- 1HiLIFE Helsinki Institute of Life Science, University of Helsinki, Helsinki, Finland
- 2Research Programme in Organismal and Evolutionary Biology, Faculty of Biological and Environmental Sciences, University of Helsinki, Helsinki, Finland
- 3Dipartimento STEBICEF, Università degli Studi di Palermo, Palermo, Italy
Hosts of brood parasitic cuckoos often employ mobbing attacks to defend their nests and, when mobbing is costly, hosts are predicted to adjust their mobbing to match parasitism risk. While evidence exists for fine-tuned plasticity, it remains unclear why mobbing does not track larger seasonal changes in parasitism risk. Here we test a possible explanation from parental investment theory: parents should defend their current brood more intensively as the opportunity to replace it declines (re-nesting potential), and therefore “counteract” any apparent seasonal decline to match parasitism risk. We take advantage of mobbing experiments conducted at two sites where reed warblers (Acrocephalus scirpaceus) experience (in Italy), or do not experience (in Finland), brood parasitism. We predicted that mobbing of cuckoos should be higher overall in Italy, but remain constant over the season as in other parasitised sites, whereas in Finland where cuckoos do not pose a local threat, we predicted that mobbing should be low at the beginning of the season but increase as re-nesting potential declined. However, while cuckoos were more likely to be mobbed in Italy, we found little evidence that mobbing changed over the season at either the parasitized or non-parasitized sites. This suggests that re-nesting potential has either little influence on mobbing behavior, or that its effects are obscured by other seasonal differences in ecology or experience of hosts.
Introduction
When encountering an intruder at the nest, many parent birds use noisy vocal and visual displays to defend their brood. However, indiscriminate mobbing behavior is rarely optimal (Montgomerie and Weatherhead, 1988) and depends on the benefits gained by protecting the survival of the current brood outweighing the risks it entails for a parent’s future reproduction (Trivers, 1972) or continued survival (Montgomerie and Weatherhead, 1988). Taking such an optimality approach to parental risk-taking behavior has advanced understanding of the ecology and evolution of predator-prey interactions (Caro, 2005), and inspired extensive research into quantifying the ecological factors that shape the costs and benefits of parental behavior (e.g., Oteyza et al., 2021). Although mobbing to protect the nest is also a widespread “frontline” defense behavior (Welbergen and Davies, 2009) employed by hosts against avian brood parasites (Feeney et al., 2012), it has rarely been investigated explicitly in terms of trade-offs against a parent’s future reproduction or continued survival. Therefore, and despite receiving increasing research interest over the last decade (Feeney, 2017), we still lack a comprehensive understanding of the ecological factors that shape the costs and benefits determining optimal mobbing responses.
During mobbing attacks, hosts attempt to prevent the brood parasite from laying its egg in their nest (e.g., Welbergen and Davies, 2009), or reduce the collateral damage of parasitism for host fitness (e.g., mobbing prevents shiny cowbirds from damaging their host’s eggs during laying, enabling more mockingbird young to survive: Gloag et al., 2013). Mobbing a brood parasite, however, also involves potential risks for hosts: noisy mobbing displays can attract attention and reveal the nest’s location to eavesdropping predators (Krama and Krams, 2005) or other brood parasites (Marton et al., 2019), and inadvertently contribute to the eventual loss of the reproductive attempt (Campobello and Sealy, 2018). Furthermore, many brood parasite species have evolved mimicry to thwart host detection. For example, female cuckoo finches (Anomalospiza imberbis) appear to be aggressive mimics of a harmless heterospecific (Feeney et al., 2015), whereas several Cuculidae cuckoos are Batesian mimics of dangerous hawks (Welbergen and Davies, 2011; Thorogood and Davies, 2013a). In the case of hawk-mimicry, recognition errors can be fatal for adults (if a hawk is misidentified as a cuckoo and approached), whereas misidentifying a virulent cuckoo as a hawk can lead to elimination of the current brood. Together these costs mean that, as in models of antipredator mobbing, hosts should adjust their mobbing to match local variation in parasitism risk.
Evidence that hosts match their mobbing behavior to parasitism risk is, however, mixed. In their landmark paper, Moksnes and colleagues systematically compared mobbing responses among hosts in Norway and found that of the 14 species parasitized by Common cuckoos (Cuculus canorus) in their study, the least aggressive species were most commonly parasitized (Moksnes et al., 1991). In a follow-up study, however, they detected a strong positive correlation between the suitability of a host species and its propensity to show aggression toward cuckoos (Røskaft et al., 2002). Nevertheless, intensive experiments with reed warblers (Acrocephalus scirpaceus) indicate fine-scale matching of mobbing to parasitism risk can occur. Mobbing occurs less often and at lower intensity at sites where parasitism is absent (Lindholm and Thomas, 2000; Thorogood and Davies, 2013b), and also matches temporal changes within sites: as parasitism rates declined dramatically over three decades at Wicken Fen in England, the proportion of reed warbler pairs that invested in mobbing also declined (Thorogood and Davies, 2013b). Furthermore, the parasitism rate of the population within 3 days of focal birds’ laying predicted the likelihood that reed warblers would mob a cuckoo at their nest, suggesting that small scale adjustments of mobbing propensity shape patterns at larger temporal and spatial scales (Thorogood and Davies, 2013b). However, there was no evidence that reed warblers adjusted their mobbing to seasonal reductions in parasitism of a similar magnitude to annual changes in parasitism risk (Thorogood and Davies, 2013b), despite becoming less likely to reject experimental cuckoo eggs (also see Brooke et al., 1998). Indeed, of the four studies investigating mobbing behavior in reed warblers that factored season into their analyses as a covariate (Table 1), only one found an effect: aggression decreased linearly through the season (Čapek et al., 2010). While positive correlations between season and mobbing behavior have been detected in other host-brood parasite systems (e.g., Japanese bush warbler, Cettia diphone, vs. little cuckoo, Cuculus poliocephalus: Hamao, 2011), this occurred when parasitism increased as the season progresses. How can we reconcile evidence for optimal mobbing at fine and coarse temporal and spatial scales, but not with season?
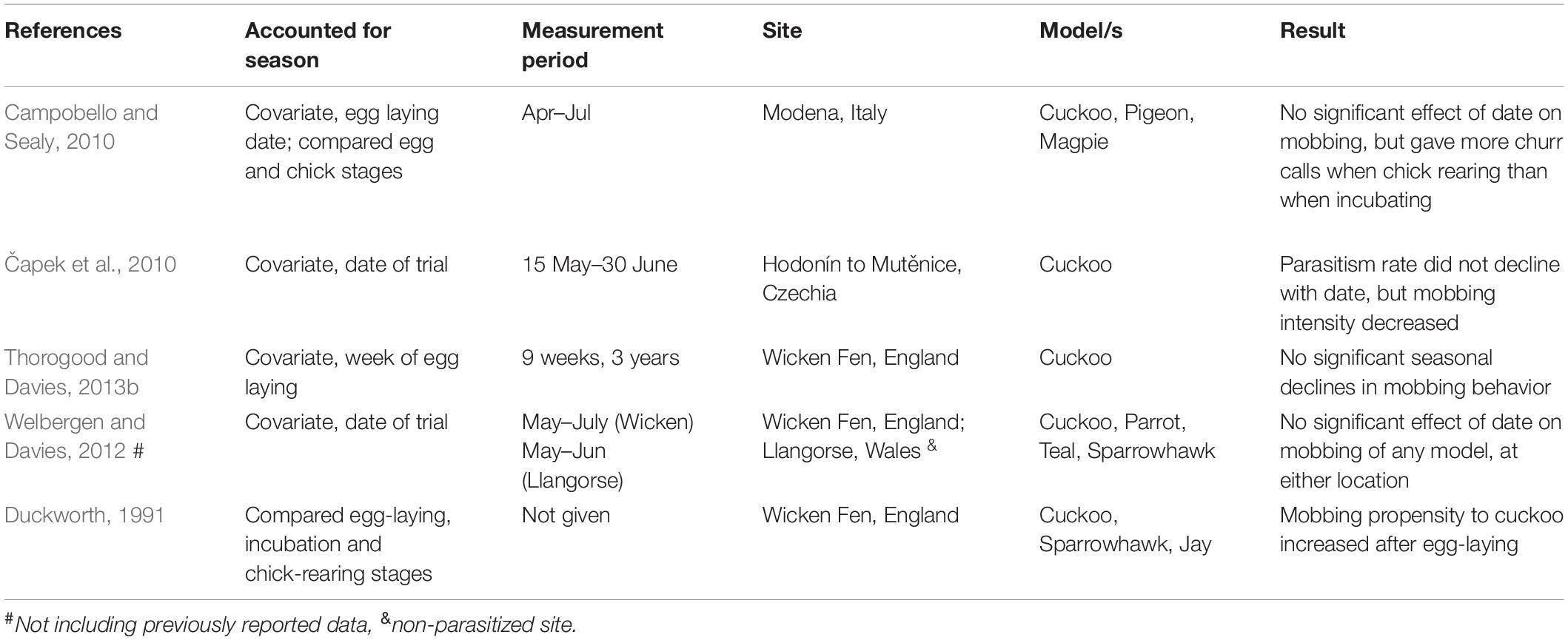
Table 1. Summary of studies with reed warblers, Acrocephalus scirpaceus that included seasonal effects in analyses of mobbing toward cuckoos (other models presented are also shown), or investigated effects of reproductive value via breeding stage.
One possible explanation may come from considering how trade-offs of protecting current against future reproduction vary during the season. Parental investment theory predicts that parents should defend their current offspring aggressively as they increase in value (Trivers, 1972), but only as long as the fitness benefit gained from mobbing is greater than the cost it imposes on a parent’s potential for reproduction in the future (i.e., residual reproductive value; Williams, 1966). Opportunities for future reproduction decline rapidly during the breeding season (“re-nesting potential”; Barash, 1975), especially when parents face uncertainty about their survival to the following year (Montgomerie and Weatherhead, 1988). If diminishing re-nesting potential increases the relative benefit of mobbing behavior, then parents might therefore continue mobbing cuckoos, even as the risk of parasitism declines. Depending on the relative strength and seasonal patterns of changing parasitism risk and re-nesting potential, this could result in either no apparent overall seasonal change in mobbing behavior, or a curvilinear relationship at parasitized sites (Figure 1). However, when parasitism risk is absent, a seasonal pattern in response to re-nesting potential should become easier to detect. Although re-nesting potential has received much attention in how it might shape optimal anti-predator mobbing (e.g., Shew et al., 2016), it remains virtually unexplored in the context of brood parasitism, and all but one of the previous studies that incorporated an effect of season into their analyses tested mobbing behavior at parasitized sites (Table 1).
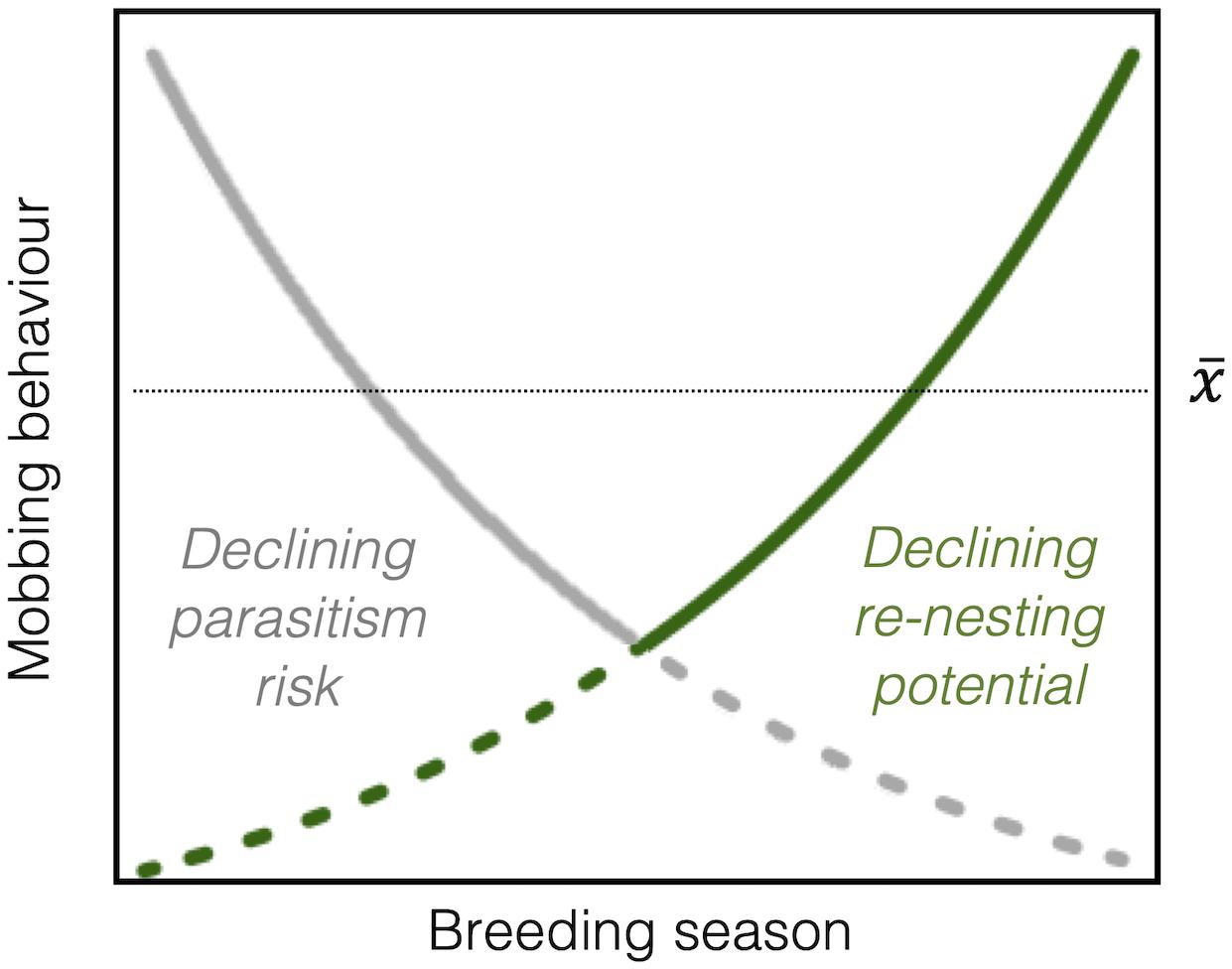
Figure 1. Conceptual example of how changes in re-nesting potential (dark green line) over the breeding season could explain no overall seasonal change (, horizontal dotted line), or a quadratic change (solid lines), in mobbing behavior of hosts toward cuckoos, despite declining parasitism risk (dark gray line). Dashed lines indicate where the benefit of reducing mobbing is outweighed by the positive effect of either factor. Note that the shape of the detected seasonal relationship will depend on the relative strength and seasonal patterns of different factors.
Here we use a non-parasitized population of reed warblers in Finland to investigate if host mobbing toward cuckoos changes as re-nesting potential declines through the breeding season. Finland lies at the northern range margin for reed warblers, and although cuckoos are common, the gens that parasitizes reed warblers is absent. Reed warblers migrate to sub-Saharan Africa to overwinter, and their adult survival is ∼50% (Stolt, 1999). Furthermore, their breeding season is several weeks shorter in Finland than in the core of the European breeding range (Halupka et al., 2008). Together this means that re-nesting potential should diminish rapidly through the season, and parents will face uncertainty about surviving to future breeding seasons (i.e., main criteria for re-nesting potential to affect mobbing; Montgomerie and Weatherhead, 1988). As models evaluating the effects of re-nesting potential emphasize changes in mobbing intensity (Montgomerie and Weatherhead, 1988), here we use an information theoretic approach to investigate seasonal changes in reed warblers’ latency to approach their nest, their propensity to mob, and the intensity of their mobbing displays. If mobbing behavior is based on parasitism risk alone, we predicted reed warblers in Finland to show low aggression overall toward cuckoos (similar to Lindholm and Thomas, 2000; Welbergen and Davies, 2012). However, if re-nesting potential informs mobbing behavior, then we predicted aggression toward nest intruders to increase as the breeding season progressed. Finally, all previous studies that included season in their analyses of mobbing behavior did so as a linear term (Table 1). As the shape of seasonal change in a parasitised population may be curvilinear (e.g., Figure 1), we re-examined a published dataset from a parasitized population of reed warblers in Italy (Campobello and Sealy, 2010) to explore whether mobbing showed a non-linear relationship with date (e.g., declining with parasitism risk until increasing later in the season as re-nesting potential declined). In both populations we compared mobbing responses to a magpie (Pica pica), a common nest predator that preys on passerine eggs and young (Birkhead, 1991). If seasonal patterns in mobbing are a function of reduced re-nesting potential, then we predicted that mobbing of both cuckoos and magpies would increase over the season in Finland, but in Italy mobbing would increase only toward magpies.
Materials and Methods
Study Area and Host Populations
We studied reed warblers nesting in 30 reed-lined bays along 100 km of the southern coast of Finland, near Helsinki (from 60.19848N 24.07305E to 60.34039N 25.71162E, EPSG:3857). From mid-May until mid-July in 2019 and 2020, we searched potential nesting sites by locating singing males and observing behavior. The majority of nests were found during building or egg-laying so their exact date of clutch initiation was known (2019: 50 nests, 2020: 62 nests), however, a small number of nests were found during incubation and we used the hatching date of the eggs to back-date their clutch initiation. Presentations of cuckoos at these latter nests were only included in the main dataset if they occurred within 7 days of clutch initiation (8 nests, all in 2019).
Reed warblers breed at low density in Finland and their territories are distributed sparsely (shortest distances among nests measured using GPS coordinates, median (lower quartile, upper quartile) 2019: 57 m (33, 95), 2020: 49 m (27, 77); range, 2019: 15–573, 2020: 9–398), meaning that we could be confident that each pair was tested only once each year. Despite attempts to color-ring as many adults as possible at the end of each breeding season, a small proportion may have been resampled in subsequent years. Nevertheless, annual survival rates of reed warblers are low (Thaxter et al., 2006), we recorded a return rate of 14.3% between 2019 and 2020 for color-ringed adults at our sites), and we expanded our study area in 2020. Therefore, replication of individuals during the study was likely to be low. For details of the study area in Italy, see Campobello and Sealy (2010). In brief, data was collected over 2 years (2004, 2005) from a single site in northern Italy (Natural Reserve of the Valli di Mortizzuolo and surrounding area, Modena, 44.89498N, 11.18025E) where 16% of reed warblers were parasitized during the study (Campobello and Sealy, 2010).
Measuring Mobbing Behavior
At each nest we presented a model of a cuckoo, and at half of the nests this was followed by presentation of a magpie, with at least 1.5 h between presentations. The model was placed adjacent to the nest rim, and we recorded the number of bill snaps and rasp calls within 5 min (intensity) after the arrival of the first reed warbler within 3 m of the nest (latency, s). Bill snaps and rasp calls are correlated with close approach, threat postures and direct attack of cuckoo models (Welbergen and Davies, 2008), and differ according to the threat posed by the model (Duckworth, 1991; Welbergen and Davies, 2008; Campobello and Sealy, 2010). We used the same conservative measure as previous studies (Welbergen and Davies, 2009; Thorogood and Davies, 2012, 2013b) to estimate mobbing propensity (at least 20 combined rasp calls and bill snaps) as this reduces the risk of erroneously identifying the sound of drying and cracking reed as bill snaps, or some “churr” warning calls with rasp calls (Welbergen and Davies, 2008). Repeating our analyses using a less conservative threshold of five combined mobbing calls did not alter our conclusions (see Supplementary Material). We aimed to assess defensive mobbing at each nest on the day the 4th egg was laid. However, reed warblers typically lay 3–5 eggs, so some nests were tested either the day before (N = 35 cuckoo presentations, N = 15 magpie presentations) or the day after (N = 1 for both cuckoo and magpie presentations) clutch completion (with N = 84 cuckoo presentations and N = 41 magpie presentations on day of clutch completion). As incubation usually starts once the penultimate egg is laid (Cramp, 1992) any differences in nest attendance among nests were nevertheless likely to be small. In total, we presented a cuckoo at 120 nests (2019: 58 nests, 2020: 62 nests), and a magpie at 57 nests (2019: 26 nests, 2020: 31 nests).
We used commercially-available plastic models; the cuckoo was 3D-printed ABS plastic (3D QuickPrinting United Kingdom) painted with acrylics by an artist, and the magpie was a hunting decoy (Live Decoys). Our cuckoo models were produced using the same template as independent studies by other research groups (e.g., Marton et al., 2019), and elicited a similar range of mobbing behaviors as studies using taxidermy models to assess mobbing behavior in reed warblers (e.g., Welbergen and Davies, 2008). The threat presentations were conducted as part of a larger experiment (unpublished) where the experimental design did not alternate the order of presentation of cuckoo and magpie models. To check if this could bias our conclusions, we used data available from a further 27 nests where magpies were presented either before or after a cuckoo using an otherwise identical protocol. There were no significant differences in mobbing responses according to the order in which the magpie was presented (propensity: binomial glm, estimate = −0.406 ± 1.008, z = −0.402, p = 0.687; intensity: negative binomial glm, estimate = −0.278 ± 0.703, z = −0.395, p = 0.693). Furthermore, at another 17 nests where we had presented a magpie twice (without presentation of a cuckoo), there was also no difference in response according to the order of the magpie’s presentation [intensity: paired t(16) = 1.033, P = 0.317]. Therefore, it is unlikely that our approach of comparing mobbing responses toward cuckoos and magpies without accounting for order influenced our results.
Nest presentations in Finland were carried out by three observers, each using a different set of models. To account for potential differences and biases among observers, we filmed each nest presentation with a small action camera mounted on a green pole 1 m from the nest. After the field season we selected a random set of 10 presentations and each observer recorded data from the videos, blind to the data collected in the field. We then calculated the consistency of the observers in their detection of the number of rasps and bill snaps from the videos, using the Intraclass Correlation Coefficient (ICC) in the package “irr” (Gamer et al., 2019) (using R statistical computing environment, see Data analysis methods). All three observers were consistent in detecting the number of rasps [ICC: 0.98 (95%CI 0.95–1.0), p < 0.001] and bill snaps [ICC: 0.92 (95%CI 0.80–0.98), p < 0.001].
Mobbing data were collected differently in Italy (see Campobello and Sealy, 2010 for details). In brief, mobbing behavior toward cuckoos and magpies was elicited using taxidermy models fixed to wooden poles painted green to match the vegetation, both models were presented to nests with at least 20 min between presentations, and alternated randomly. Mobbing intensity was measured in terms of occurrence of rasps and/or bill snaps within 10 s intervals (rather than call rate) and data on the birds’ latency to approach was not available. Therefore, we limited our analyses of the Italian dataset to the birds’ propensity to mob cuckoos and magpies (i.e., either rasps or bill snaps occurred). Although it is unlikely that these methodological differences could generate large differences among sites [e.g., presentations using wooden models elicit similar responses to taxidermy cuckoos when tested within (e.g., Welbergen and Davies, 2008) and among populations at similar risk from parasitism (e.g., Thorogood and Davies, 2013b)], we did not combine Finnish and Italian datasets for formal statistical comparisons. None of the nests included in either the Finnish or Italian datasets were parasitized or depredated at the time mobbing behavior was measured.
Data Analysis
All data analyses were conducted in R (v.3.6.2; R Core Team, 2019). We used generalized linear mixed effects models (GLMMs), implemented using the lme4 package (Bates et al., 2015) and generalized linear models (GLMs) to estimate the relationship between Julian date and each of the mobbing behavior measures (latency to approach, propensity to mob, and mobbing intensity) in Finland and Italy, respectively. For data from Finland, we included a random effect term to account for ecological variation among the different bays within each year (for example, some bays are more impacted by human activity, have larger areas of continuous reed, or are closer to forest areas with cuckoo activity). We checked for the most appropriate model error structure for each mobbing measure (i.e., the response variable) by using QQ-normality plots, residuals vs. predicted plots, and dispersion and outlier tests (as implemented in the DHARMa package (Hartig, 2020) on the most complex model (see below). Propensity to mob (yes/no) was modeled using a binomial error distribution, while latency to approach and mobbing intensity (number of rasps and bill snaps per 5 min) were modeled using a negative binomial error distribution (implemented with the glmer.nb function in lme4). Models comparing reed warblers’ propensity to mob magpies versus cuckoos in Finland included a random effect term for nest identity that accounted for repeated presentations, and in analyses of seasonal change of mobbing propensity toward magpies, we included a covariate to account for variation in the number of days elapsed between the mobbing trial and clutch initiation (magpies were occasionally presented later in incubation than cuckoos, see above).
We used a model selection approach to evaluate support for predictions of mobbing behavior to increase during the season in Finland (either linearly or exponentially) but remain constant overall (or vary quadratically) in Italy. For each measure of mobbing, we built a candidate model set where the relationship with date (mean-centered and scaled by standard deviation) was described using a third-order polynomial (Italian data only), a second-order polynomial (i.e., increasing exponentially during the season), or a linear term, as well as a null model. We then ranked these models using their corrected Akaike information criterion (AICc) values (using the AICcmodavg package; Mazerolle, 2020), and accepted strong support for (or against) a relationship if the model was at least 2 AICc values smaller (or larger, respectively) than the next best model, or if the null model had the smallest AICc we accepted inconclusive evidence if the model of interest was no more than 2 AICc values larger (Burnham et al., 2011). We repeated these analyses for data available for mobbing propensity toward a model magpie to check whether behavior varied toward an avian nest predator familiar to reed warblers at both the parasitized and non-parasitized sites. We present the estimated effect sizes and p-values of the best-supported relationships for comparison with previous analyses that included date as a covariate (see Table 1). Summaries of model outputs and analysis codes are available in the Supplementary Material.
Results
As predicted, reed warblers in Finland showed low aggression toward cuckoos: mobbing occurred at 30.8% of nests (37/120) whereas in Italy mobbing occurred at 78.6% of nests (81 out of 103). However, we found little evidence of mobbing behavior changing over the season (Figure 2). Latency to approach the cuckoo showed little change over the season; the model containing the linear term for date was 1.2 AICc units larger than the null model (Table 2A) and the relationship was not significant (negative binomial GLMM, linear term: estimate = 0.103 ± 0.104, z = 0.991, p = 0.322; Figure 2A). A linear relationship with date was also within 1.6 AICc of the null model for mobbing propensity (Table 2B), but again the relationship was not significant (binomial GLMM of linear term: estimate = 0.169 ± 0.245, z = 0.691, p = 0.490; Figure 2B). Finally, at the nests where reed warblers mobbed the cuckoo (N = 37), there was no change in their intensity of mobbing calls: no model was within 2 AICc of the null model (Table 2C, negative binomial GLMM of linear term: estimate = 0.012 ± 0.164, z = 0.076, p = 0.940; Figure 2D).
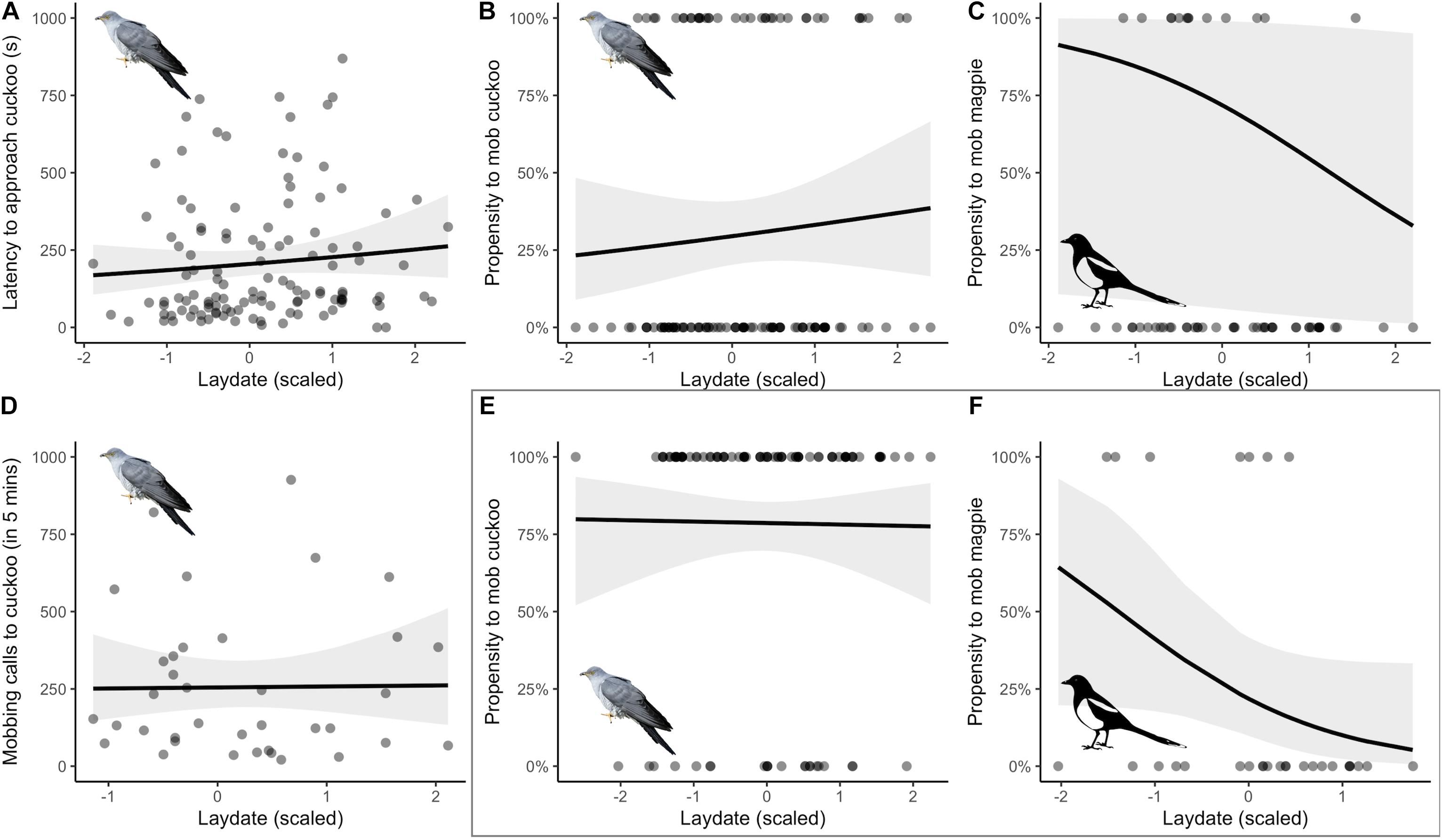
Figure 2. Investigating potential effects of re-nesting potential (estimated using laying date scaled relative to breeding season) on reed warblers’ (A) latency to approach (s) and (B,E) propensity to mob (using bill snaps and rasp calls) a cuckoo model presented at the nest, and (C,F) propensity to mob a model nest predator (magpie). (D) presents the intensity of mobbing (number of calls per 5 min) at nests with positive propensity in panel (B). Panels (A–D) represent behavior at non-parasitized sites in Finland, (E,F) (in box) show data from parasitized sites in Italy (previously published in Campobello and Sealy, 2010). Solid lines (±95% confidence intervals, shaded) indicate the relationship from generalized linear mixed effect models (A–D) and generalized linear models (E,F); no relationships were significant (see Table 2).
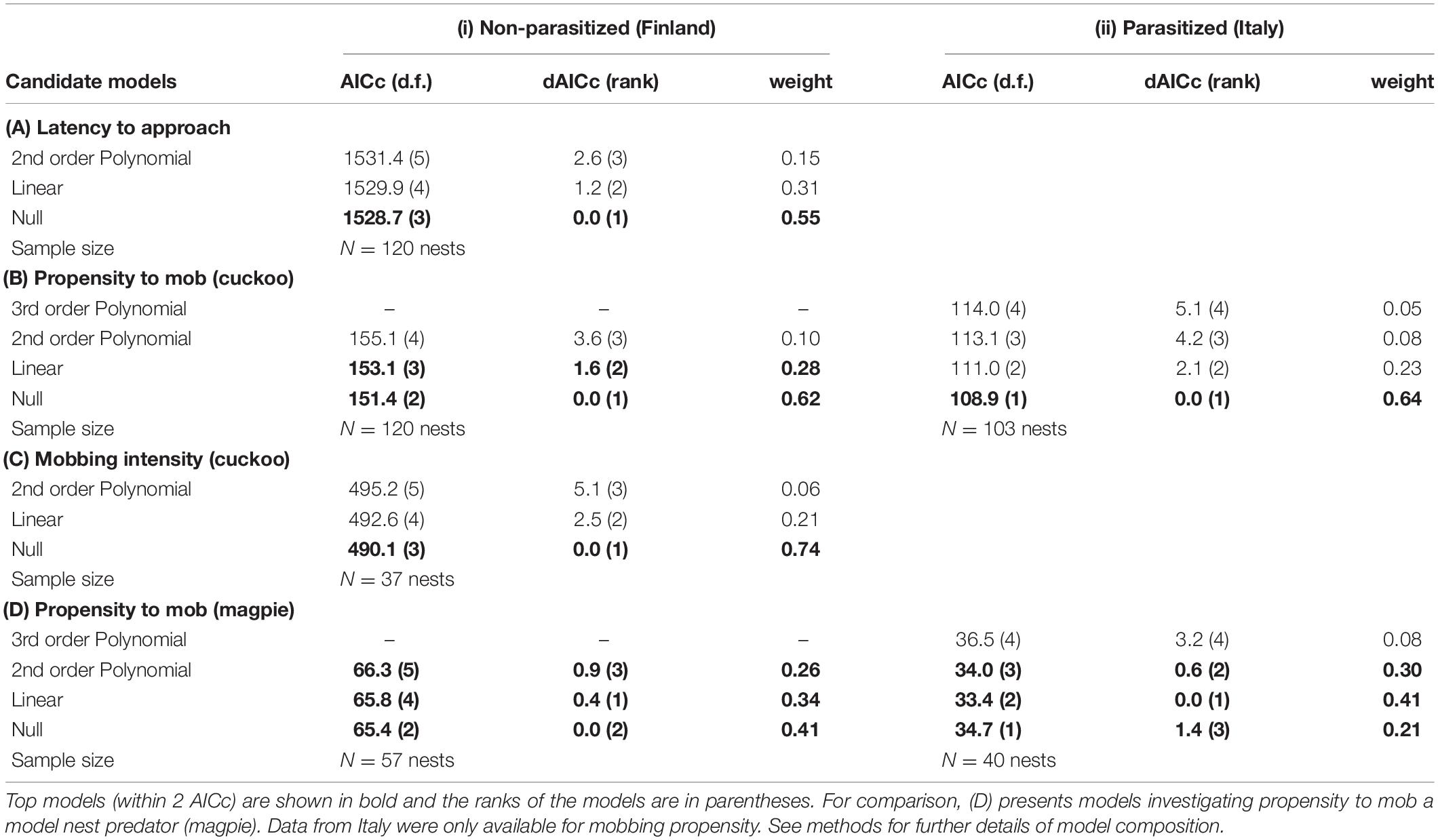
Table 2. Akaike Information Criterion (corrected for small sample size) values (AICc) for generalized linear mixed effects models investigating relationship between laying date (scaled relative to breeding season) and reed warblers’ (A) latency to approach (s), (B) propensity to mob (using bill snaps and rasp calls), and (C) intensity of mobbing (number of calls per 5 min) toward a cuckoo model presented at the nest at (i) non-parasitized sites in Finland and (ii) parasitized sites in Italy.
The lack of significant relationships with season was not because reed warblers in Finland failed to recognize our model cuckoo as a threat to their reproductive success. Reed warblers were more likely to mob the cuckoo than a magpie nest predator (of 57 nests tested with both models, 33.3% mobbed cuckoos vs. 22.8% mobbed magpies; binomial GLMM, estimate = −8.103 ± 2.446, z = −3.313, p < 0.001), and by comparison their propensity to mob magpies showed some weak evidence to decline through the season (Table 2D; binomial GLMM of linear term: estimate = −0.516 ± 0.402, z = −1.284, p = 0.199; Figure 2C). However, this difference among model types was not significant (GLMM of linear term in interaction with model type: dAICc = 2.0 greater than model without interaction, estimate = 1.083 ± 2.617, z = 0.414, p = 0.679).
Parasitized reed warblers in Italy showed a similar declining propensity across the season to mob magpies, with all three relationships with date being within 2 AICc of the null model (Table 2D; GLM of simplest linear term: estimate = −0.919 ± 0.509, z = −1.808, p = 0.071; Figure 2F). They were also less likely to mob magpies than cuckoos (of 30 nests tested with both models, 83.3% mobbed cuckoos vs. 23.0% mobbed magpies; GLM: estimate = −3.080 ± 0.694, z = −4.438, p < 0.001). However, in Italy there was no evidence of any seasonal change in their propensity to mob cuckoos as any model containing a relationship with date was > 2.1 AICc larger than the null model (Table 2B; GLM of linear term: estimate = −0.0209 ± 0.237, z = −0.121, p = 0.904; Figure 2E).
Discussion
While theory predicts that mobbing might increase toward brood parasites and nest predators through the season due to the diminishing opportunities for a pair to re-nest (Barash, 1975; Montgomerie and Weatherhead, 1988), here we found little evidence that this occurs in reed warblers. Our information theoretic approach suggested that seasonal change in the propensity to mob cuckoos was more ambiguous in non-parasitized sites in Finland (within 2 AICc of the null model, Burnham et al., 2011) than at a parasitized site in Italy. However, at both sites reed warbler’s remained as likely to mob a cuckoo at the end of the breeding season as they did when their potential to re-nest was high at the start. Interestingly, mobbing toward magpies also showed little change, and in fact tended to decline rather than increase over the season.
We ceased experiments at both non-parasitized and parasitized sites as nests became scarce later in the season. However, some clutches could possibly have been laid after our study period and this might have reduced our ability to detect a trend associated with re-nesting potential. Nevertheless, our results were similar to the five previous studies of reed warblers’ mobbing where date was included in analyses (Table 1), and to several studies of mobbing by the more aggressive great reed warbler (Acrocephalus arundinaceus) that is also often used as a host by the common cuckoo [(e.g., mobbing of cuckoos: Honza et al., 2010; Trnka et al., 2012; Trnka and Grim, 2014); mobbing of magpies: Trnka and Požgayová, 2017)]. Furthermore, the propensity of reed warblers to mob in Finland was similar to previous studies at locations where cuckoo activity is absent (e.g., 31% compared to 25% at Llangorse Lake, Wales United Kingdom.: Welbergen and Davies, 2012). Since our results are analogous to many other studies of mobbing by reed warblers, and that responses to painted models are similar to taxidermy versions (Welbergen and Davies, 2008) as well as responses to live cuckoos (Tryjanowski et al., 2018), we can assume that our model cuckoos and magpies were sufficiently accurate stimuli to detect variation. Why then do reed warblers not modify their mobbing behavior through the season?
Re-nesting potential is not the only determinant of the value of a current reproductive attempt for parents. For example, parents are also predicted to decide whether to risk mobbing an intruder based on the probability that their current brood will survive to breed themselves (Trivers, 1972). Although this theory is generally interpreted to represent increasing reproductive value as offspring progress toward independence (Shew et al., 2016), many bird species have a greater probability of fledging young when breeding earlier in the season (Grant et al., 2005; Grüebler and Naef-Daenzer, 2010). If offspring survival decreases through the season then this could make eggs laid earlier more valuable for parents to defend, regardless of the nest intruder’s identity. Indeed, one of the few studies to consider potentially opposing forces of selection found that variation in nest defense by red-winged blackbirds was best explained by an interaction between re-nesting potential and parental investment (Shew et al., 2016). For example, increasing predation risk or worsening rearing conditions could counter-act high re-nesting potential and elevate mobbing early in the season (i.e., increasing mobbing away from the green dashed line in Figure 1). This would then generate more consistent patterns of mobbing across the season than we would otherwise expect.
Besides altering the value of the brood, changing predation risk may also modify mobbing behavior via informational constraints. Nest defense is traditionally considered costly only to the parent, but mobbing calls can, paradoxically, endanger the nest by attracting other predators and brood parasites to the nest (Marton et al., 2019). If predator abundance increases through the season, warblers may be less prone to mob to avoid alerting other threats to the location of their brood, as opposed to favoring their own survival. In other words, this would suppress the predicted effects of declining renesting potential later in the season (i.e., reducing mobbing away from the solid green line in Figure 1). Measures of mobbing might thus not fully reflect parental investment in a brood since there is a trade-off between deterring a current threat and avoiding further threats in the future (in addition to the trade-off between current and future investment; Trivers, 1972). Parents might also use less detectable nest defense behaviors, such as by increasing nest attendance (Samelius and Alisauskas, 2001) rather than noisy vocal displays, to mitigate against the risk of attracting attention to the nest. Here we assessed attentiveness through latency to approach the nest, but this can be affected by the perceived risk of the nest intruder [e.g., reed warblers are slower to approach a sparrowhawk at the nest (Duckworth, 1991); including after warnings by neighbors (Thorogood and Davies, 2012)]. Unfortunately, seasonal patterns of predation incurred by reed warblers in Finland are largely unexplored, and elsewhere show variation among sites and years (e.g., no seasonal trend: Honza et al., 1998; three seasons decreasing, two increasing, and one with no seasonal trend of predation: Halupka et al., 2014). More in-depth investigation of other aspects of nest defenses against cuckoos, combined with analyses incorporating changing predation risk (e.g., Lima, 2009), may therefore help to uncover why mobbing behavior does not appear to change over the season.
Finally, we may not have detected seasonal changes in mobbing behavior if re-nesting potential was experienced unequally among individuals. Theoretically, re-nesting potential should shape nest defense according to (1) the time before a parent can make another breeding attempt and (2) the parent’s probability of survival (Montgomerie and Weatherhead, 1988; Shew et al., 2016). However, individual differences in intrinsic behavior, condition, age and experience are unlikely to be consistent across the season, and each of these could influence mobbing behavior directly (e.g., Avilés and Parejo, 2011), as well as timing of breeding and probability of survival. For example, personality can influence nest defense and survival (Vrublevska et al., 2015), including mobbing (Trnka and Grim, 2014) and egg rejection (Zhang et al., 2021) by hosts, and breeding phenology (Abbey-Lee and Dingemanse, 2019) and survival to breed again (Smith and Blumstein, 2008) can covary with differences in boldness and exploration behavior. Older individuals are also expected to take more risks as the value of their current brood increases relative to potential future breeding attempts (e.g., asset-protection principle, Clark, 1994; de Jong et al., 2021), even in relatively short-lived passerines (e.g., Class and Brommer, 2016). Indeed, older birds are more likely to reject cuckoo eggs (e.g., magpies, Pica pica: Martínez et al., 2020; great reed warbler: Lotem et al., 1992). Although reed warblers arrive on the breeding grounds in successive waves, with earlier arriving birds being typically older and more familiar with local conditions (as assessed by intensive bird ringing and monitoring, Chernetsov, 1999), it is impossible to age adult reed warblers accurately by plumage or morphometrics to incorporate age-specific survival probabilities into our study, and unfortunately it is not feasible to track individuals through multiple breeding attempts across years. Nor do we have data available on seasonal variation in personality. Integrating individual differences among breeders into studies of re-nesting potential is, however, likely to be an important next step in explaining why some studies find support for this theory (e.g., Hollander et al., 2008) while others do not (e.g., our study; Weatherhead, 1979, 1989; Thornhill, 1989).
Conclusion
Although reed warblers do adjust mobbing behavior adaptively according to reproductive value in terms of breeding stage (Duckworth, 1991; Campobello and Sealy, 2010), and fine-tune mobbing based on local variation in parasitism (Thorogood and Davies, 2013b), here we find no evidence of seasonal change in either a parasitised or a non-parasitised population. While this might suggest that re-nesting potential cannot explain the lack of seasonal trends previously described in parasitised populations (Table 1), there is still a need for quantitative modeling with realistic parameters, informed by field data, to explore the relative magnitude of the different effects of re-nesting potential, parasitism risk, and offspring survival on parental investment in nest defense. Climate change is altering both the onset and length of breeding seasons for birds, and these effects appear to vary across species (Halupka and Halupka, 2017; Hällfors et al., 2020). Reed warblers in particular have lengthened their breeding season (Halupka et al., 2008), potentially contributing to mismatches in phenology with cuckoos (Saino et al., 2009) while increasing hosts’ opportunities to re-nest. Further work is therefore required to determine whether ecological factors and individual traits interact with the length of the breeding season to shape the expression of defenses and the consequent effects on both host and cuckoo population dynamics.
Data Availability Statement
The raw data supporting the conclusions of this article will be made available by the authors, without undue reservation.
Ethics Statement
The animal study was reviewed and approved by Project Authorization Board of the Regional State Administrative Agency (ESAVI/7857/2018) and conducted under permission of Centre for Economic Development, Transport and the Environment (VARELY/758/2018 and VARELY/799/2019).
Author Contributions
EK, DT, KR, and RT conceived and designed the study. EK, KR, and RT collected the data in Finland. DC contributed previously published data from Italy. DT analyzed the data with assistance from EK and RT. DT and RT wrote the manuscript with contributions from EK, KR, DC, and RT supervised the research. All authors contributed to the manuscript and approved the submitted version.
Funding
This research was supported by Academy of Finland Project grant 1333803 and a start up grant to RT from HiLIFE Helsinki Institute of Life Science.
Conflict of Interest
The authors declare that the research was conducted in the absence of any commercial or financial relationships that could be construed as a potential conflict of interest.
Publisher’s Note
All claims expressed in this article are solely those of the authors and do not necessarily represent those of their affiliated organizations, or those of the publisher, the editors and the reviewers. Any product that may be evaluated in this article, or claim that may be made by its manufacturer, is not guaranteed or endorsed by the publisher.
Acknowledgments
In Finland we would like to thank field assistants Julius Mäkinen, Juho Jolkkonen, Anna Tuominen, Anna Välkki, Purabi Deshpande, and Nora Bergman for their essential help with nest searching and monitoring, Steve Collett for painting the model cuckoos, and Pang (Peter) Bowen who conducted preliminary analyses. We would also like to thank private land owners, Uusimaa municipalities, and Metsähallitus (permission no. MH1100/2018/06.06.02) for allowing access to reed beds. In Italy we thank the Modena Ornithological Station for their valuable logistic support.
Supplementary Material
The Supplementary Material for this article can be found online at: https://www.frontiersin.org/articles/10.3389/fevo.2021.725467/full#supplementary-material
Supplementary Data Sheet | Code for statistical analyses and results.
References
Abbey-Lee, R. N., and Dingemanse, N. J. (2019). Adaptive individual variation in phenological responses to perceived predation levels. Nat. Commun. 10:1601. doi: 10.1038/s41467-019-09138-5
Avilés, J. M., and Parejo, D. (2011). Host personalities and the evolution of behavioural adaptations in brood parasitic–host systems. Anim. Behav. 82, 613–618. doi: 10.1016/j.anbehav.2011.07.025
Barash, D. P. (1975). Evolutionary aspects of parental behavior: distraction behavior of the alpine accentor. Wilson Bull. 87, 367–373.
Bates, D., Mächler, M., Bolker, B., and Walker, S. (2015). Fitting linear mixed-effects models using lme4. J. Stat. Softw. 67, 1–48. doi: 10.18637/jss.v067.i01
Brooke, M., de, L., Davies, N. B., and Noble, D. G. (1998). Rapid decline of host defences in response to reduced cuckoo parasitism: behavioural flexibility of reed warblers in a changing world. Proc. R. Soc. Lond. B 265, 1277–1282. doi: 10.1098/rspb.1998.0430
Burnham, K. P., Anderson, D. R., and Huyvaert, K. P. (2011). AIC model selection and multimodel inference in behavioral ecology: some background, observations, and comparisons. Behav. Ecol. Sociobiol. 65, 23–35. doi: 10.1007/s00265-010-1029-6
Campobello, D., and Sealy, S. G. (2010). Enemy recognition of reed warblers (Acrocephalus scirpaceus): threats and reproductive value act independently in nest defence modulation. Ethology 116, 498–508. doi: 10.1111/j.1439-0310.2010.01764.x
Campobello, D., and Sealy, S. G. (2018). Evolutionary significance of antiparasite, antipredator and learning phenotypes of avian nest defence. Sci. Rep. 8:10569. doi: 10.1038/s41598-018-28275-3
Čapek, M., Požgayová, M., Procházka, P., and Honza, M. (2010). Repeated presentations of the Common Cuckoo increase nest defense by the Eurasian Reed Warbler but do not induce it to make recognition errors. Condor 112, 763–769. doi: 10.1525/cond.2010.100063
Caro, T. (2005). Antipredator Defenses In Birds And Mammals. Chicago IL: University of Chicago Press.
Chernetsov, N. (1999). Timing of spring migration, body condition, and fat score in local and passage populations of the Reed Warbler Acrocephalus scirpaceus on the Courish Spit. Avian Ecol. Behav. 2, 75–88.
Clark, C. W. (1994). Antipredator behavior and the asset-protection principle. Behav. Ecol. 5, 159–170. doi: 10.1093/beheco/5.2.159
Class, B., and Brommer, J. E. (2016). Senescence of personality in a wild bird. Behav. Ecol. Sociobiol. 70, 733–744. doi: 10.1007/s00265-016-2096-0
Cramp, S. (1992). in Handbook of the Birds of Europe, the Middle East and North Africa: The Birds of the Western Palearctic, Vol. Volume, ed. S. Cramp (Oxford: Oxford University Press), 6.
de Jong, M. E., Nicolaus, M., Fokkema, R. W., and Loonen, M. J. J. E. (2021). State dependence explains individual variation in nest defence behaviour in a long-lived bird. J. Anim. Ecol. 90, 809–819. doi: 10.1111/1365-2656.13411
Duckworth, J. W. (1991). Responses of breeding Reed Warblers Acrocephalus scirpaceus to mounts of Sparrowhawk Accipiter nisus. Cuckoo Cuculus canorus and Jay Garrulus glandarius. IBIS 133, 68–74. doi: 10.1111/j.1474-919X.1991.tb04812.x
Feeney, W. E. (2017). “Evidence of adaptations and counter-adaptations before the parasite lays its egg: the frontline of the arms race,” in Avian Brood Parasitism: Behaviour, Ecology, Evolution and Coevolution, ed. M. Soler (Berlin: Springer), 307–324. doi: 10.1007/978-3-319-73138-4_17
Feeney, W. E., Troscianko, J., Langmore, N. E., and Spottiswoode, C. N. (2015). Evidence for aggressive mimicry in an adult brood parasitic bird, and generalized defences in its host. Proc. R. Soc. B 282:20150795. doi: 10.1098/rspb.2015.0795
Feeney, W. E., Welbergen, J. A., and Langmore, N. E. (2012). The frontline of avian brood parasite–host coevolution. Anim. Behav. 84, 3–12. doi: 10.1016/j.anbehav.2012.04.011
Gamer, M., Lemon, J., Fellows, I., and Singh, P. (2019). irr: Various Coefficients of Interrater Reliability and Agreement. R package version 0.84.1. Available online at: https://cran.r-project.org/package=irr (accessed January 26, 2019).
Gloag, R., Fiorini, V. D., Reboreda, J. C., and Kacelnik, A. (2013). The wages of violence: mobbing by mockingbirds as a frontline defence against brood-parasitic cowbirds. Anim. Behav. 86, 1023–1029. doi: 10.1016/j.anbehav.2013.09.007
Grant, T. A., Shaffer, T. L., Madden, E. M., and Pietz, P. J. (2005). Time-specific variation in passerine nest survival: new insights into old questions. Auk 122, 661–672. doi: 10.1093/auk/122.2.661
Grüebler, M. U., and Naef-Daenzer, B. (2010). Fitness consequences of timing of breeding in birds: date effects in the course of a reproductive episode. J. Avian Biol. 41, 282–291. doi: 10.1111/j.1600-048X.2009.04865.x
Hällfors, M. H., Antaõ, L. H., Itter, M., Lehikoinen, A., Lindholm, T., Roslin, T., et al. (2020). Shifts in timing and duration of breeding for 73 boreal bird species over four decades. Proc. Natl. Acad. Sci. U.S.A. 117, 18557–18565. doi: 10.1073/pnas.1913579117
Halupka, L., Dyrcz, A., and Borowiec, M. (2008). Climate change affects breeding of reed warblers Acrocephalus scirpaceus. J. Avian Biol. 39, 95–100. doi: 10.1111/j.2008.0908-8857.04047.x
Halupka, L., and Halupka, K. (2017). The effect of climate change on the duration of avian breeding seasons: a meta-analysis. Proc. R. Soc. B 284:20171710. doi: 10.1098/rspb.2017.1710
Halupka, L., Halupka, K., Klimczuk, E., and Sztwiertnia, H. (2014). Coping with shifting nest predation refuges by European reed warblers Acrocephalus scirpaceus. PLoS One 9:e115456. doi: 10.1371/journal.pone.0115456
Hamao, S. (2011). Seasonal increase in intensity of nest defence against little cuckoos by Japanese bush warblers. Anim. Behav. 82, 869–874. doi: 10.1016/j.anbehav.2011.07.026
Hartig, F. (2020). DHARMa: Residual Diagnostics for Hierarchical (Multi-Level / Mixed) Regression Models. R package version 0.3.3.0.
Hollander, F. A., Van Overveld, T., Tokka, I., and Matthysen, E. (2008). Personality and nest defence in the great tit (Parus major). Ethology 114, 405–412. doi: 10.1111/j.1439-0310.2008.01488.x
Honza, M., Øien, I. J., Moksnes, A., and Røskaft, E. (1998). Survival of reed warbler Acrocephalus scirpaceus clutches in relation to nest position. Bird Study 45, 104–108. doi: 10.1080/00063659809461083
Honza, M., Procházka, P., Šicha, V., and Požgayová, M. (2010). Nest defence in a cuckoo host: great reed warblers risk themselves equally for their own and parasitic chicks. Behaviour 147, 741–756. doi: 10.1163/000579510X491081
Krama, T., and Krams, I. (2005). Cost of mobbing call to breeding pied flycatcher. Ficedula hypoleuca. Behav. Ecol. 16, 37–40. doi: 10.1093/beheco/arh116
Lima, S. L. (2009). Predators and the breeding bird: behavioral and reproductive flexibility under the risk of predation. Biol. Rev. 84, 485–513. doi: 10.1111/j.1469-185X.2009.00085.x
Lindholm, A. K., and Thomas, R. J. (2000). Differences between populations of reed warblers in defences against brood parasitism. Behaviour 137, 25–42. doi: 10.1163/156853900501854
Lotem, A., Nakamura, H., and Zahavi, A. (1992). Rejection of cuckoo eggs in relation to host age: a possible evolutionary equilibrium. Behav. Ecol. 3, 128–132. doi: 10.1093/beheco/3.2.128
Martínez, J. G., Molina-Morales, M., Precioso, M., and Avilés, J. M. (2020). Age-related brood parasitism and egg rejection in magpie hosts. Am. Natural. 195, 876–885. doi: 10.1086/708155
Marton, A., Fülöp, A., Ozogány, K., Moskát, C., and Bán, M. (2019). Host alarm calls attract the unwanted attention of the brood parasitic common cuckoo. Sci. Rep. 9:18563. doi: 10.1038/s41598-019-54909-1
Mazerolle, M. J. (2020). AICcmodavg: Model Selection and Multimodel Inference Based On (Q)AIC(c). R Package Version 2.3-1.
Moksnes, A., Braa, A., and Korsnes, L. (1991). Behavioural responses of potential hosts towards artificial cuckoo eggs and dummies. Behaviour 116, 64–89. doi: 10.1163/156853990x00365
Montgomerie, R. D., and Weatherhead, P. J. (1988). Risks and rewards of nest defence by parent birds. Q. Rev. Biol. 63, 167–187. doi: 10.1086/415838
Oteyza, J. C., Mouton, J. C., and Martin, T. E. (2021). Adult survival probability and body size affect parental risk-taking across latitudes. Ecol. Lett. 24, 20–26. doi: 10.1111/ele.13615
R Core Team (2019). R: A Language And Environment For Statistical Computing. Vienna: R Foundation for Statistical Computing.
Røskaft, E., Moksnes, A., Stokke, B. G., Bicik, V., and Moskat, C. (2002). Aggression to dummy cuckoos by potential European cuckoo hosts. Behaviour 139, 613–628. doi: 10.1163/15685390260136735
Saino, N., Rubolini, D., Lehikoinen, E., Sokolov, L. V., Bonisoli-Alquati, A., Ambrosini, R., et al. (2009). Climate change effects on migration phenology may mismatch brood parasitic cuckoos and their hosts. Biol. Lett. 5, 539–541. doi: 10.1098/rsbl.2009.0312
Samelius, G., and Alisauskas, R. T. (2001). Deterring arctic fox predation: the role of parental nest attendance by lesser snow geese. Can. J. Zool. 79, 861–866. doi: 10.1139/cjz-79-5-861
Shew, J. J., van der Merwe, J., Schauber, E. M., Tallitsch, B. K., and Nielsen, C. K. (2016). A classic question revisited in red-winged blackbirds: disentangling confounding hypotheses surrounding parental investment theory and nest defense intensity. Behav. Ecol. Sociobiol. 70, 1843–1856. doi: 10.1007/s00265-016-2190-3
Smith, B. R., and Blumstein, D. T. (2008). Fitness consequences of personality: a meta-analysis. Behav. Ecol. 19, 448–455. doi: 10.1093/beheco/arm144
Stolt, B. D. (1999). The Swedish Reed Warbler Acrocephalus scirpaceus population estimated by a capture-recapture technique. Ornis Svecica 9, 35–46.
Thaxter, C. B., Redfern, C. P. F., and Bevan, R. M. (2006). Survival rates of adult Reed Warblers Acrocephalus scirpaceus at a northern and southern site in England. Ringing Migr. 23, 65–79. doi: 10.1080/03078698.2006.9674347
Thornhill, R. (1989). Nest defense by Red Jungle Fowl (Gallus gallus spadiceus) hens: the roles of renesting potential, parental experience and brood reproductive value. Ethology 83, 31–42. doi: 10.1111/j.1439-0310.1989.tb00517.x
Thorogood, R., and Davies, N. B. (2012). Cuckoos combat socially transmitted defenses of reed warbler hosts with a plumage polymorphism. Science 337, 578–580. doi: 10.1126/science.1220759
Thorogood, R., and Davies, N. B. (2013a). Hawk mimicry and the evolution of polymorphic cuckoos. Chin. Birds 4, 39–50. doi: 10.5122/cbirds.2013.0002
Thorogood, R., and Davies, N. B. (2013b). Reed warbler hosts fine-tune their defenses to track three decades of cuckoo decline. Evolution 67, 3545–3555. doi: 10.1111/evo.12213
Trivers, R. L. (1972). in Parental Investment and Sexual Selection, ed. B. Campbell (Venice: Aldine Press), 136–179.
Trnka, A., and Grim, T. (2014). Testing for correlations between behaviours in a cuckoo host: why do host defences not covary? Anim. Behav. 92, 185–193. doi: 10.1016/j.anbehav.2014.04.006
Trnka, A., and Požgayová, M. (2017). Does conspicuous colouration of Magpies Pica pica influence aggressive behaviour in nesting Great Reed Warblers Acrocephalus arundinaceus? Bird Study 64, 108–111. doi: 10.1080/00063657.2016.1273878
Trnka, A., Prokop, P., and Grim, T. (2012). Uncovering dangerous cheats: how do avian hosts recognize adult brood parasites? PLoS One 7:e37445. doi: 10.1371/journal.pone.0037445
Tryjanowski, P., Morelli, F., Kwieciǹski, Z., Indykiewicz, P., and Møller, A. P. (2018). Birds respond similarly to taxidermic models and live cuckoos Cuculus canorus. J. Ethol. 36, 243–249. doi: 10.1007/s10164-018-0554-z
Vrublevska, J., Krama, T., Rantala, M. J., Mierauskas, P., Freeberg, T. M., and Krams, I. A. (2015). Personality and density affect nest defence and nest survival in the great tit. Acta Ethol. 18, 111–120. doi: 10.1007/s10211-014-0191-7
Weatherhead, P. J. (1979). Do savannah sparrows commit the concorde fallacy? Behav. Ecol. Sociobiol. 5, 373–381. doi: 10.1007/BF00292525
Weatherhead, P. J. (1989). Nest defence by song sparrows: methodological and life history considerations. Behav. Ecol. Sociobiol. 25, 129–136. doi: 10.1007/BF00302929
Welbergen, J. A., and Davies, N. B. (2008). Reed warblers discriminate cuckoos from sparrowhawks with graded alarm signals that attract mates and neighbours. Anim. Behav. 76, 811–822. doi: 10.1016/j.anbehav.2008.03.020
Welbergen, J. A., and Davies, N. B. (2009). Strategic variation in mobbing as a front line of defense against brood parasitism. Curr. Biol. 19, 235–240. doi: 10.1016/j.cub.2008.12.041
Welbergen, J. A., and Davies, N. B. (2011). A parasite in wolf’s clothing: hawk mimicry reduces mobbing of cuckoos by hosts. Behav. Ecol. 22, 574–579. doi: 10.1093/beheco/arr008
Welbergen, J. A., and Davies, N. B. (2012). Direct and indirect assessment of parasitism risk by a cuckoo host. Behav. Ecol. 23, 783–789. doi: 10.1093/beheco/ars031
Williams, G. C. (1966). Natural selection, the costs of reproduction, and a refinement of Lack’s principle. Am. Natural. 100, 687–690. doi: 10.1086/282461
Keywords: frontline defense, seasonal change, re-nesting potential, parental investment, avian brood parasitism
Citation: Tolman D, Campobello D, Rönkä K, Kluen E and Thorogood R (2021) Reed Warbler Hosts Do Not Fine-Tune Mobbing Defenses During the Breeding Season, Even When Cuckoos Are Rare. Front. Ecol. Evol. 9:725467. doi: 10.3389/fevo.2021.725467
Received: 15 June 2021; Accepted: 28 July 2021;
Published: 16 August 2021.
Edited by:
Canchao Yang, Hainan Normal University, ChinaReviewed by:
Micahel Sorenson, Boston University, United StatesJesús Miguel Avilés, Consejo Superior de Investigaciones Científicas (CSIC), Spain
Haitao Wang, Northeast Normal University, China
Copyright © 2021 Tolman, Campobello, Rönkä, Kluen and Thorogood. This is an open-access article distributed under the terms of the Creative Commons Attribution License (CC BY). The use, distribution or reproduction in other forums is permitted, provided the original author(s) and the copyright owner(s) are credited and that the original publication in this journal is cited, in accordance with accepted academic practice. No use, distribution or reproduction is permitted which does not comply with these terms.
*Correspondence: Rose Thorogood, rose.thorogood@helsinki.fi