- Centre for Ecological Sciences, Indian Institute of Science, Bengaluru, India
The ancient interaction between figs (Ficus, Moraceae) and their pollinating fig wasps is an unusual example of a mutualism between plants and gall-inducing insects. This review intends to offer fresh perspectives into the relationship between figs and the diversity of gall-inducing sycophiles which inhabit their enclosed globular inflorescences that function as microcosms. Besides gall-inducing pollinators, fig inflorescences are also inhabited by other gall-inducing wasps. This review evaluates the state of current knowledge on gall-induction by fig wasps and exposes the many lacunae in this area. This review makes connections between fig and gall-inducing wasp traits, and suggests relatively unexplored research avenues. This manuscript calls for an integrated approach that incorporates such diverse fields as life-history theory, plant mate choice, wasp sexual selection and local mate competition, plant embryology as well as seed and fruit dispersal. It calls for collaboration between researchers such as plant developmental biologists, insect physiologists, chemical ecologists and sensory biologists to jointly solve the many valuable questions that can be addressed in community ecology, co-evolution and species interaction biology using the fig inflorescence microcosm, that is inhabited by gall-inducing mutualistic and parasitic wasps, as a model system.
Introduction
Gall-inducing insects are usually plant parasites (Espírito-Santo and Fernandes, 2007; Oliveira et al., 2016; Miller and Raman, 2019). Sometimes, however, they are beneficial to plants, as in brood-site pollination mutualisms where plants trade insect development sites against seed production (Sakai, 2002; Borges, 2016). Yet, such mutualisms persist only if partner exploitation is controlled (Borges, 2015b; Dunn, 2020). Most mutualisms involving galling insects are specific, and often exhibit co-diversification, co-speciation, and co-evolution (Cruaud et al., 2012; Wang et al., 2019). This specificity mandates adaptations at many levels including unusual life history traits, e.g., 30-year diapause in yucca moth larvae to match the flowering phenology of yucca host-plant populations (Powell, 2001).
In the brood-site pollination mutualism between figs and wasps, a single pollinator wasp larva usually occupies each uniovulate galled flower within a multi-flowered closed inflorescence (Janzen, 1979; Wiebes, 1979). Pollinating fig wasps usually belong to family Agaonidae: subfamilies Agaoninae, Kradibiinae, Tetrapusiinae (Kjellberg et al., 2005; Heraty et al., 2013; Farache et al., 2017). The fig inflorescence or syconium is also occupied by non-pollinating parasitic gall-inducing wasps in the Sycophaginae (Agaonidae), Otitesellinae, and Epichrysomallinae (Pteromalidae; Heraty et al., 2013; Farache et al., 2017, 2018b). There is extreme sexual dimorphism in adult fig wasps, with the males usually being wingless and completing their life cycle within their natal syconium (Weiblen, 2002). The fig–fig wasp mutualism is a classic example of co-diversification (Cruaud et al., 2012; Wang et al., 2019), with much greater congruence in phylogenies between figs and their pollinators, and between pollinators and competing gallers, than between figs and their galler parasites (Wang et al., 2019); the latter incongruence may signify host switches, duplications, and association losses (Yang et al., 2015; Wachi et al., 2016).
Although the fig–galler fig wasp interaction has been extensively investigated (Cook and Rasplus, 2003; Kjellberg et al., 2005; Herre et al., 2008; Borges, 2015a; Dunn, 2020), vital mechanisms governing fig and fig wasp biology remain to be discovered. This review will propose possible correspondences between traits of figs and gall-inducing wasps, relevant to the mutualism or its exploitation. This review provides fresh insights and suggests new questions that await answers.
Ficus (Moraceae) Inflorescence Traits
Fig flowers or inflorescence tissues, that are exploited by galling wasps, are hidden within a globoid or urn-shaped inflorescence called a syconium. This enclosed fig inflorescence likely originated from the invagination of a flat flower-bearing capitulum (Thorogood et al., 2018; Clement et al., 2020). The fig–fig wasp mutualism, which probably arose in Eurasia, is dated at 75+ mya (Cruaud et al., 2012; Rasplus et al., 2020), but a more recent date of 41–56 mya is suggested (Zhang et al., 2019) which matches the 49 my age of fig wasps (Peters et al., 2018).
The urn-shaped fig inflorescence with its compressed internodes (Verkerke, 1989) is closed off by an involucre of bracts, leaving a single opening or ostiole. Usually, female pollinators entering through the tight-fitting ostiole suffer wing and antennal breakage and other injuries; consequently, such females, called foundresses, can usually visit only a single syconium within which they soon die. Syconia can be axillary, cauliflorous, or geocarpic being subtended on subterranean stolons (Baijnath and Ramcharun, 1983). Syconia range in size from a few mm in diameter (e.g., Ficus tsjahela in south India, RM Borges, pers. observ.) to 21 × 18 cm (Ficus dammaropsis from Papua New Guinea; Ezedin and Weiblen, 2019). Syconia contain tens (e.g., 50 in Ficus mathewsii) to thousands of flowers (e.g., 7000 in Ficus pumila) (Verkerke, 1989). Fig species are either monoecious (52%) with plants bearing syconia containing individual male and female flowers, or gynodioecious (48%) with plants bearing syconia with either only functional female flowers (female trees) or those with male and female flowers (“male” trees) (Basso-Alves et al., 2014; Rasplus et al., 2020; Figure 1). In monoecious species, all syconia give rise to seeds, pollen, and wasps; however, in gynodioecious species, syconia on female trees give rise to only seeds while those on “male” trees give rise to only wasps (Figure 1). This sex-limited seed or wasp production in dioecious species is related to the longer style lengths of flowers in female trees which prevent female wasps from depositing eggs at the level of the ovule, resulting in zero reproductive success for foundress females entering syconia on female trees (Figure 1). In syconia of monoecious figs and of male trees in gynodioecious species, there is extreme protogyny, i.e., female flowers develop first and the interval between female and male flower development can be several weeks or months. This is likely an adaptation to the brood-site pollination mutualism, since one generation of pollinators brings in pollen to fertilize female flowers, but the next generation of pollinators that develops within the syconium carries pollen out of the syconium. Very recently, gynomonoecy was discovered in Ficus umbrae in Papua New Guinea (Ezedin and Weiblen, 2019); in this case, plants bear syconia with female flowers and non-functional male flowers as well as syconia with fully functional male and female flowers. Monoecy appears ancestral in Ficus (Weiblen, 2000; Rasplus et al., 2020); gynodioecy apparently appeared once, and monoecy has reappeared at least twice in gynodioecious clades (Ezedin and Weiblen, 2019; Rasplus et al., 2020). Unisexuality in Ficus flowers is achieved by stamen absence ab initio in female flowers, and by pistil absence ab initio or abortion in male flowers (Basso-Alves et al., 2014).
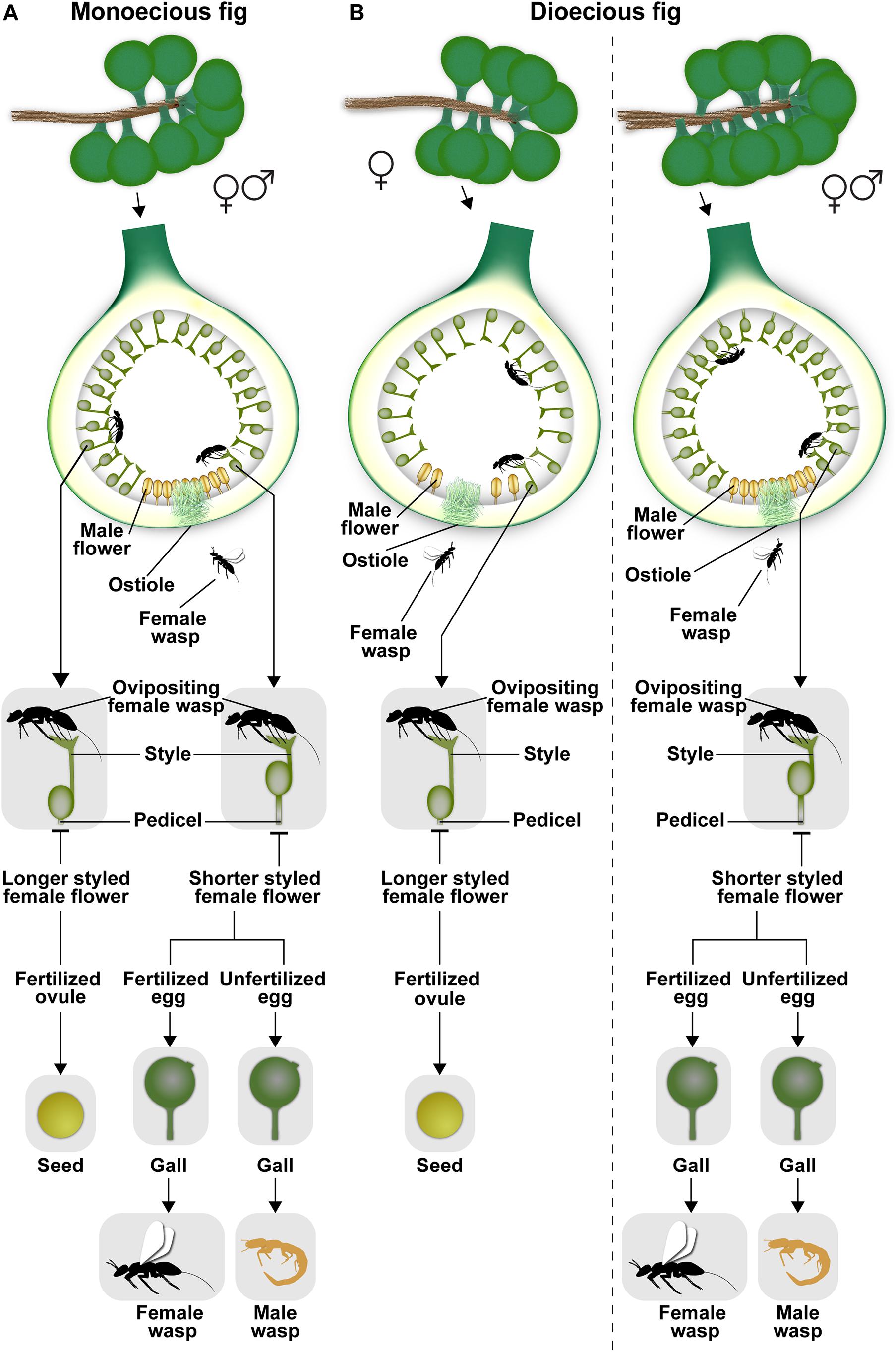
Figure 1. (A,B) The life cycles of gall-inducing pollinating fig wasps in monoecious and dioecious figs. The extreme sexual dimorphism and the lack of reproductive success in female trees in dioecious fig species is clearly depicted. Illustration by Priya B.R.
Glands on ostiolar bracts release volatiles (Souza et al., 2015; Hu et al., 2020) that attract pollinators to pollen-receptive syconia (Hossaert-McKey et al., 2010; Borges, 2016, 2018). The ostiole usually shuts after pollinators enter, but loosely arranged bracts sometimes allow the now wingless female pollinators to exit and visit other syconia on the same plant (Hu et al., 2010). The ostiole may also re-open at the wasp dispersal stage (Verkerke, 1989) so that the next generation of pollen-carrying females exit through the ostiole rather than through a passage through the syconium wall excavated by wingless males. Bracts may bear stomata (Verkerke, 1989) and secretory glands whose exudates seal the ostiole or contribute to antibiotic-containing fluid in the syconium lumen (Machado et al., 2013). The syconium wall has multi-layered parenchyma with sclerified cells and abundant latex-producing laticifers, and is covered externally by trichomes; these collectively constitute frontline defenses against parasitic gall-inducers or other herbivorous insects (Machado et al., 2013; Fan et al., 2019).
Monoecious figs have imperfect heterostyly, i.e., flowers are arranged in multiple layers with style lengths that are either normally distributed or have a positively skewed distribution; flower pedicel lengths often vary such that all stigmas reach the same level (Kathuria et al., 1995; Ganeshaiah et al., 1999) to form a fused synstigma by the coherence of groups of stigmas (Teixeira et al., 2021; Figure 2). The synstigma is typical of actively pollinated fig species (see later). Dioecious figs have perfect heterostyly; syconia on male trees have short-styled flowers, while those on female trees are long-styled (Figure 1). The synstigma allows pollen deposited at one location within this fused network to grow down a distant style at another location (Teixeira et al., 2021; Figure 2). This is analogous to the hyperstigma of Tambourissa (Monimiaceae) in which flowers are located within an invaginated cup (reminiscent of a syconium) closed by a mucilaginous hyperstigma (Endress, 1979). The reasons for apparent convergence in these traits need further investigation. The Ficus stigma is of the dry type (Heslop-Harrison and Shivanna, 1977), and must be punctured or gnawed by the pollinator ovipositor or mouthparts to initiate secretion of germination fluids (Ramírez-Benavides, 2007). Each flower has a single bitegmic ovule surrounded by two integuments (Galil et al., 1970; Baijnath and Naicker, 1989). The nucellus of each ovule is crassinucellate (i.e., massive and well developed), and therefore can serve as food, especially for gallers (Verkerke, 1989). Each ovule is either anatropous, i.e., its micropyle (entrance to the ovule) is bent downward toward the point of basal attachment to the funiculus (Figure 2), or hemi-anatropous, i.e., with its micropyle at right angles to the funiculus (Galil et al., 1970; Verkerke, 1987). Owing to anatropy, pollen tubes have to grow down the style and curve around to enter the micropyle (Figure 2), a long path which may lend itself to pollen tube competition. Possibly due to the high specificity between figs and pollinators, Ficus lacks self-incompatibility systems that recognize pollen genotypes (Hossaert-McKey and Bronstein, 2001; Ramírez-Benavides, 2007); this may explain why hybridization potential is frequently recognized between different Ficus “species” (Wei et al., 2014; Ghana et al., 2015a).
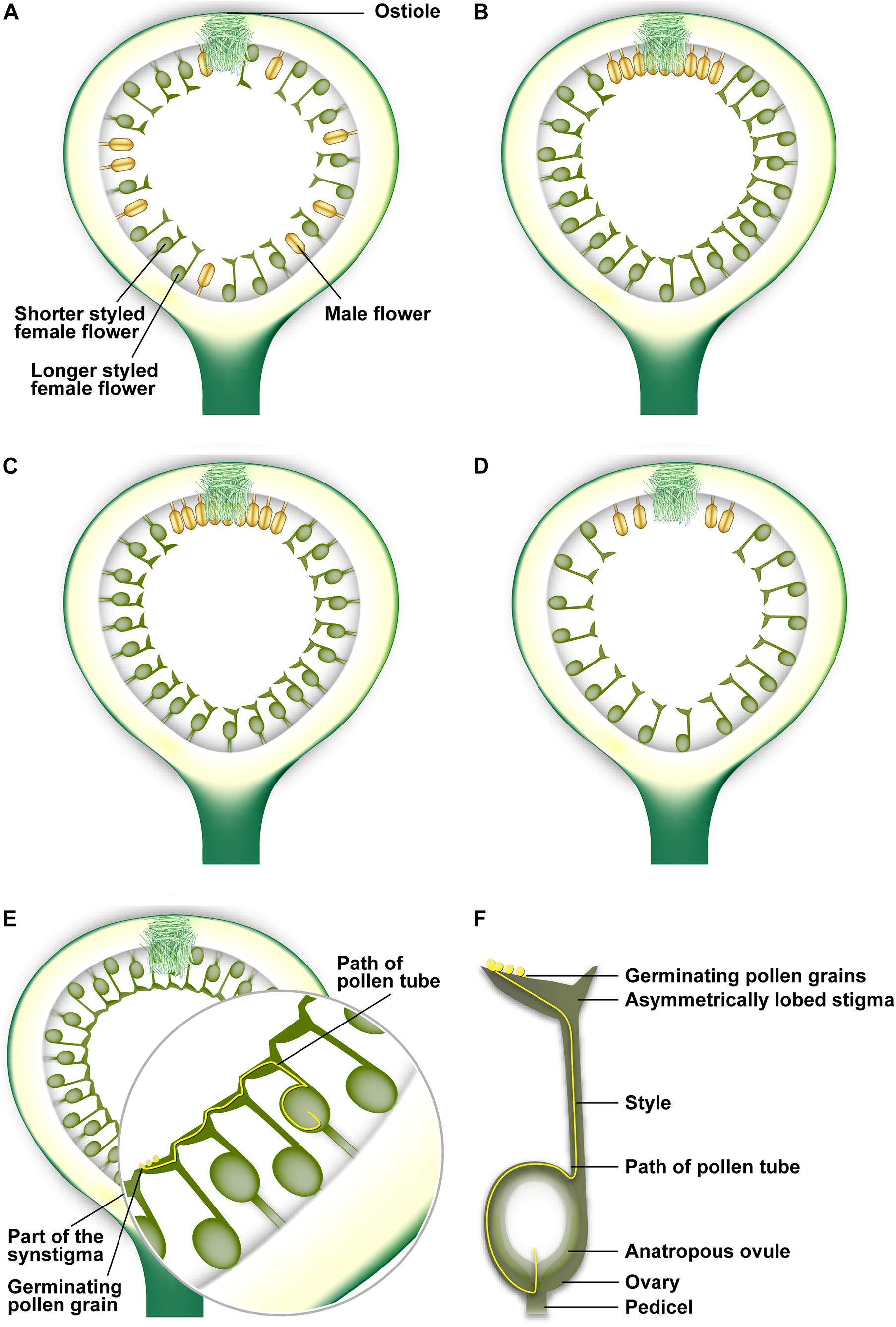
Figure 2. Arrangement of flowers within fig syconia. (A) Passively pollinated syconia usually have male flowers interspersed with female flowers throughout the syconium. (B) Actively pollinated syconia usually have few male flowers near the ostiolar opening. (C) Distribution of short-styled female flowers and a few male flowers in a syconium on a male tree in a gynodioecious fig species. (D) Distribution of long-styled female flowers in a syconium on a female tree in a gynodioecious fig species. (E) The synstigma in the syconium of an actively pollinated fig species showing longer-styled flowers with short pedicels and shorter-styled flowers with long pedicles. The potential path of a pollen tube taken in a synstigma is also shown. (F) The potential path taken by a pollen tube after germinating on an asymmetrically lobed stigma and traversing down the style to enter the micropyle of an anatropous ovule. Illustration by Priya B.R.
Syconia are well endowed with latex, which is often effective against herbivory (Ramos et al., 2019). Although laticifers occur throughout the syconium wall, they are precisely distributed in floral tissues (Marinho et al., 2018). For example, laticifers are absent from pistils, which will develop either into seeds or support gall-inducing pollinator larvae, and also do not occur in stamens; however, they are present in ostiolar bracts, sepals, and flower pedicels (Marinho et al., 2018). Inappropriate localization of latex could interfere with pollination, oviposition, stamen excision and safe exit from galls and may explain their precise distribution (Marinho et al., 2018). Moreover, laticifer distribution may also restrict galler larvae to single galls derived from uniovulate flowers, since movement between galls and feeding on extra-gall tissue could result in severance of laticifers resulting in entrapment in latex. How restricted feeding within a single gall has affected larval and adult size is unknown. Latex leakage is prevented by callose and suberin in laticifer walls (Teixeira et al., 2020) and rapid occlusion of laticifers on damage (Shi et al., 2019). Inflorescence laticifers are unlikely to be a synapomorphy in Moraceae as hitherto believed since laticifers occur within the inflorescence and other plant tissues in Urticaceae and Moraceae (Marinho and Teixeira, 2019); however, in non-Ficus species within Moraceae, laticifers also occur in the ovary and style (Marinho and Teixeira, 2019) whereas they are absent from such tissues in Ficus suggesting that the syconium may be the only brood-site in a latex-rich host plant wherein laticifer distribution is specifically adapted to the mutualism with gall-inducing pollinators.
Another trait critical to the mutualism between figs and galler fig wasps is flowering phenology (Addicott et al., 1990). Ficus flowers aseasonally, usually showing a neutral response to photoperiod; flowering is usually synchronous within plants (Gates and Nason, 2012; Krishnan and Borges, 2014). However, at the northern limit of its sub-tropical range and in fig species located in temperate regions, e.g., Ficus carica in southern Europe, flowering phenology matches seasonal temperature fluctuations and may also include over-wintering syconia that keep pollinators alive through the winter (Kjellberg et al., 1987; Zhao T. T. et al., 2014; Chen et al., 2015). While the molecular basis of short- or long-day flowering is known (Singh et al., 2017), that for day-neutral flowering, as generally occurs in Ficus, is barely understood (Lu et al., 2020). In Ficus carica, which has a 5-month fruiting period before entering winter dormancy, FcFT1, a FLOWERING LOCUS T-like gene, and FcLFY, a homolog of the FLORICAULA/LEAFY gene, are involved in inflorescence differentiation (Ikegami et al., 2013; Ma et al., 2020).
Syconium development times also vary between species; wasp and seed development phases can range from 15 to 100 days (Ramírez-Benavides, 1974) and this may be dependent on syconium size. Since syconia are largely animal-dispersed (Shanahan et al., 2001), they must become attractive to fruit dispersers only after pollinators have left the syconium; ripening prior to pollinator dispersal would result in pollinators being consumed by frugivores. Some fig fruit, however, ripen slowly while others ripen very rapidly; the reasons for these differences are barely investigated (Borges et al., 2011).
Traits of Gall-Inducing fig Wasps
Pollinator Wasps
Female pollinator wasps have morphological adaptations that facilitate entry between the ostiolar bracts into the syconium (van Noort and Compton, 1996). If only a single foundress wasp enters a syconium, her offspring will experience sib-mating. Therefore, pollinators must tolerate some level of inbreeding (Greeff et al., 2009) since syconia with only single foundresses do occur. The offspring of fig wasps with their haplo-diploid breeding system (unfertilized eggs developing into males; fertilized eggs developing into females; Figure 1) experience local mate competition (LMC) (Hamilton, 1967) since the syconium is an enclosed mating arena. The first foundress has a female-biased offspring sex ratio, while the offspring ratio of late-entering foundresses is more male-biased (Kjellberg et al., 2005). Eggs destined to be males are laid first followed by females (Raja et al., 2008); females modulate egg-laying decisions based on their own clutch sizes and those of competing foundresses (Greeff and Newman, 2011). How they achieve this is unknown, although they may estimate egg load remaining after each bout of oviposition (Raja et al., 2008; Zhang et al., 2020). Fighting between foundresses results in fewer eggs laid by the weaker competitor (Moore and Greeff, 2003).
The extreme inter-sexual dimorphism of fig wasps (Figure 1) has been selected by mating conditions of the syconium in which the syconium is the mating arena; mating usually occurs only within the syconium (Cook et al., 1997; Weiblen, 2002). Sexual dimorphism in clock gene expression results in males eclosing and leaving their galls earlier (Gu et al., 2014) to gain access to unmated females. In species in which several foundresses enter syconia, resulting in a more balanced offspring sex ratio, males engage in aggressive combat (Greeff et al., 2003).
Pollinator males also cooperate to release pollen-carrying females by collectively tunneling through the syconium wall (Suleman et al., 2012) usually near the ostiole; why the ostiole is avoided as an exit point is unknown. Males may also excise anthers of the unisexual male flowers that have only recently developed due to the extreme protogyny of the syconium; however, anthers may also dehisce spontaneously. Pollen is dusted over females (in passive pollination) or is actively gathered by females into their special thoracic pockets (in active pollination). In passive pollination, the pollen that coats females entering a female-phase syconium will passively adhere to stigmas, while in active pollination, pollinator females will actively unload pollen from their thoracic pockets using special coxal combs and deposit pollen on the stigmas. In Ficus, transitions from passive to active pollination have occurred at least five times (Cruaud et al., 2012); however, active pollination may be ancestral (Rasplus et al., 2020). Anther location near the ostiole in many fig species (Basso-Alves et al., 2014) may direct male tunneling activities near the ostiole so that pollen can be loaded on females prior to their departure.
The few reported non-agaonid gall-inducing pollinators [e.g., the pteromalids Diaziella (Sycoecinae) and Lipothymus (Otitesillinae)] enter through the ostiole like agaonids (Jousselin et al., 2001; Zhang F. et al., 2009). However, these non-agaonids can service only passively pollinated fig species and require agaonid pollinators within the syconium for successful reproduction. They may therefore be considered supplemental pollinators and have converged on agaonid traits for entering the syconium via the ostiole, e.g., flattened head and mandibular appendages (van Noort and Compton, 1996).
Adult pollinators do not feed; males usually die after mating and releasing females from the syconium. Therefore, pollinators are capital breeders that only utilize resource capital acquired during development; females are pro-ovigenic and eclose with a full complement of mature eggs, a trait that is a likely consequence of their short life spans (usually 24–48 h) (Ghara and Borges, 2010).
Non-Pollinating Gall-Inducing Wasps
Sycophilic non-pollinating gallers also exhibit extreme sexual dimorphism in addition to wingless or winged males, weapon polymorphism and competition for females (Wang et al., 2010; Cruaud et al., 2011). Some gallers enter through the ostiole (especially in the Old World, e.g., Sycophaga sycomori), while most oviposit into the syconium from outside. Those entering through the ostiole are usually unable to re-enter other syconia owing to injuries sustained during ostiolar passage while those ovipositing from outside could deposit eggs in multiple syconia. These gallers are often pro-ovigenic capital breeders with short or more extended lifespans of one day to several days (Ghara and Borges, 2010; Elias et al., 2018a). Sometimes, former mutualists have evolved into completely exploitative gallers but at the cost of reduced fitness, e.g., a cheater Eupristina species in Ficus altissima (Zhao J. B. et al., 2014). If sufficient suitable syconia are unavailable, older females distribute eggs over fewer syconia or oviposit into those that younger females would have avoided (Yadav and Borges, 2018a), indicating that age influences oviposition decisions. Since the female may deposit several eggs into the same syconium under these circumstances, possibly resulting in sib-mating, such wasps also must tolerate inbreeding as do pollinating fig wasps. Females, that oviposit from outside the syconium, use cues, such as chemical footprints left on the syconium surface by previously ovipositing conspecifics, to make oviposition decisions (Yadav et al., 2018). Since several females often oviposit in the same syconium, sex ratios are less likely to be female-biased, leading to the evolution of even greater male competitive ability (Nelson and Greeff, 2009a).
Community Structure of Fig Wasps Occupying the Syconial Microcosm
Worldwide, there is convergence in the community structure of fig wasps occupying fig syconia with a set of small-, medium-, and large-sized gallers and their corresponding parasitoids (Cruaud et al., 2011; Segar et al., 2013; Darwell et al., 2018). There appears to be a limited number of ways in which wasp communities can be assembled in a syconium, and the duration of the window of development time within which a syconium is suitable for oviposition appears to dictate the stability and diversity of its occupants (Venkateswaran and Borges, 2021). Prior to pollinator arrival at the syconium during the pollen-receptive stage, early-arriving gallers induce a few very large galls that protrude prominently into the syconium lumen. Gallers that arrive either just before or concomitant with the pollinators usually induce medium-sized galls or galls of the same size as pollinators. Generally, there is great host-plant specificity, especially among early-arriving gallers (Farache et al., 2018a). A notable exception is Idarnes flavicollis, which colonizes syconia during the pollen-receptive phase and exploits at least 6 Ficus species (Farache et al., 2018a). This may be due to its ability to recognize a generalizable blend of “Ficus” pollinator attraction scent (Proffit et al., 2020) across these species; such a hypothesis has explained polyphagy in fruit flies (Biasazin et al., 2019). The results may also be explained by cryptic Idarnes species, each associated with a different Ficus species. More research is required to distinguish between these possibilities.
Relationship Between fig Syconium Traits and Traits of Gall-Inducing Sycophiles
In this section, connections are made between fig and wasp traits (Figure 3). Here, there is no assumption about causation, and many connections are hypotheses that await testing; these patterns may have been observed in a few species, or not at all; consequently, this section intends to stimulate the focused collection of patterns across the 874 fig species described to date (POWO, 2020).
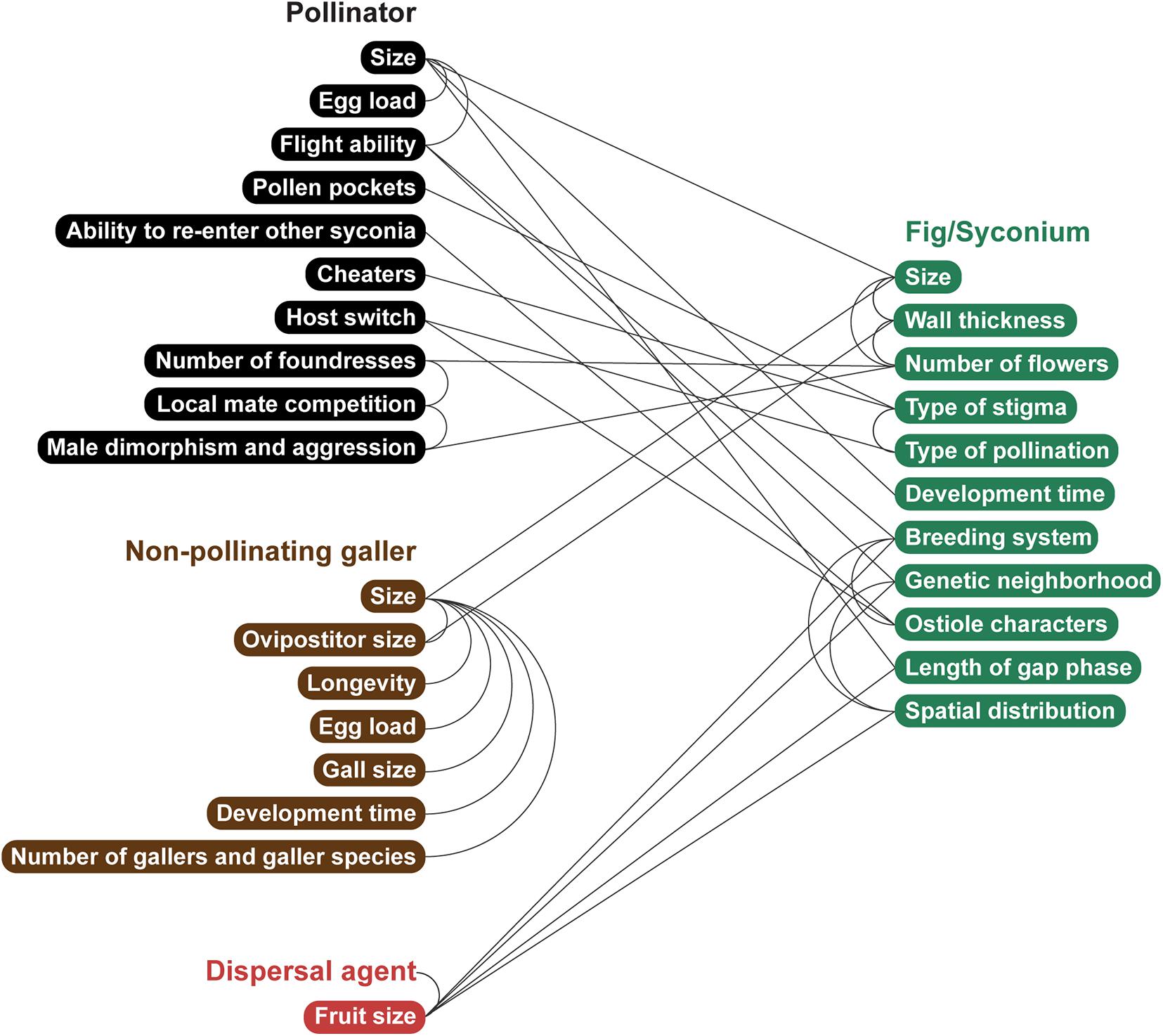
Figure 3. The possible network of causal and/or correlative connections between traits of figs, pollinators, illegitimate gallers, and fruit dispersal agents. This network does not exhaustively show all possible connections but illustrates a holistic integrative view of how multi-partner interactive traits and selection pressures may be viewed. Illustration by Priya B.R.
Syconium Size and Development Time
Syconia can vary from few mm to many cm in diameter between species; smaller syconia likely have smaller and fewer flowers. Smaller flowers are likely able to support smaller wasps which may have intrinsically lower flight ability given the energy expenditure required for flight (Venkateswaran et al., 2018). Figs with small syconia are most often pollinated by smaller wasps (Murray, 1985). Even though fig wasps are largely wind-dispersed, they vary considerably in intrinsic flight ability (Venkateswaran et al., 2018). Figs with smaller syconia may therefore have smaller genetic neighborhoods of wasp-mediated pollen transfer. Smaller syconia, when ripe, are also likely consumed by smaller frugivores with smaller dispersal distances, further contributing to more local gene movement. Since body size and ovipositor size are correlated in agaonids (Murray, 1985), and ovipositor size determines access to precise locations within each flower, it is likely that flower size and body size traits of gallers are closely related (Figure 3). The matching of these traits by either exaptive or co-evolutionary processes (Janzen, 1980) needs investigation. It is expected that trait values will stabilize to prevent over-exploitation of the mutualism by pollinators with overly long ovipositors (Murray, 1985).
If ovipositor sizes determine the exploitation of flower sizes by pollinators, then host switches in fig wasps may occur more often between figs and wasps whose flower and ovipositor sizes are compatible. Therefore, host switches may largely involve sister species as has been found in dioecious figs (Yang et al., 2015). Smaller syconia with smaller flower numbers are likely visited by fewer numbers of foundresses. Consequently, larger syconia are likely to have more inter-foundress competition, less male-biased sex ratios and more aggression among males (Nelson and Greeff, 2009a).
Syconium wall thickness may be related to syconium size and may limit exploitation by gall-inducing fig wasps that oviposit from the exterior. Early-arriving gallers have shorter ovipositors and fewer accessible sites compared to those arriving later in syconium ontogeny (Ghara et al., 2014). Ovipositors must also have appropriate armature and stiffness to penetrate the syconium wall (Ghara et al., 2011; Elias et al., 2018b). Large syconia with many more flowers likely support greater diversity of gallers compared to smaller syconia. Moreover, syconium development time is also affected by its contents, e.g., purely seed syconia take longer to develop compared to those containing only pollinators and illegitimate gallers (Galil, 1977; Krishnan and Borges, 2014). Furthermore, the duration of the pollen-receptive phase of individual syconia (which is usually only a few days) can be extended to more than 1 month if pollinators fail to enter the syconium (Gu et al., 2012; Zhang et al., 2012). While prolonged pollen-receptivity is likely an adaptation to stochasticity in pollinator arrival, older syconia, that have waited longer for pollinators to arrive, set lighter seeds and have a greater male-biased function (Gu et al., 2012); therefore, waiting for pollinators comes at a cost.
In dioecious figs, there is inter-sexual mimicry in syconium scent (Hossaert-McKey et al., 2016) to ensure equal attraction of pollinators to male and female trees, since pollinators entering syconia on female trees will have no reproductive success (Figure 1). However, in most dioecious angiosperms, flower number and size are larger in males (Barrett and Hough, 2013). In gynodioecious fig species, the constraints of intersexual mimicry may have caused syconium size to converge between the sexes, at least at the pollen-receptive stage, so that pollinator attractive scent varies neither in quality nor quantity (Raja et al., 2016) resulting in equal probabilities of visitation to both sexes. Divergence in attraction resulting in unequal probability of visitation to both sexes will destabilize the mutualism.
Ostiolar Traits
The ostiole of the syconium may be permeable without stringent filters allowing unrelated non-pollinating gall-inducing fig wasps to enter. However, cheater sister species of pollinators may even be able to breach a stringent ostiolar filter (Zhao J. B. et al., 2014). Ostiolar features may select for smaller wasps, since larger individuals may be excluded by the ostiolar passage (Liu et al., 2013). Spontaneously re-opening ostioles may contribute to greater fitness for foundress pollinator females by allowing oviposition in multiple syconia (Suleman et al., 2013) and those females that lay eggs in multiple syconia may be less prone to the consequences of LMC. In some dioecious figs, foundresses are more likely to re-emerge from older syconia, suggesting that syconium quality affects re-emergence (Raja et al., 2014).
Type of Stigma and Pollination
Synstigmas occur in 67% of figs, more often in monoecious and actively pollinated species (Teixeira et al., 2018, 2021). Stigmas of long-styled flowers in dioecious and monoecious species have greater pollen capture area compared to short-styled flowers (Jousselin et al., 2004; Teixeira et al., 2018). However, synstigmas preclude precise targeted pollination (Jousselin and Kjellberg, 2001) since pollen tubes can grow through synstigmatic tissue to enter styles distant from pollen deposition (Figure 2). Since wasps develop more successfully in pollinated flowers, how pollen tube growth in a synstigma network can be ensured in a flower in which a pollinator has deposited an egg is as yet unknown. Similarly, with a synstigma, it would be difficult if not impossible, to sanction cheater wasps that do not pollinate but only oviposit in the syconium, if the syconium has been also entered by cooperative non-cheater pollinators. These anatomical features may explain the nature of the sanctions described in figs against cheater pollinators (Dunn, 2020).
Actively pollinated figs usually have smaller pollen–ovule ratios compared to those with passive pollination since there is much less pollen wastage and more precise pollen deposition (Pellmyr et al., 2020; but see Deng et al., 2016). Pollinator traits for active pollination (pro-thoracic pockets, coxal combs for pollen removal from the pockets) appear labile and track pollen–ovule ratios of associated fig species (Cook et al., 2004). It is also interesting that although two carpels are initiated in each flower, only one carpel forms an ovule; consequently, the stigmatic surface is equivalent to that required by two carpels and two ovules, when in fact, the flower is uniovulate. The asymmetry between stigmatic surface and ovule number likely results from selection to increase pollen–ovule ratio in this highly specialized mutualism (Leite et al., 2020).
Length of Reproductive Quiescent Phase and Plant Connectivity
In many fig species, although plants flower aseasonally, plants become reproductively quiescent between flowering episodes and enter a “gap phase” (Krishnan and Borges, 2014). With a prolonged gap phase, at any given time there could be very few plants within a population that could accept pollen-carrying dispersing wasps; consequently, wasps may have to travel far to find suitable pollen-receptive trees. Wasp dispersal ability is, therefore, likely greater for those species with longer gap phases compared to those with shorter gap phases, all else being equal. Further, gallers with narrow time windows during which the syconium is suitable for oviposition are more likely to require a larger population of plants within which at least some syconia are in the right stage for oviposition (Venkateswaran and Borges, 2021).
Factors such as syconium development time, flowering synchrony, gap phase duration, and related traits such as wasp flight capabilities, which may be genetic as well as environmentally induced by variables such as syconium size and time of wasp dispersal (e.g., windy or non-windy conditions), can also affect movement between plants and thereby their connectivity. Connectivity between plants is important in determining foundress number in individual syconia (Duthie and Nason, 2016) which in turn can affect wasp traits and mutualism outcomes (Figure 3). Connectivity between plants can also be affected by syconium size and seed dispersal agents (Harrison et al., 2012).
The Gall-Inducing Process in fig Wasps: Many Lacunae in Knowledge
Although many insects engage in gall induction (Espírito-Santo and Fernandes, 2007), the galling process is still poorly understood (Hearn et al., 2019; Takeda et al., 2019: Harris and Pitzschke, 2020; Raman, 2021). Shared signaling mechanisms and hormones between plants and insects can explain the hijack of plant development machinery in gall formation (Guiguet et al., 2016; Schultz et al., 2019; Dodueva et al., 2020). Gall-inducing insects target reactive tissue sites capable of differentiation, e.g., plant meristems (Weis et al., 1988; Ferreira et al., 2019). Furthermore, since galls are fixed structures housing developing insects, their locations are important (Weis et al., 1988) in terms of factors such as access to assured resources, protection, and development on relatively long-lived plant tissues. While gall-inducing insects may stimulate plant development by effector proteins (Giron et al., 2016), gallers themselves produce hormones such as auxin and cytokinin at levels many-fold higher than those in ungalled plants (Yamaguchi et al., 2012; Andreas et al., 2020) creating hotspots of tissue growth. Since auxin and cytokinins predominantly control meristem activity (Lee et al., 2019), whether their local abundance promotes de-differentiation and neoplastic activity resulting in new meristematic sites at ectopic locations (Carneiro et al., 2017) is worth investigating. Gall-inducing adults probably also introduce compounds such as cellulases and ectoapyrase with their ovipositors or their mouthparts, compounds which are likely important in the gall-inducing process (De Lillo and Monfreda, 2004; Cambier et al., 2019).
Gall-induction in figs has received scant attention. For a pollinator or a gall-inducing exploiter, the ability to gall is fundamental to interacting with a fig species (Ghana et al., 2015b). Pollinator galls perform better when larvae develop in pollinated compared to non-pollinated flowers (Jousselin et al., 2003). According to the unbeatable seed hypothesis (West and Herre, 1994), flowers closer to the outer syconium wall in monoecious figs are more likely to become seeds; such seed flowers are presumably metabolically different and unlikely to be galled. While this hypothesis has received some experimental support (Wang et al., 2013), it has never been decisively proved. However, genes involved in carbohydrate and flavonoid metabolism are up-regulated in gall flowers compared to seed flowers (Martinson et al., 2015), suggesting metabolic differences. Flavonoids are negative regulators and modulators of auxin transport (Brown et al., 2001; Peer and Murphy, 2007); up-regulation of chalcone synthase and related flavonoid synthesis genes suggests that, by preventing transport of auxin out of gall flowers, auxin build-up occurs enabling gall formation. Vascular bundles present at the base of each uniovulate flower (Galil et al., 1970) supply nutrients to the galls from the host plant. In fig wasps, poison sac size and egg load are positively related (Martinson et al., 2014), suggesting an increased requirement for gall-inducing effector production with egg load.
Ovule dimorphism may explain the ability to induce galls in flowers as has been reported in dioecious Ficus asperifolia (Verkerke, 1987). The inner ovule integument in gall flowers does not completely surround the nucellus on the raphal side and the insect egg is deposited between the inner integument and the nucellus; consequently, the hatched larva gets easy access to the nourishing nucellus (Verkerke, 1987). However, in seed flowers, the inner ovule integument is complete on the raphal side. Periclinal (parallel) divisions of the raphal cells facilitate easier penetration of the ovipositor between raphal cell layers and those of the inner integument to reach this suitable oviposition site (Verkerke, 1987). In dioecious Ficus carica, seed flowers have a wetter stigma and obturator tissue whose secretions facilitate pollen tube growth through the style toward the micropyle (Beck and Lord, 1988) as occurs in other angiosperms (Losada and Herrero, 2017). However, gall flowers lack an obturator and have limited transmitting tissue secretions (Beck and Lord, 1988). A funnel-shaped opening in the style of gall flowers caused by the incomplete fusion of carpellary lobes and a cuticle lining the style in gall flowers likely facilitate style penetration by the ovipositor (Beck and Lord, 1988). In monoecious Ficus citrifolia, actively pollinating Pegoscapus wasps also oviposit between the nucellus and the inner integument of the ovary (Jansen-González et al., 2012). After mouth part sclerotization, the early instar larva feeds on hypertrophied nucellar tissue; later, after pollination, the endosperm proliferates and forms a syncytial nutritive tissue. Lack of pollination negatively affects larval development with an increased number of empty “galls” (bladders) signifying failed development (Jansen-González et al., 2012).
The non-pollinating galler Sycophaga sycomori enters Ficus sycomorus syconia through the ostiole and deposits its eggs into the embryo sac via the stylar route (Galil et al., 1970). Here, the proliferating nutritive tissue is nucellus and not endosperm, since even those syconia that are never pollinated (e.g., in Israel where pollinators are absent) result in completely developed syconia and production of parthenocarpic fruit. Similarly, in F. citrifolia, larvae of non-pollinating gall-inducing Idarnes species feed on hypertrophied nucellar tissue (Jansen-González et al., 2014). Although Idarnes oviposits into the syconium from the outside, its ovipositor uses the same route as the pollinators, i.e., insertion down the style (Elias et al., 2012), and the egg is deposited between the inner integument and nucellus (Jansen-González et al., 2014). That two unrelated gall-inducing wasps have converged on the same entry route to the identical egg deposition site in the same fig species, even though one wasp inserts its ovipositor from the exterior of the syconium, suggests ease of navigation via this route and/or abundant sensory cues that enable finding the right location. However, in the monoecious Ficus curtipes which lacks a synstigma, the Eupristina pollinator does not insert its ovipositor down the style, but, most unusually, penetrates the style much below the stigma; this may also occur in other monoecious species that lack a synstigma (Zhang F. P. et al., 2009). If such style-bypassing oviposition behavior can evolve, then why longer style length deters successful oviposition in female trees in gynodioecious figs needs to be addressed. Gall-inducing sycophiles Camarothorax and Otitesella in monoecious Ficus ingens oviposit into ovules through the syconium wall during the late pre-pollen receptive phase since the syconium wall and ovary are not sclerified at this stage, and are easy to penetrate (Baijnath and Naicker, 1989).
Sycophiles ovipositing before the pollen-receptive phase, induce large galls, much larger than pollinator galls, and may also negatively affect the growth of syconia (Zhang and Li, 2020). Such gallers may oviposit directly into the early syconium lumen, even before flower primordia are formed, e.g., Sycophaga stratheni in Ficus racemosa (Ghara et al., 2014; Yadav and Borges, 2018b); these gallers likely stimulate ectopic meristems within the syconium lumen. Knowledge of specific egg deposition sites will enable a better understanding of the galling process. Gall sizes are usually characteristic of galler species (Compton et al., 2018; Yadav and Borges, 2018b), and may structure the entire community of gallers, inquilines, and parasitoids occupying the syconium (Borges, 2015a; Compton et al., 2018).
Gall location within the syconium may contribute to successful reproduction. In some species, e.g., Ficus hispida, pollinators developing in the center of the fig syconium (closer to the lumen) have more mating opportunities, produce more offspring, and also have a more-female biased sex ratio (Peng et al., 2014), suggesting that gall location is important in reproductive success. For unknown reasons, gall failure in dioecious Ficus hirta occurred more frequently in peripheral galls, closer to the syconium wall (Yu et al., 2018). In Ficus hirta, the pedicels of pollinator male galls elongate to move them closer to the lumen, increasing the likelihood of escape from parasitoid attack (Yu and Compton, 2012). However, the opposite was observed in the monoecious Ficus racemosa (Li et al., 2016) suggesting a variety of processes at work in different fig species. In most fig species, male pollinators are vital to prevent fig syconia from becoming tomb blossoms wherein the developed female wasps are unable to leave their nursery owing to the lack of sufficient males that can cut through the syconium wall to release them. Whether special features and adaptations are at work to protect the successful development of male pollinators needs to be a focus of investigation. It appears that unusual behaviors in fig wasp males, that are vital to the survival of wasps in these enclosed brood-sites, are selected for under unusual circumstances. For example, in Israel, non-pollinating Sycophaga sycomori males cooperate to release females from the syconium by tunneling through the syconium wall, a behavior normally performed by male pollinators in East Africa (Galil and Eisikowitch, 1968). Such behavior is critical for the success of S. sycomori in Israel where the pollinator is absent. While pollinators only develop in male trees in dioecious species, non-pollinating gallers, ovipositing from the exterior, can develop in syconia of male and female trees (Wu et al., 2013); in such instances, male gallers release females from syconia on female trees (Wu et al., 2013). Similarly, male gall-inducing wasps Walkerella yashiroi and Sycobia hodites, associated with monoecious Ficus microcarpa and Ficus benjamina respectively, can release females independent of male pollinators (Wang et al., 2015; Farache et al., 2018b). How such behaviors have evolved is unknown. The large galls of Idarnes group flavicollis swell and block the ostiolar bracts, even entrapping pollinators at the syconium entrance (da Silva and Pereira, 2018). Since Idarnes requires pollinator males for release from syconia, a balance between pollinator and Idarnes galls within syconia is likely maintained over evolutionary time, but its mechanism is unknown.
The fig syconium is also host to inquilines, although their biology is barely understood (Cook and Rasplus, 2003). Inquilines are insects that parasitize a gall, starving out the galler larva and feeding on gall contents. In Ficus curtipes, the putative inquilines Diaziella yangi and Lipothymus sp. (Pteromalidae) oviposit into galls initiated by pollinators, but the galls and eclosing adults are larger than pollinators and pollinator galls, suggesting that these are secondary gallers, not strict inquilines, and capable of gall proliferation to some extent (Chen et al., 2013). Inquiline biology should clearly be an active area of research in this system.
A Plethora of Unanswered Questions in an Ideal Model System
The fig–fig wasp mutualism has attracted the attention of ecologists and evolutionary biologists. Consequently, a diversity of interactions within the syconial microcosms have been uncovered. However, there are many facets that still need investigation. A list of additional important lacunae is presented below; this list is certainly not exhaustive but may serve to inspire new work on this iconic and unusual mutualism between plants and gall-inducing insects.
While sanctions imposed by fig plants on cheating pollinators have been documented (Dunn, 2020), there are no studies on the mechanisms underlying these sanctions. Similarly, there is no research on mechanisms of pollen tube competition and female choice in figs compared to the rich literature on this aspect in other angiosperms (Erbar, 2003; Lora et al., 2019). How mate choice may be exercised in those fig species possessing synstigmas is enigmatic and completely unknown. The synstigma forms a compitum (transmission tract) for pollen tube competition which may also contribute to male reproductive success (Teixeira et al., 2021). We, therefore, need good plant embryology coupled with egg deposition and larval development, and also good insect oviposition behavior observations as in older classic papers (e.g., Johri and Konar, 1955; Galil and Snitzer-Pasternak, 1970; Galil and Eisikowitch, 1974). We need simple but elegant experiments; e.g., Ramya et al. (2011) demonstrated that pollinators use broken-off wing density at the ostiole (resulting from wing remains of foundresses already within the syconium) to make decisions on which syconium to enter. Such cues may determine foundress distribution across syconia within a plant helping to stabilize the mutualism (Borges, 2015b). We need to determine how syconia delay their development over longer or shorter time scales, from days to weeks to months. We need comparative studies of floral development across Ficus and its relatives (Leite et al., 2020).
We need sensory biology of oviposition coupled with the mechanobiology of ovipositor stiffness, steering, and egg-laying processes (Cerkvenik et al., 2019; Polidori and Wurdack, 2019; van Meer et al., 2020). That ovipositors of gallers can sense volatiles was established only for Sycophaga fusca in Ficus racemosa (Yadav and Borges, 2017) but must occur in other species also. We lack comparative studies of antennae and their sensilla as in cynipid gall wasps (Polidori and Nieves-Aldrey, 2014). We lack connections between life-history and sensory biology (Javoiš et al., 2019). We know nothing about waste management within the syconial microcosm as in aphid galls (Kutsukake et al., 2019) or how waste in a syconial microcosm, where hundreds of wasps develop, may be recycled. We require data on interactions between plant traits and larval trophic levels (Krishnan et al., 2015; Segar et al., 2018) and mode of feeding, since unusual reports of early instar larvae suspended in acellular gall fluid and ingesting a liquid diet (Yadav and Borges, 2018b) need wider validation.
We need observations that capture wasps in the act of a potential host shift (Michaloud et al., 2005). While monoecious figs often have large cross-continental populations serviced by single pollinator species or putative species complexes (Bain et al., 2016), they also share pollinators (Satler et al., 2019). Pollinator sharing is facilitated by matching flowering phenologies (Liu et al., 2015), but it must also be facilitated by the ability to enter syconia and induce galls, compatible development times, and so on. Similarly, dioecious species are likely pollinated by many different parapatric species of fig wasps that show genetic and morphological variation (Yu et al., 2019). We need a better understanding of the mechanisms supporting such variation. Experimental introduction of single foundress females into non-host figs resulted in successful development of more but smaller offspring, or fewer and larger offspring (Yang et al., 2012). The mechanisms underlying such results need to be discovered. While hybridization is frequently reported, we also need to understand why hybridization does not occur, e.g., possible polyploidy in Ficus tannoensis (Wachi et al., 2016).
Since syconia could be pollinated by just one foundress bringing in pollen from a single parent, this single source of pollen and of wasp eggs could give rise to traits of kin selection and cooperation between seed embryos (Bawa, 2016) and perhaps even among wasps. Sibling rivalry and parent–offspring conflicts affect brood size patterns in plants (Uma Shaanker et al., 1988) and placental animals (Fowden and Moore, 2012). However, seeds and pollinator galls usually lack obvious size variation, unless “bladders” or empty galls may be considered as possible outcomes of diverted resources resulting from resource conflict. This begs questions about sibling rivalry or lack thereof between pollinators developing in adjacent galls, and also between developing seeds. We, therefore, need genotyping of individual seeds and pollinator brood within syconia to answer these questions and to couple them with positional and growth effects in the syconium.
It is undoubtedly interesting that the majority of plant-associated arthropods with intrasexually selected weapons are gall-inducers, e.g., Acari, Thysanoptera, Hemiptera, Diptera, and Hymenoptera (Rico-Guevara and Hurme, 2019). Like the microcosm of the syconium, which selects for sexual dimorphism as well as for male intrasexual competition (Nelson and Greeff, 2009b), the microcosm of galls in other plant families could provide similar selection pressures. Research on sexual selection in fig wasps should inform and inspire investigations in other gall-inducing taxa.
The hallmark of the interaction between figs and gall-inducing fig wasps is simultaneous seed and wasp development within jointly constructed brood sites (Borges, 2017). However, this interaction can be perturbed by climate change in various ways. Global warming likely affects insect development (Rebaudo and Rabhi, 2018; Huey and Kingsolver, 2019). Higher temperatures result in smaller syconia and smaller wasps (Krishnan et al., 2014), and higher fig wasp mortality (Jevanandam et al., 2013). We urgently need more data on such aspects. That fig microcosms can become model systems for understanding the impacts of warming on insect diversity cannot be overemphasized.
Since the fig syconium develops into a fruit, effects of pollinators and dispersers on the evolution of syconium traits need to be integrated (Harrison et al., 2012; Leonard and Francis, 2017; Valenta et al., 2017). We, therefore, need crosstalk between pollination biologists, sensory ecologists, plant development biologists, insect physiologists, and evolutionary biologists since the intricacies of the fig microcosm will only be revealed with such collaboration. While there is asymmetry in evolutionary rates and generation times between fig trees and mutualistic fig wasps, which may lead to faster speciation rates in the wasps (Souto-Vilarós et al., 2019), the fig partners appear to have the upper hand, and may win the evolutionary race. Is this an example of the Red King effect (Borges, 2015b)? It is entirely possible that fig wasps are constantly chasing host figs because diverging too far away from the host would result in local extinctions. We could therefore be witnessing host chases rather than strict co-evolution (sensu Endara et al., 2017). Only good data on traits and trait evolution within a strong phylogenetic framework will provide the answers to such questions.
Author Contributions
The author confirms being the sole contributor of this work and has approved it for publication.
Funding
The work in my lab on figs and fig wasps has been generously funded over the years by the Indian Institute of Science (IISc); Ministry of Environment, Forests & Climate Change; Department of Biotechnology–IISc Partnership Programme; Department of Science and Technology; and the Indo-French Centre for the Promotion of Advanced Research.
Conflict of Interest
The author declares that the research was conducted in the absence of any commercial or financial relationships that could be construed as a potential conflict of interest.
Publisher’s Note
All claims expressed in this article are solely those of the authors and do not necessarily represent those of their affiliated organizations, or those of the publisher, the editors and the reviewers. Any product that may be evaluated in this article, or claim that may be made by its manufacturer, is not guaranteed or endorsed by the publisher.
Acknowledgments
I am grateful to all the students, researchers, and members of my lab who have made my work on figs and fig wasps possible, and the global fig biology scientific community that has made remarkable discoveries. I thank Priya B.R. for doing the illustrations. I also thank A. Raman for being an ever-constant source of encouragement on all matters related to gall inducers. I am also grateful to Don Miller and Makoto Tokuda for inviting me to contribute to this Special Research Topic.
References
Addicott, J. F., Bronstein, J., and Kjellberg, F. (1990). “Evolution of mutualistic life-cycles: yucca moths and fig wasps,” in Genetics, Evolution and Coordination of Insect Life Cycles, ed. F. Gilbert (London: Springer), 143–161. doi: 10.1007/978-1-4471-3464-0_10
Andreas, P., Kisiala, A., Emery, R. J., Clerck-Floate, D., Tooker, J. F., Price, P. W., et al. (2020). Cytokinins are abundant and widespread among insect species. Plants 9:208. doi: 10.3390/plants9020208
Baijnath, H., and Naicker, S. (1989). Developmental anatomy of Ficus ingens syconia in relation to its wasp faunula. South Afr. J. Bot. 55, 409–421. doi: 10.1016/s0254-6299(16)31164-4
Baijnath, H., and Ramcharun, S. (1983). Aspects of pollination and floral development in Ficus capensis Thunb. (Moraceae). Bothalia 14, 883–888. doi: 10.4102/abc.v14i3/4.1257
Bain, A., Borges, R. M., Chevallier, M. H., Vignes, H., Kobmoo, N., Peng, Y.-Q., et al. (2016). Geographic structuring into vicariant species-pairs in a wide-ranging, high-dispersal plant–insect mutualism: the case of Ficus racemosa and its pollinating wasps. Evol. Ecol. 30, 663–684. doi: 10.1007/s10682-016-9836-5
Barrett, S. C., and Hough, J. (2013). Sexual dimorphism in flowering plants. J. Exp. Bot. 64, 67–82. doi: 10.1093/jxb/ers308
Basso-Alves, J. P., Pereira, R. A. S., Peng, Y.-Q., and Teixeira, S. P. (2014). Different ontogenetic processes promote dicliny in Ficus L. (Moraceae). Acta Oecol. 57, 5–16. doi: 10.1016/j.actao.2013.02.012
Bawa, K. S. (2016). Kin selection and the evolution of plant reproductive traits. Proc. Roy. Soc. B 283:20160789. doi: 10.1098/rspb.2016.0789
Beck, N. G., and Lord, E. M. (1988). Breeding system in Ficus carica, the common fig. II. Pollination events. Am. J. Bot. 75, 1913–1922. doi: 10.1002/j.1537-2197.1988.tb11272.x
Biasazin, T. D., Larsson Herrera, S., Kimbokota, F., and Dekker, T. (2019). Translating olfactomes into attractants: shared volatiles provide attractive bridges for polyphagy in fruit flies. Ecol. Lett. 22, 108–118. doi: 10.1111/ele.13172
Borges, R. M. (2015a). How to be a fig wasp parasite on the fig–fig wasp mutualism. Curr. Opin. Insect Sci. 8, 34–40. doi: 10.1016/j.cois.2015.01.011
Borges, R. M. (2015b). How mutualisms between plants and insects are stabilized. Curr. Sci. 108, 1862–1868.
Borges, R. M. (2016). “On the air: broadcasting and reception of volatile messages in brood-site pollination mutualisms,” in Deciphering Chemical Language of Plant Communication, eds J. D. Blande and R. Glinwood (Cham: Springer), 227–255. doi: 10.1007/978-3-319-33498-1_10
Borges, R. M. (2017). Co-niche construction between hosts and symbionts: ideas and evidence. J. Genet. 96, 483–489. doi: 10.1007/s12041-017-0792-9
Borges, R. M. (2018). The galling truth: Limited knowledge of gall-associated volatiles in multitrophic interactions. Front. Plant Sci. 9:119. doi: 10.3389/fpls.2018.01139
Borges, R. M., Ranganathan, Y., Krishnan, A., Ghara, M., and Pramanik, G. (2011). When should fig fruit produce volatiles? Pattern in a ripening process. Acta Oecol. 37, 611–618. doi: 10.1016/j.actao.2011.06.003
Brown, D. E., Rashotte, A. M., Murphy, A. S., Normanly, J., Tague, B. W., Peer, W. A., et al. (2001). Flavonoids act as negative regulators of auxin transport in vivo in Arabidopsis. Plant Physiol. 126, 524–535. doi: 10.1104/pp.126.2.524
Cambier, S., Ginis, O., Moreau, S. J., Gayral, P., Hearn, J., Stone, G., et al. (2019). Gall wasp transcriptomes unravel potential effectors involved in molecular dialogues with oak and rose bushes. Front. Physiol. 10:926. doi: 10.3389/fphys.2019.00926
Carneiro, R. G., Isaias, R., Moreira, A. S., and Oliveira, D. C. (2017). Reacquisition of new meristematic sites determines the development of a new organ, the Cecidomyiidae gall on Copaifera langsdorffii Desf.(Fabaceae). Front. Plant Sci. 8:1622. doi: 10.3389/fpls.2017.01622
Cerkvenik, U., Van Leeuwen, J. L., Kovalev, A., Gorb, S. N., Matsumura, Y., and Gussekloo, S. W. (2019). Stiffness gradients facilitate ovipositor bending and spatial probing control in a parasitic wasp. J. Exp. Biol. 222:jeb195628. doi: 10.1242/jeb.195628
Chen, H. H., Yang, D. R., Gu, D., Compton, S. G., and Peng, Y.-Q. (2013). Secondary galling: a novel feeding strategy among ‘non-pollinating’ fig wasps from Ficus curtipes. Ecol. Entomol. 38, 381–389. doi: 10.1111/een.12030
Chen, H., Peng, Y., Zhang, Y., and Corlett, R. T. (2015). Winter cropping in Ficus tinctoria: an alternative strategy. Sci. Rep. 5:16496. doi: 10.1038/srep16496
Clement, W. L., Bruun-Lund, S., Cohen, A., Kjellberg, F., Weiblen, G. D., and Rønsted, N. (2020). Evolution and classification of figs (Ficus, Moraceae) and their close relatives (Castilleae) united by involucral bracts. Bot. J. Linn. Soc. 193, 316–339. doi: 10.1093/botlinnean/boaa022
Compton, S. G., Chen, X. Y., Chen, Y., Hatcher, M. J., Peng, Y.-Q., Quinnell, R. J., et al. (2018). Host-parasitoid relationships within figs of an invasive fig tree: a fig wasp community structured by gall size. Insect Conserv. Diver. 11, 341–351. doi: 10.1111/icad.12282
Cook, J. M., and Rasplus, J.-Y. (2003). Mutualists with attitude: coevolving fig wasps and figs. Trends Ecol. Evol. 18, 241–248. doi: 10.1016/S0169-5347(03)00062-4
Cook, J. M., Bean, D., Power, S. A., and Dixon, D. J. (2004). Evolution of a complex coevolved trait: active pollination in a genus of fig wasps. J. Evol. Biol. 17, 238–246. doi: 10.1111/j.1420-9101.2003.00683.x
Cook, J. M., Compton, S. G., Herre, E. A., and West, S. A. (1997). Alternative mating tactics and extreme male dimorphism in fig wasps. Proc. Roy. Soc. B 264, 747–754. doi: 10.1098/rspb.1997.0106
Cruaud, A., Jabbour-Zahab, R., Genson, G., Kjellberg, F., Kobmoo, N., van Noort, S., et al. (2011). Phylogeny and evolution of life-history strategies in the Sycophaginae non-pollinating fig wasps (Hymenoptera, Chalcidoidea). BMC Evol. Biol. 11:178. doi: 10.1186/1471-2148-11-178
Cruaud, A., Rønsted, N., Chantarasuwan, B., Chou, L. S., Clement, W. L., Couloux, A., et al. (2012). An extreme case of plant–insect codiversification: figs and fig-pollinating wasps. Syst. Biol. 61, 1029–1047. doi: 10.1093/sysbio/sys068
da Silva, P. C. A., and Pereira, R. A. S. (2018). How to be a good non-pollinating fig wasp: Galling wasps (Idarnes group flavicollis) do not interfere with the floral receptivity. Oecol. Austral. 22, 426–437. doi: 10.4257/oeco.2018.2204.06
Darwell, C. T., Segar, S. T., and Cook, J. M. (2018). Conserved community structure and simultaneous divergence events in the fig wasps associated with Ficus benjamina in Australia and China. BMC Ecol. 18:13. doi: 10.1186/s12898-018-0167-y
De Lillo, E., and Monfreda, R. (2004). ‘Salivary secretions’ of eriophyoids (Acari: Eriophyoidea): first results of an experimental model. Exp. Appl. Acarol. 34, 291–306. doi: 10.1023/B:APPA.0000049219.93796.11
Deng, J. Y., Fu, R. H., Compton, S. G., Hu, D. M., Zhang, L. S., Yang, F., et al. (2016). Extremely high proportions of male flowers and geographic variation in floral ratios within male figs of Ficus tikoua despite pollinators displaying active pollen collection. Ecol. Evol. 6, 607–619. doi: 10.1002/ece3.1926
Dodueva, I. E., Lebedeva, M. A., Kuznetsova, K. A., Gancheva, M. S., Paponova, S. S., and Lutova, L. L. (2020). Plant tumors: a hundred years of study. Planta 251:82. doi: 10.1007/s00425-020-03375-5
Dunn, D. (2020). Stability in fig tree–fig wasp mutualisms: how to be a cooperative fig wasp. Biol. J. Linn. Soc. 130, 1–17. doi: 10.1093/biolinnean/blaa027
Duthie, A. B., and Nason, J. D. (2016). Plant connectivity underlies plant-pollinator-exploiter distributions in Ficus petiolaris and associated pollinating and non-pollinating fig wasps. Oikos 125, 1597–1606. doi: 10.1111/oik.02905
Elias, L. G., Kjellberg, F., Farache, F. H. A., Almeida, E. A., Rasplus, J.-Y., Cruaud, A., et al. (2018b). Ovipositor morphology correlates with life history evolution in agaonid fig wasps. Acta Oecol. 90, 109–116. doi: 10.1016/j.actao.2017.10.007
Elias, L. G., Lino-Neto, J., and Pereira, R. A. S. (2018a). Oogenesis and ovarian morphology in pollinating and non-pollinating fig wasps: evidence from adult and immature stages. Invert. Reprod. Develop. 62, 162–168. doi: 10.1080/07924259.2018.1469549
Elias, L. G., Teixeira, S. P., Kjellberg, F., and Pereira, R. A. S. (2012). Diversification in the use of resources by Idarnes species: bypassing functional constraints in the fig–fig wasp interaction. Biol. J. Linn. Soc. 106, 114–122. doi: 10.1111/j.1095-8312.2012.01851.x
Endara, M. J., Coley, P. D., Ghabash, G., Nicholls, J. A., Dexter, K. G., Donoso, D. A., et al. (2017). Coevolutionary arms race versus host defense chase in a tropical herbivore–plant system. Proc. Natl. Acad. Sci. USA 114, E7499–E7505. doi: 10.1073/pnas.1707727114
Endress, P. K. (1979). Noncarpellary pollination and ‘hyperstigma’in an angiosperm (Tambourissa religiosa. Monimiaceae). Experientia 35, 45. doi: 10.1007/bf01917867
Erbar, C. (2003). Pollen tube transmitting tissue: place of competition of male gametophytes. Int. J. Plant Sci. 164, S265–S277. doi: 10.1086/377061
Espírito-Santo, M. M., and Fernandes, G. W. (2007). How many species of gall-inducing insects are there on earth, and where are they? Ann. Entomol. Soc. Am. 100, 95–99. doi: 10.1603/0013-87462007100[95:HMSOGI]2.0.CO;2
Ezedin, Z., and Weiblen, G. (2019). Additions and changes to Ficus (Moraceae) in New Guinea with comments on the world’s largest fig. Gard. Bull. Singapore 71(Suppl. 2), 197–216. doi: 10.26492/gbs71(suppl 2).2019-15
Fan, K. Y., Bain, A., Tzeng, H. Y., Chiang, Y. P., Chou, L. S., and Kuo-Huang, L. L. (2019). Comparative anatomy of the fig wall (Ficus, Moraceae). Botany 97, 417–426. doi: 10.1139/cjb-2018-0192
Farache, F. H. A., Cruaud, A., Rasplus, J.-Y., Cerezini, M. T., Rattis, L., Kjellberg, F., et al. (2018a). Insights into the structure of plant-insect communities: Specialism and generalism in a regional set of non-pollinating fig wasp communities. Acta Oecol. 90, 49–59. doi: 10.1016/j.actao.2018.02.006
Farache, F. H. A., Pereira, C. B., Koschnitzke, C., Barros, L. O., de Castro Souza, E. M., Felício, D. T., et al. (2018b). The unknown followers: Discovery of a new species of Sycobia Walker (Hymenoptera: Epichrysomallinae) associated with Ficus benjamina L. (Moraceae) in the Neotropical region. J. Hymenopt. Res. 67, 85–102. doi: 10.3897/jhr.67.29733
Farache, F. H., Cruaud, A., Genson, G., Rasplus, J.-Y., and Pereira, R. A. (2017). Taxonomic revision and molecular phylogenetics of the Idarnes incertus species-group (Hymenoptera, Agaonidae, Sycophaginae). PeerJ 5:e2842. doi: 10.7717/peerj.2842
Ferreira, B. G., Álvarez, R., Bragança, G. P., Alvarenga, D. R., Pérez-Hidalgo, N., and Isaias, R. M. (2019). Feeding and other gall facets: patterns and determinants in gall structure. Bot. Rev. 85, 78–106. doi: 10.1007/s12229-019-09207-w
Fowden, A. L., and Moore, T. (2012). Maternal-fetal resource allocation: co-operation and conflict. Placenta 33, e11–e15. doi: 10.1016/j.placenta.2012.05.002
Galil, J., and Eisikowitch, D. (1968). On the pollination ecology of Ficus sycomorus in East Africa. Ecology 49, 259–269. doi: 10.2307/1934454
Galil, J., and Eisikowitch, D. (1974). Further studies on pollination ecology in Ficus sycomorus II. Pocket filling and emptying by Ceratosolen arabicus Mayr. New Phytol. 73, 515–528. doi: 10.1111/j.1469-8137.1974.tb02130.x
Galil, J., and Snitzer-Pasternak, Y. (1970). Pollination in Ficus religiosa L. as connected with the structure and mode of action of the pollen pockets of Blastophaga quadraticeps Mayr. New Phytol. 69, 775–784. doi: 10.1111/j.1469-8137.1970.tb02462.x
Galil, J., Dulberger, R., and Rosen, D. (1970). The effects of Sycophaga sycomori L. on the structure and development of the synconia in Ficus sycomorus L. New Phytol. 69, 103–111. doi: 10.1111/j.1469-8137.1970.tb04054.x
Ganeshaiah, K. N., Kathuria, P., and Uma Shaanker, R. (1999). Does optimal packing of flowers in syconia shape style length variation in monoecious figs? Biotropica 31, 312–320. doi: 10.1111/j.1744-7429.1999.tb00143.x
Gates, D. J., and Nason, J. D. (2012). Flowering asynchrony and mating system effects on reproductive assurance and mutualism persistence in fragmented fig–fig wasp populations. Am. J. Bot. 99, 757–768. doi: 10.3732/ajb.1100472
Ghana, S., Suleman, N., and Compton, S. G. (2015a). A comparison of pollinator fig wasp development in figs of Ficus montana and its hybrids with Ficus asperifolia. Entomol. Exp. Appl. 156, 225–237. doi: 10.1111/eea.12338
Ghana, S., Suleman, N., and Compton, S. G. (2015b). Ability to gall: the ultimate basis of host specificity in fig wasps? Ecol. Entomol. 40, 280–291. doi: 10.1111/een.12183
Ghara, M., and Borges, R. M. (2010). Comparative life-history traits in a fig wasp community: implications for community structure. Ecol. Entomol. 35, 139–148. doi: 10.1111/j.1365-2311.2010.01176.x
Ghara, M., Kundanati, L., and Borges, R. M. (2011). Nature’s Swiss army knives: ovipositor structure mirrors ecology in a multitrophic fig wasp community. PLoS One 6:e23642. doi: 10.1371/journal.pone.0023642
Ghara, M., Ranganathan, Y., Krishnan, A., Gowda, V., and Borges, R. M. (2014). Divvying up an incubator: how parasitic and mutualistic fig wasps use space within their nursery microcosm. Arthropod-Plant Interac. 8, 191–203. doi: 10.1007/s11829-014-9300-9
Giron, D., Huguet, E., Stone, G. N., and Body, M. (2016). Insect-induced effects on plants and possible effectors used by galling and leaf-mining insects to manipulate their host-plant. J. Insect Physiol. 84, 70–89. doi: 10.1016/j.jinsphys.2015.12.009
Greeff, J. M., and Newman, D. V. (2011). Testing models of facultative sex ratio adjustment in the pollinating fig wasp Platyscapa awekei. Evolution 65, 203–219. doi: 10.1111/j.1558-5646.2010.01116.x
Greeff, J. M., van Noort, S., Rasplus, J.-Y., and Kjellberg, F. (2003). Dispersal and fighting in male pollinating fig wasps. C. R. Biol. 326, 121–130. doi: 10.1016/S1631-0691(03)00010-6
Greeff, J. M., van Vuuren, G. J., Kryger, P., and Moore, J. C. (2009). Outbreeding and possibly inbreeding depression in a pollinating fig wasp with a mixed mating system. Heredity 102, 349–356. doi: 10.1038/hdy.2009.2
Gu, D., Yang, D., Compton, S. G., and Peng, Y. (2012). Age at pollination modifies relative male and female reproductive success in a monoecious fig tree. Symbiosis 57, 73–81. doi: 10.1007/s13199-012-0178-y
Gu, H. F., Xiao, J. H., Dunn, D. W., Niu, L. M., Wang, B., Jia, L. Y., et al. (2014). Evidence for the circadian gene period as a proximate mechanism of protandry in a pollinating fig wasp. Biol. Lett. 10:20130914. doi: 10.1098/rsbl.2013.0914
Guiguet, A., Dubreuil, G., Harris, M. O., Appel, H. M., Schultz, J. C., Pereira, M. H., et al. (2016). Shared weapons of blood-and plant-feeding insects: surprising commonalities for manipulating hosts. J. Insect Physiol. 84, 4–21. doi: 10.1016/j.jinsphys.2015.12.006
Hamilton, W. D. (1967). Extraordinary sex ratios. Science 156, 477–478. doi: 10.1126/science.156.3774.477
Harris, M. O., and Pitzschke, A. (2020). Plants make galls to accommodate foreigners: some are friends, most are foes. New Phytol. 225, 1852–1872. doi: 10.1111/nph.16340
Harrison, R. D., Rønsted, N., Xu, L., Rasplus, J.-Y., and Cruaud, A. (2012). Evolution of fruit traits in Ficus subgenus Sycomorus (Moraceae): to what extent do frugivores determine seed dispersal mode? PLoS One 7:e38432. doi: 10.1371/journal.pone.0038432
Hearn, J., Blaxter, M., Schönrogge, K., Nieves-Aldrey, J. L., Pujade-Villar, J., Huguet, E., et al. (2019). Genomic dissection of an extended phenotype: Oak galling by a cynipid gall wasp. PLoS Genet. 15:e1008398. doi: 10.1371/journal.pgen.1008398
Heraty, J. M., Burks, R. A., Cruaud, A., Gibson, G. A., Liljeblad, J., Munro, J., et al. (2013). A phylogenetic analysis of the megadiverse Chalcidoidea (Hymenoptera). Cladistics 29, 466–542. doi: 10.1111/cla.12006
Herre, E. A., Jandér, K. C., and Machado, C. A. (2008). Evolutionary ecology of figs and their associates: recent progress and outstanding puzzles. Annu. Rev. Ecol. Evol. Syst. 39, 439–458. doi: 10.1146/annurev.ecolsys.37.091305.110232
Heslop-Harrison, Y., and Shivanna, K. R. (1977). The receptive surface of the angiosperm stigma. Ann. Bot. 41, 1233–1258. doi: 10.1093/oxfordjournals.aob.a085414
Hossaert-McKey, M., and Bronstein, J. L. (2001). Self-pollination and its costs in a monoecious fig (Ficus aurea, Moraceae) in a highly seasonal subtropical environment. Am. J. Bot. 88, 685–692. doi: 10.2307/2657069
Hossaert-McKey, M., Proffit, M., Soler, C. C. L., Chen, C., Bessière, J.-M., Schatz, B., et al. (2016). How to be a dioecious fig: Chemical mimicry between sexes matters only when both sexes flower synchronously. Sci. Rep. 6:21236. doi: 10.1038/srep21236
Hossaert-McKey, M., Soler, C., Schatz, B., and Proffit, M. (2010). Floral scents: their roles in nursery pollination mutualisms. Chemoecology 20, 75–88. doi: 10.1007/s00049-010-0043-5
Hu, H.-Y., Niu, L. -M., Ma, G. -C., Fu, Y. -G., Peng, Z. -Q., and Huang, D.-W. (2010). Permeability of receptive fig fruits and its effects on the re-emergence behaviour of pollinators. Ecol. Entomol. 35, 115–125. doi: 10.1111/j.1365-2311.2009.01170.x
Hu, R., Sun, P., Yu, H., Cheng, Y., Wang, R., Chen, X., et al. (2020). Similitudes and differences between two closely related Ficus species in the synthesis by the ostiole of odors attracting their host-specific pollinators: A transcriptomic based investigation. Acta Oecol. 105:103554. doi: 10.1016/j.actao.2020.103554
Huey, R. B., and Kingsolver, J. G. (2019). Climate warming, resource availability, and the metabolic meltdown of ectotherms. Am. Nat. 194, E140–E150. doi: 10.1086/705679
Ikegami, H., Nogata, H., Inoue, Y., Himeno, S., Yakushiji, H., Hirata, C., et al. (2013). Expression of FcFT1, a FLOWERING LOCUS T-like gene, is regulated by light and associated with inflorescence differentiation in fig (Ficus carica L.). BMC Plant Biol. 13:216. doi: 10.1186/1471-2229-13-216
Jansen-González, S., Teixeira, S. P., and Pereira, R. A. S. (2012). Mutualism from the inside: coordinated development of plant and insect in an active pollinating fig wasp. Arthropod-Plant Interac. 6, 601–609. doi: 10.1007/s11829-012-9203-6
Jansen-González, S., Teixeira, S. P., Kjellberg, F., and Pereira, R. A. S. (2014). Same but different: Larval development and gall-inducing process of a non-pollinating fig wasp compared to that of pollinating fig-wasps. Acta Oecol. 57, 44–50. doi: 10.1016/j.actao.2013.07.003
Javoiš, J., Davis, R. B., and Tammaru, T. (2019). A comparative morphometric study of sensory capacity in geometrid moths. J. Evol. Biol. 32, 380–389. doi: 10.1111/jeb.13422
Jevanandam, N., Goh, A. G., and Corlett, R. T. (2013). Climate warming and the potential extinction of fig wasps, the obligate pollinators of figs. Biol. Lett. 9:20130041. doi: 10.1098/rsbl.2013.0041
Johri, B. M., and Konar, R. N. (1955). A contribution to the morphology and embryology of Ficus religiosa Linn. Curr. Sci. 24, 382–385.
Jousselin, E., and Kjellberg, F. (2001). The functional implications of active and passive pollination in dioecious figs. Ecol. Lett. 4, 151–158. doi: 10.1046/j.1461-0248.2001.00209.x
Jousselin, E., Hossaert-McKey, M., Herre, E. A., and Kjellberg, F. (2003). Why do fig wasps actively pollinate monoecious figs? Oecologia 134, 381–387. doi: 10.1007/s00442-002-1116-0
Jousselin, E., Kjellberg, F., and Herre, E. A. (2004). Flower specialization in a passively pollinated monoecious fig: a question of style and stigma? Int. J. Plant Sci. 165, 587–593. doi: 10.1086/386558
Jousselin, E., Rasplus, J.-Y., and Kjellberg, F. (2001). Shift to mutualism in parasitic lineages of the fig/fig wasp interaction. Oikos 94, 287–294. doi: 10.1034/j.1600-0706.2001.940209.x
Kathuria, P., Ganeshaiah, K. N., Uma Shaanker, R., and Vasudeva, R. (1995). Is there dimorphism for style lengths in monoecious figs? Curr. Sci. 68, 1047–1050.
Kjellberg, F., Gouyon, P. H., Ibrahim, M., Raymond, M., and Valdeyron, G. (1987). The stability of the symbiosis between dioecious figs and their pollinators: a study of Ficus carica L. and Blastophaga psenes L. Evolution 41, 693–704. doi: 10.2307/2408881
Kjellberg, F., Jousselin, E., Hossaert-McKey, M., and Rasplus, J.-Y. (2005). “Biology, ecology, and evolution of fig-pollinating wasps (Chalcidoidea, Agaonidae),” in Biology, Ecology and Evolution of Gall-inducing Arthropods, Vol. 2, eds A. Raman, C. W. Schaefer, and T. M. Withers (Enfield, NH: Sciences Publishers), 539–572.
Krishnan, A., and Borges, R. M. (2014). Parasites exert conflicting selection pressures to affect reproductive asynchrony of their host plant in an obligate pollination mutualism. J. Ecol. 102, 1329–1340. doi: 10.1111/1365-2745.12277
Krishnan, A., Ghara, M., Kasinathan, S., Pramanik, G. K., Revadi, S., and Borges, R. M. (2015). Plant reproductive traits mediate tritrophic feedback effects within an obligate brood-site pollination mutualism. Oecologia 179, 797–809. doi: 10.1007/s00442-015-3372-9
Krishnan, A., Pramanik, G. K., Revadi, S. V., Venkateswaran, V., and Borges, R. M. (2014). High temperatures result in smaller nurseries which lower reproduction of pollinators and parasites in a brood site pollination mutualism. PLoS One 9:e115118. doi: 10.1371/journal.pone.0115118
Kutsukake, M., Uematsu, K., and Fukatsu, T. (2019). Plant manipulation by gall-forming social aphids for waste management. Front. Plant Sci. 10:933. doi: 10.3389/fpls.2019.00933
Lee, Z. H., Hirakawa, T., Yamaguchi, N., and Ito, T. (2019). The roles of plant hormones and their interactions with regulatory genes in determining meristem activity. Int. J. Mol. Sci. 20:4065. doi: 10.3390/ijms20164065
Leite, V. G., Basso-Alves, J. P., Gualberto, A. R. S., and Teixeira, S. P. (2020). A comparative ontogenetic approach to understanding the pseudomonomerous gynoecium in Moraceae. Int. J. Plant Sci. 181, 241–255. doi: 10.1086/706452
Leonard, A. S., and Francis, J. S. (2017). Plant–animal communication: past, present and future. Evol. Ecol. 31, 143–151. doi: 10.1007/s10682-017-9884-5
Li, Z. T., Peng, Y. Q., Wen, X. L., and Jandér, K. C. (2016). Selective resource allocation may promote a sex ratio in pollinator fig wasps more beneficial for the host tree. Sci. Rep. 6:35159. doi: 10.1038/srep35159
Liu, C., Yang, D.-R., Compton, S. G., and Peng, Y.-Q. (2013). Larger fig wasps are more careful about which figs to enter–with good reason. PLoS One 8:e74117. doi: 10.1371/journal.pone.0074117
Liu, G.-X., Yang, D.-R., Peng, Y.-Q., and Compton, S. G. (2015). Complementary fruiting phenologies facilitate sharing of one pollinator fig wasp by two fig trees. J. Plant Ecol. 8, 197–206. doi: 10.1093/jpe/rtv022
Lora, J., Laux, T., and Hormaza, J. I. (2019). The role of the integuments in pollen tube guidance in flowering plants. New Phytol. 221, 1074–1089. doi: 10.1111/nph.15420
Losada, J. M., and Herrero, M. (2017). Pollen tube access to the ovule is mediated by glycoprotein secretion on the obturator of apple (Malus× domestica, Borkh). Ann. Bot. 119, 989–1000. doi: 10.1093/aob/mcw276
Lu, J., Sun, J., Jiang, A., Bai, M., Fan, C., Liu, J., et al. (2020). Alternate expression of CONSTANS-LIKE 4 in short days and CONSTANS in long days facilitates day-neutral response in Rosa chinensis. J. Exp. Bot. 71, 4057–4068. doi: 10.1093/jxb/eraa161
Ma, N., An, Y., Li, J., and Wang, L. (2020). Cloning and characterization of a homologue of the FLORICAULA/LEAFY gene in Ficus carica L., FcLFY, and its role in flower bud differentiation. Sci. Hortic. 261:109014. doi: 10.1016/j.scienta.2019.109014
Machado, A. F. P., de Souza, A. M., and Leitão, C. A. E. (2013). Secretory structures at syconia and flowers of Ficus enormis (Moraceae): A specialization at ostiolar bracts and the first report of inflorescence colleters. Flora 208, 45–51. doi: 10.1016/j.flora.2012.12.005
Marinho, C. R., and Teixeira, S. P. (2019). Novel reports of laticifers in Moraceae and Urticaceae: revisiting synapomorphies. Plant Syst. Evol. 305, 13–31. doi: 10.1007/s00606-018-1548-6
Marinho, C. R., Pereira, R. A. S., Peng, Y.-Q., and Teixeira, S. P. (2018). Laticifer distribution in fig inflorescence and its potential role in the fig-fig wasp mutualism. Acta Oecol. 90, 160–167. doi: 10.1016/j.actao.2017.10.005
Martinson, E. O., Hackett, J. D., Machado, C. A., and Arnold, A. E. (2015). Metatranscriptome analysis of fig flowers provides insights into potential mechanisms for mutualism stability and gall induction. PLoS One 10:e0130745. doi: 10.1371/journal.pone.0130745
Martinson, E. O., Jandér, K. C., Peng, Y.-Q., Chen, H. H., Machado, C. A., Arnold, A. E., et al. (2014). Relative investment in egg load and poison sac in fig wasps: Implications for physiological mechanisms underlying seed and wasp production in figs. Acta Oecol. 57, 58–66. doi: 10.1016/j.actao.2013.07.009
Michaloud, G., Bossu-Dupriez, N., Chevolot, M., and Lasbleiz, C. (2005). Pollen waste and unrelated traits in a fig–fig wasp symbiosis: a new behaviour suggesting a host shift. C. R. Biol. 328, 81–87. doi: 10.1016/j.crvi.2004.11.002
Miller, D. G. III, and Raman, A. (2019). Host–plant relations of gall-inducing insects. Ann. Entomol. Soc. Am. 112, 1–19. doi: 10.1093/aesa/say034
Moore, J. C., and Greeff, J. M. (2003). Resource defence in female pollinating fig wasps: two’s a contest, three’s a crowd. Anim. Behav. 66, 1101–1107. doi: 10.1006/anbe.2003.2304
Murray, M. G. (1985). Figs (Ficus spp.) and fig wasps (Chalcidoidea, Agaonidae): hypotheses for an ancient symbiosis. Biol. J. Linn. Soc. 26, 69–81. doi: 10.1111/j.1095-8312.1985.tb01552.x
Nelson, R. M., and Greeff, J. M. (2009a). Evolution of the scale and manner of brother competition in pollinating fig wasps. Anim. Behav. 77, 693–700. doi: 10.1016/j.anbehav.2008.12.003
Nelson, R. M., and Greeff, J. M. (2009b). Male pollinator fig wasps (Chalcidoidea: Agaonidae) do not need an arena to fight. Afr. Entomol. 17, 228–231. doi: 10.4001/003.017.0215
Oliveira, D. C., Isaias, R. M. S., Fernandes, G. W., Ferreira, B. G., Carneiro, R. G. S., and Fuzaro, L. (2016). Manipulation of host plant cells and tissues by gall-inducing insects and adaptive strategies used by different feeding guilds. J. Insect Physiol. 84, 103–113. doi: 10.1016/j.jinsphys.2015.11.012
Peer, W. A., and Murphy, A. S. (2007). Flavonoids and auxin transport: modulators or regulators? Trends Plant Sci. 12, 556–563. doi: 10.1016/j.tplants.2007.10.003
Pellmyr, O., Kjellberg, F., Herre, E. A., Kawakita, A., Hembry, D. H., Holland, J. N., et al. (2020). Active pollination drives selection for reduced pollen-ovule ratios. Am. J. Bot. 107, 164–170. doi: 10.1002/ajb2.1412
Peng, Y.-Q., Zhang, Y., Compton, S. G., and Yang, D. R. (2014). Fig wasps from the centre of figs have more chances to mate, more offspring and more female-biased offspring sex ratios. Anim. Behav. 98, 19–25. doi: 10.1016/j.anbehav.2014.09.017
Peters, R. S., Niehuis, O., Gunkel, S., Bläser, M., Mayer, C., Podsiadlowski, L., et al. (2018). Transcriptome sequence-based phylogeny of chalcidoid wasps (Hymenoptera: Chalcidoidea) reveals a history of rapid radiations, convergence, and evolutionary success. Mol. Phylogenet. Evol. 120, 286–296. doi: 10.1016/j.ympev.2017.12.005
Polidori, C., and Nieves-Aldrey, J. L. (2014). Diverse filters to sense: great variability of antennal morphology and sensillar equipment in gall-wasps (Hymenoptera: Cynipidae). PLoS One 9:e101843. doi: 10.1371/journal.pone.0101843
Polidori, C., and Wurdack, M. (2019). Mg-enriched ovipositors as a possible adaptation to hard-skinned fruit oviposition in Drosophila suzukii and D. subpulchrella. Arthropod-Plant Interact. 13, 551–560. doi: 10.1007/s11829-018-9641-x
Powell, J. A. (2001). Longest insect dormancy: Yucca moth larvae (Lepidoptera: Prodoxidae) metamorphose after 20, 25, and 30 years in diapause. Ann. Entomol. Soc. Am. 94, 677–680. doi: 10.1603/0013-87462001094[0677:LIDYML]2.0.CO;2
POWO (2020). Plants of the World Online. Facilitated by the Royal Botanic Gardens, Kew. Available online at: http://www.plantsoftheworldonline.org.(Accessed 16 July 2020)
Proffit, M., Lapeyre, B., Buatois, B., Deng, X. X., Arnal, P., Gouzerh, F., et al. (2020). Chemical signal is in the blend: bases of plant-pollinator encounter in a highly specialized interaction. Sci. Rep. 10:10071. doi: 10.1038/s41598-020-66655-w
Raja, S., Suleman, N., and Compton, S. G. (2014). Foundress fig wasps are more likely to re-emerge from older figs. J. Insect Behav. 27, 786–790. doi: 10.1007/s10905-014-9469-x
Raja, S., Suleman, N., and Compton, S. G. (2016). Floral constraint resulting from intersexual mimicry in a gynodioecious fig tree. Entomol. Sci. 19, 290–295. doi: 10.1111/ens.12202
Raja, S., Suleman, N., Compton, S. G., and Moore, J. C. (2008). The mechanism of sex ratio adjustment in a pollinating fig wasp. Proc. Roy. Soc. B 275, 1603–1610. doi: 10.1098/rspb.2008.0136
Ramírez-Benavides, W. (1974). Coevolution of Ficus and Agaonidae. Ann. Miss. Bot. Gard. 61, 770–780. doi: 10.2307/2395028
Ramírez-Benavides, W. (2007). Pollination analogies between Orchidaceae, Ficus (Moraceae) and Asclepiadaceae. Lankesteriana 7, 450–457. doi: 10.15517/lank.v7i1-2.19668
Ramos, M. V., Demarco, D., Souza, I. C. C., and de Freitas, C. D. T. (2019). Laticifers, latex, and their role in plant defense. Trends Plant Sci. 24, 553–567. doi: 10.1016/j.tplants.2019.03.006
Ramya, K. T., Fiyaz, R. A., Uma Shaanker, R., and Ganeshaiah, K. N. (2011). Pollinators for a syconium: How do wasps choose among syconia? Curr. Sci. 101, 520–527.
Rasplus, J.-Y., Rodriguez, L. J., Sauné, L., Peng, Y.-Q., Bain, A., Kjellberg, F., et al. (2020). Exploring systematic biases, rooting methods and morphological evidence to unravel the evolutionary history of the genus Ficus (Moraceae). Cladistics 2020:42259. doi: 10.1101/2020.04.15.042259
Rebaudo, F., and Rabhi, V. B. (2018). Modeling temperature-dependent development rate and phenology in insects: review of major developments, challenges, and future directions. Entomol. Exp. Appl. 166, 607–617. doi: 10.1111/eea.12693
Rico-Guevara, A., and Hurme, K. J. (2019). Intrasexually selected weapons. Biol. Rev. 94, 60–101. doi: 10.1111/brv.12436
Sakai, S. (2002). A review of brood-site pollination mutualism: plants providing breeding sites for their pollinators. J. Plant Res. 115, 161–168. doi: 10.1007/s102650200021
Satler, J. D., Herre, E. A., Jandér, K. C., Eaton, D. A., Machado, C. A., Heath, T. A., et al. (2019). Inferring processes of coevolutionary diversification in a community of Panamanian strangler figs and associated pollinating wasps. Evolution 73, 2295–2311. doi: 10.1111/evo.13809
Schultz, J. C., Edger, P. P., Body, M. J., and Appel, H. M. (2019). A galling insect activates plant reproductive programs during gall development. Sci. Rep. 9:1833. doi: 10.1038/s41598-018-38475-6
Segar, S. T., Mardiastuti, A., Wheeler, P. M., and Cook, J. M. (2018). Detecting the elusive cost of parasites on fig seed production. Acta Oecol. 90, 69–74. doi: 10.1016/j.actao.2018.03.002
Segar, S. T., Pereira, R. A., Compton, S. G., and Cook, J. M. (2013). Convergent structure of multitrophic communities over three continents. Ecol. Lett. 16, 1436–1445. doi: 10.1111/ele.12183
Shanahan, M., So, S., Compton, S. G., and Corlett, R. (2001). Fig-eating by vertebrate frugivores: a global review. Biol. Rev. 76, 529–572. doi: 10.1017/S1464793101005760
Shi, M., Li, Y., Deng, S., Wang, D., Chen, Y., Yang, S., et al. (2019). The formation and accumulation of protein-networks by physical interactions in the rapid occlusion of laticifer cells in rubber tree undergoing successive mechanical wounding. BMC Plant Biol. 19:8. doi: 10.1186/s12870-018-1617-6
Singh, R. K., Svystun, T., AlDahmash, B., Jönsson, A. M., and Bhalerao, R. P. (2017). Photoperiod-and temperature-mediated control of phenology in trees–a molecular perspective. New Phytol. 213, 511–524. doi: 10.1111/nph.14346
Souto-Vilarós, D., Machac, A., Michalek, J., Darwell, C. T., Sisol, M., Kuyaiva, T., et al. (2019). Faster speciation of fig-wasps than their host figs leads to decoupled speciation dynamics: snapshots across the speciation continuum. Mol. Ecol. 28, 3958–3976. doi: 10.1111/mec.15190
Souza, C. D., Pereira, R. A., Marinho, C. R., Kjellberg, F., and Teixeira, S. P. (2015). Diversity of fig glands is associated with nursery mutualism in fig trees. Am. J. Bot. 102, 1564–1577. doi: 10.3732/ajb.1500279
Suleman, N., Raja, S., and Compton, S. G. (2012). Only pollinator fig wasps have males that collaborate to release their females from figs of an Asian fig tree. Biol. Lett. 8, 344–346. doi: 10.1098/rsbl.2011.1016
Suleman, N., Raja, S., Quinnell, R. J., and Compton, S. G. (2013). Putting your eggs in several baskets: oviposition in a wasp that walks between several figs. Entomol. Exp. Appl. 149, 85–93. doi: 10.1111/eea.12108
Takeda, S., Yoza, M., Amano, T., Ohshima, I., Hirano, T., Sato, M. H., et al. (2019). Comparative transcriptome analysis of galls from four different host plants suggests the molecular mechanism of gall development. PLoS One 14:e0223686. doi: 10.1371/journal.pone.0223686
Teixeira, S. P., Costa, M. F., Basso-Alves, J. P., Kjellberg, F., and Pereira, R. A. (2018). Morphological diversity and function of the stigma in Ficus species (Moraceae). Acta Oecol. 90, 117–131. doi: 10.1016/j.actao.2018.02.008
Teixeira, S. P., Costa, M. F., Basso-Alves, J. P., Kjellberg, F., and Pereira, R. A. S. (2021). The synstigma turns the fig into a large flower. Bot. J. Linn. Soc. 195, 93–105. doi: 10.1093/botlinnean/boaa061
Teixeira, S. P., Marinho, C. R., and Leme, F. M. (2020). “Structural diversity and distribution of laticifers,” in Latex, Laticifers and Their Molecular Components, Vol. 93, ed. R. Nowrot (Amsterdam: Elsevier), 27–54. doi: 10.1016/bs.abr.2019.09.003
Thorogood, C., Dalton, N., Irvine, A., and Hiscock, S. (2018). The reproductive biology of two poorly known relatives of the fig (Ficus) and insights into the evolution of the fig syconium. Nordic J. Bot. 36:01832. doi: 10.1111/njb.01832
Uma Shaanker, R., Ganeshaiah, K. N., and Bawa, K. S. (1988). Parent-offspring conflict, sibling rivalry, and brood size patterns in plants. Annu. Rev. Ecol. Syst. 19, 177–205. doi: 10.1146/annurev.es.19.110188.001141
Valenta, K., Nevo, O., Martel, C., and Chapman, C. A. (2017). Plant attractants: integrating insights from pollination and seed dispersal ecology. Evol. Ecol. 31, 249–267. doi: 10.1007/s10682-016-9870-3
van Meer, N. M., Cerkvenik, U., Schlepütz, C. M., van Leeuwen, J. L., and Gussekloo, S. W. (2020). The ovipositor actuation mechanism of a parasitic wasp and its functional implications. J. Anat. 237, 689–703. doi: 10.1111/joa.13216
van Noort, S., and Compton, S. G. (1996). Convergent evolution of agaonine and sycoecine (Agaonidae, Chalcidoidea) head shape in response to the constraints of host fig morphology. J. Biogeogr. 23, 415–424. doi: 10.1111/j.1365-2699.1996.tb00003.x
Venkateswaran, V., and Borges, R. M. (2021). Staying in the club: Exploring criteria governing metacommunity membership for obligate symbionts under host–symbiont feedback. J. Theor. Biol. 510:110512. doi: 10.1016/j.jtbi.2020.110512
Venkateswaran, V., Kumble, A. L., and Borges, R. M. (2018). Resource dispersion influences dispersal evolution of highly insulated insect communities. Biol. Lett. 14:20180111. doi: 10.1098/rsbl.2018.0111
Verkerke, W. (1987). Ovule dimorphism in Ficus asperifolia Miquel. Acta Bot. Neerl. 36, 121–124. doi: 10.1111/j.1438-8677.1987.tb01983.x
Verkerke, W. (1989). Structure and function of the fig. Experientia 45, 612–622. doi: 10.1007/bf01975678
Wachi, N., Kusumi, J., Tzeng, H. Y., and Su, Z. H. (2016). Genome-wide sequence data suggest the possibility of pollinator sharing by host shift in dioecious figs (Moraceae, Ficus). Mol. Ecol. 25, 5732–5746. doi: 10.1111/mec.13876
Wang, A. Y., Peng, Y.-Q., Harder, L. D., Huang, J. F., Yang, D. R., Zhang, D. Y., et al. (2019). The nature of interspecific interactions and co-diversification patterns, as illustrated by the fig microcosm. New Phytol. 224, 1304–1315. doi: 10.1111/nph.16176
Wang, H., Ridley, J., Dunn, D. W., Wang, R., Cook, J. M., and Yu, D. W. (2013). Biased oviposition and biased survival together help resolve a fig–wasp conflict. Oikos 122, 533–540. doi: 10.1111/j.1600-0706.2012.20463.x
Wang, R., Segar, S. T., Harper, M., Yu, H., Quinnell, R. J., and Compton, S. G. (2015). Between-species facilitation by male fig wasps in shared figs. Ecol. Entomol. 40, 428–436. doi: 10.1111/een.12201
Wang, Z. J., Peng, Y.-Q., Compton, S. G., and Yang, D. R. (2010). Reproductive strategies of two forms of flightless males in a non-pollinating fig wasp under partial local mate competition. Ecol. Entomol. 35, 691–697. doi: 10.1111/j.1365-2311.2010.01228.x
Wei, Z. D., Kobmoo, N., Cruaud, A., and Kjellberg, F. (2014). Genetic structure and hybridization in the species group of Ficus auriculata: can closely related sympatric Ficus species retain their genetic identity while sharing pollinators? Mol. Ecol. 23, 3538–3550. doi: 10.1111/mec.12825
Weiblen, G. D. (2000). Phylogenetic relationships of functionally dioecious Ficus (Moraceae) based on ribosomal DNA sequences and morphology. Am. J. Bot. 87, 1342–1357. doi: 10.2307/2656726
Weiblen, G. D. (2002). How to be a fig wasp. Annu. Rev. Entomol. 47, 299–330. doi: 10.1146/annurev.ento.47.091201.145213
Weis, A. E., Walton, R., and Crego, C. L. (1988). Reactive plant tissue sites and the population biology of gall makers. Annu. Rev. Entomol. 33, 467–486. doi: 10.1146/annurev.en.33.010188.002343
West, S. A., and Herre, E. A. (1994). The ecology of the New World fig-parasitizing wasps Idarnes and implications for the evolution of the fig–pollinator mutualism. Proc. Roy. Soc. B 258, 67–72. doi: 10.1098/rspb.1994.0143
Wiebes, J. T. (1979). Co-evolution of figs and their insect pollinators. Annu. Rev. Ecol. Syst. 10, 1–12. doi: 10.1146/annurev.es.10.110179.000245
Wu, T., Dunn, D. W., Hu, H. Y., Niu, L. M., Xiao, J. H., Pan, X. L., et al. (2013). The occurrence of fig wasps in the fruits of female gynodioecious fig trees. Acta Oecol. 46, 33–38. doi: 10.1016/j.actao.2012.10.009
Yadav, P., and Borges, R. M. (2017). The insect ovipositor as a volatile sensor within a closed microcosm. J. Exp. Biol. 220, 1554–1557. doi: 10.1242/jeb.152777
Yadav, P., and Borges, R. M. (2018a). Why resource history matters: Age and oviposition history affect oviposition behaviour in exploiters of a mutualism. Ecol. Entomol. 43, 473–482. doi: 10.1111/een.12520
Yadav, P., and Borges, R. M. (2018b). Host–parasitoid development and survival strategies in a non-pollinating fig wasp community. Acta Oecol. 90, 60–68. doi: 10.1016/j.actao.2017.04.001
Yadav, P., Desireddy, S., Kasinathan, S., Bessière, J.-M., and Borges, R. M. (2018). History matters: oviposition resource acceptance in an exploiter of a nursery pollination mutualism. J. Chem. Ecol. 44, 18–28. doi: 10.1007/s10886-017-0914-0
Yamaguchi, H., Tanaka, H., Hasegawa, M., Tokuda, M., Asami, T., and Suzuki, Y. (2012). Phytohormones and willow gall induction by a gall-inducing sawfly. New Phytol. 196, 586–595. doi: 10.1111/j.1469-8137.2012.04264.x
Yang, L. Y., Machado, C. A., Dang, X. D., Peng, Y.-Q., Yang, D. R., Zhang, D. Y., et al. (2015). The incidence and pattern of copollinator diversification in dioecious and monoecious figs. Evolution 69, 294–304. doi: 10.1111/evo.12584
Yang, P., Li, Z., Peng, Y., and Yang, D. (2012). Exchange of hosts: can agaonid fig wasps reproduce successfully in the figs of non-host Ficus? Naturwissenschaften 99, 199–205. doi: 10.1007/s00114-012-0885-5
Yu, H., and Compton, S. G. (2012). Moving your sons to safety: galls containing male fig wasps expand into the centre of figs, away from enemies. PLoS One 7:e30833. doi: 10.1371/journal.pone.0030833
Yu, H., Compton, S. G., and Wu, L. (2018). Spatial variation in pollinator gall failure within figs of the gynodioecious Ficus hirta. Acta Oecol. 90, 75–80. doi: 10.1016/j.actao.2017.11.009
Yu, H., Tian, E., Zheng, L., Deng, X., Cheng, Y., Chen, L., et al. (2019). Multiple parapatric pollinators have radiated across a continental fig tree displaying clinal genetic variation. Mol. Ecol. 28, 2391–2405. doi: 10.1111/mec.15046
Zhang, F. P., Peng, Y.-Q., Compton, S. G., and Yang, D. R. (2009). Floral characteristics of Ficus curtipes and the oviposition behavior of its pollinator fig wasp. Ann. Entomol. Soc. Am. 102, 556–559. doi: 10.1603/008.102.0328
Zhang, F., Peng, Y., Compton, S. G., Zhao, Y., and Yang, D. (2009). Host pollination mode and mutualist pollinator presence: net effect of internally ovipositing parasite in the fig–wasp mutualism. Naturwissenschaften 96, 543–549. doi: 10.1007/s00114-008-0502-9
Zhang, Q., Onstein, R. E., Little, S. A., and Sauquet, H. (2019). Estimating divergence times and ancestral breeding systems in Ficus and Moraceae. Ann. Bot. 123, 191–204. doi: 10.1093/aob/mcy159
Zhang, X. W., and Li, L. H. (2020). An early gall-inducing parasitic wasp adversely affects the fitness of its host Ficus tree but not the pollinator. Sci. Rep. 10:14941. doi: 10.1038/s41598-020-71738-9
Zhang, X. W., Dunn, D. W., and Wang, R. W. (2020). Egg load is a cue for offspring sex ratio adjustment in a fig-pollinating wasp with male-eggs-first sex allocation. J. Evol. Biol. 33, 366–376. doi: 10.1111/jeb.13572
Zhang, Y., Yang, D. R., Peng, Y.-Q., and Compton, S. G. (2012). Costs of inflorescence longevity for an Asian fig tree and its pollinator. Evol. Ecol. 26, 513–527. doi: 10.1007/s10682-011-9525-3
Zhao, J. B., Peng, Y.-Q., Quinnell, R. J., Compton, S. G., and Yang, D. R. (2014). A switch from mutualist to exploiter is reflected in smaller egg loads and increased larval mortalities in a ‘cheater’ fig wasp. Acta Oecol. 57, 51–57. doi: 10.1016/j.actao.2013.04.003
Keywords: Ficus, fig wasps, gall induction, microcosm, multitrophic interactions, mutualism, parasitism
Citation: Borges RM (2021) Interactions Between Figs and Gall-Inducing Fig Wasps: Adaptations, Constraints, and Unanswered Questions. Front. Ecol. Evol. 9:685542. doi: 10.3389/fevo.2021.685542
Received: 25 March 2021; Accepted: 08 July 2021;
Published: 10 August 2021.
Edited by:
Makoto Tokuda, Saga University, JapanReviewed by:
Iain James Gordon, Australian National University, AustraliaMaría Del Carmen Viera, Universidad de la República, Uruguay
Copyright © 2021 Borges. This is an open-access article distributed under the terms of the Creative Commons Attribution License (CC BY). The use, distribution or reproduction in other forums is permitted, provided the original author(s) and the copyright owner(s) are credited and that the original publication in this journal is cited, in accordance with accepted academic practice. No use, distribution or reproduction is permitted which does not comply with these terms.
*Correspondence: Renee M. Borges, renee@iisc.ac.in