB) regions. The 3D reconstruction shows the relative sizes of the SEG (purple) and AB (pink). (B) Brain volume was compared between subsocial (blue) species, which form nests of single mothers and their offspring, who disperse at adulthood, and social (pink) species, which form permanent communal nests with multiple mothers and offspring. (C) Phylogenetic relationships among the sampled species (Luo et al., 2020).
Specimens were collected and preserved in Prefer aldehyde-based fixative solution (Anatech, Ltd.). We sampled adult females from 5 social and 6 subsocial species of Anelosimus spiders (adult males from 5 social and 4 subsocial species), representing at least 3 independent transitions to social behavior (Agnarsson et al., 2006; Figure 1C). Subsocial species: A. arizona (n = 3 females, 3 males); A. baeza (n = 4 females, 3 males); A. elegans (n = 3 females, 3 males); A. jabaquara (n = 3 females); A. studiosus (n = 3 females, 3 males); A. tosum (n = 2 females). Social species: A. domingo (n = 1 female, 3 males); A. dubiosus (n = 3 females, 1 male); A. eximius (n = 3 females, 3 males); A. guacamayos (n = 3 females, 3 males); A. oritoyacu (n = 3 females, 3 males). Specimen collection date and location are detailed in Supplementary Material.
For each subject we removed the legs and opisthosoma and opened the cephalothorax dorsum to remove the venom gland, then embedded in plastic resin comprised of Embed-812 resin, DDSA, DBP, and DMP 30 (Electron Microscopy Services, Inc.), and cured at 60°C for 72 h. Embedded tissue was sectioned into 8–10 um thick slices using a rotary microtome and stained using Toluidine Blue O (Fisher Scientific). Sections were photographed using a light microscope and areas containing neuropil were outlined using ImageJ version 1.53a (Schneider et al., 2012), multiplied by slice thickness, and then converted to volume (mm3) using a pixel-to-millimeter conversion factor. Detailed embedding protocol (O’Donnell et al., 2011).
For each specimen, we measured the protocerebrum, which is considered to be the “true brain” of spiders and is comprised of the supraesophageal ganglion and the arcuate body (Babu and Barth, 1984). The supraesophageal ganglion receives visual sensory input (Babu and Barth, 1984). The arcuate body is an integration center between the subesophageal and supraesophageal ganglia (Babu and Barth, 1984), and may be important for navigating complex environments (Steinhoff et al., 2018). We focused on the arcuate body as a region particular relevant to maternal care tasks and prey capture, due to the necessity to integrate visual and mechanosensory information and coordinate locomotion during these tasks (Steinhoff et al., 2018). We quantified supraesophageal volume (as a brain size reference) and arcuate body volume (Steinhoff et al., 2017).
We used General Linear Models (GLM; SPSS v. 28) and Phylogenetically Generalized Least Squares (PGLS; R version 4.0.2 and the packages ape (Paradis and Schliep, 2019) and phytools (Revell, 2012) to test whether subsocial and social species differed in relative arcuate body investment. We performed separate analyses for male and female brain volume data. Because we expected an allometric relationship between arcuate body volume and brain size (Napiórkowska and Kobak, 2018), we first accounted for brain size effects using supraesophageal ganglion volume as a covariate in the models, then tested for the effects of species sociality category (subsocial vs. social) as a fixed factor. We performed GLM analyses (with type I sums of squares) on the raw species-mean brain data and on log10-transformed brain region volumes to assess the allometry of brain region evolution (Mascaro et al., 2014). To account for possible effects of phylogeny on brain region/behavior relationships we also performed separate phylogenetically generalized least squares regression (PGLS). PGLS allows accounting for linear covariates in the analysis of categorical differences in response variables (in our study, social behavior category differences in arcuate body volume) (Mundry, 2014). We used a published phylogeny of Anelosimus species from Luo et al. (2020), with all branch lengths set to one. We used a PGLS to test the relationship between arcuate body investment and sociality level, with supraesophageal ganglion volume as a covariate (controlling for brain size, as we did in the GLM). Mean species values of brain region volumes used in PGLS analysis were log10 transformed.
For females, social category was a significant predictor of arcuate body volume. After accounting for significant effects of brain size [supraesophageal ganglion volume: GLM F(1, 8) = 12.02, p = 0.008], arcuate body volume was significantly greater in subsocial than in social species [GLM F(1, 8) = 5.72, p = 0.04]. Similar patterns were found in the analysis of log-log transformed brain volume data [Figure 2; supraesophageal ganglion volume effect: GLM F(1, 8) = 16.15, p = 0.004; social category effect: GLM F(1,8) = 7.03, p = 0.03]. The log-log slope of the arcurate body vs. supraesophageal ganglion volume regression was significantly lower than 1.0 (slope = 0.39 ± 0.24 95% CI), demonstrated an allometrically decelerating rate of increase in arcuate body size. Using the PGLS to account for phylogeny supported the effects of sociality category: arcuate body size was greater in subsocial than in social species [Figure 2; PGLS on log transformed data: F(1, 8) = 14.84, p = 0.005]. For males, social behavior category did not predict arcuate body size [GLM linear analysis F(1, 6) = 1.11, p = 0.33; GLM log-log analysis F(1, 6) = 1.72, p = 0.24; PGLS on log transformed data F(1, 6) = 3.3, p = 0.12].
Figure 2. Relationships between log supraesophageal ganglion volume and log arcuate body volume for Anelosimus spiders. (A) Females. (B) Males. Data points represent mean-species values for either social (blue) or subsocial (green) species. Lines represents slopes of linear regression calculated using data from all species. ari A, Arizona; bae A, baeza; dom A, domingo; dub A, dubiosus; ele A, elegans; exi A, eximius; gua A, guacamayos; jab A, jabaquara; ori A,oritoyacu; stu A, studiosus; tos A, tosum.
Female Anelosimus of social species engage in cooperative brood care and prey capture (Avilés and Tufino, 1998; Yip et al., 2008; Harwood and Avilés, 2013, 2018; Samuk and Avilés, 2013; Avilés and Guevara, 2017), and we predicted that individuals’ redundancy in these tasks would reduce individual cognitive demands and relax selection for neural investment compared to subsocial congeners. As predicted, females of social species had significantly reduced relative investment in the arcuate body compared to subsocial species. This pattern did not hold for males which do not differ in task redundancy between subsocial and social species. These results indicate that behavioral redundancy is an attribute of sociality which may promote reduced brain region investment, independently of other social attributes such as task specialization. Application of the behavioral framework we develop to other social systems may encourage the identification of behavioral attributes driving brain evolution that have previously gone unnoticed.
The raw data supporting the conclusions of this article will be made available by the authors, without undue reservation.
LA collected and supplied specimens. All authors listed have made substantial contributions to the work and approved it for publication.
The authors declare that the research was conducted in the absence of any commercial or financial relationships that could be construed as a potential conflict of interest.
All claims expressed in this article are solely those of the authors and do not necessarily represent those of their affiliated organizations, or those of the publisher, the editors and the reviewers. Any product that may be evaluated in this article, or claim that may be made by its manufacturer, is not guaranteed or endorsed by the publisher.
The Supplementary Material for this article can be found online at: https://www.frontiersin.org/articles/10.3389/fevo.2021.733228/full#supplementary-material
Agnarsson, I., Avilés, L., Coddington, J. A., and Maddison, W. P. (2006). Sociality in theridiid spiders: repeated origins of an evolutionary dead end. Evolution 60, 2342–2351.
Amador-Vargas, S., Gronenberg, W., Wcislo, W. T., and Mueller, U. (2015). Specialization and group size: brain and behavioural correlates of colony size in ants lacking morphological castes. Proc. R. Soc. B Biol. Sci. 282:20142502. doi: 10.1098/rspb.2014.2502
Aviles, L. (1986). Sex-ratio bias and possible group selection in the social spider Anelosimus eximius. Am. Nat. 128, 1–12. doi: 10.1086/284535
Aviles, L., and Bukowski, T. C. (2006). Group living and inbreeding depression in a subsocial spider. Proc. Biol. Sci. 273, 157–163. doi: 10.1098/rspb.2005.3308
Avilés, L., and Gelsey, G. (1998). Natal dispersal and demography of a subsocial Anelosimus species and its implications for the evolution of sociality in spiders. Can. J. Zool. 76, 2137–2147.
Avilés, L., and Guevara, J. (2017). “Sociality in spiders,” in Comparative Social Evolution, eds D. R. Rubenstein and P. Abbot (Cambridge: Cambridge University Press), 188–223. doi: 10.1017/9781107338319.008
Avilés, L., and Salazar, P. (1999). Notes on the social structure, life cycle, and behavior of Anelosimus rupununi. J. Arachnol. 27, 497–502.
Avilés, L., and Tufino, P. (1998). Colony size and individual fitness in the social spider Anelosimus eximius. Am. Nat. 152, 403–418. doi: 10.1086/286178
Babu, K. S., and Barth, F. G. (1984). Neuroanatomy of the central nervous system of the wandering spider, Cupiennius salei (Arachnida, Araneida). Zoomorphology 104, 344–359. doi: 10.1007/bf00312185
Barchuk, A. R., dos Santos, G. D., Dias Caneschi, R., de Paula Junior, D. E., and Moda, L. M. R. (2018). The ontogenetic saga of a social brain. Apidologie 49, 32–48. doi: 10.1007/s13592-017-0540-4
Bergman, T. J., and Beehner, J. C. (2015). Measuring social complexity. Anim. Behav. 103, 203–209. doi: 10.1016/j.anbehav.2015.02.018
Chapais, B. (1995). Alliances as a means of competition in primates: evolutionary, developmental, and cognitive aspects. Am. J. Phys. Anthropol. 38, 115–136.
Drews, C. (1993). The concept and definition of dominance in animal behaviour. Behaviour 125, 283–313.
Dunbar, R. I. M. (1992). Neocortex size as a constraint size in primates on group ecologically. J. Hum. Evol. 20, 469–493. doi: 10.1016/0047-2484(92)90081-j
Emery, N. J., Seed, A. M., Von Bayern, A. M. P., and Clayton, N. S. (2007). Cognitive adaptations of social bonding in birds. Philos. Trans. R. Soc. Lond. B. Biol. Sci. 362, 489–505. doi: 10.1098/rstb.2006.1991
Faulkes, C. G., and Bennett, N. C. (2001). Family values: group dynamics and social control of reproduction in African mole-rats. Trends Ecol. Evol. 16, 184–190. doi: 10.1016/S0169-5347(01)02116-4
Fedorova, N., Evans, C. L., and Byrne, R. W. (2017). Living in stable social groups is associated with reduced brain size in woodpeckers (Picidae). Biol. Lett. 13:20170008. doi: 10.1098/rsbl.2017.0008
Gonzalez-Voyer, A., Winberg, S., and Kolm, N. (2009). Social fishes and single mothers: brain evolution in African cichlids. Proc. R. Soc. B Biol. Sci. 276, 161–167. doi: 10.1098/rspb.2008.0979
Gordon, D. G., Zelaya, A., Arganda-Carreras, I., Arganda, S., and Traniello, J. F. A. (2019). Division of labor and brain evolution in insect societies: neurobiology of extreme specialization in the turtle ant Cephalotes varians. PLoS One 14:e0213618. doi: 10.1371/journal.pone.0219036
Gronenberg, W., and Riveros, A. J. (2009). “Social brains and behavior: past and present,” in Organization of Insect Societies: From Genome to Sociocomplexity, eds J. Gadau and J. Fewell (Cambridge, MA: Harvard University Press), 377–401.
Guttal, V., Romanczuk, P., Simpson, S. J., Sword, G. A., and Couzin, I. D. (2012). Cannibalismcan drive the evolution of behavioural phase polyphenism in locusts. Ecol. Lett. 15, 1158–1166. doi: 10.1111/j.1461-0248.2012.01840.x
Harwood, G., and Avilés, L. (2013). Differences in group size and the extent of individual participation in group hunting may contribute to differential prey-size use among social spiders. Biol. Lett. 9:20130621. doi: 10.1098/rsbl.2013.0621
Harwood, G., and Avilés, L. (2018). The shortfall of sociality: group-living affects hunting performance of individual social spiders. Behav. Ecol. 29, 1487–1493. doi: 10.1093/beheco/ary099
Heisenberg, M., Heusipp, M., and Wanke, C. (1995). Structural plasticity in the Drosophila brain. J. Neurosci. 15, 1951–1960. doi: 10.1523/jneurosci.15-03-01951.1995
Herbers, J. M. (1981). Reliability theory and foraging by ants. J. Theor. Biol. 89, 175–189. doi: 10.1016/0022-5193(81)90184-3
Iwaniuk, A. N. (2017). “Functional correlates of brain and brain region sizes in nonmammalian vertebrates,” in Evolution of Nervous Systems, Vol. 1, ed. J. Kaas (Oxford: Elsevier), 335–348. doi: 10.1007/s002390010256
Jeanne, R. (1986). The evolution of the organization of work in social insects. Monit. Zool. Ital. 20, 119–133.
Jeanne, R. (1999). “Group size, productivity, and information flow in social wasps,” in Information Processing in Social Insects, eds C. Detrain, J. L. Deneubourg, and J. M. Pasteels (Switzerland: Birkhauser Verlag Basel), 3–30. doi: 10.1007/978-3-0348-8739-7_1
Jones, T. C., and Parker, P. G. (2000). Costs and benefits of foraging associated with delayed dispersal in the spider Anelosimus studiosus (Araneae, Theridiidae). J. Arachnol. 28, 61–69. doi: 10.1636/0161-8202(2000)028[0061:cabofa]2.0.co;2
Keller, L., Duarte, A., Weissing, F. J., and Pen, I. (2011). An evolutionary perspective on self-organized division of labor in social insects. Annu. Rev. Ecol. Evol. Syst. 42, 91–110. doi: 10.1146/annurev-ecolsys-102710-145017
Korb, J., and Thorne, B. (2017). “Sociality in termites,” in Comparative Social Evolution, eds D. R. Rubenstein and P. Abbot (Cambridge: Cambridge University Press), 124–153.
Kotrschal, A., Corral-Lopez, A., Amcoff, M., and Kolm, N. (2015). A larger brain confers a benefit in a spatial mate search learning task in male guppies. Behav. Ecol. 26, 527–532. doi: 10.1093/beheco/aru227
Kverková, K., Belíkova, T., Olkowicz, S., Pavelkova, Z., O’Riain, M. J., Sumbera, R., et al. (2018). Sociality does not drive the evolution of large brains in eusocial African mole-rats. Sci. Rep. 8:9203.
Liedtke, J., and Schneider, J. M. (2017). Social makes smart: rearing conditions affect learning and social behaviour in jumping spiders. Anim. Cogn. 20, 1093–1106. doi: 10.1007/s10071-017-1125-3
Lihoreau, M., Latty, T., and Chittka, L. (2012). An exploration of the social brain hypothesis in insects. Front. Physio. 3:442. doi: 10.3389/fphys.2012.00442
Lubin, Y. (1995). Is there division-of-labor in the social spider Achaearanea wau (Theridiidae)? Anim. Behav. 49, 1315–1323.
Luo, Y., Goh, S. P., Li, D., Gonzaga, M. O., Santos, A. J., Tanikawa, A., et al. (2020). Global diversification of anelosimus spiders driven by long-distance overwater dispersal and neogene climate oscillations. Syst. Biol. 69, 1122–1136. doi: 10.1093/sysbio/syaa017
Mascaro, J., Litton, C. M., Hughes, R. F., Uowolo, A., and Schnitzer, S. A. (2014). Is logarithmic transformation necessary in allometry? Ten, one-hundred, one-thousand-times yes. Biol. J. Linn. Soc. 111, 230–233. doi: 10.1111/bij.12177
McBlain, M., Jones, K. A., and Shannon, G. (2020). Sleeping eurasian oystercatchers adjust their vigilance in response to the behaviour of neighbours, human disturbance and environmental conditions. J. Zool. 312, 75–84. doi: 10.1111/jzo.12812
Mundry, R. (2014). “Statistical issues and assumptions of phylogenetic generalized least squares,” in Modern Phylogenetic Comparative Methods and Their Application in Evolutionary Biology, ed. L. Z. Garamszegi (Berlin: Springer), 131–153. doi: 10.1007/978-3-662-43550-2_6
Napiórkowska, T., and Kobak, J. (2018). The allometry of the arcuate body in the postembryonic development of the giant house spider Eratigena atrica. Invert. Neurosci. 18:3. doi: 10.1007/s10158-018-0208-4
Niven, J. E., and Laughlin, S. B. (2008). Energy limitation as a selective pressure on the evolution of sensory systems. J. Exp. Biol. 211, 1792–1804. doi: 10.1242/jeb.017574
Noirot, C. (1989). Social structure in termite societies. Ethol. Ecol. Evol. 1, 1–17. doi: 10.1080/08927014.1989.9525528
O’Donnell, S., Clifford, M., and Molina, Y. (2011). Comparative analysis of constraints and caste differences in brain investment among social paper wasps. Proc. Natl. Acad. Sci. U.S.A. 108, 7107–7112. doi: 10.1073/pnas.1017566108
O’Donnell, S., Bulova, S., Barrett, M., and von Beeren, C. (2018). Brain investment under colony-level selection: soldier specialization in Eciton army ants (Formicidae: Dorylinae). BMC Zool. 3:3. doi: 10.1186/s40850-018-0028-3
O’Donnell, S., Bulova, S. J., DeLeon, S., Barrett, M., and Fiocca, M. (2017). Caste differences in the mushroom bodies of swarm-founding paper wasps: implications for brain plasticity and brain evolution (Vespidae, Epiponini). Behav. Ecol. Sociobiol. 71:116. doi: 10.1007/s00265-017-2344-y
O’Donnell, S., Bulova, S. J., DeLeon, S., Khodak, P., Miller, S., and Sulger, E. (2015). Distributed cognition and social brains: reductions in mushroom body investment accompanied the origins of sociality in wasps (Hymenoptera: Vespidae). Proc. Biol. Sci. 282:20150791. doi: 10.1098/rspb.2015.0791
Orlova, M., Treanore, E., and Amsalem, E. (2020). Built to change: dominance strategy changes with life stage in a primitively eusocial bee. Behav. Ecol. 31, 1361–1368. doi: 10.1093/beheco/araa093
Ott, S. R., and Rogers, S. M. (2010). Gregarious desert locusts have substantially larger brains with altered proportions compared with the solitarious phase. Proc. Biol. Sci. 277, 3087–3096. doi: 10.1098/rspb.2010.0694
Pahlke, S., Jaumann, S., Seid, M. A., and Smith, A. R. (2019). Brain differences between social castes precede group formation in a primitively eusocial bee. Sci. Nat. 106, 2015–2018. doi: 10.1007/s00114-019-1644-7
Pahlke, S., Seid, M. A., Jaumann, S., and Smith, A. (2020). The loss of sociality is accompanied by reduced neural investment in mushroom body volume in the sweat bee Augochlora pura (Hymenoptera: Halictidae). Ann. Entomol. Soc. Am. 114, 637–642. doi: 10.1093/aesa/saaa019
Paradis, E., and Schliep, K. (2019). Ape 5.0: an environment for modern phylogenetics and evolutionary analyses in R. Bioinformatics 35, 526–528. doi: 10.1093/bioinformatics/bty633
Pedroso, M. (2020). Blind cooperation: the evolution of redundancy via ignorance. Br. J. Philos. Sci. 72:3. doi: 10.1093/BJPS/AXZ022
Pollen, A. A., Dobberfuhl, A. P., Scace, J., Igulu, M. M., Renn, S. C. P., Shumway, C. A., et al. (2007). Environmental complexity and social organization sculpt the brain in Lake Tanganyikan cichlid fish. Brain Behav. Evol. 70, 21–39. doi: 10.1159/000101067
Rehan, S. M., Bulova, S. J., and O’Donnell, S. (2015). Cumulative effects of foraging behavior and social dominance on brain development in a facultatively social bee (Ceratina australensis). Brain Behav. Evol. 85, 117–124. doi: 10.1159/000381414
Revell, L. J. (2012). phytools: an R package for phylogenetic comparative biology (and other things). Methods Ecol. Evol. 3, 217–223. doi: 10.1111/j.2041-210X.2011.00169.x
Riehl, C. (2013). Evolutionary routes to non-kin cooperative breeding in birds. Proc. Soc. Sci. 280:20132245. doi: 10.1098/rspb.2013.2245
Samuk, K., and Avilés, L. (2013). Indiscriminate care of offspring predates the evolution of sociality in alloparenting social spiders. Behav. Ecol. Sociobiol. 67, 1275–1284. doi: 10.1007/s00265-013-1555-0
Samuk, K. M., LeDue, E. E., and Aviles, L. (2011). Sister clade comparisons reveal reduced maternal care behavior in social cobweb spiders. Behav. Ecol. 9:20130621. doi: 10.1093/beheco/arr146
Schneider, C. A., Rasband, W. S., and Eliceiri, K. W. (2012). NIH Image to imageJ: 25 years of image analysis. Nat. Methods 9, 671–675. doi: 10.1038/nmeth.2089
Settepani, V., Grinsted, L., Granfeldt, J., Jensen, J. L., and Bilde, T. (2013). Task specialization in two social spiders, Stegodyphus sarasinorum (Eresidae) and Anelosimus eximius (Theridiidae). J. Evol. Biol. 26, 51–62. doi: 10.1111/jeb.12024
Shultz, S., and Dunbar, R. I. M. (2006). Both social and ecological factors predict ungulate brain size. Proc. Biol. Sci. 273, 207–215. doi: 10.1098/rspb.2005.3283
Silk, J. B. (2007). The adaptive value of sociality in mammalian groups. Philos. Trans. R. Soc. Lond. B. Biol. Sci. 362, 539–559. doi: 10.1098/rstb.2006.1994
Smith, A. R., Seid, M. A., Jiménez, L. C., and Wcislo, W. T. (2010). Socially induced brain development in a facultatively eusocial sweat bee Megalopta genalis (Hailctidae). Proc. Biol. Sci. 277, 2157–2163. doi: 10.1098/rspb.2010.0269
Steinhoff, P. O. M., Liedtke, J., Sombke, A., Schneider, J. M., and Uhl, G. (2018). Early environmental conditions affect the volume of higher-order brain centers in a jumping spider. J. Zool. 304, 182–192. doi: 10.1111/jzo.12512
Steinhoff, P. O. M., Sombke, A., Liedtke, J., Schneider, J. M., Harzsch, S., and Uhl, G. (2017). The synganglion of the jumping spider Marpissa muscosa (Arachnida: Salticidae): insights from histology, immunohistochemistry and microCT analysis. Arthropod. Struct. Dev. 46, 156–170. doi: 10.1016/j.asd.2016.11.003
Sundström, L., and Boomsma, J. J. (2001). Conflicts and alliances in insect families. Heredity 86, 515–521. doi: 10.1046/j.1365-2540.2001.00884.x
Taborsky, B., Skubic, E., and Bruintjes, R. (2007). Mothers adjust egg size to helper number in a cooperatively breeding cichlid. Behav. Ecol. 18, 652–657. doi: 10.1093/beheco/arm026
Viera, C., Costa, F. G., Ghione, S., and Benamu-Pino, M. A. (2007). Progeny, development and phenology of the sub-social spider Anelosimus cf. studiosus (Araneae, Theridiidae) from Uruguay. Stud. Neotrop. Fauna Environ. 42, 145–153.
Wang, X., Yang, L., Zhao, Y., Yu, C., and Li, Z. (2021). The group size effect and synchronization of vigilance in the Tibetan wild ass. Curr. Zool. 67, 11–16. doi: 10.1093/cz/zoaa024
Ward, A. J. W., Herbert-Read, J. E., Sumpter, D. J. T., and Krause, J. (2011). Erratum: fast and accurate decisions through collective vigilance in fish shoals. Proc. Natl. Acad. Sci. U.S.A. 108:8. doi: 10.1073/pnas.1101616108
Yip, E. C., Powers, K. S., and Aviles, L. (2008). Cooperative capture of large prey solves scaling challenge faced by spider societies. Proc. Nat. Acad. Sci. U.S.A. 105, 11818–11822. doi: 10.1073/pnas.0710603105
Keywords: neuroecology, social behavior, social brain evolution, social spiders, distributed cognition hypothesis
Citation: Caponera V, Avilés L, Barrett M and O’Donnell S (2021) Behavioral Attributes of Social Groups Determine the Strength and Direction of Selection on Neural Investment. Front. Ecol. Evol. 9:733228. doi: 10.3389/fevo.2021.733228
Received: 30 June 2021; Accepted: 28 October 2021;
Published: 18 November 2021.
Edited by:
Mathieu Lihoreau, Centre National de la Recherche Scientifique (CNRS), FranceReviewed by:
Tom Langen, Clarkson University, United StatesCopyright © 2021 Caponera, Avilés, Barrett and O’Donnell. This is an open-access article distributed under the terms of the Creative Commons Attribution License (CC BY). The use, distribution or reproduction in other forums is permitted, provided the original author(s) and the copyright owner(s) are credited and that the original publication in this journal is cited, in accordance with accepted academic practice. No use, distribution or reproduction is permitted which does not comply with these terms.
*Correspondence: Sean O’Donnell, c28zNTZAZHJleGVsLmVkdQ==
Disclaimer: All claims expressed in this article are solely those of the authors and do not necessarily represent those of their affiliated organizations, or those of the publisher, the editors and the reviewers. Any product that may be evaluated in this article or claim that may be made by its manufacturer is not guaranteed or endorsed by the publisher.
Research integrity at Frontiers
Learn more about the work of our research integrity team to safeguard the quality of each article we publish.