- 1Department of Wildlife Ecology and Conservation, University of Florida, Gainesville, FL, United States
- 2Environmental Change Institute, School of Geography and the Environment, University of Oxford, Oxford, United Kingdom
- 3Department of Botany and Zoology, Stellenbosch University, Matieland, South Africa
- 4Department of Biological Sciences, University of Eswatini, Kwaluseni, Eswatini
- 5Mammal Research Institute, Department of Zoology and Entomology, University of Pretoria, Hatfield, South Africa
In savannas across the planet, encroaching woody plants are altering ecosystem functions and reshaping communities. Seed predation by rodents may serve to slow the encroachment of woody plants in grasslands and savannas. Our goals for this study were to determine if rodents in an African savanna selectively removed seeds of an encroaching plant and if foraging activity was influenced by the local vegetation structure or by the landscape context. From trials with two species of seeds (encroacher = Dichrostachys cinerea, non-encroaching overstory tree = Senegalia nigrescens) at 64 seed stations, we recorded 1,065 foraging events by seven species of granivorous rodents. We found a strong positive relationship between rodent activity and the number of seeds removed during trials. Foraging events were dominated by rodent seed predators, with <10.6% of events involving a rodent with the potential for secondary dispersal. Rodents selectively removed the seeds of the encroaching species, removing 32.6% more D. cinerea seeds compared to S. nigrescens. Additionally, rodent activity and the number of seeds removed increased at sites with more grass biomass. Our results suggest a potential mechanistic role for rodents in mitigating the spread of woody plants in grass dominated savannas.
Introduction
Globally, savannas are experiencing an increase in woody vegetation, known as woody encroachment (Stevens et al., 2016a,b). Savannas are characterized by a coexistence of trees and grasses, with woody vegetation historically limited by rainfall, fire, herbivory, and competition with grasses (Bond, 2008; Lehmann et al., 2014; Staver and Bond, 2014). However, in the last century, tropical savannas in Africa, South America, and Australia (Stevens et al., 2016b) have seen an increased in abundance of woody plants due to poorly understood and interacting factors, such as atmospheric carbon dioxide, fire regimes, and overgrazing (Sala and Maestre, 2014; Archer et al., 2017). Encroachment, often dominated by a few native plant species, can alter vegetation structure to shade out vegetation in the grassy layer (Scholes, 2003; Charles-Dominique et al., 2018). This structural and compositional change can alter the ecosystem functions and services that savannas provide, such as altering carbon storage, decreasing water availability, reducing biological diversity, and disrupting socioeconomic conditions through reduced grazing (Huxman et al., 2005; Soto-Shoender et al., 2018). To maintain savannas and their beneficial services, it is important to understand factors that can limit the establishment of woody encroachers, particularly at the seed germination and establishment stage.
One under-studied process that can limit woody plants during the seed germination and establishment stage is post-dispersal seed predation (Ostfeld et al., 1997; Vaz Ferreira et al., 2010). Post-dispersal seed predation can influence the colonization, distribution, and composition of plant communities (Hulme, 1997; Lindquist and Carroll, 2004; Zamora and Matías, 2014). Compositional changes can occur when predators selectively consume seeds of certain species over others (Meiners and Stiles, 1997; Orrock et al., 2006). One group of effective seed predators that can reshape the structure of vegetation are granivorous rodents (Davidson et al., 1984; Brown and Heske, 1990; Hulme, 1993). Rodents can mitigate populations of invasive and exotic plants by predating their seeds (Parker et al., 2006; Pearson et al., 2012) and may have the potential to slow woody encroachment in savannas (Busch et al., 2011; Stanton et al., 2018). However, there is minimal information on how rodents could limit the establishment and spread of encroaching woody plants (Busch et al., 2011; Bergstrom, 2013; Gordon et al., 2016).
Seed consumption by some rodents, other mammals, birds, and insects, can increase the viability of seeds and disperse them to new areas (Schupp, 1993; Miller, 1994b; Crawley, 2000). However, many non-caching granivorous rodents are effective seed predators that destroy the seed upon consumption (Ostfeld et al., 1997; Honek et al., 2009). The extent of rodents’ predation and dispersal of seeds is likely a function of environmental conditions. At a local scale, rodents’ movements are shaped by vegetation structure, such as grass biomass and shrub cover, which provide cover from predators (Hulme, 1993; Monadjem, 1999a; Loggins et al., 2019b). While less pronounced, rodent activity may also be influenced by different types and configurations of surrounding landscapes (Nupp and Swihart, 2000; Orrock and Damschen, 2005; Ness and Morin, 2008).
Our goals for this study were to determine if rodents in an African savanna selectively removed different woody seeds and varied their activity with changes in local vegetation structure or landscape context. Working in a shrub encroached savanna embedded within a complex landscape matrix, we predicted rodents would selectively remove seeds of a woody encroacher (Dichrostachys cinerea). We predicted that the nutritious D. cinerea herbivore-dispersed seeds would also be highly palatable for rodents (Irie and Tsuyuzaki, 2011; Xiao and Zhang, 2016) and selected (i.e., removed at a higher rate) over a non-herbivore dispersed tree species (Senegalia nigrescens). Additionally, we predicted that fine-scale habitat conditions would have a greater influence on seed removal than the surrounding landscape because of rodents’ strong behavioral responses to local vegetation structure (Lima and Dill, 1990; Loggins et al., 2019a).
Materials and Methods
Study Area
We conducted our study in the subtropical savannas of northeastern Eswatini. This region falls within the Maputaland-Pondoland-Albany biodiversity hotspot, recognized for its high endemism and biodiversity (Smith et al., 2008). A variety of land uses in the region creates a mosaic landscape of irrigated commercial monocultures and rain-fed subsistence agriculture, grazing lands, and protected areas (Bailey et al., 2016; Reynolds et al., 2018).
The native savanna vegetation in this area is characterized by a continuous grass layer dominated by Themeda triandra and Panicum maximum and interspersed with woody trees and shrubs. The dominant canopy tree is S. nigrescens and the shrub layer is dominated by D. cinerea. The woody shrub cover in the region has increased steadily over the last 70 years, from 2% to currently >40% (Roques et al., 2001; Sirami and Monadjem, 2011; McCleery et al., 2018; Stanton et al., 2020). The region has mild, dry winters and hot, wet summers. We conducted our study during the winter, June–July 2018, when average temperatures range from 8 to 26°C and the region receives approximately 0–50 mm of its average 600 mm of annual rainfall (Goudie and Price-Williams, 1983).
We conducted our experiments within the Mbuluzi Game Reserve, Mlawula Nature Reserve, Hlane Royal National Park, and the Inyoni Yami Swaziland Irrigation Scheme (IYSIS) cattle ranch. These savannas were embedded within a land-use matrix consisting of protected natural areas, cattle ranches, subsistence agriculture, and intensive sugarcane plantations (Monadjem and Garcelon, 2005; LaScaleia et al., 2018). Our study sites host a variety of rodents with different feeding and habitat preferences. The most common species at our sites (Mastomys natalensis, Lemniscomys rosalia, Aethomys ineptus, and Micaelamys namaquensis) have variable diets that include seeds (Field, 1975; Kerley and Erasmus, 1991;Monadjem, 1997a, 1998; Chimimba and Linzey, 2008; Hagenah et al., 2009; Mulungu et al., 2011). We have found no evidence suggesting these widespread and well-studied rodents contribute to secondary seed dispersal (Miller, 1994a; Skinner and Chimimba, 2005; Scholtz, 2008; Happold, 2013; White and Midgley, 2021). However, three less common granivorous species (Saccostomus campestris, Steatomys pratensis, and Mus minutoides) on our sites are known to eat seeds and cache food in their burrows or nests (Hanney, 1965; Ellison, 1993; Hoole et al., 2017) and may facilitate seed dispersal. However, caching by M. minutoides has only recently been demonstrated in a laboratory setting and S. campestris and S. pratensis often reduce their activity during the austral winter (Kern, 1981; Korn, 1987; Monadjem, 1999b), which is when we conducted this study because seeds from the dominant woody plants are most abundant (Donaldson, 1993; Hoffman, 2006).
Site Selection and Landscape Metrics
To understand the influence of the surrounding landscape on the interaction between rodents and seed predation, we created a land-cover map from Google Earth Engine which included savanna, agriculture, homestead, and open water (Reynolds et al., 2018; Figure 1). We derived a value for each site for compositional (the type and diversity of land cover) and configurational (the shape and arrangement of land cover) landscape heterogeneity (Gustafson, 1998). To derive these measures, we used the raster and SDMTools packages in R statistical software V 3.5.0 to implement a moving-window analysis and identify gradients in landscape composition and configuration based on variations in land cover (Zhang et al., 2013; Tolessa et al., 2016, R Core Team, 2018). To capture areas of savanna with a variety of surrounding landscapes, we set a window with a 2 km radius and centered sampling landscapes around cells surrounded by at least a 500 × 500 m buffer. Within the 2 km buffer, we calculated two measures of compositional heterogeneity (Shannon diversity index of land cover types and land cover richness) and three measures of configuration heterogeneity (total length of edge between land cover classes, total number of patches, and patch cohesion and landscape division) (Fahrig et al., 2011; Supplementary Table 1).
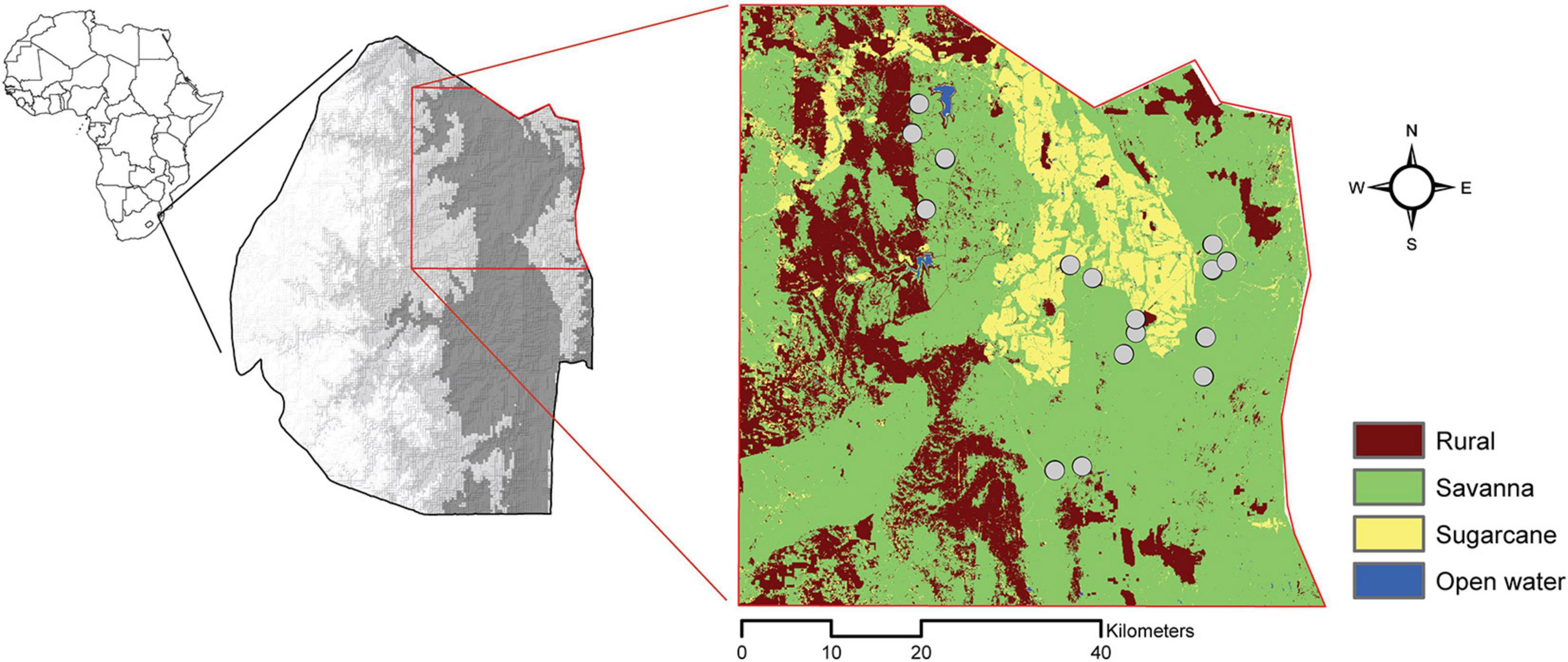
Figure 1. Map detailing the country of Eswatini (gray scale depicts elevation; darker gray is lower elevation) and our study area. Our study area is delineated in red, and our sampling sites (gray circles) are overlayed on our classification of land-cover types.
We used principal components analysis (PCA) from the psych package to create two orthogonal components of landscape heterogeneity, representing compositional, and configurational heterogeneity (Revelle, 2014). We ranked cells based on their PCA value for compositional and configurational heterogeneity. Using stratified sampling, we then selected 16 sampling landscapes across gradients of compositional and configurational heterogeneity.
We scored sites as low (<33%), medium (34–66%), or high (>67%) compositional and configurational heterogeneity. We stratified the 16 sites based on the following classifications: high compositional and high configurational heterogeneity (n = 3), high compositional and medium configurational heterogeneity (n = 2), high compositional and low configurational heterogeneity (n = 2), medium compositional and medium configurational heterogeneity (n = 1), medium compositional and low configurational heterogeneity (n = 2), low compositional and high configurational heterogeneity (n = 2), low compositional and medium configurational heterogeneity (n = 2), and low compositional and low configurational heterogeneity (n = 2).
Vegetation Structure
Our sampling sites were located within relatively open savanna habitats (woody cover 25–40%). To quantify the vegetation structure of the local environment (0.25-ha), we sampled three 50 m transects at each site. We ran two parallel transects at 10 m distance from the center of the site, and a third perpendicular transect through the center point. We measured grass biomass with a disc pasture meter (DPM) every 5 m on each transect (Bransby and Tainton, 1977). We averaged the measures and used a previously calibrated estimate to generate an estimate of biomass in kg/ha (Zambatis et al., 2006). We measured the average canopy cover at each site by taking spherical densiometer readings at every 5 m along each of the three transects and we estimated shrub cover (woody cover <5 m) using the line intercept method on each transect (Canfield, 1941).
Foraging Trials
We conducted foraging trials with seeds from the two dominant woody species in our study area, S. nigrescens and D. cinerea (family Fabaceae). They are both widespread in southern African savannas (Palgrave and Palgrave, 2015). D. cinerea is a common woody encroacher that relies on ingestion by non-rodent herbivores (e.g., ungulates) for both dispersal and dormancy-breaking (Van Staden et al., 1994; Miller, 1995; Dudley, 1999; Tjelele et al., 2012, 2015). The seeds of D. cinerea are round and ≈ 4 mm × 5 mm (Supplementary Figure 1). The pods are nutritious and are eaten by large herbivores. S. nigrescens is a canopy tree with disc-shaped seeds that form in a pod and are dispersed through ballistic dispersal. The seeds of S. nigrescens are ≈ 10 mm × 10 mm and flat (Supplementary Figure 1). These larger seeds do not require herbivore-assisted dispersal to break dormancy and are damaged if consumed (Miller, 1994b, 1995).
At each sampling site (50 × 50 m plot, 0.25 ha) we established four seed stations around the center of the site. We placed the seed stations (30 cm diameter tray) halfway under shrubs to increase foraging rates (Loggins et al., 2019b). Prior to trials, we pre-baited trays for 24 h with oats and peanut butter. Then we randomly assigned two seed stations to receive S. nigrescens seeds (n = 40) and two to receive D. cinerea seeds (n = 60). We obtained our seeds from Silverhill Seeds and Books1. We used 50% more D. cinerea seeds than S. nigrescens seeds to account for the former’s smaller mass and volume. We ran each trial for 24 h and then collected and recorded the number of intact seeds remaining. We classified seeds as remaining seeds if they were scarred or damaged but had an intact cotyledon that could potentially germinate (Bell and van Staden, 1993). The small and hard seeds used in this study often shatter with damage to the cotyledon; thus, to be conservative we considered seeds with >25% damage to the cotyledon as removed (Barnes, 2001). For the next 24-h period, we switched seed species at each station and ran the trial again. Finding no evidence that the common seed predators (M. natalensis, L. rosalia, A. ineptus, and M. namaquensis) contribute to secondary seed dispersal (Miller, 1994a; Skinner and Chimimba, 2005; Scholtz, 2008; Happold, 2013; White and Midgley, 2021), we assumed the seeds removed by these species were predated. Alternatively, we acknowledged that foraging by the less common M. minutoides, S. campestris, and S. pratensis may have led to secondary dispersal.
Rodent Activity
To link seed predation to rodent activity, we monitored each seed station with a short-focused camera [Spartan, model SR1-IR(S100), Norcross, GA, United States]. We placed the camera 55 cm above each seed station facing downwards (Supplementary Figure 2). Seed trays were marked with a scale bar to aid in identification of rodents using tail and body length (McCleery et al., 2014). We set the motion-sensitive camera to photo, three bursts, with a 5 s trigger interval. To calculate the length of foraging bout we measured the duration of time each individual rodent first and last appeared on camera, with new bouts starting after 20 min of non-detection (Loggins et al., 2019b). When multiple individuals of the same species appeared on camera simultaneously, we noted a foraging bout for the maximum number of individuals seen in the frame. We grouped the ecologically similar A. ineptus and M. namaquensis as a single species due to the difficulty of distinguishing them on camera; however, the latter species is rarely captured away from rocky outcrops in Eswatini (Monadjem, 1997b) and was therefore not likely to be encountered in this study. All methods were approved by the University of Florida’s Institutional Animal Care and Use Committee (#201509045).
Statistical Analyses
Seed Selection
To determine if rodents selected one seed species over the other, we modeled the proportion of seeds removed during each trial as a function of the species of seed. We used proportions to account for the different number of seeds used for each species. We used a mixed effects model with each seed station as a random effect and fitted to a binomial distribution using the lme4 package for R (Bates et al., 2015). We considered the proportion of seeds removed to be significantly different if the 95% confidence intervals (CI) of β estimate for seed species did not include zero.
Variation in Seed Removal
To explain variation in seed removal for each species separately, we used the raw count of the number of seeds remaining at each tray. For each species, we considered variation as a function of factors at two different scales, the local scale (vegetation structure at the 0.25 ha scale) and the landscape scale (land use composition and configuration at the 2 km scale). We developed seven a priori models to explain differences in the number D. cinerea and S. nigrescens seeds remaining. We included models with only one fixed variable (canopy cover, shrub cover, grass biomass, compositional heterogeneity, and configurational heterogeneity), as well as global and null models (Table 1). We fitted generalized linear mixed models using a generalized Poisson distribution and site as a random effect using the glmmTMB package in R (Brooks et al., 2017). We evaluated model fit using Akaike’s information criterion (AICc) corrected for small sample size. We considered models with AICc scores lower than the null model and within four AICc units of the best model to be competing models (Burnham and Anderson, 2002). We considered parameters from competing models to be significant if their 95% CIs did not include zero.
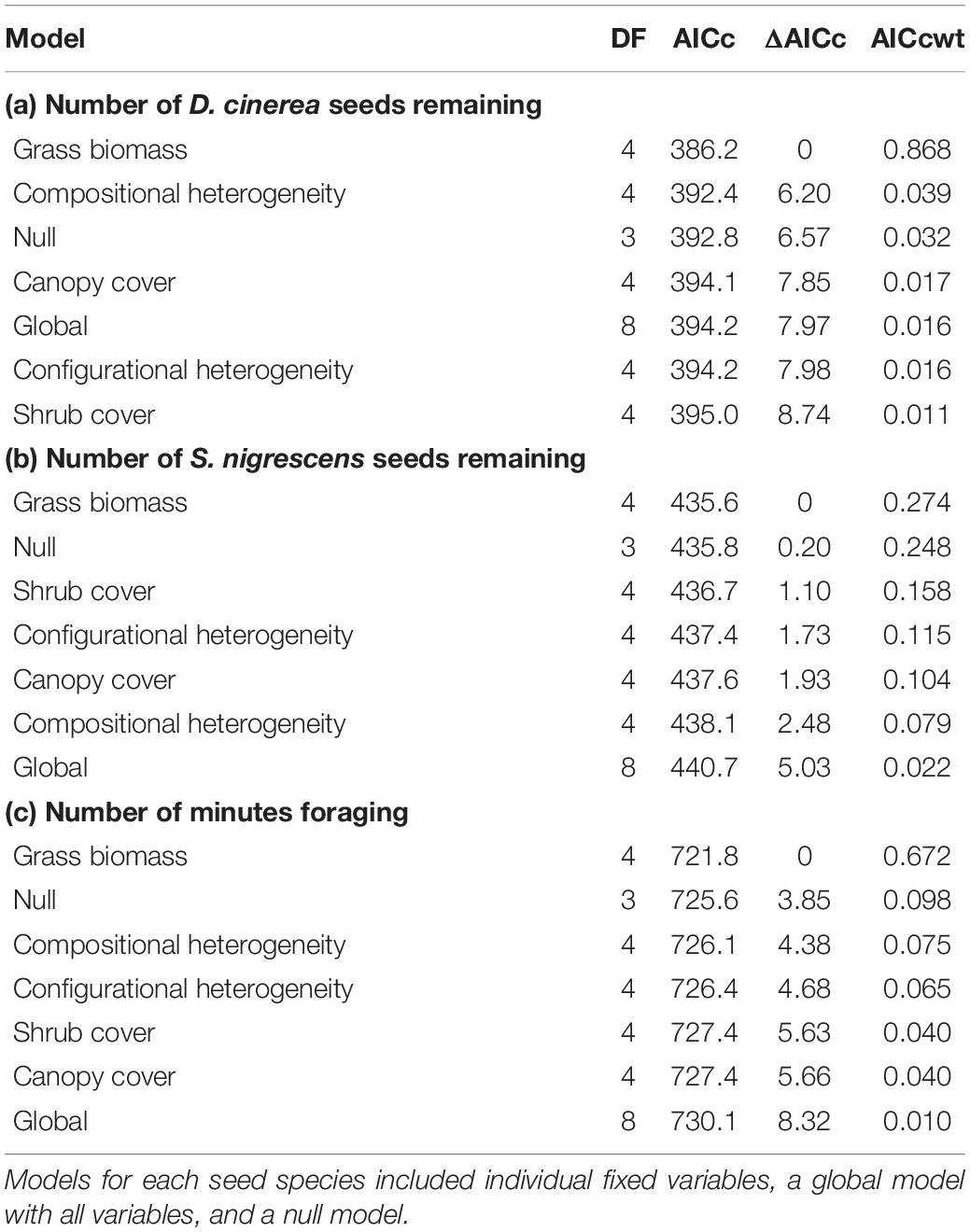
Table 1. Degrees of freedom (DF), Akaike’s information criterion corrected for small sample size (AICc), difference in AIC scores (ΔAICc), and AICc weight (AICcwt) of models to explain differences in the numbers of Dichrostachys cinerea seeds remaining at seed trays (a), the number of Senegalia nigrescens seeds remaining at seed trays (b) and the total time (i.e., number of minutes) rodents foraged at each seed station (c).
Rodent Activity
To link rodent activity to overall seed removal (i.e., both species), we related the proportion of seeds removed during each trial to the total number of minutes that rodents foraged at each tray. Using both species, we modeled the proportion of seeds removed during each trial as a function of rodent activity with a mixed effects model fitted to a binomial distribution with seed station as random effect. In addition to examining 95% CIs, we evaluated the strength of the relationship by calculating the marginal pseudo-R2 using the sjstats package (Lüdecke, 2021). Next, to determine if activity varied as a function of the species of seed, we modeled the foraging activity during each trial (i.e., total number of minutes of rodent activity). We correlated these count data with the seed species used during the trial using a generalized Poisson distribution and seed station as the random effect. Finally, to determine if rodent activity varied with environmental factors in a manner that reflected variation in measures of seed removal, we fitted generalized linear mixed models to rodent activity using a generalized Poisson distribution and site as a random effect to the same seven models (Table 1) and assessed model parsimony and significance as described above.
Results
We recorded 1,065 foraging events by seven species of granivorous rodents during seed experiments that were conducted from June 23–July 26, 2018 (Supplementary Table 2). The rodents most frequently detected at the seed stations were the seed predators M. natalensis (39.0%, n = 415), A. ineptus/M. namaquensis (25.4%, n = 270), and L. rosalia (25.1%, n = 267). Rodents with the potential for secondary seed dispersal accounted for only 10.6% of the foraging events. The diminutive M. minutoides accounted for 10.1% (n = 108) of the activity and we only recorded five foraging events by S. pratensis (0.3%, n = 3), and S. campestris (0.2%, n = 2). Of the 64 seed stations used in the experiment, one had no sign of seed removal or rodent activity and two were incorrectly set up; hence, all three were removed from further analysis (n = 61).
Comparing the proportion of seeds removed by species, we found that rodents removed a greater proportion of D. cinerea than S. nigrescens (β = −1.30, 95% CI −2.52 to −0.26, reference = D. cinerea). Model predicted estimates suggested that rodents removed 32.6% more D. cinerea seeds (x̄ = 0.87, SE = 0.06) than S. nigrescens seeds (x̄ = 0.66, SE = 0.10).
Examining the influence of the surrounding environment on the number of seeds removed, for both seed species, we found a model with only the fixed effect of grass biomass was the best model with no competing models (Table 1). Grass biomass had a significant negative relationship with the number of D. cinerea seeds remaining in trays (β = −0.76, 95% CI −1.26 to −0.26), where the number of D. cinerea seeds remaining decreased from 31 to 1 as grass biomass increased from a minimal 1,000 with a maximum of 4,500 kg/ha (Figure 2). The relationship between grass biomass and the number of S. nigrescens remaining was not significant (β = −0.478, 95% CI −1.07 to 0.12), suggesting a weaker relationship. We found little evidence that seed removal was affected by the broader landscape context (i.e., composition and configuration; Table 1).
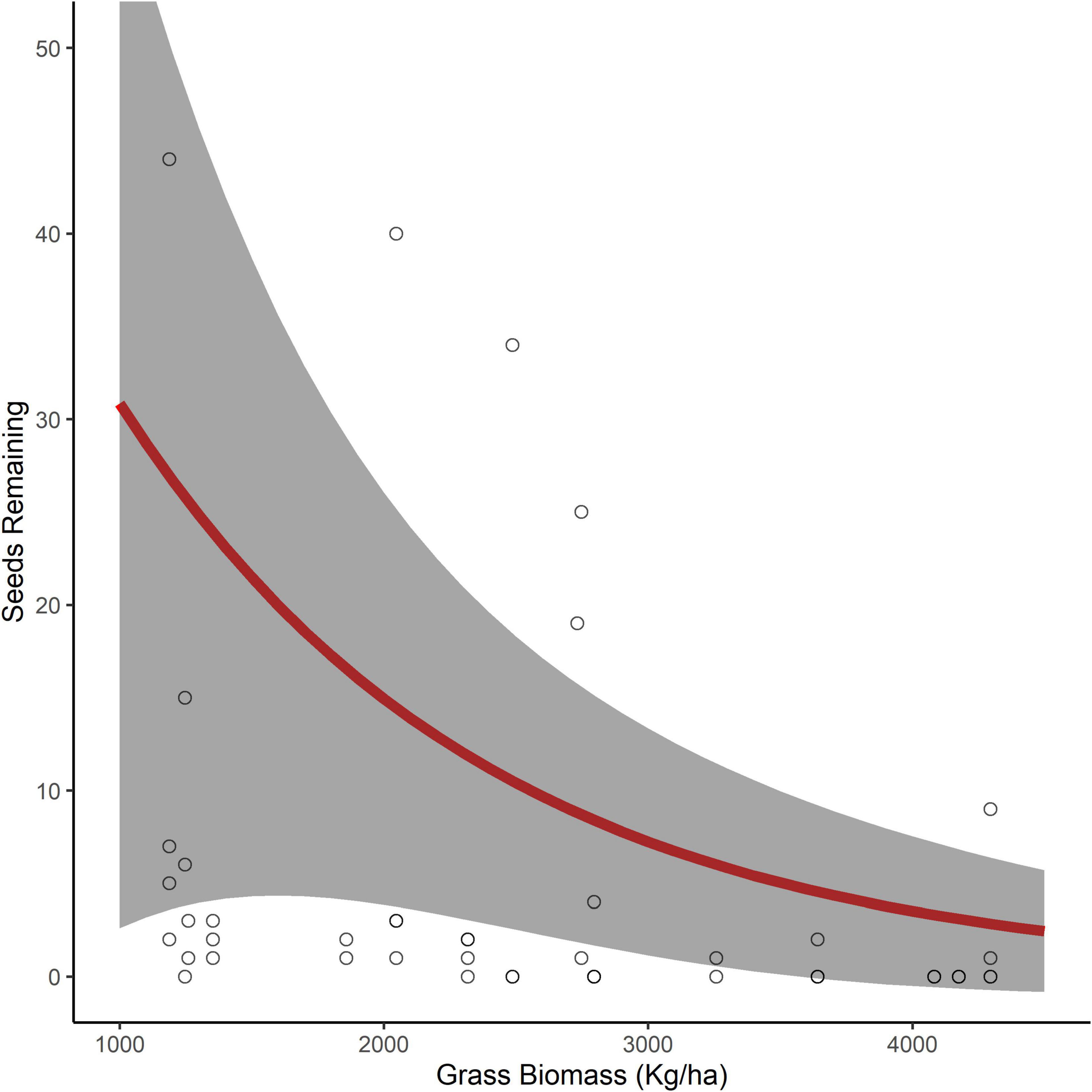
Figure 2. The predicted number of Dichrostachys cinerea seeds remaining in seed trays (95% confidence interval in gray) as a function of grass biomass (kg/ha) in the surrounding environment (0.25 ha).
Of the 61 seed stations used to understand seed removal, six had camera failures. Using the seed stations with operational cameras (n = 55), we found a strong positive relationship between the proportion of seeds removed and rodent activity (β = 3.19, 95% CI 1.75–4.65, pseudo R2 = 0.76). However, we found no indication that activity varied with the seed species used during the trial (β = 1.15, 95% CI −0.24 to 0.19, reference = D. cinerea). Similar to our investigation of seed removal, we found the best model, with no competing models, to explain variation in rodent activity was a model that included only the fixed effect of grass biomass (Table 1). Rodents spent more time foraging in areas with more grass biomass (β = 0.46, 95% CI 0.13–0.80). The total number of minutes increased from approximately 96 to 450 over the range of grass biomass recorded at our sites (Figure 3).
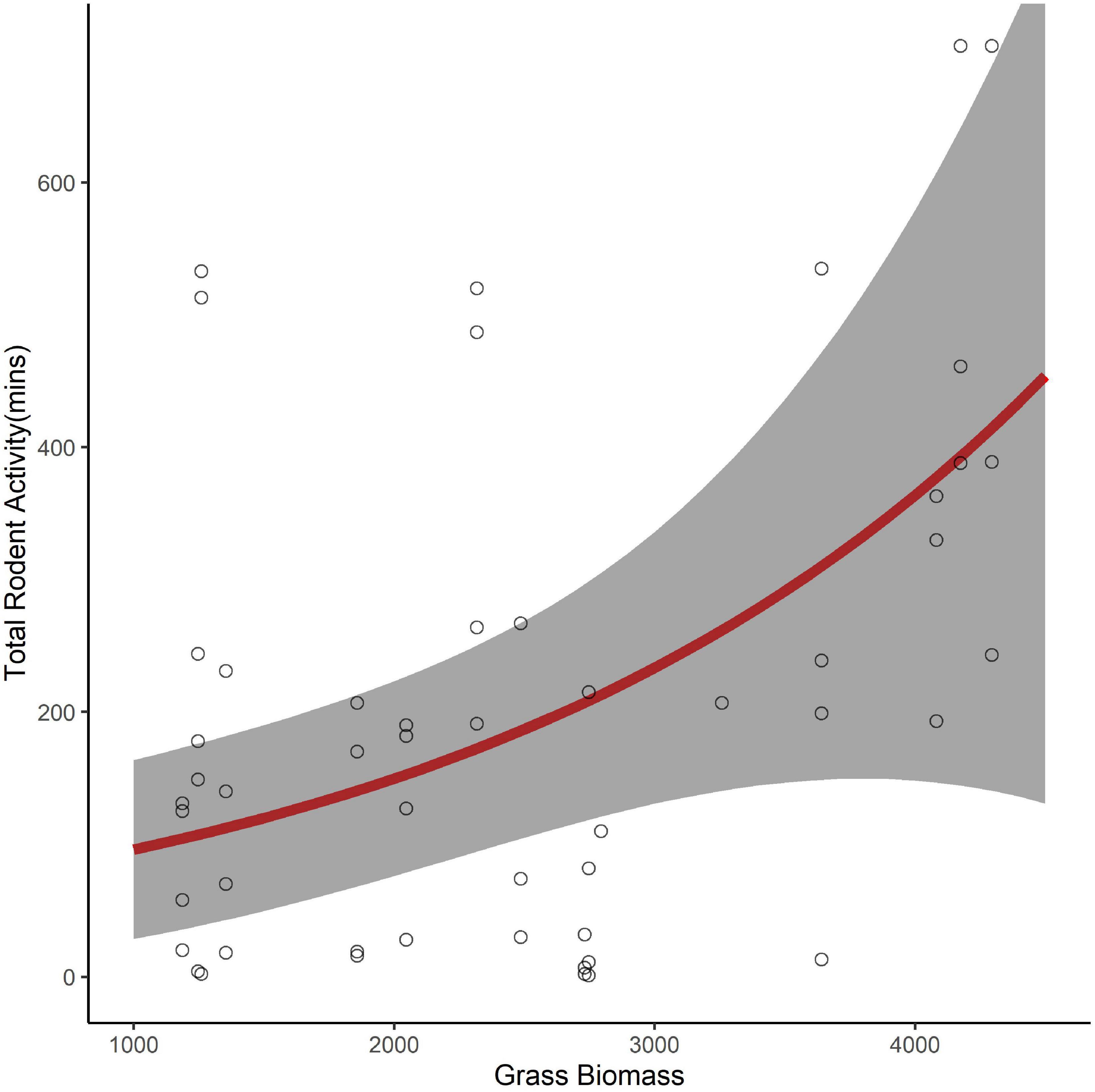
Figure 3. The predicted number of minutes that rodents foraged at seed trays (95% confidence interval in gray) as a function of grass biomass (kg/ha) in the surrounding environment (0.25 ha).
Discussion
We found a strong linkage between the removal of seeds and rodent activity, suggesting a potentially important role for rodents in shaping the composition and structure of woody vegetation in savannas. With only a small percentage of rodent activity from rodents with the known ability to cache seeds, we assumed the majority of rodent-seed interactions ended with seed predation. This was particularly true for rodents’ interactions with the seeds of the woody encroaching shrub D. cinerea. Despite spending similar amounts of time with both seed species, rodents removed 33% more of the seeds from D. cinerea.
Rodents’ selection of D. cinerea may be based on seed properties (e.g., morphology, nutrition, and palatability). The larger S. nigrescens seeds may provide rodents with a greater volume of food, but D. cinerea likely has a higher nutritional value (Janzen, 1984; Tiffney, 2004). This difference has been documented in the seed pods and may extend to the seeds (Tsopito and Adogla-Bessa, 1998; Aganga and Motshewa, 2007). Additionally, optimal foraging theory predicts that animals select seeds that provide the most nutritional gain for the least amount of energy expended (Charnov, 1976; Brown et al., 1999). The larger S. nigrescens seeds may require more energy to handle or increase the rodent’s vigilance behavior during foraging (Quenette, 1990). Accordingly, rodents may have selected the smaller seeds of D. cinerea to consume food with minimal effort while remaining vigilant.
We also found that the number of seeds removed by rodents and the amount of time rodents spent foraging increased with increases in grass biomass (Figures 2, 3). This supports our prediction that rodents are more strongly influenced by localized vegetation than the surrounding landscape. Thick grass cover is likely to provide rodents with ample food resources and reduce their perception of fear (Banasiak and Shrader, 2016). These findings are consistent with the “landscape of fear” framework (Laundré et al., 2014), where animals alter their movements and behaviors to minimize predation risk while maximizing benefits (e.g., foraging on seeds) (Lima and Dill, 1990; Brown, 1999; Bleicher, 2017).
In savannas, grasses and shrubs compete for resources (e.g., water and light) and areas with a thick grass layer have lower rates of seedling survival, reducing the chances of shrub establishment (Köchy and Wilson, 2000; Rinella et al., 2015; Pierce et al., 2018). Additionally, rodent predation of seeds of encroaching shrubs in areas with thick grass may create a feedback loop, where high grass biomass limits shrub establishment and facilitates rodent seed predation which helps to maintain the dominance of grassy vegetation. This feedback loop may be more pronounced for plants that are herbivore-dispersed such as D. cinerea, which rodents appear to select. Nonetheless, the role of rodents may be substantially reduced if the system switches from an open grassy savanna to an encroached savanna with minimal grassy cover.
Through the removal of seeds, rodents can decrease seedling recruitment (Ostfeld et al., 1997; Nuñez et al., 2008; Yu et al., 2014) and the density of adult plants (Maron and Kauffman, 2006; Larios et al., 2017), altering composition (Louda, 1982; Pearson et al., 2012, 2013) and shaping vegetation (Brown and Heske, 1990; Edwards and Crawley, 1999; Moorhead, 2017). In our savanna system, rodents have the potential to reduce the recruitment of woody encroaching plants; however, we did not consider the role of other herbivores. The nutritious seeds and seed pods of woody encroachers are often consumed and deposited in the dung of non-rodent herbivores, where they can germinate rapidly (Campos-Arceiz and Blake, 2011). This mechanism of dispersal may protect seeds from rodent predation and enhance secondary dispersal (Vander Wall et al., 2005; Enders and Vander Wall, 2011). Additionally, it is plausible that the root suckering version of D. cinerea and other encroaching species may be more important than seed dispersal in the establishment of D. cinerea and other encroachers (Wakeling and Bond, 2007).
Shrub encroachment is altering tropical savannas (Stevens et al., 2016a,b) and our results suggest a potential role for rodents in mitigating their spread. However, rodent-shrub interactions will need to be evaluated on more ecologically relevant scales, while accounting for variability in the placement and relative abundance of seeds (Lichti et al., 2014; Yi and Wang, 2015) before we can interpret the role of rodents in limiting the encroachment of woody plants. Future work should explicitly examine rodent and seed interactions (Forget and Wenny, 2005; Wróbel and Zwolak, 2013), instead of assuming seed predation or dispersal based on previous research, laboratory studies, and natural history accounts. If rodents provide this critical service, it highlights the consequences of the loss and decline of seed-eating mammals (Hurst et al., 2014; Mills et al., 2018) and the need to maintain savannas’ thick grassy-layer that facilities seed predation, which is threatened by chronic overutilization by domestic and wild ungulates.
Data Availability Statement
The raw data supporting the conclusions of this article will be made available by the authors, without undue reservation.
Ethics Statement
The animal study was reviewed and approved by the University of Florida’s Institutional Animal Care and Use Committee (#201509045).
Author Contributions
ST collected the data and wrote the initial draft of the manuscript. RM and ST conducted the data analysis. All authors helped design the study and contributed to the writing and review of the manuscript.
Funding
This project was funded by NSF IRES Grant (No. 1459882) and U.S. National Institute of Food and Agriculture, Hatch project FLA-WEC-005125.
Conflict of Interest
The authors declare that the research was conducted in the absence of any commercial or financial relationships that could be construed as a potential conflict of interest.
Acknowledgments
This research was part of the BROWSE program and conducted out of the Savannah Research Center. We thank Phumlile Simelane, Jack Hartfelder, Muzi Sibya, and all the members of the IRES program for their support in the field. We also thank Tal Fineberg and the staff at the Savannah Research Center and the Mbuluzi Game Reserve for their support.
Supplementary Material
The Supplementary Material for this article can be found online at: https://www.frontiersin.org/articles/10.3389/fevo.2021.676572/full#supplementary-material
Footnotes
References
Aganga, A. A., and Motshewa, C. B. (2007). Nutritive value of urea molasses block containing acacia erubescens or Dichrostachys cinerea as natural protein sources. J. Anim. Vet. Adv. 6, 1280–1283.
Archer, S. R., Andersen, E. M., Predick, K. I., Schwinning, S., Steidl, R. J., and Woods, S. R. (2017). “Woody plant encroachment: causes and consequences,” in Rangeland Systems Springer Series on Environmental Management, ed. D. David (Berlin: Springer).
Bailey, K. M., McCleery, R. A., Binford, M. W., and Zweig, C. (2016). Land-cover change within and around protected areas in a biodiversity hotspot. J. Land Use Sci. 11, 154–176. doi: 10.1080/1747423x.2015.1086905
Banasiak, N., and Shrader, A. M. (2016). Similarities in perceived predation risk prevent temporal partitioning of food by rodents in an African grassland. J. Mammal. 97, 483–489. doi: 10.1093/jmammal/gyv192
Barnes, M. E. (2001). Seed predation, germination and seedling establishment of Acacia erioloba in northern Botswana. J. Arid Environ. 49, 541–554. doi: 10.1006/jare.2001.0805
Bates, D. M., Maechler, M., Bolker, B. M., and Walker, S. (2015). Fitting linear mixed-effects models using lme4. J. Stat. Softw. 67, 1–48.
Bell, W. E., and van Staden, J. (1993). Seed structure and germination of Dichrostachys cinerea. S. Afr. J. Bot. 59, 9–13. doi: 10.1016/s0254-6299(16)30768-2
Bergstrom, B. J. (2013). Would East African savanna rodents inhibit woody encroachment? evidence from stable isotopes and microhistological analysis of feces. J. Mammal. 94, 436–447. doi: 10.1644/12-mamm-a-146.1
Bleicher, S. S. (2017). The landscape of fear conceptual framework: definition and review of current applications and misuses. PeerJ 5:e3772. doi: 10.7717/peerj.3772
Bond, W. J. (2008). What limits trees in C4 grasslands and savannas? Annu. Rev. Ecol. Evol. Syst. 39, 641–659. doi: 10.1146/annurev.ecolsys.39.110707.173411
Bransby, D., and Tainton, N. (1977). The disc pasture meter: possible applications in grazing management. Proc. Annu. Congresses Grassland Soc. Southern Afr. 12, 115–118. doi: 10.1080/00725560.1977.9648818
Brooks, M., Kristensen, K., Koenj van, B., Magnusson, A., Casperw, B., et al. (2017). glmmTMB balances speed and flexibility among packages for Zero-inflated Generalized linear mixed modeling. R J. 9:378. doi: 10.32614/rj-2017-066
Brown, J. H., and Heske, E. J. (1990). Control of a desert-grassland transition by a keystone rodent guild. Science 250, 1705–1707. doi: 10.1126/science.250.4988.1705
Brown, J. S. (1999). Vigilance, patch use and habitat selection: foraging under predation risk. Evol. Ecol. Res. 1, 49–71.
Brown, J. S., Laundre, J. W., and Gurung, M. (1999). The ecology of fear: optimal foraging, game theory, and trophic interactions. J. Mammal. 80, 385–399. doi: 10.2307/1383287
Burnham, K. P., and Anderson, D. R. (2002). Model Selection and Multimodel Inference: a Practical Information-Theoretic Approach, 2nd Edition. New York: Springer.
Busch, M., Knight, C., Mazía, C. N., Hodara, K., Muschetto, E., and Chaneton, E. (2011). Rodent seed predation on tree invader species in grassland habitats of the inland Pampa. Ecol. Res. 27, 369–376. doi: 10.1007/s11284-011-0909-1
Campos-Arceiz, A., and Blake, S. (2011). Megagardeners of the forest – the role of elephants in seed dispersal. Acta Oecol. 37, 542–553. doi: 10.1016/j.actao.2011.01.014
Canfield, R. H. (1941). Application of the line interception method in sampling range vegetation. J. For. 39, 388–394.
Charles-Dominique, T., Midgley, G. F., Tomlinson, K. W., and Bond, W. J. (2018). Steal the light: shade vs fire adapted vegetation in forest-savanna mosaics. New Phytol. 218, 1419–1429. doi: 10.1111/nph.15117
Charnov, E. L. (1976). Optimal foraging, the marginal value theorem. Theor. Popul. Biol. 9, 129–136. doi: 10.1016/0040-5809(76)90040-x
Chimimba, C. T., and Linzey, A. V. (2008). Aethomys ineptus (Rodentia: Muridae). Mammalian Species 809, 1–7. doi: 10.1644/809.1
Crawley, M. J. (2000). “Seed predators and plant population dynamics,” in Seeds: the Ecology of Regeneration in Plant Communities, eds M. Fenner and R. S. Gallagher (Wallingford: CABI).
Davidson, D. W., Inouye, R. S., and Brown, J. H. (1984). Granivory in a desert ecosystem: experimental evidence for indirect facilitation of ants by rodents. Ecology 65, 1780–1786. doi: 10.2307/1937774
Donaldson, J. S. (1993). Mast-seeding in the cycad genus Encephalartos: a test of the predator satiation hypothesis. Oecologia 94, 262–271. doi: 10.1007/bf00341326
Dudley, J. (1999). Seed dispersal of Acacia erioloba by African bush elephants in Hwange National Park, Zimbabwe. Afr. J. Ecol. 37, 375–385. doi: 10.1046/j.1365-2028.1999.00191.x
Edwards, G. R., and Crawley, M. J. (1999). Rodent seed predation and seedling recruitment in mesic. Oecologia 118, 288–296. doi: 10.1007/s004420050729
Ellison, G. T. H. (1993). Group size, burrow structure and hoarding activity of pouched mice (Saccostomus campestris: Cricetidae) in southern Africa. Afr. J. Ecol. 31, 135–155. doi: 10.1111/j.1365-2028.1993.tb00527.x
Enders, M. S., and Vander Wall, S. B. (2011). Black bears Ursus americanus are effective seed dispersers, with a little help from their friends. Oikos 121, 589–596. doi: 10.1111/j.1600-0706.2011.19710.x
Fahrig, L., Baudry, J., Brotons, L., Burel, F. G., Crist, T. O., Fuller, R. J., et al. (2011). Functional landscape heterogeneity and animal biodiversity in agricultural landscapes. Ecol. Lett. 14, 101–112. doi: 10.1111/j.1461-0248.2010.01559.x
Field, A. C. (1975). Seasonal changes in reproduction, diet and body composition of two equatorial rodents. East Afr. Wildlife J. 13, 221–235. doi: 10.1111/j.1365-2028.1975.tb00136.x
Forget, P. M., and Wenny, D. (2005). “How to elucidate seed fate? a review of methods used to study seed removal and secondary seed dispersal,” in Seed Fate. Predation, Dispersal and Seedling Establishment, ed. P. M. Forget (Wallingford: CABI Publishing), 379–393. doi: 10.1079/9780851998060.0379
Gordon, C. E., Eldridge, D. J., Ripple, W. J., Crowther, M. S., Moore, B. D., and Letnic, M. (2016). Shrub encroachment is linked to extirpation of an apex predator. J. Anim. Ecol. 86, 147–157. doi: 10.1111/1365-2656.12607
Goudie, A. S., and Price-Williams, D. (1983). The Atlas of Swaziland. Lobamba: Swaziland National Trust Commission.
Gustafson, E. J. (1998). Quantifying landscape spatial pattern: what is the state of the art? Ecosystems 1, 143–156. doi: 10.1007/s100219900011
Hagenah, N., Prins, H. H., and Olff, H. (2009). Effects of large herbivores on murid rodents in a South African savanna. J. Trop. Ecol. 25, 483–492. doi: 10.1017/s0266467409990046
Hanney, P. (1965). The muridae of malawi (Africa: Nyasaland). J. Zool. 146, 577–633. doi: 10.1111/j.1469-7998.1965.tb05224.x
Happold, D. (2013). “Order LAGOMORPHA hares, rock-hares, rabbits, pikas,” in Mammals of Africa Vol III, Rodents, Hares and Rabbits. London: Bloomsbury Publishing.
Hoffman, E. W. (2006). Flower Initiation and Development of Protea cv. Carnival. Doctoral dissertation. Stellenbosch: Stellenbosch University.
Honek, A., Martinkova, Z., Saska, P., and Koprdova, S. (2009). Role of post-dispersal seed and seedling predation in establishment of dandelion (Taraxacum agg.) plants. Agric. Ecosyst. Environ. 134, 126–135. doi: 10.1016/j.agee.2009.06.001
Hoole, C., McKechnie, A. E., Parker, D. M., and Bennett, N. C. (2017). The endogenous activity patterns of Africa’s smallest terrestrial mammal, the pygmy mouse (Mus minutoides). Can. J. Zool. 95, 745–752. doi: 10.1139/cjz-2016-0250
Hulme, P. E. (1993). Post-dispersal seed predation by small mammals. Symp. Zool. Soc. Lond. 65, 269–287.
Hulme, P. E. (1997). Post-dispersal seed predation and the establishment of vertebrate dispersed plants in Mediterranean scrublands. Oecologia 111, 91–98. doi: 10.1007/s004420050212
Hurst, Z. M., McCleery, R. A., Collier, B. A., Silvy, N. J., Taylor, P. J., and Monadjem, A. (2014). Linking changes in small mammal communities to ecosystem functions in an agricultural landscape. Mamm. Biol. 79, 17–23. doi: 10.1016/j.mambio.2013.08.008
Huxman, T. E., Wilcox, B. P., Breshears, D. D., Scott, R. L., Snyder, K. A., Small, E. E., et al. (2005). Ecohydrological implications of woody plant encroachment. Ecology 86, 308–319. doi: 10.1890/03-0583
Irie, K., and Tsuyuzaki, S. (2011). Dispersal timing, palatability and caching of acorns of Aesculus turbinata Bl. Plant Biosyst. Int. J. Dealing All Aspects Plant Biol. 145, 798–801. doi: 10.1080/11263504.2011.606986
Janzen, D. H. (1984). Dispersal of small seeds by big herbivores: foliage is the fruit. Am. Nat. 123, 338–353. doi: 10.1086/284208
Kerley, G. I. H., and Erasmus, T. (1991). What do mice select for in seeds? Oecologia 86, 261–267. doi: 10.1007/bf00317539
Kern, N. G. (1981). The influence of fire on populations of small mammals of the Kruger National Park. Koedoe 24, 125–157.
Köchy, M., and Wilson, S. D. (2000). Competitive effects of shrubs and grasses in prairie. Oikos 91, 385–395. doi: 10.1034/j.1600-0706.2000.910219.x
Korn, H. (1987). Densities and biomasses of non-fossorial southern African savanna rodents during the dry season. Oecologia 72, 410–413. doi: 10.1007/bf00377572
Larios, L., Pearson, D. E., and Maron, J. L. (2017). Incorporating the effects of generalist seed predators into plant community theory. Funct. Ecol. 31, 1856–1867. doi: 10.1111/1365-2435.12905
LaScaleia, M. C., Reynolds, C., Magagula, C. N., Roets, F., and Mccleery, R. A. (2018). Dung beetle richness decreases with increasing landscape structural heterogeneity in an African savanna-agricultural mosaic. Insect Conserv. Divers. 11, 396–406. doi: 10.1111/icad.12290
Laundré, J. W., Hernández, L., Medina, P. L., Campanella, A., López-Portillo, J., González-Romero, A., et al. (2014). The landscape of fear: the missing link to understand top-down and bottom-up controls of prey abundance? Ecology 95, 1141–1152. doi: 10.1890/13-1083.1
Lehmann, C. E. R., Anderson, T. M., Sankaran, M., Higgins, S. I., Archibald, S., Hoffmann, W. A., et al. (2014). Savanna vegetation-fire-climate relationships differ among continents. Science 343, 548–552. doi: 10.1126/science.1247355
Lichti, N. I., Steele, M. A., Zhang, H., and Swihart, R. K. (2014). Mast species composition alters seed fate in North American rodent-dispersed hardwoods. Ecology 95, 1746–1758. doi: 10.1890/13-1657.1
Lima, S. L., and Dill, L. M. (1990). Behavioral decisions made under the risk of predation: a review and prospectus. Can. J. Zool. 68, 619–640. doi: 10.1139/z90-092
Lindquist, E. S., and Carroll, C. R. (2004). Differential seed and seedling predation by crabs: impacts on tropical coastal forest composition. Oecologia 141, 661–671. doi: 10.1007/s00442-004-1673-5
Loggins, A. A., Monadjem, A., Kruger, L. M., Reichert, B. E., and Mccleery, R. A. (2019a). Vegetation structure shapes small mammal communities in African savannas. J. Mammal. 100, 1243–1252. doi: 10.1093/jmammal/gyz100
Loggins, A. A., Shrader, A. M., Monadjem, A., and Mccleery, R. A. (2019b). Shrub cover homogenizes small mammals’ activity and perceived predation risk. Sci. Rep. 9:16857.
Louda, S. M. (1982). Distribution ecology: variation in plant recruitment over a gradient in relation to insect seed predation. Ecol. Monogr. 52, 25–41. doi: 10.2307/2937343
Maron, J. L., and Kauffman, M. J. (2006). Habitat-specific impacts of multiple consumers on plant population dynamics. Ecology 87, 113–124. doi: 10.1890/05-0434
McCleery, R. A., Monadjem, A., Baiser, B., Fletcher, R. J., Vickers, K., and Kruger, L. (2018). Animal diversity declines with broad-scale homogenization of canopy cover in African savannas. Biol. Conserv. 226, 54–62. doi: 10.1016/j.biocon.2018.07.020
McCleery, R. A., Zweig, C. L., Desa, M. A., Hunt, R., Kitchens, W. M., and Percival, H. F. (2014). A novel method for camera-trapping small mammals. Wildlife Soc. Bull. 38, 887–891. doi: 10.1002/wsb.447
Meiners, S. J., and Stiles, E. W. (1997). Selective predation on the seeds of woody plants. J. Torrey Bot. Soc. 124:67. doi: 10.2307/2996599
Miller, M. F. (1994a). Seed predation by nocturnal rodents in an African savanna ecosystem. S. Afr. J. Zool. 29, 262–266. doi: 10.1080/02541858.1994.11448361
Miller, M. F. (1994b). The costs and benefits of acacia seed consumption by ungulates. Oikos 71:181. doi: 10.2307/3546187
Miller, M. F. (1995). Acacia seed survival, seed germination and seedling growth following pod consumption by large herbivores and seed chewing by rodents. Afr. J. Ecol. 33, 194–210. doi: 10.1111/j.1365-2028.1995.tb00797.x
Mills, C. H., Gordon, C. E., and Letnic, M. (2018). Rewilded mammal assemblages reveal the missing ecological functions of granivores. Funct. Ecol. 32, 475–485. doi: 10.1111/1365-2435.12950
Monadjem, A. (1997a). Stomach contents of 19 species of small mammals from Swaziland. S. Afr. J. Zool. 32, 23–26. doi: 10.1080/02541858.1997.11448423
Monadjem, A. (1997b). Habitat preferences and biomasses of small mammals in Swaziland. Afr. J. Ecol. 35, 64–72.
Monadjem, A. (1998). Reproductive biology, age structure, and diet of Mastomys natalensis (Muridae: Rodentia) in a Swaziland grassland. Zeitschrift für Säugetierkunde 63, 347–356.
Monadjem, A. (1999a). Geographic distribution patterns of small mammals in Swaziland in relation to abiotic factors and human land-use activity. Biodivers. Conserv. 8, 223–237.
Monadjem, A. (1999b). Population dynamics of Mus minutoides and Steatomys pratensis (Muridae: Rodentia) in a subtropical grassland in Swaziland. Afr. J. Ecol. 37, 202–210. doi: 10.1046/j.1365-2028.1999.00169.x
Monadjem, A., and Garcelon, D. K. (2005). Nesting distribution of vultures in relation to land use in Swaziland. Biodivers. Conserv. 14, 2079–2093. doi: 10.1007/s10531-004-4358-9
Moorhead, L. C. (2017). Effects of Rodents on Ecosystem Structure and Function. Doctoral dissertation. Knoxville: University of Tennessee.
Mulungu, L. S., Themb’alilahlwa, A. M., Massawe, A. W., Kennis, J., Crauwels, D., Eiseb, S., et al. (2011). Dietary differences of the multimammate mouse, Mastomys natalensis (Smith, 1834), across different habitats and seasons in Tanzania and Swaziland. Wildlife Res. 38, 640–646. doi: 10.1071/wr11028
Ness, J. H., and Morin, D. F. (2008). Forest edges and landscape history shape interactions between plants, seed-dispersing ants and seed predators. Biol. Conserv. 141, 838–847. doi: 10.1016/j.biocon.2007.12.029
Nuñez, M. A., Simberloff, D., and Relva, M. A. (2008). Seed predation as a barrier to alien conifer invasions. Biol. Invasions 10, 1389–1398. doi: 10.1007/s10530-007-9214-x
Nupp, T. E., and Swihart, R. K. (2000). Landscape-level correlates of small-mammal assemblages in forest fragments of farmland. J. Mammal. 81, 512–526. doi: 10.1093/jmammal/81.2.512
Orrock, J. L., and Damschen, E. I. (2005). Corridors cause differential seed predation. Ecol. Appl. 15, 793–798. doi: 10.1890/04-1129
Orrock, J. L., Levey, D. J., Danielson, B. J., and Damschen, E. I. (2006). Seed predation, not seed dispersal, explains the landscape-level abundance of an early-successional plant. J. Ecol. 94, 838–845. doi: 10.1111/j.1365-2745.2006.01125.x
Ostfeld, R. S., Manson, R. H., and Canham, C. D. (1997). Effects of rodents on survival of tree seeds and seedlings invading old fields. Ecology 78, 1531–1542. doi: 10.1890/0012-9658(1997)078[1531:eoroso]2.0.co;2
Palgrave, K. C., and Palgrave, M. C. (2015). Palgraves Trees of Southern Africa. Cape Town: Penguin Random House South Africa.
Parker, J. D., Burkepile, D. E., and Hay, M. E. (2006). Opposing effects of native and exotic herbivores on plant invasions. Science 311, 1459–1461. doi: 10.1126/science.1121407
Pearson, D. E., Hierro, J. L., Chiuffo, M., and Villarreal, D. (2013). Rodent seed predation as a biotic filter influencing exotic plant abundance and distribution. Biol. Invasions 16, 1185–1196. doi: 10.1007/s10530-013-0573-1
Pearson, D. E., Potter, T., and Maron, J. L. (2012). Biotic resistance: exclusion of native rodent consumers releases populations of a weak invader. J. Ecol. 100, 1383–1390. doi: 10.1111/j.1365-2745.2012.02025.x
Pierce, N. A., Archer, S. R., Bestelmeyer, B. T., and James, D. K. (2018). Grass-shrub competition in arid lands: an overlooked driver in grassland–shrubland state transition? Ecosystems 22, 619–628. doi: 10.1007/s10021-018-0290-9
Quenette, P. Y. (1990). Functions of vigilance behavior in mammals: a review. Acta Oecol. 11, 801–818.
R Core Team (2018). R: a Language and Environment for Statistical Computing. Vienna: R Foundation for Statistical Computing.
Revelle, W. (2014). Psych: Procedures for Personality and Psychological Research. R Package Version 1. Evanston: Northwestern University.
Reynolds, C., Fletcher, R. J., Carneiro, C. M., Jennings, N., Ke, A., LaScaleia, M. C., et al. (2018). Inconsistent effects of landscape heterogeneity and land-use on animal diversity in an agricultural mosaic: a multi-scale and multi-taxon investigation. Landsc. Ecol. 33, 241–255. doi: 10.1007/s10980-017-0595-7
Rinella, M. J., Hammond, D. H., Bryant, A.-E. M., and Kozar, B. J. (2015). High precipitation and seeded species competition reduce seeded shrub establishment during dryland restoration. Ecol. Appl. 25, 1044–1053. doi: 10.1890/14-1110.1
Roques, K. G., Oconnor, T. G., and Watkinson, A. R. (2001). Dynamics of shrub encroachment in an African savanna: relative influences of fire, herbivory, rainfall and density dependence. J. Appl. Ecol. 38, 268–280. doi: 10.1046/j.1365-2664.2001.00567.x
Sala, O. E., and Maestre, F. T. (2014). Grass-woodland transitions: determinants and consequences for ecosystem functioning and provisioning of services. J. Ecol. 102, 1357–1362. doi: 10.1111/1365-2745.12326
Scholes, R. J. (2003). Convex relationships in ecosystems containing mixtures of trees and grass. Environ. Resour. Econ. 26, 559–574. doi: 10.1023/b:eare.0000007349.67564.b3
Scholtz, R. (2008). Small Mammal Community Structure (Rodentia: Muridae) in Punda Maria, Kruger National Park, South Africa: Causes and consequences. Ph.D. thesis. South Africa: University of Cape Town.
Schupp, E. W. (1993). Quantity, quality and the effectiveness of seed dispersal by animals. Vegetatio 107, 15–29. doi: 10.1007/978-94-011-1749-4_2
Sirami, C., and Monadjem, A. (2011). Changes in bird communities in Swaziland savannas between 1998 and 2008 owing to shrub encroachment. Divers. Distrib. 18, 390–400. doi: 10.1111/j.1472-4642.2011.00810.x
Skinner, J. D., and Chimimba, C. T. (2005). The Mammals of the Southern African Sub-region. Cambridge: Cambridge University Press.
Smith, R. J., Easton, J., Nhancale, B. A., Armstrong, A. J., Culverwell, J., Dlamini, S. D., et al. (2008). Designing a transfrontier conservation landscape for the Maputaland centre of endemism using biodiversity, economic and threat data. Biol. Conserv. 141, 2127–2138. doi: 10.1016/j.biocon.2008.06.010
Soto-Shoender, J. R., McCleery, R. A., Monadjem, A., and Gwinn, D. C. (2018). The importance of grass cover for mammalian diversity and habitat associations in a bush encroached savanna. Biol. Conserv. 221, 127–136. doi: 10.1016/j.biocon.2018.02.028
Stanton, R. A., Boone, W. W., Soto-Shoender, J., Fletcher, R. J., Blaum, N., and McCleery, R. A. (2018). Shrub encroachment and vertebrate diversity: a global meta-analysis. Global Ecol. Biogeogr. 27, 368–379. doi: 10.1111/geb.12675
Stanton, R. A., Fletcher, R. J., Sibiya, M. D., Monadjem, A., and McCleery, R. A. (2020). The effects of shrub encroachment on bird occupancy vary with land use in an African savanna. Animal Conserv. 24, 194–205. doi: 10.1111/acv.12620
Staver, A. C., and Bond, W. J. (2014). Is there a ‘browse trap’? dynamics of herbivore impacts on trees and grasses in an African savanna. J. Ecol. 102, 595–602. doi: 10.1111/1365-2745.12230
Stevens, N., Erasmus, B. F. N., Archibald, S., and Bond, W. J. (2016a). Woody encroachment over 70 years in South African savannahs: overgrazing, global change or extinction aftershock? Philos. Trans. R. Soc. B Biol. Sci. 371:20150437. doi: 10.1098/rstb.2015.0437
Stevens, N., Lehmann, C. E. R., Murphy, B. P., and Durigan, G. (2016b). Savanna woody encroachment is widespread across three continents. Global Change Biol. 23, 235–244. doi: 10.1111/gcb.13409
Tiffney, B. H. (2004). Vertebrate dispersal of seed plants through time. Annu. Rev. Ecol. Evol. Syst. 35, 1–29. doi: 10.1146/annurev.ecolsys.34.011802.132535
Tjelele, J. T., Dziba, L. E., and Pule, H. T. (2012). Recovery and Germination of Dichrostachys cinerea seeds fed to Goats (Capra hircus). Rangel. Ecol. Manag. 65, 105–108. doi: 10.2111/rem-d-09-00161.1
Tjelele, J., Ward, D., and Dziba, L. (2015). The effects of seed ingestion by livestock, dung fertilization, trampling, grass competition and fire on seedling establishment of two woody plant species. PLoS One 10:e0117788. doi: 10.1371/journal.pone.0117788
Tolessa, T., Senbeta, F., and Kidane, M. (2016). Landscape composition and configuration in the central highlands of Ethiopia. Ecol. Evol. 6, 7409–7421. doi: 10.1002/ece3.2477
Tsopito, C. M., and Adogla-Bessa, T. (1998). Feed potential of Acacia species to ruminants in Botswana. Archivos De Zootecnia 47, 659–668.
Vander Wall, S. B., Kuhn, K. M., and Beck, M. J. (2005). Seed removal, seed predation, and secondary dispersal. Ecology 86, 801–806. doi: 10.1890/04-0847
Van Staden, J., Kelly, K. M., and Bell, W. E. (1994). The role of natural agents in the removal of coat-imposed dormancy in Dichrostachys cinerea (L.) Wight et Arn. seeds. Plant Growth Regul. 14, 51–59. doi: 10.1007/bf00024141
Vaz Ferreira, A., Bruna, E. M., and Vasconcelos, H. L. (2010). Seed predators limit plant recruitment in Neotropical savannas. Oikos 120, 1013–1022. doi: 10.1111/j.1600-0706.2010.19052.x
Wakeling, J., and Bond, W. J. (2007). Disturbance and the frequency of root suckering in an invasive savanna shrub, Dichrostachys cinerea. S. Afr. J. Bot. 24, 73–76. doi: 10.2989/ajrfs.2007.24.2.3.157
White, J. D., and Midgley, J. J. (2021). Seed dispersal, directed deterrence and germination in gifboom (Hyaenanche globosa; Picrodendraceae) seeds. S. Afr. J. Bot. 137, 434–439. doi: 10.1016/j.sajb.2020.11.022
Wróbel, A., and Zwolak, R. (2013). The choice of seed tracking method influenced fate of beech seeds dispersed by rodents. Plant Ecol. 214, 471–475. doi: 10.1007/s11258-013-0183-0
Xiao, Z., and Zhang, Z. (2016). Contrasting patterns of short-term indirect seed-seed interactions mediated by scatter-hoarding rodents. J. Anim. Ecol. 85, 1370–1377. doi: 10.1111/1365-2656.12542
Yi, X., and Wang, Z. (2015). Context-dependent seed dispersal determines acorn survival of sympatric oak species. Plant Ecol. 216, 123–132. doi: 10.1007/s11258-014-0421-0
Yu, F., Shi, X., Wang, D., Wang, T., Yi, X., and Lou, Y. (2014). Seed predation patterns favor the regeneration of dominant species in forest gaps compared with the understory in an oak-pine mixed forest. Acta Theriol. 59, 495–502. doi: 10.1007/s13364-014-0192-y
Zambatis, N., Zacharias, P., Morris, C., and Derry, J. (2006). Re-evaluation of the disc pasture meter calibration for the Kruger National Park, South Africa. Afr. J. Range Forage Sci. 23, 85–97. doi: 10.2989/10220110609485891
Zamora, R., and Matías, L. (2014). Seed dispersers, seed predators, and browsers act synergistically as biotic filters in a mosaic landscape. PLoS One 9:e0107385.
Keywords: ecosystem service, Eswatini, foraging, Dichrostachys cinerea, Senegalia nigrescens
Citation: Teman SJ, Stevens N, Monadjem A, Fletcher RJ Jr, Austin JD and McCleery R (2021) Savanna Rodents’ Selective Removal of an Encroaching Plant’s Seeds Increased With Grass Biomass. Front. Ecol. Evol. 9:676572. doi: 10.3389/fevo.2021.676572
Received: 05 March 2021; Accepted: 19 May 2021;
Published: 15 June 2021.
Edited by:
Balázs Deák, Centre for Ecological Research, Hungarian Academy of Science, HungaryReviewed by:
Laura Godó, University of Debrecen, HungaryJennifer Lesley Silcock, The University of Queensland, Australia
Copyright © 2021 Teman, Stevens, Monadjem, Fletcher, Austin and McCleery. This is an open-access article distributed under the terms of the Creative Commons Attribution License (CC BY). The use, distribution or reproduction in other forums is permitted, provided the original author(s) and the copyright owner(s) are credited and that the original publication in this journal is cited, in accordance with accepted academic practice. No use, distribution or reproduction is permitted which does not comply with these terms.
*Correspondence: Robert McCleery, cmFtY2NsZWVyeUB1ZmwuZWR1