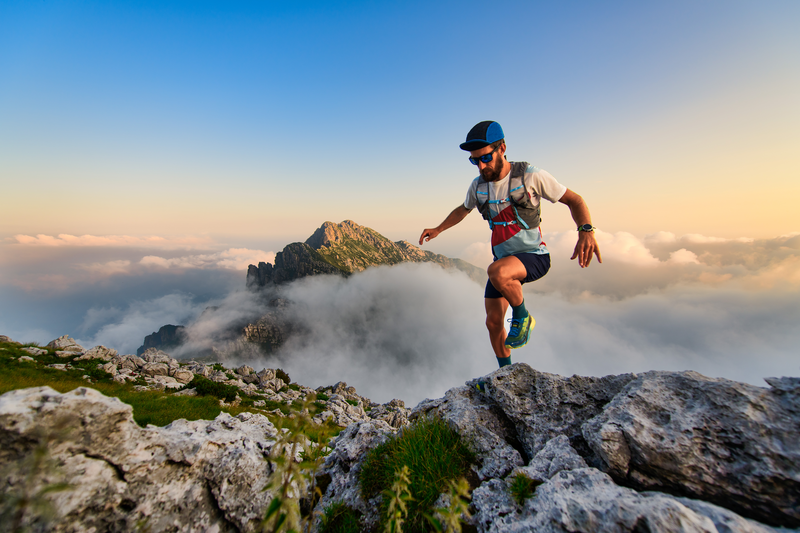
95% of researchers rate our articles as excellent or good
Learn more about the work of our research integrity team to safeguard the quality of each article we publish.
Find out more
ORIGINAL RESEARCH article
Front. Ecol. Evol. , 01 July 2021
Sec. Chemical Ecology
Volume 9 - 2021 | https://doi.org/10.3389/fevo.2021.678277
This article is part of the Research Topic The Chemical Ecology of Host and Mate Selection View all 9 articles
Illumina-based transcriptome sequencing of chemosensory organs has become a standard in deciphering the molecular bases of chemical senses in insects, especially in non-model species. A plethora of antennal transcriptomes is now available in the literature, describing large sets of chemosensory receptors and binding proteins in a diversity of species. However, little is still known on other organs such as mouthparts, legs and ovipositors, which are also known to carry chemosensory sensilla. This is the case of the noctuid Spodoptera littoralis, which has been established as a model insect species in molecular chemical ecology thanks to the description of many—but not all—chemosensory genes. To fulfill this gap, we present here an unprecedented transcriptomic survey of chemosensory tissues in this species. RNAseq from male and female proboscis, labial palps, legs and female ovipositors allowed us to annotate 115 putative chemosensory gene transcripts, including 30 novel genes in this species. Especially, we doubled the number of candidate gustatory receptor transcripts described in this species. We also evidenced ectopic expression of many chemosensory genes. Remarkably, one third of the odorant receptors were found to be expressed in the proboscis. With a total of 196 non-overlapping chemosensory genes annotated, the S. littoralis repertoire is one of the most complete in Lepidoptera. We further evaluated the expression of transcripts between males and females, pinpointing sex-specific transcripts. We identified five female-specific transcripts, including one odorant receptor, one gustatory receptor, one ionotropic receptor and one odorant-binding protein, and one male-specific gustatory receptor. Such sex-biased expression suggests that these transcripts participate in sex-specific behaviors, such as host choice for oviposition in females and/or mating partner recognition in both sexes.
Chemosensation—olfaction and taste—are at the basis of insects’ environmental perception, triggering vital behaviors such as feeding, mating, ovipositing and avoiding dangers and enemies (Stocker, 1994; Chapman, 2003; Dahanukar et al., 2005; Nishino et al., 2005). Several organs for chemical reception are found on the insect body. In moths, the head bears the antennae, the proboscis and the labial palps. The thorax and the abdomen also harbor sensory organs, such as the tarsi and the female ovipositor. Antennae have been the most studied because of their important role in olfaction, but they are also involved in other senses such as taste and mechano/hydroreception (Altner et al., 1977; Keil, 1999). Mouth parts are known for their role in taste but labial palps are also involved in sensing carbon dioxide. The tarsi and the abdomen extremities are also crucial for partner, food and oviposition site recognition and approval by insects (Fan et al., 1998; Chapman, 2003; Calas et al., 2007; Hansson and Stensmyr, 2011; Seada, 2015; Seada et al., 2018). These chemosensory organs contain plethora of different types of innervated hair structures, the sensilla, adapted to detect a wide range of molecules. Electrophysiological observations in combination with immunostaining and behavioral studies showed functional differences of the different types of chemosensory sensilla (Blaney and Simmonds, 1988, 1990; Marion-Poll et al., 1992; Marion-Poll and Van Der Pers, 1996; Laue and Steinbrecht, 1997; Calas et al., 2009; De Brito Sanchez et al., 2014; Seada, 2015; Seada et al., 2016, 2018). For instance, sensilla chaetica are devoted to the detection of soluble molecules, trichoid/basiconic sensilla to volatiles, coeloconic sensilla to acids and amines (Yao et al., 2005; Vosshall and Stocker, 2007; Hill et al., 2010).
Olfactory and gustatory cues are detected with these sensilla by chemoreceptor proteins embedded in the membrane of the neurons. After interaction with chemoreceptors, the chemical signals are transduced into electrical signals that are transmitted to the brain through the primary neuronal axons, where they are integrated, eventually resulting in a behavioral response. In insects, there are three main families of chemoreceptors: the odorant receptors (ORs), the gustatory receptors (GRs)k, and the ionotropic receptors (IRs), the latter being likely involved in both olfaction and taste (Benton et al., 2009; Fleischer et al., 2018). ORs and IRs function as complexes with co-receptors, such as Orco for ORs and IR8a/IR25a for IRs (Fleischer et al., 2018). Whether GRs also function with co-receptor(s) is not clearly established, but it is known that several GR genes are co-expressed within the same neuron, suggesting they form multimeric complexes as well (Freeman and Dahanukar, 2015; Xu and Anderson, 2015; Ning et al., 2016; Dweck and Carlson, 2020; Xu et al., 2020). Sensory neuron membrane proteins (SNMPs) are transmembrane proteins, some of which found expressed in olfactory neurons and possibly involved in pheromone detection (Benton et al., 2007; Leal, 2013; Suh et al., 2014; Lemke et al., 2019). Families of small soluble proteins are also found within chemosensory sensilla: the odorant-binding proteins (OBPs) and the chemosensory proteins (CSPs). They have been proposed to facilitate the diffusion of hydrophobic stimulants within the sensillum lymph up to the neuronal membrane (Pelosi et al., 2018).
Sequences of ORs, IRs, GRs, SNMPs, OBPs, and CSPs have been accumulated in the recent years for various insect species thanks to the progress in sequencing technologies. Most of the transcriptomic studies focused on antennae, describing a plethora of OBPs and CSPs thanks to their high expression facilitating their detection, and of ORs because of their role in long distance chemical communication. Larger repertoires of chemosensory genes have come from genome analyses, including vast arrays of GRs and IRs. For instance, recent genomic data from Lepidoptera revealed that Noctuidae genomes contain an incomparable high number of GR genes compared to ORs, with up to 250 GRs (and only 70–80 ORs) in polyphagous species such as Helicoverpa armigera, Spodoptera frugiperda, and S. litura (Cheng et al., 2017; Gouin et al., 2017; Pearce et al., 2017). However, little is known on their expression pattern, because transcriptomic data from taste tissues is scarce (Xu et al., 2016; Cheng et al., 2017; Guo et al., 2018).
Among Noctuidae, the cotton leafworm S. littoralis has been established for several years as a model in molecular chemical ecology. The sex pheromone and many plant volatiles detected by the antennae and triggering specific behaviors have been identified. Transcriptomic data have been accumulated on chemosensory organs such as male and female antennae (Legeai et al., 2011; Jacquin-Joly et al., 2012; Poivet et al., 2013), larval antennae and palps (Poivet et al., 2013), and adult non-chemosensory tissues such as brain, body and proboscis (Walker et al., 2019), leading to the description of a substantial number of expressed chemosensory genes in a Lepidoptera. A total of 60 OR transcripts have been described, and the recent functional characterization of one third of them has been a landmark in Lepidoptera olfaction (de Fouchier et al., 2017). However, only 17 GR transcripts could be reconstituted in this species (Poivet et al., 2013; Walker et al., 2019), probably due to the lack of taste organs in the tissues investigated.
The aim of the present study was to fulfill this gap, by performing in-depth RNA sequencing of different tissues known to carry taste sensilla but never investigated before. We generated new RNAseq data from male and female adult labial palps and forelegs as well as female ovipositors, and completed previous data on proboscis. We identified new candidate chemosensory genes in S. littoralis, and expanded coding sequences of previously described transcripts. Altogether, these transcriptomic data offer incomparable resources to explore the molecular mechanisms of olfaction and taste in a single model and revealed ectopic expression of most chemosensory gene families.
All S. littoralis individuals were from an inbred colony reared in the laboratory at 24°C, 70% relative humidity and under a 16 h:8 h light:dark photoperiod. Larvae were fed on a semi-artificial diet (Poitout and Bues, 1974) and adults were provided with sugar water. Males and females were sexed as pupae and kept in separate rooms, since it has been shown that smelling the sex pheromone can impact chemosensory gene expression in this species (Guerrieri et al., 2012).
Thirty proboscis and 15 pairs of labial palps and forelegs were dissected from both males and females. For females, 30 ovipositors were also dissected. Adults were 2–3 days old upon dissection and all dissections were conducted between the second and third hours of the scotophase. Proboscis RNAs (one sample from males, one sample from females) were extracted separately from the rest of the tissues, which were pooled together, and formed two samples (one for males and one for females). These tissue mix samples (labial palps, forelegs plus ovipositors for the females) are further designed as “palp/leg” tissue mix to facilitate description. RNAs were extracted using TRIzolTM Reagent (Thermo Fisher Scientific, Waltham, MA, United States) and quality was checked using a NanoDropTM ND-2000 spectrophotometer (Thermo Fisher Scientific). About 5 μg of total RNA per sample were used for cDNA paired-end library construction using the Truseq RNA Stranded Sample Preparation Illumina Kit at the Institut de Génomique Fonctionnelle, Plateforme Montpellier GenomiX IBiSA (MGX). Illumina HiSeq2000 sequencing (2 × 100 bp) was performed at MGX.
MGX provided raw reads cleaned by removing 3′ adaptors and poly-A/T tails. Data were further processed using Galaxy Version 2.8.3. FastQC was run on each sample and low-quality reads (<30) and sequences shorter than 20 bp were trimmed using Trimmomatic (Bolger et al., 2014). Ribosomal RNAs were removed using Ribopicker (Schmieder et al., 2011). A reference transcriptome was assembled with all the data generated in this study using Trinity (Grabherr et al., 2011) with default parameters. After reconstruction, the transcriptome was filtered using two criteria: (1) transcripts with no read support (expected count < 1) were identified using Bowtie (v1.0.0) (Langmead et al., 2009) and discarded, (2) CD-HIT-EST was used to remove transcript redundancy with a similarity threshold of 0.90 and a word size of 8. Open reading frames (ORFs) encoding proteins of more than 50 amino acids were predicted by TransDecoder (Haas et al., 2013). We used the Benchmarking Universal Single-Copy Orthologs tool (BUSCO v3.0.2) (Simão et al., 2015) against the Insecta odb10 dataset (1,367 reference genes) to assess the completeness of the assembled transcriptome.
To identify putative chemosensory genes (ORs, GRs, IRs, OBPs, CSPs, and SNMPs), we used amino acid sequences of proteins previously annotated in S. littoralis and the related species S. frugiperda and S. litura (Legeai et al., 2011; Jacquin-Joly et al., 2012; Poivet et al., 2013; Cheng et al., 2017; Gouin et al., 2017; Walker et al., 2019) as queries in a blastp search on the translated ORFs predicted from the reference transcriptome. Annotations of all retrieved sequences were confirmed by blastp on the NCBI non-redundant database (NR). Sequences described for the first time in S. littoralis were named according to their ortholog in S. frugiperda (Gouin et al., 2017), except IRs that were named following orthology with S. litura (Zhu et al., 2018). For sequences previously described we followed the nomenclature of Walker et al. (2019), except for SlitGR70 that was renamed SlitGR272.
Phylogenetic analyses were performed using the newly identified protein sequences (ORs, GRs, IRs and OBPs) together with previously annotated amino acid sequences in Spodoptera spp. (S. littoralis, S. frugiperda, S. litura), sequences from other Lepidoptera (Bombyx mori, Helicoverpa armigera, Heliconius melpomene) and from species from other insect orders when relevant (Drosophila melanogaster, Tribolium castaneum, and Apis melifera). MAFFT v7 (Katoh and Standley, 2013) was employed for amino acid sequence alignment and maximum-likelihood phylogenies were constructed using PhyML 3.0 (Guindon et al., 2010) with default parameters. The best-fit model of amino acid substitution was determined by SMS (Lefort et al., 2017). Node support was estimated via SH-like aLRT (Anisimova and Gascuel, 2006). Trees were visualized with FigTree v1.4.3 and images were edited with Adobe Illustrator software.
The de novo transcriptome presented in this paper was generated from four libraries: proboscis from each sex, labial palps and forelegs from males, and labial palps, forelegs and ovipositors from females. A total of 418 million raw reads were obtained and deposited at the NCBI Sequence Read Archive (BioProject ID PRJNA693435, Biosamples SAMN17386877, SAMN17386878, SAMN17386879, SAMN17386880). After cleaning, a total of 325 million reads were pooled and assembled to generate a de novo transcriptome of 234,643 transcripts. Removing transcripts with no read support led to an assembly of 203,642 transcripts. Last, CD-HIT-EST was applied to remove transcript redundancy, leading to a final assembly of 154,302 transcripts. Out of the transcripts, 47,853 ORFs could be identified. All metrics are provided in Supplementary Material 1. BUSCO analysis revealed that the de novo transcriptome contained 89.9% of complete sequences, with 78.6% as single copy and 11.3% as duplicated, thus reflecting a good representative transcriptome (Supplementary Material 1).
We sequenced previously unexplored tissues (adult labial palps, legs and ovipositors) from S. littoralis. These tissues have been selected as surface contact tissues and candidate tissues for close range chemoreception (taste), without neglecting their potential long range (olfaction) chemosensory capacities. These tissues all carry chemosensory sensilla and are expected to play a role in assessing the quality and toxicity of food and oviposition substrates, as well as in close range mate detection. We thus expected to extend our current knowledge on S. littoralis chemosensory transcripts. This proved to be rewarding since we identified a total of 115 candidate transcripts from the three chemosensory receptor families (GRs, ORs and IRs), the pheromone detection associated SNMPs and the two lipid transporter families (OBPs and CSPs), including 30 new chemosensory transcripts compared to the latest published repertoire (Walker et al., 2019; Table 1). We also identified full-length sequences for 13 previously published sequences (Supplementary Material 2). In addition, we got insight into the presence of S. littoralis chemosensory transcripts according to tissue and sex, as summarized in Figure 1.
Figure 1. Venn diagrams showing the expression of chemosensory transcripts in the different tissues and sexes.
Details per family are described below.
We identified 34 candidate GRs in our S. littoralis gustatory transcriptome and we obtained complete ORFs for 14 of them (Supplementary Material 2). We could retrieve 13 out of the 17 GRs already known from previous work on this species (Jacquin-Joly et al., 2012; Poivet et al., 2013; Walker et al., 2019) and identified 21 new GRs (Table 1 and Supplementary Material 2). A total of 38 GRs are now described for this species (SlitGR158 and SlitGR206, previously described in Walker et al. (2019), as expressed in the proboscis, were not found here).
The number of 38 GR genes found expressed in the different S. littoralis transcriptomes is far behind the numbers of GR genes found in the genomes of Noctuidae (Cheng et al., 2017; Gouin et al., 2017; Pearce et al., 2017). For instance, 231 GRs have been annotated in the genome of S. frugiperda and it has been hypothesized that this extended number of GRs could be linked to the acquisition of polyphagy. With only 38 GR transcripts described by now in S. littoralis, we can speculate that the vast majority of GRs have probably not been identified yet in this species. GRs are known to be expressed at very low levels and their identification may need very deep sequencing. Alternatively, some may be expressed in tissues not investigated here, for example the gut, or at different periods of the life cycle, for example in larvae as already demonstrated for S. litura (Cheng et al., 2017). Further genomic data will help to establish a complete set of GRs in S. littoralis. Anyhow, SlitGRs were found in each of the major clades in the GR phylogeny (Figure 2). We extended the candidate sugar receptor subfamily to six GRs (one more than previous studies), all having orthologs in S. frugiperda, and we identified one of the two candidate fructose receptor orthologs found in the genome of S. frugiperda (Gouin et al., 2017). We found the three candidate CO2 receptors SlitGR1, 2 and 3 (Erdelyan et al., 2012). All other candidate SlitGRs clustered in the so-called “bitter” GR subfamily.
Figure 2. Phylogenetic position of Spodoptera littoralis gustatory receptors (SlitGRX, black). The phylogeny was built using GR repertoires of the moths S. frugiperda (red) and Bombyx mori (blue), and the butterfly Heliconius melpomene (green). SlitGRs found in the present transcriptome are written in bold. Candidate CO2, sugar and fructose receptor clades are pictured with color shades. The CO2 receptor clade was used as an outgroup. Clades supported by an aLRT value over 0.9 are indicated by a black dot.
SlitGR6, 12, 13, and 14 (candidate sugar GRs) were expressed both in the proboscis and the palps/legs. This suggests their importance for sugar detection during food intake but also palps/legs implication in sugar detection in addition to proboscis and antennae (Walker et al., 2019).
Three candidate CO2 receptors SlitGR1, 2 and 3 were expressed both in the proboscis and in the palp/leg samples (Supplementary Material 2). This is in accordance with the observation that the labial palps—and more specifically the labial pit organ—are involved in CO2 detection in Lepidoptera (Bogner et al., 1986; Kent et al., 1986). Previous studies have revealed that SlitGR2 and 3 are also highly expressed in antennae (Jacquin-Joly et al., 2012; Walker et al., 2019) and that SlitGR1 is enriched in female ovipositor (Legeai et al., 2011). This may suggest that most chemosensory organs participate in CO2 sensing in S. littoralis, although functional studies on orthologous CO2-sensing GRs in H. armigera have shown that co-expression of GR1 and 3 is necessary and sufficient for CO2 detection (Xu and Anderson, 2015; Ning et al., 2016).
More than half (57%) of the S. littoralis candidate bitter GRs were specifically expressed in the proboscis (Supplementary Material 2) and have not been described in previous studies (Jacquin-Joly et al., 2012; Poivet et al., 2013; Walker et al., 2019). The remaining candidate bitter GRs were expressed in both proboscis and palps/legs at the exception of SlitGR16, only found in male palps/legs. Two other candidate bitter GRs were sex-biased: SlitGR79 and 149 were exclusively found in the female proboscis, which may be related with the need for females to eat for their sexual maturation or to find adequate surface for oviposition and progeny nutrition. Indeed, it is known that S. littoralis premating behavior consists in female foraging for flower nectar while postmating females are more attracted to larval host odors (Ahmed et al., 2012). Almost no functional data are available for moth candidate bitter GRs, but impressive expansions of these GRs have been described in Noctuidae (Cheng et al., 2017; Gouin et al., 2017; Pearce et al., 2017).
Although legs are known to harbor taste sensilla and play an important role in gustation, SlitGRs were surprisingly poorly represented in the palp/leg tissue mix (Supplementary Material 2). We did not evidence any female-specific GRs in this tissue mix, although the female palp/leg mix also included ovipositors. This is intriguing since female moths are known to use their tarsi and ovipositor to taste oviposition sites before egg laying. Sensilla chaetica have been found at the external distal border of the ovipositor papillae in S. littoralis (Seada et al., 2016). They are uniporous (a hallmark of taste sensilla) and contain four gustatory sensory neurons responding mainly to salt, sugars, caffeine and water (Seada et al., 2016). Although several molecules have been shown to promote or deter oviposition in moths and several chemosensory genes seem to be specifically expressed in the ovipositor, no chemoreceptor/molecule association has been established (Qiu et al., 1998; van der Goes van Naters and Carlson, 2006; Xu et al., 2017; Chen et al., 2019).
Sixty S. littoralis ORs (SlitORs) have been previously described in the literature (Legeai et al., 2011; Jacquin-Joly et al., 2012; Poivet et al., 2013; Walker et al., 2019). We identified 22 of these in our transcriptome, including the co-receptor SlitOrco. In addition, we annotated four ORs that have never been described in S. littoralis before (Table 1). We named these new ORs as SlitOR61, SlitOR65, SlitOR68—according to their orthologs in the S. frugiperda genome (Gouin et al., 2017)—and SlitOR70 (no identified ortholog in S. frugiperda). We obtained complete ORFs for 7 SlitORs (Supplementary Material 2). As a result, the S. littoralis OR family reached a total of 64 expressed ORs in this species. This number is close to the number of OR genes annotated in the genomes of other noctuid species (69 in S. frugiperda, 73 in S. litura, 84 in H. armigera and 82 in H. zea) (Cheng et al., 2017; Gouin et al., 2017; Pearce et al., 2017), suggesting we have identified most—if not all—of the ORs expressed in S. littoralis. The phylogenetic analysis (Figure 3) showed that ORs expressed in the mouth parts, legs and ovipositor are found in the vast majority of lepidopteran OR clades, thus mirroring the wide diversity of ORs expressed in taste tissues. Interestingly, there was no representative in the classical pheromone receptor clade (Figure 3).
Figure 3. Phylogenetic position of S. littoralis odorant receptors (SlitORX, black). The phylogeny was built using OR repertoires of the moths S. frugiperda (red), H. armigera (yellow) and B. mori (blue), and the butterfly H. melpomene (green). SlitGRs found in the present transcriptome are written in bold. Clades of candidate receptors for pheromones and aromatics are shown with purple and green color shades, respectively. The odorant receptor coreceptor (Orco) clade is shaded in gray and was used as an outgroup. Clades supported by an aLRT value over 0.9 are indicated by a black dot.
Out of the 64 SlitORs, we found that one third (21 ORs) were expressed in the proboscis (Table 1 and Figure 1). We found a set of 22 ORs expressed in the palp/leg samples of both males and females (Table 1 and Figure 1), some of which being also expressed in the proboscis. Seven ORs described as antennal specific in Walker et al. (2019) and 19 others described as enriched in the antennae (with only one or two reads in the brain and/or body) were not retrieved in our transcriptome, supporting their antennal specific expression or enrichment.
This tissue distribution of ORs in a moth is surprising (half of the SlitORs expressed in the proboscis, one third in the palps/legs) and suggests that these organs participate in odorant sensing. Out of the 83 known ORs in adult H. armigera (Pearce et al., 2017), only 4 and 3 ORs, including Orco, have been found expressed in proboscis and palps, respectively (Guo et al., 2018). One type of multiporous sensilla (sensilla styloconica) was found in the proboscis of H. armigera, suggesting a role in olfaction (Guo et al., 2018). Few studies on S. littoralis have investigated the sensilla on mouthparts, tarsi and ovipositor (Chadha and Roome, 1980; El-Degwi and Gabarty, 2015; Seada et al., 2018, 2016) but none has described their numbers or their morphology in a resolution that would permit to distinguish uniporous from multiporous sensilla. Overall, the number of ORs described here likely exceeds the number of olfactory sensilla carried by these organs. This suggests that they might be transcribed but that not all of them are functional in these tissues.
SlitOrco was found expressed in the proboscis and in the palp/leg tissue mix of both sexes. This was expected in view of its role as a co-receptor for ORs and it predicts that at least some of the ORs found in these gustatory tissues should form functional olfactory ligand gated ion channels.
SlitOR45, 61, and 68 were found to be expressed in the proboscis samples but not in the palps/legs (Supplementary Material 2). On the contrary, SlitOR20, 28, 58, 60, and 70 were found exclusively expressed in the palp/leg tissue mix.
We found one OR, SlitOR37, expressed in the female proboscis but not in male ones, with no expression in palp/leg mixes (Supplementary Material 2). This transcript was not previously found expressed in the proboscis (Walker et al., 2019) but was found in both male and female antennae with a female bias. Interestingly, SlitOR37 clustered in the phylogeny with BmorOR19 and MsexOR5, which were found to be female-specific (Anderson et al., 2009; Grosse-Wilde et al., 2010; Koenig et al., 2015). BmorOR19 has been functionally characterized as responding to linalool (Anderson et al., 2009), an oviposition cue for many female moths (Rostelien et al., 2005) and a male pheromone component in some Noctuidae, e.g., Mamestra brassicae (Heath et al., 1992). The SlitOR37 ortholog in Helicoverpa assulta, HassOR62, also exhibited the same female-biased expression pattern (Zhang et al., 2015). Although SlitOR37 has not been functionally characterized yet in S. littoralis, its expression pattern suggests it may play a role in the detection of suitable oviposition sites or in male mate recognition. SlitOR38 was clearly male-biased in palps/legs, as it is in antennae (Walker et al., 2019), suggesting that this receptor presents a general male-enriched expression. Additionally, SlitOR38 belongs to a recently described candidate pheromone receptor clade (Bastin-Héline et al., 2019), which highlights an eventual role in female-pheromone recognition.
According to the OR phylogeny, none of the SlitORs retrieved from our transcriptome belonged to the classical pheromone receptor group (Figure 3). However, we detected SlitOR5 expressed in all tissues examined from both sexes (Supplementary Material 2). This receptor does not belong to the classical pheromone receptor clade but has been recently described as highly expressed in male antennae (Walker et al., 2019) and functionally characterized as an unconventional pheromone receptor tuned to the major component of the S. littoralis female sex pheromone (Bastin-Héline et al., 2019). SlitOR5 expression in antennal and non-antennal tissue suggests that other tissues than the antennae may be involved in sex pheromone detection. However, its low expression levels in the non-antennal tissues calls for further investigation of its precise expression pattern before any assumptions and interpretations could be given on its function in these tissues. Interestingly, we found SlitSNMP1, encoding a membrane protein probably involved in pheromone delivery to pheromone receptors (Vogt et al., 2009), in the present transcriptome (Table 1 and Supplementary Material 2). Detailed observations of S. littoralis premating behavior are also needed to investigate if mouthpart and/or leg contacts occur during courtship and if courtship and mating are disturbed when these appendices are blocked. Alternatively, sex pheromone cues may be used in non-sexual behaviors. For instance, females may detect these cues via mouthparts, legs or ovipositor in order to regulate their pheromone production (as proposed in the moth Heliothis virescens, Widmayer et al., 2009) or to detect traces of sex pheromone from other females in oviposition sites to avoid over-oviposition on the same plant and high competition between larvae for the same food resources.
Thirteen IR transcripts were identified in our transcriptome (Figure 1 and Table 1)and complete ORFs were obtained for five of them (Supplementary Material 2). Among these 13 IRs, we found the three candidate IR coreceptors (SlitIR8a, 25a, and 76b), 4 members of the so-called antennal IR clades (SlitIR41a, 64a, 75d, and 75p.2) supposedly involved in olfaction, and 6 divergent IRs (SlitIR7d.1, 7d.4, 60a, 85a, 100b, and 100i) more likely to be involved in taste (Figure 4). Five of these divergent IRs are described for the first time as they were not found in previous S. littoralis transcriptomes. The total number of IRs found expressed in S. littoralis is now 22. This is approximately half of the number of IR genes described in the genomes of S. frugiperda (43 IRs, Gouin et al., 2017) and H. armigera (39 IRs, Liu et al., 2018). Out of these 22 SlitIRs, 17 have been found to be expressed in the antennae (Walker et al., 2019), 14 in the proboscis (Walker et al., 2019 and our study) and 12 were described, for the first time in this paper, in the palps/legs of S. littoralis. Of the 5 new S. littoralis divergent IRs discovered here (thus not previously found expressed in antennae), 4 were expressed in both the proboscis and the palp/leg tissue mix and one (SlitIR7d.4) was exclusively expressed in the proboscis of both males and females. This expression pattern, together with their phylogenetic position within divergent IRs, makes these SlitIRs good candidate taste receptors.
Figure 4. Phylogenetic position of S. littoralis ionotropic receptors (SlitIRX, black). The phylogeny was built using IR repertoires of the moths S. frugiperda (red), H. armigera (yellow) and the butterfly H. melpomene (green), and three non-Lepidoptera species (blue): Drosophila melanogaster, Apis melifera, and Tribolium castaneum. SlitIRs found in the present transcriptome are written in bold. Three coreceptor clades are pictured with blue color shades and the coreceptor IR93a for hygro- and thermosensation is pink shaded. The ionotropic glutamate receptor clade is shaded in gray and was used as an outgroup. Candidate ionotropic receptors for hygrosensation and thermosensation according to their homology with Drosophila IRs are designated with the accolade of a self-explaining pictogram. Clades supported by an aLRT value over 0.9 are indicated by a black dot.
As already observed in H. armigera (Liu et al., 2018), we showed that expression of the so-called antennal IRs was not restricted to antennae (Supplementary Material 2), as four antennal IRs were expressed either in the proboscis and the palp/leg tissue mix (SlitIR41a, 64a, 75d) or specifically in the female palp/leg/ovipositor tissue mix (SlitIR75p.2). As this latter IR was not expressed in the male palp/leg mix, one could hypothesize that it is expressed in the ovipositor, playing a potential role in host quality evaluation for oviposition. Deeper studies of its expression pattern would be needed to precisely identify in which tissues of the palp/leg/ovipositor mix this IR is expressed.
Looking at the three candidate IR coreceptor transcripts, SlitIR25a appeared expressed in the proboscis and in the palp/leg tissue mix from both sexes, SlitIR76b was also expressed in all tissues examined here, and SlitIR8a was found only in the palp/leg tissue mix (Supplementary Material 2). All have been previously found highly expressed in the antennae (Olivier et al., 2011; Walker et al., 2019). The expression pattern of these coreceptors is in line with previous observation in insects. They are usually described as highly expressed in all insect taxa in which they have been described. Most are expressed in more than one tissue and the different expression patterns between different taxa suggests that insect sensing is differently distributed between the chemosensory tissues among taxa (Leal et al., 2013; Sparks et al., 2014; van Schooten et al., 2016; Walker et al., 2019). Following knowledge on Drosophila IR coreceptors, our results are not surprising since DmelIR8a has been associated to olfactory neurons in the antennae and palps and DmelIR76b has been associated to gustatory neurons which are more prominent in the Drosophila antennae, labellum and legs (Benton et al., 2009; Croset et al., 2010; Abuin et al., 2011; Zhang et al., 2013; Koh et al., 2014; Hussain et al., 2016; Rimal and Lee, 2018). IR25a is known to be a more generally and highly expressed coreceptor, which could be considered as the equivalent of Orco (Benton et al., 2009; Croset et al., 2010; Abuin et al., 2011; Rimal and Lee, 2018).
Interestingly, the IR phylogeny showed that we found no SlitIR in the clades housing IRs involved in hygrosensation and thermosensation (Figure 4). The cool temperature sensing candidate SlitIR21a and the humidity sensing candidates SlitIR40a and SlitIR68a (Knecht et al., 2017, 2016) have been found previously in S. littoralis antennae (Olivier et al., 2011; Poivet et al., 2013; Walker et al., 2019), yet the candidate coreceptor involved in hygrosensation and thermosensation, IR93a (Benton et al., 2009; Corey et al., 2013; Rytz et al., 2013; Groh-Lunow et al., 2015) was not retrieved in our transcriptome nor in previous S. littoralis antennal transcriptomes (Olivier et al., 2011; Poivet et al., 2013; Walker et al., 2019). All together, these observations suggest that proboscis, palps and legs are not involved in hygro and thermosensation in S. littoralis.
CSPs are known to be largely expressed in a variety of chemosensory and non-chemosensory tissues in insects, including Noctuidae (Zhang et al., 2015; Guo et al., 2018; Walker et al., 2019). Our results comfort these observations, since among the 22 SlitCSPs previously identified in S. littoralis antennal transcriptomes (Poivet et al., 2013; Walker et al., 2019), 10 were found and they were all expressed in both the proboscis and palps/legs (Supplementary Material 2). The current hypothesis is that CSPs would play a general role in hydrophobic molecule transport (Pelosi et al., 2018). It is thus difficult for now to propose any specific function of CSPs in olfaction and/or taste in S. littoralis.
Thirty one OBP transcripts were found in the present transcriptome, all previously described in adult antennae and larval tissues (Legeai et al., 2011; Jacquin-Joly et al., 2012; Poivet et al., 2013; Walker et al., 2019). Twenty-seven had a full-length ORF (Supplementary Material 2). Among the 31 SlitOBPs, we retrieved the two OBPs classified as general OBPs (GOBPs) and three of the four candidate pheromone-binding proteins (PBPs), as shown in Figure 5. At the exception of SlitOBP8 and SlitOBP33, we also found all OBPs belonging to the Noctuidae-specific OBP expansion.
Figure 5. Phylogenetic position of S. littoralis odorant-binding proteins (SlitOBPX, black). The midpoint rooted phylogeny was built using OBP repertoires of the moths S. frugiperda (red), H. armigera (yellow) and B. mori (blue), and the butterfly H. melpomene (green). SlitOBPs found in the present transcriptome are written in bold. The General OBP (GOBP) and Pheromone-Binding Protein (PBP) clade is designated with a light blue color shade. Plus and minus-C clades are highlighted with green and yellow shades, respectively. The noctuidae-specific OBP expansion is indicated by a red line. Clades supported by an aLRT value over 0.9 are indicated by a black dot.
First studies on OBPs have suggested that, contrary to CSPs, they would be antennae-specific and only involved in olfaction, with some (the PBPs) being sex-specific and involved in pheromone transportation in the sensillum lymph. Here, we found 31 SlitOBPs expressed in proboscis and palps/legs (Supplementary Material 2) SlitOBP6 was expressed only in the female palp/leg/ovipositor tissue mix. This OBP has been previously detected in female adult antennae and caterpillar antennae (Poivet et al., 2013), suggesting that SlitOBP6 may be female-specific but not tissue-specific. Whether this OBP is involved in female specific behaviors, such as oviposition site selection, remains to be investigated.
Four OBPs expressed in the proboscis (i.e., SlitOBP11, 25, 32, and 34) belong to the Noctuidae-specific expansion mentioned above. Interestingly, some of these have also been found to be more expressed in the proboscis than in antennae (Walker et al., 2019). Expression of these OBPs in gustatory tissues has been observed also in H. assulta (five out of seven OBPs of this clade are also expressed in legs; Zhang et al., 2015) and in H. armigera (all OBPs of this clade are also expressed in caterpillar mouthparts; Chang et al., 2017). Whether these OBPs from the Noctuidae OBP expansion play a role in olfaction has not yet been determined.
Interestingly enough and rather surprisingly, we found three SlitPBPs expressed in all tissues tested in this study. This expression pattern was unexpected for PBPs that are believed to bind volatile hydrophobic pheromone molecules and to be expressed in antennae. In addition to the expression of SlitOR5 and SlitSNMP1 in all samples (see upper), expression of PBPs revealed that all the machinery for sex pheromone detection is present in proboscis and palp/leg tissues. This reinforces our hypothesis that S. littoralis may use sensory appendages such as proboscis, palps and/or legs to evaluate mating partners via the detection of long range sex pheromone and/or close range pheromonal cues yet unidentified.
S. littoralis is a model species in chemical ecology. It is crucial to combine long term knowledge and accumulate data on this model for further advances in deciphering the mechanisms of chemosensation in moths. Our study adds a large amount of data on chemosensory genes and their expression specificities in this species, revealing unexpected expression of many. We pinpointed interesting genes for further functional studies that will help understanding the intimate link of the different sensory appendages to olfaction and taste. Also, we contribute additional evidence that the sex pheromone communication may be more complex than previously thought in this species, with involvement of a probable close range communication. S. littoralis is also an economically important agricultural pest and a representative of the Spodoptera genus that contains some of the most dangerous invasive pest species, such as the fall armyworm S. frugiperda. Chemosensation drives many detrimental behaviors in pests, such as food, oviposition site and mate choice. A better understanding of the chemoreception pathways will lead to identifying key molecular targets to disturb such chemical communication in a context of sustainable pest management.
The data generated in this study have been deposited in NCBI Sequence Read Archive, (BioProject ID PRJNA693435, Biosamples SAMN17386877, SAMN17386878, SAMN17386879, and SAMN17386880).
EJ-J conceived the research. FAK performed the laboratory work and the transcriptomic data analysis. FAK, CMo, and M-CF annotated the transcripts. CMe helped with the transcriptomic data analysis and NM with the phylogenetic analysis. FAK, NM, CMe, and EJ-J contributed to the writing and reviewing of the manuscript. DS was responsible for transcriptomic sequencing. All authors contributed to the article and approved the submitted version.
The authors declare that the research was conducted in the absence of any commercial or financial relationships that could be construed as a potential conflict of interest.
We thank the BioInformatics Platform for Agroecosystems Anthropods (BIPAA), Rennes, France, for assistance on Galaxy transcriptomic data analysis. We also thank Pascal Roskam (iEES) for insect rearing. We acknowledge the French National Research Agency (ANR-16-CE02-0003-01 and ANR-16-CE21-0002-01 grants) and the SPE department at INRAE for funding.
The Supplementary Material for this article can be found online at: https://www.frontiersin.org/articles/10.3389/fevo.2021.678277/full#supplementary-material
Abuin, L., Bargeton, B., Ulbrich, M. H., Isacoff, E. Y., Kellenberger, S., and Benton, R. (2011). Functional architecture of olfactory ionotropic glutamate receptors. Neuron 69, 44–60. doi: 10.1016/j.neuron.2010.11.042
Ahmed, S., Kromann, S., Birgersson, G., Bengtsson, M., Lindblom, T., Balkenius, A., et al. (2012). Floral to green: Mating switches olfactory coding and preference. Proc. R. Soc. Lond. B Biol. Sci. 279, 2314–2322. doi: 10.1098/rspb.2011.2710
Altner, H., Sass, H., and Altner, I. (1977). Relationship between structure and function of antennal chemo-, hygro-, and thermoreceptive sensilla in Periplaneta americana. Cell Tissue Res. 176, 389–405.
Anderson, A. R., Wanner, K. W., Trowell, S. C., Warr, C. G., Jaquin-Joly, E., Zagatti, P., et al. (2009). Molecular basis of female-specific odorant responses in Bombyx mori. Insect Biochem. Mol. Biol. 39, 189–197. doi: 10.1016/j.ibmb.2008.11.002
Anisimova, M., and Gascuel, O. (2006). Approximate Likelihood-Ratio Test for branches: A fast, accurate, and powerful alternative. Syst. Biol. 55, 539–552. doi: 10.1080/10635150600755453
Bastin-Héline, L., de Fouchier, A., Cao, S., Koutroumpa, F., Caballero-Vidal, G., Robakiewicz, S., et al. (2019). A novel lineage of candidate pheromone receptors for sex communication in moths. Elife 8:e49826.
Benton, R., Vannice, K. S., Gomez-Diaz, C., and Vosshall, L. B. (2009). Variant ionotropic glutamate receptors as chemosensory receptors in Drosophila. Cell 136, 149–162. doi: 10.1016/j.cell.2008.12.001
Benton, R., Vannice, K. S., and Vosshall, L. B. (2007). An essential role for a CD36-related receptor in pheromone detection in Drosophila. Nature 450, 289–93. doi: 10.1038/nature06328
Blaney, W. M., and Simmonds, M. S. J. (1988). Food selection in adults and larvae of three species of Lepidoptera: a behavioural and electro physiological study. Entomol. Exp. Appl. 49, 111–121. doi: 10.1111/j.1570-7458.1988.tb02482.x
Blaney, W. M., and Simmonds, M. S. J. (1990). A behavioural and electrophysiological study of the role of tarsal chemoreceptors in feeding by adults of Spodoptera, Heliothis virescens and Helicoverpa armigera. J. Insect Physiol. 36, 743–756. doi: 10.1016/0022-1910(90)90048-k
Bogner, F., Boppré, M., Ernst, K.-D., and Boeckh, J. (1986). CO2 sensitive receptors on labial palps of Rhodogastria moths (Lepidoptera: arctiidae): physiology, fine structure and central projection. J. Comp. Physiol. A 158, 741–749. doi: 10.1007/bf01324818
Bolger, A. M., Lohse, M., and Usadel, B. (2014). Trimmomatic: a flexible trimmer for Illumina sequence data. Bioinformatics 30, 2114–2120. doi: 10.1093/bioinformatics/btu170
Calas, D., Berthier, A., and Marion-Poll, F. (2007). Do European corn borer females detect and avoid laying eggs in the presence of 20-hydroxyecdysone? J. Chem. Ecol. 33, 1393–1404. doi: 10.1007/s10886-007-9302-5
Calas, D., Marion-Poll, F., and Steinbauer, M. J. (2009). Tarsal taste sensilla of the autumn gum moth, Mnesampela privata: morphology and electrophysiological activity. Entomol. Exp. Appl. 133, 186–192. doi: 10.1111/j.1570-7458.2009.00921.x
Chadha, G. K., and Roome, R. E. (1980). Oviposition behaviour and the sensilla of the ovipositor of Chilo partellus and Spodoptera littoralis (Lepidoptera: noctuidae). J. Zool. 192, 169–178. doi: 10.1111/j.1469-7998.1980.tb04228.x
Chang, H., Ai, D., Zhang, J., Dong, S., Liu, Y., and Wang, G. (2017). Candidate odorant binding proteins and chemosensory proteins in the larval chemosensory tissues of two closely related noctuidae moths, Helicoverpa armigera and H. assulta. PLoS One 12:e0179243. doi: 10.1371/journal.pone.0179243
Chapman, R. F. (2003). Contact chemoreception in feeding by phytophagous insect. Annu. Rev. Entomol. 48, 455–484.
Chen, W.-W., Kang, K., Yang, P., and Zhang, W.-Q. (2019). Identification of a sugar gustatory receptor and its effect on fecundity of the brown planthopper Nilaparvata lugens. Insect Sci. 26, 441–452. doi: 10.1111/1744-7917.12562
Cheng, T., Wu, J., Wu, Y., Chilukuri, R. V., Huang, L., Yamamoto, K., et al. (2017). Genomic adaptation to polyphagy and insecticides in a major East Asian noctuid pest. Nat. Ecol. Evol. 1, 1747–1756. doi: 10.1038/s41559-017-0314-4
Corey, E. A., Bobkov, Y., Ukhanov, K., and Ache, B. W. (2013). Ionotropic crustacean olfactory receptors. PLoS One 8:e60551. doi: 10.1371/journal.pone.0060551
Croset, V., Cummins, S. F., and Benton, R. (2010). Ancient protostome origin of chemosensory ionotropic glutamate receptors and the evolution of insect taste and olfaction. J. Neurogenet. 24, 30–31.
Dahanukar, A., Hallem, E. A., and Carlson, J. R. (2005). Insect chemoreception. Curr. Opin. Neurobiol. 15, 423–430.
De Brito Sanchez, M. G., Lorenzo, E., Songkung, S., Liu, F., and Giurfa, M. (2014). The tarsal taste of honey bees: behavioral and electrophysiological analyses. Front. Behav. Neurosci. 8:25. doi: 10.3389/fnbeh.2014.00025
de Fouchier, A., Walker, W. B. III., Montagné, N., Steiner, C., Binyameen, M., Schlyter, F., et al. (2017). Functional evolution of Lepidoptera olfactory receptors revealed by deorphanization of a moth repertoire. Nat. Commun. 8:15709.
Dweck, H. K. M., and Carlson, J. R. (2020). Molecular logic and evolution of bitter taste in Drosophila. Curr. Biol. 30, 17–30.e3.
El-Degwi, M. S., and Gabarty, A. (2015). Morphological changes induced by thermal treatment and gamma irradiation on the males’ hind legs of Spodoptera littoralis (Noctuidae; Lepidoptera). J. Radiat. Res. Appl. Sci. 8, 508–515. doi: 10.1016/j.jrras.2015.06.002
Erdelyan, C. N. G., Mahood, T. H., Bader, T. S. Y., and Whyard, S. (2012). Functional validation of the carbon dioxide receptor genes in Aedes aegypti mosquitoes using RNA interference. Insect Mol. Biol. 21, 119–127. doi: 10.1111/j.1365-2583.2011.01120.x
Fan, R. J., Anderson, P., and Hansson, B. (1998). Behavioural analysis of olfactory conditioning in the moth Spodoptera littoralis (Boisd.)(Lepidoptera: Noctuidae). J. Exp. Biol. 200, 2969–2976. doi: 10.1242/jeb.200.23.2969
Fleischer, J., Pregitzer, P., Breer, H., and Krieger, J. (2018). Access to the odor world: olfactory receptors and their role for signal transduction in insects. Cell. Mol. Life Sci. 75, 485–508. doi: 10.1007/s00018-017-2627-5
Freeman, E. G., and Dahanukar, A. (2015). Molecular neurobiology of Drosophila taste. Curr. Opin. Neurobiol. 34, 140–148. doi: 10.1016/j.conb.2015.06.001
Gouin, A., Bretaudeau, A., Nam, K., Gimenez, S., Aury, J.-M., Duvic, B., et al. (2017). Two genomes of highly polyphagous lepidopteran pests (Spodoptera frugiperda, Noctuidae) with different host-plant ranges. Sci. Rep. 7:11816.
Grabherr, M. G., Haas, B. J., Yassour, M., Levin, J. Z., Thompson, D. A., Amit, I., et al. (2011). Full-length transcriptome assembly from RNA-Seq data without a reference genome. Nat. Biotechnol. 29, 644–652. doi: 10.1038/nbt.1883
Groh-Lunow, K. C., Getahun, M. N., Grosse-Wilde, E., and Hansson, B. S. (2015). Expression of ionotropic receptors in terrestrial hermit crab’s olfactory sensory neurons. Front. Cell. Neurosci. 8:448. doi: 10.3389/fncel.2014.00448
Grosse-Wilde, E., Stieber, R., Forstner, M., Krieger, J., Wicher, D., and Hansson, B. S. (2010). Sex-specific odorant receptors of the tobacco hornworm Manduca sexta. Front. Cell. Neurosci. 4:22. doi: 10.3389/fncel.2010.00022
Guerrieri, F., Gemeno, C., Monsempes, C., Anton, S., Jacquin-Joly, E., Lucas, P., et al. (2012). Experience-dependent modulation of antennal sensitivity and input to antennal lobes in male moths (Spodoptera littoralis) pre-exposed to sex pheromone. J. Exp. Biol. 215, 2334–2341. doi: 10.1242/jeb.060988
Guindon, S., Dufayard, J.-F., Lefort, V., Anisimova, M., Hordijk, W., and Gascuel, O. (2010). New algorithms and methods to estimate maximum-likelihood phylogenies: assessing the performance of PhyML 3.0. Syst. Biol. 59, 307–321. doi: 10.1093/sysbio/syq010
Guo, M., Chen, Q., Liu, Y., Wang, G., and Han, Z. (2018). Chemoreception of mouthparts: sensilla morphology and discovery of chemosensory genes in proboscis and labial palps of adult Helicoverpa armigera (Lepidoptera: noctuidae). Front. Physiol. 9:970. doi: 10.3389/fphys.2018.00970
Haas, B. J., Papanicolaou, A., Yassour, M., Grabherr, M., Blood, P. D., Bowden, J., et al. (2013). De novo transcript sequence reconstruction from RNA-seq using the Trinity platform for reference generation and analysis. Nat. Protoc. 8, 1494–1512. doi: 10.1038/nprot.2013.084
Hansson, B. S., and Stensmyr, M. C. (2011). Evolution of Insect Olfaction. Neuron 72, 698–711. doi: 10.1016/j.neuron.2011.11.003
Heath, R. R., Landolt, P. J., Dueben, B. D., Murphy, R. E., and Schneider, R. E. (1992). Identification of male cabbage looper sex pheromone attractive to females. J. Chem. Ecol. 18, 441–453. doi: 10.1007/bf00994243
Hill, S. R., Zaspel, J., Weller, S., Hansson, B. S., and Ignell, R. (2010). To be or not to be…a vampire: A matter of sensillum numbers in Calyptra thalictri? Arthropod. Struct. Dev. 39, 322–333. doi: 10.1016/j.asd.2010.05.005
Hussain, A., Zhang, M., Üçpunar, H. K., Svensson, T., Quillery, E., Gompel, N., et al. (2016). Ionotropic chemosensory receptors mediate the taste and smell of polyamines. PLoS Biol. 14:e1002454. doi: 10.1371/journal.pbio.1002454
Jacquin-Joly, E., Legeai, F., Montagne, N., Monsempes, C., Francois, M. C., Poulain, J., et al. (2012). Candidate chemosensory genes in female antennae of the noctuid moth Spodoptera littoralis. Int. J. Biol. Sci. 8, 1036–1050. doi: 10.7150/ijbs.4469
Katoh, K., and Standley, D. M. (2013). MAFFT multiple sequence alignment software version 7: improvements in performance and usability. Mol. Biol. Evol. 30, 772–780. doi: 10.1093/molbev/mst010
Keil, T. A. (1999). “Morphology and development of the peripheral olfactory organs,” in Insect Olfaction, ed. B. S. Hansson (Berlin: Springer), 5–47. doi: 10.1007/978-3-662-07911-9_2
Kent, K. S., Harrow, I. D., Quartararo, P., and Hildebrand, J. G. (1986). An accessory olfactory pathway in Lepidoptera: the labial pit organ and its central projections in Manduca sexta and certain other sphinx moths and silk moths. Cell Tissue Res. 245, 237–245.
Knecht, Z. A., Silbering, A. F., Cruz, J., Yang, L., Croset, V., Benton, R., et al. (2017). Ionotropic Receptor-dependent moist and dry cells control hygrosensation in Drosophila. Elife 6:e26654.
Knecht, Z. A., Silbering, A. F., Ni, L., Klein, M., Budelli, G., Bell, R., et al. (2016). Distinct combinations of variant ionotropic glutamate receptors mediate thermosensation and hygrosensation in Drosophila. Elife 5:e17879.
Koenig, C., Hirsh, A., Bucks, S., Klinner, C., Vogel, H., Shukla, A., et al. (2015). A reference gene set for chemosensory receptor genes of Manduca sexta. Insect Biochem. Mol. Biol. 66, 51–63. doi: 10.1016/j.ibmb.2015.09.007
Koh, T.-W., He, Z., Gorur-Shandilya, S., Menuz, K., Larter, N. K., Stewart, S., et al. (2014). The Drosophila IR20a clade of ionotropic receptors are candidate taste and pheromone receptors. Neuron 83, 850–865. doi: 10.1016/j.neuron.2014.07.012
Langmead, B., Trapnell, C., Pop, M., and Salzberg, S. L. (2009). Ultrafast and memory-efficient alignment of short DNA sequences to the human genome. Genome Biol. 10:R25.
Laue, M., and Steinbrecht, R. A. (1997). Topochemistry of moth olfactory sensilla. Int. J. Insect Morphol. Embryol. 26, 217–228. doi: 10.1016/s0020-7322(97)00023-8
Leal, W. S. (2013). Odorant reception in insects: roles of receptors, binding proteins, and degrading enzymes. Annu. Rev. Entomol. 58, 373–391. doi: 10.1146/annurev-ento-120811-153635
Leal, W. S., Choo, Y. M., Xu, P., da Silva, C. S., and Ueira-Vieira, C. (2013). Differential expression of olfactory genes in the southern house mosquito and insights into unique odorant receptor gene isoforms. Proc. Natl. Acad. Sci. U. S. A. 110, 18704–18709. doi: 10.1073/pnas.1316059110
Lefort, V., Longueville, J.-E., and Gascuel, O. (2017). SMS: Smart Model Selection in PhyML. Mol. Biol. Evol. 34, 2422–2424. doi: 10.1093/molbev/msx149
Legeai, F., Malpel, S., Montagne, N., Monsempes, C., Cousserans, F., Merlin, C., et al. (2011). An Expressed Sequence Tag collection from the male antennae of the Noctuid moth Spodoptera littoralis: a resource for olfactory and pheromone detection research. BMC Genomics 12:88. doi: 10.1186/1471-2164-12-86
Lemke, R.-S., Pregitzer, P., Eichhorn, A.-S., Breer, H., Krieger, J., and Fleischer, J. (2019). SNMP1 and odorant receptors are co-expressed in olfactory neurons of the labial and maxillary palps from the desert locust Schistocerca gregaria (Orthoptera: acrididae). Cell Tissue Res. 379, 275–289. doi: 10.1007/s00441-019-03083-x
Liu, N.-Y., Xu, W., Dong, S.-L., Zhu, J.-Y., Xu, Y.-X., and Anderson, A. (2018). Genome-wide analysis of ionotropic receptor gene repertoire in Lepidoptera with an emphasis on its functions of Helicoverpa armigera. Insect Biochem. Mol. Biol. 99, 37–53. doi: 10.1016/j.ibmb.2018.05.005
Marion-Poll, F., Guillaumin, D., and Masson, C. (1992). Sexual dimorphism of tarsal receptors and sensory equipment of the ovipositor in the European corn borer, Ostrinia nubilalis. Cell Tissue Res. 267, 507–518. doi: 10.1007/bf00319373
Marion-Poll, F., and Van Der Pers, J. (1996). Un-filtered recordings from insect taste sensilla. Entomol. Exp. Appl. 80, 113–115. doi: 10.1007/978-94-009-1720-0_27
Ning, C., Yang, K., Xu, M., Huang, L.-Q., and Wang, C.-Z. (2016). Functional validation of the carbon dioxide receptor in labial palps of Helicoverpa armigera moths. Insect Biochem. Mol. Biol. 73, 12–19. doi: 10.1016/j.ibmb.2016.04.002
Nishino, H., Nishikawa, M., Yokohari, F., and Mizunami, M. (2005). Dual, multilayered somatosensory maps formed by antennal tactile and contact chemosensory afferents in an insect brain. J. Comp. Neurol. 493, 291–308. doi: 10.1002/cne.20757
Olivier, V., Monsempes, C., Francois, M. C., Poivet, E., and Jacquin-Joly, E. (2011). Candidate chemosensory ionotropic receptors in a Lepidoptera. Insect Mol. Biol. 20, 189–199. doi: 10.1111/j.1365-2583.2010.01057.x
Pearce, S. L., Clarke, D. F., East, P. D., Elfekih, S., Gordon, K. H. J., Jermiin, L. S., et al. (2017). Genomic innovations, transcriptional plasticity and gene loss underlying the evolution and divergence of two highly polyphagous and invasive Helicoverpa pest species. BMC Biol. 15:63. doi: 10.1186/s12915-017-0402-6
Pelosi, P., Iovinella, I., Zhu, J., Wang, G., and Dani, F. R. (2018). Beyond chemoreception: diverse tasks of soluble olfactory proteins in insects. Biol. Rev. 93, 184–200. doi: 10.1111/brv.12339
Poitout, S., and Bues, R. (1974). Linolenic acid requirements of some Lepidoptera-noctuidae of quadriginae group reared on artificial diets. Ann. Nutr. Aliment. 28, 173–187.
Poivet, E., Gallot, A., Montagne, N., Glaser, N., Legeai, F., and Jacquin-Joly, E. (2013). A comparison of the olfactory gene repertoires of adults and larvae in the noctuid moth Spodoptera littoralis. PLoS One 8:e60263. doi: 10.1371/journal.pone.0060263
Qiu, Y.-T., van Loon, J. J. A., and Roessingh, P. (1998). Chemoreception of oviposition inhibiting terpenoids in the diamondback moth Plutella xylostella. Entomol. Exp. Appl. 87, 143–155. doi: 10.1046/j.1570-7458.1998.00316.x
Rimal, S., and Lee, Y. (2018). The multidimensional ionotropic receptors of Drosophila melanogaster. Insect Mol. Biol. 27, 1–7. doi: 10.1111/imb.12347
Rostelien, T., Stranden, M., Borg-Karlson, A. K., and Mustaparta, H. (2005). Olfactory receptor neurons in two Heliothine moth species responding selectively to aliphatic green leaf volatiles, aromatic compounds, monoterpenes and sesquiterpenes of plant origin. Chem. Senses 30, 443–461. doi: 10.1093/chemse/bji039
Rytz, R., Croset, V., and Benton, R. (2013). Ionotropic Receptors (IRs): Chemosensory ionotropic glutamate receptors in Drosophila and beyond. Insect Biochem. Mol. Biol. 43, 888–897. doi: 10.1016/j.ibmb.2013.02.007
Schmieder, R., Lim, Y. W., and Edwards, R. (2011). Identification and removal of ribosomal RNA sequences from metatranscriptomes. Bioinformatics 28, 433–435. doi: 10.1093/bioinformatics/btr669
Seada, M. A. (2015). Antennal morphology and sensillum distribution of female cotton leaf worm Spodoptera littoralis (Lepidoptera: Noctuidae). J. Basic Appl. Zool. 68, 10–18. doi: 10.1016/j.jobaz.2015.01.005
Seada, M. A., Ignell, R., Al Assiuty, A. N., and Anderson, P. (2018). Functional Characterization of the Gustatory Sensilla of Tarsi of the Female Polyphagous Moth Spodoptera littoralis. Front. Physiol. 9:1606. doi: 10.3389/fphys.2018.01606
Seada, M. A., Ignell, R., and Anderson, P. (2016). Morphology and distribution of ovipositor sensilla of female cotton leaf worm Spodoptera littoralis (Lepidoptera: noctuidae), and evidence for gustatory function. Entomol. Sci. 19, 9–19. doi: 10.1111/ens.12160
Simão, F. A., Waterhouse, R. M., Ioannidis, P., Kriventseva, E. V., and Zdobnov, E. M. (2015). BUSCO: assessing genome assembly and annotation completeness with single-copy orthologs. Bioinformatics 31, 3210–3212. doi: 10.1093/bioinformatics/btv351
Sparks, J. T., Bohbot, J. D., and Dickens, J. C. (2014). The genetics of chemoreception in the labella and tarsi of Aedes aegypti. Insect Biochem. Mol. Biol. 48, 8–16. doi: 10.1016/j.ibmb.2014.02.004
Stocker, R. F. (1994). The organization of the chemosensory system in Drosophila melanogaster: a rewiew. Cell Tissue Res. 275, 3–26. doi: 10.1007/bf00305372
Suh, E., Bohbot, J., and Zwiebel, L. J. (2014). Peripheral olfactory signaling in insects. Curr. Opin. Insect Sci. 6, 86–92. doi: 10.1016/j.cois.2014.10.006
van der Goes van Naters, W., and Carlson, J. R. (2006). Insects as chemosensors of humans and crops. Nature 444, 302–307. doi: 10.1038/nature05403
van Schooten, B., Jiggins, C. D., Briscoe, A. D., and Papa, R. (2016). Genome-wide analysis of ionotropic receptors provides insight into their evolution in Heliconius butterflies. BMC Genomics 17:254. doi: 10.1186/s12864-016-2572-y
Vogt, R. G., Miller, N. E., Litvack, R., Fandino, R. A., Sparks, J., Staples, J., et al. (2009). The insect SNMP gene family. Insect Biochem. Mol. Biol. 39, 448–456. doi: 10.1016/j.ibmb.2009.03.007
Vosshall, L. B., and Stocker, R. E. (2007). Molecular architecture of smell and taste in Drosophila. Annu. Rev. Neurosci. 30, 505–533.
Walker, W. B. III., Roy, A., Anderson, P., Schlyter, F., Hansson, B. S., and Larsson, M. C. (2019). Transcriptome analysis of gene families involved in chemosensory function in Spodoptera littoralis (Lepidoptera: noctuidae). BMC Genomics 20:428. doi: 10.1186/s12864-019-5815-x
Widmayer, P., Heifetz, Y., and Breer, H. (2009). Expression of a pheromone receptor in ovipositor sensilla of the female moth (Heliothis virescens). Insect Mol. Biol. 18, 541–547. doi: 10.1111/j.1365-2583.2009.00894.x
Xu, P., Wen, X., and Leal, W. S. (2020). CO2 per se activates carbon dioxide receptors. Insect Biochem. Mol. Biol. 117:103284. doi: 10.1016/j.ibmb.2019.103284
Xu, W., and Anderson, A. (2015). Carbon dioxide receptor genes in cotton bollworm Helicoverpa armigera. Sci. Nat. 102:11.
Xu, W., Liu, N., Liao, Y., and Anderson, A. (2017). Molecular characterization of sugar taste receptors in the cotton bollworm Helicoverpa armigera. Genome 60, 1037–1044. doi: 10.1139/gen-2017-0086
Xu, W., Papanicolaou, A., Zhang, H.-J., and Anderson, A. (2016). Expansion of a bitter taste receptor family in a polyphagous insect herbivore. Sci. Rep. 6:23666.
Yao, C. A., Ignell, R., and Carlson, J. R. (2005). Chemosensory coding by neurons in the coeloconic sensilla of the Drosophila antenna. J. Neurosci. 25, 8359–67. doi: 10.1523/jneurosci.2432-05.2005
Zhang, J., Wang, B., Dong, S., Cao, D., Dong, J., Walker, W. B., et al. (2015). Antennal transcriptome analysis and comparison of chemosensory gene families in two closely related Noctuidae moths, Helicoverpa armigera and H. assulta. PLoS One 10:e0117054. doi: 10.1371/journal.pone.0117054
Zhang, Y. V., Ni, J., and Montell, C. (2013). The molecular basis for attractive salt-taste coding in Drosophila. Science 340, 1334–1338. doi: 10.1126/science.1234133
Keywords: RNAseq, chemosensory transcriptome, Spodoptera littoralis, proboscis, palps, legs, ovipositor
Citation: Koutroumpa FA, Monsempes C, François M-C, Severac D, Montagné N, Meslin C and Jacquin-Joly E (2021) Description of Chemosensory Genes in Unexplored Tissues of the Moth Spodoptera littoralis. Front. Ecol. Evol. 9:678277. doi: 10.3389/fevo.2021.678277
Received: 09 March 2021; Accepted: 25 May 2021;
Published: 01 July 2021.
Edited by:
Ahmed M. Saveer, North Carolina State University, United StatesReviewed by:
Sneha Mokashi, Clemson University, United StatesCopyright © 2021 Koutroumpa, Monsempes, François, Severac, Montagné, Meslin and Jacquin-Joly. This is an open-access article distributed under the terms of the Creative Commons Attribution License (CC BY). The use, distribution or reproduction in other forums is permitted, provided the original author(s) and the copyright owner(s) are credited and that the original publication in this journal is cited, in accordance with accepted academic practice. No use, distribution or reproduction is permitted which does not comply with these terms.
*Correspondence: Fotini A. Koutroumpa, Zm90aW5pLmtvdXRyb3VtcGFAZ21haWwuY29t; Emmanuelle Jacquin-Joly, ZW1tYW51ZWxsZS5qb2x5QGlucmFlLmZy
Disclaimer: All claims expressed in this article are solely those of the authors and do not necessarily represent those of their affiliated organizations, or those of the publisher, the editors and the reviewers. Any product that may be evaluated in this article or claim that may be made by its manufacturer is not guaranteed or endorsed by the publisher.
Research integrity at Frontiers
Learn more about the work of our research integrity team to safeguard the quality of each article we publish.