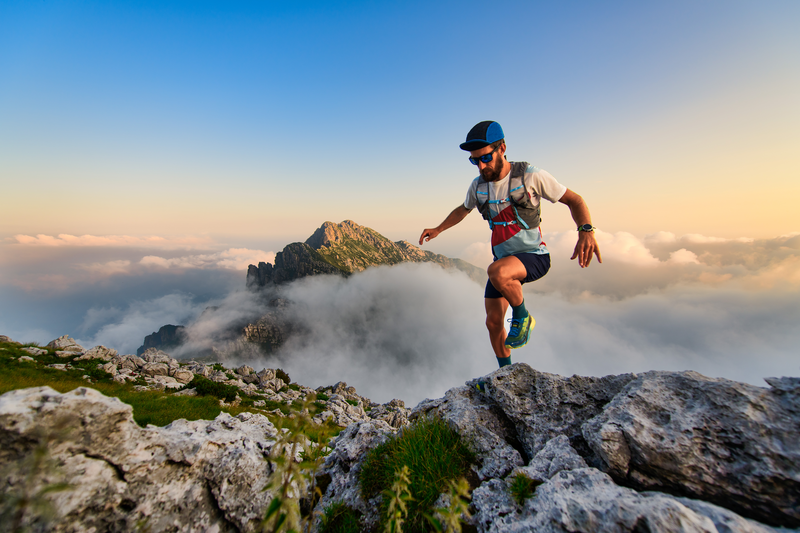
94% of researchers rate our articles as excellent or good
Learn more about the work of our research integrity team to safeguard the quality of each article we publish.
Find out more
ORIGINAL RESEARCH article
Front. Ecol. Evol. , 05 October 2021
Sec. Population, Community, and Ecosystem Dynamics
Volume 9 - 2021 | https://doi.org/10.3389/fevo.2021.676993
This article is part of the Research Topic Fluctuating Habitats: Ecological Relevance of Environmental Variability and Predictability on Species, Communities, and Ecosystems View all 9 articles
Studies on population dynamics are helpful for understanding the factors determining population development and predicting the effects of disturbances, such as harvesting of plant species. In an investigation of the demography of a terrestrial medicinal orchid known as Crepidium acuminatum, the effects of harvesting on its population dynamics were recorded. Data on recruitment, growth and survival were collected in three populations of C. acuminatum over a 6-year period (2012–2017) in central Nepal. A matrix modeling method was used to determine the effect of different harvesting regimes on the population growth and survival of this species. Population growth rates (λ) of unharvested populations were relatively similar and stable in different years of the study. Harvesting significantly reduced λ. The results of this study indicate that the sustainable survival of a population that is subject to harvesting can only occur when it is either selective (only flowering individuals or only small amounts of vegetative individuals) or rotational (once every 3–5 or more years). This study demonstrates the necessity of using a sustainable method when harvesting natural populations. Our results are useful for developing efficient management strategies for this species. As each species has a different biology, similar studies are needed for other rare and/or economically important species in the Himalayan region and in other understudied parts of the world.
Collecting and analyzing long-term data on population dynamics is essential for understanding factors determining future fates of populations (Hutchings, 2010; Černá and Münzbergová, 2013; Rokaya et al., 2017; Dostálek et al., 2018; Shefferson et al., 2020). The basic information obtained from studies on population dynamics allows us to assess the present population status, its future fates and predict the effects of different factors, such as harvesting or herbivore damage, on a population’s performance (Caswell, 2000a; Coates et al., 2006; Crone et al., 2013; Gamelon et al., 2017; Rokaya et al., 2017; Dostálek et al., 2018) or effects of stochastic effects such as large storms or hurricanes on population viability estimates (Crain et al., 2019). Population dynamic studies can therefore be used to formulate plans for species recovery and management (Caswell, 2000a; Crone et al., 2013; Nurfadilah, 2017; Rokaya et al., 2017; Ticktin et al., 2019).
Studies dealing with demographic structure of plant populations involve investigation of the complete life cycle of the species, including seed production, seedling recruitment, plant growth, survival and flowering (Münzbergová, 2005, 2007; Münzbergová and Ehrlén, 2005; Heinken-Šmídová and Münzbergová, 2012; Dostálek and Münzbergová, 2013; Castro et al., 2015). Determining the processes in population dynamics involves detailed observations of fate of individual plants at different stages of development over time (Caswell, 2000a; Ticktin, 2004; Schmidt et al., 2011). The stages can be based on plant size (Castro et al., 2015; Dostálek et al., 2018), leaf size or leaf number (Münzbergová, 2007; Heinken-Šmídová and Münzbergová, 2012), petiole length (Rokaya et al., 2017), age (Bucharová et al., 2012), etc. (Crone et al., 2011).
Demographic studies are especially important, when endangered species with special demands, such as orchids, are considered. The orchid life cycle is quite unique because of their symbiosis with specific fungi and pollinators, special nature of their substrates or host species on which they grow. In addition, orchid seeds are tiny and lack any food reserves, thus they need to form an association with a symbiotic fungus to germinate (Rasmussen et al., 2015; Sonkoly et al., 2016). There are currently very few studies on the population dynamics of different terrestrial species of orchids (e.g., Shefferson et al., 2020) and none from biodiversity rich Nepal (Shefferson et al., 2020), where there are 450 species and 107 genera of orchids (Paudel, 2020). Most of the species of orchids in Nepal are either threatened or even at the verge of extinction (Raskoti, 2009; Pant and Raskoti, 2013; Subedi et al., 2013; Vaidya, 2019). Thus, there is an immediate need to conserve these species (Wraith et al., 2020).
In addition, many orchids are economically important as aphrodisiacs, for medicine, as food or ornamental species (Teoh, 2016, 2019; Singh et al., 2019). Such species are collected indiscriminately for the local use (Charitonidou et al., 2019) or widely traded legally/illegally (Subedi et al., 2013; Fay, 2018; Hinsley et al., 2018; Ticktin et al., 2019). Thus, there is a need to develop sustainable harvesting strategies for these economically important species of orchids (Ghimire et al., 2008; Mondragón Chaparro and Ticktin, 2011; Schmidt et al., 2011; Klimas et al., 2012).
Both successful conservation and sustainable harvesting depend on detailed studies of demographic structure and population performance, using simulation methods in population projection matrices, as previously done for different medicinal plants (Nantel et al., 1996; Ticktin, 2004; Ghimire et al., 2008; Rokaya et al., 2017) including orchids (García-González et al., 2017; Ticktin et al., 2019; Orozco-Ibarrola et al., 2021). Such studies may help elucidate best practice strategies for population persistence and use by the local people (Ghimire et al., 2008; Rokaya et al., 2017).
The present study concentrates on three populations of Crepidium acuminatum (D. Don) Szlach., a terrestrial medicinal orchid, growing in central Nepal. The effects of harvesting on the dynamics of this species were studied, in order to answer the following questions:
(1) Are there any differences in population dynamics of the three populations of C. acuminatum studied in Nepal?
(2) What is the effect of harvesting on the population dynamics of C. acuminatum and how does it differ between the populations studied?
The permissions required for entering the localities in Shivpuri Nagarjun National Park were obtained from the Department of National Parks and Wildlife Conservation (DNPWC), Nepal. This study of individual plants was only observational and did not involve damaging the plants.
Crepidium acuminatum (D. Don) Szlach. (Synonym Malaxis acuminata D. Don, Malaxis acuminata forma biloba (Lindl.) Tuyama, Microstylis wallichii Lindl.) of the family Orchidaceae is a long-lived 20–40 cm tall perennial terrestrial herbaceous plant with pseudobulbs at its base. Basal portion of shoot is covered by 1 or 2 sheathing bracts and the bases of the 3 or 4 or more elliptic-lanceolate 7–13 × 2–5 cm sized leaves. Leaf tip is acute to acuminate, sheathing at the base. Inflorescence is terminal and scape ridged. Flowers are dark purple. It is quite rare, but locally abundant and found in moist places in deciduous or subtropical forests. Flowering and fruiting are from May to July (Pearce and Cribb, 2002). In Nepal, it occurs in the central and eastern part (450–3,050 m a.s.l.) and in the rest of the world in Australia, Bhutan, China (Guang-dong, SW Guizhou, Taiwan, SE Xizang, S Yunnan), Cambodia, India, Indonesia, Laos, Myanmar, Philippines, Thailand and Vietnam (Raskoti, 2009; Rokaya et al., 2013). In Nepal, it is locally known as Jivak. This plant species is reproduced by means of seeds and also through propagation of pseudobulbs (Pearce and Cribb, 2002).
Pseudobulbs are used in the ayurvedic medicine as one of the ingredients of “ashtawarga” used in tonic preparations (Khare, 2015). The taste of the pseudobulbs is slightly sweet. They are used to treat fever, seminal weakness, burning sensations, excessive thirst, hematemesis or vomiting blood, bronchitis, pulmonary tuberculosis and weakness (Khare, 2007, 2015; Pant and Raskoti, 2013; Vaidya, 2019). Although it is widely used as a medicine, the exact amount traded is unknown. Mostly the whole plant, but some time only underground parts, are harvested, dried and illegally sold in the market for use in local traditional medicine and international trade. However, some traded plants are not well identified up to species level, especially when sterile individuals are harvested (Subedi et al., 2013), and may thus represent other related species.
A demographic study of three populations of C. acuminatum was carried out on Shivapuri hill in central Nepal. All three populations were at an altitude of about 2,150 m a.s.l. in the forest and Population 1 and 3 were close to trails, whereas population 2 was at least 100–150 m from a trail in a deep forest.
In August 2012, we selected approximately 150–200 individuals per population (usually all plants within the population), permanently tagged them and inspected them every year in August until 2017, i.e., for 6 subsequent years. We ensured that individuals at different stages (as defined below) were marked in similar proportions (Münzbergová and Ehrlén, 2005) and also ensured that data are collected each year from 2012 to 2017 as incomplete matrices (holey matrices) may result in biologically non-sensical matrices (Tremblay et al., 2021). Every year, any newly developing plants found at each locality were also tagged. These plants were in areas where previously C. acuminatum was not recorded and very likely had germinated from seed or developed from pseudobulbs. In each census, we recorded the number of leaves, total height and status (vegetative/flowering) of each plant. Mortality of plants was also recorded the following year.
After flowering C. acuminatum produces capsules containing many minute dust-like seeds. Due to the difficulty of monitoring the germination of its dust-like seeds, we marked all the plants in specific areas in this study of its population dynamics. In addition, we marked C. acuminatum plants growing close to the areas studied in 2012 and recorded new seedlings that appeared the following year, 2013. This was repeated again each year until 2017, the end of study period. The seedling recruitment recorded in this study is the number of new seedlings that appeared each year over the total number of flowering plants the previous year. Thus, we assumed that there is germination of all seeds to seedlings (small plants) within 1 year in our species. It is also possible that some of the new individuals arise vegetatively from the pseudobulbs. While this assumption is likely not correct, it is considered as the best solution to the problem that the seeds can stay in dormancy for a variable number of years and the time of development to seedling is unknown (Shefferson, 2002; Gregg, 2011). This approach is used also in other studies on other species of orchids (Ackerman et al., 1996; García-González et al., 2017; Raventós et al., 2018).
Using generalized linear model (GLM) with a binomial link, we looked for thresholds in plant traits (number of leaves and height of plant individuals) that differentiated between vegetative plants with low and high probability of flowering the following year. In the regression, flowering the following year (yes/no) was used as dependent variable and plant traits as independent variables. GLM analysis was carried out using glm() function in lmerTest package (Kuznetsova et al., 2017) in R 4.0.0 (R Development Core Team, 2020). We used the knowledge obtained in the tests to classify individuals into three stages, based on the number of leaves and flowering status: small vegetative (with fewer than 3 leaves and not flowering), large vegetative (more than 4 leaves and not flowering) and flowering individuals (bearing flowers, but leaves ranged from 1 to 7 in numbers, meaning that flowering plants do not always bear high number of leaves). Small vegetative plants included small plants with zero or very low probability of flowering the following year and made up approximately one-third of the tagged plants. Small vegetative plants were usually large vegetative plants the following year. Most of the large vegetative plants remain in this stage for more than one year and until they gather enough resources for flowering and producing dust-like seeds. However, there were very less flowering plant individuals and it shows that this particular species is resource limited for reproduction (Tremblay et al., 2004). In general, the plant usually dies after flowering, although it is not strictly monocarpic—it can be vegetative the next year and flower the following year. In very rare cases, it can flower in two consecutive years. We thus consider this species to be perennial and non-clonal with polycyclic bulbs (Klimešová et al., 2016).
We compared differences in the probability of stasis, growth, retrogression, flowering and mortality in different years and populations. For this comparison, we used logistic regression with stage in the previous year, population type and interaction of year × population as independent variables. Stasis was defined as plants surviving and remaining in the same stage, growth as development to a later stage, i.e., growth from a small vegetative to a large vegetative or flowering plant, or large vegetative to flowering plants and retrogression as a reduction in growth from a large to a smaller stage, such as a flowering plant to a large vegetative plant. Analyses of vital rates were carried out using GLM using glm() function in lmerTest package (Kuznetsova et al., 2017) in R 4.0.0 (R. Development Core Team, 2020).
Data on recruitment, growth and survival of individuals, classified by size, were gathered over a period of 6 years (2012–2017). Stage-based population projection matrix models were used to estimate demographic parameters (Caswell, 2000a). The model used was:
where n is a column vector containing the number of individuals in each stage at time t or t+1, and A is a square matrix with the matrix elements representing transition probabilities between stages. Three stages were used in this study: small vegetative (a1) termed as seedling (S), large vegetative (a2) termed as non-flowering adults (V) and flowering individuals (a3) termed as flowering adults (FA) (Figures 1A,B). aij indicates transitions in matrix A from stage j to stage i in 1-year time intervals. From each transition matrix constructed for each population and year (Supplementary Table 1), lambda (λ) was calculated, which is known as the population growth rate (Caswell, 2000a), and elasticity (Supplementary Table 1), which is usually used as a measure of the relative contribution of a matrix element to fitness (de Kroon et al., 2000). Elasticity analyses can be used to identify stages for potential management because the high elasticity of changes in vital rates will result in large changes in λ (Caswell, 2000b).
Figure 1. (A) Life cycle diagram for Crepidium acuminatum. Circles represent individual life stages: (S) seedlings, (V) non-flowering adults, (FA) flowering adults. Arrows represent all possible transitions: (Gnm) growth, (Snm) survival), (Rnm) retrogression and (Fnm) fertility, between each of three life stages, where m is the initial stage and n is the subsequent stage. (B) Generalized population projection matrix corresponding with the life cycle diagram in (A).
Both the 95% confidence intervals (CI) of λ and elasticity values of each transition matrix were estimated using the bootstrap percentile-interval method (Alvarez-Buylla and Slatkin, 1994). Bootstrapping the original data to derive the transition matrices was done 105 times (see Dostálek and Münzbergová, 2013; Rokaya et al., 2017). For this purpose, we used a MATLAB script developed for a previous study by Münzbergová (2006).
In summary, for information on λ and elasticity for populations and years, we used the simulation approach suggested by Caswell (2000a) and Rydgren et al. (2007). We calculated two types of population growth rate, λS. (1) There were five λS values for each year, which were based on years 2012–2017. (2) Overall stochastic λS values were based on a combination of data from all years 2012–2017 and populations. Simulations were performed using a MATLAB script developed in a previous study (Münzbergová, 2005). We ran the same simulations for the bootstrapped matrices as described above. Therefore, we were able to construct 95% confidence intervals for the population growth rates and elasticity (Černá and Münzbergová, 2013).
A life-table response experiment (LTRE) (Caswell, 2000a) with a fixed factorial design was conducted to examine the contribution of each transition to the observed variation in λ between populations in different populations growing sites. LTRE is a form of retrospective analysis that allows quantification of factors responsible for the observed variation in population growth rates and is analogous to ANOVA. Compared to the elasticity analysis, the LTRE analysis quantifies the observed effects of single matrix elements on observed variation in the population growth rate, as opposed to expected effects. Potential best management actions identified by elasticity analysis are sometimes not realized, because it is difficult to change vital rates with high elasticity values. In addition, LTRE analyses enable the identification of variable matrix elements with the highest positive or negative contributions to the population growth rate (λ). The significance of LTRE was estimated using the permutation test used by Münzbergová (2007) and based on 10,000 permutations. To visualize the LTRE values and compare the three populations, we also summed the positive and negative contributions for survival separately (including transitions a11, a12, a22, a23, a33), fecundity (a13) and growth (a21, a31, a32) and plotted these values (Jongejans and De Kroon, 2005; Münzbergová, 2013; Černá and Münzbergová, 2013; Rokaya et al., 2017).
To assess the effects of harvesting on C. acuminatum, we produced projections of population size over 50 years under four different levels of harvesting (see below). To simulate environmental stochasticity, one of the five matrices (five transition intervals) available for each type of population was randomly selected at each simulation step. At each step, the resulting population vector (number of plants in each stage) was replaced by values drawn from a Poisson distribution with the appropriate mean, which is the standard procedure for simulating demographic stochasticity (Caswell, 2000a). This projection was repeated 1,000 times for each type of population (Münzbergová, 2005). Harvesting was simulated by removing 0, 25, 50, 75, and 100% of the natural population every 1, 3, 5, and 10 years (Ghimire et al., 2008; Rokaya et al., 2017). This was carried out in different scenarios, including removal of (i) only plants in the large vegetative and flowering stages, (ii) only large vegetative plants, (iii) only flowering plants and (iv) all plants (small and large vegetative and flowering plants). Small vegetative plants are not intentionally harvested for pseudobulbs. However, they are usually destroyed when commercial collectors randomly dig up mature plants and during this process destroy other small plant individuals too, leading to the destruction of all plant individuals and thus destruction of the whole population. Therefore, in simulation tests, we also used a scenario in which all of the plants are removed. For the initial population vector for the harvesting simulations, we used 100 plants divided according to the stable stage distribution (Caswell, 2000a) and averaged for all populations, i.e., 49 small vegetative, 36 large vegetative and 15 flowering plants. The stable stage distribution was very similar for the populations. By using the mean stable stage distribution, we ensured that all populations started under exactly the same conditions in our simulations. The MATLAB script used during the analysis is that used previously in other studies (Münzbergová, 2005, 2006).
All simulations were performed using Matlab, version 6.0.0.88-The MathWorks, Inc., Natick, Massachusetts, United States (MATLAB, 2002).
All the vital rates (probability of stasis, growth, retrogression, flowering and mortality) tested were significantly affected by stage in the previous year and except for growth, which varied significantly between years. Growth, flowering and mortality significantly varied among populations. The differences in flowering and mortality only varied in population 2. Individuals in population 2 flowered more than in populations 1 and 3 and mortality in population 2 was lower than in populations 1 and 3 (Table 1 and Figure 2).
Table 1. Effects of stage in previous year, year, populations and their interactions on probability of stasis (survival and remaining in the same stage), growth (growth to larger stage), retrogression (shrinkage from large stage to small stage), flowering and mortality in the next year.
Figure 2. Comparison of the vital rates of three populations. The vital rates are the probability of stasis (survival and remaining in the same stage), growth (growth to a larger stage), retrogression (shrinkage from a large to a small stage), flowering and mortality the following year. Boxes show means, standard errors and 1.96 * standard errors (defining 95% confidence interval of the mean) of the percentage of individuals undergoing a given transition. Different letters indicate significant differences in vital rates between populations (Tukey’s post hoc test, P< 0.05).
There was no significant variation in the population growth rate between populations and years. The population growth rates (λS) within 1 year varied from 0.81 to 1.05 for population 1, 0.91–1.22 for population 2 and 0.73–1.13 for population 3 over 5 transition intervals (Figure 3). Overall stochastic population growth rates (λS) based on a combination of data from 2012 to 2017 showed that each λ had equal probabilities and population 1 had the lowest growth rate (λS = 1.05), followed by population 3 (λS = 1.12) and population 2 (λS = 1.27; see Figure 3).
Figure 3. Population growth rates and their 95% confidence intervals for three populations. The last value for each population (indicated by asterisks) shows stochastic population growth rate for all transition intervals combined (2012–2017). Dashed line (λ = 1) indicates a stable population.
The transition with the highest elasticity value for all populations was stasis of large vegetative plants (a22) (Supplementary Table 1). Other important transitions were associated with growth (a21 and a32) and fecundity (a13). There were no differences in elasticity values among populations (Supplementary Figure 1).
According to the LTRE analyses, population growth of C. acuminatum in population 1 was negatively affected by fecundity, survival and growth (mainly the transition from small vegetative to large vegetative plants). Population 2 was positively affected by fecundity and survival-related transitions (mainly the high production of large vegetative plants from large vegetative and flowering from large vegetative stages), but negatively affected by growth (the transition from large vegetative plants to flowering plants). Population 3 was positively affected by fecundity, survival and growth (mainly the transition from small vegetative to large vegetative stages) (Table 2, Figure 4, and Supplementary Figure 2).
Table 2. Results of LTRE analyses comparing the contribution of single matrix elements to the recorded variation in overall population growth rate between populations.
Figure 4. Result of LTRE analysis showing positive and negative contributions of three populations to the observed variation in overall stochastic population growth rate grouped by life history components (survival, i.e., stasis and retrogression, fecundity and growth).
Harvesting 25% or more of all plant individuals every year led to a significant decline in population size and increase in the probability of extinction within 30 years in all three populations. Population 3 was more vulnerable than population 1 and 2 (Figures 5, 6 and Supplementary Table 2).
Figure 5. Probabilities of three populations of C. acuminatum becoming extinct in 30 years when 25% of the individuals in all stages, only the large vegetative and flowering stages (Large veg. and flow.), only the large vegetative stage (Large vegetative) or only the flowering (Flowering) stage are harvested at different frequencies. 1, 3, 5, 10 on X-axis indicates the interval of harvesting in years.
Figure 6. Probabilities of three populations of C. acuminatum becoming extinct in 30 years when different percentages (0, 25, 50, 75, and 100%) of all individuals, only large vegetative and flowering, only large vegetative or only flowering stages are harvested every 3 years.
The negative effect of harvesting was partly reduced when only large vegetative and flowering plants were harvested separately. The lowest extinction probabilities were achieved when only flowering plants were harvested (Figures 5, 6).
Harvesting is sustainable (i.e., the extinction probabilities for all populations in 30 years is less) when harvesting is carried out at the rate of 25% of all plant stages in a rotation of 3–5 years (Figures 5, 6 and Supplementary Table 2).
A long-term study of the population dynamics of C. acuminatum showed that the three populations studied were relatively stable with their growth rates (λS) of near to 1. However, all populations were seriously affected by harvesting as even in low harvesting percentages (i.e., 25% of all available plants) every year led to a significant decline in population size and increased in the probability of extinction in all populations. Our study showed that harvesting can be sustainable, if the period between harvests is long (at least 3–5 years) or when it is restricted to certain plant stages, such as small numbers of vegetative individuals or only flowering individuals.
C. acuminatum is capable of reproducing through bulbs and removing large individuals with fruits may not have very strong effect on seedling recruitment. It was also observed that most of the plant individuals in studied populations were in non-flowering stages meaning that flowering or seed production plays less role in population growth. Experiment related to harvesting of pseudobulb has shown death of no plants as more than 55% produced new pseudobulbs yearly in Laelia autumnalis orchid in Mexico (Emeterio-Lara et al., 2021).
Most of the studies on the effect of harvesting on the population dynamics of different economically important plants in the world are based only on data collected during 2–3 years (Ghimire et al., 2008; Schmidt et al., 2011; Ticktin et al., 2019). However, the previous study on medicinal plants (Rheum acuminatum and R. australe) in Nepal (Rokaya et al., 2017) was based on 6-year study period as the present study. Results of both studies were similar showing a significant decline in population size and increase in the probability of extinction within 30 years in all populations due to harvesting of the plant. The similarity in results in both studies is because studied species were perennial in nature and underground parts of both species were used as medicine (Khare, 2007, 2015; Pant and Raskoti, 2013; Rokaya et al., 2017; Dostálek et al., 2018; Vaidya, 2019). We focused in having a long-term study because such study can result in novel insights into the temporal variability in the overall dynamics of plant species (Oostermeijer et al., 1996; Fréville et al., 2004; Marrero-Gómez et al., 2007; Jongejans et al., 2010; Crone et al., 2011; Bucharová et al., 2012; Reinke et al., 2019).
Our study showed variability in stasis, growth, retrogression, flowering and mortality over time that depended on the stages present in previous years. There was also variability in growth, flowering and mortality among populations. These findings are similar to previous findings for other perennial plants (Münzbergová, 2005, 2013; Heinken-Šmídová and Münzbergová, 2012; Černá and Münzbergová, 2013; Rokaya et al., 2017; Dostálek et al., 2018) and terrestrial orchids (Shefferson et al., 2020). The variability in stasis, growth, retrogression, flowering and mortality over time is mostly driven by fluctuations in climate from 1 year to the next (Gamelon et al., 2017; Shefferson et al., 2020), which can markedly affect flowering, fruiting, seed production and also seedling recruitment (Fréville et al., 2004). In addition to this, finding of variability in growth, flowering and mortality among populations is due to the presence of variation in the local environmental factors that ultimately have influence in the growth of plant individuals in different habitats (Rokaya et al., 2017).
Population growth rates (λ) for C. acuminatum in all the years were close to 1 meaning that the populations were almost stable and do not fluctuate. This is because the studied species is perennial in nature and similar results were obtained for many other perennial plant species in the world (García et al., 2008; Ramula et al., 2008; Shefferson et al., 2020). It is pointed out that stable population dynamics is a common feature of perennial species with tuberous bulbs that usually store resources in underground parts and are as a consequence better able to withstand changing environmental conditions (García et al., 2008).
In this study, we report a high elasticity value for stasis, which is similar to that reported for epiphytic orchids in Southeast México (Mondragón, 2009) and other epiphytic angiosperms (Mondragón et al., 2015). High elasticity value for statis, among the different vital rates (such as statis, growth, retrogression and reproduction), means that stasis plays a vital role and contributes most to population growth rates. The retrospective (LTRE) analysis revealed both positive and negative contributions of fecundity, survival and growth to the population growth of C. acuminatum. From our results, we found that population 1 can be best managed by increasing fecundity, survival and growth and population 2 by increasing growth as variation in these vital rates contributed negatively to population growth rates. However, there is no need to manage population 3 as all the vital rates contributed positively (Heinken-Šmídová and Münzbergová, 2012).
This study revealed that the effects of harvesting on population dynamics of C. acuminatum depend on different plant stages that are harvested for economic use. Populations are negatively affected by frequent harvesting large vegetative individuals with high elasticity values, as was reported in previous studies (Ghimire et al., 2008; Rokaya et al., 2017) or by frequent harvesting all the plants would also completely eradicate the species (Rock et al., 2004; Ticktin, 2004; Lázaro-Zermeño et al., 2011; Ticktin et al., 2019). However, selective harvesting of numbers of vegetative individuals or only flowering individuals in our study species or a long-term rotational harvesting (preferably once in 3–5 years) ensures the survival of populations in their natural habitat and this practice helps to protect C. acuminatum from complete eradication in their habitat as in Rheum austral and R. acuminatum in Nepal (Rokaya et al., 2017). Similar to our findings, previous studies have reported that populations of perennial plants are not seriously endangered by harvesting their flowers or flowering individuals (Ticktin, 2004; Emanuel et al., 2005; Schmidt and Ticktin, 2012; Castro et al., 2015). Our study indicated that frequent harvesting of all plants is not sustainable as shown by Ticktin et al. (2019). As most of the plant individuals are in vegetative stage and this species can also reproduce through bulb, selective harvesting of only flowering individuals or a small number of vegetative individuals will not deplete the population and such practices will ensure the survival of the studied terrestrial orchid, C. acuminatum.
Our sampling was carried out for a long time period (for 6 years), and was quite extensive. Different demographic processes and future fates of our study species are well illustrated for less studied orchid species in Nepal. Despite the lack of field harvesting experiments, we have used simulations that are commonly used in demographic studies to show the effect of harvesting on demographic structure and population performance (Ticktin et al., 2019) and also to evaluate demographics and extinction risk of rare and endemic plants such as Lepanthes caritensis (Orchidaceae) (Crain et al., 2019). These simulations allow us to formulate management plans to protect plant species from getting extinct (Ghimire et al., 2008; Rokaya et al., 2017; Crain et al., 2019; Ticktin et al., 2019).
Overharvesting and random harvesting of many medicinal plant species throughout the Himalayan region has negatively affected the performance and also their existence. It is thus important to protect these plant species. As our study is in line with previous studies from Nepal (Ghimire et al., 2008; Rokaya et al., 2017; Dostálek et al., 2018) and results can serve as baseline information for other similar species that grow in the similar climatic conditions and habitat, mainly in Nepal.
This study revealed that although the natural populations of C. acuminatum studied are almost stable, they are negatively affected by harvesting. If harvesting is random or too frequent, this species may be seriously threatened or even become extinct. Thus, sustainable harvesting strategies are necessary as: (i) selective harvesting or rotation harvesting is needed to protect natural populations of C. acuminatum and (ii) transplantation/cultivation or tissue culture techniques should be used to protect natural populations that are over harvested or destroyed. The present research results indicate that it is possible to maintain natural populations of orchids, as reported for 15 Mexican orchids (Ticktin et al., 2019) and long-term research should be undertaken on other vulnerable species of plants, especially orchids that are highly endangered (Fay, 2018).
The raw data supporting the conclusions of this article will be made available by the authors, without undue reservation.
BT, PK, and MR conceived the idea. BT and MR collected field data and wrote the manuscript. BT, MR, and ZM analyzed the data. PK and ZM contributed significantly to the ideas presented and edited the manuscript. All authors contributed critically to the final manuscript and approved its publication.
This work was supported by the Ministry of Education, Youth and Sports of Czech Republic within the CzeCOS Program (grant no. LM2018123) to BT and project GACR (grant no. 17-10280S) to ZM. MR acknowledges institutional support RVO (grant no. 67985939).
The authors declare that the research was conducted in the absence of any commercial or financial relationships that could be construed as a potential conflict of interest.
All claims expressed in this article are solely those of the authors and do not necessarily represent those of their affiliated organizations, or those of the publisher, the editors and the reviewers. Any product that may be evaluated in this article, or claim that may be made by its manufacturer, is not guaranteed or endorsed by the publisher.
We are indebted to Tony Dixon for editing the language. We also thank the Department of National Parks and Wildlife Conservation (DNPWC) for providing permission to collect data during this study.
The Supplementary Material for this article can be found online at: https://www.frontiersin.org/articles/10.3389/fevo.2021.676993/full#supplementary-material
Ackerman, J. D., Sabat, A., and Zimmerman, J. K. (1996). Seedling establishment in an epiphytic orchid: an experimental study of seed limitation. Oecologia 106, 192–198. doi: 10.1007/BF00328598
Alvarez-Buylla, E. R., and Slatkin, M. (1994). Finding confidence limits on population growth rates: three real examples revised. Ecology 75:255. doi: 10.2307/1939401
Bucharová, A., Brabec, J., and Münzbergová, Z. (2012). Effect of land use and climate change on the future fate of populations of an endemic species in central Europe. Biol. Conserv. 145, 39–47. doi: 10.1016/j.biocon.2011.09.016
Castro, S., Dostálek, T., Meer, S., van der Oostermeijer, G., and Münzbergová, Z. (2015). Does pollen limitation affect population growth of the endangered Dracocephalum austriacum L.? Popul. Ecol. 57, 105–116. doi: 10.1007/s10144-014-0458-x
Caswell, H. (2000b). Prospective and retrospective perturbation analyses: their roles in conservation biology. Ecology 81, 619–627. doi: 10.2307/177364
Černá, L., and Münzbergová, Z. (2013). Comparative population dynamics of two closely related species differing in ploidy level. PLoS One 8:e75563. doi: 10.1371/journal.pone.0075563
Charitonidou, M., Stara, K., Kougioumoutzis, K., and Halley, J. M. (2019). Implications of salep collection for the conservation of the Elder-flowered orchid (Dactylorhiza sambucina) in Epirus. Greece. J. Biol Res. Thessaloniki 26:18. doi: 10.1186/s40709-019-0110-1
Coates, F., Lunt, I. D., and Tremblay, R. L. (2006). Effects of disturbance on population dynamics of the threatened orchid Prasophyllum correctum D.L. Jones and implications for grassland management in south-eastern Australia. Biol. Conserv. 129, 59–69. doi: 10.1016/j.biocon.2005.06.037
Crain, B. J., Tremblay, R. L., and Ferguson, J. M. (2019). Sheltered from the storm? Population viability analysis of a rare endemic under periodic catastrophe regimes. Popul. Ecol. 61, 1–19. doi: 10.1002/1438-390X.1002
Crone, E. E., Ellis, M. M., Morris, W. F., Stanley, A., Bell, T., Bierzychudek, P., et al. (2013). Ability of matrix models to explain the past and predict the future of plant populations. Conserv. Biol. 27, 968–978. doi: 10.1111/cobi.12049
Crone, E. E., Menges, E. S., Ellis, M. M., Bell, T., Bierzychudek, P., Ehrlén, J., et al. (2011). How do plant ecologists use matrix population models? Ecol. Lett. 14, 1–8. doi: 10.1111/j.1461-0248.2010.01540.x
de Kroon, H., van Groenendael, J., and Ehrlén, J. (2000). Elasticities: a review of methods and model limitations. Ecology 81, 607–618.
Dostálek, T., and Münzbergová, Z. (2013). Comparative population biology of critically endangered Dracocephalum austriacum (Lamiaceae) in two distant regions. Folia Geobot 48, 75–93. doi: 10.1007/s12224-012-9132-2
Dostálek, T., Rokaya, M. B., and Münzbergová, Z. (2018). Altitude, habitat type and herbivore damage interact in their effects on plant population dynamics. PLoS One 13:e0209149. doi: 10.1371/journal.pone.0209149
Emanuel, P. L., Shackleton, C. M., and Baxter, J. S. (2005). Modelling the sustainable harvest of Sclerocarya birrea subsp. caffra fruits in the South African lowveld. For. Ecol. Manag. 214, 91–103. doi: 10.1016/j.foreco.2005.03.066
Emeterio-Lara, A., García-Franco, J. G., Hernández-Apolinar, M., Toledo-Hernández, V. H., Valencia-Díaz, S., and Flores-Palacios, A. (2021). Is pseudobulb harvest a sustainable management strategy in wild orchid populations? An experiment with Laelia autumnalis. For. Ecol. Manag. 491:119205. doi: 10.1016/j.foreco.2021.119205
Fay, M. F. (2018). Orchid conservation: how can we meet the challenges in the twenty-first century? Bot. Stud. 59:16. doi: 10.1186/s40529-018-0232-z
Fréville, H., Colas, B., Riba, M., Caswell, H., Mignot, A., Imbert, E., et al. (2004). Spatial and temporal demographic variability in the endemic plant species Centaurea corymbosa (Asteraceae). Ecology 85, 694–703. doi: 10.1890/03-0119
Gamelon, M., Grøtan, V., Nilsson, A. L. K., Engen, S., Hurrell, J. W., Jerstad, K., et al. (2017). Interactions between demography and environmental effects are important determinants of population dynamics. Sci. Adv. 3:e1602298. doi: 10.1126/sciadv.1602298
García, M. B., Pico, F. X., and Ehrlén, J. (2008). Life span correlates with population dynamics in perennial herbaceous plants. Am. J. Bot. 95, 258–262. doi: 10.3732/ajb.95.2.258
García-González, A., Damon, A., Raventós, J., Riverón-Giró, F. B., Mújica, E., and Solís-Montero, L. (2017). Impact of different shade coffee management scenarios, on a population of Oncidium poikilostalix (Orchidaceae), in Soconusco, Chiapas, Mexico. Plant Ecol. Diver. 10, 185–196. doi: 10.1080/17550874.2017.1315840
Ghimire, S. K., Gimenez, O., Pradel, R., McKey, D., and Aumeeruddy-Thomas, Y. (2008). Demographic variation and population viability in a threatened Himalayan medicinal and aromatic herb Nardostachys grandiflora: matrix modelling of harvesting effects in two contrasting habitats. J. Appl. Ecol. 45, 41–51. doi: 10.1111/j.1365-2664.2007.01375.x
Gregg, K. B. (2011). Recovery from bud disappearance explains prolonged dormancy in Cleistes bifaria (Orchidaceae). Am. J. Bot. 98, 326–330. doi: 10.3732/ajb.1000262
Heinken-Šmídová, A., and Münzbergová, Z. (2012). Population dynamics of the endangered, long-lived perennial species, Ligularia sibirica. Folia Geobot 47, 193–214. doi: 10.1007/s12224-011-9116-7
Hinsley, A., de Boer, H. J., Fay, M. F., Gale, S. W., Gardiner, L. M., Gunasekara, R. S., et al. (2018). A review of the trade in orchids and its implications for conservation. Bot. J. Linnean Soc. 186, 435–455.
Hutchings, M. J. (2010). The population biology of the early spider orchid Ophrys sphegodes Mill. III. Demography over three decades: population biology of early spider orchid. J. Ecol. 98, 867–878. doi: 10.1111/j.1365-2745.2010.01661.x
Jongejans, E., and De Kroon, H. (2005). Space versus time variation in the population dynamics of three co-occurring perennial herbs. J. Ecol. 93, 681–692. doi: 10.1111/j.1365-2745.2005.01003.x
Jongejans, E., Jorritsma-Wienk, L. D., Becker, U., Dostál, P., Mildén, M., and De Kroon, H. (2010). Region versus site variation in the population dynamics of three short-lived perennials. J. Ecol. 98, 279–289. doi: 10.1111/j.1365-2745.2009.01612.x
Khare, C. P. (2007). Indian Medicinal Plants: An Illustrated Dictionary. Berlin: Springer Science+Business Media.
Khare, C. P. (2015). Ayurvedic Pharmacopoeial Plant Drugs: Expanded Therapeutics, 1edn Edn. Boca Raton, FL: CRC Press.
Klimas, C. A., Cropper, W. P., Kainer, K. A., and de Oliveira Wadt, L. H. (2012). Viability of combined timber and non-timber harvests for one species: a Carapa guianensis case study. Ecol. Modell. 246, 147–156. doi: 10.1016/j.ecolmodel.2012.07.022
Klimešová, J., Nobis, M. P., and Herben, T. (2016). Links between shoot and plant longevity and plant economics spectrum: environmental and demographic implications. Perspect. Plant Ecol. Evol. Syst. 22, 55–62. doi: 10.1016/j.ppees.2016.09.002
Kuznetsova, A., Brockhoff, P. B., and Christensen, R. H. B. (2017). lmerTest package: tests in linear mixed effects models. J. Stat. Soft. 82, 1–26. doi: 10.18637/jss.v082.i13
Lázaro-Zermeño, J. M., González-Espinosa, M., Mendoza, A., Martínez-Ramos, M., and Quintana-Ascencio, P. F. (2011). Individual growth, reproduction and population dynamics of Dioon merolae (Zamiaceae) under different leaf harvest histories in Central Chiapas. Mexico For. Ecol. Manag. 261, 427–439. doi: 10.1016/j.foreco.2010.10.028
Marrero-Gómez, M. V., Oostermeijer, J. G. B., Carqué-Álamo, E., and Bañares-Baudet, Á (2007). Population viability of the narrow endemic Helianthemum juliae (Cistaceae) in relation to climate variability. Biol. Conserv. 136, 552–562. doi: 10.1016/j.biocon.2007.01.010
MATLAB (2002). MATLAB® -The Language of Technical Computing Version 6.0.0.88. Portola Valley. CA: The MathWorks Inc.
Mondragón, D. (2009). Population viability analysis for Guarianthe aurantiaca, an ornamental epiphytic orchid harvested in Southeast México. Plant Species Biol. 24, 35–41. doi: 10.1111/j.1442-1984.2009.00230.x
Mondragón, D., Valverde, T., and Hernández-Apolinar, M. (2015). Population ecology of epiphytic angiosperms: a review. Trop. Ecol. 56, 1–39.
Mondragón Chaparro, D., and Ticktin, T. (2011). Demographic effects of harvesting epiphytic Bromeliads and an alternative approach to collection. Conserv. Biol. 25, 797–807. doi: 10.1111/j.1523-1739.2011.01691.x
Münzbergová, Z. (2005). Determinants of species rarity: population growth rates of species sharing the same habitat. Am. J. Bot. 92, 1987–1994. doi: 10.3732/ajb.92.12.1987
Münzbergová, Z. (2006). Effect of population size on the prospect of species survival. Folia Geobotanica 41, 137–150. doi: 10.1007/BF02806475
Münzbergová, Z. (2007). Population dynamics of diploid and hexaploid populations of a perennial herb. Ann.f Bot. 100, 1259–1270. doi: 10.1093/aob/mcm204
Münzbergová, Z. (2013). Comparative demography of two co-occurring Linum species with different distribution patterns. Plant Biol. J. 15, 963–970. doi: 10.1111/plb.12007
Münzbergová, Z., and Ehrlén, J. (2005). How best to collect demographic data for population viability analysis models. J. Appl. Ecol. 42, 1115–1120. doi: 10.1111/j.1365-2664.2005.01099.x
Nantel, P., Gagnon, D., and Nault, A. (1996). Population viability analysis of American Ginseng and wild Leek harvested in stochastic environments. Conserv. Biol. 10, 608–621. doi: 10.1046/j.1523-1739.1996.10020608.x
Nurfadilah, S. (2017). Population study of Peristylus goodyeroides (Orchidaceae) in five habitats and implication for its conservation. Biodiversitas 18, 1084–1091. doi: 10.13057/biodiv/d180328
Oostermeijer, J. G. B., Brugman, M. L., De Boer, E. R., and Nijs, H. C. M. D. (1996). Temporal and spatial variation in the demography of Gentiana pneumonanthe, a rare perennial herb. J. Ecol. 84, 153–166. doi: 10.2307/2261351
Orozco-Ibarrola, O., Solano, R., and Valverde, T. (2021). Sustainable harvesting and conservation of Laelia furfuracea, a rare epiphytic orchid from Oaxaca, Mexico. Biotropica 53, 142–151. doi: 10.1111/btp.12854
Pant, B., and Raskoti, B. B. (2013). Medicinal Orchids of Nepal. Kathmandu: Himalayan Map House Pvt. Ltd.
Paudel, H. R. (2020). The first record of the genus Tropidia Lindl. (Orchidaceae) from Nepal. Int. J. Appl. Sci. Biotechnol. Sci. 1, 153–157.
Pearce, N. R., and Cribb, P. J. (2002). Orchids of Bhutan: Including a record of plants from Sikkim and Darjeeling. Edinburgh: Royal Botanic Garden Edinburgh.
R Development Core Team (2020). R: A Language and Environment for Statistical Computing. Vienna: R Development Core Team.
Ramula, S., Knight, T. M., Burns, J. H., and Buckley, Y. M. (2008). General guidelines for invasive plant management based on comparative demography of invasive and native plant populations. J. Appl. Ecol. 45, 1124–1133. doi: 10.1111/j.1365-2664.2008.01502.x
Rasmussen, H. N., Dixon, K. W., Jersáková, J., and Těšitelová, T. (2015). Germination and seedling establishment in orchids: a complex of requirements. Ann. Bot. 116, 391–402. doi: 10.1093/aob/mcv087
Raventós, J., García-González, A., Riverón-Giró, F. B., and Damon, A. (2018). Comparison of transient and asymptotic perturbation analyses of three epiphytic orchid species growing in coffee plantations in Mexico: effect on conservation decisions. Plant Ecol. Diver. 11, 133–145. doi: 10.1080/17550874.2018.1444110
Reinke, B. A., Miller, D. A. W., and Janzen, F. J. (2019). What have long-term field studies taught us about population dynamics? Annu. Rev. Ecol. Evol. Syst. 50, 261–278. doi: 10.1146/annurev-ecolsys-110218-024717
Rock, J. H., Beckage, B., and Gross, L. J. (2004). Population recovery following differential harvesting of Allium tricoccum Ait. In the southern Appalachians. Biol. Conserv. 116, 227–234. doi: 10.1016/S0006-3207(03)00193-9
Rokaya, M. B., Münzbergová, Z., and Dostálek, T. (2017). Sustainable harvesting strategy of medicinal plant species in Nepal – results of a six-year study. Folia Geobotanica 52, 239–252. doi: 10.1007/s12224-017-9287-y
Rokaya, M. B., Raskoti, B. B., Timsina, B., and Münzbergová, Z. (2013). An annotated checklist of the orchids of Nepal. Nordic J. Bot. 31, 511–550. doi: 10.1111/j.1756-1051.2013.01230.x
Rydgren, K., Økland, R. H., Picó, F. X., and de Kroon, H. (2007). Moss species benefits from breakdown of cyclic rodent dynamics in boreal forests. Ecology 88, 2320–2329. doi: 10.1890/06-1634.1
Schmidt, I. B., Mandle, L., Ticktin, T., and Gaoue, O. G. (2011). What do matrix population models reveal about the sustainability of non-timber forest product harvest? J. Appl. Ecol. 48, 815–826. doi: 10.1111/j.1365-2664.2011.01999.x
Schmidt, I. B., and Ticktin, T. (2012). When lessons from population models and local ecological knowledge coincide – Effects of flower stalk harvesting in the Brazilian savanna. Biol. Conserv. 152, 187–195. doi: 10.1016/j.biocon.2012.03.018
Shefferson, R. P. (2002). “Dormancy and survival in rare, terrestrial orchids,” in Trends and Fluctuations and Underlying Mechanisms in Terrestrial Orchid Populations, eds P. Kindlmann, J. H. Willems, and D. F. Whigham (Leiden: Backhuys Publishers), 53–64.
Shefferson, R. P., Jacquemyn, H., Kull, T., and Hutchings, M. J. (2020). The demography of terrestrial orchids: life history, population dynamics and conservation. Bot. J. Linnean Soc. 192, 315–332. doi: 10.1093/botlinnean/boz084
Singh, S. K., Agrawala, D. K., Jalal, J. S., Dash, S. S., Mao, A. A., and Singh, P. (2019). Orchids of India: A Handbook. Dehra Dun: M/s Bishen Singh Mahendra Pal Singh.
Sonkoly, J., Vojtkó, A. E., Tökölyi, J., Török, P., Sramkó, G., Illyés, Z., et al. (2016). Higher seed number compensates for lower fruit set in deceptive orchids. J. Ecol. 104, 343–351. doi: 10.1111/1365-2745.12511
Subedi, A., Kunwar, B., Choi, Y., Dai, Y., van Andel, T., Chaudhary, R. P., et al. (2013). Collection and trade of wild-harvested orchids in Nepal. J. Ethnobiol. Ethnomed. 9:64. doi: 10.1186/1746-4269-9-64
Teoh, E. S. (2016). Medicinal Orchids of Asia. Berlin: Springer International Publishing, doi: 10.1007/978-3-319-24274-3
Teoh, E. S. (2019). Orchids as Aphrodisiac, Medicine or Food. Berlin: Springer International Publishing, doi: 10.1007/978-3-030-18255-7
Ticktin, T. (2004). The ecological implications of harvesting non-timber forest products. J. Appl. Ecol. 41, 11–21. doi: 10.1111/j.1365-2664.2004.00859.x
Ticktin, T., Mondragón, D., Lopez-Toledo, L., Dutra-Elliott, D., Aguirre-León, E., and Hernández-Apolinar, M. (2019). Synthesis of wild orchid trade and demography provides new insight on conservation strategies. Conserv. Lett. 13:e12697. doi: 10.1111/conl.12697
Tremblay, R. L., Ackerman, J. D., Zimmerman, J. K., and Calvo, R. N. (2004). Variation in sexual reproduction in orchids and its evolutionary consequences: a spasmodic journey to diversification. Biol. J. Linnean Soc. 84, 1–54. doi: 10.1111/j.1095-8312.2004.00400.x
Tremblay, R. L., Tyre, A. J., Pérez, M.-E., and Ackerman, J. D. (2021). Population projections from holey matrices: using prior information to estimate rare transition events. Ecol. Modell. 447:109526. doi: 10.1016/j.ecolmodel.2021.109526
Vaidya, B. N. (2019). “Nepal: a global hostspot for medicinal orchids,” in Medicinal Plants: From Farm to Pharmacy, eds N. Joshee, S. A. Dhekney, and P. Parajuli (Berlin: Springer Nature), 35–80. doi: 10.1007/978-3-030-31269-5_3
Keywords: elasticity, Himalayan region, Orchidaceae, simulation, sustainable harvesting
Citation: Timsina B, Kindlmann P, Münzbergová Z and Rokaya MB (2021) Six-Year Demographic Study of the Terrestrial Orchid, Crepidium acuminatum: Implications for Conservation. Front. Ecol. Evol. 9:676993. doi: 10.3389/fevo.2021.676993
Received: 06 March 2021; Accepted: 14 September 2021;
Published: 05 October 2021.
Edited by:
Su Wang, Beijing Academy of Agricultural and Forestry Sciences, ChinaReviewed by:
Raymond L. Tremblay, University of Puerto Rico, Puerto RicoCopyright © 2021 Timsina, Kindlmann, Münzbergová and Rokaya. This is an open-access article distributed under the terms of the Creative Commons Attribution License (CC BY). The use, distribution or reproduction in other forums is permitted, provided the original author(s) and the copyright owner(s) are credited and that the original publication in this journal is cited, in accordance with accepted academic practice. No use, distribution or reproduction is permitted which does not comply with these terms.
*Correspondence: Binu Timsina, YmludS50aW1zaW5hQGdtYWlsLmNvbQ==; Maan B. Rokaya, cm9rYXlhbWFhbkBnbWFpbC5jb20=
Disclaimer: All claims expressed in this article are solely those of the authors and do not necessarily represent those of their affiliated organizations, or those of the publisher, the editors and the reviewers. Any product that may be evaluated in this article or claim that may be made by its manufacturer is not guaranteed or endorsed by the publisher.
Research integrity at Frontiers
Learn more about the work of our research integrity team to safeguard the quality of each article we publish.