- School of Biological Sciences, University of Adelaide, Adelaide, SA, Australia
Although indirect selection through relatives (kin selection) can explain the evolution of effectively sterile offspring that act as helpers at the nest (eusociality) in the ants, bees, and stinging wasps (aculeate Hymenoptera), the genetic, ecological, and life history conditions that favor transitions to eusociality are poorly understood. In this study, ancestral state reconstruction on recently published phylogenies was used to identify the independent transitions to eusociality in each of the taxonomic families that exhibit eusociality. Semisociality, in which a single nest co-foundress monopolizes reproduction, often precedes eusociality outside the vespid wasps. Such a route to eusociality, which is consistent with groups consisting of a mother and her daughters (subsocial) at some stage and ancestral monogamy, is favored by the haplodiploid genetic sex determination of the Hymenoptera (diploid females and haploid males) and thus may explain why eusociality is common in the Hymenoptera. Ancestral states were also reconstructed for life history characters that have been implicated in the origins of eusociality. A loss of larval diapause during unfavorable seasons or conditions precedes, or coincides with, all but one transition to eusociality. This pattern is confirmed using phylogenetic tests of associations between state transition rates for sweat bees and apid bees. A loss of larval diapause may simply reflect the subsocial route to eusociality since subsociality is defined as females interacting with their adult daughters. A loss of larval diapause and a gain of subsociality may be associated with an extended breeding season that permits the production of at least two broods, which is necessary for helpers to evolve. Adult diapause may also lower the selective barrier to a first-brood daughter becoming a helper. Obligate eusociality meets the definition of a major evolutionary transition, and such transitions have occurred five times in the Hymenoptera.
Introduction
The evolution of an effectively sterile worker caste, known as eusociality (see Table 1 for definitions of social structures), has occurred many times in the aculeate Hymenoptera (ants, bees, and stinging wasps), but explaining these transitions remains a challenge. Although sterile workers are understood to evolve through kin selection (Hamilton, 1964a,b; see Table 2 for definitions of key life history terms), the genetic, ecological, and life history conditions favoring reproductive altruism remain poorly understood (Bourke, 2019; Field and Toyoizumi, 2020). At a minimum, a nest-founding female must live long enough in an environment with a sufficiently long breeding season to produce at least two broods, giving first-brood daughters the opportunity to help raise second-brood siblings. This is known as the subsocial route to eusociality (Wheeler, 1923; Alexander et al., 1991; Bourke, 2011). In addition, it is understood that because monogamy is ancestral to eusocial lineages, a daughter is as related to her own offspring as she is to her siblings (Hughes et al., 2008), whereas a mother remains more closely related to her own offspring than to her daughter’s offspring. This relatedness asymmetry means that a mother may be selected to coerce her first-brood daughters to help raise their second-brood siblings and that daughters may offer little or no resistance (Stubblefield and Charnov, 1986; Boomsma, 2009). Thus, the barriers to a first-brood daughter’s independent breeding, or the advantages to helping, do not have to be great in order for a worker caste to evolve, as long as a daughter can help raise a sufficient number of siblings. The question then is, what genetic, ecological, and life history conditions favor a daughter foregoing her own reproduction to help her mother?
Numerous traits, including genetic, morphological, behavioral, demographic, life history, and ecological traits, have been hypothesized to favor eusociality, but without any consensus as to which are sufficient and which are necessary (reviewed by Andersson, 1984; Crespi, 1996). For example, whether haplodiploidy favors eusociality remains disputed (Hamilton, 1964b; Trivers and Hare, 1976; Seger, 1983; Fromhage and Kokko, 2011; Gardner et al., 2012; Gardner and Ross, 2013). Considering life history traits, multiple nest foundresses, underground nests, progressive brood provisioning, and breeding aseasonality have each been proposed to select for a long lifespan in a nest foundress, resulting in the required overlap between a foundress and her offspring (Cowan, 1991; Matthews, 1991; Queller, 1996b; Brockmann, 1997; Keller and Genoud, 1997; O’Neill, 2001; Danforth et al., 2019; da Silva, in press). It has also been suggested that aboveground nests favor eusociality because they provide greater opportunities to expand the nest and thus allow greater capitalization of workers’ efforts or require the help of workers for the nest’s defense (Alexander et al., 1991; Matthews, 1991). A recent phylogenetic comparative study reports a strong association between a loss of larval diapause and the evolution of social living in bees (Santos et al., 2019), and argues that this pattern supports the hypothesis that eusociality is favored by “split sex ratios” between broods that may arise in partially bivoltine species with mated adult diapause (Seger, 1983).
In the present study, information was collected from the literature for life history characters that have been implicated in the evolution of eusociality and for which data are commonly available across the Aculeata. Using recently published phylogenies, ancestral character states were reconstructed to describe the evolutionary histories of life history characters in relation to transitions to eusociality in all four taxonomic families that exhibit eusociality: Vespidae (vespid wasps), Pemphredonidae (aphid wasps), Halictidae (sweat bees), and Apidae (apid bees). This, the first analysis of its kind across the aculeate Hymenoptera, is possible because of new data on the sociality and life histories of poorly studied taxa and because of the recent stabilization of Hymenopteran phylogeny (Branstetter et al., 2017; Peters et al., 2017; Piekarski et al., 2018; Sann et al., 2018; Bossert et al., 2019). Eusociality was found to have originated more often than previously thought in the Apidae and Halictidae and to be preceded by semisociality outside the Vespidae. A semisocial route to eusociality is consistent with a subsocial route and with ancestral functional monogyny and, because semisociality is favored by haplodiploidy, may explain why eusociality is common in the Hymenoptera. A loss of larval diapause precedes, or coincides with, all but one of the transitions to eusociality. This may simply reflect that subsociality is defined as the interaction between a mother and her adult offspring. In addition, a loss of larval diapause and a gain of subsociality may be associated with an extended breeding season, permitting the production of two broods. Obligate eusociality, which meets the definition of a major evolutionary transition, has evolved five times in the Hymenoptera. An important consequence of these transitions is that inclusive fitness can no longer be calculated for individuals within obligately eusocial groups but must be calculated for the new level of individuality, the colony.
Materials and Methods
Data
All available data were collected for sociality and other life history characters for species in taxonomic families exhibiting eusociality: Vespidae (vespid wasps), Pemphredonidae (aphid wasps), Halictidae (sweat bees), and Apidae (apid bees). The life history characters are:
(1) Sociality (solitary, subsocial, communal, quasisocial, semisocial, primitively eusocial, advanced eusocial)
(2) Number of nest foundresses (single, multiple, swarm)
(3) Nest type (ground, stem/wood, cavity, aerial)
(4) Brood provisioning (mass, progressive)
(5) Breeding seasonality (seasonal, aseasonal)
(6) Diapause (prepupal, adult, none, reproductive quiescence)
The definitions of social organizations of Hymenoptera are those of Cowan (1991), which are modified from those of earlier workers (Wilson, 1971; Michener, 1974; Eickwort, 1981; Table 1). The highest level of social structure reported for a species was used. Life history characters are defined in Table 2. The category of the number of nest foundresses assigned to a species is the highest category recorded. Swarming refers to one or more foundresses and multiple workers establishing a nest. Nests may be excavated in the ground, produced by boring in the soft pith of stems or in soft wood, built in available cavities or constructed in exposed areas. Brood provisioning is either mass, in which a female provides the food required by a larva to complete development all at once, or progressive, in which food is provided incrementally to the growing larva. Diapause refers to a period of developmental dormancy. Prepupal diapause occurs during the last larval instar. Reproductive quiescence refers to a cessation in reproduction without diapause. These data were collected for 317 species and are available as a supplementary dataset for each family (Supplementary Data).
Statistical Analyses
All statistical tests and inferences were carried out using the R language and environment for statistical computing and graphics (R Core Team, 2019).
Ancestral State Reconstruction
Discrete ancestral character states were reconstructed on recent phylogenies using maximum likelihood to fit hidden Markov models (Beaulieu et al., 2013; Beaulieu and O’Meara, 2014), as implemented in the R package corHMM (version 2.1). This method allows rates of transitions between character states to vary across a phylogeny as different rate classes. All transition rates between character states were allowed to differ. Transitions between states could be constrained to not permit certain transitions. The state of the root node was fixed to the ancestral state when there was strong supporting evidence, otherwise the method of FitzJohn et al. (2009) was used to infer the root state (root.p = “maddfitz”). This method weights each root state according to its probability of giving rise to the extant data, given the model parameters and the phylogeny. The states of other internal nodes could also be fixed to test hypotheses about character evolution. Marginal reconstruction was used to estimate the empirical Bayesian posterior probability of each state at each internal node. The state with the highest probability was assigned to each node. One hundred random restarts were used to ensure that the maximum likelihood solution was found. Models with different numbers of rate classes were compared using the Akaike information criterion (AIC). The smallest number of rate classes giving the smallest AIC was used (a difference of 2 AIC units is considered significant). A single rate class is used unless stated otherwise.
For each taxonomic family, the states of the root node for each character were fixed as solitary living, a single nest foundress, ground nesting, mass brood provisioning, seasonal breeding, and prepupal diapause (Wilson, 1971; Michener, 1974; Cowan, 1991; Matthews, 1991; Hunt, 2007; Danforth et al., 2019; Santos et al., 2019), unless stated otherwise. For sociality, state transitions were constrained to follow the subsocial route to eusociality (Alexander et al., 1991; Bourke, 2011; Figure 1A). This may occur directly from subsociality to primitive eusociality or indirectly via parasociality. All forms of parasocial living appear to be also subsocial (Lin and Michener, 1972; Alexander et al., 1991; Cowan, 1991). It is assumed that semisocial living evolved from less complex forms of parasociality, in which mutual tolerance and reproductive cooperation evolved (Cowan, 1991). Of the parasocial social structures, only semisociality is assumed to precede primitive eusociality since monogamy is ancestral to eusociality in the Hymenoptera, and this may be functional in the sense that one co-foundress does all or most of the egg laying (Hughes et al., 2008). It is also assumed that primitive eusociality precedes advanced eusociality (Wilson, 1971; Michener, 1974) and that advanced eusociality represents a point of no return, from which there are no reversions to other forms of sociality (Wilson and Hölldobler, 2005; Boomsma and Gawne, 2018). For diapause, transitions were constrained to be sequential (Figure 1B), reflecting a reduction in diapause as species adapt incrementally to reduced breeding seasonality (e.g., Saito et al., 2009). Reproductive quiescence represents a non-diapause adaptation to breeding seasonality. This model is supported by examples of sequential pairs of states, but no non-sequential pairs, observed within the same genus (e.g., adult diapause ↔ no diapause within the same genus in the Polistinae and the Stenogastrinae).
Testing the Phylogenetic Dependence of Eusociality on Life History Characters
Whether a transition from non-eusociality to eusociality is dependent on the state of a life history character was tested using Pagel’s method of detecting correlated evolution between binary characters on phylogenies (Pagel, 1994). The method uses maximum likelihood to fit models of character state transition rates for each character evolving independently and for the two characters evolving in a correlated fashion, and then compares these models using the log likelihood ratio test to determine if the correlated model provides a better fit. Tests were carried out with sociality as the dependent variable, and P-values were corrected for multiple comparisons within each family using the Holm method (Holm, 1979). Analyses were conducted using the function fitPagel (method = “fitMk”) in the R package phytools (version 0.7-70) (Revell, 2012). Sociality and each life history character were converted to binary characters. For example, the states of sociality were converted to non-eusocial and eusocial. The state of the root of each phylogeny was specified for each character as the ancestral states non-eusocial, solitary foundress, ground nesting, mass brood provisioning, seasonal breeding, and prepupal diapause (or diapause, depending on the form of the binary character).
Phylogenies
Vespidae
The phylogeny for the family Vespidae (vespid wasps) is based on nucleotide sequence data from 378 loci across 136 species (Piekarski et al., 2018). Branch lengths represent the amount of evolutionary change. Species for which life history data were collected, but which were not included in the published tree, were added. When the tree included a single species of the same genus, or a closely related genus, a species was added to the terminal node of the included species and given a terminal branch length of zero. Otherwise, species were added to the deepest node of the smallest clade to which they belong (most recent common ancestor, MRCA), and their terminal branch given a length of zero. In some cases, these additions created polytomies. Other phylogenies were used as guides in the placement of species in the following genera: Vespula (Lopez-Osorio et al., 2015); Vespa (Perrard et al., 2013; Lopez-Osorio et al., 2015); Polistes (Santos et al., 2015).
Pemphredonidae
The phylogeny for the family Pemphredonidae (aphid wasps) is extracted from a time-calibrated phylogeny of the superfamily Apoidea (bees and digger wasps) based on target DNA enrichment and transcriptomic sequence data from 195 single-copy protein-coding genes for 174 species (Sann et al., 2018). Species for which life history data were collected were added to the tree as for the Vespidae, except their terminal branches were extended to the present. The placements of added species were based on their taxonomic classification.
Halictidae
The phylogeny for the family Halictidae (sweat bees) is a composite of two time-calibrated phylogenies. One phylogeny comprises the subfamily Halictinae and is based on sequences from three nuclear genes (elongation factor-1 alpha, wingless, and long-wavelength rhodopsin) and one mitochondrial gene (cytochrome c oxidase 1) for 206 species (Gibbs et al., 2012). The placements of the subfamilies Nomioidinae, Nomiinae, and Rophitinae is from a second phylogeny, of bees (clade Anthophila), based on sequences from two nuclear ribosomal genes (18S and 28S) and five nuclear protein-coding genes (elongation factor-1 alpha, wingless, opsin, pol II, and Nak) for 152 species (Cardinal and Danforth, 2013). The ages for the relevant overlapping nodes from the two phylogenies are consistent. Species for which life history data were collected were added, as for the Vespidae, except their terminal branches were extended to the present. Other phylogenies were used in the placement of species in the following groups: Rophitinae (Patiny et al., 2008); Nomiinae (Brady et al., 2006; Patiny et al., 2008); Augochlorini (Gonçalves, 2016); Agapostemon (Janjic and Packer, 2003); Sphecodes (Habermannova et al., 2013).
Apidae
The phylogeny for the family Apidae (apid bees) is based on genome, transcriptome and ultraconserved element sequences for 79 species (Bossert et al., 2019). Branch lengths represent the amount of evolutionary change. Species for which life history data were collected were added as for the Vespidae. Other phylogenies were used in the placement of species in the following groups: Anthophorinae (Dubitzky, 2007); Nomadinae (Litman et al., 2013); Xylocopinae (Rehan et al., 2012); Eucera (Dorchin et al., 2018); Centridini (Martins and Melo, 2016); Meliponini (Rasmussen and Cameron, 2007); Bombus (Cameron et al., 2007).
Results
Results for ancestral character state reconstructions are presented for each family, with the origins of eusociality inferred first, followed by the evolutionary histories of life history characters in relation to the origins of eusociality. Phylogenies depicting ancestral state reconstructions for each life history character are given in Supplementary Figures 1–5. These results are summarized in Figure 2. Results of tests for the dependence of eusociality on each life history character are then presented.
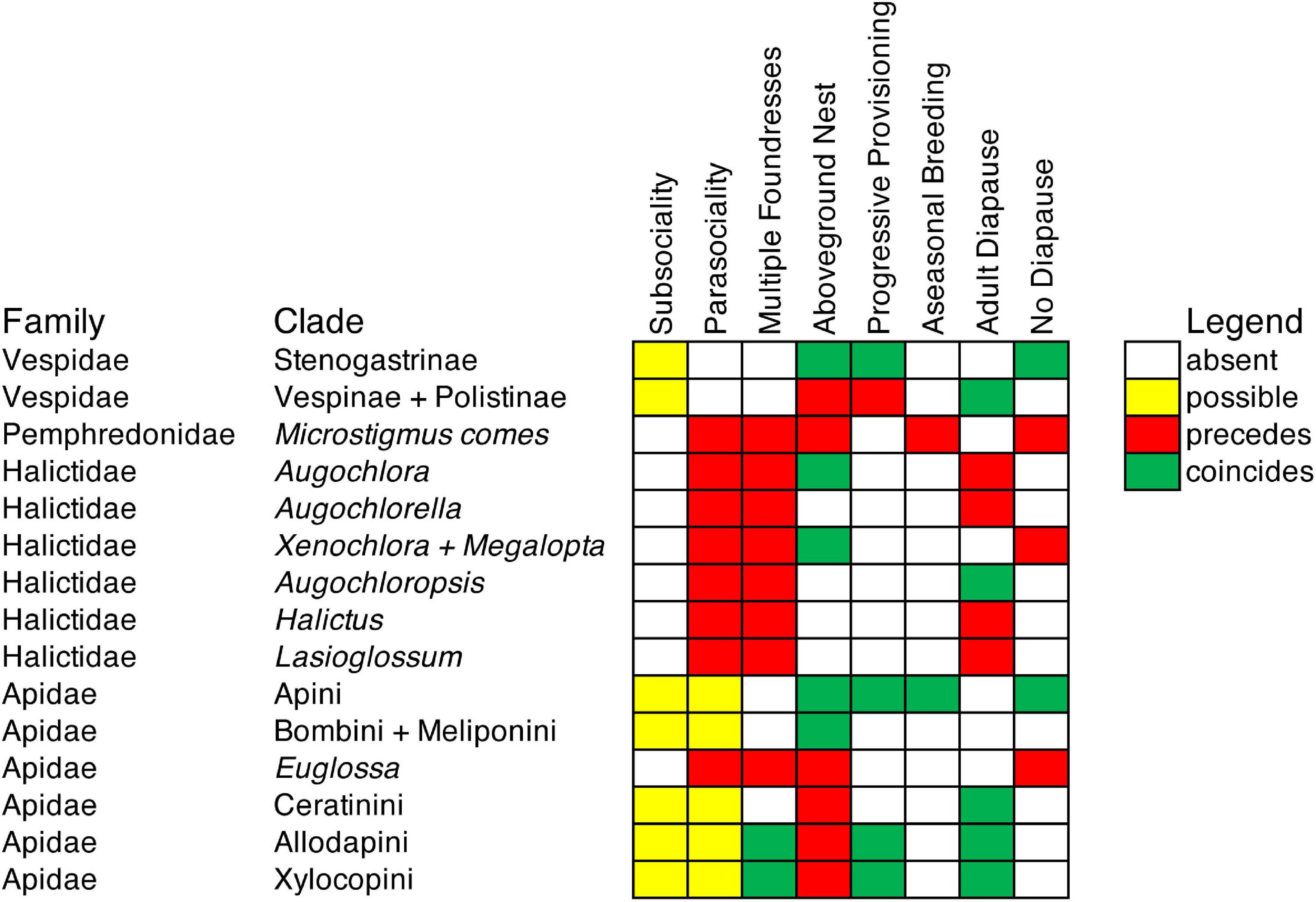
Figure 2. Summary of life history character state transitions in relation to transitions to eusociality. Derived life history character states are shown. An independent transition to eusociality occurred at the MRCA of each clade.
Vespidae
Sociality
Solitary living at the root node is consistent with the solitary parasitoid family Rhopalosomatidae as the extant sister group to the Vespidae (Branstetter et al., 2017). Ancestral state reconstruction infers that primitive eusociality arose independently in the MRCAs of the subfamilies Vespinae + Polistinae and Stenogastrinae (Figure 3). These origins descend from nodes inferred to be solitary, but would have passed through subsocial stages, as constrained by the model. This assumption is supported by the subsocial subfamily Zethinae as the sister group to the Vespinae + Polistinae. Advanced eusociality evolved once, in the MRCA of the Vespinae.
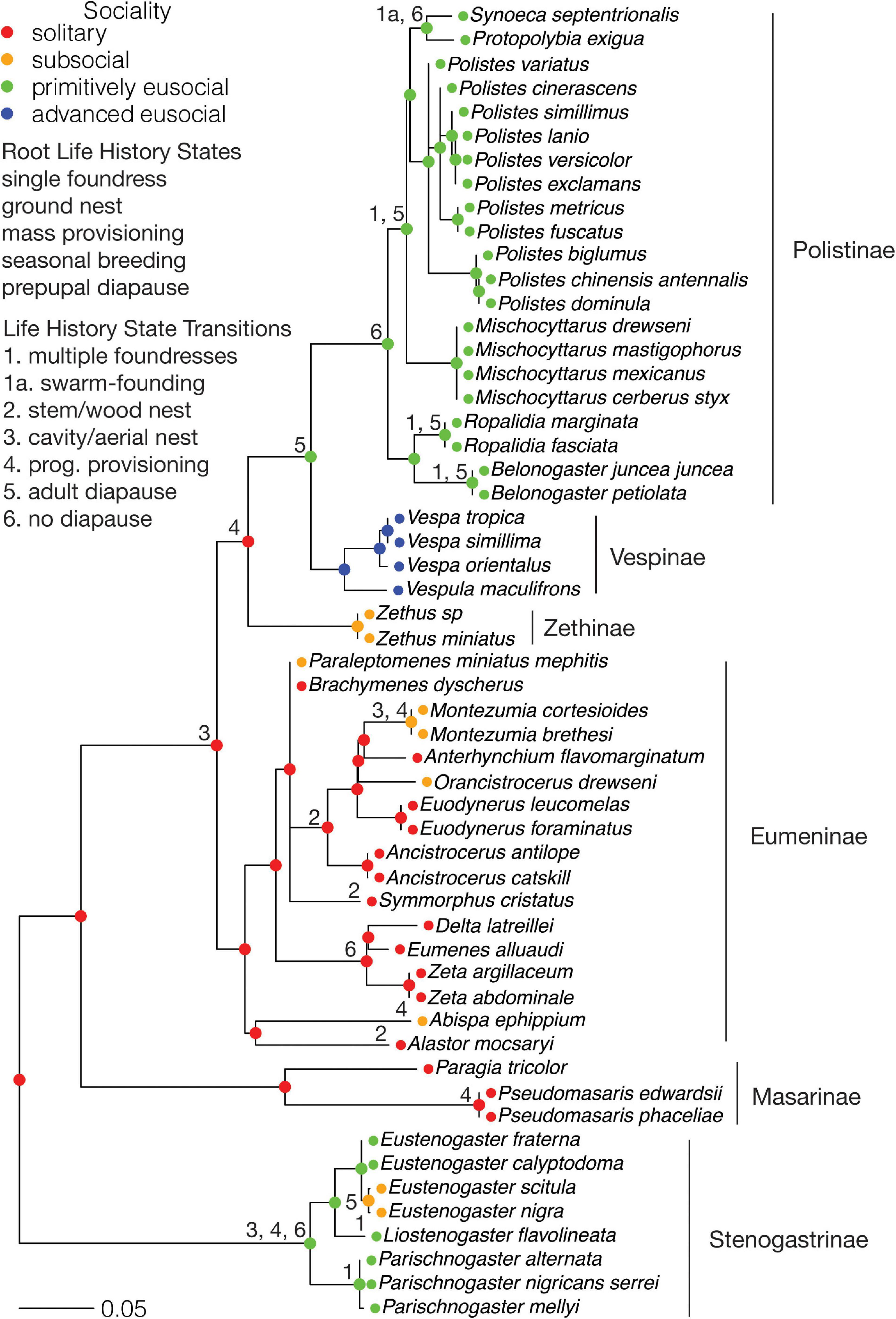
Figure 3. Vespidae: Ancestral states for sociality and transitions from the root state for each life history character. Subfamilies are indicated. The scale bar is in units of substitutions per site.
Although the swarm-founding Polistinae (Jeanne, 1991) were classified as primitively eusocial because many species do not exhibit discrete caste-specific difference in morphology, size and behavior and workers may become queens in some species (Strassmann et al., 2002; Noll and Wenzel, 2008), they could be considered as advanced eusocial because of their dependence on workers to establish new nests and because of caste-specific differences in some species. The dataset contains two swarm-founding species, Synoeca septentrionalis and Protopolybia exigua, both in the tribe Epiponini, which forms the sister group to the primitively eusocial Polistini (Polistes) (Figure 3). Reclassifying these species as advanced eusocial changes their MRCA from primitively eusocial to advanced eusocial but does not affect the state of any other node. This change also does not affect any associations with other life history characters, for which transitions from the root state precede the origin of swarming.
Life History
Both nodes at the origins of primitive eusociality maintain the ancestral state of a single nest foundress (Figure 3). Some nodes within the primitively eusocial Polistinae and Stenogastrinae are inferred to have multiple foundresses. Ground nesting was assumed to be ancestral for the Vespidae, although as the extant sister group to the Vespidae are the parasitoid Rhopalosomatidae, there is no direct supporting evidence. However, inferring the state of the root from the data supports ground nesting. The origin of primitive eusociality in the Stenogastrinae coincides with a transition to aerial nesting. However, for the origin of primitive eusociality in Vespinae + Polistinae, the node state is cavity nesting, which is retained from an earlier transition from ground nesting at the node for the MRCA of the Eumeninae + [Zethinae + (Vespinae + Polistinae)]. The primitively eusocial Polistinae have an MRCA with aerial nesting. A transition from mass brood provisioning to progressive provisioning coincides with the origin of primitive eusociality in the Stenogastrainae. Another transition to progressive provisioning occurs in the MRCA of the Zethinae + (Vespinae + Polistinae), thus preceding the origin of primitive eusociality in the Vespinae + Polistinae. The other progressive provisioners are the subsocial genera Paraleptomenes, Montezumia, and Abispa in the Eumeninae. There are no transitions from ancestral seasonal breeding that coincide with or precede the origins of eusociality, although most of the Stenogastrinae live in aseasonal environments. A reversion from primitive eusociality to subsociality in the Stenogastrinae coincides with a reversion from an aseasonal environment to a seasonal environment. Ancestral prepupal diapause is consistent with prepupal diapause in the Rhopalosomatidae (Gurney, 1953). A transition to adult diapause from prepupal diapause coincides with the origin of primitive eusociality in the Vespinae + Polistinae. A transition to no diapause from prepupal diapause coincides with the origin of primitive eusociality in the Stenogastrinae. A reversion from primitive eusociality to subsociality in the Stenogastrinae coincides with a reversion from no diapause to adult diapause, consistent with the associated reversion from an aseasonal environment to a seasonal one.
Pemphredonidae
Sociality
The state of the root was fixed as solitary living, consistent with solitary living in the subtribe Pemphredonina, which forms part of the sister group to the subtribe Spilomenina (Figure 4). The Spilomenina contain the only primitively eusocial species in the Pemphredonidae, Microstigmus comes. The other members of the Spilomenina, including the congener Microstigmus nigrophthalmus, are quasisocial. A transition from solitary living to quasisociality occurs in the MRCA of the Spilomenina. Therefore, the transition to primitive eusociality in M. comes was preceded by quasisociality and unobserved semisociality.
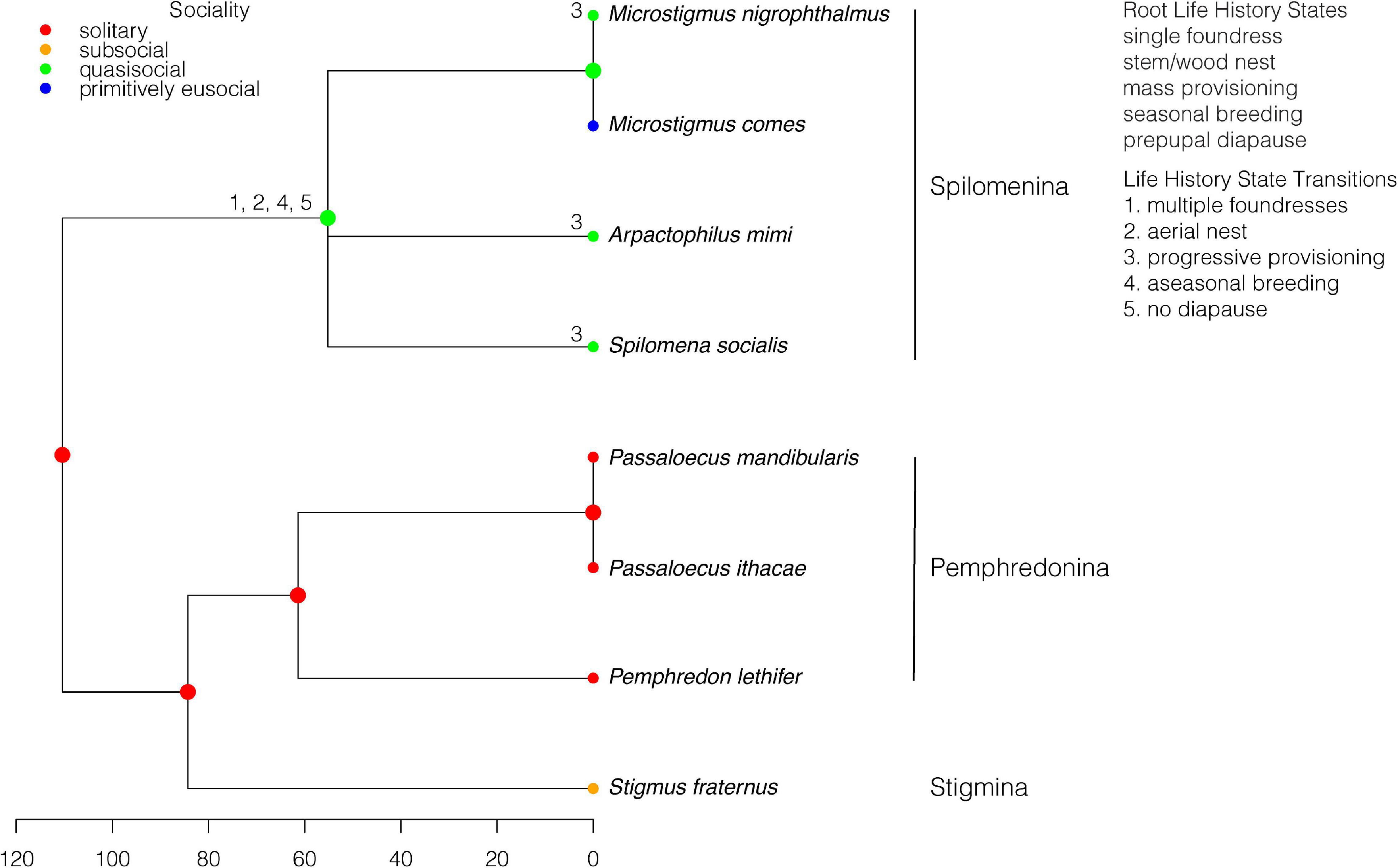
Figure 4. Pemphredonidae: Ancestral states for sociality and transitions from the root state for each life history character. Subtribes are indicated. The scale is in millions of years.
Life History
A transition to multiple foundresses coincides with the transition to quasisociality in Spilomenina, and therefore multiple founding preceded the origin of primitive eusociality (Figure 4). The stem/wood nesting of the Pemphredonina + Stigmina is supported as ancestral when the root state is inferred from the data. A transition to aerial nesting occurs at the MRCA of the Spilomenina and therefore precedes the origin of primitive eusociality. All the members of the Pemphredonina + Stigmina in this dataset are mass provisioners, supporting ancestral mass provisioning. Microstigmus comes is a mass provisioner, whereas its congener, M. nigrophthalmus, is a progressive provisioner. The other two species in the Spilomenina included in the tree are also progressive provisioners. Most internal nodes are inferred to be mass or progressive provisioning with equal probabilities. Therefore, although progressive provisioning is common in the Spilomenina, the only primitively eusocial species is a mass provisioner. A transition from ancestral seasonal breeding to aseasonal breeding occurs in the MRCA of the Spilomenina and therefore precedes the origin of primitive eusociality. A transition from ancestral prepupal diapause to no diapause occurs in the MRCA of the Spilomenina and therefore also precedes the origin of primitive eusociality.
Halictidae
Sociality
The root node for the Halictidae was fixed as solitary living, consistent with solitary living in the subfamily Rophitinae, the sister group to the remaining Halictidae [Halictinae + (Nomiinae + Nomioidinae)]. A model with two rate classes provides the best fit to the data (AIC: one class 498.97; two classes 413.35; three classes 428.56). A transition from solitary living to communal living occurs at the MRCA of the Halictinae + (Nomiinae + Nomioidinae) (Figure 5). A subsequent transition to semisociality occurs at the MRCA of the Augochlorini, followed by independent transitions to primitive eusociality in Augochlora, Augochlorella, Xenochlora + Megalopta, and Augochloropsis. The state of the MRCA of the Halictini is communal living and there are independent transitions to semisociality in the Hemihalictus series of the genus Lasioglossum and the MRCA of Halicuts + Thrincohalictus. Primitive eusociality then evolves in the MRCA of Halictus and several times in the Hemihalictus series of Lasioglossum.
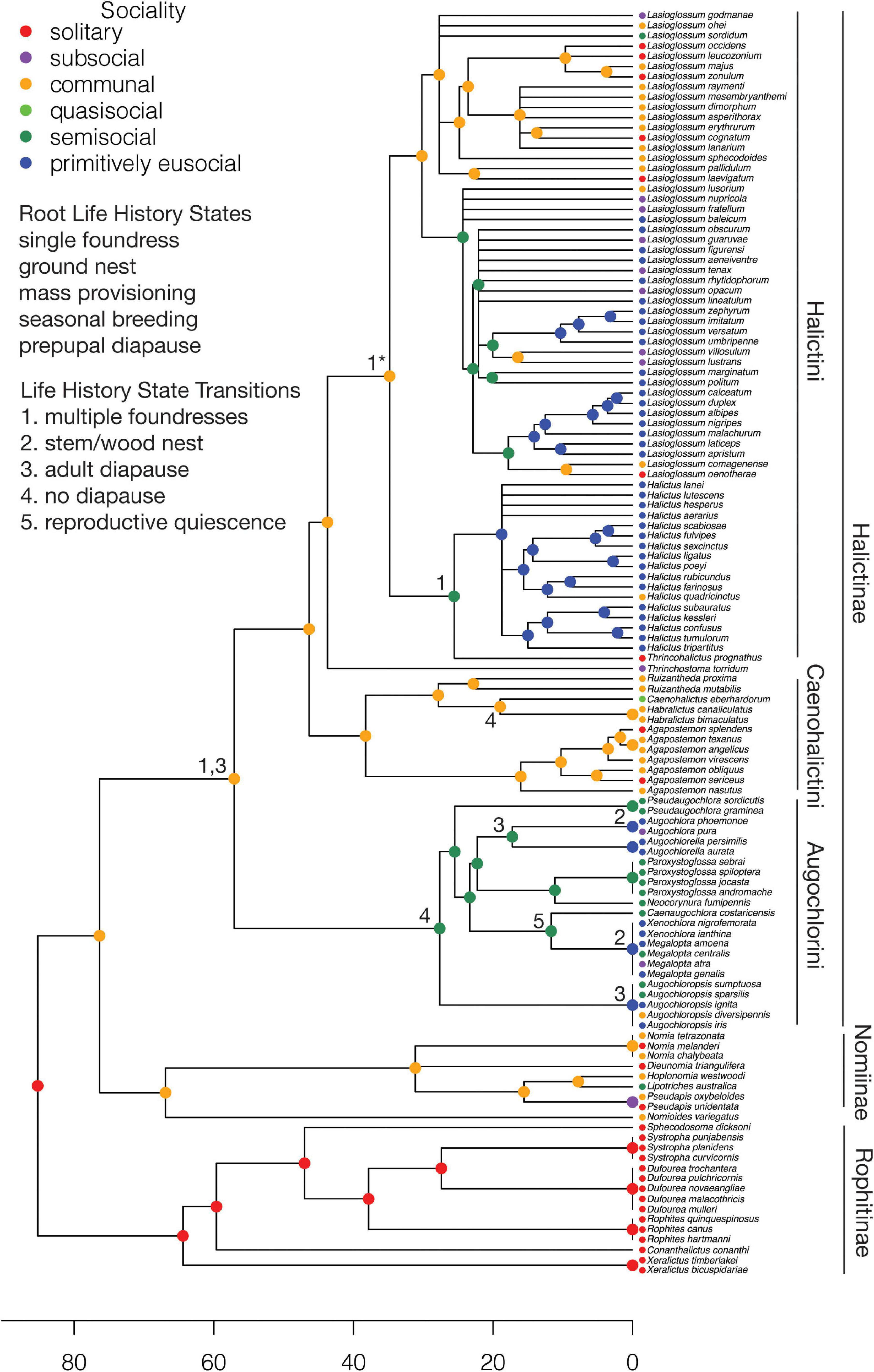
Figure 5. Halictidae: Ancestral states for sociality and transitions from the root state for each life history character. An asterisk indicates a reversion to the root state. Major subfamilies and tribes are indicated. The scale is in millions of years.
Previous studies have proposed either two origins (Danforth, 2002; Brady et al., 2006), or a single origin (Gibbs et al., 2012), of primitive eusociality in the Halictini. To test the hypothesis of a single origin, the node for the MRCA of Halictini was fixed as primitively eusocial, but this model gave a marginally poorer fit to the data than fixing the node as communal (AIC 415.87 vs. 414.98). Previous studies of the Augochlorini, based on limited data on social behavior, have proposed a single origin of eusociality within the clade and possibly one reversion to solitary living (Danforth and Eickwort, 1997; Danforth, 2002; Brady et al., 2006). The addition of newer data for this group suggests at least four independent origins of eusociality within the Augochlorini. The number of reversions from primitive eusociality within the clade cannot be determined because of polytomies.
The two rate classes (rates scaled to a maximum of 100) in the preferred model for the Halictidae correspond to low transition rates between semisociality (sem) and primitive eusociality (prim) in the Halictini (qsem→prim = 0.13; qprim→sem < 0.01) and high rates in the Augochlorini (qsem→prim = 60.99; qprim→sem = 100).
Life History
The number of foundresses could not be reconstructed accurately; most nodes were inferred to have nearly equal probabilities of single and multiple foundresses, suggesting that this character is very labile. Nevertheless, a transition from ancestral single founding to multiple founding is inferred for the MRCA of the subfamily Halictinae, preceding the origins of eusociality in the subfamily (Figure 5). Nearly all species are ground nesters. Transitions from ancestral ground nesting to stem/wood nesting occur in some lineages of the Augochlorini that evolve eusociality: Augochlora and Xenochlora + Megalopta. All species are mass provisioners. Nearly all species have seasonal breeding. The root node was fixed as prepupal diapause, consistent with prepupal diapause in the Rophitinae. A transition from ancestral prepupal diapause to adult diapause is inferred for the MRCA of the Halictinae, thus preceding the origins of eusociality in the subfamily. However, there is a transition to no diapause in the MRCA of the Augochlorini followed by reversions to adult diapause in the MRCAs of Augochlora + Augochlorella and Augochloropsis and a transition to reproductive quiescence in the MRCA of the clade Caenaugochlora + (Xenochlora + Megalopta), all of which contain lineages that evolved eusociality.
Apidae
The subfamilies Apinae and Xylocopinae were analyzed separately since eusociality appears to have evolved independently in them and advanced eusociality is exclusive to the Apinae (Danforth et al., 2013). In addition, transition rates, including reversions, between character states may be significantly different between the two subfamilies. Frequent gains and losses of primitive eusociality have been inferred for the Xylocopinae (Wcislo and Danforth, 1997; Rehan et al., 2012).
Apinae
Sociality
The root node was fixed as solitary, consistent with the mostly solitary tribe Centridini, the sister group to the remaining Apinae. Eusociality is inferred to have evolved at least three times (Figure 6). Primitive eusociality evolved at least once in the genus Euglossa (tribe Euglossini) from a node with communal living, and therefore through unobserved semisociality. Primitive eusociality also evolved in the MRCA of the tribes Meliponini + Bombini, with advanced eusociality evolving in the MRCA of the Meliponini. This transition to primitive eusociality occurred from a node with solitary living and therefore is assumed to have passed through either subsociality or semisociality. Advanced eusociality also evolved in the MRCA of the tribe Apini. This transition is from a node with solitary living and is therefore assumed to have passed through either subsociality or semisociality and then primitive eusociality. To test whether there is a single origin of eusociality for the exclusively eusocial Apini + (Bombini + Meliponini), the MRCA for this clade was fixed as primitively eusocial, but this model gave a poorer fit to the data than when the node was fixed as solitary (AIC 197.46 vs. 195.05). Eusociality has also been inferred to have evolved in the MRCA of the corbiculate Apinae (Euglossini, Apini, Bombini, and Meliponini) (Cardinal and Danforth, 2011). This hypothesis was tested by fixing the MRCA of the corbiculates as primitively eusocial, but this model also gave a poorer fit to the data than fixing the node as solitary (AIC 201.36 vs. 194.21). Therefore, eusociality evolved twice in the Apini + (Bombini + Meliponini) and advanced eusociality evolved independently in the Meliponini and the Apini.
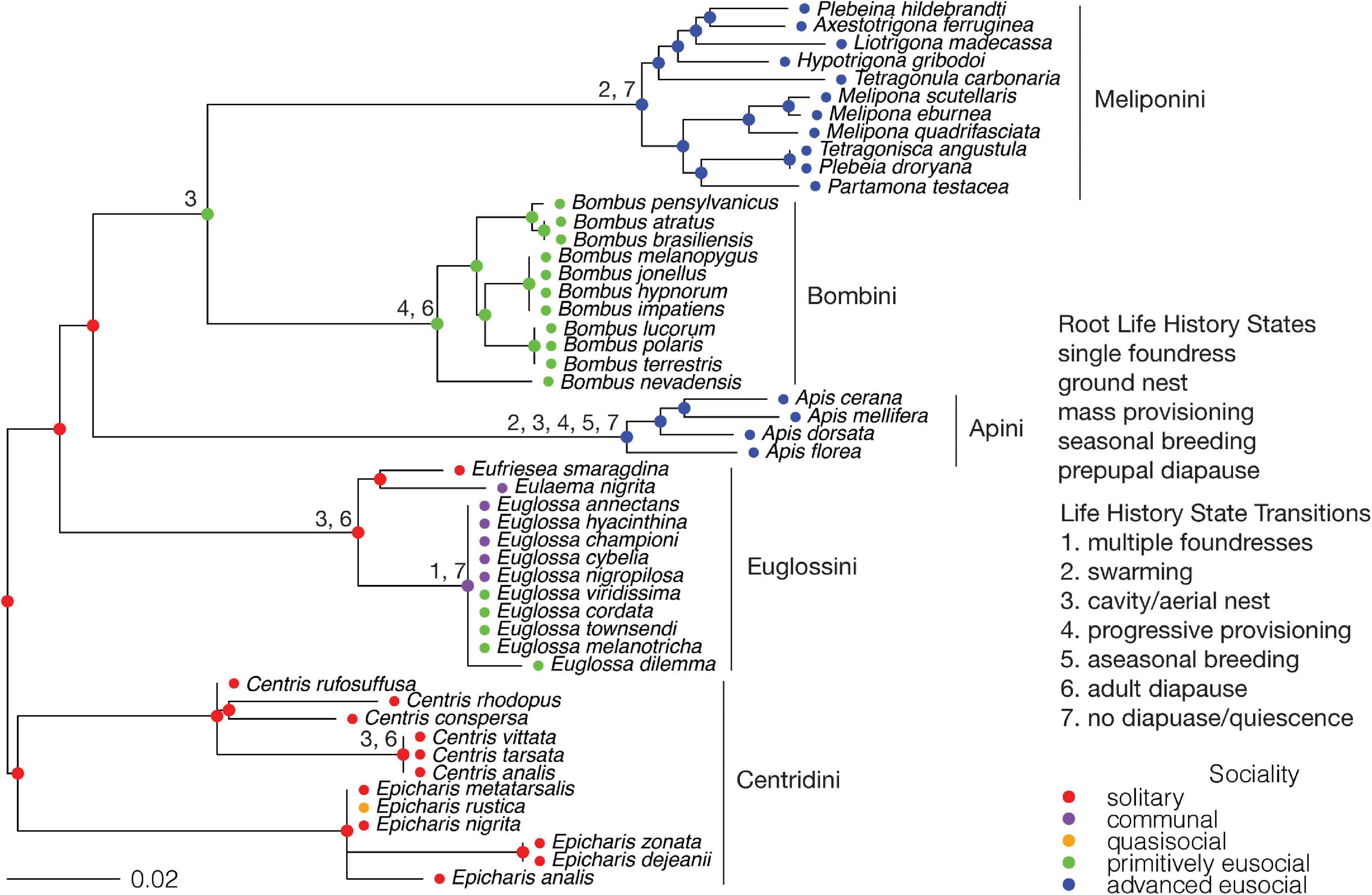
Figure 6. Apinae: Ancestral states for sociality and transitions from the root state for each life history character. Tribes are indicated. The scale bar is in units of substitutions per site.
There have been suggestions that the non-parasitic Bombus (bumblebees) be reclassified as advanced eusocial, rather than primitively eusocial as they are classified here, on the basis of various criteria (Crespi and Yanega, 1995; Kocher and Paxton, 2014; Boomsma and Gawne, 2018; Richards, 2019; Holland and Bloch, 2020). This reclassification has the effect of changing the MRCA of the Meliponini + Bombini from primitively eusocial to advanced eusocial. However, the MRCA of the Apini + (Bombini + Meliponini) remains solitary, and therefore the conclusion of two origins of eusociality in the Apini + (Bombini + Meliponini) does not change.
Life History
The state of the root for the number of foundresses was fixed as single, consistent with a solitary ancestor. Separate transitions from solitary founding to swarming coincide with both origins of advanced eusociality, supporting two origins of advanced eusociality (Figure 6). The primitively eusocial Bombini mostly retain ancestral single founding. A transition to multiple founding from single founding preceded the origin of primitive eusociality in the Euglossini. The state of the root for nest type was fixed as ground nesting, consistent with the mostly ground nesting Centridini. Cavity and aerial nesting were merged into a single state, cavity/aerial nesting. Separate transitions from ground nesting to cavity/aerial nesting coincide with the two origins of eusociality in the Meliponini + Bombini and Apini. A transition from ground nesting to cavity/aerial nesting occurs at the MRCA of the Euglossini, and thus cavity/aerial nesting precedes the origin of primitive eusociality in Euglossa. The state of the root for brood provisioning was fixed as mass provisioning, consistent with mass provisioning in the Centridini. A transition from mass provisioning to progressive provisioning occurs in the MRCA of the Apini, coinciding with this origin of advanced eusociality. A transition from mass provisioning to progressive provisioning also occurs in the MRCA of the primitively eusocial Bombini, while the advanced eusocial Meliponini retain ancestral mass provisioning. Therefore, the Apini and Bombini evolved progressive provisioning independently. The state of the root for breeding seasonality was fixed as seasonal. Most species live in a seasonal environment. A transition from seasonality to aseasonality occurs at the MRCA of the advanced eusocial Apini. The state of the root for diapause was fixed as prepupal, consistent with prepupal diapause being common in the Centridini. A transition from prepupal to no diapause coincides with the origin of advanced eusociality in the MRCA of the Apini. The state of the MRCA of the Meliponini + Bombini is prepupal diapause, which coincides with the origin of primitive eusociality for this clade. There is a transition to adult diapause for the MRCA of the Bombini and reproductive quiescence for the MRCA of the Meliponini. A transition from prepupal to adult diapause occurs in the MRCA of the Euglossini and is followed by a transition to no diapause in Euglossa, which thus precedes the evolution of eusociality in this group.
Xylocopinae + Eucerinae
Sociality
The subfamilies Xylocopinae + Eucerinae form the sister group to the Apinae. The state of the root for the Xylocopinae + Eucerinae was fixed as solitary, consistent with the mostly solitary Eucerinae. Transitions from solitary living to primitive eusociality (through subsociality or semisociality) occur for the MRCAs of the tribes Ceratinini, Allodapini, and Xylocopini of the Xylocopinae (Figure 7). In each of these tribes there are reversals to presociality and parasociality. Therefore, primitive eusociality evolved independently in the Ceratinini, Allodapini, and Xylocopini. A previous study suggested that sociality, defined broadly to cover all forms of sociality, evolved once in the Xylocopinae (Rehan et al., 2012). To test the hypothesis that eusociality evolved once in the Xylocopinae and was subsequently lost in some lineages, the state of the MRCA of the clade (Ceratinini + Allodapini) + (Xylocopini + Manueliini) was fixed as primitively eusocial, but this gave a poorer fit to the data than fixing the node as solitary (AIC 236.38 vs. 230.17).
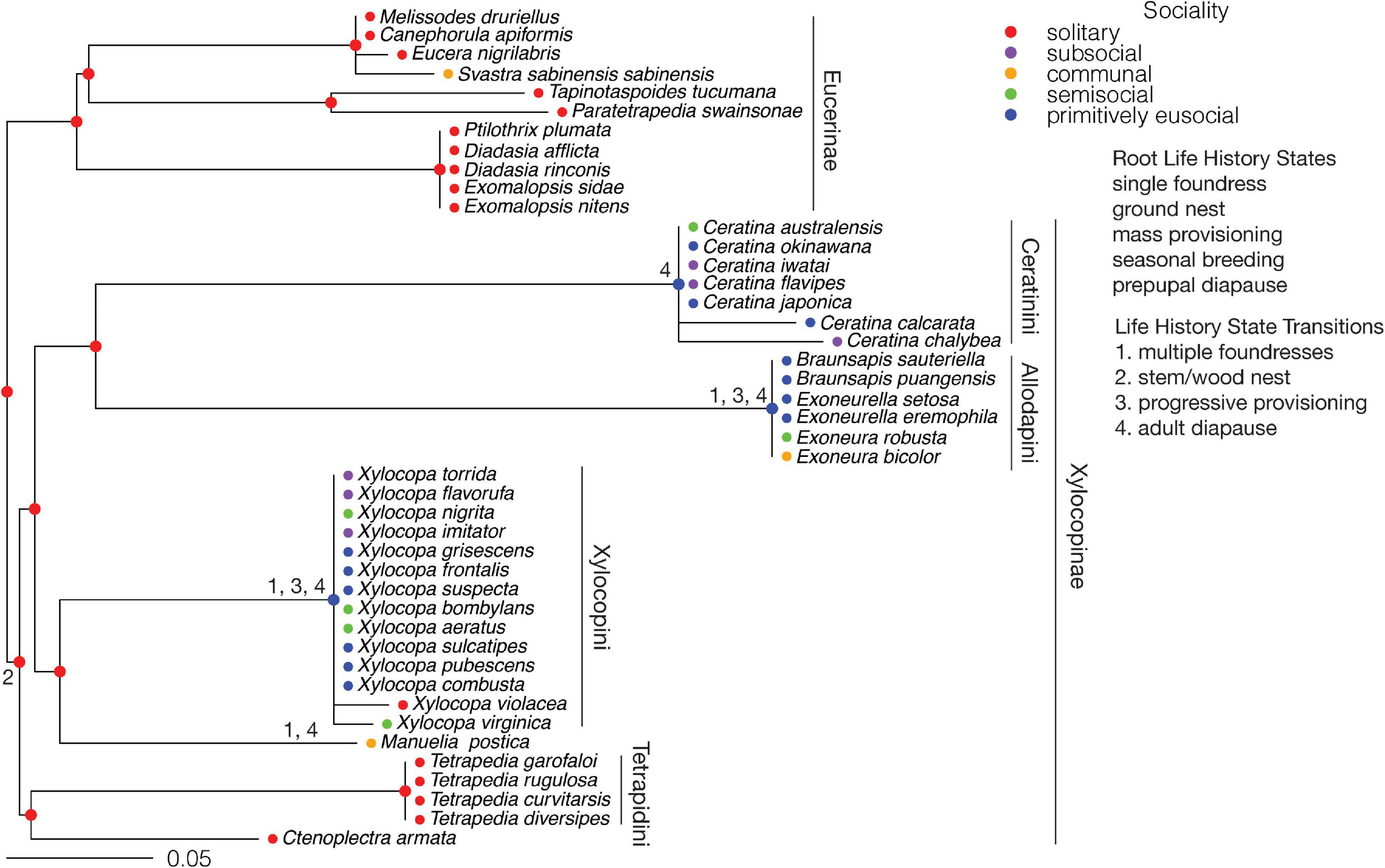
Figure 7. Xylocopinae and Eucerinae: Ancestral states for sociality and transitions from the root state for each life history character. Major tribes of the Xylocopinae are indicated. The scale bar is in units of substitutions per site.
Life History
The state of the root for the number of foundresses was fixed as single, consistent with ancestral solitary living. A transition from a single foundress to multiple foundresses coincides with each of the transitions to primitive eusociality in the MRCAs of the Allodapini and Xylocopini (Figure 7). The state of the root for nest type was fixed as ground nesting, consistent with ground nesting in the Eucerinae. A transition to stem/wood nesting from ground nesting occurs in the MRCA of the Xylocopinae, and therefore precedes the origins of eusociality within this subfamily. The state of the root for brood provisioning was fixed as mass provisioning, consistent with mass provisioning in the Eucerinae. A transition from mass provisioning to progressive provisioning coincides with each of the transitions to primitive eusociality in the MRCAs of the Allodapini and Xylocopini. All species live in seasonal environments. The state of the root for diapause was fixed as prepupal, consistent with prepupal diapause in the Eucerinae. Transitions to adult diapause from prepupal diapause occur at the MRCAs of the Ceratinini, Allodapini, and Xylocopini. Therefore, transitions to adult diapause coincide with all three transitions to eusociality.
Statistical Tests of the Dependence of Eusociality on Life History
Tests for the dependence of sociality on each life history character, using binary versions of the characters, were carried out for each family except the Pemphredonidae, which contain a single eusocial species. The effect of diapause on the evolution of eusociality was tested in three ways to account for: (1) a loss of prepupal diapause, (2) a loss of diapause altogether, and (3) the effect of adult diapause on its own. After correcting for multiple comparisons within the family, none of the life history characters was significantly associated with sociality in the Vespidae (P > 0.3; Table 3). For the Halictidae, nest type and form of diapause have highly significant associations with sociality. For nest type, the transition from non-eusociality to primitive eusociality occurs at a much higher rate when nests are in a stem or wood (q = 8523.124) than when they are in the ground (q = 0.002) (P = 1.705 × 10–10). For diapause, primitive eusociality evolves only after prepupal diapause has been lost (P = 2.214 × 10–11). For the Apidae, nest type, brood provisioning, and diapause are significantly associated with sociality. For nest type, eusociality evolves only when nests are aboveground (P = 0.0132), with the effect being due to cavity or aerial nesting (P = 0.0008). For brood provisioning, eusociality evolves at a higher rate with progressive provisioning (q = 29.57) than with mass provisioning (q = 1.042) (P = 0.0105). For diapause, eusociality evolves only after the loss of prepupal diapause (P = 0.0132), due to transitions to adult diapause (P = 0.0250).
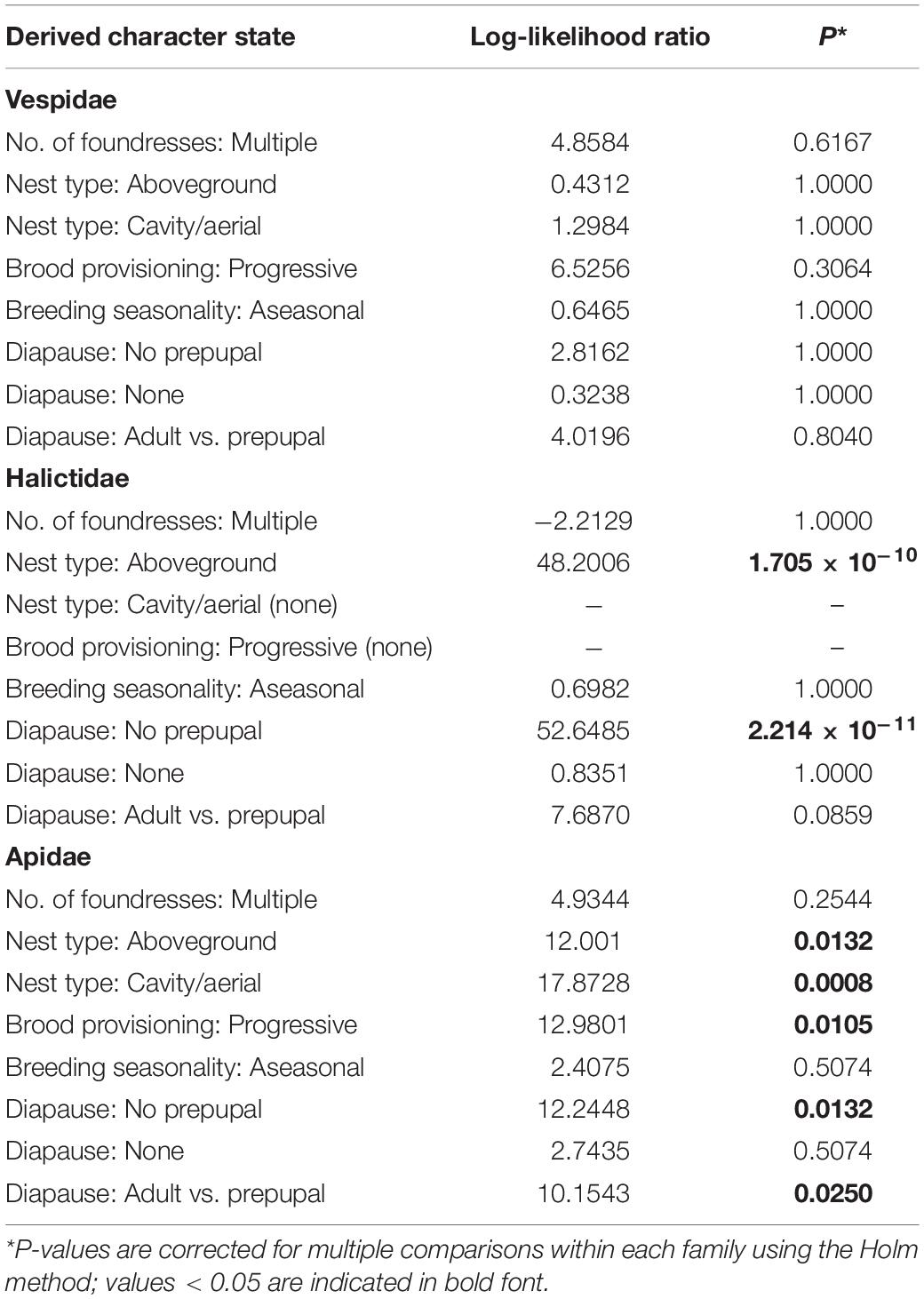
Table 3. Tests for the dependence of eusociality on binary life history characters within each family using Pagel’s method.
Discussion
Transitions to Eusociality
Eusociality is estimated here to have originated at least 15 times across the aculeate Hymenoptera, not counting a single origin in the ants (Figure 2). The two origins of primitive eusociality in the Vespidae, in the Stenogastrinae and the Vespinae + Polistinae, are consistent with previous analyses (Hines et al., 2007; Piekarski et al., 2018). Primitive eusociality is inferred to have arisen at least six times in the Halictinae: at least twice in the Halictini (Halictus and the Hemihalictus series of Lasioglossum) and four times in the Augochlorini. Previous studies have proposed either two origins (Danforth, 2002; Brady et al., 2006), or a single origin (Gibbs et al., 2012), of primitive eusociality in the Halictini and a single origin of primitive eusociality within the Augochlorini (Danforth and Eickwort, 1997; Danforth, 2002; Brady et al., 2006). The several independent origins of eusociality in the Augochlorini inferred in this study are the result of the addition of more recent data on social behavior. The Augochlorini exhibit much higher rates of transition to and from eusociality than the Halictini. In the Apinae, eusociality is inferred to have evolved three times, twice in the Apini + (Bombini + Meliponini) and at least once in Euglossa, with advanced eusociality evolving independently in the Apini and Meliponini. Based on a tree topology that is no longer supported, a previous analysis inferred a single origin of eusociality in the Apinae (Cardinal and Danforth, 2011). Two origins of advanced eusociality in the Apinae are consistent with previous analyses (Winston and Michener, 1977; Cameron, 1993; Cardinal and Danforth, 2011). In the Xylocopinae, primitive eusociality is inferred to have evolved independently in each of the Ceratinini, Allodapini, and Xylocopini. A previous study proposed a single origin of eusociality for the Allodapini (Schwarz et al., 2006). However, a more recent study proposed that sociality, defined broadly, evolved once in the Xylocopinae and was followed by reversions to non-social living (Rehan et al., 2012). In the present study, the MRCA of the Xylocopinae is inferred to be solitary. This discrepancy may reflect the more recent and accurate data on sociality and phylogeny in the present study. Frequent gains and losses of primitive eusociality had been inferred for the Xylocopinae (Wcislo and Danforth, 1997; Rehan et al., 2012).
Routes to Eusociality and Life History
To summarize the routes to eusociality and the associations of life history character states with transitions to eusociality determined through ancestral state reconstruction, the derived states subsociality, parasociality, multiple founding of nests, aboveground nesting, progressive brood provisioning, aseasonal breeding, adult diapause, and no diapause were each classified as being absent, possibly present but unobserved, preceding or coinciding with respect to each origin of eusociality (Figure 2). A state may be possibly present in the case of subsociality or parasociality when a transition occurs from solitary living to eusociality on a phylogeny because it is assumed to have passed through a subsocial or semisocial stage that has not been observed. Parasociality is observed to precede or possibly precede 13 of the 15 origins of eusociality, suggesting that semisociality provides a common route to eusociality. The loss of prepupal diapause, in the form of either adult diapause or no diapause, precedes, or coincides with, all except one origin of eusociality.
A semisocial route to eusociality is consistent with a subsocial route since all semisocial species appear to also be subsocial (Lin and Michener, 1972; Alexander et al., 1991; Cowan, 1991). It is also consistent with eusocial lineages being ancestrally monogamous since monogyny is often functional, involving multiple foundresses but with only one foundress responsible for most or all of the egg laying; functional monogyny is ancestral for eusocial vespid wasps, ants, and corbiculate bees (Hughes et al., 2008). A semisocial route to eusociality has been proposed previously and may remain evident as a semisocial stage in the lifecycle of many primitively eusocial species (Lin and Michener, 1972).
Interestingly, a semisocial stage in the evolution of eusociality could resurrect a causal link between haplodiploidy and eusociality (Hamilton, 1964b). Haplodiploidy is not currently considered to favor eusociality because although it increases the genetic relatedness between full sisters it also reduces the relatedness between sisters and brothers, resulting in no advantage of haplodiploidy when the sex ratio of reproductive siblings is even (Trivers and Hare, 1976). However, subordinate, worker-like co-foundresses in semisocial groups of full sisters may experience indirect fitness benefits by raising nieces and nephews, and these are more closely related to them under haplodiploidy than under diploidy. This argument has been made in passing (Trivers and Hare, 1976; West-Eberhard, 1978; Maynard Smith, 1989, p. 178; Pamilo, 1991; Bourke and Franks, 1995, p. 84) but does not appear to have been developed further. This argument depends on co-foundresses being closely related, which they are in primitively eusocial paper wasps (Strassmann et al., 1989; Ross and Carpenter, 1991), hover wasps (Turillazzi, 2012), sweat bees (Brand and Chapuisat, 2016), carpenter bees (Schwarz, 1987; Hurst et al., 1997; Hogendoorn and Velthuis, 1999), and orchid bees (Andrade et al., 2016; Freiria et al., 2017). The conditions that favor multiple females founding a nest may include higher nest survival (Queller, 1996b; Itô and Kasuya, 2005), higher per capita reproduction (Bartz and Hölldobler, 1982; Schwarz, 1988), assured fitness returns for the dominant co-foundress (Lucas and Field, 2011), or subordinate co-foundresses (Gadagkar, 1990; Shreeves et al., 2003) and the opportunity for nest inheritance by subordinate co-foundresses (Leadbeater et al., 2011).
Another interesting consequence of a semisocial stage in the transition to eusociality is that if semisocial groups have a higher number of offspring per capita, the result of local resource enhancement (Schwarz, 1988), then females may have higher a reproductive value than males, which may select for a female-biased sex ratio (West, 2009). This in turn favors eusociality under haplodiploidy since helpers then help to raise closely related sisters disproportionately. Semisociality may also be a preadaptation to the mutual tolerance and reproductive altruism necessary for primitive eusociality (Lin and Michener, 1972).
The Vespidae are an exception in that there is no evidence of parasociality preceding, or coinciding with, their two origins of eusociality (Piekarski et al., 2018; Figure 2), although multiple nest foundresses are common in the paper wasps (Polistinae). The eusocial Vespidae, however, exhibit progressive brood provisioning exclusively, in contrast to several other eusocial clades across the Hymenoptera that are mass provisioners. A possible explanation is that multiple founding and progressive provisioning are alternative strategies for dealing with scarce larval food. When food is scarce, having multiple foundresses may increase the chances of adequately mass provisioning the first brood. Alternatively, a single foundress would have to provision progressively to meet the demands of her first brood. Some solitary eumenine vespid wasps may practice mass provisioning when prey is abundant but shift to progressive or delayed provisioning when prey is scarce (Evans, 1977; Cowan, 1991). This may explain the evolution of progressive provisioning (O’Neill, 2001). Whichever strategy is used, food scarcity would also favor a female-biased first brood if they could help raise a second brood. Both progressive provisioning and multiple founding may also select for an extended foundress lifespan, which could overlap that of her first brood and thus permit eusociality to evolve (da Silva, in press). Progressive provisioning may select for an extended foundress lifespan because it extends parental care. Multiple founding may do so because the subordinate co-foundresses do most of the risky foraging, thereby reducing the extrinsic mortality of the dominant foundress (Metcalf and Whitt, 1977; Strassmann et al., 1984; Garofalo, 1985; Giannotti and Machado, 1994; Shreeves and Field, 2002; Itô and Kasuya, 2005). Perhaps an impetus for eusociality was scarcity of larval food.
These roles for progressive provisioning and multiple founding may explain clades of eusocial species that are exclusively mass provisioning, but with multiple founding or swarming, such as stingless bees (Meliponini) and some clades of sweat bees (Halictinae), while other clades are exclusively progressive provisioning, but with single founding, such as bumblebees (Bombini), and vespine wasps (Vespinae) (Figure 2). Most other eusocial clades contain species that combine progressive provisioning and multiple founding: clades of paper wasps (Polistinae), honeybees (Apini), and some clades of carpenter bees (Xylocopinae). The combination of strategies may be common because multiple founding reduces the dependence of the first brood on a single progressively provisioning female over the long provisioning period (Field, 2005).
Life History Dependencies
A more rigorous assessment of the association of sociality and life history characters involved testing for dependence of the transition from non-eusociality to eusociality on transitions in binary life history characters. These tests were done for each family separately to allow for differences in natural history and life history between families. No significant dependencies were observed for the Vespidae, very likely because there are only two transitions to eusociality in this family. The strongest dependencies were identified in the Halictidae, for aboveground nesting and a loss of prepupal diapause. The Apidae also showed these dependencies in addition to a dependency on progressive brood provisioning. Progressive provisioning is discussed above and the loss of prepupal diapause is discussed below. An aboveground nest may favor eusociality because it provides a greater opportunity to expand the nest and therefore allows greater capitalization of the efforts of workers (Alexander et al., 1991; Matthews, 1991). Alternatively, an aboveground nest may require the help of workers for its defense. However, this is unlikely to be important since nest defense is associated with the origin of workers of both sexes in other eusocial taxa, while the origin of exclusively female workers in the Hymenoptera is explained by their primary role in alloparental care (Ross et al., 2013).
The Loss of Prepupal Diapause
The loss of prepupal diapause precedes, or coincides with, all but one transition to eusociality identified by ancestral state reconstruction and is a statistically significant predictor of transitions to eusociality in the Halictidae and Apidae. The only exception to this pattern is the transition to primitive eusociality in the MRCA of the Bombini + Meliponini, which retains ancestral prepupal diapause (Figure 2). Prepupal diapause is, however, subsequently lost in the MRCAs of the Bombini (adult diapause) and Meliponini (reproductive quiescence). This sequence of events may suggest that either the assignment of prepupal diapause to the MRCA of the Bombini + Meliponini is incorrect or that eusociality evolved independently in the Bombini and Meliponini. The latter possibility would explain the very different life histories of these two groups, with the Bombini having single foundresses and progressive provisioning, while the Meliponini exhibit swarming and retain ancestral mass provisioning.
In a recent phylogenetic comparative study, Santos et al. (2019) report that a loss of prepupal diapause is associated with nearly every origin of sociality in bees, with sociality defined as subsociality, parasociality, or eusociality. The present study shows that this applies to most transitions to eusociality specifically. The authors of the previous study argue that this pattern supports the “Seger hypothesis,” that eusociality is favored by a female bias in the sex ratio of reproductive offspring in the foundress’ second brood or the second generation of offspring, caused by first-brood males mating with both first-brood females and second-brood/generation females before these enter diapause (Seger, 1983). The logic is that because of haplodiploidy, female workers will experience higher indirect fitness by helping to raise a female-biased second brood/generation.
However, there does not appear to be any evidence in support of the Seger hypothesis. In particular, the limited number of cases of sex ratio differences between broods/generations have many other possible explanations (Cowan, 1991; O’Neill, 2001; West, 2009), there is no evidence that males mate across broods/generations (Yanega, 1996), the requirement for mated adult diapause does not explain the evolution of eusociality in carpenter bees (Xylocopinae), which have unmated adult diapause (Danforth et al., 2019), and the occurrence of sex ratio differences between broods/generations and between nests is deemed too rare to have had any effect on the evolution of eusociality in the Hymenoptera (Gardner et al., 2012).
A simpler explanation for the strong association between a loss of prepupal diapause and the evolution of eusociality reported here is that eusociality evolves via the subsocial route and subsociality is defined as females interacting with their adult offspring. Thus, a loss of prepupal diapause is simply a prerequisite for subsociality. Indeed, bee species with prepupal diapause are solitary and not closely related to any eusocial lineages (Danforth et al., 2019). In contrast, as shown in this and other studies (Danforth et al., 2019; Santos et al., 2019), all subsocial bees exhibit adult diapause or no diapause. Similarly, wasps with prepupal diapause appear to be exclusively solitary (Fye, 1965; Brockmann and Grafen, 1992), whereas subsocial species exhibit adult or no diapause.
Life cycles in which daughters may either enter prepupal diapause or complete development and breed in the same season appear to be bet-hedging strategies in unpredictable environments (Fye, 1965; Brockmann and Grafen, 1992; Danforth et al., 2019). Prepupal diapause is especially common in solitary bees living in arid environments, likely because prepupae are resistant to desiccation, permitting them to remain in diapause for a considerable amount of time, sometimes for several years (Danforth et al., 2019). In more predictable and less arid environments, females may be selected to complete development with the option of entering diapause as adults. One advantage of adult diapause is that it permits females to begin nesting earlier in the next season than females that had entered diapause as prepupae and must first complete development (Matthews, 1991; Brockmann, 1997; O’Neill, 2001; Danforth et al., 2019). Earlier emergence from adult diapause in the solitary mining bee Andrena vaga is associated with a longer lifespan (Straka et al., 2014). This scenario may favor eusociality if it permits the production of two broods, thus allowing first-brood females the opportunity to raise second-brood reproductive siblings. There are several species of bees that are primitively eusocial at low latitudes or elevations, where the length of the breeding season permits the production of two broods, but are otherwise subsocial (Purcell, 2011).
In addition, with adult diapause, if a first-brood daughter has no opportunity to breed either in her natal nest or independently, because of a high cost of independent breeding, and thus her only options are to enter diapause or to help raise the second brood, the selective barrier to helping is extremely low (Figure 8). This univoltine lifecycle is observed in paper wasps (Polistinae) and sweat bees (Halictinae) (Yanega, 1988; Reeve et al., 1998; Schwarz et al., 2007). In this situation, a first-brood daughter is in a genetic sense simply trading off herself against her contribution to the current production of full siblings in the second brood; she would have to help produce only two full siblings to come out even with an even sex ratio because each sibling is related to her by one half on average. In other words, the cost of helping in terms of personal reproduction is simply the female’s genome; her future reproduction, had she opted to enter diapause, is not relevant since any siblings she helps to produce may also breed in the future. This conclusion was derived by Pamilo (1991) using a formal inclusive fitness model, but has apparently not attracted any attention. The idea is supported by the observation that in vespid wasps and bumblebees the ontogeny of gynes, but not workers, is guided toward an adult diapause phenotype (Hunt and Amdam, 2005; Hunt et al., 2007; Amsalem et al., 2015; Piekarski et al., 2018). The idea is also supported by what may represent a step on the path to full eusociality: brood divalency in the sweat bee Halictus rubicundus, in which some first-brood females become workers while others mate and enter diapause (Yanega, 1988). The hypothesis that adult diapause favors eusociality in this manner could potentially be tested in the Halictinae, which exhibit considerable inter- and intra-specific variation in life cycles and social systems that is often associated with season length (Yanega, 1997; Schwarz et al., 2007; Field et al., 2010; Purcell, 2011; Kocher et al., 2018).
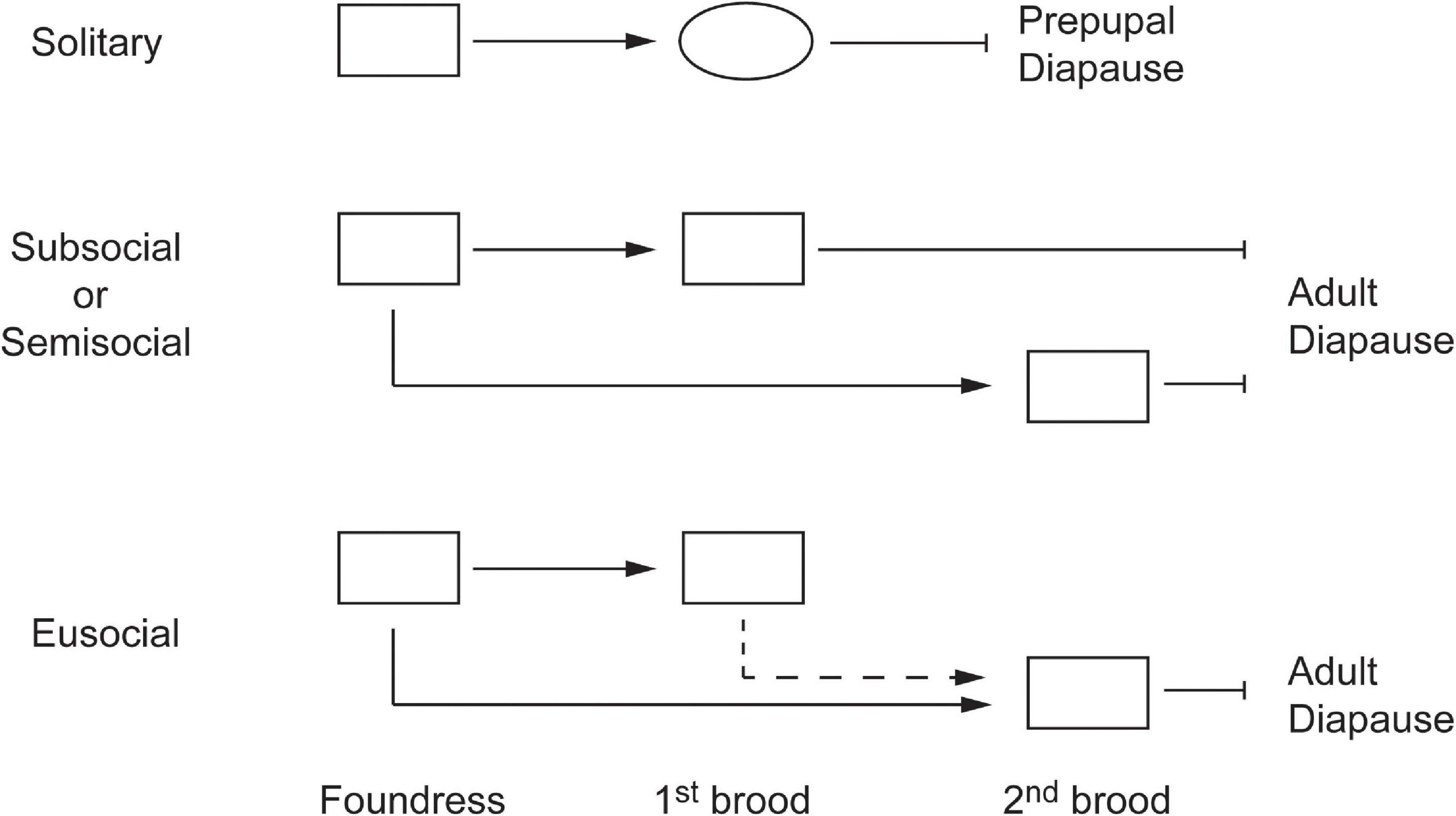
Figure 8. Schematic diagrams of life cycles with prepupal and adult diapause. Adults are represented by rectangles and prepupae are represented by an oval. Solid arrows indicate breeding, and the dashed arrow indicates helping. The distinction between subsociality and semisociality is whether there is a single foundress (subsocial) or multiple foundresses (semisocial).
Obligate Eusociality Is a Major Evolutionary Transition
A major evolutionary transition is a change in the way genetic information is transmitted between generations (Maynard Smith and Szathmáry, 1995). Such transitions help explain the hierarchical nature of living systems, including the origins of new levels of individuality, such as unicells, eukaryotic cells, multicellular organisms, and eusocial societies (Bourke, 2011; West et al., 2015). Therefore, to qualify as a major evolutionary transition, eusociality must be obligate, in the sense that reproduction cannot occur outside the social group, thus defining the social group as an individual or superorganism. Boomsma and Gawne (2018) suggest defining such groups by workers remaining unmated throughout their lives, which is associated with distinct morphological differences between queens and workers. This allows for worker production of males. I believe this is insufficient, however, since it leaves open the possibility that a foundress may reproduce independently of workers. Crespi and Yanega (1995) define obligate eusociality as a lack of totipotency of both the reproductive and non-reproductive castes, making castes mutually dependent, thus creating a new level of individuality. Crespi and Yanega (1995) define castes by the commitment of individuals throughout adulthood to a behavioral role, usually involving a distinct morphology, in order to distinguish eusociality from cooperative breeding, in which individuals, usually offspring, act as helpers for only their early adult years. The requirement for commitment to a caste by Crespi and Yanega (1995) and Boomsma and Gawne (2018) is motivated by the analogy with multicellular organisms, with their distinct germ line and soma. However, then this requirement seems too stringent since in both animals and plants reproduction sometimes occurs through somatic tissue. For example, cnidarians such as Hydra, may reproduce by budding, and plants commonly reproduce vegetatively.
If we disregard commitment to caste, then the swarm-founding Polistinae (paper wasps) (Jeanne, 1991), which rely on workers to establish new nests, but in which workers may become queens (Strassmann et al., 2002), are nevertheless obligately eusocial and thus would meet the criteria for a new level of individuality. On this basis, in addition to the swarm-founding Polistinae, the non-parasitic bumblebees, stingless bees, honeybees, non-parasitic hornets, and yellowjackets and most ants are obligately eusocial (Crespi and Yanega, 1995; Boomsma and Gawne, 2018). Thus, in the Hymenoptera there have been five cases of a major evolutionary transition to obligate eusociality, in the MRCAs of the clades Bombini + Meliponini, Apini, Formicidae, Vespinae, and Epiponini (Figure 9).
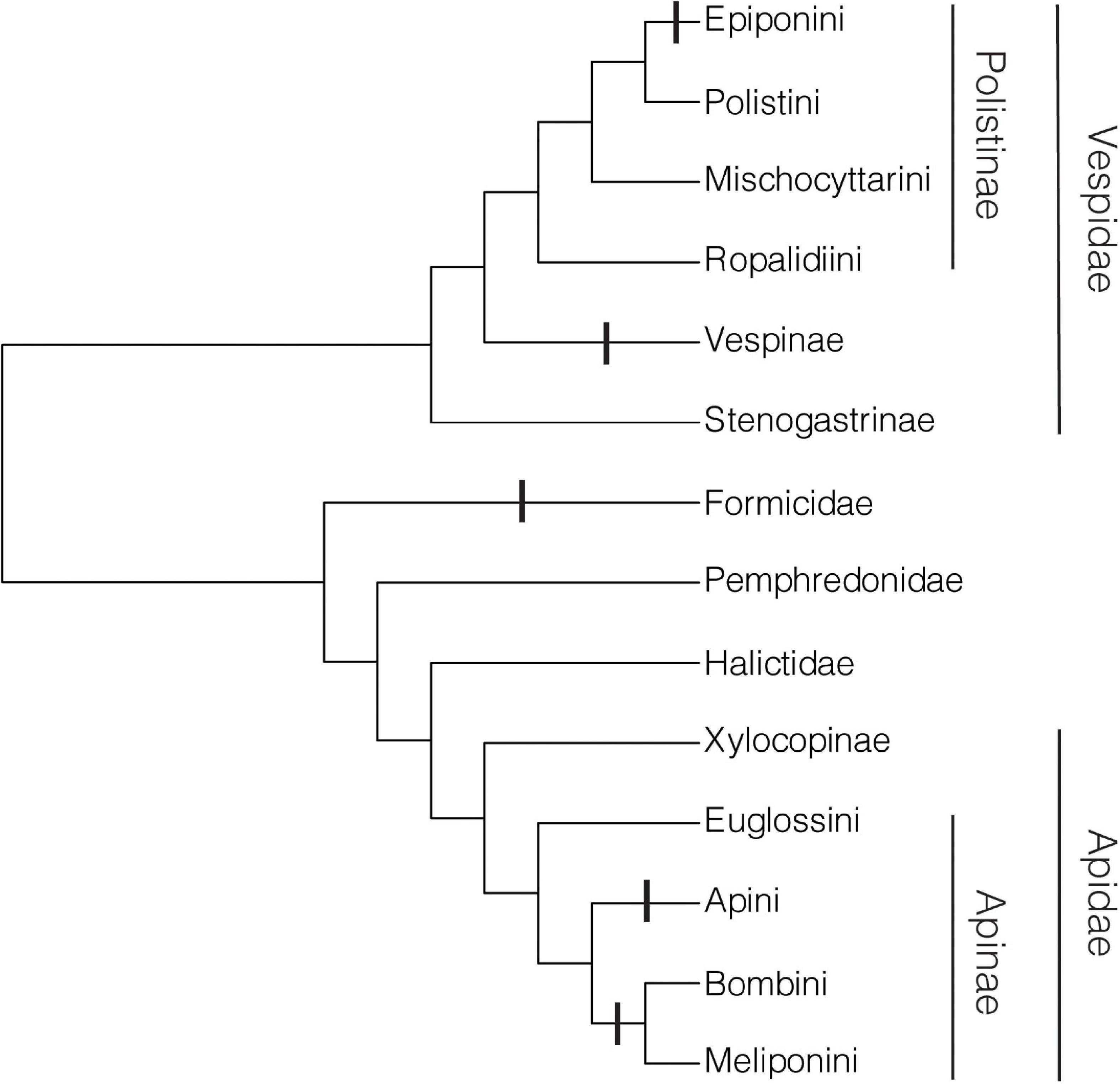
Figure 9. Major evolutionary transitions to obligate eusociality. Vertical bars indicate transitions. Cladogram of groups exhibiting eusociality is based on recent phylogenies (Peters et al., 2017; Piekarski et al., 2018; Sann et al., 2018; Bossert et al., 2019).
The process of a major evolutionary transition to a new level of individuality can be broken down into three steps: social group formation, social group maintenance, and social group transformation (Bourke, 2011). The first step, social group formation, has been the focus of the present attempt to understand the life history factors that favor the origin of eusociality, in the form of primitive eusociality. The third step, social group transformation, is the set of processes that transforms a stable social group (primitive eusociality) to an obligate social group, and is the stage of a major evolutionary transition where the new level of individuality emerges. In the case of obligate eusociality, none of the life history characters studied here are associated directly with social group transformation. However, unlike facultatively eusocial clades, and with the exception of bumblebees, obligately eusocial clades are characterized by species that form very large colonies. This supports the size-complexity hypothesis (Bourke, 2011). In a larger group, an individual worker has a lower probability of attaining reproductive status, thus selecting for greater reproductive altruism, and because of relatedness asymmetries, for worker policing of worker reproduction. Such specialization permits the production of larger groups, which selects for even greater reproductive division of labor, producing a positive feedback loop. The size-complexity hypothesis is supported in the Hymenoptera by positive correlations with colony size for social complexity and morphological and lifespan differences between castes (Fjerdingstad and Crozier, 2006; Rodriguez-Serrano et al., 2012; Ferguson-Gow et al., 2014; da Silva, in press).
An interesting consequence of the major evolutionary transition to obligate eusociality is that inclusive fitness accounting (Hamilton, 1964a) can no longer be used to explain the evolutionary forces maintaining the social group. Inclusive fitness is calculated as the reproductive output of a focal individual (or mated pair), exclusive of any offspring produced due to help from others, plus any additional offspring produced by others due to the focal individual’s help, weighted by the relatedness between the focal individual and those receiving help. This means that a queen in an obligately eusocial species has no inclusive fitness, since all her reproduction is dependent on help from workers (Queller, 1996a). This does not imply that kin selection no longer operates; it simply reflects that inclusive fitness, which is calculated for individuals, must now be calculate for the new level of individual, the colony. I suggest that the inclusive fitness of an obligately eusocial colony may help explain the paradoxical evolution of super-colonies of ants (Helanterä et al., 2009).
Conclusion
This study represents the most comprehensive assessment of the transitions to eusociality in the Hymenoptera to date. Eusociality has evolved more frequently in the Apidae and Halictidae than previously thought. Interestingly, semisociality either precedes or possibly precedes every transition to eusociality outside the Vespidae. Given that semisociality should be favored by haplodiploidy, this pattern may help explain why eusociality is common in the Hymenoptera. Although ancestral state reconstruction and statistical tests of transition rate dependencies show that different life histories may favor eusociality in different families, a loss of prepupal diapause appears to be a general prerequisite. The association with a loss of prepupal diapause may simply reflect the subsocial route to eusociality, both of which may be associated with an extension of the breeding season that permits the production of more than one brood. An intriguing possibility is that with adult diapause a first-brood female that does not have the option of breeding immediately has a very low selective barrier to becoming a worker because she is simply trading off herself against any second-brood offspring she helps to raise. A fuller understanding of the conditions favoring eusociality will require knowledge of the interactions between life cycles and season length that favor first-brood females that opt to help rather than breed or enter diapause. Obligate eusociality, which meets the definition of a major evolutionary transition, has evolved five times in the Hymenoptera. And consistent with the evolution of a new level of individuality, inclusive fitness can no longer be calculated for individuals in obligately eusocial groups but must be calculated at the new level of individuality, the colony.
Data Availability Statement
Data are available in figshare: https://doi.org/10.6084/m9.figshare.16691290.v1.
Author Contributions
The author confirms being the sole contributor of this work and has approved it for publication.
Conflict of Interest
The author declares that the research was conducted in the absence of any commercial or financial relationships that could be construed as a potential conflict of interest.
Publisher’s Note
All claims expressed in this article are solely those of the authors and do not necessarily represent those of their affiliated organizations, or those of the publisher, the editors and the reviewers. Any product that may be evaluated in this article, or claim that may be made by its manufacturer, is not guaranteed or endorsed by the publisher.
Acknowledgments
This work was supported by the School of Biological Sciences, University of Adelaide.
Supplementary Material
The Supplementary Material for this article can be found online at: https://www.frontiersin.org/articles/10.3389/fevo.2021.727124/full#supplementary-material
References
Alexander, R. D., Noonan, K. M., and Crespi, B. J. (1991). “The evolution of eusociality,” in The Biology of the Naked Mole-Rat, eds P. W. Sherman, J. U. M. Jarvis, and R. D. Alexander (Princeton, NJ: Princeton University Press), 3–44.
Amsalem, E., Galbraith, D. A., Cnaani, J., Teal, P. E. A., and Grozinger, C. M. (2015). Conservation and modification of genetic and physiological toolkits underpinning diapause in bumble bee queens. Mol. Ecol. 24, 5596–5615. doi: 10.1111/mec.13410
Andrade, A. C. R., Miranda, E. A., Del Lama, M. A., and Nascimento, F. S. (2016). Reproductive concessions between related and unrelated members promote eusociality in bees. Sci. Rep. 6:26635. doi: 10.1038/srep26635
Bartz, S. H., and Hölldobler, B. (1982). Colony founding in Myrmecocystus mimicus wheeler (Hymenoptera: Formicidae) and the evolution of foundress associations. Behav. Ecol. Sociobiol. 10, 137–147.
Beaulieu, J. M., and O’Meara, B. C. (2014). “Hidden markov models for studying the evolution of binary morphological characters,” in Modern Phylogenetic Comparative Methods and Their Application in Evolutionary Biology, ed. L. Z. Garamszegi (Berlin: Springer-Verlag), 395–408.
Beaulieu, J. M., O’meara, B. C., and Donoghue, M. J. (2013). Identifying hidden rate changes in the evolution of a binary morphological character: the evolution of plant habit in campanulid angiosperms. Syst. Biol. 62, 725–737. doi: 10.1093/sysbio/syt034
Boomsma, J. J. (2009). Lifetime monogamy and the evolution of eusociality. Philos. Trans. R. Soc. B Biol. Sci. 364, 3191–3207.
Boomsma, J. J., and Gawne, R. (2018). Superorganismality and caste differentiation as points of no return: how the major evolutionary transitions were lost in translation. Biol. Rev. 93, 28–54. doi: 10.1111/brv.12330
Bossert, S., Murray, E. A., Almeida, E. A., Brady, S. G., Blaimer, B. B., and Danforth, B. N. (2019). Combining transcriptomes and ultraconserved elements to illuminate the phylogeny of Apidae. Mol. Phylogenet. Evol. 130, 121–131. doi: 10.1016/j.ympev.2018.10.012
Bourke, A. F. G. (2019). Inclusive fitness and the major transitions in evolution. Curr. Opin. Insect Sci. 34, 61–67.
Bourke, A. F. G., and Franks, N. R. (1995). Social Evolution in Ants. Princeton, NJ: Princeton University Press.
Brady, S. G., Sipes, S., Pearson, A., and Danforth, B. N. (2006). Recent and simultaneous origins of eusociality in halictid bees. Proc. R. Soc. B Biol. Sci. U.S.A. 273, 1643–1649. doi: 10.1098/rspb.2006.3496
Brand, N., and Chapuisat, M. (2016). Low relatedness and frequent inter-nest movements in a eusocial sweat bee. Insectes Soc. 63, 249–256. doi: 10.1007/s00040-015-0460-0
Branstetter, M. G., Danforth, B. N., Pitts, J. P., Faircloth, B. C., Ward, P. S., Buffington, M. L., et al. (2017). Phylogenomic insights into the evolution of stinging wasps and the origins of ants and bees. Curr. Biol. 27, 1019–1025. doi: 10.1016/j.cub.2017.03.027
Brockmann, H. J. (1997). “Cooperative breeding in wasps and vertebrates: the role of ecological constraints,” in The Evolution of Social Behaviour in Insects and Arachnids, eds B. J. Crespi and J. C. Choe (Cambridge: Cambridge University Press), 347–371.
Brockmann, H. J., and Grafen, A. (1992). Sex ratios and life-history patterns of a solitary wasp, Trypoxylon (Trypargilum) politum (Hymenoptera : Sphecidae). Behav. Ecol. Sociobiol. 30, 7–27.
Cameron, S. A. (1993). Multiple origins of advanced eusociality in bees inferred from mitochondrial DNA sequences. Proc. Natl. Acad. Sci. U.S.A. 90, 8687–8691. doi: 10.1073/pnas.90.18.8687
Cameron, S. A., Hines, H. M., and Williams, P. (2007). A comprehensive phylogeny of the bumble bees (Bombus). Biol. J. Linn. Soc. 91, 161–188.
Cardinal, S., and Danforth, B. N. (2011). The antiquity and evolutionary history of social behavior in bees. PLoS One 6:e21086. doi: 10.1371/journal.pone.0021086
Cardinal, S., and Danforth, B. N. (2013). Bees diversified in the age of eudicots. Proc. R. Soc. B Biol. Sci. 280:20122686. doi: 10.1098/rspb.2012.2686
Cowan, D. P. (1991). “The solitary and presocial Vespidae,” in The Social Biology of Wasps, eds K. G. Ross and R. W. Matthews (Ithaca, NY: Cornell University Press), 33–73. doi: 10.1016/j.ympev.2017.08.020
Crespi, B. J. (1996). “Comparative analysis of the origins and losses of eusociality: causal mosaics and historical uniqueness,” in Phylogenies and the Comparative Method in Animal Behavior, ed. E. P. Martins (New York, NY: Oxford University Press), 253–287.
Crespi, B. J., and Yanega, D. (1995). The definition of eusociality. Behavioral Ecology 6, 109–115. doi: 10.1093/beheco/6.1.109
da Silva, J. (in press). The extension of foundress lifespan and the evolution of eusociality in the Hymenoptera. Am. Nat.
Danforth, B. N. (2002). Evolution of sociality in a primitively eusocial lineage of bees. Proc. Natl. Acad. Sci. U.S.A. 99, 286–290. doi: 10.1073/pnas.012387999
Danforth, B. N., Cardinal, S., Praz, C., Almeida, E. A., and Michez, D. (2013). The impact of molecular data on our understanding of bee phylogeny and evolution. Annu. Rev. Entomol. 58, 57–78. doi: 10.1146/annurev-ento-120811-153633
Danforth, B. N., and Eickwort, G. C. (1997). “The evolution of social behavior in the augochlorine sweat bees (Hymenoptera: Halictidae) based on a phylogenetic analysis of the genera,” in The Evolution of Social Behaviour in Insects and Arachnids, eds B. J. Crespi and J. C. Choe (Cambridge: Cambridge University Press), 270–292. doi: 10.1017/cbo9780511721953.014
Danforth, B. N., Minckley, R. L., Neff, J. L., and Fawcett, F. (2019). The Solitary Bees: Biology, Evolution, Conservation. Princeton, NJ: Princeton University Press.
Dorchin, A., López-Uribe, M. M., Praz, C. J., Griswold, T., and Danforth, B. N. (2018). Phylogeny, new generic-level classification, and historical biogeography of the Eucera complex (Hymenoptera: Apidae). Mol. Phylogenet. Evol. 119, 81–92. doi: 10.1016/j.ympev.2017.10.007
Dubitzky, A. (2007). Phylogeny of the world Anthophorini (Hymenoptera: Apoidea: Apidae). Syst. Entomol. 32, 585–600.
Eickwort, G. C. (1981). “Presocial insects,” in Social Insects, ed. H. R. Hermann (New York, NY: Academic Press), 199–280. doi: 10.1016/b978-0-12-342202-6.50010-1
Evans, H. E. (1977). Observations on the nests and prey of eumenid wasps (Hymenoptera, Eumenidae). Psyche 84, 255–259. doi: 10.1155/1977/49726
Ferguson-Gow, H., Sumner, S., Bourke, A. F., and Jones, K. E. (2014). Colony size predicts division of labour in attine ants. Proc. R. Soc. B Biol. Sci. 281:20141411. doi: 10.1098/rspb.2014.1411
Field, J. (2005). The evolution of progressive provisioning. Behav. Ecol. 16, 770–778. doi: 10.1093/beheco/ari054
Field, J., Paxton, R. J., Soro, A., and Bridge, C. (2010). Cryptic plasticity underlies a major evolutionary transition. Curr. Biol. 20, 2028–2031. doi: 10.1016/j.cub.2010.10.020
Field, J., and Toyoizumi, H. (2020). The evolution of eusociality: no risk-return tradeoff but the ecology matters. Ecol. Lett. 23, 518–526. doi: 10.1111/ele.13452
FitzJohn, R. G., Maddison, W. P., and Otto, S. P. (2009). Estimating trait-dependent speciation and extinction rates from incompletely resolved phylogenies. Syst. Biol. 58, 595–611. doi: 10.1093/sysbio/syp067
Fjerdingstad, E. J., and Crozier, R. H. (2006). The evolution of worker caste diversity in social insects. Am. Nat. 167, 390–400. doi: 10.2307/3844761
Freiria, G. A., Garófalo, C. A., and Del Lama, M. A. (2017). The primitively social behavior of Euglossa cordata (Hymenoptera, Apidae, Euglossini): a view from the perspective of kin selection theory and models of reproductive skew. Apidologie 48, 523–532. doi: 10.1007/s13592-017-0496-4
Fromhage, L., and Kokko, H. (2011). Monogamy and haplodiploidy act in synergy to promote the evolution of eusociality. Nat. Commun. 2:397. doi: 10.1038/ncomms1410
Fye, R. (1965). The biology of the Vespidae, Pompilidae, and Sphecidae (Hymenoptera) from trap nests in Northwestern Ontario. Can. Entomol. 97, 716–744.
Gadagkar, R. (1990). Evolution of eusociality: the advantage of assured fitness returns. Philos. Trans. R. Soc. Lond. Ser. B Biol. Sci. 329, 17–25. doi: 10.1098/rstb.1990.0146
Gardner, A., Alpedrinha, J., and West, S. A. (2012). Haplodiploidy and the evolution of eusociality: split sex ratios. Am. Nat. 179, 240–256. doi: 10.1086/663683
Gardner, A., and Ross, L. (2013). Haplodiploidy, sex-ratio adjustment, and eusociality. Am. Nat. 181, E60–E67. doi: 10.1086/669147
Garofalo, C. A. (1985). Social structure of Euglossa cordata nests (Hymenoptera: Apidae: Euglossini). Entomol. Gener. 11, 77–83. doi: 10.1127/entom.gen/11/1985/77
Giannotti, E., and Machado, V. L. L. (1994). Longevity, life table and age polyethism in Polistes lanio lanio (Hymenoptera: Vespidae), a primitive eusocial wasp. J. Adv. Zool. 15, 95–101.
Gibbs, J., Brady, S. G., Kanda, K., and Danforth, B. N. (2012). Phylogeny of halictine bees supports a shared origin of eusociality for Halictus and Lasioglossum (Apoidea: Anthophila: Halictidae). Mol. Phylogenet. Evol. 65, 926–939. doi: 10.1016/j.ympev.2012.08.013
Gonçalves, R. B. (2016). A molecular and morphological phylogeny of the extant Augochlorini (Hymenoptera, Apoidea) with comments on implications for biogeography. Syst. Entomol. 41, 430–440. doi: 10.1111/syen.12166
Gurney, A. B. (1953). Notes on the biology and immature stages of a cricket parasite of the genus Rhopalosoma. Proc. U.S. Natl. Mus. 103, 19–34. doi: 10.5479/si.00963801.103-3313.19
Habermannova, J., Bogusch, P., and Straka, J. (2013). Flexible host choice and common host switches in the evolution of generalist and specialist cuckoo bees (Anthophila: Sphecodes). PLoS One 8:e64537. doi: 10.1371/journal.pone.0064537
Hamilton, W. D. (1964a). The genetical evolution of social behaviour. I. J. Theor. Biol. 7, 1–16. doi: 10.1016/0022-5193(64)90038-4
Hamilton, W. D. (1964b). The genetical evolution of social behaviour. II. J. Theor. Biol. 7, 17–52. doi: 10.1016/0022-5193(64)90039-6
Helanterä, H., Strassmann, J. E., Carrillo, J., and Queller, D. C. (2009). Unicolonial ants: where do they come from, what are they and where are they going? Trends Ecol. Evol. 24, 341–349. doi: 10.1016/j.tree.2009.01.013
Hines, H. M., Hunt, J. H., O’connor, T. K., Gillespie, J. J., and Cameron, S. A. (2007). Multigene phylogeny reveals eusociality evolved twice in vespid wasps. Proc. Natl. Acad. Sci. U.S.A. 104, 3295–3299. doi: 10.1073/pnas.0610140104
Hogendoorn, K., and Velthuis, H. H. W. (1999). Task allocation and reproductive skew in social mass provisioning carpenter bees in relation to age and size. Insectes Soc. 46, 198–207. doi: 10.1007/s000400050135
Holland, J. G., and Bloch, G. (2020). The complexity of social complexity: a quantitative multidimensional approach for studies of social organization. Am. Nat. 196, 525–540. doi: 10.1086/710957
Hughes, W. O. H., Oldroyd, B. P., Beekman, M., and Ratnieks, F. L. W. (2008). Ancestral monogamy shows kin selection is key to the evolution of eusociality. Science 320, 1213–1216. doi: 10.1126/science.1156108
Hunt, J. H. (2007). The Evolution of Social Wasps : History, Dynamics, and Paradigm. New York, NY: Oxford University Press USA–OSO.
Hunt, J. H., and Amdam, G. V. (2005). Bivoltinism as an antecedent to eusociality in the paper wasp genus Polistes. Science 308, 264–267. doi: 10.1126/science.1109724
Hunt, J. H., Kensinger, B. J., Kossuth, J. A., Henshaw, M. T., Norberg, K., Wolschin, F., et al. (2007). A diapause pathway underlies the gyne phenotype in Polistes wasps, revealing an evolutionary route to caste-containing insect societies. Proc. Natl. Acad. Sci. U.S.A. 104:14020. doi: 10.1073/pnas.0705660104
Hurst, P. S., Gray, S., Schwarz, M. P., Tilly, J. A., Foran, A. C., and Adams, M. (1997). Increased nest cofounding and high intra-colony relatedness in the bee Exoneura bicolor (Hymenoptera: Apidae): results from an experimental situation. Austral Ecol. 22, 419–424. doi: 10.1111/j.1442-9993.1997.tb00692.x
Itô, Y., and Kasuya, E. (2005). Demography of the Okinawan eusocial wasp Ropalidia fasciata (Hymenoptera: Vespidae) I. Survival rate of individuals and colonies, and yearly fluctuations in colony density. Entomol. Sci. 8, 41–64.
Janjic, J., and Packer, L. (2003). Phylogeny of the bee genus Agapostemon (Hymenoptera: Halictidae). Syst. Entomol. 28, 101–124. doi: 10.1046/j.1365-3113.2003.00204.x
Jeanne, R. L. (1991). “The swarm-founding Polistinae,” in The Social Biology of Wasps, eds K. G. Ross and R. W. Matthews (Ithaca, NY: Cornell University Press), 191–231. doi: 10.7591/9781501718670-009
Keller, L., and Genoud, M. (1997). Extraordinary lifespans in ants: a test of evolutionary theories of ageing. Nature 389, 958–960. doi: 10.1038/40130
Kocher, S. D., Mallarino, R., Rubin, B. E. R., Yu, D. W., Hoekstra, H. E., and Pierce, N. E. (2018). The genetic basis of a social polymorphism in halictid bees. Nat. Commun. 9:4338. doi: 10.1038/s41467-018-06824-8
Kocher, S. D., and Paxton, R. J. (2014). Comparative methods offer powerful insights into social evolution in bees. Apidologie 45, 289–305. doi: 10.1007/s13592-014-0268-3
Leadbeater, E., Carruthers, J. M., Green, J. P., Rosser, N. S., and Field, J. (2011). Nest inheritance is the missing source of direct fitness in a primitively eusocial insect. Science 333, 874–876. doi: 10.1126/science.1205140
Litman, J. R., Praz, C. J., Danforth, B. N., Griswold, T. L., and Cardinal, S. (2013). Origins, evolution, and diversification of cleptoparasitic lineages in long-tongued bees. Evolution 67, 2982–2998. doi: 10.1111/evo.12161
Lopez-Osorio, F., Perrard, A., Pickett, K. M., Carpenter, J. M., and Agnarsson, I. (2015). Phylogenetic tests reject Emery’s rule in the evolution of social parasitism in yellowjackets and hornets (Hymenoptera: Vespidae, Vespinae). R. Soc. Open Sci. 2:150159. doi: 10.1098/rsos.150159
Lucas, E. R., and Field, J. (2011). Assured fitness returns in a social wasp with no worker caste. Proc. R. Soc. B Biol. Sci. 278, 2991–2995. doi: 10.1098/rspb.2011.0128
Martins, A. C., and Melo, G. A. R. (2016). The new world oil-collecting bees Centris and Epicharis (Hymenoptera, Apidae): Molecular phylogeny and biogeographic history. Zool. Scr. 45, 22–33. doi: 10.1111/zsc.12133
Matthews, R. W. (1991). “Evolution of social behavior in sphecid wasps,” in The Social Biology of Wasps, eds K. G. Ross and R. W. Matthews (Ithaca, NY: Cornell University Press), 570–602. doi: 10.7591/9781501718670-020
Maynard Smith, J., and Szathmáry, E. (1995). The Major Transitions in Evolution. Oxford: W.H. Freeman Spektrum.
Metcalf, R., and Whitt, G. (1977). Relative inclusive fitness in the social wasp Polistes metricus. Behav. Ecol. Sociobiol. 2, 353–360. doi: 10.1007/bf00299505
Michener, C. D. (1974). ). The Social Behavior of the Bees : A Comparative Study. Cambridge, MA: Belknap Press of Harvard University Press.
Noll, F. B., and Wenzel, J. W. (2008). Caste in the swarming wasps:‘queenless’ societies in highly social insects. Biol. J. Linn. Soc. 93, 509–522. doi: 10.1186/s12983-014-0078-5
O’Neill, K. M. (2001). Solitary Wasps: Behavior and Natural History. Ithaca, NY: Cornell University Press.
Pagel, M. (1994). Detecting correlated evolution on phylogenies: a general method for the comparative analysis of discrete characters. Proc. R. Soc. Lond. Ser. B Biol. Sci. 255, 37–45.
Patiny, S., Michez, D., and Danforth, B. N. (2008). Phylogenetic relationships and host-plant evolution within the basal clade of Halictidae (Hymenoptera. Apoidea). Cladistics 24, 255–269.
Perrard, A., Pickett, K. M., Villemant, C., Kojima, J.-I., and Carpenter, J. (2013). Phylogeny of hornets: a total evidence approach (Hymenoptera, Vespidae, Vespinae, Vespa). J. Hymenopt. Res. 32, 1–15.
Peters, R. S., Krogmann, L., Mayer, C., Donath, A., Gunkel, S., Meusemann, K., et al. (2017). Evolutionary history of the Hymenoptera. Curr. Biol. 27, 1013–1018.
Piekarski, P. K., Carpenter, J. M., Lemmon, A. R., Moriarty Lemmon, E., and Sharanowski, B. J. (2018). Phylogenomic evidence overturns current conceptions of social evolution in wasps (Vespidae). Mol. Biol. Evol. 35, 2097–2109. doi: 10.1093/molbev/msy124
Purcell, J. (2011). Geographic patterns in the distribution of social systems in terrestrial arthropods. Biol. Rev. 86, 475–491.
Queller, D. C. (1996b). “The origin and maintenance of eusociality: the advantage of extended parental care,” in Natural History and Evolution of Paper-Wasps, eds S. Turillazzi and M. J. West-Eberhard (Oxford: Oxford University Press), 218–234.
R Core Team (2019). R: A Language and Environment for Statistical Computing. Vienna: R Foundation for Statistical Computing.
Rasmussen, C., and Cameron, S. A. (2007). A molecular phylogeny of the Old World stingless bees (Hymenoptera: Apidae: Meliponini) and the non-monophyly of the large genus Trigona. Syst. Entomol. 32, 26–39. doi: 10.1111/j.1365-3113.2006.00362.x
Reeve, H. K., Peters, J. M., Nonacs, P., and Starks, P. T. (1998). Dispersal of first “workers” in social wasps: causes and implications of an alternative reproductive strategy. Proc. Natl. Acad. Sci. U.S.A. 95, 13737–13742. doi: 10.1073/pnas.95.23.13737
Rehan, S. M., Leys, R., and Schwarz, M. P. (2012). A mid-cretaceous origin of sociality in xylocopine bees with only two origins of true worker castes indicates severe barriers to eusociality. PLoS One 7:e34690. doi: 10.1371/journal.pone.0034690
Revell, L. J. (2012). phytools: an R package for phylogenetic comparative biology (and other things). Methods Ecol. Evol. 3, 217–223.
Richards, M. H. (2019). Social trait definitions influence evolutionary inferences: a phylogenetic approach to improving social terminology for bees. Curr. Opin. Insect Sci. 34, 97–104. doi: 10.1016/j.cois.2019.04.006
Rodriguez-Serrano, E., Inostroza-Michael, O., Avaria-Llautureo, J., and Hernandez, C. E. (2012). Colony size evolution and the origin of eusociality in corbiculate bees (Hymenoptera: Apinae). PLoS One 7:e40838. doi: 10.1371/journal.pone.0040838
Ross, K. G., and Carpenter, J. M. (1991). “Population genetic structure, relatedness, and breeding systems,” in The Social Biology of Wasps, eds K. G. Ross and R. W. Matthews (Ithaca, NY: Comstock Pub. Associates), 451–479.
Ross, L., Gardner, A., Hardy, N., and West, S. A. (2013). Ecology, not the genetics of sex determination, determines who helps in eusocial populations. Curr. Biol. 23, 2383–2387.
Saito, F., Nguyen, L. T. P., and Kojima, J. (2009). Colony cycle of a “temperate” hover wasp, Eustenogaster nigra, with special reference to overwintering of males in an enveloped nest together with virgin females (Hymenoptera, Vespidae, Stenogastrinae). Insectes Soc. 56, 49–54.
Sann, M., Niehuis, O., Peters, R. S., Mayer, C., Kozlov, A., Podsiadlowski, L., et al. (2018). Phylogenomic analysis of Apoidea sheds new light on the sister group of bees. BMC Evol. Biol. 18:71. doi: 10.1186/s12862-018-1155-8
Santos, B. F., Payne, A., Pickett, K. M., and Carpenter, J. M. (2015). Phylogeny and historical biogeography of the paper wasp genus Polistes (Hymenoptera: Vespidae): implications for the overwintering hypothesis of social evolution. Cladistics 31, 535–549.
Santos, P. K. F., Arias, M. C., and Kapheim, K. M. (2019). Loss of developmental diapause as prerequisite for social evolution in bees. Biol. Lett. 15:20190398.
Schwarz, M. P. (1987). Intra-colony relatedness and sociality in the allodapine bee Exoneura bicolor. Behav. Ecol. Sociobiol. 21, 387–392.
Schwarz, M. P. (1988). Local resource enhancement and sex ratios in a primitively social bee. Nature 331, 346–348.
Schwarz, M. P., Fuller, S., Tierney, S. M., and Cooper, S. J. (2006). Molecular phylogenetics of the exoneurine allodapine bees reveal an ancient and puzzling dispersal from Africa to Australia. Syst. Biol. 55, 31–45.
Schwarz, M. P., Richards, M. H., and Danforth, B. N. (2007). Changing paradigms in insect social evolution: insights from halictine and allodapine bees. Annu. Rev. Entomol. 52, 127–150.
Seger, J. (1983). Partial bivoltinism may cause alternating sex-ratio biases that favour eusociality. Nature 301, 59–62.
Shreeves, G., Cant, M. A., Bolton, A., and Field, J. (2003). Insurance–based advantages for subordinate co–foundresses in a temperate paper wasp. Proc. R. Soc. Lond. Ser. B Biol. Sci. 270, 1617–1622.
Shreeves, G., and Field, J. (2002). Group size and direct fitness in social queues. Am. Nat. 159, 81–95.
Straka, J. Černá, K., Macháčková, L., Zemenová, M., and Keil, P. (2014). Life span in the wild: the role of activity and climate in natural populations of bees. Funct. Ecol. 28, 1235–1244.
Strassmann, J. E., Hughes, C. R., Queller, D. C., Turillazzi, S., Cervo, R., Davis, S. K., et al. (1989). Genetic relatedness in primitively eusocial wasps. Nature 342:268.
Strassmann, J. E., Meyer, D. C., and Matlock, R. L. (1984). Behavioral castes in the social wasp Polistes exclamans (Mymenoptera: Vespidae). Sociobiology 8, 211–224.
Strassmann, J. E., Sullender, B. W., and Queller, D. C. (2002). Caste totipotency and conflict in a large–colony social insect. Proc. R. Soc. Lond. Ser. B Biol. Sci. 269, 263–270.
Stubblefield, J. W., and Charnov, E. L. (1986). Some conceptual issues in the origin of eusociality. Heredity 57(Pt 2), 181–187.
Trivers, R. L., and Hare, H. (1976). Haploidploidy and the evolution of the social insect. Science 191, 249–263.
Wcislo, W. T., and Danforth, B. N. (1997). Secondarily solitary: the evolutionary loss of social behavior. Trends Ecol. Evol. 12, 468–474.
West, S. A., Fisher, R. M., Gardner, A., and Kiers, E. T. (2015). Major evolutionary transitions in individuality. Proc. Natl. Acad. Sci. U.S.A. 112, 10112–10119.
West-Eberhard, M. J. (1978). Polygyny and the evolution of social behavior in wasps. J. Kans. Entomol. Soc. 51, 832–856.
Wheeler, W. M. (1923). Social Life Among the Insects; Being a Series of Lectures Delivered at the Lowell Institute in Boston in March 1922. New York, NY: Harcourt, Brace and Company.
Wilson, E. O. (1971). The Insect Societies. Cambridge, MA: Belknap Press of Harvard University Press.
Wilson, E. O., and Hölldobler, B. (2005). Eusociality: origin and consequences. Proc. Natl. Acad. Sci. U.S.A. 102, 13367–13371.
Winston, M. L., and Michener, C. D. (1977). Dual origin of highly social behavior among bees. Proc. Natl. Acad. Sci. U.S.A. 74:1135.
Yanega, D. (1988). Social plasticity and early-diapausing females in a primitively social bee. Proc. Natl. Acad. Sci. U.S.A. 85, 4374–4377.
Yanega, D. (1996). Sex ratio and sex allocation in sweat bees (Hymenoptera: Halictidae). J. Kans. Entomol. Soc. 69, 98–115.
Keywords: eusociality, life history, diapause, bees, wasps, major evolutionary transition
Citation: da Silva J (2021) Life History and the Transitions to Eusociality in the Hymenoptera. Front. Ecol. Evol. 9:727124. doi: 10.3389/fevo.2021.727124
Received: 18 June 2021; Accepted: 28 September 2021;
Published: 09 December 2021.
Edited by:
Karen Marie Kapheim, Utah State University, United StatesReviewed by:
Adam R. Smith, George Washington University, United StatesPriscila Santos, The Pennsylvania State University (PSU), United States
Copyright © 2021 da Silva. This is an open-access article distributed under the terms of the Creative Commons Attribution License (CC BY). The use, distribution or reproduction in other forums is permitted, provided the original author(s) and the copyright owner(s) are credited and that the original publication in this journal is cited, in accordance with accepted academic practice. No use, distribution or reproduction is permitted which does not comply with these terms.
*Correspondence: Jack da Silva, amFjay5kYXNpbHZhQGFkZWxhaWRlLmVkdS5hdQ==