- 1Department of Biology, Centre for Animal Movement Research, Lund University, Lund, Sweden
- 2Institute of Biodiversity and Ecosystem Research, Bulgarian Academy of Sciences, Sofia, Bulgaria
Juvenile songbirds rely on an endogenous program, encoding direction, distance, fueling, and timing of migration. Migratory distance is species-specific, expressed as a period of migratory restlessness, for which the length is correlated with distance, while fueling is modified to meet anticipated flight distances controlled by geomagnetic cues and amount of day-light available for foraging. How daylength affect onset and level of migratory activity and fueling decisions in wild birds have so far received limited attention. Here we study how photoperiod controls onset, level and extent of autumn migratory activity and fueling in juvenile diurnally migrating dunnocks, and nocturnally migrating European robins by experimentally increasing daylength. For both species, we kept a control group indoors at the location of capture in southern Sweden exposed to the natural photoperiod, and an experimental group with increased and advanced photoperiod by 2 h in the morning. Dunnocks initiated migratory activity at sunrise (or artificial sunrise) in both groups, demonstrating a highly responsive and flexible component for the onset of migration triggered by light. Experimental robins anticipated the end of nocturnal migratory activity predicting the earlier sunrise immediately after the time-shift and expressed this behavior already under darkness, supporting a fast-resetting mechanism to the new diel period. Timing of end of morning activity was not affected by the earlier sunrise in both species, suggesting a fixed endogenous control that persisted throughout the 13-day study period. Experimental dunnocks expressed higher overall activity and lower fuel loads than controls, while robins did not change their overall activity and fuel load in response to the shifted and increased photoperiod. These results reveal important adaptations for circadian timekeeping including both a flexible onset open to fast modifications and a more rigid end, with differential effects by the treatment on migratory activity and fueling in the two species.
Introduction
Migratory naïve songbirds rely on an endogenous migration program guiding them from the sites where they are born to their wintering areas (Gwinner, 1975, 1996; Berthold, 1996). The genetic program encode time, distance, direction, and fueling in preparation of migration flights that enable birds to follow population-specific routes (e.g., Berthold and Querner, 1981; Helbig et al., 1989; Berthold, 1996; Helbig, 1996, 2003; Pulido, 2007; Liedvogel et al., 2011). Additional phenotypical adaptations are needed to enable successful long-distance migrations, including morphology, physiology, and behavior (Åkesson and Hedenström, 2007). Several of those adaptations are tightly connected to the endogenous migration program (Gwinner, 1975, 1996; Åkesson et al., 2017) and the ability to navigate (e.g., Emlen, 1975; Able, 1980; Åkesson et al., 2014), while others are related to disposition of time between fueling and flight (Hedenström and Alerstam, 1997).
Both timing and length of the autumn migratory restlessness period have been shown to be correlated with migratory distance in songbirds (Berthold, 1973) and contribute to the individual variation and flexibility in migratory traits observed in birds (Åkesson and Helm, 2020). The periods of controlled migratory restlessness in individual birds are regulated by two environmental cycles, extending over a day or a full year (Aschoff, 1960; Gwinner, 1975, 1996; Gwinner and Brandstätter, 2001). The annual migratory restlessness period is controlled by an endogenous oscillator with external diel input, which in absence of seasonal variations has been shown to free run for several years (Gwinner, 1986). The circadian oscillator is dependent on control mechanisms from the day and night cycle, including variations of light levels (e.g., Aschoff, 1960; Daan, 1976; Gwinner, 1996). The complex circadian pace-making system in birds include three main components (i.e., encephalic photoreceptors, pineal gland, and retina), shown to enable coordination of time over annual and circadian cycles (Gwinner and Brandstätter, 2001).
The migration process involves periods of fueling and flight, which has been predicted to approach a fueling: flight ratio of 7:1 (Hedenström and Alerstam, 1997). Endogenous control of fueling rate and mass increase have been shown to be timed and triggered by geomagnetic information to increase in front of predicted barriers (Fransson et al., 2001), and to be regulated by daylength (Kvist and Lindström, 2000). Migratory restlessness and compass orientation have extensively been investigated in caged songbirds (e.g., Gwinner, 1975, 1986; Åkesson, 1994; Bäckman et al., 1997; Muheim et al., 2006; Helm et al., 2009; Ilieva et al., 2018). However, the combination of recording fueling, and migratory restlessness in the same experimental setup, has so far been limited (Bulte et al., 2017; Ilieva et al., 2018) as well as studies of how daylength affects migration behavior including timing of departure, activity pattern, and fueling (Kvist and Lindström, 2000).
To investigate if diel schedule has any effect on the timing and level of migratory activity, and fueling in juvenile songbirds, we designed an experiment where we experimentally increased the daylength by 2 h in the morning (i.e., prolonged photoperiod shifted forward; Müller et al., 2018b) and kept the geomagnetic parameters at the local site. By this procedure, we could separate the anticipated responses relative to the diel cycle from the geomagnetic cues (i.e., field intensity, angle of inclination) providing latitudinal information (Skiles, 1985). This was important since geomagnetic information have been shown to affect both migratory activity (Ilieva et al., 2018) and fueling (Fransson et al., 2001; Boström et al., 2010) in songbirds. We compared the behavior of the experimental group with control birds kept in the local photoperiod of southern Sweden in autumn. We studied two species of temperate zone passerine migrants captured in autumn on passage to their wintering areas in southwestern Europe, the dunnock, Prunella modularis and the European robin, Erithacus rubecula (Zink, 1975) (hereafter robin). Dunnocks migrate primarily at daytime (Dorka, 1966; Ilieva et al., 2018; Michalik et al., 2020), while the European robin is a strictly nocturnal migrant (Cramp, 1988; Åkesson et al., 2015). The daylength manipulation was substantial (2 h), and therefore out of range from what the species’ normally meet on a daily basis during natural migrations, and in a direction that would be expected during spring rather than during autumn. However, since the period of the increase was abrupt and with a fixed duration (not gradual) it is unlikely that birds will interpret this change as springtime. The length of the light manipulation was selected because we wanted to investigate how the birds reacted to an earlier onset of the day and at the same time reliably measure the time needed for adjusting the clock to a new time. We were interested to investigate if the birds would shift the onset of activity and if the length of the period of migratory restlessness would stay unaffected or change in response to the manipulation. The increase in photoperiod by 2 h correspond to a 1 month shift back in time, or a geographical shift to the south of the expected wintering area in late autumn, where the experimentally increased daylength would occur (Supplementary Figure 1). We expected the two migrants may respond differently to the treatment, since they predominantly migrate at day (dunnock) and at night (robin). The advanced and increased photoperiod was used to investigate if the dunnock responded to the increased daylength by shifting the (fixed) period of migration restlessness in relation to the new sunrise time, or if the sunrise may result in an extended period of expressed migratory activity. For the nocturnally migrating robin we instead expected the earlier sunrise, and thus increased day-length, not to affect the start of migratory restlessness, but rather result in a shorter period of nocturnal restlessness (or no change in length) and an increased fueling rate in response to the increased period available for foraging (Kvist and Lindström, 2000).
We set up the following predictions:
(i) Dunnocks will show the highest activity in the morning, when they naturally migrate (Dorka, 1966; Michalik et al., 2020), and nocturnally migrating robins will be most active at night starting shortly after sunset (Bolshakov et al., 2000, 2007).
(ii) The length of the daily migratory restlessness period will be species-specific, related to the migration phenotype and time of year (Berthold, 1973). Therefore, we predicted that this period will be constant, and only the starting time will change with the earlier sunrise for dunnocks, while robins will not change starting time, but shorten the period of nocturnal restlessness as the migration period at night becomes shorter after light manipulation.
(iii) As an alternative to (ii), we predicted that if the sunrise is an important trigger for the onset of migration independent of a circadian time-keeper regulating the circadian rhythm and the end of the period is set relative to an endogenous dial time sense unaffected by the treatment, dunnocks would respond to the earlier sunrise by extending the period of migratory restlessness, while robins instead should be unaffected by the start and end the restlessness at the same time as controls.
(iv) Fueling rate will be negatively related to level of expressed migratory activity in both species and daylight available for foraging. With an increased daylength, longer diurnal foraging times may thus potentially increased fueling rates in both species (Kvist and Lindström, 2000), but if the daylight is primarily used for migration activity the fueling rate may instead decrease in response to the increased level of overall activity. This may lead to differences in fueling rates between the diurnal and nocturnal migrant.
Materials and Methods
We used two species of temperate zone passerine migrants, the dunnock and the European robin, breeding in large parts of Sweden (Fransson, and Hall-Karlsson, 2008), where different populations are exposed to largely different photoperiods in summer depending on breeding latitude. Dunnock is a diurnal migrant (Dorka, 1966; Michalik et al., 2020) passing through southern Sweden on autumn migration in September and October. Swedish dunnocks migrate to wintering areas in southern France (Zink, 1975; Fransson, and Hall-Karlsson, 2008). The European robin is a nocturnal migrant, captured in large numbers on autumn migration passage in southern Sweden, migrating to wintering areas from Germany and Denmark in the north to North Africa in the south (Zink, 1975; Fransson, and Hall-Karlsson, 2008).
First-year migratory European robins (n = 24) and dunnocks (n = 24) were captured with mist-nets at stopover sites near Stensoffa Ecological Field Station (55°41′N 13°26′E) in southern Sweden on 23–24th September and 6–10th October 2018, respectively. We kept the birds indoors in individual cages for a few days (maximum 5 days) until they were all simultaneously moved to the experimental facility (on 26th September for robins and 11th October for dunnocks) when the experiments started. In the individual cages, the birds were provided with food and water ad libitum.
The experiments were performed in the experimental facilities at the Lund University Stensoffa Ecological Field Station in southwest Sweden (Ilieva et al., 2016). The facility is composed of six wooden houses built in non-magnetic material placed a 5–10 m apart. Each house accommodates four birds in separate circular cages (n = 24 birds in total). For each species, we used three of the houses as control and three houses for altering the photoperiod. All houses have a semi-transparent roof that provided natural light conditions (transmission spectrum visible in Supplementary Figure 2). The daylength was altered adding a LED light source (Lumak Pro; 8,000 lm luminous flux) with daylight color temperature (emitting spectrum in Supplementary Figure 2) in each house that was automatically switched on and off by an electronic timer. The light source was mounted pointing toward the translucent plastic roof of the house, hence, providing indirect illumination to the cages with similar diffuse light as the incoming natural light during daytime. The LED lights were switched on at 07:00 (local sunrise time) in the control houses and 2 h earlier in the treatment houses (05:00) resulting in 2 h longer days for the experimental birds. Light irradiance provided by the lamps was measured on top of the cages with an electronic radiometer (IL 1400A, International Light Technologies, Inc., United States) to be around 0.3 mW/cm2 when complete dark outside and comparable with the light intensity measured soon after sunrise in the houses. The lights were automatically switched off in all houses at 10:00 (local time), when the natural light irradiance was above 4 mW/cm2 (measured under different weather conditions) and the contribution due to the LED lights to the internal luminosity of the houses was not measurable anymore. We introduced the birds into the cages in the early afternoon to familiarize with the new environment and kept them in the cages during 14 days in total.
The cages were designed to electronically measure the weight of the individual birds while sitting on the perch positioned in the middle of the cage (for more details on setup see, Ilieva et al., 2016), and to let an operator provide food and water from the bottom of the cage. With these procedures, the captive birds were not able to see the operator during the weighting and while providing water and food (once a day at 12:00 local time) reducing potential stress-related effects on the birds.
In each house, a network camera was filming all four cages from above and continuously recording videos on external storing devices. Videos were then used to extract the detailed birds’ activity using the computer vision procedure detailed in Ilieva et al. (2018). Briefly, the algorithm automatically detected and tracked the motion of each bird individually and measured the fraction of time that the birds were in flying mode (i.e., excluding any fluttering, jumping or walking) in 20-min intervals. Raw data from the current experiments and extracted by the above procedure are available as Supplementary Appendix 1, and summarized in the actogram in Figure 1. The daily activity recorded for the individual bird was measured as the average number of 20 min active intervals (i.e., intervals when the bird was flying for more than 1 min; Ilieva et al., 2018), and presented for the full experimental day (from 12:00 to 12:00 of the next day, local time), but also divided into first half of the day (from 00:00 to 12:00) and second half of the day (from 12:00 to 00:00) periods (Figure 2). In addition, we performed statistical tests in intervals before the sunrise time (either at natural or experimentally manipulated time) to investigate whether nocturnal activity in European robins was affected by the expected sunrise time. We finally tested both species for difference in “departure” and “arrival” times calculated relative to the overall diurnal activity for dunnocks (between midnight and feeding time: 00:00–12:00, local time) and nocturnal activity for robins (between sunset and sunrise: 19:00–07:00). The departure (i.e., start of migratory activity) was defined as the time at which each individual reached the 10th percentile of the cumulative activity in the considered migratory period that has been demonstrated to be an accurate predictor by Schmaljohann et al. (2015). Similarly, the arrival (i.e., end of migratory activity) was defined as the time at which the individual reached the 90th percentile of the cumulative activity, that is, when the bird is close to landing and possibly searching for shelter or a foraging ground.
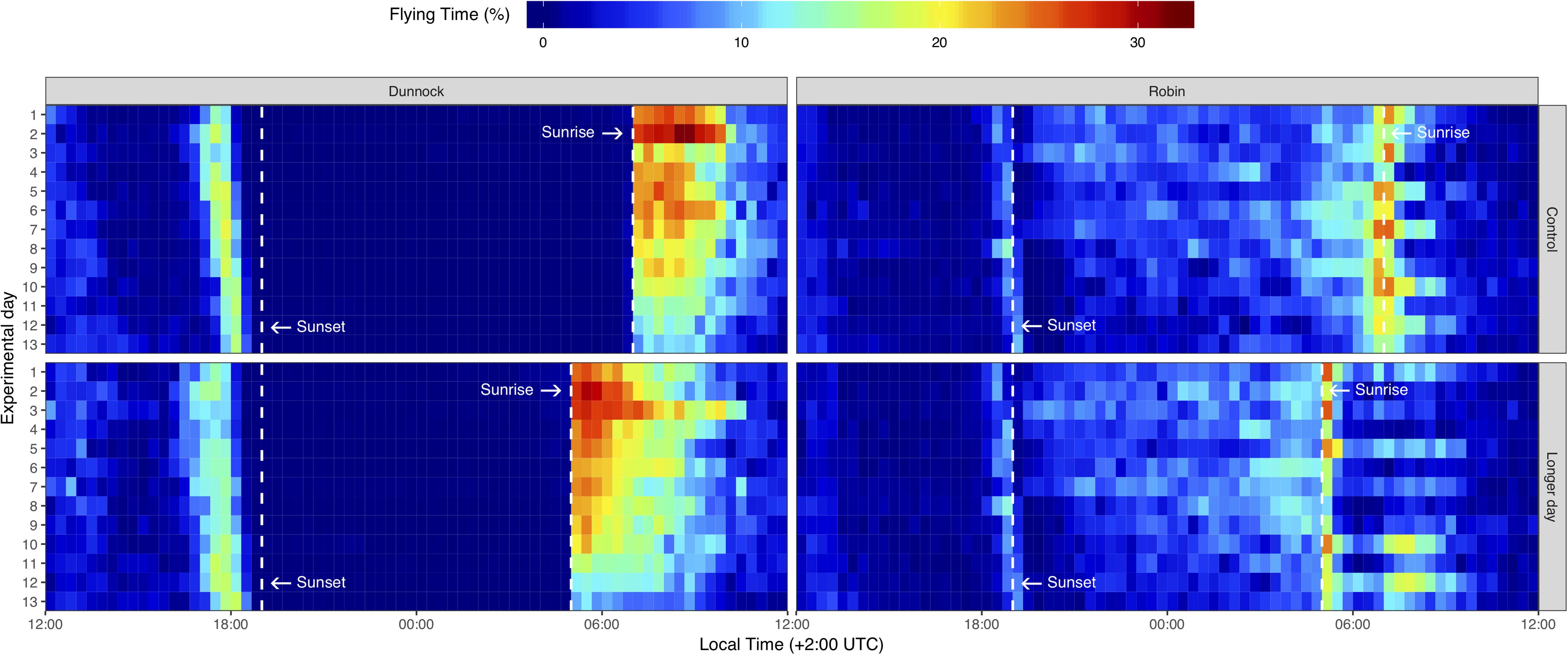
Figure 1. Actogram of first-year migratory dunnocks (Prunella modularis) and European robins (Erithacus rubecula) monitored under local daylength timing (Control), and experimentally 2 h advanced early sunrise (Longer day). Each color-coded horizontal line shows the mean time spent in flying mode by 12 birds per group (n = 24 for each species) from 12:00 of each day to 12:00 of the successive day. Dashed vertical lines indicate the time of sunset (19:00 for all groups), as well as sunrise at local time (07:00 for the Control groups) and 2 h earlier (05:00 for the Longer day groups).
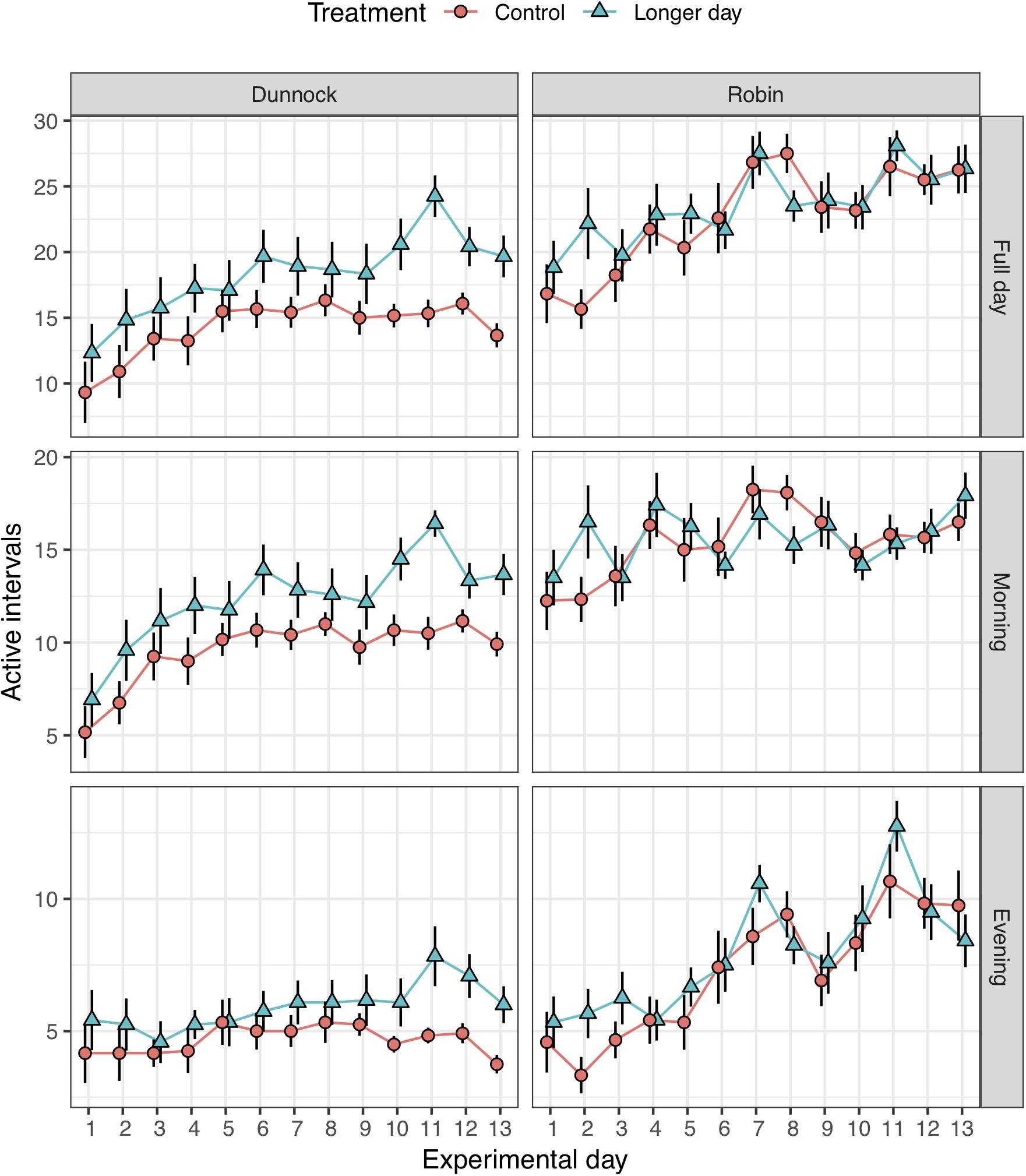
Figure 2. Average (±SE) daily activity of first-year migratory dunnocks (Prunella modularis) and European robins (Erithacus rubecula) monitored under local daylength timing (Control) and early sunrise (Longer day) conditions (n = 12 for each treatment group; n = 24 for each species). The daily activity was measured as the average number of 20-min active intervals (i.e., intervals when birds were flying for more than 1 min) presented for the full day, and divided in morning and evening periods.
All the plots and statistical tests were done in the software R version 3.6.1 (R Core Team, 2019). One-way and repeated-measures ANOVA were used for testing differences in activity, food intake, fuel load and arrival/departure time between the treatment and control groups of each species.
Results
The actograms show clear differences in activity patterns between dunnocks and robins (Figure 1), with the highest activity in the first half of the day starting at sunrise for dunnocks, and a prolonged period with elevated level of activity during the entire night for robins. The actograms also show a strong effect of the treatment on the timing of activity in both species.
Dunnocks showed no activity at night and initiated their migratory activity at sunrise (Figure 1). Thereafter, the activity continued for several hours, and ended before noon. The start of activity coincided with local sunrise time for the control group exposed to the local diel cycle and was initiated 2 h before for the experimental group (a time corresponding to the apparent sunrise time in this treatment group; Figure 1). Dunnocks in the local photoperiod were active for the rest of the day and expressed a peak of increased activity just before sunset (Figure 1). The highest level of activity in dunnocks was expressed in the first half of the day, starting at sunrise and ending before noon (Figure 1). The experimental dunnocks expressed a similar temporal pattern of activity throughout the day, but with an extended morning activity period starting at the experimentally advanced sunrise and ending at the normal time before noon. The morning activity (higher) is primarily associated with migration, while afternoon activity (lower) is associated with foraging in dunnocks.
Robins expressed a short peak of activity just before sunset, which for this nocturnal migratory species is expected to be associated with preparations for the migratory departure. Immediately after this peak of activity at sunset, the robins showed a restricted period of lower activity corresponding to a “quiescence period,” before the elevated nocturnal restlessness period started. Robins were active for the entire night, but with a reduced activity level, and showed an increased activity ending with a peak at sunrise (Figure 1). The timing of the peak of morning activity for the robins, probably associated with the end of the daily migratory activity and search for shelter, was affected by the treatment. That is, for the control group the peak of activity was at local sunrise time (07:00), whereas, for the experimental group the peak of activity occurred almost 2 h earlier around 05:00 when lamps were turned on for the experimental group (Figure 1; see also below). Both robins held in the local and extended photoperiod continued to be active during the mornings, and showed relatively limited activity during the afternoons (Figure 1).
To investigate whether there were differences in restlessness between groups, we analyzed the duration of activity (i.e., number of active intervals), and we found that dunnocks exposed to experimentally increased photoperiod were more active than the control group (Figure 2). The difference was significant during mornings (repeated measures ANOVA, days 1–13: F1,22 = 4.394, p = 0.048), but not during evenings (F1,22 = 2.239, p = 0.149). The higher activity during the morning, is a consequence of the fact that dunnocks in the daylength treatment group started their migratory activity earlier (see above) but continued to be active until around 10:00 which is similar to the time when migratory activity ceased in the control group (Figure 1; see also below).
Robins, on the other hand, were not affected in their overall activity by the increased photoperiod with earlier sunrise and did not change activity pattern (p > 0.486 for all time periods; Figure 2). However, we investigated if the peak activity at sunrise for robins (see above) was only affected by an external factor (i.e., the earlier sunrise of the treatment) or by an internal factor (i.e., birds can predict time of sunrise with their circadian clock). We compared the activity of the control group with the experimental group during 5 h before the sunrise, either at natural sunrise timing (07:00) or at the artificial sunrise time (05:00). The activity of robins 5 h before the natural sunrise (02:00–07:00) was not affected by treatment (repeated measures ANOVA, days 1–13: F1,22 = 1.515, p = 0.231). However, the activity 5 h before the artificial sunrise (00:00–05:00) showed that the experimental group was significantly more active than the control group (F1,22 = 8.887, p = 0.007), with higher level of activity for the experimental group. The latter difference was measured before any light was yet present in the houses. We did not find this response in the dunnocks relative to the earlier sunrise, as they expressed no activity at night (Figure 1).
As predicted by the actogram in Figure 1, we found a strong effect on time for onset of migratory activity between the control and the experimental group in dunnocks (repeated measures ANOVA, days 1–13: F1,22 = 106.4, p < 0.001) with the experimental group starting to express migratory restlessness earlier following the artificial sunrise (Figure 3). We did, however, not find any time difference in the end of restlessness for the dunnocks (F1,22 = 2.04, p = 0.167). Furthermore, we did not find any difference in start of migration activity between the control and the experimental group in robins (F1,22 = 0.005, p = 0.945; Figure 3), but we did find that the end of activity for the experimental group was consistently earlier than the control group (F1,22 = 47.33, p < 0.001), and this difference lasted during the entire experimental period (Figure 3).
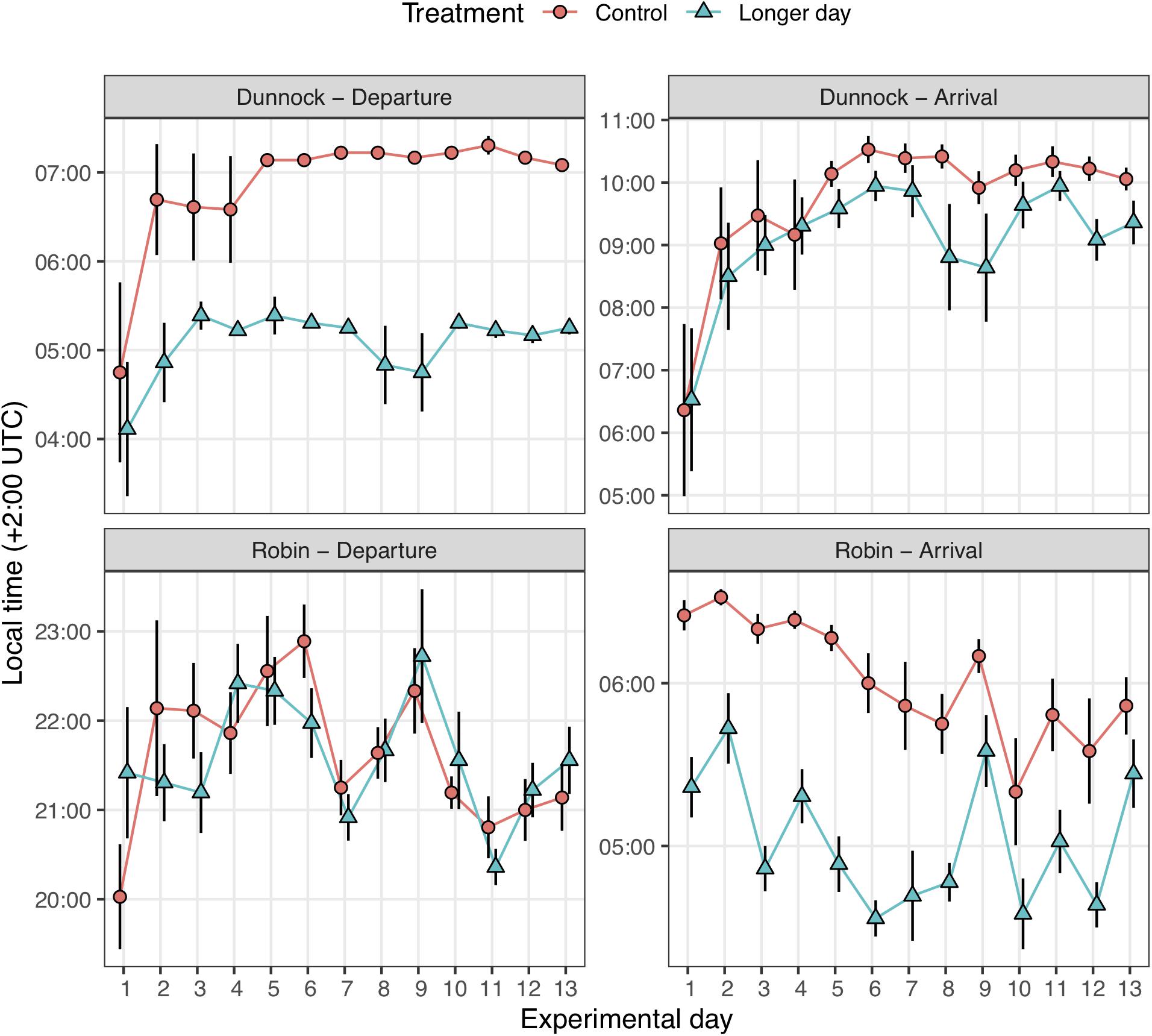
Figure 3. Time (±SE) of migratory departure and arrival for first-year migratory dunnocks (Prunella modularis) and European robins (Erithacus rubecula) monitored under local daylength timing (Control) and 2 h experimentally advanced early sunrise (Longer day) conditions (n = 12 for each treatment group; n = 24 for each species). For information on categories, see main text.
We also measured how body mass, foraging activity and fueling varied across the experimental period, as well as between the experimental groups for the two species. There were no initial body mass differences between the control and experimental groups for both dunnocks (control: mean ± SD: 21.8 ± 3.0 g; treatment: 21.4 ± 1.6 g; one-way ANOVA: F1,22 = 0.139, p = 0.713) and robins (control: 16.9 ± 0.9 g; treatment: 17.0 ± 1.3 g; F1,22 = 0.096, p = 0.759), nor was there a significant difference between groups in food intake throughout the experiment (repeated measures ANOVA, days 1–13: dunnocks: F1,22 = 2.488, p = 0.129; robins: F1,22 = 0.064, p = 0.802; Figure 4). Both species showed a tendency to increase body mass during the experimental period (Figure 4). However, the fuel load (i.e., fraction of body mass increase relative to individual’s lean body mass) for dunnocks, was significantly lower for the daylength treatment group as compared to the control group (repeated measures ANOVA, days 1–13: F1,22 = 5.806, p = 0.025; Figure 4), but there was no difference in fuel load between the two groups for robins (F1,22 = 0.029, p = 0.866; Figure 4).
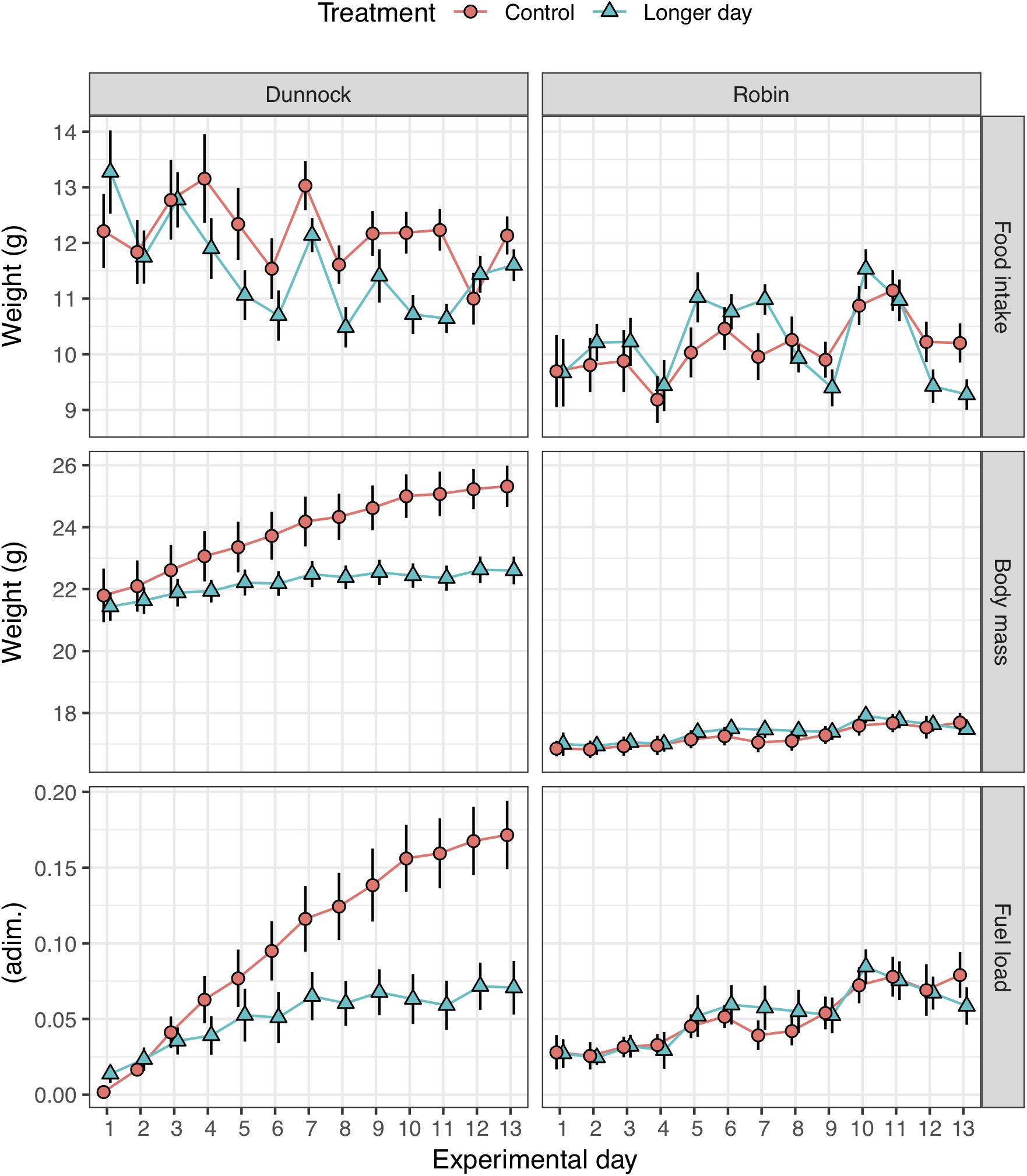
Figure 4. Average (±SE) of food intake, body mass and fuel load for first-year migratory dunnocks (Prunella modularis) and European robins (Erithacus rubecula) monitored under local daylength timing (Control), and 2 h experimentally advanced early sunrise (Longer day) conditions (n = 12 for each treatment group; n = 24 for each species).
Discussion
Dunnocks
The dunnocks expressed the highest activity in the early morning, as confirmed in our previous study (Ilieva et al., 2018), which corresponds to the time when dunnocks normally migrate (Dorka, 1966; Michalik et al., 2020). We found that the experimental juvenile dunnocks responded to our earlier onset of sunrise (increased photoperiod) experiment, by increasing the period of diurnal migratory activity, in contrast to what has been proposed for diurnal animals (Daan, 1976). This change occurred instantly and was prolonged during the complete study period lasting 13 days. Dunnocks in the local photoperiod further expressed a peak of increased activity just before sunset, which presumably involves foraging and movements to find shelter for resting during the upcoming night (Ilieva et al., 2018). At the same time, the dunnocks exposed to an increased photoperiod reduced their fuel load, which likely was a result of the increased activity.
Our results show that the time for initiation of migratory activity was flexible and possible to experimentally advance in dunnocks. The ending of the diurnal migratory activity, however, occurred at a similar time for both the experimental group and controls ending just before noon (ca. 10:00–11:00 local time; Figures 1, 3), suggesting rigid endogenous control. It, thus, seems that in diurnally migrating dunnocks the sunrise time and associated change of light level dictates the start of the migratory activity (functioning as an external trigger; DeCoursey, 1960, 1961), and an internal circadian clock dictates when the migratory activity ends rather than an inherited period length of activity. The timing of the end of activity, furthermore, did not seem to be affected by the experimentally prolonged photoperiod over time, but rather stayed constant over the 13-day study period. These results provide support that the circadian timekeeper in migratory dunnocks contain both flexible (start) and rigid (end) parts, not affected by changes of photoperiod. Inherited migration programs of diurnally migrating birds have received limited attention in experimental work so far (Åkesson and Helm, 2020). Our study of dunnocks, thus, provide new information on how photoperiodic changes affect onset of migratory activity, the length of it, and how the end is kept constant despite an increased photoperiod, with an increased level of activity and reduced fueling as a result.
A flexible light-dependent control mechanism triggering migratory activity (DeCoursey, 1960, 1961), will enable adaptive responses to local photoperiods and weather conditions during migrations (e.g., Åkesson et al., 2001, 2002; Sjöberg et al., 2015, 2017; Müller et al., 2016), but may also be an important regulator of speed of migration (Hedenström and Alerstam, 1998; cf. Lindström et al., 2019) by controlling fueling rates (Kvist and Lindström, 2000) and energy consumed. Such a light-dependent triggering mechanism affecting migratory activity may, however, also be vulnerable to human impact from light pollution as reported for nocturnal bird migrants (e.g., La Sorte et al., 2017; Horton et al., 2019), with elevated risk of mortality and fatal conservation consequences (Van Doren et al., 2017; McLaren et al., 2018). The influence of artificial light on ecosystems are multiple and widespread (Gaston et al., 2013) and will predictably be strong for diurnal bird migrants as shown in our experiments with migratory dunnocks, leading to extended activity periods, and reduced fueling rates with likely negative fitness consequences. Therefore, a more extensive study of this problem with respect to artificial lights in natural environments would be of great interest for the future.
European Robins
The European robins in our experiments showed strictly nocturnal migratory activity, which has been observed in previous experiments in our facility (Bianco et al., 2019), and reduced activity at daytime (mid-day and afternoon). The robins also expressed an increased peak of activity associated with the time of sunset, as well as with the early morning hours (Figure 1). The sunset peak of activity was followed by a period of lower activity, i.e., a quiescence period, before the nocturnal migration restlessness was initiated (Figure 1), and this period is expected to be associated with preparations for migration departure observed in birds both in captivity (e.g., Watts et al., 2017) and during natural migrations (e.g., Hebrard, 1971; Åkesson et al., 1996a; Bolshakov et al., 2007; Schofield et al., 2018). The time for nocturnal migration departure shows substantial inter-individual variation for different bird species (Hebrard, 1971; Åkesson et al., 1996a; Bolshakov et al., 2007; Schofield et al., 2018), where several ecological factors such as internal state, stopover time, and weather likely have important influences on the timing of migration (e.g., Åkesson and Hedenström, 2000; Åkesson et al., 2001, 2002; Bolshakov et al., 2007; Sjöberg et al., 2015, 2017; Müller et al., 2016). Migratory intensity, recorded in diurnal and nocturnal free-flying songbird migrants, follows a predictable temporal pattern relative to sunset and sunrise with increased migration traffic rate starting at civil twilight at dusk, with the peak activity recorded 2–4 h thereafter, and again starting 1 h before civil twilight at dawn with continued activity after (Zehnder et al., 2001; Åkesson et al., 2001). European robins may initiate the migration at different times of the night, and show the highest migration activity during the second half of the night as shown for tracked birds in field studies (Åkesson et al., 1996a; Bolshakov et al., 2000, Bolshakov et al., 2007). Our data from diurnally migrating dunnocks initiating their migration activity at sunrise, and nocturnally migrating robins showing the highest activity starting 1–2 h after local sunset and continuing for the rest of the night support these findings.
We found an interesting difference in response to the increased and advanced photoperiod for the robins associated with one of the two sunrise and sunset activity peaks. Robins were not affected in the start of the migration activity period by the advanced and increased day length, but they were affected in the timing of end of migration activity. Since the pre-dawn activity increased when the birds were still in the dark, it means that the experimental robins were able to predict the new sunrise time in their house immediately after the first day experiencing a shifted sunrise time. The time of sunrise was anticipated before any light was present in the houses and supports the hypothesis that the robins were able to use their circadian time sense to predict the artificial sunrise time and adjust their migratory activity accordingly. Our experiments suggest that this response was expressed instantly, involving unexpected flexibility (Figures 1, 3), in line with the findings reported by DeCoursey (1960, 1961) for flying squirrels reacting to a short pulse of light by increasing activity. Thus, our results suggest that indeed the robins were affected in their behavior by the extended morning period, not in the overall daily activity, but in the timing of their activity including the activity expressed before sunrise. This light-level-trigger associated with the night-day transition could for nocturnally migrating robins be used to decide the landing time after a nocturnal migratory flight, and the increased desire to move at daybreak may be used to visually find safe shelter, to avoid diurnal predators and search for good foraging sites (Alerstam, 1978). In coastal areas, re-orientation by nocturnal avian migrants to sheltered areas have frequently been observed in early morning hours, and may be associated with movements to inland stop-over sites (e.g., Åkesson et al., 1996b, 2001; Zehnder et al., 2002; Nilsson and Sjöberg, 2016).
Fueling and Activity
We found an overall but limited increase in mass and fueling rate in our control birds over the study period. At the same time, there was no effect on mass gain by the increased photoperiod in robins, and a very strong negative effect on fueling in dunnocks. In the experimental dunnocks, the decreased fueling rate was associated with an increased level and prolonged period of migratory activity expressed during the day. Time of season has been shown to affect fueling decisions and mass gain in caged songbird migrants (Kullberg et al., 2003, 2007), with a higher rate in late birds. Availability of food has further been shown to affect the level of migratory activity in songbirds, resulting in reduced activity after food being presented ad libitum (Biebach, 1985; Gwinner et al., 1985). All our control and experimental birds were given access to water and food ad libitum during the study period, and still we observed an increased level of activity in dunnocks in response to the earlier sunrise, suggesting the light cue was more important as a trigger of migratory activity than food availability. It was further interesting to note that the experimental robins did not explore the increased daylength for foraging, to increase the fueling rate, as has been predicted for bird migrants (Kvist and Lindström, 2000). Instead, it seems like the fueling rate may be controlled by other factors for the robin, which may be interesting to study in the future. In line with this, Maggini and Bairlein (2010) found that different populations of Northern wheatears (Oenanthe oenanthe) expressed migratory activity in relation to anticipated migration distance while fueling were related to the distance of barrier crossings which differed between populations. Thus, the two components, activity and fueling, seems to be controlled by different factors in the wheatears, leading to adaptive levels of fueling during migration instead of maximizing fuel intake at any given time. One additional factor limiting fueling rate (mass gain) could, for instance, also be the risk of being taken by a predator, which would lead to reduced fueling in response to increased predation risk (Lind et al., 2010).
Photoperiodic Time-Keeping as an Adaptation to Control Migration
Our experimental treatment, increasing day length by 2 h is far off what our species are experiencing during natural migrations at this time of year, but have been used in previous experiments with songbirds (Müller et al., 2018b). Daylength may provide information on latitude, and as such potentially also anticipated migration distance, passed, and remaining. Our experiments were performed with caged songbird migrants during the autumn equinox period, when birds naturally will experience no difference in day length between latitudes at the autumn equinox (23 September), but fast changing day length near to this date, and different lengths of the twilight period depending on latitude (Daan and Aschoff, 1975). Despite this, we found very pronounced effects on timing and level of migratory activity in diurnal dunnocks, and a shift in timing-related activity peaks in nocturnal robins in response to the 2 h earlier sunrise. Since we kept the geomagnetic parameters (i.e., field intensity and angle of inclination) at local values, we can be sure that the birds responded to the daylength manipulations and not the geomagnetic field.
It is important for migratory birds to time their movements with respect to environmental conditions, geographical areas and circannual cycles, and to manage this an efficient control mechanism will be needed (Åkesson et al., 2017). Migratory birds seem to have evolved a complex navigational toolbox, shaped by generations of successful exploration of wide geographical space (Wehner, 1998). Still, several of the navigational tools used for global navigation remain unknown, and underlying sensory mechanisms and the ways they are combined even more so. Clearly space and time needs to be combined in the endogenous migration program of birds (Gwinner, 1986, 1996; Wallraff, 1990; Wehner, 1998). Latitudinal information can be provided by geophysical information from the geomagnetic field (total field intensity and angle of inclination; Skiles, 1985), and have been shown to affect fueling and migratory activity in juvenile songbirds (e.g., Fransson et al., 2001; Boström et al., 2010; Ilieva et al., 2018). However, a photoperiodic control mechanism with high adaptability to the transition between day and night may further assist birds to adjust to the local time on migration. Such mechanism may for instance be part of the re-setting of the time-compensated sun compass prior to migration flights (Alerstam and Pettersson, 1991). It may also trigger level and extent of migratory activity as suggested here, and could contribute to a photoperiodic control mechanism recording daylength that could be used to adjust the endogenous program to local cues (Müller et al., 2016). At this point, we cannot exclude that a flexible diel control mechanism may be combined with the perception of geomagnetic information to define latitude, and we suggest that this possible association should be studied in the future.
Our results have revealed some interesting differences between two passerine species migrating similar distances, along similar southwestern routes and at the same time of year but adapted to migrate at different time of day. Still, there are several aspects of their endogenous migration program that would be interesting to investigate more closely in the future, such as the effect of decreased (and increased) photoperiod on activity and fueling rate. Comparative experiments on these two and other species of songbird migrants outside of the equinox period may also be of priority since species-specific differences in circadian pace making may be expected (Gwinner and Brandstätter, 2001). It will also be interesting to study how different phenotypic components related to the endogenous migration program may be affected by an extended daylength at sunset rather than at sunrise. In such scenario, we may at first expect opposite responses between diurnal and nocturnal songbird migrants as observed here, but also potentially species- and population-specific adaptations depending on migration timing and distance (Maggini and Bairlein, 2010). We hope that future research will reveal in what aspects and level of detail migration programs and control mechanisms vary between species, and how these adaptations have evolved to cope with differences in migration routes and strategies for different bird species and populations (Müller et al., 2018a). We believe that our experimental setting applied here may be a useful approach to resolve some of these challenging and still open questions on the functional characteristics of endogenous migration programs that can be applied to birds and other animals.
Data Availability Statement
The original contributions presented in the study are included in the article/Supplementary Material, further inquiries can be directed to the corresponding author.
Ethics Statement
The animal study was reviewed and approved by Malmö/Lund Ethical Committee for scientific work on animals (Dnr 5.8.18-12719/2017), the Swedish Board of Agriculture for housing facilities (Dnr 5.2.18-5398/16), and work with animals (Dnr 5.2.18-10992/18).
Author Contributions
MI performed the fieldwork with support from GB and SÅ. GB analyzed the data. All authors conceived the study, discussed the outcome of the results, and wrote the first draft of the manuscript.
Funding
The project received financial support from the Swedish Research Council (621-2013-4361; 2016-05342), Carl Tryggers Stiftelse för Vetenskaplig Forskning (17-518) to SÅ, and the Centre for Animal Movement Research (CAnMove) financed by a Linnaeus grant (349-2007-8690) from the Swedish Research Council and Lund University.
Conflict of Interest
The authors declare that the research was conducted in the absence of any commercial or financial relationships that could be construed as a potential conflict of interest.
Acknowledgments
We would like to thank Christoffer Sjöholm for assistance during fieldwork, and Johan Bäckman, Xier Chen, Elena Martinez Hernandez, and Shujie Lang for help with light measurements.
Supplementary Material
The Supplementary Material for this article can be found online at: https://www.frontiersin.org/articles/10.3389/fevo.2021.666176/full#supplementary-material
References
Able, K. P. (1980). “Mechanisms of orientation, navigation and homing,” in Animal Migration, Orientation and Navigation, ed. S. A. Gauthreaux (New York, NY: Academic Press), 283–373. doi: 10.1016/b978-0-08-091833-4.50010-9
Åkesson, S. (1994). Comparative orientation experiments with different species of passerine long-distance migrants: effect of magnetic field manipulation. Anim. Behav. 48, 1379–1393. doi: 10.1006/anbe.1994.1374
Åkesson, S., Alerstam, T., and Hedenström, A. (1996a). Flight initiation of nocturnal passerine migrants in relation to celestial orientation conditions at twilight. J. Avian Biol. 27, 95–102. doi: 10.2307/3677138
Åkesson, S., Boström, J., Liedvogel, M., and Muheim, R. (2014). “Animal navigation,” in Animal Movement Across Scales, eds L.-A. Hansson and S. Åkesson (Oxford: Oxford University Press), 151–178.
Åkesson, S., and Hedenström, A. (2000). Wind selectivity of migratory flight departures in birds. Behav. Ecol. Sociobiol. 47, 140–144. doi: 10.1007/s002650050004
Åkesson, S., and Hedenström, A. (2007). How migrants get there: migratory performance and orientation. BioScience 57, 123–133. doi: 10.1641/b570207
Åkesson, S., and Helm, B. (2020). Endogenous programs and flexibility in bird migration. Front. Ecol. Evol. 8:78. doi: 10.3389/fevo.2020.00078
Åkesson, S., Ilieva, M., Karagicheva, J., Rakhimberdiev, E., Tomotani, B., and Helm, B. (2017). Timing avian long-distance migration: from internal clock mechanisms to global flights. Phil. Trans. R. Soc. B 372:20160252. doi: 10.1098/rstb.2016.0252
Åkesson, S., Karlsson, L., Walinder, G., and Alerstam, T. (1996b). Bimodal orientation and the occurrence of temporary reverse bird migration during autumn in south Scandinavia. Behav. Ecol. Sociobiol. 38, 293–302. doi: 10.1007/s002650050245
Åkesson, S., Odin, C., Hegedüs, R., Ilieva, M., Sjöholm, C., Farkas, A., et al. (2015). Testing avian compass calibration: comparative experiments with diurnal and nocturnal passerine migrants in South Sweden. Biol. Open 4, 35–47. doi: 10.1242/bio.20149837
Åkesson, S., Walinder, G., Karlsson, L., and Ehnbom, S. (2001). Reed warbler orientation: initiation of nocturnal migratory flights in relation to visibility of celestial cues at dusk. Anim. Behav. 61, 181–189. doi: 10.1006/anbe.2000.1562
Åkesson, S., Walinder, G., Karlsson, L., and Ehnbom, S. (2002). Nocturnal migratory flight initiation in reed warblers Acrocephalus scirpaceus: effect of wind on orientation and timing of migration. J. Avian Biol. 33, 349–357. doi: 10.1034/j.1600-048x.2002.02951.x
Alerstam, T. (1978). Reoriented bird migration in coastal areas: dispersal to suitable resting grounds? Oikos 30, 405–408. doi: 10.2307/3543491
Alerstam, T., and Pettersson, S. G. (1991). Orientation along great circles by migrating birds using a sun compass. J. Theor. Biol. 152, 191–202. doi: 10.1016/s0022-5193(05)80452-7
Aschoff, J. (1960). Exogenous and endogenous components in circadian rhythms. Cold Spr. Harb. Symp. Quant. Biol. 25, 11–28. doi: 10.1101/sqb.1960.025.01.004
Bäckman, J., Pettersson, J., and Sandberg, R. (1997). The influence of fat stores on magnetic orientation in day−migrating chaffinch, Fringilla coelebs. Ethology 103, 247–256. doi: 10.1111/j.1439-0310.1997.tb00120.x
Berthold, P. (1973). Relationships between migratory restlessness and migration distance in six Sylvia species. Ibis 115, 594–599. doi: 10.1111/j.1474-919x.1973.tb01998.x
Berthold, P., and Querner, U. (1981). Genetic basis of migratory behavior in European warblers. Science 212, 77–79. doi: 10.1126/science.212.4490.77
Bianco, G., Ilieva, M., and Åkesson, S. (2019). Magnetic storms disrupt nocturnal migratory activity in songbirds. Biol. Lett. 15:20180918. doi: 10.1098/rsbl.2018.0918
Biebach, H. (1985). Sahara stopover in migratory flycatchers: fat and food affect the time program. Experientia 41, 695–697. doi: 10.1007/bf02007727
Bolshakov, C. V., Bulyuk, V. N., and Sinelschikova, A. (2000). Study of nocturnal departures in small passerine migrants: retrapping of ringed birds in high mist-nets. Vogelwarte 40, 250–257.
Bolshakov, C. V., Chernetsov, N., Mukhin, A., Bulyuk, V. N., Kosarev, V., Ktitorov, P., et al. (2007). Time of nocturnal departures in European robins, Erithacus rubecula, in relation to celestial cues, season, stopover duration and fat stores. Anim. Behav. 74, 855–865. doi: 10.1016/j.anbehav.2006.10.024
Boström, J. E., Fransson, T., Henshaw, I., Jakobsson, S., Kullberg, C., and Åkesson, S. (2010). Autumn migratory fuelling: a response to simulated magnetic displacements in juvenile wheatears, Oenanthe oenanthe. Behav. Ecol. Sociobiol. 64, 1725–1732. doi: 10.1007/s00265-010-0985-1
Bulte, M., Heyers, D., Mouritsen, H., and Bairlein, F. (2017). Geomagnetic information modulates nocturnal migratory restlessness but not fueling in a long distance migratory songbird. J. Avian Biol. 48, 75–82. doi: 10.1111/jav.01285
Daan, S. (1976). Light intensity and the timing of daily activity of finches (Fringillidae). Ibis 118, 223–236. doi: 10.1111/j.1474-919x.1976.tb03068.x
Daan, S., and Aschoff, J. (1975). Circadian rhythms of locomotor activity in captive birds and mammals: their variations with season and latitude. Oecologia 18, 269–316. doi: 10.1007/bf00345851
DeCoursey, P. J. (1960). Daily rhythm of light sensitivity in a rodent. Science 131, 33–35. doi: 10.1126/science.131.3392.33
DeCoursey, P. J. (1961). Effect of light on the circadian activity rhythm of the flying squirrel, Glaucomys volans. Z. Vergl. Physiol. 44, 331–354. doi: 10.1007/bf00388033
Dorka, V. (1966). Das jahres- und tageszeitliche Zugmuster von Kurz- und Langstreckenziehern nach Beobachtungen auf den Alpenpassen Cou/Bretolet (Wallis). Orn. Beob. 63, 165–223.
Emlen, S. T. (1975). The stellar-orientation system of a migratory bird. Sci. Am. 233, 102–111. doi: 10.1038/scientificamerican0875-102
Fransson, T., and Hall-Karlsson, S. (2008). “Svensk Ringmärkningsatlas,” in Tättingar, (Stockholm: SOF förlag), 3.
Fransson, T., Jakobsson, S., Johansson, P., Kullberg, C., Lind, J., and Vallin, A. (2001). Bird migration: magnetic cues trigger extensive refuelling. Nature 414, 35–36. doi: 10.1038/35102115
Gaston, K. J., Bennie, J., Davies, T. W., and Hopkins, J. (2013). The ecological impacts of nighttime light pollution: a mechanistic appraisal. Biol. Rev. 88, 912–927. doi: 10.1111/brv.12036
Gwinner, E. (1975). “Circadian and circannual rhythms in birds,” in Avian Biology, Vol. 5, eds D. S. Farner and J. R. King (New York, NY: Academic Press), 221–285. doi: 10.1016/b978-0-12-249405-5.50012-4
Gwinner, E. (1986). Circannual Rhythms. Endogenous Annual Clocks in the Organization of Seasonal Processes. Berlin: Springer.
Gwinner, E. (1996). Circadian and circannual programmes in avian migration. J. Exp. Biol. 199, 39–48.
Gwinner, E., Biebach, H., and Kries, I. V. (1985). Food availability affects migratory restlessness in caged garden warblers (Sylvia borin). Naturwissenschaften 72, 51–52. doi: 10.1007/bf00405336
Gwinner, E., and Brandstätter, R. (2001). Complex bird clocks. Phil. Trans. R. Soc. B 356, 1801–1810. doi: 10.1098/rstb.2001.0959
Hebrard, J. J. (1971). The nightly initiation of passerine migration in spring: a direct visual study. Ibis 113, 8–18. doi: 10.1111/j.1474-919x.1971.tb05119.x
Hedenström, A., and Alerstam, T. (1998). How fast can birds migrate? J. Avian Biol. 29, 424–432. doi: 10.2307/3677161
Hedenström, A., and Alerstam, T. (1997). Optimum fuel loads in migratory birds: distinguishing between time and energy minimization. J. Theor. Biol. 189, 227–234. doi: 10.1006/jtbi.1997.0505
Helbig, A. J. (1996). Genetic basis, mode of inheritance and evolutionary changes of migratory directions in palearctic warblers (Aves: Sylviidae). J. Exp. Biol. 199, 49–55.
Helbig, A. J. (2003). “Evolution of bird migration: a phylogenetic and biogeographic perspective,” in Avian Migration, eds P. Berthold, E. Gwinner, and E. Sonnenschein (Berlin: Springer), 3–20. doi: 10.1007/978-3-662-05957-9_1
Helbig, A. J., Berthold, P., and Wiltschko, W. (1989). Migratory orientation in blackcaps (Sylvia atricapilla): population-specific shifts of direction during the autumn. Ethology 82, 307–315. doi: 10.1111/j.1439-0310.1989.tb00510.x
Helm, B., Schwabl, I., and Gwinner, E. (2009). Circannual basis of geographically distinct bird schedules. J. Exp. Biol. 212, 1259–1269. doi: 10.1242/jeb.025411
Horton, K. G., Nilsson, C., Van Doren, B. M., La Sorte, F. A., Dokter, A. M., and Farnsworth, A. (2019). Bright lights in the big cities: migratory birds’ exposure to artificial light. Front. Ecol. Environm. 17:209–214. doi: 10.1002/fee.2029
Ilieva, M., Bianco, G., and Åkesson, S. (2016). Does migratory distance affect fuelling in a medium-distance passerine migrant: results from direct and step-wise simulated magnetic displacements. Biol. Open 5, 272–278. doi: 10.1242/bio.014779
Ilieva, M., Bianco, G., and Åkesson, S. (2018). Effect of geomagnetic field on migratory activity in a diurnal passerine migrant, the dunnock, Prunella modularis. Anim. Behav. 146, 79–85. doi: 10.1016/j.anbehav.2018.10.007
Kullberg, C., Henshaw, I., Jakobsson, S., Johansson, P., and Fransson, T. (2007). Fuelling decisions in migratory birds: geomagnetic cues override the seasonal effect. Proc. R. Soc. B Biol. Sci. 274, 2145–2151. doi: 10.1098/rspb.2007.0554
Kullberg, C., Lind, J., Fransson, T., Jakobsson, S., and Vallin, A. (2003). Magnetic cues and time of season affect fuel deposition in migratory thrush nightingales (Luscinia luscinia). Proc. R. Soc. B Biol. Sci. 270, 373–378. doi: 10.1098/rspb.2002.2273
Kvist, A., and Lindström, Å (2000). Maximum daily energy intake: it takes time to lift the metabolic ceiling. Physiol. Biochem. Zool. 73, 30–36. doi: 10.1086/316719
La Sorte, F. A., Fink, D., Buler, J. J., Farnsworth, A., and Cabrera−Cruz, S. A. (2017). Seasonal associations with urban light pollution for nocturnally migrating bird populations. Global Change Biol. 23, 4609–4619. doi: 10.1111/gcb.13792
Liedvogel, M., Åkesson, S., and Bensch, S. (2011). The genetics of migration on the move. Trends Ecol. Evol. 26, 561–569.
Lind, J., Jakobsson, S., and Kullberg, C. (2010). “Impaired predator evasion in the life history of birds: behavioral and physiological adaptations to reduced flight ability,” in Current Ornithology, Vol. 17, ed. C. F. Thompson (New York, NY: Springer), 1–30. doi: 10.1007/978-1-4419-6421-2_1
Lindström, Å, Alerstam, T., and Hedenström, A. (2019). Faster fuelling is the key to faster migration. Nat. Clim. Change 9:288. doi: 10.1038/s41558-019-0443-7
Maggini, I., and Bairlein, F. (2010). Endogenous rhythms of seasonal migratory body mass changes and nocturnal restlessness in different populations of northern wheatear Oenanthe oenanthe. J. Biol. Rhythms 25, 268–276. doi: 10.1177/0748730410373442
McLaren, J. D., Buler, J. J., Schreckengost, T., Smolinsky, J. A., Boone, M., Emiel van Loon, E., et al. (2018). Artificial light at night confounds broad−scale habitat use by migrating birds. Ecol. Lett. 21, 356–364. doi: 10.1111/ele.12902
Michalik, B., Brust, V., and Hüppop, O. (2020). Are movements of daytime and nighttime passerine migrants as different as day and night? Ecol. Evol. 10, 11031–11042. doi: 10.1002/ece3.6704
Muheim, R., Moore, F. R., and Phillips, J. B. (2006). Calibration of magnetic and celestial compass cues in migratory birds-a review of cue-conflict experiments. J. Exp. Biol. 209, 2–17. doi: 10.1242/jeb.01960
Müller, F., Eikenaar, C., Crysler, Z. J., Taylor, P. D., and Schmaljohann, H. (2018a). Nocturnal departure timing in songbirds facing distinct migratory challenges. J. Anim. Ecol. 87, 1102–1115. doi: 10.1111/1365-2656.12821
Müller, F., Rüppel, G., and Schmaljohann, H. (2018b). Does the length of the night affect the timing of nocturnal departures in a migratory songbird? Anim. Behav. 141, 183–194. doi: 10.1016/j.anbehav.2018.05.018
Müller, F., Taylor, P. D., Sjöberg, S., Muheim, R., Tsvey, A., Mackenzie, S. A., et al. (2016). Towards a conceptual framework for explaining variation in nocturnal departure time of songbird migrants. Movem. Ecol. 4, 1–12.
Nilsson, C., and Sjöberg, S. (2016). Causes and characteristics of reverse bird migration: an analysis based on radar, radio tracking and ringing at Falsterbo. Sweden. J. Avian Biol. 47, 354–362. doi: 10.1111/jav.00707
Pulido, F. (2007). The genetics and evolution of avian migration. BioScience 57, 165–174. doi: 10.1641/b570211
R Core Team (2019). R: A Language and Environment for Statistical Computing. Vienna: R Foundation for Statistical Computing.
Schmaljohann, H., Kämpfer, S., Fritzsch, A., Kima, R., and Eikenaar, C. (2015). Start of nocturnal migratory restlessness in captive birds predicts nocturnal departure time in free-flying birds. Behav. Ecol. Sociobiol. 69, 909–914. doi: 10.1007/s00265-015-1902-4
Schofield, L. N., Deppe, J. L., Diehl, R. H., Ward, M. P., Bolus, R. T., Zenzal, T. J., et al. (2018). Occurrence of quiescence in free-ranging migratory songbirds. Behav. Ecol. Sociobiol. 72:36.
Sjöberg, S., Alerstam, T., Åkesson, S., and Muheim, R. (2017). Ecological factors influence timing of departures in nocturnally migrating songbirds at Falsterbo, Sweden. Anim. Behav. 127, 253–269. doi: 10.1016/j.anbehav.2017.03.007
Sjöberg, S., Alerstam, T., Åkesson, S., Schulz, A., Weidauer, A., Coppack, T., et al. (2015). Weather and fuel reserves determine departure and flight decisions in passerines migrating across the Baltic Sea. Anim. Behav. 104, 59–68. doi: 10.1016/j.anbehav.2015.02.015
Skiles, D. D. (1985). “The geomagnetic field its nature, history, and biological relevance,” in Magnetite Biomineralization and Magnetoreception in Organisms, eds J. L. Kirschvink, D. S. Jones, and B. J. MacFadden (Boston, MA: Springer), 43–102. doi: 10.1007/978-1-4613-0313-8_3
Van Doren, B. M., Horton, K. G., Dokter, A. M., Klinck, H., Elbin, S. B., and Farnsworth, A. (2017). High-intensity urban light installation dramatically alters nocturnal bird migration. Proc. Nat. Acad. Sci. U.S.A. 114, 11175–11180. doi: 10.1073/pnas.1708574114
Wallraff, H. G. (1990). Conceptual approaches to avian navigation systems. Experientia 46, 379–388. doi: 10.1007/978-3-0348-7208-9_7
Watts, H. E., Robart, A. R., Chopra, J. K., Asinas, C. E., Hahn, T. P., and Ramenofsky, M. (2017). Seasonal expression of migratory behavior in a facultative migrant, the pine siskin. Behav. Ecol. Sociobiol. 71:9.
Wehner, R. (1998). Navigation in context: grand theories and basic mechanisms. J. Avian Biol. 29, 370–386. doi: 10.2307/3677156
Zehnder, S., Åkesson, S., Liechti, F., and Bruderer, B. (2001). Nocturnal autumn bird migration at Falsterbo, South Sweden. J. Avian Biol. 32, 239–248. doi: 10.1111/j.0908-8857.2001.320306.x
Zehnder, S., Åkesson, S., Liechti, F., and Bruderer, B. (2002). Observation of free-flying nocturnal migrants at Falsterbo: occurrence of reverse flight directions in autumn. Avian Sci. 2, 103–113.
Keywords: circadian rhythm, daylength, endogenous migration program, photoperiod, migration
Citation: Åkesson S, Ilieva M and Bianco G (2021) Flexibility and Control of Circadian Activity, Migratory Restlessness and Fueling in Two Songbird Migrants. Front. Ecol. Evol. 9:666176. doi: 10.3389/fevo.2021.666176
Received: 09 February 2021; Accepted: 22 March 2021;
Published: 14 April 2021.
Edited by:
Ivan Maggini, University of Veterinary Medicine Vienna, AustriaReviewed by:
Nikita Chernetsov, Zoological Institute (RAS), RussiaEmily Cohen, University of Maryland Center for Environmental Science (UMCES), United States
Copyright © 2021 Åkesson, Ilieva and Bianco. This is an open-access article distributed under the terms of the Creative Commons Attribution License (CC BY). The use, distribution or reproduction in other forums is permitted, provided the original author(s) and the copyright owner(s) are credited and that the original publication in this journal is cited, in accordance with accepted academic practice. No use, distribution or reproduction is permitted which does not comply with these terms.
*Correspondence: Susanne Åkesson, c3VzYW5uZS5ha2Vzc29uQGJpb2wubHUuc2U=