- 1Institute of Omic Sciences and Applied Biotechnology, Pontifical Catholic University of Peru, Lima, Peru
- 2Department of Evolutionary Biology, Faculty of Life Sciences, University of Vienna, Vienna, Austria
- 3Department of Community Ecology, Helmholtz Centre for Environmental Research - UFZ, Halle, Germany
- 4German Centre for Integrative Biodiversity Research (iDiv) Halle-Jena-Leipzig, Leipzig, Germany
- 5Department of Biosciences, Paris-Lodron University of Salzburg, Salzburg, Austria
- 6Centre for Functional Biodiversity, School of Life Sciences, University of KwaZulu-Natal, Pietermaritzburg, South Africa
- 7Institute of Evolutionary Ecology and Conservation Genomics, University of Ulm, Ulm, Germany
- 8Museum of Evolution, Uppsala University, Uppsala, Sweden
- 9Faculty of Science, University of South Bohemia, České Budějovice, Czechia
Despite increased focus on elucidating the various reproductive strategies employed by orchids, we still have only a rather limited understanding of deceptive pollination systems that are not bee- or wasp-mediated. In Europe, the orchid Neotinea ustulata has been known to consist of two phenologically divergent varieties, neither of which provide rewards to its pollinators. However, detailed studies of their reproductive biology have been lacking. Our study aimed to characterize and understand the floral traits (i.e., morphology, color, and scent chemistry) and reproductive biology of N. ustulata. We found that the two varieties differ in all their floral traits; furthermore, while Neotinea ustulata var. ustulata appears to be pollinated by both bees (e.g., Anthophora, Bombus) and flies (e.g., Dilophus, Tachina), var. aestivalis is pollinated almost entirely by flies (i.e., Nowickia, Tachina). Tachinids were also found to be much more effective than bees in removing pollinaria, and we show experimentally that they use the characteristic dark inflorescence top as a cue for approaching inflorescences. Our results thus suggest that while both N. ustulata varieties rely on tachinids for pollination, they differ in their degree of specialization. Further studies are, however, needed to fully understand the reproductive strategy of N. ustulata varieties.
Introduction
The orchid family is one of the largest plant families in the world, accounting for around 28,000 described species (Willis, 2017; Fay, 2018). At least one third of these species are deceptive, not providing their pollinators with a reward (Renner, 2005; but see Shrestha et al., 2020). Deceptive orchids employ various strategies to lure in their pollinators, which range from the advertisement of false nectar or pollen sources to the imitation of brood sites or mating partners (Jersáková et al., 2006; Johnson and Schiestl, 2016). Each type of deception is thought to be associated with a particular combination of floral traits, often influenced by their reliance on particular groups of pollinators (Johnson and Schiestl, 2016; Valenta et al., 2017). Conspicuous visual displays coupled with reduced floral scent emission and the attraction of male and female pollinators are traditionally considered an indication of food-deceptive pollination (Galizia et al., 2004; Jersáková et al., 2012, 2016; Johnson and Schiestl, 2016). Less conspicuous visual displays, the exclusive attraction of either male or female insects and the emission of particular scent bouquets are, in contrast, associated with pollination by sexual deception or brood-site mimicry (Vereecken and Schiestl, 2009; Ayasse et al., 2011; Bohman et al., 2016; Martel et al., 2019; but see Streinzer et al., 2010). For many deceptive orchids the investigation of the floral traits employed can provide an accurate estimate of their pollination strategy; however, some orchid species may have complex strategies that are much more difficult to categorize (e.g., Vogel, 1972; Bino et al., 1982; Vöth, 1989; Valterová et al., 2007; Scopece et al., 2009). This seems also to be the case for the European orchid species, Neotinea ustulata (L.) R. M. Bateman, Pridgeon and M. W. Chase.
Neotinea ustulata, like up to 81% of orchid taxa occurring in Europe, Asia Minor and North Africa (Paulus, 2005; Delforge, 2006), is deceptive, i.e., does not offer any readily identifiable reward to potential floral visitors (Claessens and Kleynen, 2011). While most of these deceptive orchids rely on either bumblebees or solitary bees for reproduction, N. ustulata has been considered to be potentially fly- and beetle-pollinated (Vöth, 1984; van der Cingel, 1995). The evidence for N. ustulata’s pollination system has, however, been mostly anecdotal. The identification of its reproductive strategy has been further complicated by the occurrence of two phenologically distinct varieties, the early flowering (from April to June, or even until July) Neotinea ustulata var. ustulata and the late flowering (from June to August) N. ustulata var. aestivalis (Kümpel) Tali, M. F. Fay and R. M. Bateman. The first recorded pollination event in N. ustulata var. ustulata probably dates back to Godfery (1933), who observed two Tachina (Echinomyia) aff. magnicornis (Zett.) flies, one of them with pollinaria attached to its proboscis, visiting flowers in a population from Eastern France. Further records stem from Vöth (1984), who observed several individuals of T. aff. magnicornis carrying pollinaria in Austria. While tachinid flies seem to be common visitors, other records include bees (Borsos, 1962), bumblebees (Paulus, 2005; Claessens and Kleynen, 2016), and empidid flies (Paulus, 2005; Claessens and Kleynen, 2016). Pollinator records are even more scarce for N. ustulata var. aestivalis, for which there have been only observations of beetles, namely Chrysanthia sp. (Oedemeridae) (Danesch and Danesch, 1962) and Vadonia (Leptura) livida (F.) (Cerambycidae) (Mrkvicka, 1991), carrying pollinaria on various parts of their head. These records are, however, insufficient to determine the reproductive system of N. ustulata as they only account for sporadic and isolated visitation events.
Throughout their range of distribution, the two N. ustulata varieties do not usually occur in sympatry (Tali et al., 2004); var. ustulata being mostly found in dry meadows, whereas var. aestivalis in both dry and wet meadows (e.g., Molinetum vegetation) (Paulus, pers. obs.). Plants of var. aestivalis are also usually taller than those of var. ustulata (Haraštová-Sobotková et al., 2005). Despite these differences, the two varieties are genetically undifferentiated (Tali et al., 2006; but see Haraštová-Sobotková et al., 2005) and have the same chromosome number (Tali, 2004), which indicates no association of autopolyploidy with the phenological differences as reported in others plants (e.g., Simón-Porcar et al., 2017). The mechanisms driving their divergence, thus remains unknown. It is possible that both pollinator- and non-pollinator-mediated selection could have played a role in driving the phenological divergence among populations, leading to the evolution of these two varieties. However, testing the role of pollinator as selection mediators in N. ustulata requires firstly a better assessment of its pollinators and of the traits involved in pollinator attraction. To this date there are only very few assessments of floral traits of N. ustulata varieties (i.e., Haraštová-Sobotková et al., 2005; Schiestl and Cozzolino, 2008; D’auria et al., 2021). Inflorescences of both varieties have a similar color pattern (Paulus, 2005), both having a very dark brown-purple top (hereafter referred as “dark top”). This provides a strong contrast with the remaining part of the inflorescence (Godfery, 1933; Stace, 2010), and may serve as a signal for attracting pollinators; however, this hypothesis so far has not been tested. Perhaps the most unusual trait of N. ustulata is its floral scent. Food-deceptive orchids rarely rely upon flower scent for pollinator attraction, this floral trait is, however, essential in sexually deceptive species (Ayasse et al., 2011; Bohman et al., 2016). Schiestl and Cozzolino (2008) were the first to show that the floral scent of N. ustulata contains alkenes, which, although uncommon in most flowers, are frequently associated with sexually deceptive ones (Ayasse et al., 2011; Bohman et al., 2016); in particular, N. ustulata flowers produce high relative quantities of 11-tricosene. The role of this specific compound in pollinator attraction is so far unknown, but other tricosene isomers are known to act as sex pheromones in the house fly Musca domestica (Carlson et al., 1971; Rogoff et al., 1973) or aggregation pheromones in Drosophila (Hedlund et al., 1996). At the moment, we do not know if alkenes could be found in both varieties or these are restricted to just one of them. The particular combination of visual signals associated with the chemical composition of the flower scent raises the interesting possibility that N. ustulata may rely on intricate signals to attract its pollinators.
In order to understand the reproductive strategy and, eventually, the evolution of N. ustulata, we need to accurately quantify the differences in floral traits between its two varieties as well as identify their reproductive biology. Therefore, our study aims are to compare N. ustulata varieties in terms of (i) morphological, visual and chemical floral traits, (ii) pollination success and patterns of fruit set, and (iii) pollinator spectra. Furthermore, we aimed to (iv) assess efficiency in pollinaria removal among the principal pollinator guilds, and (v) identify the role of the inflorescence dark top in the attraction of predominant pollinators.
Materials and Methods
Study Species
Neotinea ustulata is a perennial tuberous herb, which is typically found on extensively used meadows (Tali et al., 2004). Each plant of this species bears a single inflorescence, which has a burnt appearance when its flowers are in bud; the species is therefore known as the burnt-tip orchid. Neotinea ustulata flowers have three semi-fused sepals, which form a hood; the outer side of the sepals (especially in buds) displays a dark reddish to blackish color (Figure 1). The lip is white with dark red spots and is deeply divided into three parts (Figure 1). The flowers have a nectarless spur, which is blunt. Neotinea ustulata occurs in central and southern Europe with populations reaching Spain and Greece in the south, England and southern Sweden in the north, and as far east as the Caucasus and Ural Mountains (Tali et al., 2004; Delforge, 2006). Despite its broad geographical range, the species is vulnerable to extinction and the number of populations have been greatly reduced during the last century (Neiland, 2001; Tali et al., 2004; Baumann et al., 2005; Jacquemyn et al., 2005).
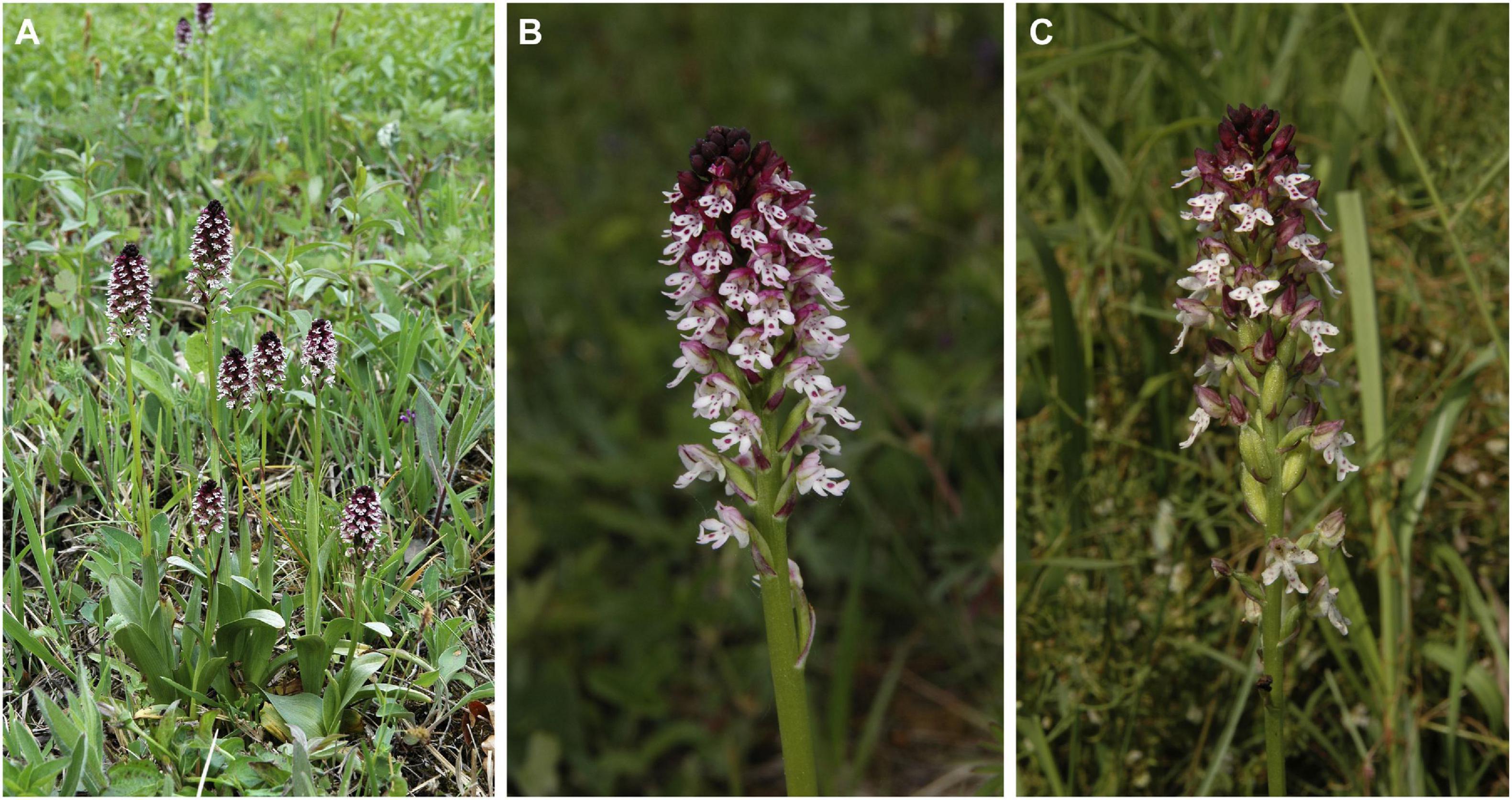
Figure 1. The burnt tip orchid Neotinea ustulata. (A) Habitus of N. ustulata. (B) Inflorescence from a var. ustulata plant. (C) Inflorescence from a var. aestivalis plant. Pictures by J. Jersáková.
The species consists of two varieties, which, while phenologically distinct, do not differ strongly in their vegetative traits (Kümpel and Mrkvicka, 1990; Wucherpfennig, 1992; Haraštová-Sobotková et al., 2005): the early flowering N. ustulata var. ustulata and the late flowering var. aestivalis. Neotinea ustulata var. ustulata is 10–35 cm tall, rarely larger, and its flowering period ranges from April till June in most parts of Europe (Tali et al., 2004; Baumann et al., 2005), but from June to July at high altitudes in the Alps (Paulus, pers. obs.). The dense, narrow, up to 8 cm long inflorescence is composed on an average of 30 flowers (Tali et al., 2004; Baumann et al., 2005; Haraštová-Sobotková et al., 2005; Figure 1). Neotinea ustulata var. aestivalis has in turn a somewhat taller stem, of 33 cm long in average, (rarely up to 80 cm; Haraštová-Sobotková et al., 2005) with longer and narrower leaves, and sharply pointed inflorescences (especially in young stage) (Figure 1). The inflorescences have on average around 40 flowers (Jensen and Pedersen, 1999). Plants of this variety flower from the end of June or July till mid-August (Tali et al., 2004; Baumann et al., 2005; Paulus, pers. obs.). In order to confirm the identity of the two varieties, detailed digital photographs of individuals from our sampling localities were compared against material deposited in diverse herbaria (e.g., CB, BRNM, OHN, PR, PRC, UPS, VN, and W).
Study Sites
Field observations and experiments were carried out in Austria, the Czech Republic, the Slovak Republic and Sweden (Figure 2), during May–June (for details see Supplementary Table 1). In total, thirteen populations of var. ustulata and ten populations of var. aestivalis were sampled to analyze different aspects of their reproductive biology (Figure 2; for population details see Supplementary Table 1).
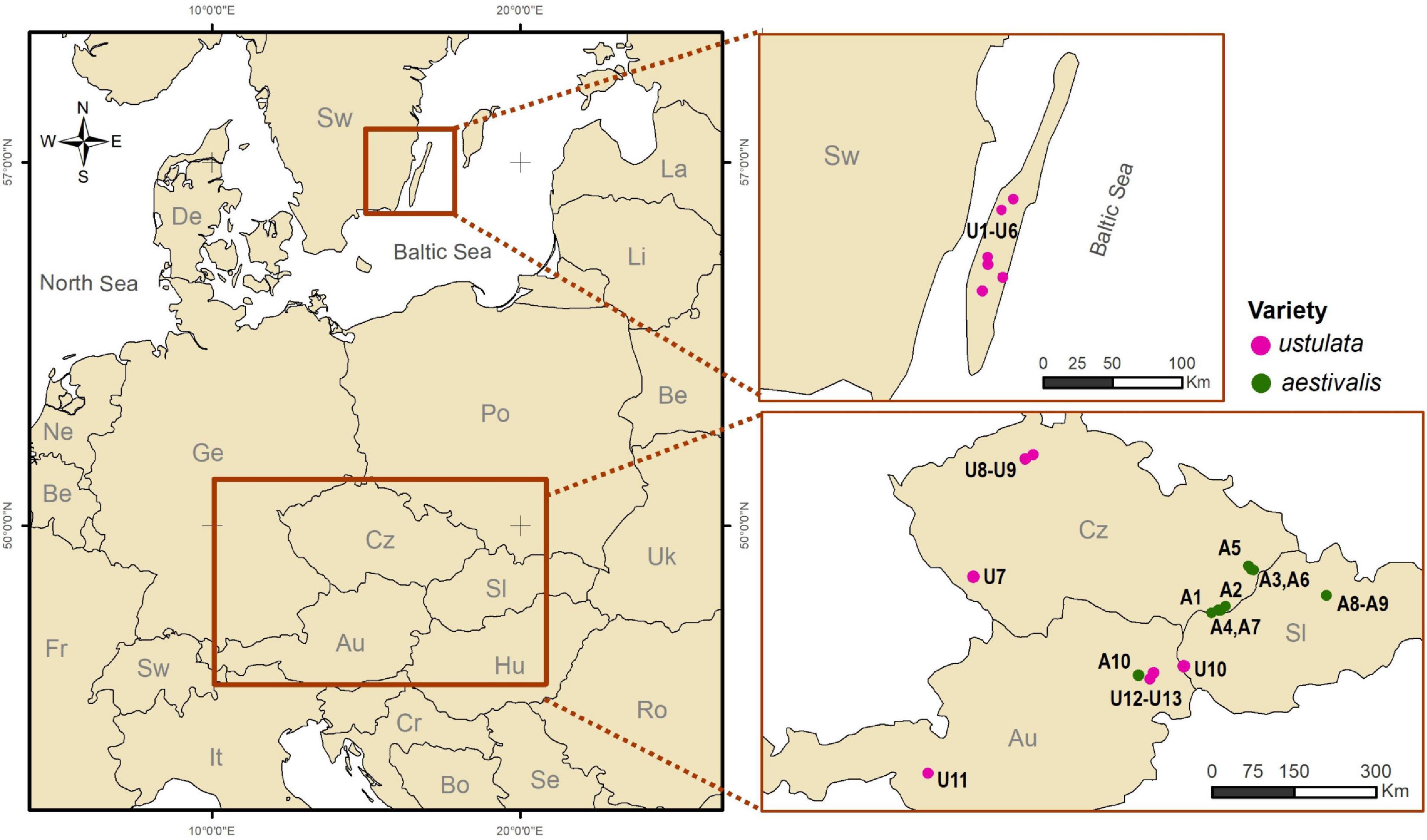
Figure 2. Maps showing the localities where field work was carried out in diverse European countries. The variety identity of Neotinea ustulata populations is indicated by circle colors (violet: var. aestivalis; green: var. ustulata). Populations codes follow Supplementary Table 1.
Morphological, Visual, and Chemical Floral Traits
Morphology
We sampled inflorescences of N. ustulata var. ustulata and var. aestivalis from two populations each (i.e., 30, 30, 23, and 15 inflorescences from U8, U9, A2, and A4, respectively, see Supplementary Table 1 for population codes). From the middle of each inflorescence, we picked a single, fully opened and yet unpollinated flower. Flowers were placed in 70% ethanol (Sigma Aldrich, Munich, Germany) and stored until further usage. Individual flowers were mounted on a styrofoam base and then photographed from three different angles with the aid of a Dino-Lite digital microscope (AnMo Electronics Corporation, Taiwan). Size calibration and distance measurements were performed with the in-house software DinoCapture (AnMo Electronics Corporation, Taiwan). Eight floral traits (i.e., hood opening width, hood opening height, spur opening width, spur width at 3/4 spur length, width at spur bottom, spur length, distance from column to the spur opening, and lip length) were measured. To identify which morphological traits, if any, are responsible for differences among varieties of N. ustulata, we performed a principal component analysis (PCA) using the factoextra package (Kassambara and Mundt, 2020) of the R software (R version 3.6.1; R Core Team., 2017). Morphological data were scaled and normalized prior to conducting the PCA. To explore differences in morphology between the two varieties, we performed a permutational multivariate analysis of variance (PERMANOVA) with the raw morphological data. We constructed a matrix based on Euclidean distances to run the PERMANOVA with 10,000 permutations, considering “variety” as fixed factor and “population” as nested factor in “variety.” PERMANOVA was performed using the vegan package (Oksanen et al., 2019) of R.
Color
The spectral reflectance was measured for 44 flowers (one flower per plant) from two populations per N. ustulata variety (i.e., 14, 10, 10, and 10 flowers from U8, U9, A2, and A4, respectively). We specifically measured the reflectance of the dark top, outer sepals and lips. All floral reflectance measurements were performed using an Ocean optics UV-VIS Spectrometer JAZ and an Ocean Optics DT-mini light source (200–1,100 nm; Dunedin, FL, United States), calibrated with a white reflection standard (WS-1-SL, Ocean Optics). Readings were taken through a fiber-optic reflection probe (UV/VIS 400 μm) held at 45°ca. 5 mm from the surface of the object. The visual similarity between varieties, as perceived by their visitors, was determined by plotting their reflectance spectra as loci in bee- and fly-specific color spaces, assuming adaptation to foliage background (Chittka and Kevan, 2005). Selection of these two insect models was based on our pollinator list (see section “Results”). To model bee color vision, we used the color hexagon (Chittka, 1992), based on the spectral sensitivities of Apis mellifera (L.). To model fly color vision, we used Vorobyev and Osorio, 1998) receptor noise model. This method uses spectral sensitivities and the relative numbers of each fly photoreceptor type to calculate the perceived color contrast between objects. We did not use Troje’s (1993) fly categorical color vision model because it was already shown to be unreliable when contrasted with experimental data (e.g., Jersáková et al., 2012; Renoult et al., 2014; An et al., 2018). Based on color loci, we also run a PERMANOVA, considering “variety” and “inflorescence part” as fixed factors and “population” as a nested factor in variety, to test for differences in spectral reflectance between varieties while considering population origin. The visual modeling and PERMANOVA were performed using the pavo (Maia et al., 2021) and vegan packages of R, respectively.
Floral Scent
Highly volatile compounds of the floral scents of both N. ustulata varieties were collected using dynamic headspace techniques (i.e., 10, 9, and 5 flowers from U8, U9, and A2, respectively), whereas semi- and low-volatile compounds were collected through solvent extracts (i.e., 10, 10, 11, and 10 flowers from U8, U9, A2, and A4, respectively). Samples were analyzed by a gas-chromatograph and gas chromatograph/mass spectrometer using a DB-5 non-polar column (for detailed description of the analytical techniques see Supplementary Methods 1). Chemical identification was carried out by comparing the mass spectra of pure compounds with the ones of commercial libraries (NIST, ADAMS) or of our own libraries, and the retention indexes of detected compounds with those from the literature (e.g., Adams, 2017). Mass spectra were processed using the Automated Mass Spectral Deconvolution and Identification System—AMDIS—software (National Institute of Standards and Technology, Gaithersburg, United States). Differences in floral scent bouquets of both varieties were analyzed by comparing the relative proportions of compounds identified in our two type of samples. We calculated the Bray-Curtis similarity index to determine semi-quantitative differences in odor bouquets. Then, we performed a PERMANOVA with 10,000 permutations to test for differences in scent bouquets between the two varieties while considering population origin. A non-metric multidimensional scaling (NMDS) was performed to graphically display the (dis)similarities in floral scent patterns among samples. PERMANOVA and NMDS were performed using the vegan package and displayed graphically using the ggplot2 package (Wickham et al., 2020) of R.
Reproductive Success Patterns
During 6 years (i.e., 1978, 1981, 1983, 1984, 2000, and 2001), we examined seven populations of N. ustulata var. ustulata and four populations of var. aestivalis for fruit production (see Supplementary Table 1 for population details). We recorded the total number of flowers and position of fruits within inflorescences for each inflorescence sampled. The differences in the number of flowers, the number of fruits produced and the proportion of flowers that set fruits between the N. ustulata varieties were tested by means of generalized linear mixed-models (GLMMs) with Poisson model distribution with log link function for number of flowers and fruits, and binomial model distribution with logit link function for the proportion of flowers that set fruits; “population” was set as a random factor.
In deceptive systems, it is expected to find position effects on fruit production, as first open flowers are more likely to be visited by yet unexperienced insects than late flowers; however, it is unknown if this pattern generalized and also happens in N. ustulata. Therefore, we checked whether the development of fruits is affected by the relative position of the flower in the inflorescence by dividing each inflorescence into three sections (i.e., bottom, middle, and top), calculated mean reproductive success for each section, and then performed a GLMM with a binomial model distribution and log link functions, and conducted Tukey post hoc tests to compare pairs of means; “plant individual” was set as a random factor. GLMMs and post hoc tests were carried out using the lme4 (Bates et al., 2019) and emmeans packages (Lenth et al., 2020), respectively, in R.
Diversity of Pollinator Guilds and Their Efficiency
Flower Visitors, Pollinators, and Their Overall Efficiency
Insect visitors on N. ustulata flowers were recorded by video footages (73 and 37 h of footage on N. ustulata var. ustulata and var. aestivalis, respectively) and complemented by direct observations in our study sites (see Supplementary Table 1 for population details). Floral visitor identity and identification of visitors as pollinators were assessed based on both direct observations and video recordings; whereas frequency of visits and floral visitor behavior on the inflorescence were assessed based on the video footage only. Visitors were identified to the order and family level, and when possible up to genus/species level. To test for differences in the flower visitor composition, a G-test of independence comparing the frequency of species within orders between varieties was performed using the DescTools package (Signorell, 2020) of R. Representatives of each floral visiting species were collected using entomological nets in parallel to the video recordings in search of attached pollinaria and for insect identification. We also checked for presence and placement of pollinaria in insects feeding on rewarding flowers of plants co-occurring with N. ustulata, such as species of Aegopodium L. (Apiaceae), Crataegus L. (Rosaceae), Dorycnium Mill. (Fabaceae) and Euonymus L. (Celastraceae). To corroborate the pollinaria identity, they were compared with pollinaria directly collected from N. ustulata individuals. Insects were considered pollinators based on both the presence of attached pollinaria and evidence of pollinaria removal (assessed from the video footage or direct observations).
Furthermore, we also calculate male (i.e., pollinaria removed/total flowers × 100) and female (i.e., pollinia deposited/total flowers × 100) reproductive success, and pollen transfer efficiency (i.e., ratio of pollinia deposited to pollinaria removed × 100; Nunes et al., 2016), which can be linked to specialization. We performed a GLMM with Poisson model distribution and log link function to estimate the effects of variety on pollen transfer efficiency and with “population” set as a random factor. GLMM was carried out using the lme4 package of R.
Efficiency in Pollinaria Removal Between Pollinator Guilds
We compared the pollinaria removal efficiency between the tachinid fly Tachina fera (L.) and the bee Anthophora plumipes (Pallas), in order to identify whether pollinators of different guilds are equally efficient. Our set up was as follows: one inflorescence of N. ustulata var. ustulata with pollinaria present in all its open flowers was placed in a plastic container (100 × 50 mm i.d., Greiner Bio-One GmbH., Frickenhausen, Germany) in which a single individual of either T. fera (n = 6) or A. plumipes (n = 12) was introduced. We recorded the removal of pollinaria in up to three attempts (i.e., insertion of the proboscis in the flower) of each T. fera and A. plumipes. We performed a GLMM with binomial model distribution and logit link function to test for removal efficiency between pollinators. We also conducted Tukey post hoc tests to compare pairs of means. Our GLMM for repeated measures, compound symmetry was used as covariance pattern model, included “pollinator guild” and “attempt number” as fixed factors and “insect individual” as a random factor. GLMM and post hoc tests were performed using the lme4 and emmeans packages in R.
Role of Visual Traits in Pollination Success
In 1999 we sampled inflorescences from eight populations from Sweden and Czech Republic belonging to both varieties (see Supplementary Table 1 for population details). They were examined for inflorescence display and dark top display. Neotinea ustulata inflorescences have a cylindrical arrangement and, therefore, the inflorescence display was calculated as its two-dimensional projection (i.e., the area of a rectangle: length × width of inflorescence); while the dark top has a circular shape and, therefore, dark top display was approximated as the area of a circle (i.e., π × {width of dark top/2}2). We also assessed the number of flowers with removed pollinaria, deposited pollinia and flowers where pollination events occur; they were then transformed to proportions by dividing them by the number of open flowers. We performed GLMMs with binomial model distribution and logit link function to estimate the effects of visual display on the probability of a flower to be pollinated. Our models included “inflorescence display,” “dark top display” and “variety” as fixed effects, and “population” as random factor. GLMMs were performed using the lme4 package in R.
Role of Dark Top in Fly Pollinator Attraction
We tested the importance of the dark top in the attraction of tachinids since it might be an attractant to flies (Godfery, 1933; van der Cingel, 1995). Tachina flies have been previously considered the main pollinators of N. ustulata (Vöth, 1984; Trunschke et al., 2017), and their importance as pollinators is supported by our own observations (see section “Results”). Experiments were carried out with both varieties. For var. ustulata, four inflorescence pairs were selected for experiments. For each trial, two inflorescences of equal height and similar phenological state were placed less than 10 cm apart. Each inflorescence pair was first observed for about 30 min, during which the number of tachinids attracted by each inflorescence was recorded. After the initial observations the dark top of one of the two inflorescences was arbitrarily cut off. The inflorescence pairs (i.e., one intact, one without the dark top) were then observed again for the same amount of time and the number of tachinids which landed and/or probed the flowers was recorded. In case of var. aestivalis, only two inflorescence pairs were selected, but the experimental setup was similar to that described for var. ustulata, with two exceptions: (i) For the second trial, the cut off dark top of var. aestivalis was placed below the selected plant, in such a way that it was hidden from the view to approaching flies. This setup ensured that olfactory cues released by the dark top were still present when the dark top was cut off. (ii) An additional trial was carried out, in which the dark top was reattached to the plant. Then, the inflorescence pairs were offered again to tachinids. This trial controlled for any effect of the damage (e.g., green leaf volatiles after plant tissue damage) to the inflorescence on their attractiveness to Tachina flies. We performed GLMMs with negative binomial model distribution and log link function to test for differences in the attractiveness of manipulated and non-manipulated inflorescences within each variety. Our GLMMs for repeated measures incorporated a compound symmetry covariance matrix included “treatment stage” and “manipulation condition” as fixed factors, “plant individual” as the subject for repeated measures before and after the manipulation, and “number of visits” as the response variable. GLMMs were performed using the MASS package (Ripley et al., 2020) in R.
Results
Comparison of Morphological, Visual, and Chemical Floral Traits
Morphology
The flowers of N. ustulata var. ustulata are larger in all measured traits in comparison to var. aestivalis (Table 1). In our PCA, the first two PCs accounted for 62.1% of the total variance (PC1: 50.5%, PC2: 11.6%). All the morphological floral traits loaded negatively on the PC1 (Supplementary Table 2), which separates var. ustulata (negative scores) from var. aestivalis (positive scores) (Figure 3). Indeed, the PERMANOVA analysis revealed that the floral morphology differed significantly between var. ustulata and var. aestivalis [Pseudo-F(1, 97) = 81.12, p < 0.0001], and between populations [Pseudo-F(2, 97) = 5.32, p < 0.001].
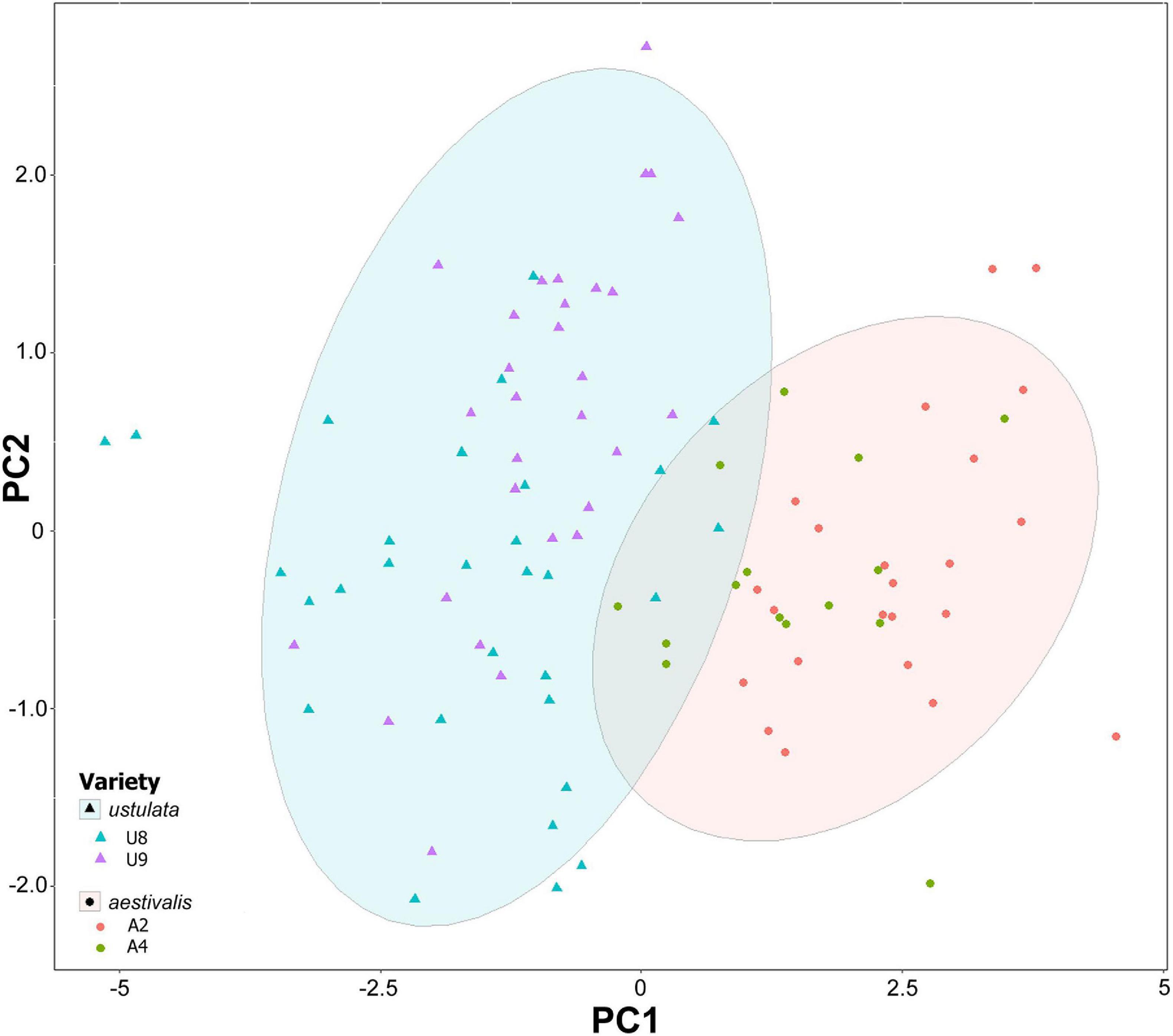
Figure 3. Plotting of Neotinea ustulata samples based on floral morphology traits in PC1 and PC2. Different symbols represent different varieties and ellipses display 95% confidence area of each variety. Different symbol colors represent different populations. Population codes as in Supplementary Table 1.
Color
The dark top and the lightly colored lip plotted apart in both the fly and bee color models, indicating that these two parts of the flower appear highly contrasting, and are therefore easily discriminated by bees as well as flies. Loci of the individual color measurements of the dark top (n = 44) plotted, except by one case, in the UV-blue color category within the bee hexagon and also grouped together within the fly tetrahedron (Figure 4). Similarly, color loci of the lip (n = 44) clustered together within both the bee hexagon and the fly tetragon (Figure 4). The color loci of the hood (n = 44) were more broadly distributed, spanning the space from the dark top loci to the lip loci (Figure 4). In terms of color information, the dark top and hood may often be perceived as uncolored against the leaf background by bees based on color hexagon distances. The color loci of different inflorescence parts were different, independently of variety, as our PERMANOVA analysis confirmed [Pseudo-F(7, 131) = 52.64, p < 0.0001]. Furthermore, PERMANOVA analyses also indicated that there are statistical differences in the spectral reflectance between N. ustulata varieties [Pseudo-F(1, 131) = 9.28, p < 0.01] and within varieties among populations [Pseudo-F(3, 131) = 2.81, p < 0.05].
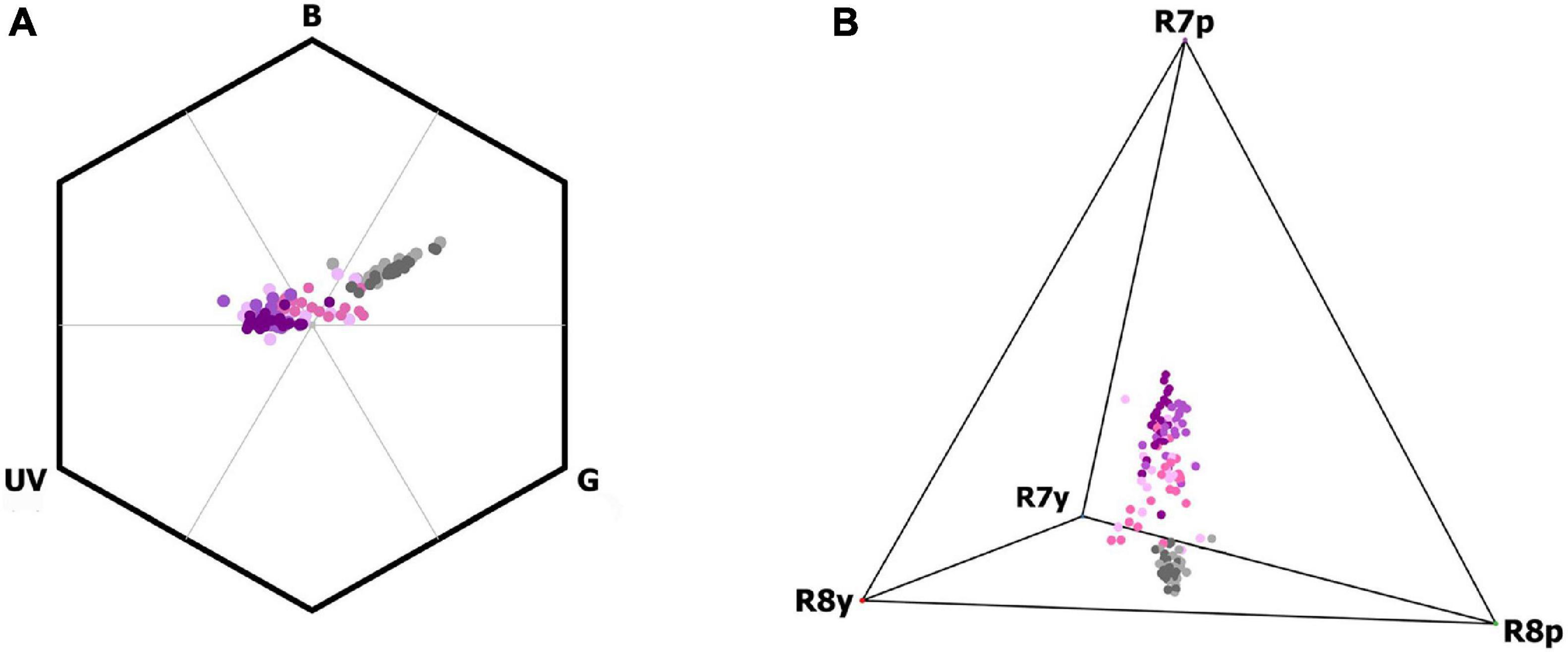
Figure 4. Color loci (circles) of different inflorescence parts of Neotinea ustulata varieties (purple: dark top; pink: hood; gray: lip) plotted within the vision color models of (A) bees (bee-hexagon) and (B) flies (fly-tetrahedron). Var. ustulata: dark-colored tones (i.e., light purple, light pink, light gray); var. aestivalis: light-colored tones (i.e., dark purple, dark pink, dark gray). Bee (UV, B, G) and fly (R7p, R7y, R8p, R8y) receptors are shown.
Floral Scent
We detected 44 floral volatiles in the headspace samples and 54 chemical compounds in the floral extracts of N. ustulata with the majority of the compounds present in both varieties (see Supplementary Tables 3, 4). Headspace samples were dominated by terpenes (e.g., eucalyptol, limonene, linalool, β-myrcene, (E)-β-ocimene), although benzenoids (e.g., 2-phenylethyl alcohol) were also identified (see Supplementary Table 3); whereas extracts were dominated by alkanes (e.g., pentacosane, heptacosane) and alkenes (e.g., tricosene and heptacosene isomers), but also aldehydes (see Supplementary Table 4). The (dis)similarity of floral scent composition between N. ustulata var. ustulata and var. aestivalis is shown in Figure 5. Although there were differences in floral scent patterns among populations of the same varieties [PERMANOVA headspace samples: Pseudo-F(1, 23) = 4.72, p < 0.01; PERMANOVA extract samples: Pseudo-F(2, 40) = 12.65, p < 0.0001], we did not find any significant differences between the two varieties when comparing headspace samples [Pseudo-F(1, 23) = 1.48, p = 0.171]. Significant differences were only found when comparing the composition of the floral extracts of the two varieties [Pseudo-F(1, 40) = 37.33, p < 0.0001]. Neotinea ustulata var. ustulata could thus be distinguished from var. aestivalis through the low- or semi-volatile compounds emitted, but not by the more volatile fraction of the flower scent.
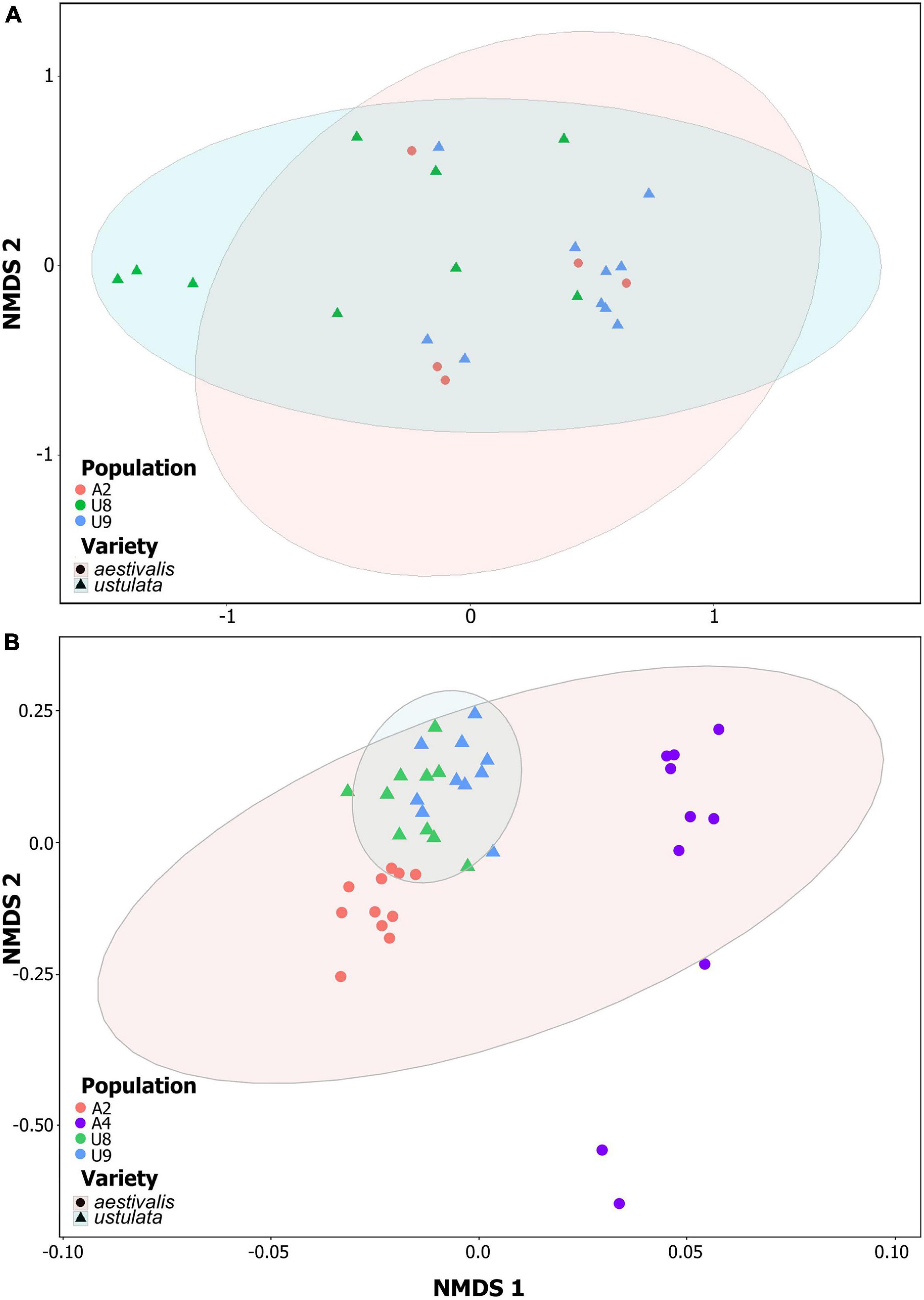
Figure 5. Comparison of floral scent composition between Neotinea ustulata varieties in a non-metric multidimensional scaling (NMDS) based on semi-quantitative Bray-Curtis similarities: (A) headspace (stress = 0.15), and (B) solvent extracts (stress = 0.09). Different symbols represent different varieties and different symbol colors represent different populations (see Supplementary Table 1 for population codes). Ellipses display 95% confidence area of each variety.
Pollination Success and Patterns of Fruit Set
Differences in the number of flowers between varieties were significant (var. aestivalis: mean ± SD: 40.89 ± 13.57; var. ustulata: mean ± SD: 33.34 ± 11.37; χ2 = 4.83, df = 1, p < 0.05; Figure 6), while differences in the number of fruits approached statistical significance (var. aestivalis: mean ± SD: 9.82 ± 9.32; var. ustulata: mean ± SD: 3.93 ± 5.01; χ2 = 3.59, df = 1, p = 0.058; Figure 6); however, no differences were found comparing the proportion of flowers that set fruits (var. aestivalis: mean ± SD: 0.22 ± 0.19; var. ustulata: mean ± SD: 0.13 ± 0.17; χ2 = 1.41, df = 1, p = 0.24; Figure 6). The number of flowers per inflorescence had a positive impact on the probability to set at least one fruit (χ2 = 6.23, df = 2, p < 0.05), and the estimated probability to set a fruit increased 4% for each 10 flowers.
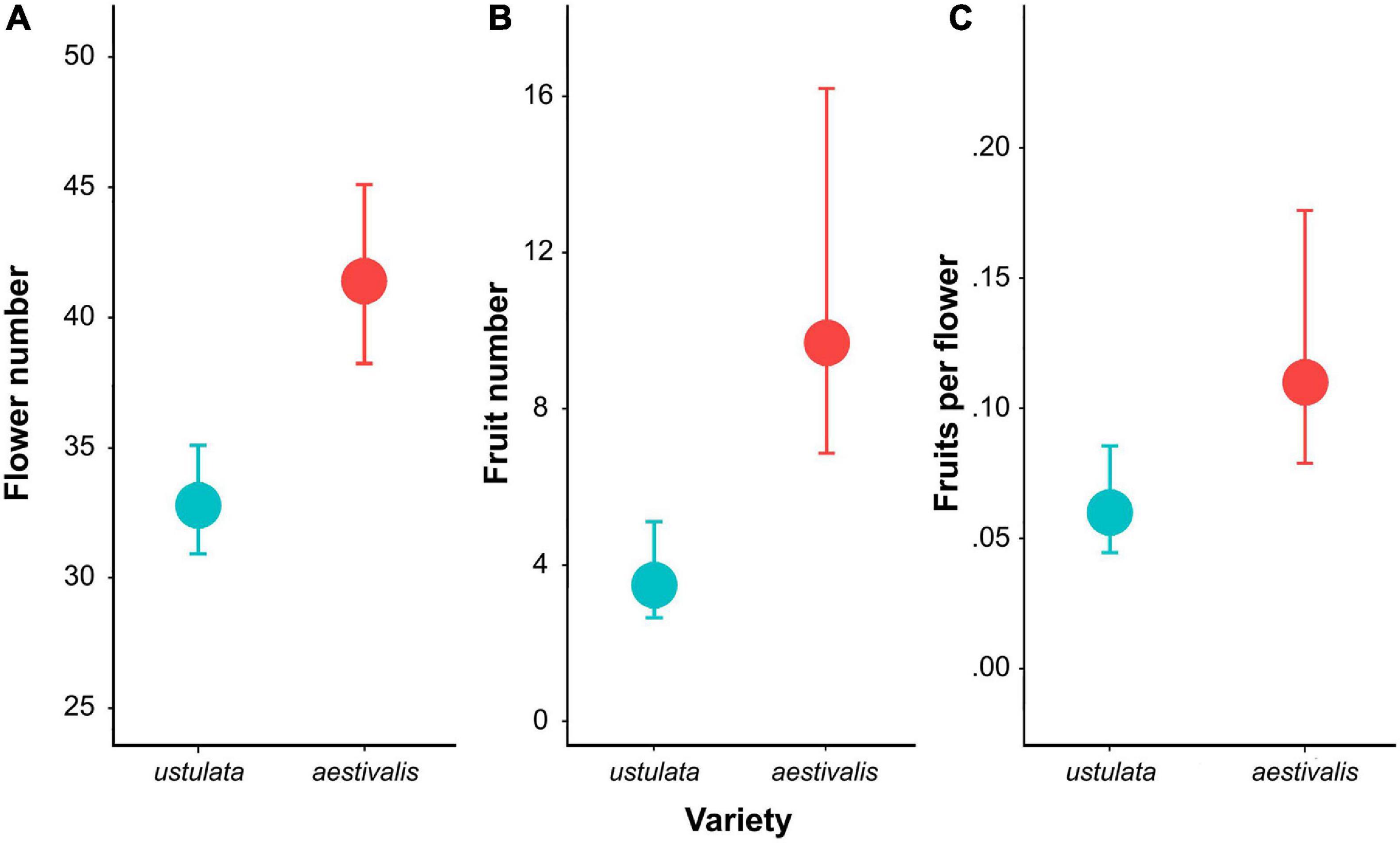
Figure 6. Floral traits related to reproductive success: (A) the number of flowers, (B) the number of fruits, and (C) proportion of flowers that set fruit between Neotinea ustulata var. ustulata and var. aestivalis. Back-transformed means and standard errors are shown.
The probability to set a fruit varied significantly with the position of a flower within the inflorescence (χ2 = 30.88, df = 2, p < 0.0001; Supplementary Figure 1), but not the variety (χ2 = 1.54, df = 1, p = 0.21) or the interaction of variety and position (χ2 = 1.71, df = 2, p = 0.42; Supplementary Figure 1). Post hoc tests comparing the fruit set among the sections within each variety showed that compared to the top section, probability to set fruit was higher in the bottom (aestivalis: z = 4.040, p < 0.001; ustulata: z = 3.995, p < 0.001) and middle sections (aestivalis: z = 2.882, p < 0.05; ustulata: z = 2.915, p < 0.05); while no differences were found when comparing bottom and middle sections (aestivalis: z = 1.556, p = 0.63; ustulata: z = 1.210, p = 0.83; Supplementary Figure 1).
Pollinator Guilds and Their Efficiency in Pollinaria Removal
Flower Visitors, Pollinators, and Their Overall Efficiency
Inflorescences of both varieties were visited by a broad range of insect taxa within the Coleoptera, Diptera, Hymenoptera and Lepidoptera. Diptera and Hymenoptera were more frequently observed in our video recordings and together accounted for around 90% of the total insect visits (i.e., 415 insect visitors) to both N. ustulata varieties (Figure 7 and see Supplementary Table 5 for details of insect identity and frequency). Based on the same video recordings, the frequencies of the attracted insect orders significantly differed between var. aestivalis and var. ustulata (G = 71.68, df = 3, p < 0.0001; Figure 7). Anthophora plumipes and Lasioglossum sp. bees were particularly frequent on N. ustulata var. ustulata, and T. aff. magnicornis flies were most abundant on var. aestivalis (Supplementary Table 5). Despite the high number of insect visits, only three Anthophora bees carrying pollinaria of N. ustulata var. ustulata and one T. aff. magnicornis removing pollinaria of var. aestivalis were observed in our videos. By direct observations, we recorded some Tachina individuals visiting the flowers and carrying pollinaria (Supplementary Table 6), thereby acting as pollinators of N. ustulata var. ustulata and var. aestivalis. While bees almost exclusively landed on the white part of the inflorescence moving upwards in their search for a reward, Tachina flies always settled briefly (for 1–4 s.) on the dark top of the inflorescence; then they searched for potential nectar in the uppermost freshly open flowers and introduced their head to reach the base of the flower spur with their body in downright position (Figure 8). The pollinaria then became attached on the ventral side of the lower part of the proboscis (Figure 8). Identification of tachinid specimens revealed that they belong to three morphologically similar species, Tachina fera, T. magnicornis and Nowickia ferox. Both taxa pollinate flowers of N. ustulata var. ustulata, while var. aestivalis was only observed to be pollinated by T. magnicornis and N. ferox (Supplementary Table 6). Beside tachinids, solitary bees and bumblebees acted as regular pollinators, whereas beetles and other flies were highly occasional (see Supplementary Table 6 and Supplementary Figure 2).
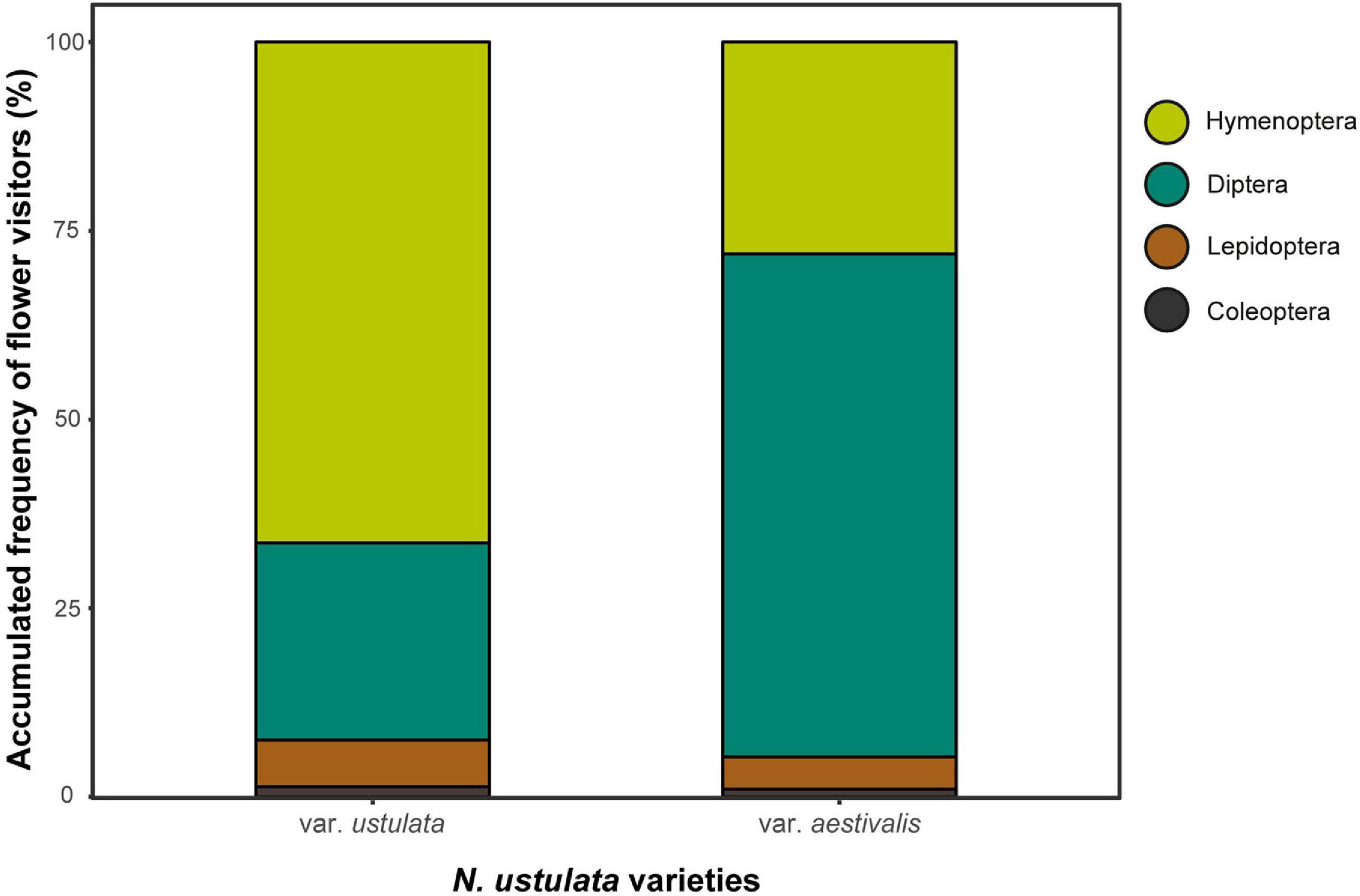
Figure 7. Percentage of floral visits by insect groups to Neotinea ustulata var. ustulata and var. aestivalis. Insects were classified at the taxonomic order rank. Note that the graphs are based solely on video recordings. For records of insect diversity and insect responses see Supplementary Table 5.
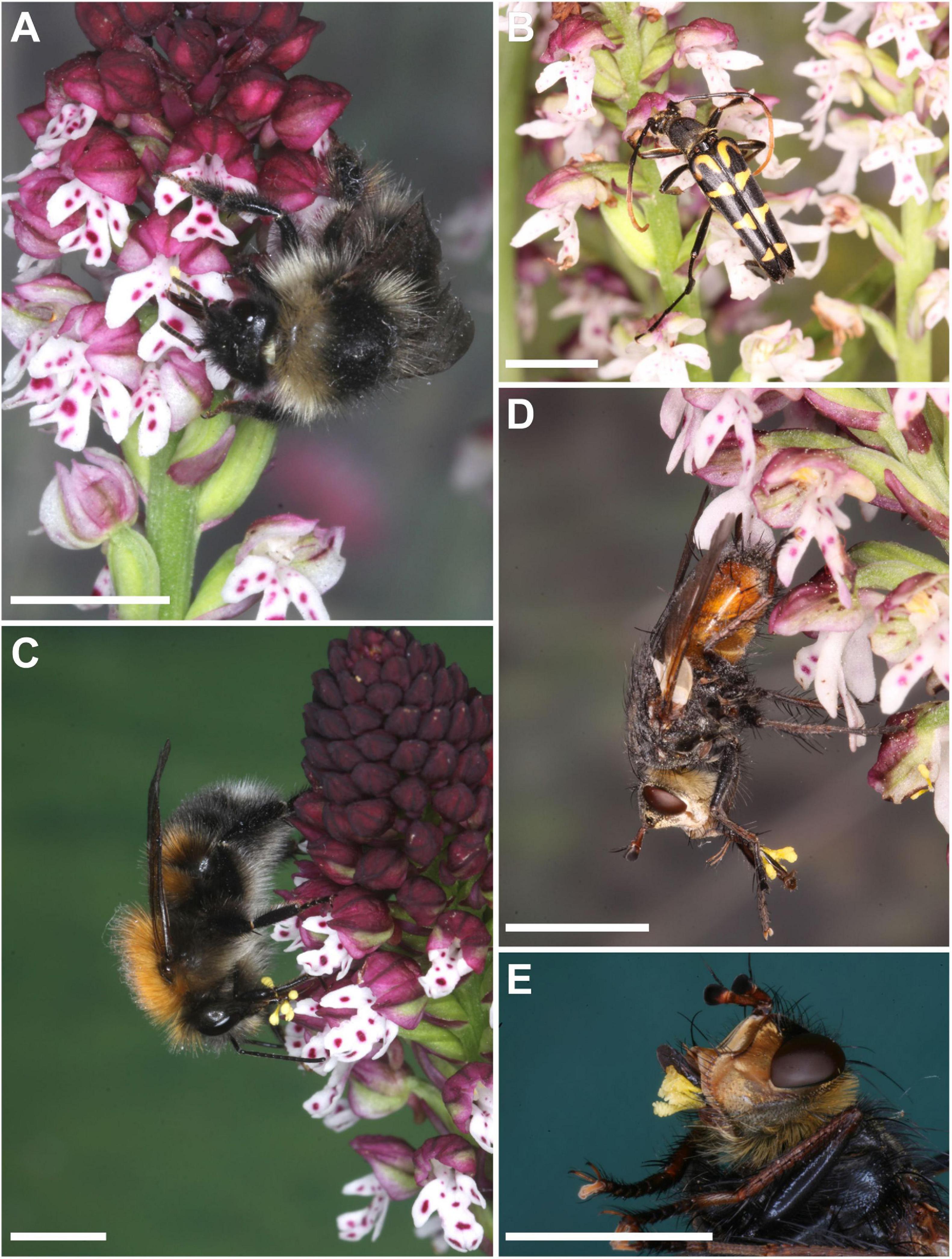
Figure 8. Pollinators of Neotinea ustulata: (A) Bombus mucidus, (B) Leptura annularis, (C) Bombus hypnorum, (D) Tachina fera, (E) Tachina fera close-up. They were recorded visiting flowers of N. ustulata var. ustulata (A,B,D,E) and var. aestivalis (C). White bars represent scale of 5 mm. Photographs by H. Paulus.
Differences in female success between both varieties (var. aestivalis: mean ± SD: 6.73 ± 15.57, n = 86; var. ustulata: 20.80 ± 22.74, n = 172) were marginally significant (χ2 = 3.787, df = 1, p = 0.052). However, we did not find differences in male success (χ2 = 0.444, df = 1, p = 0.51) and pollen transfer efficiency (χ2 = 0.477, df = 1, p = 0.49) between var. aestivalis (male success: mean ± SD: 23.03 ± 20.28, n = 86; pollen transfer efficiency: mean ± SD: 55.14 ± 237.41, n = 70) and var. ustulata (male success: mean ± SD: 24.29 ± 19.81, n = 172; pollen transfer efficiency: mean ± SD: 83.07 ± 81.82, n = 151).
Efficiency in Pollinaria Removal Between Pollinator Guilds
While all tachinids but one, which probed the flower with the body in an upright position, removed pollinaria at their first attempt, some bees did not remove pollinaria even after three attempts. When testing the efficiency of pollinaria removal, we found that pollinator type had significant effects on pollinaria removal (χ2 = 7.01, df = 1, p < 0.01; Figure 9) but no effects of number of attempts on pollinaria removal success (χ2 = 2.34, df = 2, p = 0.31; Figure 9). Post hoc tests indicated that the efficiency of pollinaria removal was significantly higher within each attempt for T. fera compared to A. plumipes (z = −2.850, p ≤ 0.05; Figure 9).
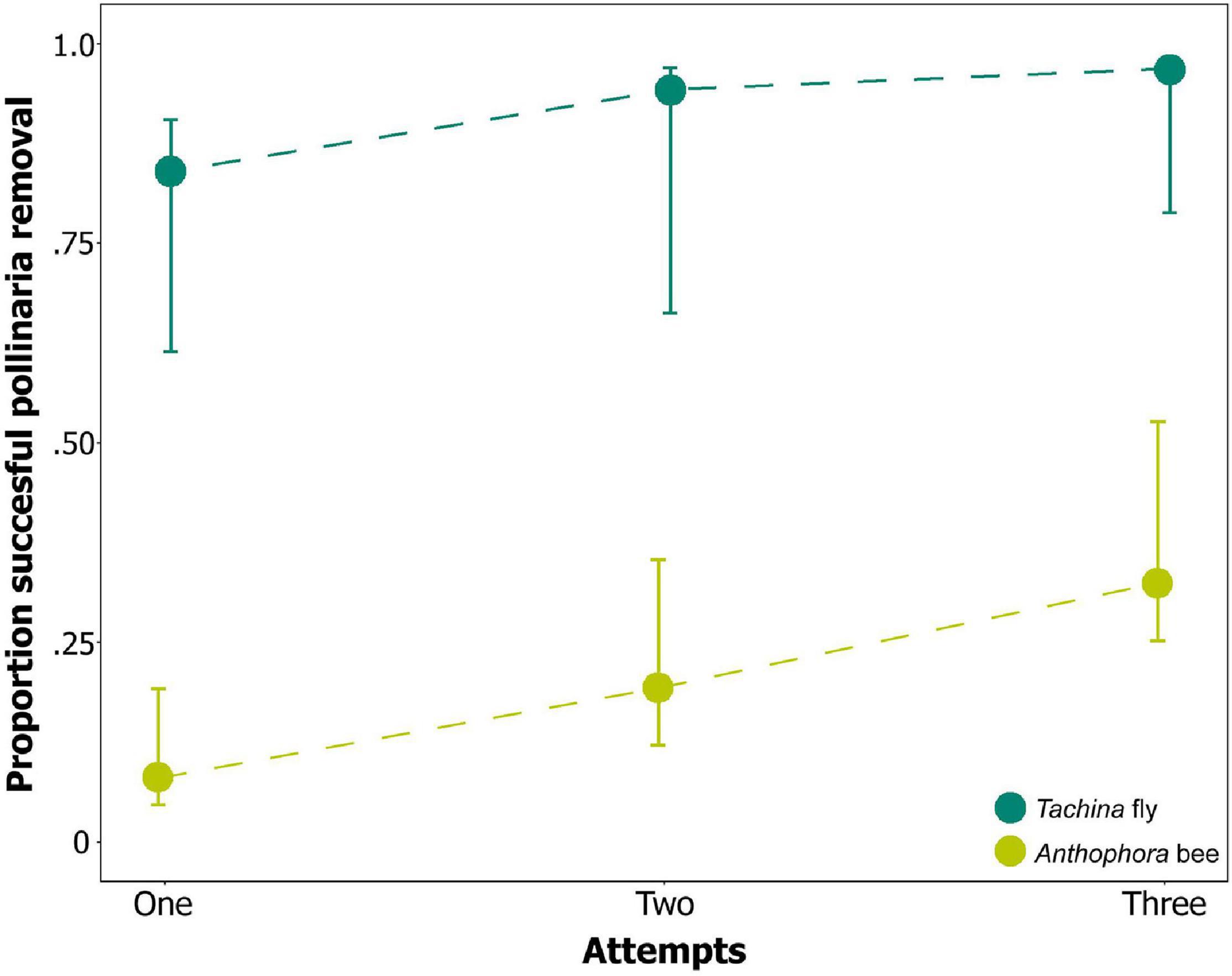
Figure 9. Pollinaria removal success in up to three attempts by the two main pollinator guilds of Neotinea ustulata, bees (Anthophora plumipes; n = 12) and flies (Tachina fera; n = 6). Back-transformed means and standard errors are shown.
The Role of Visual Traits in Pollination Success
Inflorescence display was significantly larger in N. ustulata var. aestivalis (mean ± SD: 758.1 ± 320.1 mm2) than in var. ustulata (mean ± SD: 543.9 ± 325.4 mm2; χ2 = 62.71, df = 1, p < 0.0001); whereas dark top display did not differ between N. ustulata var. ustulata (mean ± SD: 121.0 ± 35.8 mm2) and var. aestivalis (mean ± SD: 123.0 ± 31.5 mm2; χ2 = 0.20, df = 1, p = 0.655). We also did not find an effect of the dark top display on the probability of pollinaria removal (χ2 = 0.54, df = 1, p = 0.464), pollinia deposition (χ2 = 2.30, df = 1, p = 0.130) and pollination event (χ2 = 1.29, df = 1, p = 0.255). Although we also did not detect an effect of the total inflorescence display on the probability of pollinaria removal (χ2 = 2.11, df = 1, p = 0.15), effect of inflorescence display on pollinia deposition approached statistical significance (χ2 = 3.27, df = 1, p = 0.070) and was marginally non-significant for pollination events (χ2 = 3.74, df = 1, p = 0.053).
Role of Dark Top in Fly Pollinator Attraction
In our experiment with manipulation of the inflorescence dark top, there were significant effects of the treatment stage (χ2 = 5.68, df = 1, p < 0.05), the inflorescence type (i.e., selected for dark top excision or not; χ2 = 10.29, df = 1, p < 0.01) and the interaction treatment stage × inflorescence type (χ2 = 15.97, df = 1, p < 0.001) on the number of Tachina aff. fera visits to var. ustulata (Figure 10). In the case of var. aestivalis, we did not find significant effects of the treatment stage on the number of Nowickia ferox visits (χ2 = 0.017, df = 2, p = 0.99), however the inflorescence type (χ2 = 10.27, df = 1, p < 0.01) and the interaction treatment stage × inflorescence type (χ2 = 6.98, df = 1, p < 0.05) had significant effects on pollinator visits (Figure 10). Post hoc tests indicated that inflorescences with the dark top had significantly more visits than inflorescences without it (z = 3.507, p < 0.01; Figure 9), but no other pair-wise differences were found.
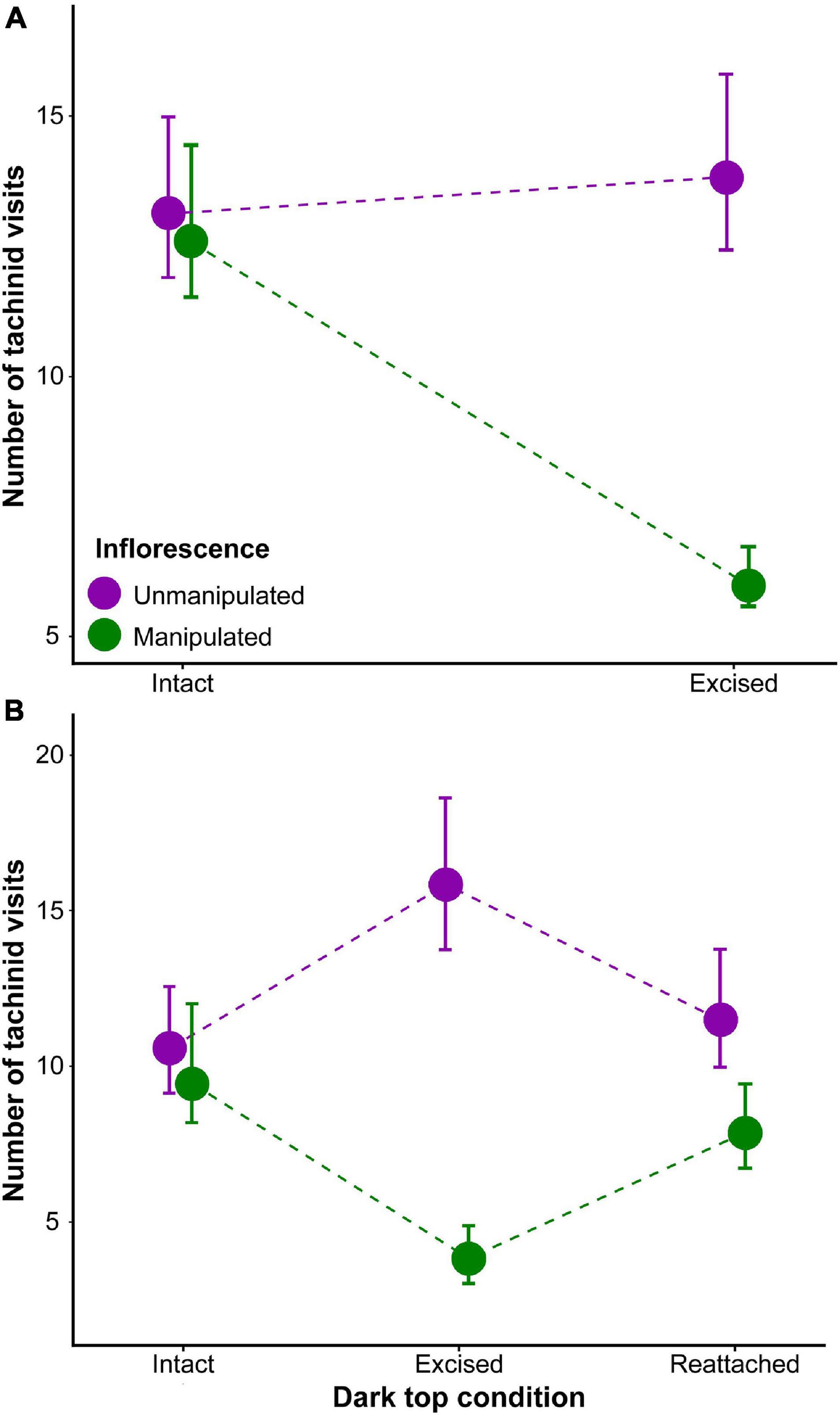
Figure 10. Attractiveness of inflorescence pairs of Neotinea ustulata varieties to pollinators: (A) Tachina aff. fera visits to var. ustulata inflorescences with and without the dark top excised from one of them. (B) Nowickia ferox visits to var. aestivalis inflorescences with, without and reattached dark top. Note the visits to the inflorescence decrease when dark top is visually absent to pollinators. Back-transformed means and standard errors are shown.
Discussion
Despite being widely distributed, Neotinea ustulata is one of the most enigmatic European orchids in terms of its reproductive biology. Our study reveals that morphological (e.g., larger flowers in var. ustulata), visual (e.g., spectral reflectance pattern) and chemical (e.g., pattern of semi- and low- volatile compounds) floral traits distinguish N. ustulata var. ustulata from var. aestivalis. Our observations across different locations in Europe also support some early records that N. ustulata is mainly pollinated by tachinid flies (Godfery, 1933; Vöth, 1984). However, the relative importance of tachinids differs between the two varieties, playing a predominant role as pollinator for the late flowering var. aestivalis. The complexity of floral traits and pollinator spectra of N. ustulata may indicate a complex, and not yet fully understood, food-deceptive mechanism.
Morphological, Visual, and Chemical Differences Between N. ustulata Varieties
Morphological Cues
Flower morphology can play a key role in pollination by promoting reproductive isolation, particularly in highly specialized pollination systems (Schiestl and Schlüter, 2009). For example, in the sexually deceptive orchid genus Chiloglottis R. Br., differences in floral morphology have been shown to be enough to achieve pollinator specificity in species relying on the same chemical attractant (de Jager and Peakall, 2016). Variations in floral size can also affect morphological fit and, therefore, pollen/pollinia transfer by their respective pollinators (Anderson and Johnson, 2008; Solís-Montero and Vallejo-Marín, 2017; de Jager and Peakall, 2019). Even relatively subtle differences in flower structures can have an impact on floral isolation such as the position of pollinaria in the moth pollinated Platanthera bifolia (L.) Rich. and P. chlorantha (Custer) Rchb., which are then attached on different parts of the pollinators (Nilsson, 1983; Schiestl and Schlüter, 2009). Although the differences in size among floral structures of N. ustulata var. ustulata and var. aestivalis were usually quite small, they are consistent even at the population level, and suggest that the two varieties might be morphologically adapted to different pollinator spectra, although phenotypic plasticity and non-pollinator selection should not be ruled out (Caruso et al., 2019; Sletvold, 2019). For instance, the width of the hood, the length of the lip and the length of the spur could all act as filters for potential pollinators. In fact, a previous study has already shown that the spur length of N. ustulata could be under pollinator-mediated selection (Trunschke et al., 2017). The role of flower traits for ensuring mechanical fit has been documented in diverse plant groups (e.g., Steiner and Whitehead, 1990; Muchhala, 2007; Anderson and Johnson, 2008; Pauw et al., 2009), including orchids (e.g., Chapurlat et al., 2015; Gögler et al., 2015; Rakosy et al., 2017; de Jager and Peakall, 2019). The smaller dimensions of N. ustulata var. aestivalis flowers could thus favor tachinids as pollinators and, conversely, larger flowers with deeper spur could favor a wider range of pollinators and improve pollinaria transfer. Assessment of mechanical fitting and behavioral experiments are necessary to test the role of morphology in N. ustulata pollinator filtering.
Visual Cues
Floral color is one of the main traits used by pollinators to identify both rewarding flowering plants and their deceptive mimics (Reverté et al., 2016). While all pollinators are able to respond to flower color signals, their preference for particular color hues and their ability to discriminate between them varies among groups (Reverté et al., 2016). Color reflectance patterns were structured at the population within and between varieties of N. ustulata. Although these differences can be related to phenotypic plasticity and non-pollinator-mediated selection (Ellis and Johnson, 2009; Caruso et al., 2019; Sletvold, 2019), pollinator preferences could be also involved. For instance, pollinator preferences have shaped daisy communities, which function as fly pollination specialist (Ellis et al., 2021). Variation in color hue among N. ustulata populations can be related to pollinator detectability and environmental conditions such as background context (e.g., coloration of surrounding plants and soil). Independently of the population or variety origin, the stark chromatic contrast between the dark top and the white lips can be discriminated by both bees and flies, and its presence may be result of pollinator selection (see below). This is supported by the occurrence of bright top forms (e.g., light pink or white top) in N. ustulata inflorescences, which are not stable and appear from time to time in different populations (Foley, 1990). Color pattern and color contrast have been shown to be important in pollinator attraction (Zhang et al., 2017), and the dark top coloration would play a similar role (see below). Experiments are needed to test the role of color pattern in the pollination success of N. ustulata varieties.
Chemical Cues
Both N. ustulata varieties produced a rather complex floral scent and the scent bouquets of N. ustulata individuals were highly variable. This pattern is not restricted to N. ustulata populations from Czech Republic since the floral scent of a var. ustulata plant from Sweden was similarly dominated by α-pinene, β-myrcene, limonene, eucalyptol, and (E)-β-ocimene (Kaiser, unpublished data). A study based on a plant from Italy also reported a complex bouquet including eucalyptol and D-limonene (i.e., D’auria et al., 2021); although we are cautious with the identity of some compounds also reported by the authors as they seem to be environmental contaminants. Differences between N. ustulata varieties were only detected when comparing compounds with low- and semi-volatility; however, larger scale sampling would be required to confirm that there are not differences between varieties when comparing highly volatile compounds. Differences in hydrocarbon compounds, which were predominant among compounds with lower volatility, could induce differential responses of pollinators once they approach or land on the flowers. Indeed, the function of these compounds, especially alkenes, has been intensively investigated in sexually deceptive orchids (Ayasse et al., 2011; Bohman et al., 2016). In fact, it is extremely unusual for food-deceptive species to produce such a high proportion of alkenes (Schiestl and Cozzolino, 2008), and in particular the production of (Z)-11-tricosene, which is extremely rare even among sexually deceptive plants but has been recorded in some tachinids (Martel et al., unpublished data). Therefore, alkenes and other low-volatile compounds could play a major role at short-range attraction in N. ustulata by eliciting certain behaviors in tachinid pollinators, while highly volatile compounds and visual cues remain probably the most important stimuli for long-range attraction. Further physiological and behavioral analyses are needed to elucidate the differential importance of N. ustulata floral scent in pollination.
Reproductive Success in N. ustulata Varieties
As typical for deceptive orchids, fruit set was rather low in most populations of N. ustulata. Reproductive success of N. ustulata was found to be higher than previously reported (i.e., Neiland, 2001; Harder and Johnson, 2008), but matches with those of some food-deceptive orchids (Gill, 1989; Fritz and Nilsson, 1994; Scopece et al., 2009, 2010; Trunschke et al., 2017). Contrary to previous reports (i.e., Tali et al., 2004; Haraštová-Sobotková et al., 2005), we did find differences in the characters associated with reproductive success between varieties. Furthermore, N. ustulata var. aestivalis was found to be slightly more successful than var. ustulata. Nevertheless, independently of the variety, the bottom and middle section of the inflorescence developed more fruits than the top section. This is consistent with gradual acropetalous opening of N. ustulata inflorescences and with tachinid behavior, as they always visit the freshest flowers on the top, but as flies habituate and more buds open, later opening flowers get less visits. This pattern is similar to those recorded in food-deceptive plants pollinated by bumblebees, which contrary to tachinids usually pursue the inflorescence from the bottom toward the top (Nilsson, 1980, 1983, 1984; Fritz, 1990; Jersáková and Kindlmann, 1998) and in sexually deceptive plants pollinated by bees and wasps, which learn to avoid deceitful plants (Peakall, 1990; Peakall and Handel, 1993; Ayasse et al., 2000). Thus, reproductive success pattern of N. ustulata indicates that pollinators are initially attracted to visit the plants, but then learn to avoid them.
Pollinator Spectra in N. ustulata Varieties
Diverse insect taxa visited flowers of both N. ustulata varieties, but only a few acted as true pollinators and the two varieties differ in their main pollinators. Both Anthophora and Tachina are able to remove pollinaria of N. ustulata var. ustulata with their proboscis, but Tachina flies were more efficient in doing so. Furthermore, while the tachinids were unable to detach the pollinaria from their proboscis, we frequently observed Anthophora bees grooming them off. Our observations of N. ustulata var. ustulata are in line with previous records in which bees and flies have been recorded (Paulus, 2005; Claessens and Kleynen, 2016). In contrast, our observations do not support the assumption that N. ustulata var. aestivalis is adapted to beetle pollination (see Danesch and Danesch, 1962; Mrkvicka, 1991; van der Cingel, 1995), though beetles can also act as occasional pollinators. While we observed a broad range of beetles, bees and even butterflies visiting the flowers of var. aestivalis, only Nowickia and Tachina were was consistently observed removing pollinaria and occasionally Bombus spp. Thus, N. ustulata var. aestivalis may have become more specialized for fly pollination than var. ustulata and this adaptation could be triggered by differences in availability of insects through space and time of flowering, and the higher efficiency of tachinids in transferring pollinia compared to bees. Despite the difference in pollinator spectra, pollen transfer efficiency was similar between both varieties, which would also be linked to their similar degrees of specialization or adaptation to fly pollinators. Our tachinid collection from Austrian and Czech populations suggests that the two N. ustulata varieties have one species of Nowickia and two of Tachina as main pollinators (i.e., N. ustulata var. ustulata is pollinated by T. fera and T. magnicornis, and var. aestivalis by T. magnicornis and N. ferox); however, differences in tachinid attraction can also be due to differences in species availability throughout the year and not specific adaption to one of them. Tachina fera and T. magnicornis are morphologically very similar, can co-occur and can be easily misidentified without a close inspection by a trained person. Although previous reports indicated that T. magnicornis flies were visiting N. ustulata var. ustulata flowers (e.g., Vöth, 1984; Paulus, 2005), some of those flies could have actually been T. fera (see Supplementary Table 6), which is more abundant and common than T. magnicornis (Paulus, pers. obs.). Fly pollination is rather rare among European orchids (Jersáková et al., 2016) and, to the best of our knowledge, no tachinid species has been recorded as the main pollinator of any European orchid. Tachinids have been previously reported in only a couple of specialized pollination systems such as those of Schizochilus (van der Niet et al., 2010) and Telipogon (Martel et al., 2016). Independently of the identity of their pollinators, both varieties have a similar male success and pollen transfer efficiency, but different female success. Hence, based on pollen transfer efficiency, pollinators of both varieties seem to be very efficient in delivering pollinia (i.e., a ratio of pollinia deposited to pollinia removed above 0.50). The pollinator efficiency is linked to the pollinator constancy as well as pollinator identity (Peter and Johnson, 2009). We therefore suggest that the reported efficiency may be related to tachinids, which seem to be more constant and are more efficient in removing pollinaria.
Role of the Dark Top in N. ustulata
We have reliably demonstrated that the dark top is an attractant signal of N. ustulata as inflorescences bearing the dark top received significantly more visits of tachinids than inflorescences without it. This increasing attractiveness can be result of a combined effect of color, shape and size of the dark top (i.e., visual stimuli). These findings appear to support the theory put forward almost 90 years ago by Godfery (1933), which considered that the dark top may be an adaptation to attract fly pollinators. The dark top of N. ustulata is quite unique among European orchids and although other species can also present a dark top (e.g., Orchis purpurea Huds.), they seem to have a far weaker contrast against the lips. Dark spots have also arisen in diverse plant groups such as Apiaceae, Asteraceae, Geraniaceae, Iridaceae, Liliaceae, Papaveraceae and Ranunculaceae (Dafni et al., 1990; Westmoreland and Muntan, 1996; Johnson and Midgley, 1997; van Kleunen et al., 2007; Goulson et al., 2009; Ellis and Johnson, 2010). It was suggested that dark, contrasting spots in flowers and inflorescences might even act as an insect mimic as demonstrated in some beetle- and fly-pollinated plants (e.g., Johnson and Midgley, 1997; van Kleunen et al., 2007; Goulson et al., 2009; Ellis and Johnson, 2010); however, at this point, it is unknown how tachinids are perceiving the contrasting dark top of N. ustulata. Although dark spots in flowers promotes pollinator attraction in some plants (e.g., Johnson and Midgley, 1997; van Kleunen et al., 2007; Goulson et al., 2009; Ellis and Johnson, 2010), it does not necessarily involve enhancement of fruit set (e.g., Westmoreland and Muntan, 1996; Johnson and Midgley, 1997; Ellis and Johnson, 2010). However, as dark spots promote visits of the main pollinators, their presence is likely to impact the fruit set of N. ustulata. In situ manipulation experiments would be needed to know how tachinids are perceiving the contrasting dark and its impact on pollination success.
Pollination Strategy of N. ustulata
Neotinea ustulata varieties are visited by generalist insects that seek for nectar and pollen (e.g., bees and flies), and the lip is bright white as in other food-deceptive plants (Johnson and Schiestl, 2016). However, other floral traits such as a strong and complex scent including alkenes, the evolutionary innovation of a dark top on the inflorescence and the predominance of tachinid male visitors (Martel et al., unpublished data) suggest that N. ustulata may have evolved a complex food-deceptive pollination system. Based on previous studies (i.e., Vogel, 1972; Bino et al., 1982; Valterová et al., 2007; Scopece et al., 2009), it seems that some orchid species potentially classified as food-deceptive have also evolved signals not usually associated with this strategy (e.g., some kind of chemical mimicry of their pollinators) and their pollinators are gender biased (i.e., attract predominantly female or male pollinators). This may also occur in N. ustulata as most abundant alkenes have been also detected in Tachina flies (Martel et al., unpublished data). Most floral visitors of N. ustulata are only seeking food, but tachinids would in addition perceive alkenes as a signal of other yet unknown resources. For instance, alkenes are key in the chemical mimicry and pollinator attraction of the sexually deceptive Telipogon peruvianus T.Hashim. to its tachinid specialist pollinator (Martel et al., 2019). In other complex pollination systems, the fly-pollinated Gorteria diffusa Thunb. (Asteraceae) attracts flies seeking food, but male flies are also sexually attracted and try to copulate with the black petal ornamentation (Ellis and Johnson, 2010); similarly, some populations of the beetle-pollinated Luisia teres Gaudich attracts nectar-feeding females and male beetles, whereas others sexually attract males (Sugiura et al., 2021). Hence, the combination of traits present in N. ustulata indicates that this orchid would exploit not only food-based signals but also sexual ones (e.g., gender-specific pheromones). To address this issue further studies should identify physiologically active scent compounds and carry out behavioral bioassays.
Data Availability Statement
The raw data supporting the conclusions of this article will be made available by the authors, without undue reservation.
Author Contributions
CM, DR, MA, HP, and JJ conceived the study. CM, DR, SD, SJ, MA, HP, and JJ designed the study. CM, DR, HP, LN, HM, and JJ collected the data. CM, DR, SD, SJ, and JJ analyzed the data. CM, DR, and JJ drafted the first version of the manuscript. CM wrote the final version. All authors contributed to manuscript revision and read and approved the submitted version.
Funding
This work was partially funded by DAAD and MŠMT to MA and JJ (DAAD-16-13/2016-2017 and 7AMB16DE003, respectively).
Conflict of Interest
The authors declare that the research was conducted in the absence of any commercial or financial relationships that could be construed as a potential conflict of interest.
The reviewer, GS, declared a past co-authorship with several of the authors, CM, MA, to the handling editor.
Acknowledgments
We thank Jaromír Vaňhara, Peter Sehnal, and Hans-Peter Tschorsnig for tachinid determination; Ivana Jongepierová, Karel Fajmon, Vlastimil Vlačiha, Dano Dítě for locations of N. ustulata populations. We also thank Casper van der Kooi and Ewan Bodenham for the invitation and support to publish our study in this special issue. Roxana Rojas kindly prepared the map shown in Figure 2. The reviewers make very helpful comments that improved an earlier version of the manuscript. DAAD and MŠMT supported this study by a shared Grant to JJ (7AMB16DE003) and MA (DAAD-16-13/2016-2017). CM thanks the Max Planck Partner Group for providing financial supports at PUCP.
Supplementary Material
The Supplementary Material for this article can be found online at: https://www.frontiersin.org/articles/10.3389/fevo.2021.659176/full#supplementary-material
References
Adams, R. P. (2017). Identification of Essential Oil Components by Gas Chromatograph/Mass Spectrometry, 5th Edn. Carol Stream, IL: Allured Publishing Corporation.
An, L., Neimann, A., Eberling, E., Algora, H., Brings, S., and Lunau, K. (2018). The yellow specialist: dronefly Eristalis tenax prefers different yellow colours for landing and proboscis extension. J. Exp. Biol. 221:jeb184788. doi: 10.1242/jeb.184788
Anderson, B., and Johnson, S. D. (2008). The geographical mosaic of coevolution in a plant-pollinator mutualism. Evolution 62, 220–225. doi: 10.1111/j.1558-5646.2007.00275.x
Ayasse, M., Schiestl, F. P., Paulus, H. F., Löfstedt, C., Hansson, B. S., Ibarra, F., et al. (2000). Evolution of reproductive strategies in the sexually deceptive orchid Ophrys sphegodes: how does flower-specific variation of odour signals influence reproductive success? Evolution 54, 1995–2006. doi: 10.1111/j.0014-3820.2000.tb01243.x
Ayasse, M., Stokl, J., and Francke, W. (2011). Chemical ecology and pollinator-driven speciation in sexually deceptive orchids. Phytochemistry 72, 1667–1677. doi: 10.1016/j.phytochem.2011.03.023
Bates, D., Maechler, M., Bolker, B., Walker, S., Bojesen Christensen, R. H., Singmann, H., et al. (2019). lme4: linear mixed-effects models using ‘eigen’ and s4. R package version 1.1-21. Available Online at: https://cran.r-project.org/package=lme4/.
Baumann, H., Blatt, H., Kretzschmar, H., and Eccarius, W. (2005). “Die Orchideentaxa,” in Die Orchideen Deutschlands, eds H. Blatt, W. Eccarius, and H. Kretzschmar (Uhlstädt-Kirchhasel: Verlag Arbeitskreise Heimische Orchideen), 223–685.
Bino, R. J., Dafni, A., and Meeuse, A. D. J. (1982). The pollination ecology of Orchis galilaea (Bornm. et Schulze) Schltr. (Orchidaceae). New Phytol. 90, 315–319. doi: 10.1111/j.1469-8137.1982.tb03263.x
Bohman, B., Flematti, G. R., Barrow, R. A., Pichersky, E., and Peakall, R. (2016). Pollination by sexual deception - it takes chemistry to work. Curr. Opin. Plant Biol. 32, 37–46. doi: 10.1016/j.pbi.2016.06.004
Borsos, O. (1962). Geobotanische monographie der orchideen der pannonischen und karpatischen Flora VI. Sec. Biol. 5, 27–61.
Carlson, D. A., Mayer, M. S., Silhacek, D. L., James, L. D., Beroza, M., and Bierl, B. A. (1971). Sex attractant pheromone of the house fly: isolation, identification and synthesis. Science 174, 76–77. doi: 10.1126/science.174.4004.76
Caruso, C. M., Eisen, K. E., Martin, R. A., and Sletvold, N. (2019). A meta-analysis of the agents of selection on floral traits. Evolution 73, 4–14. doi: 10.1111/evo.13639
Chapurlat, E., Ågren, J., and Sletvold, N. (2015). Spatial variation in pollinator-mediated selection on phenology, floral display and spur length in the orchid Gymnadenia conopsea. New Phytol. 208, 1264–1275. doi: 10.1111/nph.13555
Chittka, L. (1992). The colour hexagon: a chromaticity diagram based on photoreceptor excitations as a generalized representation of colour opponency. J. Comp. Physiol. A 170, 533–543. doi: 10.1007/BF00199331
Chittka, L., and Kevan, P. G. (2005). “Flower colour as advertisement,” in Practical Pollination Biology, eds A. Dafni, P. G. Kevan, and B. C. Husband (Cambridge, ON: Enviroquest), 157–196.
Claessens, J., and Kleynen, J. (2011). The Flower of the European Orchid. Form and Function. Geulle: Jean Claessens and Jacques Kleynen Publishers.
Claessens, J., and Kleynen, J. (2016). Orchidées d’Europe - Fleur et Pollinisation. Mèze: Biotope éditions.
Dafni, A., Bernhardt, P., Schmida, A., Ivri, Y., Greenbaum, S., O’Toole, C., et al. (1990). Red bowl-shaped flowers: convergence for beetle pollination in the Mediterranean region. Israel J. Bot. 39, 81–92. doi: 10.1080/0021213X.1990.10677134
D’auria, M., Lorenz, R., Mecca, M., Racioppi, R., Romano, V. A., and Viggiani, L. (2021). The scent of Neotinea orchids from basilicata (southern Italy). Nat. Prod. Res. 2021, 1–3. doi: 10.1080/14786419.2020.1871343
de Jager, M. L., and Peakall, R. (2016). Does morphology matter? An explicit assessment of floral morphology in sexual deception. Funct. Ecol. 30, 537–546. doi: 10.1111/1365-2435.12517
de Jager, M. L., and Peakall, R. (2019). Experimental examination of pollinator-mediated selection in a sexually deceptive orchid. Ann. Bot. 123, 347–354. doi: 10.1093/aob/mcy083
Delforge, P. (2006). Orchids of Europe, North Africa and the Middle East. London: A and C Black Publishers Ltd.
Ellis, A. G., Anderson, B., and Kemp, J. E. (2021). Geographic mosaics of fly pollinators with divergent color preferences drive landscape-scale structuring of flower color in daisy communities. Front. Plant Sci. 12:617761. doi: 10.3389/fpls.2021.617761
Ellis, A. G., and Johnson, S. D. (2009). The evolution of floral variation without pollinator shifts in Gorteria diffusa (Asteraceae). Am. J. Bot. 96, 793–801. doi: 10.3732/ajb.0800222
Ellis, A. G., and Johnson, S. D. (2010). Floral mimicry enhances pollen export: the evolution of pollination by sexual deceit outside of the Orchidaceae. Am. Nat. 176, E143–E151. doi: 10.1086/656487
Fay, M. F. (2018). Orchid conservation: how can we meet the challenges in the twenty-first century? Bot. Stud. 9:16. doi: 10.1186/s40529-018-0232-z
Foley, M. J. Y. (1990). The current distribution and abundance of Orchis ustulata L. in southern England. Watsonia 18, 37–48.
Fritz, A., and Nilsson, L. A. (1994). How pollinator-mediated mating varies with population size in plants. Oecologia 100, 451–462. doi: 10.1093/beheco/arh147
Fritz, A. L. (1990). Deceit pollination of Neotinea spitzelii (Orchidaceae) on the island of Gotland in the Baltic: a suboptimal system. Nord. J. Bot. 9, 577–587. doi: 10.1111/j.1756-1051.1990.tb00548.x
Galizia, C. G., Kunze, J., Gumbert, A., Borg-Karlson, A.-K., Sachse, S., Markl, C., et al. (2004). Relationship of visual and olfactory signal parameters in a food-deceptive flower mimicry system. Behav. Ecol. 16, 159–168.
Gill, D. E. (1989). “Fruiting failure, pollinator inefficiency and speciation in orchids,” in Speciation and its Consequences, eds D. Otte and J. A. Endler (Sunderland, MA: Sinauer), 458–481.
Godfery, M. J. (1933). Monograph and Iconograph of Native British Orchidaceae. Cambridge: Cambridge University Press.
Gögler, J., Stökl, J., Cortis, P., Beyrle, H., Rosaria, M., Lumaga, B., et al. (2015). Increased divergence in floral morphology strongly reduces gene flow in sympatric sexually deceptive orchids with the same pollinator. Evol. Ecol. 29, 703–717. doi: 10.1007/s10682-015-9779-2
Goulson, D., McGuire, K., Munro, E. E., Adamson, S., Colliar, L., Park, K. J., et al. (2009). Functional significance of the dark central floret of Daucus carota (Apiaceae) L.; is it an insect mimic? Plant Species Biol. 24, 77–82. doi: 10.1111/j.1442-1984.2009.00240.x
Haraštová-Sobotková, M., Jersáková, J., Kindlmann, P., and Čurn, L. (2005). Morphometric and genetic divergence among populations of Neotinea ustulata (Orchidaceae) with different flowering phenologies. Folia Geobot. 40, 385–405. doi: 10.1007/BF02804287
Harder, L. D., and Johnson, S. D. (2008). Function and evolution of aggregated pollen in angiosperms. Int. J. Plant Sci. 169, 59–78. doi: 10.1086/523364
Hedlund, K., Bartelt, R. J., Dicke, M., and Vet, L. E. M. (1996). Aggregation pheromones of Drosophila immigrans, D. phalerata, and D. suboscura. J. Chem. Ecol. 22, 1835–1844. doi: 10.1007/BF02028507
Jacquemyn, H., Brys, R., Hermy, M., and Willems, J. O. (2005). Does nectar reward affect rarity and extinction probabilities of orchid species? An assessment using historical records from Belgium and the Netherlands. Biol. Conserv. 121, 226–257. doi: 10.1016/j.biocon.2004.05.002
Jensen, J. M., and Pedersen, H. A. (1999). Ny lokalitet for Bakke-Gögehurt (Orchis ustulata) - med noter om artens fänologiske og morfologiske variation. Flora og fauna 105, 29–36.
Jersáková, J., Johnson, S. D., and Kindlmann, P. (2006). Mechanisms and evolution of deceptive pollination in orchids. Biol. Rev. 81, 219–235. doi: 10.1017/S1464793105006986
Jersáková, J., Jürgens, A., Smilauer, P., and Johnson, S. D. (2012). The evolution of floral mimicry: identifying traits that visually attract pollinators. Funct. Ecol. 26, 1381–1389. doi: 10.1111/j.1365-2435.2012.02059.x
Jersáková, J., and Kindlmann, P. (1998). Patterns of pollinator-generated fruit set in Orchis morio (Orchidaceae). Folia Geobot. 33, 377–390. doi: 10.1007/BF02803641
Jersáková, J., Spaethe, J., Streinzer, M., Neumayer, J., Paulus, H., Dötterl, S., et al. (2016). Does Traunsteinera globosa (the globe orchid) dupe its pollinators through generalized food deception or mimicry? Bot. J. Linn. Soc. 180, 269–294. doi: 10.1111/boj.12364
Johnson, S. D., and Midgley, J. J. (1997). Fly pollination of Gorteria diffusa (Asteraceae), and a possible mimetic function for dark spots on the capitulum. Am. J. Bot. 84, 429–436. doi: 10.2307/2446018
Kassambara, A., and Mundt, F. (2020). factoextra: extract and visualize the results of multivariate data analyses. R package version 1.0.7. Available Online at: https://cran.r-project.org/package=factoextra/.
Kümpel, H., and Mrkvicka, A. C. (1990). Untersuchungen zur Abtrennung der Neotinea ustulata L. subsp. aestivalis (Kümpel) Kümpel and Mrkvicka. Mitt. Bl. Arbeitskr. Heim. Orch. Baden-Württ. 22, 306–324.
Lenth, R., Singmann, H., Love, J., Buerkner, P., and Herve, M. (2020). emmeans: estimated marginal means, aka least-squares means. R package version 1.4.8. Available Online at: http://cran.r-project.org/package=emmeans/
Maia, R., White, T., Gruson, H., Endler, J., Eliason, C., and Bitton, P.-P. (2021). pavo: perceptual analysis, visualization and organization of spectral colour data. R package version 2.4.0. Available Online at: https://cran.r-project.org/package=pavo/.
Martel, C., Cairampoma, L., Stauffer, F. W., and Ayasse, M. (2016). Telipogon peruvianus (Orchidaceae) flowers elicit pre-mating behaviour in Eudejeania (Tachinidae) males for pollination. PLoS One 11:e0165896. doi: 10.1371/journal.pone.0165896
Martel, C., Francke, W., and Ayasse, M. (2019). The chemical and visual bases of the pollination of the Neotropical sexually deceptive orchid Telipogon peruvianus. New Phytol. 223, 1989–2001. doi: 10.1111/nph.15902
Mrkvicka, A. C. (1991). Bestäuber, Chromosomenzahl und weitere Beobachtungen zu Neotinea ustulata L. subsp. aestivalis (Kümpel) Kümpel and Mrkvicka. Mitt. Bl. Arbeitskr. Heim. Orch. Baden-Württ. 23, 331–338.
Muchhala, N. (2007). Adaptive trade-off in floral morphology mediates specialization for flowers pollinated by bats and hummingbirds. Am. Nat. 169, 494–504.
Neiland, R. M. (2001). “Neotinea: ecology,” in Genera Orchidacearum 2. Orchidoideae, Part 1, eds A. M. Pridgeon, P. J. Cribb, M. W. Chase, and F. N. Rasmussen (Oxford, UK: Oxford University Press), 320–323.
Nilsson, L. A. (1980). The pollination ecology of Dactylorhiza sambucina (Orchidaceae). Bot. Not. 133, 367–385.
Nilsson, L. A. (1983). Processes of isolation and introgressive interplay between Platanthera bifolia (L.) Rich. and Platanthera chlorantha (Custer) Reichb. (Orchidaceae). Bot. J. Linn. Soc. 87, 325–350. doi: 10.1111/j.1095-8339.1983.tb00997.x
Nilsson, L. A. (1984). Anthecology of O. morio (Orchidaceae) and its outpost in the North. Nova Acta Regiae Soc. Sci. Upsal. 3, 167–180.
Nunes, C. E. P., Amorim, F. W., Mayer, J. L. S., and Sazima, M. (2016). Pollination ecology of two species of Elleanthus (Orchidaceae): novel mechanisms and underlying adaptations to hummingbird pollination. Plant Biol. 18, 15–25. doi: 10.1111/plb.12312
Oksanen, J., Blanchet, F. G., Friendly, M., Kindt, R., Legendre, P., McGlinn, D., et al. (2019). vegan: community ecology package. R package version 2.5-6. Available Online at: https://cran.r-project.org/package=vegan/.
Paulus, H. F. (2005). “Zur Bestäubungsbiologie der Orchideen,” in Die Orchideen Deutschlands, eds H. Blatt, W. Eccarius, and H. Kretzschmar (Uhlstädt-Kirchhasel: Verlag Arbeitskreise Heimische Orchideen), 98–140.
Pauw, A., Stofberg, J., and Waterman, R. J. (2009). Flies and flowers in Darwin’s race. Evolution 63, 268–279. doi: 10.1111/j.1558-5646.2008.00547.x
Peakall, R. (1990). Responses of male Zaspilothynnus trilobatus Turner wasps to females and the sexually deceptive orchid it pollinates. Funct. Ecol. 4, 159–167. doi: 10.2307/2389335
Peakall, R., and Handel, S. N. (1993). Pollinators discriminate among floral heights of a sexually deceptive orchid: implications for selection. Evolution 47, 1681–1687. doi: 10.1111/j.1558-5646.1993.tb01260.x
Peter, C. I., and Johnson, S. D. (2009). Reproductive biology of Acrolophia cochlearis (Orchidaceae): estimating rates of cross-pollination in epidendroid orchids. Ann. Bot. 104, 573–581. doi: 10.1093/aob/mcn218
R Core Team. (2017). R: a language and environment for statistical computing. Vienna: R Foundation for Statistical Computing.
Rakosy, D., Cuervo, M., Paulus, H. F., and Ayasse, M. (2017). Looks matter: changes in flower form affect pollination effectiveness in a sexually deceptive orchid. J. Evol. Biol. 30, 1978–1993. doi: 10.1111/jeb.13153
Renner, S. S. (2005). “Rewardless flowers in the angiosperms and the role of insect cognition in their evolution,” in Plant-Pollinator Interactions: from Specialization to Generalization, eds N. M. Waser and J. Olerton (Chicago, IL: University of Chicago Press), 123–144.
Renoult, J. P., Valido, A., Jordano, P., and Schaefer, H. M. (2014). Adaptation of flower and fruit colours to multiple, distinct mutualists. New Phytol. 201, 678–686. doi: 10.1111/nph.12539
Reverté, S., Retana, J., Gómez, J. M., and Bosch, J. (2016). Pollinators show flower colour preferences but flowers with similar colours do not attract similar pollinators. Ann. Bot. 118, 249–257. doi: 10.1093/aob/mcw103
Ripley, B., Venables, B., Bates, D. M., Hornik, K., Gebhardt, A., and Firth, D. (2020). MASS: support functions and datasets for Venables and Ripley’s MASS. R package version 7.3-51.6. Available Online at: http://cran.r-project.org/package=MASS/
Rogoff, W. M., Gretz, G. H., Jacobson, M., and Beroza, M. (1973). Confirmation of (Z)-9-tricosene as a sex pheromone of the housefly. Ann. Entomol. Soc. Am. 66, 739–741. doi: 10.1093/aesa/66.4.739
Schiestl, F. P., and Cozzolino, S. (2008). Evolution of sexual mimicry in the orchid subtribe Orchidinae: the role of preadaptations in the attraction of male bees as pollinators. BMC Evol. Biol. 8:27. doi: 10.1186/1471-2148-8-27
Schiestl, F. P., and Schlüter, P. M. (2009). Floral isolation, specialized pollination, and pollinator behavior in orchids. Annu. Rev. Entomol. 54, 425–446. doi: 10.1146/annurev.ento.54.110807.090603
Scopece, G., Cozzolino, S., Johnson, S. D., and Schiestl, F. P. (2010). Pollination efficiency and the evolution of specialized deceptive pollination systems. Am. Nat. 175, 98–105. doi: 10.1086/648555
Scopece, G., Juillet, N., Müller, A., Schiestl, F. P., and Cozzolino, S. (2009). Pollinator attraction in Anacamptis papilionacea (Orchidaceae): a food or a sex promise? Plant Species Biol. 24, 109–114. doi: 10.1111/j.1442-1984.2009.00244.x
Shrestha, M., Dyer, A. G., Dorin, A., Ren, Z. X., and Burd, M. (2020). Rewardlessness in orchids: how frequent and how rewardless? Plant Biol. 22, 555–561. doi: 10.1111/plb.13113
Signorell, A. (2020). DescTools: tools for descriptive statistics. R package version 0.99.36. Available Online at: http://cran.r-project.org/package= DescTools/Google Scholar
Simón-Porcar, V. I., Silva, J. L., Meeus, S., Higgins, J. D., and Vallejo-Marín, M. (2017). Recent autopolyploidization in a naturalized population of Mimulus guttatus (Phrymaceae). Bot. J. Linn. Soc. 185, 189–207. doi: 10.1093/botlinnean/box052
Sletvold, N. (2019). The context dependence of pollinator-mediated selection in natural populations. Int. J. Plant Sci. 180, 934–943. doi: 10.1086/705584
Solís-Montero, L., and Vallejo-Marín, M. (2017). Does the morphological fit between flowers and pollinators affect pollen deposition? An experimental test in a buzz-pollinated species with anther dimorphism. Ecol. Evol. 7, 2706–2715. doi: 10.1002/ece3.2897
Stace, C. A. (2010). New Flora of the British Isles, 3rd Edn. Cambridge: Cambridge University Press.
Steiner, K. E., and Whitehead, V. B. (1990). Pollinator adaptation to oil-secreting flowers-Rediviva and Diascia. Evolution 44, 1701–1707. doi: 10.2307/2409348
Streinzer, M., Ellis, T., Paulus, H. F., and Spaethe, J. (2010). Visual discrimination between two sexually deceptive Ophrys species by a bee pollinator. Arthropod-Plant Int. 4, 141–148. doi: 10.1007/s11829-010-9093-4
Sugiura, N., Matsumura, S., and Yokota, M. (2021). Beetle pollination of Luisia teres (Orchidaceae) and implications of a geographic divergence in the pollination system. Plant Species Biol. 36, 52–59. doi: 10.1111/1442-1984.12294
Tali, K. (2004). Species structure of Neotinea ustulata. Doctoral thesis. Estonia: Institute of Botany and Ecology, University of Tartu.
Tali, K., Fay, M. F., and Bateman, R. M. (2006). Little genetic differentiation across Europe between early-flowering and late-flowering populations of the rapidly declining orchid Neotinea ustulata. Biol. J. Linn. Soc. 87, 13–25. doi: 10.1111/j.1095-8312.2006.00550.x
Tali, K., Foley, M. J. Y., and Kull, T. (2004). Orchis ustulata L. J. Ecol. 92, 174–184. doi: 10.1111/j.1365-2745.2004.00858.x
Trunschke, J., Sletvold, N., and Ågren, J. (2017). Interaction intensity and pollinator-mediated selection. New Phytol. 214, 1381–1389. doi: 10.1111/nph.14479
Valenta, K., Nevo, O., Martel, C., and Chapman, C. A. (2017). Plant attractants: integrating insights from pollination and seed dispersal ecology. Evol. Ecol. 31, 249–267. doi: 10.1007/s10682-016-9870-3
Valterová, I., Kunze, J., Gumbert, A., Luxová, A., Liblikas, I., Kalinová, B., et al. (2007). Male bumble bee pheromonal components in the scent of deceit pollinated orchids; unrecognized pollinator cues? Arthropod-Plant Int. 1, 137–145. doi: 10.1007/s11829-007-9019-y
van der Cingel, N. A. (1995). An Atlas of Orchid Pollination: European orchids. Rotterdam: A. A. Balkema.
van der Niet, T., Jürgens, A., and Johnson, S. D. (2010). Pollinators, floral morphology and scent chemistry in the southern African orchid genus Schizochilus. S. Afr. J. Bot. 76, 726–738. doi: 10.1016/j.sajb.2010.07.004
van Kleunen, M., Nanni, I., Donaldson, J. S., and Manning, J. C. (2007). The role of beetle marks and flower colour on visitation by monkey beetles (Hopliini) in the greater cape floral region, South Africa. Ann. Bot. 100, 1483–1489. doi: 10.1093/aob/mcm256
Vereecken, N. J., and Schiestl, F. P. (2009). On the roles of colour and scent in a specialized floral mimicry system. Ann. Bot. 104, 1077–1084. doi: 10.1093/aob/mcp208
Vogel, S. (1972). Pollination von Orchis papilionacea L. in den Schwarmbahnen von Eucera tuberculata F. Jahresber. Naturw. Ver. Wuppertal 25, 67–74.
Vorobyev, M., and Osorio, D. (1998). Receptor noise as a determinant of colour thresholds. Proc. R. Soc. Lond. B Biol. Sci. 265, 351–358. doi: 10.1098/rspb.1998.0302
Vöth, W. (1984). Echinomyia magnicornis Zett. Bestäuber von Neotinea ustulata L. Die Orchidee 35, 189–192.
Vöth, W. (1989). Die Bestäuber von Orchis papilionacea L. (Orchidaceae). Linzer Biol. Beitr. 21, 391–404.
Westmoreland, D., and Muntan, C. (1996). The influence of dark central florets on insect attraction and fruit production in Queen Anne’s lace (Daucus carota L.). Am. Midl. Nat. 135, 122–129. doi: 10.1111/nph.13555
Wickham, H., Chang, W., Henry, L., Pedersen, T. L., Takahashi, K., Wilke, C., et al. (2020). ggplot2: create elegant data visualisations using the grammar of graphics. R package version 3.3.0. Available Online at: https://cran.r-project.org/package=ggplot2/.
Wucherpfennig, W. (1992). Spätblühende sippen von Orchis ustulata L. Ber. Bayer. Bot. Ges. 63, 33–35.
Keywords: food deception, flower color, flower morphology, floral scent, pollinator efficiency, pollinator specialization
Citation: Martel C, Rakosy D, Dötterl S, Johnson SD, Ayasse M, Paulus HF, Nilsson LA, Mejlon H and Jersáková J (2021) Specialization for Tachinid Fly Pollination in the Phenologically Divergent Varieties of the Orchid Neotinea ustulata. Front. Ecol. Evol. 9:659176. doi: 10.3389/fevo.2021.659176
Received: 27 January 2021; Accepted: 14 May 2021;
Published: 16 June 2021.
Edited by:
Johannes Spaethe, Julius Maximilian University of Würzburg, GermanyReviewed by:
Judith Trunschke, Kunming Institute of Botany, Chinese Academy of Sciences, ChinaGiovanni Scopece, University of Naples Federico II, Italy
Copyright © 2021 Martel, Rakosy, Dötterl, Johnson, Ayasse, Paulus, Nilsson, Mejlon and Jersáková. This is an open-access article distributed under the terms of the Creative Commons Attribution License (CC BY). The use, distribution or reproduction in other forums is permitted, provided the original author(s) and the copyright owner(s) are credited and that the original publication in this journal is cited, in accordance with accepted academic practice. No use, distribution or reproduction is permitted which does not comply with these terms.
*Correspondence: Carlos Martel, Y2FybG9zLm1hcnRlbEBwdWNwLnBl
†These authors have contributed equally to this work