- 1State Key Laboratory of Agricultural Ecology of Black Soil Region, Northeast Institute of Geography and Agroecology, Chinese Academy of Sciences, Changchun, China
- 2Jilin Provincial Key Laboratory of Plant Resource Science and Green Production, Jilin Normal University, Siping, China
- 3State Key Laboratory of Desert and Oasis Ecology, Xinjiang Institute of Ecology and Geography, Chinese Academy of Sciences, Ürümqi, China
- 4Shandong Key Laboratory of Eco-Environmental Science for Yellow River Delta, Binzhou University, Binzhou, China
Soil salinity is important abiotic stress affecting various ecosystems worldwide such as grassland. Distinct ecotypes often evolve within species by natural selection to facilitate adaptation to different types of environmental stress. Leymus chinensis is a perennial rhizomatous grass that is widely distributed in the eastern Eurasian steppe; it has two main ecotypes, namely, yellow-green (YG) and gray-green (GG), which differ in their strategy for coping with salinity stress. Few studies have examined the seed germination of the two ecotypes under salinity stress. In this study, the seed germination and seedling growth of two ecotypes of L. chinensis in response to different levels of salinity (NaCl) stress [0 (control), 20, 50, 100, and 200 mM] were examined. Then, ungerminated seeds were placed under normal conditions to evaluate seedling growth following exposure to salt stress (i.e., regermination). The germination percentage was significantly higher, and the mean germination time was significantly shorter in the GG ecotype than in the YG ecotype at all NaCl concentrations. As the salinity level increased, the radicle length of the two ecotypes decreased; however, GG had longer radicles and a higher number of radicles, even at 200 mM NaCl when no radicle protruding from the seed coat was detected in YG. The shoot length of GG was significantly longer than that of YG at all NaCl levels. After salinity stress was removed, the seed germination percentage increased as the original concentration of NaCl applied increased, but the total germination percentage did not significantly differ among NaCl concentrations. The total seed germination percentage of GG was approximately 80%, whereas that of the YG was approximately 20%. The seedling length of regerminated seeds for both GG and YG was similar. The thousand-grain weight of GG was significantly higher than that of YG. GG was more salt-tolerant than YG and might be better capable of surviving in harsher environments, suggesting that GG might be particularly useful for saline grassland restoration.
Introduction
Salinity is an important cause of abiotic stresses in various ecosystems, especially agriculture and grassland (Cramer et al., 2011; Tavakkoli et al., 2011). Increases in temperature associated with climate change have caused evaporation to exceed precipitation, and this has resulted in a gradual increase in soil salinity. The exacerbation of soil salinity poses a serious threat to the development of agriculture and horticulture (Yang and Wang, 2015; Qadir, 2016). Understanding the response of plants to the changes in the external environment is essential for improving agriculture and land management, as well as predicting how plants will respond to climate change (Chaves et al., 2003; Randin et al., 2009).
Natural selection has led to the evolution of different ecotypes in many plant species to improve adaptation to environmental stress, such as salinity, drought, and temperature (Chaves et al., 2003; Hameed and Ashraf, 2008; Randin et al., 2009). Ecotypes can vary in their salt tolerance, and variation in ecotypes is associated with variation in physiological, anatomical, and molecular adaptive strategies (Chen and Wang, 2009; Kheloufi et al., 2019). Seeds are often collected from natural habitats, and environmental factors such as soil salinity, vegetation composition, or the conditions of mother plants might affect some of the physiological and morphological characteristics of seeds (Hameed and Ashraf, 2008; Kheloufi et al., 2019). The purpose of common garden experiments is to subject individuals collected from different habitats to the same growth environment to eliminate the effect of environmental factors (Bender et al., 2002).
Seed germination and seedling growths are the first and the most vulnerable stages in the life cycle of plants, and these stages are especially important for plants growing in saline soil (Ungar, 1995; Kitajima and Fenner, 2000; El-Keblawy and Al-Rawai, 2005). Salts in the soil mainly inhibit these two important stages through osmotic stress, ion toxicity, and nutrient deficiency (Ashraf, 1993; Ashraf and Harris, 2004). The best approach for dealing with saline soil and restoring degraded grassland ecosystems is to screen for plants with higher salt tolerance, as these plants have physiological and biochemical mechanisms that allow them to grow under various types of environmental stresses (Maggio et al., 2000; Tipirdamaz et al., 2006). Generally, plants can adapt to environmental stress by either evolving new ecotypes by natural selection or changing their phenotype to match the environment by phenotypic plasticity (Jump and Penuelas, 2005; Hoffmann and Sgrò, 2011; Anderson et al., 2012).
Leymus chinensis is a perennial rhizome and salt-tolerant grass with high ecological and economic values; it is widely distributed in the eastern Eurasian steppe and mainly occurs in saline-alkali soil in the Songnen Plain in China (Bai et al., 1994; Zhou and Yang, 2003). Previous studies have shown that there are two ecotypes of L. chinensis, namely, gray-green (GG) and yellow-green (YG), which differ in their survival strategies. GG has a wider distribution and is more resistant to salt stress compared with YG (Zhou et al., 2014; Lu et al., 2020). Thus, differences in L. chinensis ecotypes may reflect adaptive evolution (Kawecki and Ebert, 2004; Savolainen et al., 2007; Delker et al., 2010; Liu et al., 2016). Although some studies have examined physiological differences in the response of L. chinensis to salt-alkali stress, few studies have examined differences in the response of L. chinensis seeds of different ecotypes to salinity stress during the germination period (Chen and Wang, 2009; Zhou et al., 2014, 2017).
In this study, two ecotypes of L. chinensis ramets were transplanted from natural Songnen grassland to a common garden in 2018 and cultured for 2 years. Matured seeds were collected from L. chinensis of GG and YG in July 2020. The aim of this study was to investigate (1) the germination of seeds and seedling growth from the GG and YG ecotypes in response to a gradient of NaCl concentrations and (2) the seed germination and seedling growth characteristics following the removal of the salinity.
Materials and Methods
Seed Materials
L. chinensis ramets of GG and YG were collected from Songnen grassland in August 2018 and transplanted to separate common garden plots. The experiments were conducted at the Northeast Institute of Geography and Agricultural Ecology, Chinese Academy of Sciences (125.38° E, 43.98° N, altitude 190 m). There were 25 ramets in each plot and three replicates for each ecotype. After transplantation, ramets were regularly watered, and weeds were removed to ensure the normal growth of L. chinensis. The study site is characterized by a continental monsoon climate with an annual average temperature of 7.1°C and annual precipitation of 663 mm. The soil type is black soil, the average organic matter content is 2.83%, the total N content is 1,368.56 mg·kg−1, and the total P content is 669.18 mg·kg−1 (Zhao et al., 2019).
When the transplanted L. chinensis started to head for the first time in July 2020, 50 mature spikes were collected for each ecotype, placed in a paper bag, and dried at room temperature (25°C, 50% humidity). The length of collected spikes, the number of total seeds, the number of fully developed seeds in each spike, length and width of seeds, and the seed mass were determined for each ecotype.
Germination Test
Seeds of GG and YG were sterilized in 0.1% HgCl2 solution for 10 min, rinsed three times in distilled water, and then dried at room temperature before seed germination. A total of 25 seeds were sown in 9-cm Petri dishes of 0.7% agar-solidified medium containing 0, 20, 50, 100, and 200 mM NaCl. There were four replicates for each treatment; all treatments were incubated at a fluctuating temperature of 28/16°C (12 h light/12 h dark, 26% humidity, and light intensity of 54 μmol m−2 s−1). Seeds were considered germinated when the radicle protruded through the seed coat. The number of seeds germinated was determined every day, and after 30 days of cultivation, the radicle length, seedling length, and radicle numbers were determined. After the germination experiment, ungerminated seeds were transferred to 0.7% water agar and cultivated for another 30 days to determine the germination capacity of different ecotypes of L. chinensis seeds following exposure to salt stress (i.e., regermination).
Mean germination time (MGT) was calculated for each L. chinensis individual using the following formula:
where Gt is the number of seed germination at different times and Dt is the seed germination days corresponding to Gt (Ellis and Roberts, 1980).
where A is the number of seeds germinated initially, B is the number of seeds recovered to germination, and C is the total number of seeds used in the experiment (Khan and Ungar, 1984).
Data Analysis
Generalized linear models (GLMs) with a binomial error structure were used to compare the initial germination and total germination percentage; GLMs with a Poisson error structure were used to analyze the seed traits, MGT, the number of radicles, and the length of radicles and seedlings. Tukey's test was used to determine the significance of differences between treatments (Liyanage and Ooi, 2015; Ma et al., 2018). Figures were drawn with Origin 2019.
Results
Seed Traits of Different L. chinensis Ecotypes
Ecotype significantly affected the length of the spike, the number of spikelets in each spike, and the number of seeds in the spike (p < 0.05). The length and width of YG seeds were larger than the length and width of GG seeds; the thousand-grain weight of YG seeds was less than that of GG seeds; YG had longer spike lengths, with more spikelets and a greater total number of seeds per spike, and the percentage of empty seeds was low (Table 1).
Seed Germination
Effects of Salt Stress on the Seed Germination and MGT of Different L. chinensis Ecotypes
Salt concentration and ecotype had significant effects on the germination of L. chinensis seeds (p < 0.001, Table 2). Ecotypes had significant effects on MGT (p < 0.001, Table 2). In the initial germination, the germination percentage of GG in the control group was the highest (83%), and that in the 200 mM NaCl group was the lowest (5%). YG had the highest germination percentage at 20 mM NaCl (19%); YG did not germinate when the salt concentration exceeded 100 mM (Figure 1). At all salt concentrations, the MGT of GG was shorter than that of YG; the MGT of GG was ~two times shorter than that of YG (Figure 2).
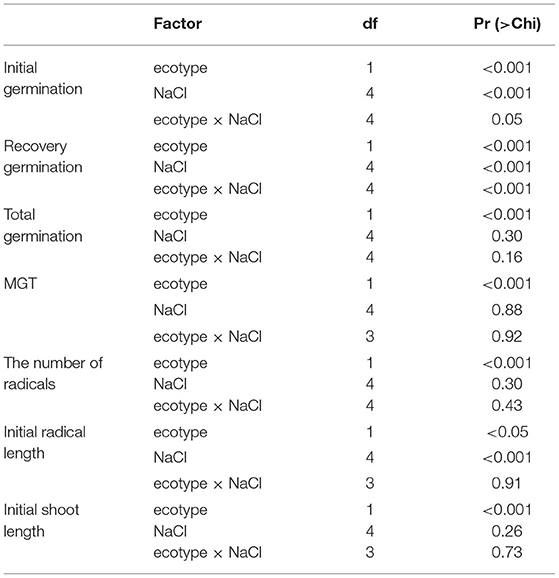
Table 2. Generalized linear models of the effects of ecotypes, salt concentration, and their interactions on the initial and total germination percentage, mean germination time, the number of radicles, and the length of the radicle and shoot during and after salt exposure.
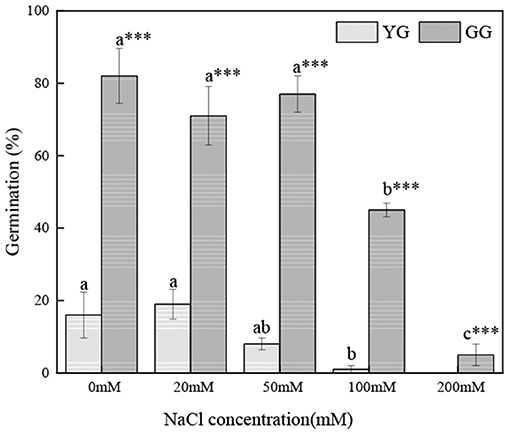
Figure 1. Effects of salt stress on the seed germination of different L. chinensis ecotypes incubated at 28/16°C (12 h light/12 h dark) for 30 d. There were four replicates for each treatment. Values are means ± standard error (SE); Different lowercase letters indicate significant differences between the different NaCl concentrations under the same ecotype. *, **, ***Indicate significant differences between the different ecotypes under the same NaCl concentration at 0.05, 0.01, and 0.001 level respectively, and “ns” indicates no significant differences.
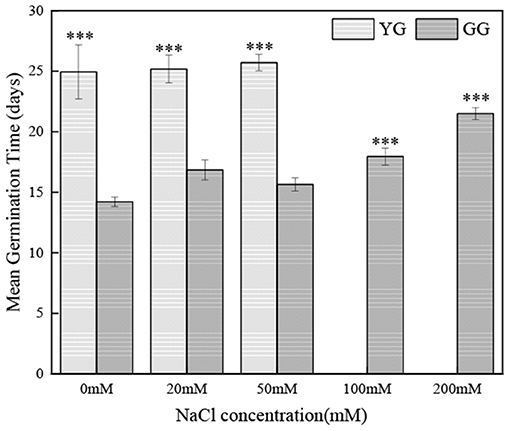
Figure 2. Effects of salt stress on mean germination time of different L. chinensis ecotypes incubated at 28/16°C (12 h light/12 h dark) for 30 d. There were four replicates for each treatment. Values are means ± standard error (SE). *, **, ***Indicate significant differences between the different ecotypes under the same NaCl concentration at 0.05, 0.01, and 0.001 level respectively, and ns indicates no significant differences.
Effects of Salt Stress on the Recovery Germination of Different L. chinensis Ecotypes
Ecotypes, salt concentration, and salt concentration × ecotype significantly affected the regermination percentage (p < 0.001, Table 2). As the pretreatment salt concentration increased, the regermination percentage increased; under the high pretreatment salt concentrations (100 and 200 mM), the regermination percentage of GG was higher than that of YG. Under 200 mM of salt pretreatment, the highest regermination percentage of GG was 66%, whereas that of YG was only 18% (Figure 3).
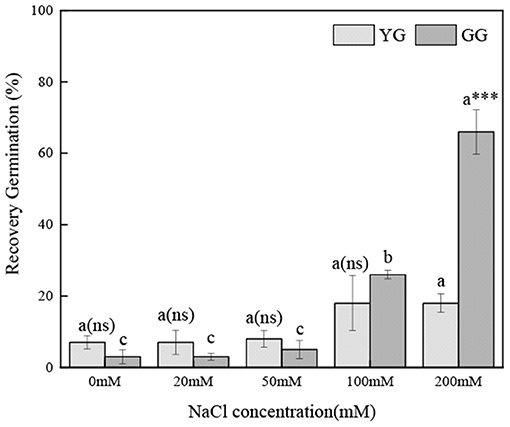
Figure 3. Effects of salt stress on the seed re-germination of different L. chinensis ecotypes incubated at 28/16°C (12 h light/12 h dark) for 30 d. There were four replicates for each treatment. Values are means ± standard error (SE); Different lowercase letters indicate significant differences between the different NaCl concentrations under the same ecotype. *, **, ***Indicate significant differences between the different ecotypes under the same NaCl concentration at 0.05, 0.01, and 0.001 level respectively, and “ns” indicates no significant differences.
Effects of Salt Stress on the Total Germination of Different L. chinensis Ecotypes
Ecotypes had a significant effect on the total germination percentage (p < 0.001, Table 2); the salt concentration had no effect on the total germination percentage. The total germination percentage of GG ranged from 71 to 85% and that of YG ranged from 16 to 23%; the total germination percentage of GG was ~four times greater than that of YG (Figure 4).
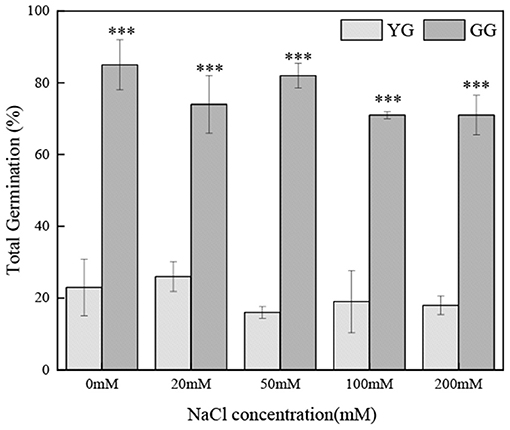
Figure 4. Effects of salt stress on the seed total germination of different L. chinensis ecotypes, incubated at 28/16°C (12 h light/12h dark) for 30 d. There were four replicates for each treatment. Values are means ± standard error (SE). *, **, ***Indicate significant differences between the different ecotypes under the same NaCl concentration at 0.05, 0.01, and 0.001 level respectively, and “ns” indicates no significant differences.
Seedling Growth
Effects of Salt Stress on Radicle Length of Seedlings Grown From Different L. chinensis Ecotypes
Salt concentration significantly affected the radicle length (p < 0.001, Table 2). Ecotypes had effects on the radicle length (p < 0.05, Table 2). As the salt concentration increased, the radicle lengths of GG and YG decreased, but the radicle length of GG was always longer than that of YG (Figure 5A). During regermination, the radicle length of YG was longer than that of GG when the pretreatment concentration was <100 mM. The radicle length of the seedlings during regermination was ~three times longer than the radicle length of the seedlings that germinated under salt stress (Figure 5B).
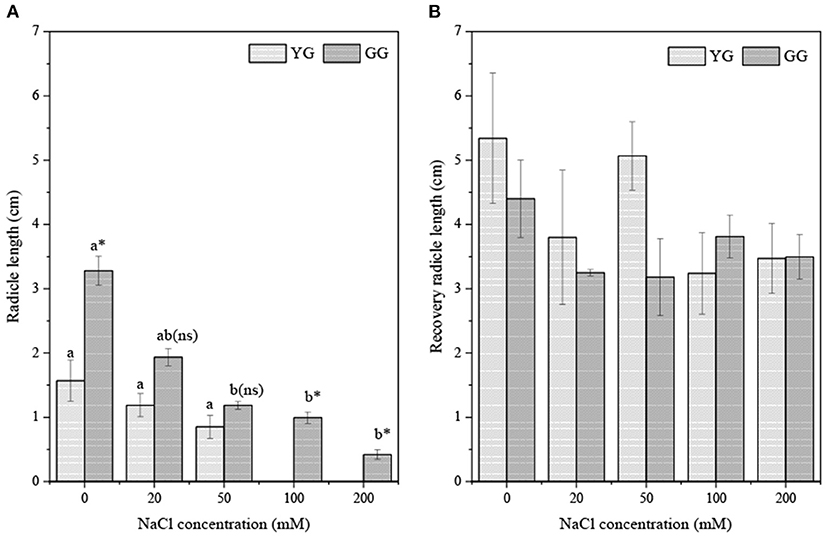
Figure 5. Effects of salt stress on radicle length of seedlings grown from different L. chinensis ecotypes (A) the initially germination (B) the recovery germination; There were twenty replicates for each treatment. Values are presented as means ± standard error (SE); Different lowercase letters indicate significant differences between the different NaCl concentrations under the same ecotype. *, **, ***Indicate significant differences between the different ecotypes under the same NaCl concentration at 0.05, 0.01, and 0.001 level respectively, and “ns” indicates no significant differences.
Effects of Salt Stress on Shoot Length of Seedlings Grown From Different L. chinensis Ecotypes
Ecotypes significantly affected the shoot length (p < 0.001, Table 2). The shoot length of GG seedlings was consistently longer than that of YG seedlings (Figure 6A). The shoot length of GG seedlings only increased significantly under the pretreatment concentration of 200 mM. The shoot length of YG seedlings increased significantly under all pretreatment concentrations. The growth of seedlings after the ungerminated seeds was transferred to a salt-free medium was better than that of seedlings that grew under salt stress, and the growth of the radicle was greater than that of the shoot (Figure 6B).
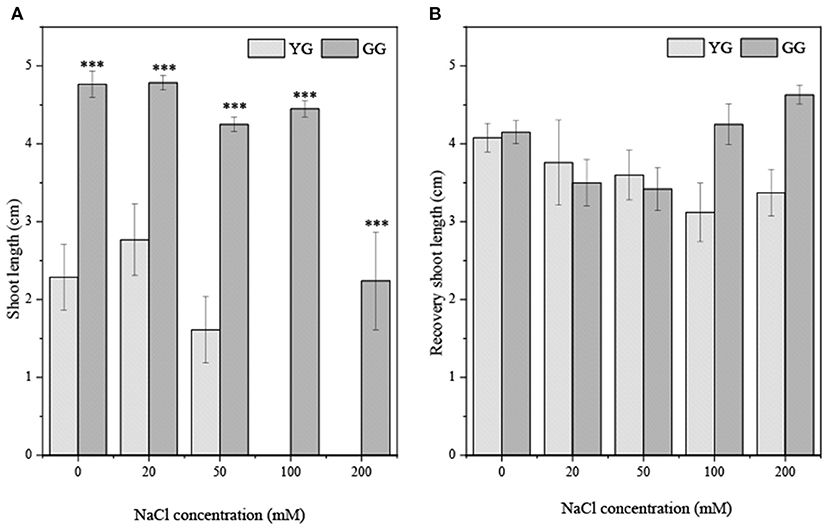
Figure 6. Effects of salt stress on shoot length of seedlings grown from different L. chinensis ecotypes (A) the initially germination (B) the recovery germination; There were twenty replicates for each treatment. Values are presented as means ± standard error (SE). *, **, ***Indicate significant differences between the different ecotypes under the same NaCl concentration at 0.05, 0.01, and 0.001 level respectively, and “ns” indicates no significant differences.
Effects of Salt Stress on the Number of Radicles of Seedlings Grown From Different L. chinensis Ecotypes
Ecotypes significantly affected the number of radicles (p < 0.001, Table 2). At all salt concentrations, the number of radicals of GG was greater than that of YG; the number of radicles of GG seedlings germinated under 200 mM salt solution was approximately two times that of the YG ecotype under salt-free conditions (Figure 7).
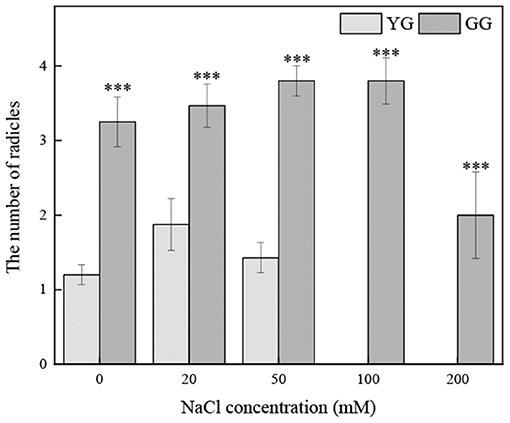
Figure 7. Effects of salt stress on the number of radicles of seedlings grown from different of L. chinensis ecotypes. There were twenty replicates for each treatment. Values are presented as means ± standard error (SE). *, **, ***Indicate significant differences between the different ecotypes under the same NaCl concentration at 0.05, 0.01, and 0.001 level respectively, and “ns” indicates no significant differences.
Discussion
There were significant differences in the germination percentage of different L. chinensis ecotypes. Under high salt stress, GG had higher germination percentages (including regermination), greater numbers of radicles, longer radicle lengths, shorter germination times, and higher thousand-grain weight compared with YG. These findings indicate that GG had stronger salt tolerance than YG during the seed germination and seedling growth stages. Variation in salt sensitivity is related to ecotype identity and salt concentration, which is consistent with the results of previous studies (Megdiche et al., 2007). We found that the GG seeds are more salt-tolerant and could be of value for plant breeding and grassland restoration.
Increases in salinity inhibit the germination of L. chinensis, and the degree of inhibition varied among L. chinensis ecotypes. GG is less affected by salt stress. Genetic differences between populations play a key role in determining salt tolerance, and some experiments have supported this hypothesis. For example, differences in the germination percentage of the seeds of different carob ecotypes under drought and salt stress have been detected. The goal is to select genotypes with strong stress tolerance for cultivation (Cavallaro et al., 2016). Ecotypes of milk thistle (Silybum marianum) differ in their tolerance to drought and salt stress, which may be caused by genetic variation (Hammami et al., 2020). GG could be particularly useful for the study of salt tolerance mechanisms. Salt inhibits the germination of seeds in two ways. First, it reduces the osmotic pressure of plants and limits the absorption of water and nutrients. It may also inhibit enzyme activity to weaken the hydrolysis and metabolism of plants (Munns, 2002; Chaves et al., 2003; Sidari et al., 2008). Second, ion toxicity reduces the absorption of K+, which results in the accumulation of toxic substances, photosynthesis, and a decrease in respiration (Munns, 2002; Chen and Wang, 2009; Zhou et al., 2014). Some studies have also shown that the MGT of seeds under salt stress is prolonged, which may be caused by a temporary delay in germination or forced dormancy by osmosis. This is an adaptive strategy for seeds to survive under harsh conditions (Ghoulam and Fares, 2001; Gill et al., 2003). GG seeds might maintain a relatively high water content under salt stress, which allows them to germinate under high salinity (200 mM NaCl), and their MGT is shorter (Bandurska, 2000). However, the low seed germination of YG might be due to the deeper seed dormancy than GG ecotype seeds. Therefore, it is necessary to break seed dormancy by GA3 or cold stratification before comparing their salt-tolerant abilities in the natural environment in future studies.
The seeds of different L. chinensis ecotypes varied in their response to salinity. Although the germination percentage generally decreased with salinity, GG has a higher salt tolerance than YG. At 100 mM NaCl, 45% of GG seeds germinated, but only 1% of YG seeds germinated. This pattern may stem from differences in the antioxidant capacity of the two ecotypes (Cavallaro et al., 2016; Zhou et al., 2017). After the ungerminated seeds exposed to salt stress were transferred to distilled water, the germination percentage of YG and GG ecotypes gradually increased as the salt concentration that they were previously exposed to increased. This pattern indicates that the salt concentration only temporarily inhibits the germination of seeds, and this is one of the mechanisms permitting L. chinensis seeds to germinate under salt stress. This finding is consistent with the results of previous studies (Khan and Gulzar, 2003; Wang et al., 2008; Cao et al., 2012). Higher salinity in spring results in low germination of L. chinensis seeds; high precipitation in summer decreases soil salinity, which increases the rapidity of germination, especially for seeds in the shallow layer of the soil.
Seedling establishment is another critical period (Jamil et al., 2005). Under salt stress, plants experience a series of morphological, physiological, and biochemical changes. The morphological changes mainly include changes in radicle length, shoot length, and the number of radicles. The length of shoots and radicles can be used as an early indicator of plant salt tolerance (Misra et al., 1995; Lopez and Satti, 1996; Evers et al., 1997). Under abiotic stress, the root system of plants is the key since the roots are in close contact with salt in the soil, and the Na+ absorbed by the shoot is regulated by the root system. The absorption and transport of Na+ are also important (Anil et al., 2005; Krishnamurthy et al., 2009). When the soil has too much salt, the nutrients in the soil are lacking, and plant dehydration is serious, which reduces the turgor pressure of the cells and causes the cells to stop growing and dividing (Bewley and Black, 1983; Neumann, 1997). The radicle is more sensitive to salinity, which is consistent with the results of previous studies (Misra and Dwivedi, 2004; Lin et al., 2014). Although salt inhibits the radicle length, salt does not inhibit the number of radicles. At all salt concentrations, the number of radicles of GG was greater than that of YG (Figure 7), which may indicate that GG has better salt tolerance. Under appropriate concentrations, salt promotes rooting, the absorption of water, the maintenance of osmotic pressure, and the dynamic balance of ions and nutrients for growth. Several radicles facilitate the maintenance of osmotic pressure and increase vegetative reproduction. We dissected the ungerminated YG seeds with a scalpel and found that the embryos of the seeds were hard and intact, indicating that most YG seeds were dormant. This may be a characteristic of seed adaptability to ensure germination in an environment conducive to seedling emergence. The germination requirements of YG seeds are very high, and the ungerminated seeds may be due to environmental conditions and the characteristics of the seed (i.e., the thickness of the seed coat, the vitality of the embryo, and the provision of nutrients) that did not meet their germination standards. Therefore, GG is more tolerant to salt stress than YG, and the degree of dormancy is lower. Thus, it is a better choice for restoring land degraded by salinity. The selection of cultivars with optimal genotypes during the germination period and seedling establishment period is essential for ensuring the success of saline-alkali soil restoration.
Data Availability Statement
The original contributions presented in the study are included in the article/Supplementary Material, further inquiries can be directed to the corresponding author.
Author Contributions
H-YM contributed to the conception of the study. M-YM performed the experiment. M-YM and H-YM contributed significantly to analysis, manuscript preparation, performed the data analyses and wrote the manuscript. LW, H-YM, D-DZ, S-YL, and W-WQ helped to perform the analysis with constructive discussions. All authors contributed to the article and approved the submitted version.
Funding
This research was supported by grants from the National Natural Science Foundation of China (41771058 and 41977424), and the Provincial Natural Science Foundation of Jilin (20200201026JC), and the Strategic Priority Research Program of the Chinese Academy of Sciences (XDA28110300 and XDA2306040303).
Conflict of Interest
The authors declare that the research was conducted in the absence of any commercial or financial relationships that could be construed as a potential conflict of interest.
Publisher's Note
All claims expressed in this article are solely those of the authors and do not necessarily represent those of their affiliated organizations, or those of the publisher, the editors and the reviewers. Any product that may be evaluated in this article, or claim that may be made by its manufacturer, is not guaranteed or endorsed by the publisher.
Supplementary Material
The Supplementary Material for this article can be found online at: https://www.frontiersin.org/articles/10.3389/fevo.2021.703287/full#supplementary-material
References
Anderson, J. T., Panetta, A. M., and Mitchell-Olds, T. (2012). Evolutionary and ecological responses to anthropogenic climate change: update on anthropogenic climate change. Plant Physiol. 160, 1728–1740. doi: 10.1104/pp.112.206219
Anil, V. S., Krishnamurthy, P., Kuruvilla, S., Sucharitha, K., Thomas, G., and Mathew, M. K. (2005). Regulation of the uptake and distribution of Na+ in shoots of rice (Oryza sativa) variety Pokkali: role of Ca2+ in salt tolerance response. Physiol. Plant. 124, 451–464. doi: 10.5194/amt-5-1333-2012
Ashraf, M. (1993). Effect of sodium chloride on water relations and some organic osmotica in arid zone plant species Melilotus indica (L.) All. J. Agric. Trop. Subtrop. 94, 95–102.
Ashraf, M., and Harris, P. J. C. (2004). Potential biochemical indicators of salinity tolerance in plants. Plant Sci. 166, 3–16. doi: 10.1016/j.plantsci.2003.10.024
Bai, Y. F., Xu, Z. X., and Li, D. X. (1994). Study on seasonal fluctuations of biomass for Leymus chinensis grassland. Grassland China 3, 1–5,9. Available online at: https://europepmc.org/article/cba/274330
Bandurska, H. (2000). Does proline accumulated in leaves of water deficit stressed barley plants confine cell membrane injury? I. Free proline accumulation and membrane injury index in drought and osmotically stressed plants. Acta Physiol. Plant. 22, 409–415. doi: 10.1007/s11738-000-0081-7
Bender, M. H., Baskin, J. M., and Baskin, C. C. (2002). Phenology and common garden and reciprocal transplant studies of Polymnia canadensis (Asteraceae), a monocarpic species of the North American temperate deciduous forest. Plant Ecol. 161, 15–39. doi: 10.1023/A:1020363517550
Bewley, J. D., and Black, M. (1983). Physiology and biochemistry of seeds in relation to germination: 1. development, germination, and growth. Ecology 1, 106–107.
Cao, D., Baskin, C. C., Baskin, J. M., Yang, F., and Huang, Z. (2012). Comparison of germination and seed bank dynamics of dimorphic seeds of the cold desert halophyte Suaeda corniculata subsp. mongolica. Ann. Bot. 110, 1545–1558. doi: 10.1093/aob/mcs205
Cavallaro, V., Barbera, A. C., Maucieri, C., Gimma, G., Scalisi, C., and Patanè, C. (2016). Evaluation of variability to drought and saline stress through the germination of different ecotypes of carob (Ceratonia siliqua L.) using a hydrotime model. Ecol. Eng. 95, 557–566. doi: 10.1016/j.ecoleng.2016.06.040
Chaves, M. M., Maroco, J. P., and Pereira, J. S. (2003). Understanding plant responses to drought—from genes to the whole plant. Funct. Plant Biol. 30, 239–264. doi: 10.1071/FP02076
Chen, L., and Wang, R. (2009). Anatomical and physiological divergences and compensatory effects in two Leymus chinensis (Poaceae) ecotypes in Northeast China. Agric. Ecosyst. Environ. 134, 46–52. doi: 10.1016/j.agee.2009.05.015
Cramer, G. R., Urano, K., Delrot, S., Pezzotti, M., and Shinozaki, K. (2011). Effects of abiotic stress on plants: a systems biology perspective. BMC Plant Biol. 11, 1–14. doi: 10.1186/1471-2229-11-163
Delker, C., Pöschl, Y., Raschke, A., Ullrich, K., Ettingshausen, S., Hauptmann, V., et al. (2010). Natural variation of transcriptional auxin response networks in Arabidopsis thaliana. Plant Cell 22, 2184–2200. doi: 10.1105/tpc.110.073957
El-Keblawy, A., and Al-Rawai, A. (2005). Effects of salinity, temperature and light on germination of invasive Prosopis juliflora (Sw.) DC. J. Arid Environ. 61, 555–565. doi: 10.1016/j.jaridenv.2004.10.007
Ellis, R. H., and Roberts, E. H. (1980). Improved equations for the prediction of seed longevity. Ann. Bot. 45, 13–30. doi: 10.1093/oxfordjournals.aob.a085797
Evers, D., Schmit, C., Mailliet, Y., and Hausman, J. F. (1997). Growth characteristics and biochemical changes of poplar shoots in vitro under sodium chloride stress. J. Plant Physiol. 151, 748–753. doi: 10.1016/S0176-1617(97)80073-9
Ghoulam, C., and Fares, K. (2001). Effect of salinity on seed germination and early seedling growth of sugar beet (Beta vulgaris L.). Seed Sci. Technol. 29, 357–364. doi: 10.1023/A:1009023320116
Gill, P. K., Sharma, A. D., Singh, P., and Bhullar, S. S. (2003). Changes in germination, growth and soluble sugar contents of Sorghum bicolor (L.) Moench seeds under various abiotic stresses. Plant Growth Regul. 40, 157–162. doi: 10.1023/A:1024252222376
Hameed, M., and Ashraf, M. (2008). Physiological and biochemical adaptations of Cynodon dactylon (L.) Pers. from the Salt Range (Pakistan) to salinity stress. Flora Morphol. Distrib. Funct. Ecol. Plants 203, 683–694. doi: 10.1016/j.flora.2007.11.005
Hammami, H., Saadatian, B., and Hosseini, S. A. H. (2020). Geographical variation in seed germination and biochemical response of milk thistle (Silybum marianum) ecotypes exposed to osmotic and salinity stresses. Ind. Crops Prod. 152, 112507–112519. doi: 10.1016/j.indcrop.2020.112507
Hoffmann, A. A., and Sgrò, C. M. (2011). Climate change and evolutionary adaptation. Nature 470, 479–485. doi: 10.1038/nature09670
Jamil, M., Lee, C. C., Rehman, S. U., Lee, D. B., Ashraf, M., and Rha, E. S. (2005). Salinity (NaCl) tolerance of Brassica species at germination and early seedling growth. Electron. J. Environ. Agric. Food Chem. 4, 970–976. doi: 10.4141/cjps95-137
Jump, A. S., and Penuelas, J. (2005). Running to stand still: adaptation and the response of plants to rapid climate change. Ecol. Lett. 8, 1010–1020. doi: 10.1111/j.1461-0248.2005.00796.x
Kawecki, T. J., and Ebert, D. (2004). Conceptual issues in local adaptation. Ecol. Lett. 7, 1225–1241. doi: 10.1111/j.1461-0248.2004.00684.x
Khan, M. A., and Gulzar, S. (2003). Germination responses of Sporobolus ioclados: a saline desert grass. J. Arid Environ. 53, 387–394. doi: 10.1006/jare.2002.1045
Khan, M. A., and Ungar, I. A. (1984). Seed polymorphism and germination responses to salinity stress in Atriplex triangularis Willd. Bot. Gaz. 145, 487–494. doi: 10.1086/337483
Kheloufi, A., Mansouri, L. M., Bouafia, B., Khamari, Y., Kheloufi, H., and Bouguern, Y. (2019). Morphological characteristics and seed germination improvement of two ecotypes of Astragalus armatus Willd. subsp. armatus in Algeria. Cercet. Agronomice Moldova 51, 96–107. doi: 10.2478/cerce-2018-0039
Kitajima, K., and Fenner, M. (2000). Ecology of seedling regeneration. Seeds 2, 331–359. doi: 10.1079/9780851994321.0331
Krishnamurthy, P., Ranathunge, K., Franke, R., Prakash, H. S., Schreiber, L., and Mathew, M. K. (2009). The role of root apoplastic transport barriers in salt tolerance of rice (Oryza sativa L.). Planta 230, 119–134. doi: 10.1007/s00425-009-0930-6
Lin, J., Li, Z., Wang, Y., and Mu, C. (2014). Effects of various mixed salt-alkaline stress conditions on seed germination and early seedling growth of Leymus chinensis from Songnen Grassland of China. Notulae Botanicae Horti Agrobotanici Cluj-Napoca 42, 154–159. doi: 10.15835/nbha4219388
Liu, Y., Zhang, L., Xu, X., and Niu, H. (2016). Understanding the wide geographic range of a clonal perennial grass: plasticity versus local adaptation. AoB Plants 8, 141–149. doi: 10.1093/aobpla/plv141
Liyanage, G. S., and Ooi, M. K. (2015). Intra-population level variation in thresholds for physical dormancy-breaking temperature. Ann. Bot. 116, 123–131. doi: 10.1093/aob/mcv069
Lopez, M. V., and Satti, S. M. E. (1996). Calcium and potassium-enhanced growth and yield of tomato under sodium chloride stress. Plant Sci. 114, 19–27. doi: 10.1016/0168-9452(95)04300-4
Lu, Q., Meng, X., Yang, F., Liu, X., and Cui, J. (2020). Characterization of LcGAPC and its transcriptional response to salt and alkali stress in two ecotypes of Leymus chinensis (Trin.) Tzvelev. Biotechnol. Biotechnol. Equip. 34, 115–125. doi: 10.1080/13102818.2020.1719020
Ma, H., Wu, H., and Ooi, M. K. (2018). Within population variation in germination response to smoke cues: convergent recruitment strategies and different dormancy types. Plant Soil 427, 281–290. doi: 10.1007/s11104-018-3641-6
Maggio, A., Reddy, M. P., and Joly, R. J. (2000). Leaf gas exchange and solute accumulation in the halophyte Salvadora persica grown at moderate salinity. Environ. Exp. Bot. 44, 31–38. doi: 10.1016/S0098-8472(00)00051-4
Megdiche, W., Amor, N. B., Debez, A., Hessini, K., Ksouri, R., Zuily-Fodil, Y., et al. (2007). Salt tolerance of the annual halophyte Cakile maritima as affected by the provenance and the developmental stage. Acta Physiol. Plant. 29, 375–384. doi: 10.1007/s11738-007-0047-0
Misra, M., Das, N., and Misra, A. N. (1995). Sodium chloride salt stress induced changes in protein content and protease activity in callus culture of pearl millet [Pennisetum glaucum LR Br.]. Acta Physiol. Plant. 17 371–374.
Misra, N., and Dwivedi, U. N. (2004). Genotypic difference in salinity tolerance of green gram cultivars. Plant Sci. 166, 1135–1142. doi: 10.1016/j.plantsci.2003.11.028
Munns, R. (2002). Comparative physiology of salt and water stress. Plant Cell Environ. 25, 239–250. doi: 10.1046/j.0016-8025.2001.00808.x
Neumann, P. (1997). Salinity resistance and plant growth revisited. Plant Cell Environ. 20, 1193–1198. doi: 10.1046/j.1365-3040.1997.d01-139.x
Qadir, M. (2016). Policy note: reversing salt-induced land degradation requires integrated measures. Water Econ. Policy 2, 1671001–1671009. doi: 10.1142/S2382624X16710016
Randin, C. F., Engler, R., Normand, S., Zappa, M., Zimmermann, N. E., Pearman, P. B., et al. (2009). Climate change and plant distribution: local models predict high-elevation persistence. Global Change Biol. 15, 1557–1569. doi: 10.1111/j.1365-2486.2008.01766.x
Savolainen, O., Pyhäjärvi, T., and Knürr, T. (2007). Gene flow and local adaptation in trees. Annu. Rev. Ecol. Evol. Syst. 38, 595–619. doi: 10.1146/annurev.ecolsys.38.091206.095646
Sidari, M., Mallamaci, C., and Muscolo, A. (2008). Drought, salinity and heat differently affect seed germination of Pinus pinea. J. For. Res. 13, 326–330. doi: 10.1007/s10310-008-0086-4
Tavakkoli, E., Fatehi, F., Coventry, S., Rengasamy, P., and McDonald, G. K. (2011). Additive effects of Na+ and Cl− ions on barley growth under salinity stress. J. Exp. Bot. 62, 2189–2203. doi: 10.1093/jxb/erq422
Tipirdamaz, R., Gagneul, D., Duhazé, C., Aïnouche, A., Monnier, C., and Özkum, D. (2006). Clustering of halophytes from an inland salt marsh in Turkey according to their ability to accumulate sodium and nitrogenous osmolytes. Environ. Exp. Bot. 57, 139–153. doi: 10.1016/j.envexpbot.2005.05.007
Ungar, I. A. (1995). Seed germination and seed-bank ecology in halophytes. Seed Dev. Germin. 41, 599–628. doi: 10.1201/9780203740071
Wang, L., Huang, Z., Baskin, C. C., Baskin, J. M., and Dong, M. (2008). Germination of dimorphic seeds of the desert annual halophyte Suaeda aralocaspica (Chenopodiaceae), a C4 plant without Kranz anatomy. Ann. Bot. 102, 757–769. doi: 10.1093/aob/mcn158
Yang, Z., and Wang, B. S. (2015). Present status of saline soil resources and countermeasures for improvement and utilization in China. Shandong Agric. Sci. 47, 125–130. doi: 10.14083/j.issn.1001-4942.2015.04.032
Zhao, D. D., Ma, H. Y., Li, Y., Wei, J. P., and Wang, Z. C. (2019). Effects of water and nutrient additins on fuctional trits and aboveground biomass of Leymus chinensis. Chin. J. Plant Ecol. 43, 501–511. doi: 10.17521/cjpe.2019.0041
Zhou, C., Busso, C. A., Yang, Y. G., Zhang, Z., Wang, Z. W., Yang, Y. F., et al. (2017). Effect of mixed salt stress on malondialdehyde, proteins and antioxidant enzymes of Leymus chinensis in three leaf colors. Phyton 86, 205–213. doi: 10.32604/phyton.2017.86.205
Zhou, C., and Yang, Y. (2003). Physiological response to salt-alkali stress in experimental populations in two ecotypes of Leymus chinensis in the Songnen Plains of China. Chin. J. Appl. Ecol. 14, 1842–1846. doi: 10.13287/j.1001-9332.2003.0407
Keywords: ecotype, salt-tolerant, adaptive evolution, grassland conservation, climate change
Citation: Ma M-Y, Ma H-Y, Wang L, Qi W-W, Li S-Y and Zhao D-D (2021) Differences in the Seed Germination of Leymus chinensis (Poaceae) Ecotypes Reveal Distinct Strategies for Coping With Salinity Stress: A Common Garden Experiment. Front. Ecol. Evol. 9:703287. doi: 10.3389/fevo.2021.703287
Received: 30 April 2021; Accepted: 27 September 2021;
Published: 28 October 2021.
Edited by:
Mesenbet Yibeltal, Bahir Dar University, EthiopiaReviewed by:
Xiaowen Hu, Lanzhou University, ChinaJixiang Lin, Northeast Forestry University, China
Copyright © 2021 Ma, Ma, Wang, Qi, Li and Zhao. This is an open-access article distributed under the terms of the Creative Commons Attribution License (CC BY). The use, distribution or reproduction in other forums is permitted, provided the original author(s) and the copyright owner(s) are credited and that the original publication in this journal is cited, in accordance with accepted academic practice. No use, distribution or reproduction is permitted which does not comply with these terms.
*Correspondence: Hong-Yuan Ma, bWFob25neXVhbkBpZ2EuYWMuY24=