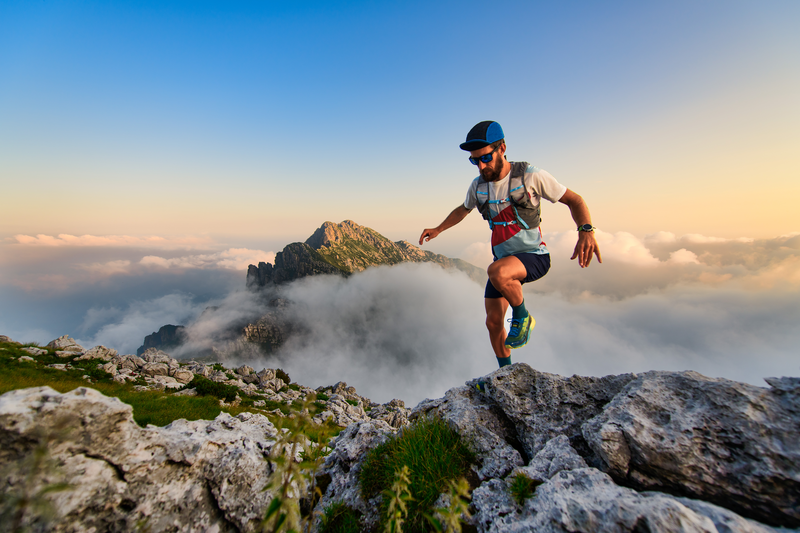
94% of researchers rate our articles as excellent or good
Learn more about the work of our research integrity team to safeguard the quality of each article we publish.
Find out more
ORIGINAL RESEARCH article
Front. Ecol. Evol. , 10 September 2021
Sec. Conservation and Restoration Ecology
Volume 9 - 2021 | https://doi.org/10.3389/fevo.2021.673148
This article is part of the Research Topic Nature-Based Solutions to Optimize Marine Ecosystems View all 8 articles
Estuaries have historically been subject to considerable habitat loss, and continue to be subjected to such in areas where the natural landward migration of intertidal habitats is constrained by hard coastal defences. Thus, in estuaries where direct (e.g., port development) or indirect (e.g., sea level rise) processes are predicted to threaten intertidal habitats and associated waterbird species, there is a regulatory requirement to produce compensatory intertidal habitats. Managed realignment (MR) is a shoreline management practise that is undertaken to build sustainable coastal defences and create intertidal habitats in estuaries. This nature-based solution brings multiple benefits in the form of carbon storage, increased resilience to flooding, and, potentially, the formation of new habitats, which is the topic of this study. A 75-ha site at the Paull Holme Strays (Humber Estuary, United Kingdom) was monitored over a 10-year period following MR to examine the change in the abundance of waterbirds in the chosen site in response to the physical processes occurring there. Using digital terrain models (DTMs) collected via light detection and ranging (LiDAR), we examined how four compensatory target species responded to changes in elevation after the creation of the site. It was shown that the very rapid accretion of estuarine sediment occurred in the first decade of the new re-created intertidal, which, over time, led to changes in the numbers of benthic foraging birds supported. Furthermore, elevation change was also driven by this sediment accretion, the rate of which depended on the initial bed elevation of the sectors within the site. Ten years after the recreation of the habitat, the spatial heterogeneity in the bed elevation remained high; however, the sectors with the lowest elevations accreted the most over the 10-year period. The foraging number of the four waterbird species that colonised the MR site significantly declined above a certain elevation, with this effect being most pronounced for the Eurasian curlew (Numenius arquata). The number of common shelducks (Tadorna tadorna), dunlins (Calidris alpina), and common redshanks (Tringa totanus) declined significantly after initial peaks 5–7 years after the creation of the site, reflecting the ongoing elevation changes. Thus, this study highlighted the need for long-term studies to understand how species respond to large-scale habitat construction. It can also aid in predicting the suitability of an MR site for waterbirds in the medium and long term.
Historically, large intertidal areas have been claimed from the sea. Estuarine wetlands, however, continue to be lost at an alarming rate across the globe. For example, in the the Yellow Sea (East Asia), the rate of loss of intertidal areas has risen dramatically over the last decades (Yang et al., 2011). In Europe, most countries have estimated the losses of coastal wetlands and sea grasses as exceeding 50% of the original area (Airoldi and Beck, 2007). In the United Kingdom (UK), the land claims for agricultural, residential, and industrial developments have historically affected at least 85% of estuaries (Davidson et al., 1991), with an overall estimated loss of 913 km2 of estuary area and 550 km2 of saltmarsh area by the end of the twentieth century (Davidson et al., 1991). With the implementation of greater site protection (Stroud et al., 2016), large-scale intertidal land reclamations for agricultural and residential developments have eased; however, small expanses of intertidal areas continue to be claimed for infrastructure projects that are deemed to be of public interest, such as port developments and coastal protection.
Waterbird species that rely heavily on intertidal areas during the non-breeding season may be significantly affected by habitat loss. By reducing the carrying capacity of an estuary, habitat loss can increase the potential for interference competition among waterbird species in remaining areas (Goss-Custard et al., 2002). While waterbirds can respond to estuarine habitat loss by emigrating to another estuary (Yang et al., 2011), many of them are site-faithful and, thus, may only move locally in response to a loss of habitat (Burton and Armitage, 2008), which increases local densities and, consequently, competition for resources (Stillman et al., 1997). This may affect individual fitness, i.e., body condition and survival rates (Burton et al., 2006), while effects can also be carried over to impacts on the breeding productivity of birds, with ultimate impacts on population size (Baker et al., 2004; Piersma et al., 2016; Studds et al., 2017). In response to these observations, the creation or restoration of intertidal habitats can help to mitigate the effects of non-breeding habitat loss and the deterioration of intertidal habitats (Atkinson, 2003). By increasing the overall carrying capacity of estuaries, the creation of new intertidal habitats may reduce the effects of interference competition on the individual fitness of waterbirds.
With the loss of intertidal habitats being of growing conservation concern to wader populations across the globe (Sutherland et al., 2012; Pearce-Higgins et al., 2017), the re-creation of intertidal habitats offers a solution to mitigate impacts on waterbird populations and other benefits for carbon and nitrogen storage and increased flood protection. Habitat restoration and creation are driven either directly or indirectly by environmental policies (e.g., Clean Air Act in the United States of America, USA) and the European Union Habitats Directive (92/43/EEC) in European Union (EU) countries. In northwest Europe, where tidal flats in estuaries are often constrained by flood defences, the realignment of flood defences is the preferred shoreline management practise to re-create intertidal areas (Esteves, 2014). This shoreline management practise is also known as managed realignment (MR), managed retreat, or depolderisation. It consists of moving the flood defences landward while breaching the existing defences and allowing the tidal flooding of terrestrial habitats to increase the intertidal area. Of a total of 98 MR sites across the globe, 51 are located in the UK, equating to a total of 24 km2 of intertidal habitats (ABPmer., 2020). These sites were created to offset the loss of intertidal habitats due to port developments, flood defence work, and environmental changes, e.g., sea level rise.
The ecological success of re-created intertidal habitats is difficult to measure, although it is usually based on the broad principle that the re-created intertidal areas should exhibit processes that are found in natural intertidal flats (Atkinson, 2003). Geist and Hawkins (2016) emphasised the need to define a clear target state in an aquatic restoration scheme. However, when creating intertidal habitats, outcomes can be unpredictable and may not match the desired goals, for example, as waterbird communities evolve over time in response to physical and biological processes (Evans et al., 1998; Brusati et al., 2001; Armitage et al., 2007).
In the United Kingdom, monitoring studies at MR sites have been short-term but have focussed on both physical and biological developments (Garbutt et al., 2006; Mazik et al., 2007; Garbutt and Boorman, 2009) with some examples of studies on waterbird colonisation (Evans et al., 1998; Atkinson et al., 2004; Mander et al., 2007). The re-created intertidal habitats monitored for wintering waterbirds in the UK have been small in size, but the studies at these sites have shown that natural waterbird communities may be successfully reproduced in just a few years (Atkinson et al., 2004; Mander et al., 2007). In these studies, the ecological state of the MR was judged against “reference” or “control” sites.
The use of newly created intertidal areas by foraging waterbirds depend primarily on the colonisation of benthic invertebrates, which themselves are driven by the deposition of sediment. This could be rapid in highly turbid environments where siltation rates are high (Mazik et al., 2010). However, over time, these high siltation rates may also result in the increase in the elevation of mudflats through accretion and eventual colonisation by saltmarsh vegetation, consequently lessening the suitability of re-created intertidal habitats for foraging waterbirds (Morris, 2013; Pontee, 2014). Thus, there needs to be a better understanding of the physical factors that control the abundance of waterbirds in MR sites and their long-term sustainability.
At the Paull Holme Strays MR (Humber Estuary, UK, Figure 1), 0.75 km2 of intertidal areas were re-created through the realignment of flood defences. Initially, the aim was to create ~ 0.32 km2 of mudflat (42%) and 0.43 km2 of saltmarsh (56%) (Edwards and Winn, 2006). Besides habitat creation, specific biodiversity targets were set by the stakeholders in the form of minimum population targets: the site was to support the foraging populations of a total of 30 individuals consisting of the common shelduck (Tadorna tadorna), Eurasian curlew (Numenius arquata), dunlin (Calidris alpina), and common redshank (Tringa totanus). These species were affected by habitat losses resulting from flood defence improvements in the Humber Estuary (Edwards and Winn, 2006; Environment Agency, 2007). All four target compensatory species are dependent on intertidal benthic prey in the winter and, thus, expected to forage on the developing mudflat in the MR site. Across the Humber Estuary, moderate (25–50%) decreases in the abundances of Eurasian curlews and common redshanks were recorded by the national Wetland Bird Survey between 2000/2001 and 2015/2016, while common shelduck and dunlin numbers remained stable (Woodward et al., 2018).
Figure 1. Location of the Paull Holme Strays managed realignment (MR) site on the Humber Estuary and sectors surveyed.
The MR site was created in 2003 and subjected to an 11-year monitoring programme on waterbirds. A previous study at the site indicated a rapid change in the waterbird community structure in the first 3 years of habitat creation following the transition from agricultural land to intertidal areas (Mander et al., 2007). High accretion rates, low elevation, and a lack of compaction in created borrow pits resulted in rapid colonisation by estuarine invertebrates (Mazik et al., 2007).
This study extended this temporal and spatial dataset to assess the long-term trends of waterbirds and changes in bird abundance in response to geomorphological changes occurring in the re-created intertidal habitats. The focus was on the four benthivorous species that were listed as compensatory requirement targets at the Paull Holme Strays MR: the common Shelduck and three waders, namely, the Eurasian curlew, dunlin, and common redshank. A generalised additive modelling approach was then followed, aiming to investigate the hypothesis that the relative use of areas within the site by foraging benthivorous species is determined by differences in elevation, which are associated with the pre-breach natural landscape conditions of the MR site and driven by sedimentary processes taking place over time (i.e., change in elevation due to accretion). It was also predicted that foraging benthivorous species would favour the intertidal habitats of a given elevation range and that, above this shore level, usage would decrease.
The study was carried out at the Paull Holme Strays MR site (53°44′N, 0°16′W) in the Humber Estuary Special Protection Area (SPA) and Ramsar site, UK (Figure 1). The Humber Estuary is an important wintering and stopover site for several East Atlantic Flyway waterbirds, supporting over 150,000 waterbirds in the winter (Stroud et al., 2016; Frost et al., 2020). Although, historically, the site was a wetland habitat before being claimed for agriculture (in ca. 1,700), the Paull Holme Strays site was opened to the estuary through the realignment of the sea wall in September 2003. Two breaches were then created, allowing the tidal flooding of the site and the subsequent development of intertidal habitats (Figure 1).
The MR site provides compensation for direct and immediate habitat losses resulting from the tidal defence improvement works carried out in the middle estuary in 2003. Flood defence work improvement in the middle Humber Estuary produced immediate losses of 0.005 km2 of mudflat and 0.003 km2 of saltmarsh, with a compensation ratio of 3:1 then being applied to these direct losses. The estuary also aimed to provide compensation for future losses associated with coastal squeeze, with a further 0.056 km2 of mudflat and 0.05 km2 of saltmarsh predicted to be lost because of this phenomenon as a result of flood defence work improvements in the middle estuary (Environment Agency, 2007). Thus, a compensation ratio of 1:1 was applied to these indirect losses.
Following the breach of the existing seawall at the Paull Holme Strays in September 2003, both intertidal saltmarshes and mudflats developed in line with the predicted or desired habitat ratio before the breach, with a high proportion of saltmarshes established (58% in 2013) at the expense of mudflats (39% in 2013), with the rest (3%) being terrestrial habitats in 2013 (Brown, 2014). The mean high-water spring tidal height in the area was 3.7 m, while the neap tidal height was 2.1 m [measured at King George Dock, 6 km upstream from the Paull Holme Strays site and heights are given above UK ordinance datum (OD, the mean sea level)]. The MR site was high in tidal frame, as the elevation of the sediment surface varied between 2.78 and 3.65 m above OD in 2013. Because of its position on the tidal frame, the site remained dry on neap tides.
Nine areas (sectors) were defined within the site, delimited by remnant drainage channels from the former agricultural landscape (Figure 1). The size of the sectors varied between 0.07 (sector A) and 0.18 km2 (sector D), with each sector being associated with distinct geomorphological characteristics (e.g., different geometry and bed elevations) and dominant habitat types at the pre-breach stage (Table 1). Before habitat creation, the MR site consisted of arable fields intersected by drains. The site also featured a soak dyke beyond the existing wall. A site intervention before the breach further modified the site through the creation of a series of borrow pits/pools, which resulted in sectors of the MR site varying considerably in initial elevation (Figure 2).
Table 1. Size and dominant prior habitat creation of sectors within the Paull Holme Strays managed realignment (MR) site.
Figure 2. Changes in the elevation in the MR site between 2003 (Year 1) and 2012 (Year 10) derived from a digital terrain model (DTM). For the dates of the measurements, see Table 2.
The MR site was well connected to established intertidal habitats, as it was fronted by an extensive mudflat and sandflat supporting an assemblage dominated by foraging waterbirds during tidal emersion, such as common shelducks and waders such as Eurasian curlews, dunlins, and common redshanks. Other foraging wildfowl such as Eurasian teals (Anas crecca), Eurasian wigeons (Anas penelope), and mallards (Anas platyrhynchos) were also present, albeit in lower numbers (Mander and Cutts, 2005; Calbrade, 2013).
We used digital terrain models (DTMs) collected via light detection and ranging (LiDAR) to investigate the temporal geomorphological changes following the tidal inundation of the site. LiDAR is an airborne mapping technique that uses a laser to measure the distance between the aircraft and the ground, allowing highly detailed terrain models to be generated at spatial resolutions of between 25 cm and 2 m. All LiDAR data have a vertical accuracy of ±15 cm root mean square error (RMSE). The LiDAR data in this study are open-source and can be obtained from environment.data.gov.uk. Digital terrain model (DTM) data were processed in ArcGIS to examine changes in elevation on a sectoral basis over the years.
Following the breach of the flood defence in September 2003, waterbird abundance was monitored at the MR site between the winters of 2003/2004 (Year 1) and 2013/2014 (Year 11). Over half a tidal cycle (either on the flood or ebb), counts were undertaken at low, mid, and high tide once a month each winter from October to March. While the survey dates coincided with the half tidal cycle during hours of daylight, they were also determined by weather conditions (heavy rain and strong winds were avoided). In total, 174 counts were made over an 11-year period.
The sectors of the MR site (A to I, Figure 1) were used as recording units. The counts of sectors A to H were made from vantage points located along the new and old flood embankments, with each sector surveyed consecutively from one or two specific vantage points (depending on wind, glare, and tidal state). The counts of sector I were undertaken from a single vantage point (a bird hide located on the old flood embankment). A single observer carried out the counts on foot using a telescope with 25× and 60× eye pieces and 10 × 50 binoculars. Counts of the site took approximately 2 h. Disturbance to the avifauna was minimised by accessing the vantage points from below the bank. Species abundance and behaviour (foraging and non-foraging) were recorded within each sector of the MR site from Year 2 to Year 11. Counts were recorded for the MR site as a whole in Year 1, with bird recording sectors not being defined until Year 2. Waterbirds were categorised as foraging when actively looking for food or non-foraging when they were roosting, loafing, preening, etc.
The temporal changes in the abundance of functional groups and individual species over the 11-year monitoring period were assessed by analysing counts of foraging birds alone, as this was reflective of the quality of the intertidal habitat, with low-, mid-, and high-water counts for each monthly survey considered in the analysis.
While assemblages can be defined according to the taxonomic identities and abundances of those species, it has been increasingly viewed as valuable for defining communities according to species functional characteristics (Mendez et al., 2012). Accordingly, waterbird species were grouped into five trophic guilds based on their winter diet preferences on UK estuaries (Cramp et al., 1983): (i) herbivorous geese, swans, and ducks; (ii) benthivorous ducks; (iii) omnivorous ducks and rails; (iv) piscivorous grebes, cormorants, and herons; (v) benthivorous waders (Supplementary Table S1 in Supplementary Material). The annual abundance of each functional group (i.e., foraging guild) at the MR site was calculated by summing the monthly counts undertaken between October and March and the relative abundance of each functional group considered as the proportion of the abundance of the overall waterbird assemblage.
Temporal changes in the monthly foraging abundances of the four target benthivorous species associated with the MR compensatory requirement site, namely, common shelducks, Eurasian curlews, dunlins, and common redshanks, were also examined using box plots.
Finally, the annual changes in the elevation of sectors, which were used as a proxy for the geomorphological changes in the MR site, were examined between 2003 (Year 1) and 2012 (Year 10). The mean elevation (in mm) was calculated for each recording sector for the following years: 2003, 2004, 2005, 2007, 2010, and 2012. Data for the years 2006, 2008, 2009, and 2013 were excluded from the analysis because they only had partial LiDAR coverage of the MR site (Table 2).
The effects of elevation on the foraging abundances of dunlins, Eurasian curlews, common redshanks, and common shelducks were tested using generalised additive models (GAMs) in the mgcv package (R Core Team, 2019). These GAMs were selected in order to account for the potential non-linearity in the responses of the birds to elevation changes (Wood and Augustin, 2002). The analysis considered the monthly winter counts of each species at low, mid, and high water in each sector. The dataset used for the analysis included Years 2, 3, 5, 8, and 10. Year 1 was excluded because bird counts were not undertaken at an individual sector level, while Years 4, 6, 7, 9, and 11 were excluded because the elevation data from the LiDAR were incomplete. Measurements of elevation for each year were included as a predictor using thin-plate regression splines as the smoothing function (Wood, 2003). Assuming that the state of the tide would influence the use of MR habitats by foraging birds, tidal height at the time of observation was included as a continuous predictor in the model structure. We also fitted a factor-smooth interaction between tide height and elevation to account for variable relationships between the two variables. Furthermore, year was included to account for the possible annual differences in the overall numbers of birds using the site, reflecting the development of the habitat of the site following its creation in 2003 and wider patterns across the estuary. To disentangle absolute elevation and elevation change, an interaction between sector and year was included, with sector being used as a random factor. Day within the winter (since October 1) was also included to account for seasonal variations in numbers. A Poisson distribution in the GAM structure was initially performed and tested for over-dispersion using the DHARMa package. Since the results indicated the model to be mis-specified, we chose to perform a negative binomial distribution to model bird abundance, as negative binomials are used for count data when the variance is much larger than the mean (Zuur et al., 2009). In the dataset, the mean number of birds was 10.48 and the variance was 1702.22. The significance of each variable included in the models was tested using Wald tests (Wood, 2013a,b), and the importance of the model terms was assessed accordingly.
Correlations in the residuals from the models were examined using the autocorrelation function (ACF) and partial autocorrelation function (PACF) in R (R Core Team, 2019). A model diagnostic was undertaken (gam.check in the mgcv package); this command provided graphical model outputs that examined the homogeneity and normality of the unstandardised residuals (Supplementary Figure S3 in Supplementary Material).
There was a steady, year-on-year increase in the annual elevation across the MR site as a whole, but the greatest increase in elevation occurred in parts of the site with the lowest elevations at the times of breach, namely, sectors B, C, and D (Figure 3). In these sectors, the increase in elevation varied between 0.51 and 0.57 m. In comparison, the sectors with the highest elevations (F and H) at the times of the breach showed some inter-annual variability in subsequent elevations, but there was no clear increasing trend in elevation since the creation of the site (Figure 3).
Figure 3. Changes in the mean elevation of each sector of the Paull Holme Strays MR site over the study period.
Of the total 49 species recorded at the site between 2003/2004 and 2013/2014, 45 were recorded during the winter months. Specialist benthivorous (waders and ducks) were well represented by a total of 12 species: Eurasian oystercatcher (Haematopus ostralegus), common ringed plover (Charadrius hiaticula), grey plover (Pluvialis squatarola), red knot (Calidris canutus), sanderling (Calidris alba), dunlin, black-tailed godwit (Limosa limosa), bar-tailed godwit (Limosa lapponica), Eurasian curlew, common redshank, common shelduck, and northern pintail (Anas acuta) (Supplementary Figure S2 in Supplementary Material). The proportion of benthivorous waterbirds at the MR site was lower during the first 3 years (<55% of the total assemblage). However, this guild became more important over time, and its proportion increased to over 80% in Years 5, 7, 9, and 11 (Figure 4). Omnivorous waterbirds represented a major component of the foraging assemblage at the MR site, especially in the first 3 years of habitat creation, whereas the herbivorous and piscivorous guilds contributed little to the foraging community (Figure 4).
Figure 4. Temporal guild composition (% abundance) of the foraging assemblage at the MR site. Guilds are abbreviated as follows: BWad, benthivorous waders; BDuc, benthivorous ducks; Herb, herbivorous ducks, geese, and swans; Omni, omnivorous ducks and rails; Pisc, piscivorous grebes, cormorant, and herons.
Foraging benthivorous species (i.e., waders and common shelducks) exhibited markedly contrasting trends in abundance over the study period (Figure 5). Foraging dunlin numbers exhibited a gradual increase during the first 5 years of habitat creation at the MR site, followed by the relative stabilisation of numbers in the following years (Figure 5). In contrast, the abundance of common shelducks and common redshanks rapidly increased in the first few years of habitat creation at the MR site, but their abundance decreased markedly after Years 5 and 7, respectively (Figure 5). Eurasian curlews, on the other hand, showed a marked rise in abundance in Year 2, with numbers being maintained at that level for the rest of the monitoring programme then on, although some inter-annual fluctuations were observed.
Figure 5. Boxplot of the number of individuals foraging per annum. Box plots represent the median values (horizontal line), interquartile distances (boxes), non-outlier range (whiskers), outliers (circles), and extreme values (asterisks).
For the four compensatory requirement target species, the results of the modelling conformed to the prediction of the study that increasing elevation had a negative effect on the abundance of benthivorous foraging birds (Figure 6). “Day within the winter” was also significant in models for all the species except for Eurasian curlews, indicating a strong seasonal effect on bird abundance, while the effect of tide height was non-significant in all the models. The effect of “year since breaching” was also significant, reflecting the rapid change in abundance that took place across the years.
Figure 6. Generalised additive model (GAM) smoothing temporal terms (line) fitted to centre of gravity data for the four selected benthivorous species at Paul Holme Strays. Shaded areas and points represent point-wise confidence bands and partial residuals, respectively.
The relationship with elevation indicated that birds avoided foraging at the highest elevations of the MR site. Results varied among the species: common redshank abundance decreased above elevations of 2.75 m (Figure 6), whereas Eurasian curlew abundance decreased above elevations of 2 m and fell sharply above elevations of 2.75 m, although the model for this species explained a lower proportion of deviance (40.5%) compared with that for common redshanks (48.3%) (Table 3). The models for common shelducks and dunlins explained 57.7 and 53.6% of the deviance, respectively. A similar decreasing trend with increasing elevation was also noted in the abundance of other waterbirds (Supplementary Material S2), with similar marked declines in the abundance of grey plovers and bar-tailed godwits being noted at elevations of 2.75 m and above.
As the models demonstrated, the suitable elevations for most foraging benthivorous species were found below 2.75 m at the MR site. After 10 years of sediment accretion, only two sectors of the Paull Holme Strays MR site featured a mean elevation below this level (sectors B and D). Interestingly, these two sectors were modified before the breaching of the original embankment. Sediment was excavated from these sectors to create a new defensive earth bank, and while these areas accreted the fastest since habitat creation, bed elevation continued to be the lowest of the whole site after 10 years. Based on sector size and average elevation in 2013, only 38% of the site featured an elevation suitable for most benthivorous species.
Alongside the protection of existing wetlands, the creation of new habitats is an important mechanism for supporting biodiversity and ensuring that ecosystems can support varied activities. The re-created intertidal site described here was undoubtedly successful in accreting and sequestering carbon-rich mud from the estuarine water column, with a first approximation of 100,000 tonnes dry weight of sedimented material, storing 3,000–8,000 tonnes of carbon [using conversion values from Kelleway et al. (2016)]. However, the main focus of this restoration site was the creation of new intertidal habitats for internationally important wading bird communities. In this study, we showed that newly created habitats can change with time and possibly diverge from their intended plan. This is because, where habitat creation is used as compensation, the resulting bird and plant communities may not match those of the natural sites that were lost (Campbell et al., 2002; Desrochers et al., 2008).
In this study, within the MR site, there was heterogeneity of elevation resulting from the pre-breach landscape and construction, which changed over the course of the study through sediment accretion (Clapp, 2009; Mazik et al., 2010; Brown, 2014). The topography of the MR site (i.e., bed elevation in the first year) influenced the rate of sedimentation (Mazik et al., 2010; Brown, 2014). The rate of sediment accretion inside MR sites like the one chosen in this study are also driven by tidal and sediment regimes (Garbutt and Boorman, 2009). At the Paull Holme Strays MR site, the intertidal habitats at the lowest initial elevation accreted sediments at the fastest rate over the study period. From the LIDAR data and derived change in elevation (the proxy for accretion), over time, we found the accretion to range from 0.51 to 0.57 m between 2004 and 2012 in sectors B, C, and D of the MR site (which all featured a mean initial elevation of lower than 2.25 m OD in 2004).
Intertidal areas with higher elevations have shorter exposure times to water during tidal immersions, with lower food supplies, higher temperatures, and desiccation stresses for benthic invertebrate prey species. We included elevation in the models given the known relationships between the food resources of benthivorous species and cumulative accretion at MR sites (Evans et al., 1998; Atkinson et al., 2004; Garbutt et al., 2006), including the Paull Holme Strays site (Mazik et al., 2010). The models demonstrated that elevation was a major source of variability in the abundance of benthivorous species as the new habitat matured and accumulated sediment. Of the four compensatory requirement target species, Eurasian curlews showed the sharpest reduction in abundance in response to increasing elevation, while common redshank abundance started to decline at elevations above ~2.75 m. Elevation also had a significant effect on the abundance of foraging dunlins, common shelducks, and other benthivorous waterbird species that colonised the site (Supplementary Table S2 in Supplementary Material). Nevertheless, the decline in the abundance with increasing elevation across the MR site was more linear for dunlins and common shelducks, with a steady decline being noted (Figure 6).
Once elevation reached 2.75 m, the models indicated that the abundance of most of the waterbird species (except for dunlins and common shelducks) would start to decline sharply. In the Humber Estuary, tidal flats above ~2.75 m of elevation were not typically reached by neap high tides. However, Mazik et al. (2010) found the lower density and abundance of benthic invertebrates above this height at the site. Conversely, it was also found that the highest abundance of invertebrates was at the lowest elevation at the MR site. Furthermore, as sediment deposits and accretes, halophyte vegetation begins to colonise mudflats very rapidly in MR sites (Mossman et al., 2012a). While elevation within the tidal range is a primary driver of saltmarsh plant distribution, oxidation-reduction (redox) potential also affects the colonisation and distribution of saltmarsh plants in MR sites (Davy et al., 2011; Mossman et al., 2012b). Across the Paull Holme Stray MR site, saltmarsh vegetation coverage across the whole site increased over the years, with estimates of 36% in 2007, 54% in 2010, and 58% in 2013 (Brown, 2014). As a result of lower prey item density, those higher areas may not be longer profitable for the majority of benthivorous species to feed. However, the magnitude of the effect of elevation observed among the waterbird species may be linked to the species daily energy requirement and resource availability at the MR site. Dunlin and common shelduck foraging abundance, for example, did not decline sharply above a 2.75 m elevation. As small waders, such as dunlin, actively forage 80–95% of the time in the winter to meet their high daily energy requirements (Van de Kam et al., 2004), they may continue to feed across the tidal cycle, typically feeding on mobile prey associated with an incoming tide line. Additionally, they could also be feeding on microphytobenthos biofilms, which are of importance for dunlins and other small Calidris species (Mathot et al., 2010; Drouet et al., 2015). Common shelducks also continue to forage on submerged tidal flats by upending and dabbling in the shallow water; therefore, the effect of increasing elevation on abundance might be dampened.
While the elevation of intertidal habitats is one of the main abiotic factors that drive ecological processes in estuaries, other biotic factors such as predation may also control the abundance and spatial distribution of waterbirds in MR sites. Birds may avoid feeding in areas with high risks of predation despite an abundance of food (Newton, 2013). The use of these areas may, however, vary according to the fitness and ability of an individual bird to meet its daily energy requirement. In intertidal habitats, Cresswell et al. (2010) found that adult common redshanks shunned available food-rich habitats close to concealed cover (e.g., bushes) from which Sparrowhawks (Accipiter nisus) were able to attack. Conversely, juvenile birds and individuals with low fitness and close to starvation may take greater risks. Because of this predation risk, a degree of confinement may be especially important in affecting how a bird uses the different areas of an MR site. Intertidal habitats re-created through realignment sites, however, are often small in size, enclosed, and located high on the tidal frame because the land availability for shoreline management practises is limited. The presence of footpaths on embankments may also affect bird distribution, as recreational human activities taking place there (e.g., dog walking, birdwatching) may deter birds from foraging (Collop et al., 2016).
The success of habitat creation through MR for waterbirds is based on re-creating hydro-ecological processes, with the deposition of sediment from tidal inundation being a driving factor (Wolanski and Elliott, 2015; Elliott et al., 2016). Garbutt et al. (2006) showed that marine macrofaunal colonisation occurred only in newly accreted sediments and not in the original, consolidated agricultural substratum. As new MR sites are opened to tidal inundation, ecological and geomorphological changes take place in response to sediment accretion (Garbutt et al., 2006). Atkinson et al. (2004) and Evans et al. (1998) indicated rapid, positive changes in bird indicators in response to sediment accretion within the first 5 years of habitat creation. Atkinson (2003) also found that an adequate supply of sediment was the main constraint in successfully creating mudflats that might support natural avian communities. By considering a relative change in elevation over time and absolute elevation in areas of the MR site in the models, this study highlighted that increasing elevation eventually has a negative effect on the abundance of benthivorous species. While the accretion of sediments contributes to increased elevations in MR sites, the long-term provision of food-rich, self-sustained mudflats for foraging benthivorous birds is a key challenge in MR sites.
However, understanding the relationship between habitat development indicators and benthivorous birds can aid us in designing and implementing future MR site projects. For example, bed elevation and predicted current speeds within an MR site need to be considered at the project design phase to improve the effectiveness of MR for benthivorous bird species (also for intertidal fish, see Burgess et al., 2020). Furthermore, areas with higher pre-breach elevations are likely to be flooded less frequently, therefore limiting their estuarine sediment deposition (and consequent accretion and habitat change). This may be an important limitation in providing suitable foraging habitats for wading birds. It may also affect the ability of the site to achieve ecological targets that are related to compensation. Although bird and benthic communities may colonise MR sites very rapidly (Atkinson et al., 2004, Mander et al., 2007; Mazik et al., 2007), long-term monitoring is crucial in looking beyond the short-term development of sites and determining whether ecological compensatory targets have been met.
While the effect of environmental change on waterbirds at MR sites was the focus of this study, it is necessary to separate the role of habitat development within the site from the effect of any wider natural changes in order to effectively assess the development of re-created habitats. Waterbird populations may respond to wider environmental changes; however, it is unclear whether the changes in the waterbird numbers within the studied site reflected the wider estuary-level changes observed between 2000/2001 and 2015/2016 as there were no increases noted in the four compensatory target species across the Humber Estuary (Woodward et al., 2018). Furthermore, natural intertidal areas near MR sites are commonly used as a “reference” or “control” sites to assess the success of these sites in providing effective compensation for the loss of natural intertidal habitats (Atkinson et al., 2004; Mander et al., 2007). However, in this study, the “reference” site, which was established as the one immediately fronting the MR sites (i.e., further downshore of the MR site), could not strictly be treated as a “control site” given the potential for knock-on effects from the habitat re-creation. Therefore, it is recommended that monitoring should focus on neighbouring sites presenting similar habitat characteristics and encompassing similar topographies, although it has already been acknowledged that finding adequate control sites might be difficult in heterogeneous estuarine systems. Pre-construction bird data were also not available for this study, but a Before-After-Control-Impact (BACI) approach should be considered in future studies to effectively assess the development of re-created habitats.
The main focus of the study was the creation of mudflats for foraging benthivorous waders and ducks. While the development of saltmarsh vegetation due to the accretion of sediment had a negative effect on the abundance of the focal species, saltmarshes provide many ecosystem services (Turner and Schaafsma, 2015). For example, saltmarshes support roosting/feeding and nesting birds and contribute organic matter to the base of the food chain in estuaries (Hughes, 2004). Furthermore, saltmarshes containing sufficiently deep creeks and lagoons can be an important habitat for fish in estuaries (Whitfield, 2017), while colonising saltmarshes in MR sites have the potential to store carbon in the long term (Burden et al., 2013, 2019). Lastly, saltmarshes provide effective physical barriers to coastal erosion (Zhu et al., 2020). Thus, when setting up ecological targets for MR sites, it is important to consider the ecosystem services and goods/benefits that saltmarshes and mudflats provide and take a broader view of these ecological targets. Specifically, these targets should be about creating habitats that provide ecosystem services similar to those provided by neighbouring natural intertidal habitats.
In this study, within the MR site, there was heterogeneity of elevation resulting from the pre-breach landscape and construction, which then changed over the following 10-year through sediment accretion. Waterbird abundance was also closely related to elevation when the decreases in the numbers of foraging birds were at elevations above 2.75 m (OD) bed elevation. The modelling of the responses of benthic foraging birds to physical characteristics indicated that the long-term suitability of the MR site for these species was uncertain because of increasing elevation, which was a result of sediment accretion. Thus, this study recommends that the design and implementation of future projects should recognise the effects of accretion on waterbird abundance. It also highlights the need for long-term monitoring at MR sites (a minimum of 10 years) in determining whether bird targets related to habitat compensation have been met. While foraging benthivorous birds were the focus of this particular study, there are many facets to ecosystem responses to MR sites, with responses to physical changes varying among taxa and habitats. MR remains a nature-based solution that creates intertidal habitats with successive benefits as mudflats accrete and are colonised by saltmarsh vegetation.
The raw data supporting the conclusions of this article will be made available by the authors, without undue reservation.
LM, LS, and NB: conceptualisation. LM: formal analysis, investigation, validation, and visualisation. LM, LS, and CT: methodology. NB and RF: supervision and writing—review and editing. LM and LS: writing—original draft. All authors contributed to the article and approved the submitted version.
This study was partly funded by the Environment Agency (EA), who we wish to thank for the permission to publish the data. The study formed part of a PhD studentship undertaken by LM at the University of Hull and supported by the British Trust for Ornithology (BTO). The study also received financial support from IMMERSE - IMplementing MEasuRes for Sustainable Estuaries, an Interreg project supported by the North Sea Programme of the European Regional Development Fund of the European Union.
The authors declare that the research was conducted in the absence of any commercial or financial relationships that could be construed as a potential conflict of interest.
All claims expressed in this article are solely those of the authors and do not necessarily represent those of their affiliated organizations, or those of the publisher, the editors and the reviewers. Any product that may be evaluated in this article, or claim that may be made by its manufacturer, is not guaranteed or endorsed by the publisher.
We would like to thank Ray Eades for his help with the fieldwork, Anita Franco and Mike Elliott for their help with an earlier version of manuscript, and Philipp Boersch-Supan (BTO) for the statistical advice.
The Supplementary Material for this article can be found online at: https://www.frontiersin.org/articles/10.3389/fevo.2021.673148/full#supplementary-material
ABPmer. (2020). A Database of Completed Coastal Habitat Creation Schemes and Other Adaptation projects. Available online at: https://www.omreg.net/ (accessed July 14, 2020)
Airoldi, L., and Beck, M. W. (2007). Loss, status and trends for coastal marine habitats of Europe, Oceanography and marine biology. Boca Raton: CRC Press, 357–417.
Armitage, A. R., Jensen, S. M., Yoon, J. E., and Ambrose, R. F. (2007). Wintering Shorebird Assemblages and Behavior in Restored Tidal Wetlands in Southern California. Restor. Ecol. 15, 139–148. doi: 10.1111/j.1526-100X.2006.00198.x
Atkinson, P. W. (2003). Can we recreate or restore intertidal habitats for shorebirds? Wader Study Group Bull. 100, 67–72.
Atkinson, P. W., Crooks, S., Drewitt, A., Grant, A., Rehfisch, M. M., Sharpe, J., et al. (2004). The managed realignment in the UK—the first 5 years of colonisation by birds. Ibis 146, 101–110. doi: 10.1111/j.1474-919X.2004.00334.x
Baker, A. J., Gonzalez, P. M., Piersma, T., Niles, L. J., de Lima Serrano do Nascimento, I., Atkinson, P. W., et al. (2004). Rapid population decline in red knots: fitness consequences of decreased refuelling rates and late arrival in Delaware Bay. Proc. R. Soc. London. Series B: Biol. Sci. 271, 875–882. doi: 10.1098/rspb.2003.2663
Brown, S. L. (2014). Paull Holme Strays Intertidal Vegetation, Accretion and Erosion Monitoring Report 2013 Final Survey. Lynden, Marshall Row, Swanage: Dorset BH19 2AF.
Brusati, E. D., DuBowy, P. J., and Lacher, T. E. Jr. (2001). Comparing Ecological Functions of Natural and Created Wetlands for Shorebirds in Texas. Waterbirds Int. J. Waterbird Biol. 24, 371–380. doi: 10.2307/1522067
Burden, A., Garbutt, A., and Evans, C. D. (2019). Effect of restoration on saltmarsh carbon accumulation in Eastern England. Biol. Lett. 15:20180773. doi: 10.1098/rsbl.2018.0773
Burden, A., Garbutt, R. A., Evans, C. D., Jones, D. L., Cooper, D. M., et al. (2013). Carbon sequestration and biogeochemical cycling in a saltmarsh subject to coastal managed realignment. Estuar. Coast. Shelf Sci. 120, 12–20. doi: 10.1016/j.ecss.2013.01.014
Burgess, H., Nelson, K., Colclough, S., and Dale, J. (2020). The Impact that Geomorphological Development of Managed Realignment Sites has on Fish Habitat. In Coastal Management 2019: Joining forces to shape our future coasts (London: ICE Publishing), 375–389.
Burton, N. H. K., and Armitage, M. J. S. (2008). Settlement of Redshank Tringa totanus following winter habitat loss: effects of prior knowledge and age. Ardea 96, 191–205. doi: 10.5253/078.096.0205
Burton, N. H. K., Rehfisch, M. M., Clark, N. A., and Dodd, S. G. (2006). Impacts of sudden winter habitat loss on the body condition and survival of redshank Tringa totanus. J. Appl. Ecol. 43, 464–473. doi: 10.1111/j.1365-2664.2006.01156.x
Calbrade, N. A. (2013). Humber Estuary low tide count programme 2011/12. Thetford: Available online at: https://www.bto.org/our-science/publications/research-reports/humber-estuary-low-tide-programme-201112 (accessed July 14, 2020).
Campbell, D. A., Cole, C. A., and Brooks, R. P. (2002). A comparison of created and natural wetlands in Pennsylvania, USA. Wetlands Ecol. Manag. 10, 41–49. doi: 10.1023/A:1014335618914
Clapp, J. (2009). Managed Realignment in the Humber Estuary: Factors Influencing Sedimentation. Degree of Doctor of Philosophy. Hull: The University of Hull.
Collop, C., Stillman, R. A., Garbutt, A., Yates, M. G., Rispin, E., and Yates, T. (2016). Variability in the area, energy and time costs of wintering waders responding to disturbance. Ibis 158, 711–725. doi: 10.1111/ibi.12399
Cramp, S., Simmons, K. L., Brooks, D., Collar, N., Dunn, E., Gillmor, R., Hollom, P., Hudson, R., Nicholson, E., and Ogilvie, M., eds (1983). Handbook of the birds of Europe, the Middle East and North Africa. The birds of the Western Palearctic: 3. Waders to gulls. Oxford: Oxford University
Cresswell, W., Lind, J., and Quinn, J. L. (2010). Predator-hunting success and prey vulnerability: quantifying the spatial scale over which lethal and non-lethal effects of predation occur. J. Anim. Ecol. 79, 556–562. doi: 10.1111/j.1365-2656.2010.01671.x
Davidson, N., Laffoley, D. D. A., Doody, J. P., Way, L. S., Gordon, J., Key, R. E., et al. (1991). Nature Conservation and Estuaries in Great Britain. Peterborough: Nature Conservancy Council, 1–76.
Davy, A. J., Brown, M. J., Mossman, H. L., and Grant, A. (2011). Colonization of a newly developing salt marsh: disentangling independent effects of elevation and redox potential on halophytes. J. Ecol. 99, 1350–1357. doi: 10.1111/j.1365-2745.2011.01870.x
Desrochers, D. W., Keagy, J. C., and Cristol, D. A. (2008). Created versus natural wetlands: Avian communities in Virginia salt marshes. Ecoscience 15, 36–43. doi: 10.2980/1195-6860(2008)15[36:CVNWAC]2.0.CO;2
Drouet, S., Turpin, V., Godet, L., Cognie, B., Cosson, R. P., and Decottignies, P. (2015). Utilisation of intertidal mudflats by the Dunlin Calidris alpina in relation to microphytobenthic biofilms. J. Ornithol. 156, 75–83. doi: 10.1007/s10336-014-1133-x
Edwards, A. M. C., and Winn, P. S. J. (2006). The Humber Estuary, Eastern England: strategic planning of flood defences and habitats. Mar. Pollut. Bull. 53, 165–174. doi: 10.1016/j.marpolbul.2005.09.012
Elliott, M., Mander, L., Mazik, K., Simenstad, C., Valesini, F., Whitfield, A., et al. (2016). Ecoengineering with ecohydrology: successes and failures in estuarine restoration. Estuar. Coast. Shelf Sci. 176, 12–35. doi: 10.1016/j.ecss.2016.04.003
Environment Agency (2007). Humber Estuary Flood Defence Strategy Paull Holme Strays Environmental Monitoring Report. Leeds: Environment Agency.
Esteves, L. S. (2014). Managed Realignment: a Viable Long-term Coastal Management Strategy? Springer Briefs in Environmental Science, New York: Springer. doi: 10.1007/978-94-017-9029-1
Evans, P. R., Ward, R. M., Bone, M., and Leakey, M. (1998). Creation of temperate-climate intertidal mudflats: factors affecting colonization and use by benthic invertebrates and their bird predators. Mar. Pollut. Bull. 37, 535–545. doi: 10.1016/S0025-326X(98)00140-4
Frost, T. M., Calbrade, N. A., Birtles, G. A., Mellan, H. J., Hall, C., Robinson, A. E., et al. (2020). Waterbirds in the UK 2018/19: The Wetland Bird Survey. Ann Arbor, MI: Thetford.
Garbutt, A., and Boorman, L. A. (2009). Managed Realignment: Re-creating Intertidal Habitats on Formerly Reclaimed Land. New York: Elseiver, 763–786.
Garbutt, R. A., Reading, C. J., Wolters, M., Gray, A. J., and Rothery, P. (2006). Monitoring the development of intertidal habitats on former agricultural land after the managed realignment of coastal defences at Tollesbury, Essex, UK. Mar. Pollut. Bull. 53, 155–164. doi: 10.1016/j.marpolbul.2005.09.015
Geist, J., and Hawkins, S. J. (2016). Habitat recovery and restoration in aquatic ecosystems: current progress and future challenges. Aquat. Conserv. Mar. Freshw. Ecosyst. 26, 942–962. doi: 10.1002/aqc.2702
Goss-Custard, J. D., Stillman, R. A., West, A. D., Caldow, R. W. G., and McGrorty, S. (2002). Carrying capacity in overwintering migratory birds. Biol. Conserv. 105, 27–41. doi: 10.1016/S0006-3207(01)00175-6
Hughes, R. G. (2004). Climate change and loss of saltmarshes: consequences for birds. Ibis 146, 21–28. doi: 10.1111/j.1474-919X.2004.00324.x
Kelleway, J. J., Saintilan, N., Macreadie, P. I., and Ralph, P. J. (2016). Sedimentary factors are key predictors of carbon storage in SE Australian saltmarshes. Ecosystems 19, 865–880. doi: 10.1007/s10021-016-9972-3
Mander, L., and Cutts, N. D. (2005). Humber Estuary Wetland Bird Survey. Twelve Months of Low Tide Counts. September 2003 to August 2004. English Nature, Northminster House, Peterborough, PE1 1UA, U.K: Available online at: http://publications.naturalengland.org.uk/publication/98018. (accessed July 14, 2020).
Mander, L., Cutts, N. D., Allen, J., and Mazik, K. (2007). Assessing the development of newly created habitat for wintering estuarine birds. Estuar. Coast. Shelf Sci. 75, 163–174. doi: 10.1016/j.ecss.2007.04.028
Mathot, K. J., Lund, D. R., and Elner, R. W. (2010). Sediment in Stomach Contents of Western Sandpipers and Dunlin Provide Evidence of Biofilm Feeding. Waterbirds Int. J. Waterbird Biol. 33, 300–306. doi: 10.1675/063.033.0305
Mazik, K., Musk, W., Dawes, O., Solyanko, K., Brown, S., Mander, L., et al. (2010). Managed realignment as compensation for the loss of intertidal mudflat: a short term solution to a long term problem? Estuar. Coast. Shelf Sci. 90, 11–20. doi: 10.1016/j.ecss.2010.07.009
Mazik, K., Smith, J. E., Leighton, A., and Elliott, M. (2007). Physical and biological development of a newly breached managed realignment site, Humber estuary, UK. Mar. Pollut. Bull. 55, 564–578. doi: 10.1016/j.marpolbul.2007.09.017
Mendez, V., Gill, J. A., Burton, N. H. K., Austin, G. E., Petchey, O. L., and Davies, R. G. (2012). Functional diversity across space and time: trends in wader communities on British estuaries. Diver. Distrib. 18, 356–365. doi: 10.1111/j.1472-4642.2011.00868.x
Morris, R. K. A. (2013). Managed realignment as a tool for compensatory habitat creation—a re-appraisal. Ocean Coast. Manag. 73, 82–91. doi: 10.1016/j.ocecoaman.2012.12.013
Mossman, H. L., Brown, M. J., Davy, A. J., and Grant, A. (2012b). Constraints on salt marsh development following managed coastal realignment: dispersal limitation or environmental tolerance? Restor. Ecol. 20, 65–75. doi: 10.1111/j.1526-100X.2010.00745.x
Mossman, H. L., Davy, A. J., and Grant, A. (2012a). Does managed coastal realignment create saltmarshes with ‘equivalent biological characteristics’ to natural reference sites? J. Appl. Ecol. 49, 1446–1456. doi: 10.1111/j.1365-2664.2012.02198.x
Newton, I. (2013). Bird Populations (Collins New Naturalist Library, Book 124), Vol. 124. HarperCollins, UK.
Pearce-Higgins, J. W., Brown, D. J., Douglas, D. J. T., Alves, J. A., Bellio, M., Bocher, P., et al. (2017). A global threats overview for Numeniini populations: synthesising expert knowledge for a group of declining migratory birds. Bird Conserv. Int. 27, 6–34. doi: 10.1017/S0959270916000678
Piersma, T., Lok, T., Chen, Y., Hassell, C. J., Yang, H.-Y., Boyle, A., et al. (2016). Simultaneous declines in summer survival of three shorebird species signals a flyway at risk. J. Appl. Ecol. 53, 479–490. doi: 10.1111/1365-2664.12582
Pontee, N. (2014). Accounting for siltation in the design of intertidal creation schemes. Ocean Coast. Manag. 88, 8–12. doi: 10.1016/j.ocecoaman,0.2013.10.014
R Core Team (2019). R: A Language and Environment for Statistical Computing. R Foundation for Statistical Computing. Available online at: https://www.R-project.org/ (accessed June 27, 2019).
Stillman, R. A., Goss-Custard, J. D., and Caldow, R. W. G. (1997). Modelling interference from basic foraging behaviour. J. Anim. Ecol. 66, 692–703. doi: 10.2307/5922
Stroud, D. A., Bainbridge, I. P., Maddock, A., Anthony, S., Baker, H., Buxton, N., et al. (2016). The Status of UK SPAs in the 2000s: the Third Network Review. Peterborough: JNCC.
Studds, C. E., Kendall, B. E., Murray, N. J., Wilson, H. B., Rogers, D. I., Clemens, R. S., et al. (2017). Rapid population decline in migratory shorebirds relying on Yellow Sea tidal mudflats as stopover sites. Nat. Commun. 8:14895. doi: 10.1038/ncomms14895
Sutherland, W. J., Alves, J. A., Amano, T., Chang, C. H., Davidson, N. C., Max Finlayson, C., et al. (2012). A horizon scanning assessment of current and potential future threats to migratory shorebirds. Ibis 154, 663–679. doi: 10.1111/j.1474-919X.2012.01261.x
Turner, R. K., and Schaafsma, M., (eds.) (2015). Coastal Zones Ecosystem Services: From Science to Values and Decision Making. New York, NY: Springer.
Van de Kam, J., Ens, B., Piersma, P., and Zwarts, L. (2004). Shorebirds: an Illustrated Behavioural Ecology. Utrecht: KNNV Publishers.
Whitfield, A. K. (2017). The role of seagrass meadows, mangrove forests, salt marshes and reed beds as nursery areas and food sources for fishes in estuaries. Rev. Fish Biol. Fish 27, 75–110. doi: 10.1007/s11160-016-9454-x
Wolanski, E., and Elliott, M. (2015). Estuarine Ecohydrology: An Introduction. Amsterdam: Elsevier. doi: 10.1016/B978-0-444-63398-9.00001-5
Wood, S. N. (2003). Thin plate regression splines. J. R. Stat. Soc. Ser. B 65, 95–114. doi: 10.1111/1467-9868.00374
Wood, S. N. (2013a). On p-values for smooth components of an extended generalized additive model. Biometrika 100:221–228. doi: 10.1093/biomet/ass048
Wood, S. N. (2013b). A simple test for random effects in regression models. Biometrika 100, 1005–1010. doi: 10.1093/biomet/ast038
Wood, S. N., and Augustin, N. H. (2002). GAMs with integrated model selection using penalized regression splines and applications to environmental modelling. Ecol. Modell. 157, 157–177. doi: 10.1016/S0304-3800(02)00193-X
Woodward, I. D., Calbrade, N. A, and Austin, G. E. (2018). Analysis of Wetland Bird Survey (WeBS) Data for The Humber Estuary SSSI, SAC, SPA and Ramsar site: Third Appraisal—Sector-Level Trends to Winter 2016/17. BTO Research Report No. 709. Thetford: British Trust for Ornithology.
Yang, H. Y., Chen, B., Barter, M., Piersma, T., Zhou, C. F., Li, F. S., et al. (2011). Impacts of tidal land reclamation in Bohai Bay, China: Ongoing losses of critical Yellow Sea waterbird staging and wintering sites. Bird Conserv. Int. 21, 241–259. doi: 10.1017/S0959270911000086
Zhu, Z., Vuik, V., Visser, P. J., Soens, T., van Wesenbeeck, B., van de Koppel, J., et al. (2020). Historic storms and the hidden value of coastal wetlands for nature-based flood defence. Nat. Sustain. 3, 853–862. doi: 10.1038/s41893-020-0556-z
Keywords: waterbirds, monitoring, intertidal habitats, ecological engineering, managed realignment
Citation: Mander L, Scapin L, Thaxter CB, Forster RM and Burton NHK (2021) Long-Term Changes in the Abundance of Benthic Foraging Birds in a Restored Wetland. Front. Ecol. Evol. 9:673148. doi: 10.3389/fevo.2021.673148
Received: 26 February 2021; Accepted: 13 August 2021;
Published: 10 September 2021.
Edited by:
Louise Firth, University of Plymouth, United KingdomReviewed by:
Stephen John Hawkins, University of Southampton, United KingdomCopyright © 2021 Mander, Scapin, Thaxter, Forster and Burton. This is an open-access article distributed under the terms of the Creative Commons Attribution License (CC BY). The use, distribution or reproduction in other forums is permitted, provided the original author(s) and the copyright owner(s) are credited and that the original publication in this journal is cited, in accordance with accepted academic practice. No use, distribution or reproduction is permitted which does not comply with these terms.
*Correspondence: Lucas Mander, bC5tYW5kZXItMjAxN0BodWxsLmFjLnVr
Disclaimer: All claims expressed in this article are solely those of the authors and do not necessarily represent those of their affiliated organizations, or those of the publisher, the editors and the reviewers. Any product that may be evaluated in this article or claim that may be made by its manufacturer is not guaranteed or endorsed by the publisher.
Research integrity at Frontiers
Learn more about the work of our research integrity team to safeguard the quality of each article we publish.