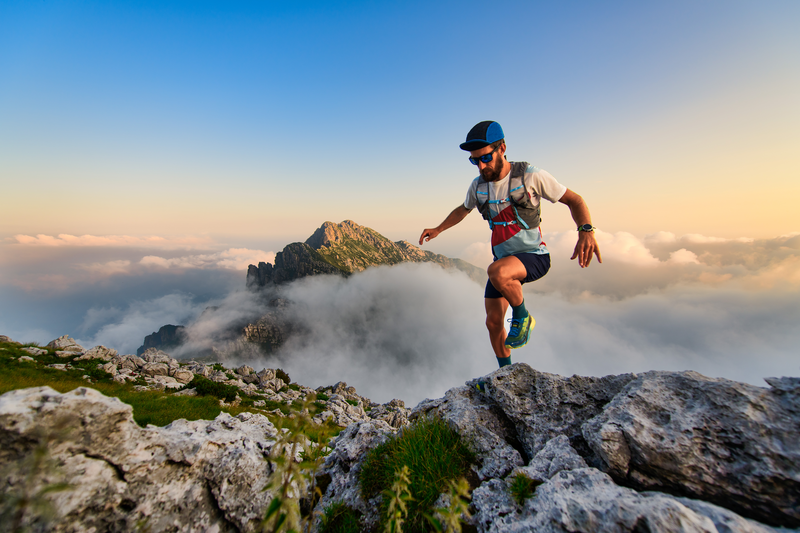
95% of researchers rate our articles as excellent or good
Learn more about the work of our research integrity team to safeguard the quality of each article we publish.
Find out more
ORIGINAL RESEARCH article
Front. Ecol. Evol. , 10 December 2021
Sec. Conservation and Restoration Ecology
Volume 9 - 2021 | https://doi.org/10.3389/fevo.2021.660513
Encroachment of rush Juncus spp. in the United Kingdom uplands poses a threat to declining wader populations due to taller, denser swards that can limit foraging and breeding habitat quality for some species. Rush management via cutting, implemented through agri-environment schemes (AESs), could thus increase wader abundance, but there is insufficient assessment and understanding of how rush management influences upland waders. Across two upland regions of England [South West Peak (SWP) and Geltsdale nature reserve, Cumbria], we surveyed waders over four visits in fields where rush was managed according to AES prescriptions (treatment; n = 21) and fields without rush management that were otherwise ecologically similar (control; n = 22) to assess how the densities of breeding wader pairs respond to rush management in the short-term. We find evidence for regional variation in the response of waders to rush management, with densities of Common Snipe Gallinago gallinago significantly higher in treatment than control fields in the SWP, but not Geltsdale. There were no statistically significant responses to treatment on densities of Eurasian Curlew Numenius arquata or Northern Lapwing Vanellus vanellus. The 95% confidence intervals for the treatment parameter estimates suggest that this may be due to limited statistical power in the case of Lapwing. For Curlew, however, any potential increases in densities are negligible. There was no evidence that variation in rush cover, which ranged from 10 to 70%, influenced densities of any of our three focal species. Our results suggest that rush management through AES prescriptions delivered in isolation of other interventions may not lead to general increases in breeding wader densities in the short-term, but benefits may arise in some situations due to regional and inter-specific variation in effectiveness. Rush management supported with interventions that improve soil conditions and thus food availability, or reduce predation pressure, may enable AES rush management to generate benefits. Additional research is required to maximise the potential benefits of rush management for each species through the development of prescriptions that tailor to individual species’ optimum sward structure.
Waders are one of several taxonomic groups of farmland breeding birds that have undergone severe Europe-wide declines in recent decades (PECBMS, 2020), with species threatened at both the national level (e.g., Common Snipe Gallinago gallinago; Amber-listed, United Kingdom; Eaton et al., 2015) and international level (e.g., Eurasian Curlew Numenius arquata and Northern Lapwing Vanellus vanellus; globally Near Threatened; IUCN, 2020). In the United Kingdom, wader population declines are occurring throughout the lowlands and uplands, with the latter containing the majority of remaining grassland-breeding wader populations (Wilson A. M. et al., 2005; Balmer et al., 2013; Siriwardena et al., 2017). Poor nest and chick survival, primarily attributable to predation and habitat degradation, are thought to be the major drivers of these declines (Roodbergen et al., 2012; Franks et al., 2018; Roos et al., 2018). Land use change including agricultural intensification, and to a lesser extent land abandonment, is driving degradation of wader habitat in United Kingdom upland regions (Baines, 1988; Fuller and Gough, 1999; Amar et al., 2011; Silcock et al., 2012; Douglas et al., 2017; Johnstone et al., 2017).
To prevent further degradation of grasslands, agri-environment schemes (AESs) encourage farming practices that benefit breeding waders through improved habitat quality (O’Brien and Wilson, 2011; Smart et al., 2013, 2014; Franks et al., 2018). Such AES prescriptions have exhibited mixed success; population trends of some wader species have been reversed at the local level, yet nationally wader population declines continue (O’Brien and Wilson, 2011; Smart et al., 2013, 2014; Siriwardena et al., 2017; Franks et al., 2018). One mechanism used in these AES prescriptions is the manipulation of vegetation structure (e.g., Natural England, 2018) with the aim of creating a mosaic of short and tall vegetation that is beneficial for foraging and breeding waders (Wilson J. D. et al., 2005). Due to the substantial inter-specific variation in wader breeding habitat requirements, creation of a mosaic aims to simultaneously provide suitable habitat for several wader species. Lapwing, for example, favour short swards with a few tussocks (Baines, 1988; Milsom et al., 2000; Durant et al., 2008), whereas Curlew and Snipe may be more tolerant of a range of sward structures with a greater preference for taller vegetation (Baines, 1988; Pearce-Higgins and Grant, 2006; Hoodless et al., 2007; Durant et al., 2008).
A recent threat to the maintenance of structurally heterogeneous grasslands in the United Kingdom uplands is encroachment by Juncus spp. (hereafter termed “rush”), with rush frequency approximately doubling between 2005 and 2018; multiple factors relating to agricultural grassland management and changes in climate have likely driven this encroachment (Ashby et al., 2020). Rush encroachment could significantly contribute to wader population declines by creating expanses of tall, dense, rush-dominated swards. It could consequently restrict physical access to the soil for foraging (Devereux et al., 2004; Robson and Allcorn, 2006), and reduce waders’ ability to detect predators and thus their willingness to breed and forage in such locations (Whittingham and Evans, 2004; Robson and Allcorn, 2006). Although, the taller, denser vegetation generated by increased rush cover could provide nests and chicks with greater concealment from predators (Valkama et al., 1998; Kelly et al., 2021).
Rush management prescriptions within AES have been developed to address the adverse impacts of rush encroachment (Natural England, 2018). These prescriptions, which typically comprise a long-term aim to reduce the extent of dense rush swards within a field to <30%, involve mowing, aftermath grazing, and occasionally herbicide application (precise prescriptions deviate slightly between United Kingdom countries; Natural England, 2012; Welsh Government, 2017; Shellswell and Humpidge, 2018). In the short-term, rush management opens up the sward and reduces vegetation height and density (Kelly et al., 2021). There is, however, little published data supporting the assumed beneficial impacts of these changes in sward structure on breeding waders. Whilst previous studies suggest that targeted rush management, or cutting of rank vegetation including rush, can increase wader abundance, these studies do not experimentally compare areas with and without rush management and in some cases are combined with additional interventions (Holton and Allcorn, 2006; Robson and Allcorn, 2006; Douglas et al., 2017). Consequently, there is insufficient assessment and understanding of how rush management influences upland waders, despite the importance of evaluating the effectiveness of AES prescriptions (Kleijn and Sutherland, 2003).
Here, we assess how the number of breeding wader pairs responds to rush management in the short-term by surveying waders in treatment fields (where rush is managed according to AES prescriptions) and control fields (without rush management) across two upland regions of England. We first test whether field size and environmental conditions that could influence wader abundance [rush cover, Holton and Allcorn (2006); Robson and Allcorn (2006); soil conditions (pH, moisture, and penetration resistance), Smart et al. (2006, 2008), Hoodless et al. (2007), McCallum et al. (2016); and woodland distance, Douglas et al. (2014), Wilson et al. (2014)] are similar between control and treatment fields. We then test whether the density of breeding wader pairs differs between treatment and control fields whilst accounting for environmental conditions and region, and test if the effects of rush management vary between regions and with the amount of rush cover.
This study was conducted during the wader breeding season (April–June 2019) in the south–west of the Peak District National Park (South West Peak, hereafter “SWP”), and Geltsdale nature reserve in Cumbria (hereafter “Geltsdale”; Figure 1). Both regions support important breeding wader populations including Curlew, Lapwing, and Snipe (Carr, 2009; Balmer et al., 2013; Douglas et al., 2017). Temperatures during the winter period preceding our surveys were not below long-term averages (1981–2010) and thus the densities of waders at our focal sites are unlikely to have been unduly influenced by cold weather-related mortality. Survey fields within the two regions were characteristic of United Kingdom upland farmed landscapes and were mostly semi-improved pasture with a smaller number of unimproved pasture, hay meadow and “white moor” fields (rough grassland with rush and Molinia). The dominant rush species was Juncus effusus with smaller amounts of other species present at some sites, particularly Juncus acutiflorus and Juncus conglomeratus at Geltsdale.
Figure 1. The two study regions showing locations of treatment fields (rush management, light grey) and control fields (no rush management, dark grey).
The study design is described in full by Kelly et al. (2021). Field selection was performed without prior knowledge on wader use of the selected fields, and was based on fields meeting our criteria on rush management, spatial configuration and obtaining permission from landowners to conduct the research. Treatment fields were selected if fields had received rush management between autumn 2018 and spring 2019 (fields may also have received management in previous years) following the EK4 and EL4 rush cutting prescriptions in Entry Level Stewardship (Natural England, 2012). These are standard AES prescriptions that are applicable to any field with at least one-third rush cover, including those on nature reserves. Rush management in treatment fields involved cutting one-third of the rush present once or twice annually on rotation (Supplementary Table 1 provides more information on the AES prescriptions). Control fields were selected if fields had not undergone rush management in the previous 2 years, had a similar extent of rush cover to treatment fields (mean rush cover ± standard error, treatment = 46.70 ± 3.67%, control = 40.00 ± 4.21%; Mann–Whitney test: W = 186, P = 0.255) and were in close proximity to treatment fields (mean distance = 90 ± 34 m standard error; Figure 1 and Supplementary Table 2). Information on field rush cover was provided by the landowners prior to selection of our survey fields. For both treatment and control fields, we only selected those that had greater than one-third rush cover so that all survey fields, regardless of treatment, qualified for the AES rush management prescriptions. Control fields were not deliberately selected to contain different levels of rush cover than treatment fields and thus both control and treatment fields constituted a representative sample of the rush cover in fields with and without AES rush management. Rush cover in our survey fields was subsequently assessed during fieldwork and varied across fields from 10 to 70% (three control fields contained less than 30% rush cover). In the SWP, there were 12 treatment and 13 control fields (one treatment field that had initially been selected was excluded as insufficient rush cutting had been conducted) and at Geltsdale, there were 9 treatment and 9 control fields, giving a total sample size of 21 treatment and 22 control fields (Figure 1).
We estimated the number of breeding wader pairs using a modified version of the standard field-by-field survey method of O’Brien and Smith (1992). Four visits were made to each survey field – two visits in the early breeding season (SWP: 16th–28th April; Geltsdale: 5th–18th May) and two visits in the late breeding season (SWP: 28th May–18th June; Geltsdale: 21st–25th June). Successive visits within the early or late breeding season were on average 7 days apart in the early breeding season and 6 days apart in the late breeding season. All visits were conducted by one researcher to ensure consistency of survey estimates and thus both regions could not be surveyed concurrently. As Geltsdale is at a higher latitude than the SWP (Figure 1), the wader breeding season commences slightly later in the former region. Survey fields in the SWP were thus visited first in both the early and late breeding seasons. Moreover, surveys were not undertaken during the first hour after sunrise or last hour before sunset, or in heavy rain, fog (<250 m visibility) or wind greater than Beaufort Force 5.
Within each field, observations were made along a survey route that started 50 m from the field edge and took the observer to within 50 m of every part of the field. All individual waders were marked on a field map with symbols to note behaviour that indicates breeding status. Surveys recorded Lapwing, Curlew, Snipe, and Common Redshank Tringa totanus, but the latter was only detected in three fields at Geltsdale (two control and one treatment) and is not considered further.
An index of the number of breeding pairs of each species per field was calculated using standard species-specific criteria. For all species, groups of more than four individuals were excluded as these may represent non-breeding flocks (following Sim et al., 2005; Douglas et al., 2021). For Lapwing, we divided the maximum number of individuals across the two early breeding season visits by two (detectability of Lapwing is high and this approach follows O’Brien and Smith, 1992; Bolton et al., 2011; O’Brien and Wilson, 2011; Smart et al., 2014). For Curlew and Snipe, two birds together, or a single (detectability of these species is expected to be lower than that of Lapwing), either in a field or associating with the field (displaying or mobbing birds above the field) were treated as a pair (following O’Brien and Smith, 1992; Henderson et al., 2002; Hoodless et al., 2006; Pearce-Higgins and Grant, 2006). The number of pairs were then estimated as the maximum per-visit number across all four visits (following Green, 1985; Smart et al., 2008; O’Brien and Wilson, 2011; Buchanan et al., 2017; Douglas et al., 2017). The restricted date range of visits used for calculating Lapwing pairs, compared to Snipe and Curlew, follows standard protocols (O’Brien and Smith, 1992; Bolton et al., 2011; O’Brien and Wilson, 2011; Smart et al., 2014). Estimates of breeding Snipe pairs from diurnal records of Snipe heard (drumming or chipping) or seen, rather than crepuscular surveys of Snipe heard drumming, are likely to be reliable in regions such as our survey locations where Snipe do not occur at very high densities, as suggested by Hoodless et al. (2006).
Rush cover was estimated once per field to the nearest 10% from multiple vantage points during the early breeding season when more accurate estimates can be obtained due to lower vegetation height [note that whilst rush grows tall, it typically spreads relatively slowly in horizontal extent (Ashby et al., 2020) and thus any spread in extent of rush cover within a field is negligible during the survey period]. Field size (ha) was measured in ArcMap™ (v10.4.1; Esri, Redlands, CA, United States) using 1:25,000 Ordnance Survey maps (Ordnance Survey, 2019). Straight-line distance (km) from the centroid of each survey field to the nearest block of woodland (defined as areas with >20% tree cover, from Land Cover Map 2015; Rowland et al., 2017) was measured using the “Near (Analysis)” tool as woodland proximity can influence breeding wader distributions and abundance (Douglas et al., 2014; Wilson et al., 2014).
Soil conditions (penetration resistance, moisture content, and pH) were measured during one early, and one late, breeding season visit to account for potential seasonal variation. Soil penetration resistance (kgF) and soil moisture content (%) were recorded at three locations within each field (field centre and two randomly selected locations toward opposite ends of the field) and at two separate points (approximately 15 cm apart) at each of these three locations – giving six measurements per field on each of the two visits. Soil penetration resistance was measured, following Green (1988), using a soil penetrometer with a 5 mm diameter metal pressure rod (20 kg Pesola macro-line spring scale and pressure set, NHBS, Devon, England). Soil moisture content (%) was measured using a soil moisture sensor and readout meter (SM150T soil moisture sensor and HH150 readout meter, Delta-T Devices, Cambridge, England). This sensor had a maximum measurement threshold of 85% and when this threshold was exceeded, we used a value of 92.5% (the mid-point between this threshold and 100%). Soil pH was recorded, using a direct soil pH meter (HI-12922 HALO wireless soil pH electrode, Hanna Instruments, Woonsocket, RI, United States), at one of the points at each of the three locations – giving three measurements per field on each visit and six measurements per field overall.
Mean soil penetration resistance, soil moisture, and soil pH values were calculated per field for the early breeding season visit (for use in models of the number of Lapwing pairs as these are estimated using data from the early breeding season only), and across the overall breeding season (for use in Curlew and Snipe models as these use data from all site visits). We note, however, that early breeding season and overall breeding season soil conditions were highly correlated (soil penetration resistance: r = 0.958; soil moisture: r = 0.931; soil pH: r = 0.919; P < 0.001 and n = 43 in all cases).
All analyses were conducted in R version 3.6.3 (R Core Team, 2020).
We tested whether treatment and control fields had similar environmental conditions. We fitted generalised linear models (GLMs) with a Gaussian error structure and identity link that modelled each environmental variable [rush cover (%), soil penetration resistance (kgF), soil pH, soil moisture (%), woodland distance (km), and field size (ha; natural logarithm transformed prior to inclusion in the models to remove the influence of outliers due to its skewed distribution)] as a function of treatment (treatment or control field) whilst accounting for region (SWP or Geltsdale).
We modelled the density of breeding waders for each species by constructing GLMs with a response variable of the estimated number of breeding pairs per field with a Poisson error structure (log link) and field size (ha; natural logarithm transformed) as an offset in all models. This offset variable converts wader pairs into densities and ensures that field size is accounted for within the models. McFadden’s pseudo-R2 was calculated to represent model fit.
We first ran preliminary checks for simple non-linear effects of our environmental variables [rush cover (%), soil penetration resistance (kgF), soil moisture (%), soil pH, and woodland distance (km)] by modelling each species’ density as a function of the selected environmental variable linear term (linear models), and linear and quadratic terms (quadratic models), whilst including region as a fixed factor and field size (ha; natural logarithm transformed) as an offset. There was no strong evidence for non-linear associations, defined as Akaike information criterion value corrected for small sample sizes (AICc) of a quadratic model being two points or more lower than that of a linear model (Supplementary Table 3) and all subsequent modelling thus used only linear terms.
Following these preliminary checks, we followed Whittingham et al. (2006) and constructed a full model of the main effects to test the prediction that rush management increased wader density, i.e., that there were significantly higher densities in treatment than control fields, whilst accounting for other environmental variables (Supplementary Table 4). For each species, we modelled estimated breeding pairs as a function of treatment (treatment or control field), region (SWP or Geltsdale), rush cover, soil moisture, soil pH, soil penetration resistance, and woodland distance, with field size (ha; natural logarithm transformed) as an offset. Early breeding season soil conditions were included in the Lapwing models, and overall breeding season soil conditions were included in the Curlew and Snipe models to match the survey dates that were used to estimate the number of breeding pairs of these species (see wader surveys section above).
In addition to the main effects full model, we constructed two extra models that also included (1) the interaction between rush cover and treatment/control field (to test if the effects of rush management varied across different amounts of rush cover), or (2) the interaction between region and treatment/control field (to test if rush management effects differed between regions; which could be the case if the factors regulating population size or the capacity of populations to respond to management vary regionally).
For each species, we compared the three full model types (main effects only, main effects plus treatment and rush cover interaction, and main effects plus treatment and region interaction) using each model’s AICc, and when interaction terms were present their statistical significance (using a P < 0.05 threshold) (Supplementary Table 5). For Curlew and Lapwing, the main effects only models had the lowest AICc values and interaction terms were not significant; inference is thus based solely on the main effects only model as there is no evidence that the effects of treatment varied with region or rush cover. For Snipe, the model with the lowest AICc value was that with the treatment and region interaction [ΔAICc relative to model with the next lowest AICc (main effects only model) = 3.605, interaction term P = 0.009]. The interaction term’s parameter estimate did, however, have a very large standard error (SE = 3621.325) demonstrating uncertainty in its effect size and we thus also report the results from the full model that only contains the main effects (Table 1).
Table 1. Generalised linear models of breeding wader pair density for Curlew, Lapwing, and Snipe – treatment, region, rush cover, soil conditions (moisture, pH, and penetration resistance), and woodland distance were included as predictor variables, with field size (natural logarithm transformed) included as an offset.
Environmental conditions (rush cover, soil penetration resistance, soil moisture, woodland distance, and field size) did not differ significantly between treatment and control fields, except for soil pH (Supplementary Tables 2, 6). In both the early and overall breeding season metrics, treatment fields had slightly more alkaline soil (mean soil pH ± standard error; early breeding season: treatment = 5.36 ± 0.15, control = 4.87 ± 0.13; overall breeding season: treatment = 5.34 ± 0.15, control = 4.88 ± 0.14).
Models that took region, rush cover, woodland distance, and soil conditions into account found no evidence that the density of Curlew pairs varied between treatment and control fields [Figures 2A,B and Table 1; profile 95% confidence interval (CI) for treatment parameter estimate = −0.73 to 1.12]. Rush cover, which varied from 10 to 70% (Supplementary Table 4), was not associated with breeding Curlew densities (Table 1). Similarly, there was no evidence that Lapwing pair densities differed between control and treatment fields or were influenced by rush cover (Figures 2C,D and Table 1; profile 95% CI for treatment parameter estimate = −0.76 to 1.91) – although it is important to note that Lapwings were extremely rare in the SWP survey fields, being observed in just a single control field (Supplementary Table 7). For Snipe, when treatment was modelled as an interaction with region, there were higher Snipe densities in treatment fields than control fields in the SWP but similar densities in the treatment and control fields at Geltsdale, and no evidence that rush cover was associated with Snipe densities (Figure 2G and Table 1). When the interaction between treatment and region was excluded from the model there was no evidence that Snipe pair densities differed between treatment and control fields or were influenced by rush cover (Figures 2E,F and Table 1; profile 95% CI for treatment parameter estimate = −0.64 to 2.09).
Figure 2. Poisson model (main effects only models) predicted breeding wader pair densities in control fields and treatment fields within Geltsdale [left hand column; n = 18 fields (9 control and 9 treatment)] and the SWP [right hand column; n = 25 fields (13 control and 12 treatment)] when taking into account rush cover, woodland distance, and soil conditions (moisture, pH, and penetration resistance) for Curlew (A,B), Lapwing (C,D), and Snipe (E,F). Bars represent model predicted densities, and errors represent model predicted 95% confidence intervals. The best fitting model (judged by AICc values) for Snipe densities included an interaction between region and treatment with model predicted densities (G) being represented by triangles (SWP) and circles (Geltsdale); error bars again represent 95% confidence intervals but note that for Snipe densities in SWP control fields these are infinite due to singularity issues with the model as no Snipe were observed in such fields.
There were trends, albeit only marginally non-significant ones, for higher densities of Curlew and Snipe in fields with more alkaline soil conditions (Table 1). Snipe densities were also higher in fields with wetter soils and in the SWP than Geltsdale (Table 1, Figure 2, and Supplementary Figures 1, 2). No other environmental variables influenced breeding wader pair densities (Table 1).
Our results reveal potential regional variation in the short-term response of Snipe breeding densities to AES rush management prescriptions, with benefits arising from rush management in the SWP but not Geltsdale. Certainty around the strength of this effect is, however, limited by the large standard error around the interaction term’s parameter estimate. When regional variation is omitted, there is no firm evidence for benefits of rush management. Breeding Lapwing densities also did not appear to be significantly influenced in the short-term by rush management. Yet, positive impacts on breeding densities cannot be excluded for either Snipe or Lapwing as the 95% CIs for the treatment parameter estimate suggest that the largest plausible values are approximately two (Snipe = 2.09; Lapwing = 1.91). For Curlew, we found negligible evidence for positive effects of rush management on breeding densities in the short-term (no significant effect; 95% CIs indicate that the largest plausible treatment parameter estimate is 1.12).
Whilst our findings are not indicative of strong, and regionally uniform increases in Lapwing and Snipe breeding densities arising from rush management, they do suggest that these species are more likely to respond positively than Curlew, especially in the case of Snipe. This is perhaps logical given (1) the preference of nesting Lapwing for short, open vegetation (Baines, 1988; Milsom et al., 2000; Durant et al., 2008) that is generated by rush cutting (Robson and Allcorn, 2006; Kelly et al., 2021), and (2) smaller- and medium-bodied species (Snipe and Lapwing, respectively) may be particularly negatively impacted by taller and denser swards that will obscure their view and thus ability to detect predators (limiting their willingness to forage and nest in such habitats) to a greater extent than larger species such as Curlew (Devereux et al., 2004; Whittingham and Evans, 2004; Wilson J. D. et al., 2005).
There is evidence for regional variation in Snipe responses to AES rush management, with Snipe densities being higher in treatment than control fields in the SWP but not Geltsdale. Such situations are expected to arise if there is regional variation in the extent to which habitat availability regulates Snipe populations. Snipe densities were significantly higher in the SWP than Geltsdale. This situation could arise if most of the habitat with structurally suitable vegetation is occupied in the SWP, whilst other regulating factors limit the Geltsdale population and prevent it from occupying all suitable habitat, including that created through AES rush management. Indeed, the general lack of strong evidence for beneficial impacts of rush management could highlight that habitat improvements alone will not enable breeding densities to increase because other factors are regulating population sizes (Smart et al., 2013). This links to the buffer effect, through which there is higher likelihood that high quality habitat remains unoccupied (Kluyver and Tinbergen, 1954; Brown, 1969; Gunnarsson et al., 2005). Thus, our results will be most applicable to wader populations at similar or lower densities to those at our study sites and we cannot exclude the possibility that rush management impacts would be greater in populations whose size is regulated by availability of fields with suitable vegetation structure. Given that increased nest and chick predation rates are a key driver of wader declines (Roodbergen et al., 2012; Roos et al., 2018), management may be required that simultaneously tackles rush encroachment and predation pressure to enable wader populations to recover and respond positively to AES rush management – especially for Curlew which exhibited negligible evidence for increased densities in response to rush management.
Alternatively, rush management may not be creating sufficiently optimal conditions for some of our focal wader species to generate consistent and detectable increases in breeding densities. Some AES prescriptions aim to reduce rush cover within a field to <30% (e.g., Natural England, 2018), yet all treatment fields had >30% rush cover due to the study design. Nevertheless, we found no evidence that rush cover (which ranged between 30 and 70% in all survey fields barring three control fields with 10–30% rush cover) influenced wader densities. As our study spanned a single breeding season, results are most applicable to the influence of rush management on breeding waders through its short-term impact on vegetation structure (i.e., vegetation height and density). Given that Lapwing favour short grass swards with sparse tussocks comprising rush and grass (Baines, 1988; Milsom et al., 2000), rush management may need to ensure a large proportion of short vegetation is retained throughout the breeding season to generate substantial increases in breeding Lapwing densities, which in the long-term could be achieved by reducing rush cover to lower than 30%. Curlew require a heterogenous sward structure for breeding (Pearce-Higgins and Grant, 2006; Durant et al., 2008), with taller areas to provide chicks with concealment from predators (Valkama et al., 1998), more open areas for foraging (Robson and Allcorn, 2006; Fisher and Walker, 2015), and a range of vegetation heights for nesting (Valkama et al., 1998; Fisher and Walker, 2015; Zielonka et al., 2019). It is plausible that current AES rush management prescriptions are not delivering sufficient within-field heterogeneity in sward structure to provide the complex habitat matrix required by Curlew. Such a situation could arise either because the current prescriptions to cut one-third of the rush within a field on an annual basis are insufficient, or because such prescriptions are too difficult for farmers to follow as they feel that they should cut a larger proportion of the field when they are able to access fields for rush cutting (this is often difficult in winter due to waterlogged conditions). It is also important to note that rush cutting through AES prescriptions has been found to increase the risk of artificial wader nest predation (Kelly et al., 2021) and thus birds may be avoiding nesting in such fields due to perceived, or realised, increases in nest predation risk.
Accounting for variation in field size via inclusion as an offset in the models, our results suggest that rush management through AES prescriptions can increase Snipe breeding densities in some but not all regions, and such benefits could also arise for Lapwing (although low population sizes, especially in the SWP, limited our ability to detect such effects). In contrast, we found evidence that Curlew are, out of our focal species, the least likely to respond to the implementation of AES rush management prescriptions. Whilst ideally surveys would be repeated in subsequent years due to the potential for environmental conditions to vary between years, this was not possible due to logistical constraints. Our study of two distinct upland regions, however, enables testing of rush management across different environmental conditions and population densities of our focal species (see Figure 2). Moreover, our results provide a snapshot of wader densities in fields with and without rush management, with results revealing the potential for rush management to increase densities of Snipe and Lapwing in the short-term. Our study thus advocates further research exploring both the short- and long-term impacts of AES rush management prescriptions on upland breeding waders. We also found evidence that more alkaline soils were associated with higher Curlew and Snipe breeding densities, which for Curlew is consistent with previous research showing lower densities where soil organic carbon (assumed to be more acidic, peaty soils) is higher (Franks et al., 2017). These patterns are presumably due to higher pH increasing abundance of soil invertebrates such as earthworms (McCallum et al., 2016), and wetter soils also increased Snipe densities. Combining rush management with additional interventions to improve habitat quality may thus be beneficial, such as installation of wetter depressions or flushes and blocking of drainage ditches (Smart et al., 2006; Douglas and Pearce-Higgins, 2014; Douglas et al., 2021), or liming of more acidic soils (but with targeted use; McCallum et al., 2016). Rush management prescriptions may, however, benefit from potential revision to increase their efficacy. Potentially beneficial changes that merit further investigation include researching the optimal total area and spatial configuration of cut and uncut rush within fields, thus ensuring heterogeneity in sward structure, perhaps particularly for Curlew (as shown by beneficial mosaic grassland management for Black-tailed Godwits Limosa limosa in The Netherlands; Schekkerman et al., 2008), and in the case of Lapwing contrastingly ensuring rush cover is below 30% (yet retaining some taller vegetation patches; Laidlaw et al., 2017) which will limit heterogeneity in the sward. In addition, where additional drivers to habitat degradation, such as increased nest and chick predation risk, are suppressing wader populations, interventions that focus solely on habitat improvements are unlikely to fully meet their potential to reverse population declines (Smart et al., 2013).
Data are archived in the Dryad Digital Repository (https://doi.org/10.5061/dryad.5dv41ns74).
LK and KE conceived and designed the study with support from other authors and led the writing. LK collected the data and analysed and interpreted the data with advice from KE and DD. All authors contributed critically to the manuscript revisions and gave final approval for the submitted version.
The National Lottery Heritage Fund provided funding for this research.
The authors declare that the research was conducted in the absence of any commercial or financial relationships that could be construed as a potential conflict of interest.
All claims expressed in this article are solely those of the authors and do not necessarily represent those of their affiliated organizations, or those of the publisher, the editors and the reviewers. Any product that may be evaluated in this article, or claim that may be made by its manufacturer, is not guaranteed or endorsed by the publisher.
We thank all farmers and landowners for site access, and Andrew Farmer, David Cooper, and Geltsdale reserve staff for advice and support. We also thank four referees for helpful comments on the manuscript. This study was undertaken within the South West Peak Landscape Partnership’s Working for Waders project, with the Peak District National Park Authority as lead partner.
The Supplementary Material for this article can be found online at: https://www.frontiersin.org/articles/10.3389/fevo.2021.660513/full#supplementary-material
Amar, A., Grant, M., Buchanan, G., Sim, I., Wilson, J., Pearce-Higgins, J. W., et al. (2011). Exploring the relationships between wader declines and current land-use in the British uplands. Bird Study 58, 13–26. doi: 10.1080/00063657.2010.513412
Ashby, M. A., Duncan Whyatt, J., Rogers, K., Marrs, R. H., and Stevens, C. J. (2020). Quantifying the recent expansion of native invasive rush species in a UK upland environment. Ann. Appl. Biol. 177, 1–13. doi: 10.1111/aab.12602
Baines, D. (1988). The effects of improvement of upland, marginal grasslands on the distribution and density of breeding wading birds (Charadriiformes) in northern England. Biol. Conserv. 45, 221–236. doi: 10.1016/0006-3207(88)90141-3
Balmer, D. E., Gillings, S., Caffrey, B. J., Swann, R. L., Downie, I. S., and Fuller, R. J. (2013). Bird Atlas 2007-2011: the breeding and wintering birds of Britain and Ireland. Thetford: BTO Books.
Bolton, M., Bamford, R., Blackburn, C., Cromarty, J., Eglington, S., Ratcliffe, N., et al. (2011). Assessment of simple survey methods to determine breeding population size and productivity of a plover, the Northern Lapwing Vanellus vanellus. Wader Study Gr. Bull. 118, 141–152.
Buchanan, G. M., Pearce-Higgins, J. W., Douglas, D. J. T., and Grant, M. C. (2017). Quantifying the importance of multi-scale management and environmental variables on moorland bird abundance. Ibis 159, 744–756. doi: 10.1111/ibi.12488
Devereux, C. L., Mckeever, C. U., Benton, T. G., and Whittingham, M. J. (2004). The effect of sward height and drainage on Common Starlings Sturnus vulgaris and Northern Lapwings Vanellus vanellus foraging in grassland habitats. Ibis 146, 115–122. doi: 10.1111/j.1474-919X.2004.00355.x
Douglas, D. J. T., Bellamy, P. E., Stephen, L. S., Pearce-Higgins, J. W., Wilson, J. D., and Grant, M. C. (2014). Upland land use predicts population decline in a globally near-threatened wader. J. Appl. Ecol. 51, 194–203. doi: 10.1111/1365-2664.12167
Douglas, D. J. T., Beresford, A., Selvidge, J., Garnett, S., Buchanan, G. M., Gullett, P., et al. (2017). Changes in upland bird abundances show associations with moorland management. Bird Study 64, 242–254. doi: 10.1080/00063657.2017.1317326
Douglas, D. J. T., Lewis, M., Thatey, Z., and Teuten, E. (2021). Wetlands support higher breeding wader densities than farmed habitats within a nature-rich farming system. Bird Study 68, 100–111. doi: 10.1080/00063657.2021.1970104
Douglas, D. J. T., and Pearce-Higgins, J. W. (2014). Relative importance of prey abundance and habitat structure as drivers of shorebird breeding success and abundance. Anim. Conserv. 17, 535–543. doi: 10.1111/acv.12119
Durant, D., Tichit, M., Kernéïs, E., and Fritz, H. (2008). Management of agricultural wet grasslands for breeding waders: integrating ecological and livestock system perspectives - a review. Biodivers. Conserv. 17, 2275–2295. doi: 10.1007/s10531-007-9310-3
Eaton, M., Aebischer, N., Brown, A., Hearn, R., Lock, L., Musgrove, A., et al. (2015). Birds of Conservation Concern 4: the population status of birds in the UK, Channel Islands and Isle of Man. Br. Birds 108, 708–746.
Fisher, G., and Walker, M. (2015). Habitat restoration for curlew Numenius arquata at the Lake Vyrnwy reserve, Wales. Conserv. Evid. 12, 48–52.
Franks, S. E., Douglas, D. J. T., Gillings, S., and Pearce-Higgins, J. W. (2017). Environmental correlates of breeding abundance and population change of Eurasian Curlew Numenius arquata in Britain. Bird Study 64, 393–409. doi: 10.1080/00063657.2017.1359233
Franks, S. E., Roodbergen, M., Teunissen, W., Carrington Cotton, A., and Pearce-Higgins, J. W. (2018). Evaluating the effectiveness of conservation measures for European grassland-breeding waders. Ecol. Evol. 8, 10555–10568. doi: 10.1002/ece3.4532
Fuller, R. J., and Gough, S. J. (1999). Changes in sheep numbers in Britain: implications for bird populations. Biol. Conserv. 91, 73–89. doi: 10.1016/s0006-3207(99)00039-7
Green, R. E. (1985). Estimating the abundance of breeding snipe. Bird Study 32, 141–149. doi: 10.1080/00063658509476869
Green, R. E. (1988). Effects of environmental factors on the timing and success of breeding of common snipe Gallinago gallinago. J. Appl. Ecol. 25, 79–93. doi: 10.2307/2403611
Gunnarsson, T. G., Gill, J. A., Petersen, A., Appleton, G. F., and Sutherland, W. J. (2005). A double buffer effect in a migratory shorebird population. J. Anim. Ecol. 74, 965–971. doi: 10.1111/j.1365-2656.2005.00994.x
Henderson, I. G., Wilson, A. M., Steele, D., and Vickery, J. A. (2002). Population estimates, trends and habitat associations of breeding lapwing Vanellus vanellus, curlew Numenius arquata and snipe Gallinago gallinago in Northern Ireland in 1999. Bird Study 49, 17–25. doi: 10.1080/00063650209461240
Holton, N., and Allcorn, R. I. (2006). The effectiveness of opening up rush patches on encouraging breeding common snipe Gallinago gallinago at Rogersceugh Farm, Campfield Marsh RSPB reserve, Cumbria, England. Conserv. Evid. 3, 79–80.
Hoodless, A. N., Ewald, J. A., and Baines, D. (2007). Habitat use and diet of Common Snipe Gallinago gallinago breeding on moorland in northern England. Bird Study 54, 182–191. doi: 10.1080/00063650709461474
Hoodless, A. N., Inglis, J. G., and Baines, D. (2006). Effects of weather and timing on counts of breeding Snipe Gallinago gallinago. Bird Study 53, 205–212. doi: 10.1080/00063650609461435
IUCN (2020). The IUCN Red List of Threatened Species. Version 2020-2. Available online at: https://www.iucnredlist.org (accessed December 4, 2020).
Johnstone, I., Elliot, D., Mellenchip, C., and Peach, W. J. (2017). Correlates of distribution and nesting success in a Welsh upland Eurasian Curlew Numenius arquata population. Bird Study 64, 535–544. doi: 10.1080/00063657.2017.1411466
Kelly, L. A., Douglas, D. J. T., Shurmer, M. P., and Evans, K. L. (2021). Upland rush management advocated by agri-environment schemes increases predation of artificial wader nests. Anim. Conserv. 24, 646–658. doi: 10.1111/acv.12672
Kleijn, D., and Sutherland, W. J. (2003). How effective are European agri-environment schemes in conserving and promoting biodiversity? J. Appl. Ecol. 40, 947–969. doi: 10.1111/j.1365-2664.2003.00868.x
Kluyver, H. N., and Tinbergen, L. (1954). Territory and the regulation of density in titmice. Arch. Neerl. Zool. 10, 265–289.
Laidlaw, R. A., Smart, J., Smart, M. A., and Gill, J. A. (2017). Scenarios of habitat management options to reduce predator impacts on nesting waders. J. Appl. Ecol. 54, 1219–1229. doi: 10.1111/1365-2664.12838
McCallum, H. M., Wilson, J. D., Beaumont, D., Sheldon, R., O’Brien, M. G., and Park, K. J. (2016). A role for liming as a conservation intervention? Earthworm abundance is associated with higher soil pH and foraging activity of a threatened shorebird in upland grasslands. Agric. Ecosyst. Environ. 223, 182–189. doi: 10.1016/j.agee.2016.03.005
Milsom, T. P., Langton, S. D., Parkin, W. K., Peel, S., Bishop, J. D., Hart, J. D., et al. (2000). Habitat models of bird species’ distribution: an aid to the management of coastal grazing marshes. J. Appl. Ecol. 37, 706–727. doi: 10.1046/j.1365-2664.2000.00529.x
Natural England (2012). Entry Level Stewardship: Environmental Stewardship Handbook. Available online at: http://publications.naturalengland.org.uk/publication/2798159 (accessed May 27, 2020).
Natural England (2018). Countryside Stewardship: Mid Tier Options, Supplements and Capital Items. Available online at: www.gov.uk/natural-england (accessed May 27, 2020).
O’Brien, M., and Smith, K. W. (1992). Changes in the status of waders breeding on wet lowland grasslands in England and Wales between 1982 and 1989. Bird Study 39, 165–176. doi: 10.1080/00063659209477115
O’Brien, M., and Wilson, J. D. (2011). Population changes of breeding waders on farmland in relation to agri-environment management. Bird Study 58, 399–408. doi: 10.1080/00063657.2011.608117
Ordnance Survey (2019). 1:25 000 Scale Colour Raster [TIFF Geospatial Data]. Southampton: Ordnance Survey.
Pearce-Higgins, J. W., and Grant, M. C. (2006). Relationships between bird abundance and the composition and structure of moorland vegetation. Bird Study 53, 112–125. doi: 10.1080/00063650609461424
PECBMS (2020). Trends of Common Birds in Europe, 2019 Update. Available online at: https://pecbms.info/trends-of-common-birds-in-europe-2019-update/ (accessed January 22, 2021).
R Core Team (2020). R: A Language and Environment for Statistical Computing. Available online at: https://www.r-project.org/ (accessed February 18, 2020).
Robson, B., and Allcorn, R. I. (2006). Rush cutting to create nesting patches for lapwings Vanellus vanellus and other waders, Lower Lough Erne RSPB reserve, County Fermanagh, Northern Ireland. Conserv. Evid. 3, 81–83.
Roodbergen, M., van der Werf, B., and Hötker, H. (2012). Revealing the contributions of reproduction and survival to the Europe-wide decline in meadow birds: review and meta-analysis. J. Ornithol. 153, 53–74. doi: 10.1007/s10336-011-0733-y
Roos, S., Smart, J., Gibbons, D. W., and Wilson, J. D. (2018). A review of predation as a limiting factor for bird populations in mesopredator-rich landscapes: a case study of the UK. Biol. Rev. 93, 1915–1937. doi: 10.1111/brv.12426
Rowland, C. S., Morton, R. D., Carrasco, L., McShane, G., O’Neil, A. W., and Wood, C. M. (2017). Land Cover Map 2015 (vector, GB). NERC Environmental Information Data Centre. Available online at: https://catalogue.ceh.ac.uk/documents/6c6c9203-7333-4d96-88ab-78925e7a4e73 (accessed September 10, 2019).
Schekkerman, H., Teunissen, W., and Oosterveld, E. (2008). The effect of ‘mosaic management’ on the demography of black-tailed godwit Limosa limosa on farmland. J. Appl. Ecol. 45, 1067–1075. doi: 10.1111/j.1365-2664.2008.01506.x
Shellswell, C. H., and Humpidge, R. (2018). Use of grazing and mowing to reduce the dominance of soft rush Juncus effusus in fen meadows in Scotland. Conserv. Evid. 15, 54–58.
Silcock, P., Brunyee, J., and Pring, J. (2012). Changing Livestock Numbers in the UK LESS Favoured Areas – An Analysis of Likely Biodiversity Implications. Available online at: http://www.cumulus-consultants.co.uk (accessed May 27, 2020).
Sim, I. M. W., Gregory, R. D., Hancock, M. H., and Brown, A. F. (2005). Recent changes in the abundance of British upland breeding birds. Bird Study 52, 261–275. doi: 10.1080/00063650509461399
Siriwardena, G., Conway, G., Stanbury, A., and Eaton, M. (2017). Breeding Waders of English Upland Farmland (BWEUF): Survey and Data Analysis for Breeding Waders on in-Bye Land. Available online at: http://sciencesearch.defra.gov.uk/Default.aspx?Menu=Menu&Module=More&Location=None&Completed=0&ProjectID=19767 (accessed January 22, 2021).
Smart, J., Amar, A., O’Brien, M., Grice, P., and Smith, K. (2008). Changing land management of lowland wet grasslands of the UK: impacts on snipe abundance and habitat quality. Anim. Conserv. 11, 339–351. doi: 10.1111/j.1469-1795.2008.00189.x
Smart, J., Bolton, M., Hunter, F., Quayle, H., Thomas, G., and Gregory, R. D. (2013). Managing uplands for biodiversity: Do agri-environment schemes deliver benefits for breeding lapwing Vanellus vanellus? J. Appl. Ecol. 50, 794–804. doi: 10.1111/1365-2664.12081
Smart, J., Gill, J. A., Sutherland, W. J., and Watkinson, A. R. (2006). Grassland-breeding waders: identifying key habitat requirements for management. J. Appl. Ecol. 43, 454–463. doi: 10.1111/j.1365-2664.2006.01166.x
Smart, J., Wotton, S. R., Dillon, I. A., Cooke, A. I., Diack, I., Drewitt, A. L., et al. (2014). Synergies between site protection and agri-environment schemes for the conservation of waders on lowland wet grasslands. Ibis 156, 576–590. doi: 10.1111/ibi.12153
Valkama, J., Currie, D., and Robertson, P. (1998). Habitat selection by breeding curlews (Numenius arquata) on farmland: the importance of grassland. Ann. Zool. Fennici 35, 141–148.
Welsh Government (2017). Glastir Advanced 2019 Rules Booklet 2: Whole Farm Code and Management Options. Caernarfon Available online at: https://gov.wales/sites/default/files/publications/2018-01/glastir-advanced-2019-rules-booklet-1.pdf (accessed October 27, 2020).
Whittingham, M. J., and Evans, K. L. (2004). The effects of habitat structure on predation risk of birds in agricultural landscapes. Ibis 146, 210–220. doi: 10.1111/j.1474-919X.2004.00370.x
Whittingham, M. J., Stephens, P. A., Bradbury, R. B., and Freckleton, R. P. (2006). Why do we still use stepwise modelling in ecology and behaviour? J. Anim. Ecol. 75, 1182–1189. doi: 10.1111/j.1365-2656.2006.01141.x
Wilson, A. M., Vickery, J. A., Brown, A., Langston, R. H. W., Smallshire, D., Wotton, S., et al. (2005). Changes in the numbers of breeding waders on lowland wet grasslands in England and Wales between 1982 and 2002. Bird Study 52, 55–69. doi: 10.1080/00063650509461374
Wilson, J. D., Anderson, R., Bailey, S., Chetcuti, J., Cowie, N. R., Hancock, M. H., et al. (2014). Modelling edge effects of mature forest plantations on peatland waders informs landscape-scale conservation. J. Appl. Ecol. 51, 204–213. doi: 10.1111/1365-2664.12173
Wilson, J. D., Whittingham, M. J., and Bradbury, R. B. (2005). The management of crop structure: a general approach to reversing the impacts of agricultural intensification on birds? Ibis 147, 453–463. doi: 10.1111/j.1474-919x.2005.00440.x
Keywords: agricultural change, conservation effectiveness, farmland birds, habitat management, Juncus spp., rush pasture, shorebirds, upland grasslands
Citation: Kelly LA, Douglas DJT, Shurmer MP and Evans KL (2021) Inter-Specific Variation in the Potential for Upland Rush Management Advocated by Agri-Environment Schemes to Increase Breeding Wader Densities. Front. Ecol. Evol. 9:660513. doi: 10.3389/fevo.2021.660513
Received: 29 January 2021; Accepted: 09 November 2021;
Published: 10 December 2021.
Edited by:
Jose Marcelo Domingues Torezan, State University of Londrina, BrazilReviewed by:
Orsolya Kiss, University of Szeged, HungaryCopyright © 2021 Kelly, Douglas, Shurmer and Evans. This is an open-access article distributed under the terms of the Creative Commons Attribution License (CC BY). The use, distribution or reproduction in other forums is permitted, provided the original author(s) and the copyright owner(s) are credited and that the original publication in this journal is cited, in accordance with accepted academic practice. No use, distribution or reproduction is permitted which does not comply with these terms.
*Correspondence: Leah A. Kelly, bGVhaC5rZWxseUByc3BiLm9yZy51aw==
Disclaimer: All claims expressed in this article are solely those of the authors and do not necessarily represent those of their affiliated organizations, or those of the publisher, the editors and the reviewers. Any product that may be evaluated in this article or claim that may be made by its manufacturer is not guaranteed or endorsed by the publisher.
Research integrity at Frontiers
Learn more about the work of our research integrity team to safeguard the quality of each article we publish.