- 1Plant Ecology, Department of Biosciences, Paris Lodron University of Salzburg, Salzburg, Austria
- 2Institut für Botanik, Technische Universität Dresden, Dresden, Germany
- 3Institute of Biology, Universität Graz, Graz, Austria
- 4Department of Zoology, University of Cambridge, Cambridge, United Kingdom
Deceptive flowers decoy pollinators by advertising a reward, which finally is not provided. Numerous deceptive plants are pollinated by Diptera, but the attractive cues and deceptive strategies are only identified in a few cases. A typical fly-deceptive plant genus is Aristolochia, which evolved sophisticated trap flowers to temporarily capture pollinators. Though rarely demonstrated by experimental approaches, Aristolochia species are believed to chemically mimic brood sites, food sources for adult flies, or utilize sexual deception. Indeed, for most species, studies on scent composition and attractive signals are lacking. In this study, we focused on Aristolochia microstoma, a peculiar Greek endemic with flowers that are presented at ground level in the leaf litter or between rocks and are characterized by a unique morphology. We analyzed flower visitor and pollinator spectra and identified the floral scent composition using dynamic headspace and gas chromatography coupled to mass spectrometry (GC/MS). Female and male phorid flies (Phoridae) are the exclusive pollinators, although the flowers are also frequently visited by Sciaridae, as well as typical ground-dwelling arthropods, such as Collembola and arachnids. The carrion-like floral scent mainly consists of the oligosulphide dimethyldisulfide and the nitrogen-bearing compound 2,5-dimethylpyrazine. These compounds together are known to be released from decomposing insects, and thus, we conclude that pollinators are likely deceived by chemical imitation of invertebrate carrion, a deceptive strategy not described from another plant species so far.
Introduction
Deceptive pollination evolved in 4–6% of angiosperms (Renner, 2006), and relies on the inability of pollinators to distinguish between a true resource (e.g., mating partners, brood-sites, and food) and the flower/inflorescence that imitates the reward (Brodmann et al., 2008, 2009; Urru et al., 2011). Pollinators are cheated by deceptive flowers through sophisticated olfactory, visual, and tactile traits (Vogel, 1978; Dafni, 1984; Stensmyr et al., 2002; Schiestl et al., 2003; Schiestl, 2005; Stökl et al., 2010; Woodcock et al., 2014). In such systems, Diptera are common pollinators (Renner, 2006; Woodcock et al., 2014). Fly-deceptive pollination strategies include mimicry of brood-sites (Stensmyr et al., 2002; Urru et al., 2011; Jürgens et al., 2013), food (e.g., Heiduk et al., 2016), and mating partners (Martel et al., 2016). However, the specific signals involved in fly attraction and the deceptive strategies are identified in a few cases only (Stökl et al., 2010; Heiduk et al., 2015, 2016; Oelschlägel et al., 2015).
A prominent example of fly-pollinated deceptive plants is the genus Aristolochia (Aristolochiaceae). The different species are visited by a wide range of dipteran families, but often information on the actual pollinators is lacking (reviewed in Berjano et al., 2009). However, there is evidence that each Aristolochia species is specialized in just one or few pollinator families (e.g., Phoridae, Drosophilidae, and Chloropidae), and in some species fly attraction is sex-specific (Hime and Costa, 1985; Wolda and Sabrosky, 1986; Hall and Brown, 1993; Rulik et al., 2008; Berjano et al., 2009). Aristolochia species are long known for their spectacular, highly derived trap flowers (Knoll, 1929). To assure cross-pollination, the plants have evolved elaborate micro- and macromorphological features, enabling them to trap, retain, and release insects [described in detail by Oelschlägel et al. (2009)]. Pollinators enter the protogynous flower in the female phase through the tube, where downward-bending trichomes lead them to the utricle, and prevent them from escaping during the female flower phase (Oelschlägel et al., 2009). The trapped pollinators are able to deposit pollen, previously picked up from another flower, on the receptive stigmatic lobes before the flower enters the male phase. In the early male phase the pollen is released, but trapping trichomes still block the exit, before they finally shrink and allow pollinators to leave the trap, loaded with pollen (Oelschlägel et al., 2009).
Due to their often obvious and strong scents during the female phase, many authors suggested that Aristolochia flowers generally attract their pollinators by floral scent (Vogel, 1978; Hall and Brown, 1993; Bänziger and Disney, 2006; Trujillo and Séric, 2006; Rulik et al., 2008; Martin et al., 2017), which indeed was substantiated by behavioral assays in a few species (Cammerloher, 1923; Daumann, 1971; Oelschlägel et al., 2015). Based on the type of scent released, it is believed that the flowers generally mimic brood-sites of their respective pollinators, such as carrion, feces, decaying plants, or fungi, by chemical deception (Cammerloher, 1923; Vogel, 1978; Proctor et al., 1996; Martin et al., 2017). In some weakly odored species with strongly male- or female-biased pollinator attraction, mimicry of sex pheromones was suggested (Wolda and Sabrosky, 1986; Hall and Brown, 1993). First attempts to identify floral scent compounds in Aristolochia date back almost 100 years (Cammerloher, 1923, 1933), but the scent composition was only studied recently in four species by quantitative chemical analytics (Stashenko et al., 2009; Johnson and Jürgens, 2010; Oelschlägel et al., 2015; Martin et al., 2017). Among other compounds (e.g., citral), all these studies identified substances characteristic of brood-site deceptive plants (e.g., dimethyldisulfide), with one exception. Oelschlägel et al. (2015) mainly identified aliphatic hydrocarbons and esters in the Mediterranean A. rotunda. More detailed physiological and behavioral analyses with the pollinators of this species rejected brood-site deception and discovered a novel pollination strategy in plants, called kleptomyiophily (Oelschlägel et al., 2015). A. rotunda deceives its chloropid pollinators by mimicking alarm pheromones of preyed-upon mirid bugs, which are a food source of these kleptoparasitic adult flies (Oelschlägel et al., 2015).
Most of the approximately 500 Aristolochia species are native to tropical and subtropical regions, but about 50 species occur in the Mediterranean and adjacent Near East (Nardi, 1984, 1991; Neinhuis et al., 2005; Wanke et al., 2006). Among those, Aristolochia microstoma BOISS. & SPRUNER, a species endemic to Greece, stands out due to its unique perianth morphology and flower presentation (Wanke, 2006). The limb of the small, purplish-brownish flowers (Figures 1A–C) is reduced to a small beak or missing, and the entrance into the floral tube is reduced to a small pore, responsible for the name of the species (Nardi, 1991). While most Aristolochia species display their often showy flowers above the ground, A. microstoma flowers are presented close to or partly buried in the ground, among leaf litter (Figures 1A,B) or between rocks (Figures 1C,D), often hidden from above (Nardi, 1991; Wanke, 2006). Another unusual feature is the more or less horizontal orientation of the floral tube, which is vertical in other species. Pollinators were hypothesized to be small arthropods living near the ground or in leaf litter (Nardi, 1991; Wanke, 2006). So far, the flower visitors and pollinators, the reproductive biology, and the floral scent of A. microstoma remained unknown.
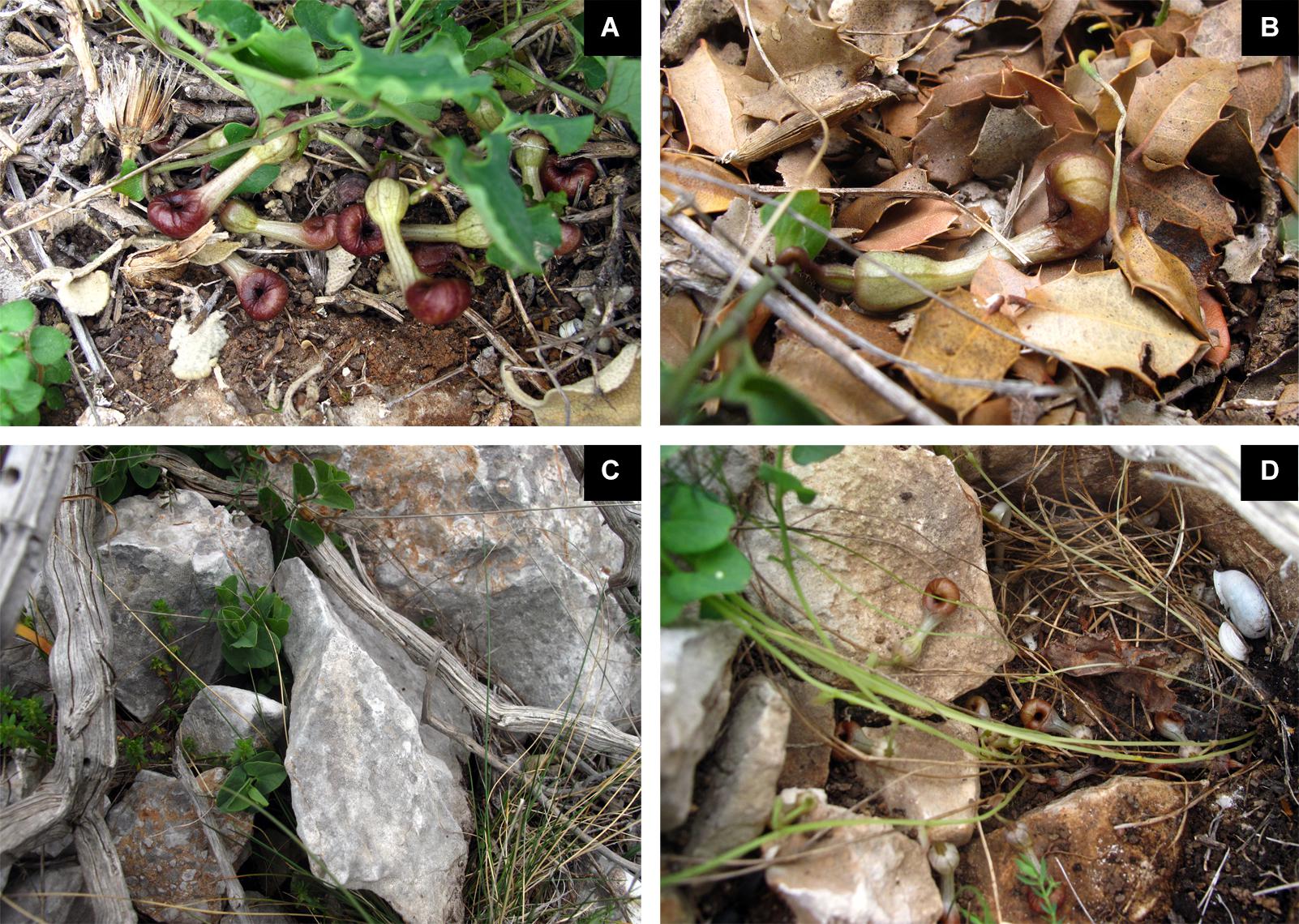
Figure 1. Aristolochia microstoma and its flowers in their natural habitat in Greece (Athens, Mt. Egaleo): The small flowers (length = 2 cm to 3 cm) are presented on ground-level, either well hidden in the leaf litter (A,B), or between rocks (C), where they are not visible from above (D; rock removed to see the flowers).
In this study, we recorded flower visitors and pollinators, and analyzed floral scents in three natural populations of A. microstoma. Specifically, we asked: (1) Are the flowers, as in congeners, also pollinated by flies, or by other ground-dwelling arthropods, and is the pollinator spectrum similar among populations? (2) Do the flowers produce scent, and if so, what is the composition, and is it similar among populations? Based on the obtained data, we discuss possible deceptive strategies of this unusual flower.
Materials and Methods
Study Sites
Aristolochia microstoma is endemic to Central Greece and Northern Peloponnesus, where it colonizes dry, stony, calcareous places in open woodlands, garrigue, and macchia (Nardi, 1991). Samples were collected during field trips in March 2019 and 2020, around the peak of flowering, in “Egaleo” (Athens, Mt. Egaleo, 37.999377N, 23.641652E, 225 m a.s.l.), “Arachneo” (surroundings of Arachneo, near Moní Panagías Talantíou, 37.6714204N, 22.9114465E, 420 m a.s.l.), and “Methana” (Methana peninsula, south of Kypseli, 37.6002630N, 23.4050652E, 65 m a.s.l.). Voucher specimens of the plants are deposited at Herbarium Dresdense (DR).
Flower Visitor and Pollinator Collection and Identification
A total of 1,457 flowers (1,044 female phase, 413 male phase) were randomly collected at the three study sites. As a rhizome may or may not produce several shoots (Nardi, 1991), it was difficult to identify a plant individual. Thus, we recorded the visitors at the level of populations. The flowers were opened and checked for trapped arthropods in the field or stored in 80% isopropanol for later processing in the lab. For each flower, we recorded the flowering phase (female or male), and the number of trapped arthropods with and without pollen. Applying the most conservative approach, only arthropods collected from female phase flowers that carried Aristolochia pollen were treated as pollinators (Rulik et al., 2008; Oelschlägel et al., 2015). The inaperturate exine is characteristic of Aristolochia-pollen (unpublished data), and since no other Aristolochia species were co-flowering at the study sites, we assumed that all Aristolochia pollen belonged to A. microstoma. Collected arthropods were conserved in 80% isopropanol and identified to the order level. Diptera were further identified to family level. The sex of visitors was determined in the two main visitor families, i.e., Phoridae and Sciaridae (see section “Results”). Morphological determinations were performed mainly with the help of Disney (1994) and Oosterbroek (2006). Voucher specimens of the collected arthropods are deposited at the Department of Biosciences, University of Salzburg.
Molecular Analyses of Pollinators
In addition to the identification based on morphological characters, pollinators were characterized by molecular data as well. DNA was extracted from all specimens carrying pollen in female stage flowers (total 25 individuals). In order to preserve the specimens as intact as possible for subsequent morphological identification only one single hind leg of each isopropanol conserved fly was used for DNA isolation.
Genomic DNA was extracted using the NucleoSpin® Tissue kit (MACHEREY-NAGEL, Düren, Germany) according to the manufacturer’s protocol. The extracted DNA samples were stored at –20°C until use. The quality and quantity of each extracted DNA sample was assessed using InvitrogenTM Qubit 3 Fluorometer (Thermo Fisher Scientific Inc., Waltham, MA, United States).
The barcoding marker COI, a 658 bp fragment of cytochrome oxidase I, was amplified using the two primer pairs COI-Dip-F5 (CWACWAAYCAYAARGATATTGG)/COI-Dip R3 (TNGTRATAAAATTWACDGCNCC) and COI-Dip-F7 (CWAT TATAATTGGDGGDTTYGG)/COI-Dip-R4 (CCAAARAATC ARAATARRTGTTG), respectively (newly designed for this study). The PCR reactions were performed in a total volume of 20 μL containing 5.5 μL ddH2O, 4 μL 1 × GoTaq Flexi buffer, 0.1 μL GoTaq G2 Flexi DNA polymerase (Promega, Fitchburg, MA, United States), 3.2 μL dNTPs (1,25 mM each), 1.2 μL 25mM MgCl2, 1 μL of 10mM F- and R-primer, and 4 μL of 1:10 diluted genomic DNA. Amplification was performed in Biometra T3000 thermocycler (Analytic Jena, Jena, Germany) according to the following protocol: initial denaturation for 30 s at 98°C, 35 cycles of denaturation at 98°C for 10 s, annealing at 60°C for 10 s and extension at 72°C for 60 s, and a final extension step at 72°C for 5 min. Quality of PCR products was assessed by gel electrophoresis employing a 1% agarose gel. 5 μL of each PCR, 1.5 μL Gelstar (Bio-RAD Laboratories Inc., Hercules, CA, United States), 2 μL 6× loading dye was run at 80V and amplicons were visualized under UV light (Biometra BioDoc, Analytic Jena, Jena, Germany).
PCR products were purified employing the NucleoSpin® Gel and PCR Clean-up kit (MACHEREY-NAGEL, Düren, Germany). The manufacturers protocol was followed. Samples were diluted in 30 μL elution buffer and directly sequenced using the Macrogen Europe sequencing service (Amsterdam, Netherlands). Sequence quality and trimming was done by eye accessing the pherograms. Forward and reverse sequences for each PCR product were aligned manually using PhyDE® – Phylogenetic Data Editor version 0.99711. Thus, each COI region for each fly individual was amplified and sequenced in two broadly overlapping parts resulting in up to 4x coverage for each nucleotide. Quality controlled sequences were submitted to NCBI nucleotid blast (megablast) as well as BOLD. First 100 hits were checked for query coverage, and percentage identity. Only BLAST search results with at least 90% query coverage and >95% identity were considered.
Floral Scent Sampling
The volatiles emitted by single female phase flowers were collected by dynamic headspace methods (Dötterl et al., 2005) in the field during daytime (11:00–17:30) at the three study sites (Egaleo: n = 7; Arachneo: n = 10; Methana: n = 6). Due to their short fragile stems and hidden position, it was often necessary to cut the flowers for scent sampling. The effect of cutting was found to be minor, as scent collected in situ from still attached flowers (n = 4) yielded the same compounds in comparable ratios (see section “Results,” Table 1 and Figure 2). Therefore, cut and uncut flower samples were pooled for further analyses. Single flowers were inserted into oven bags (10 × 5 cm; Toppits®, Minden, Germany), and scent collection was initiated immediately after bagging. The air containing the volatiles was sucked through an adsorbent tube for 30 min at a flow rate of 200 ml min–1 by a membrane pump (G12/01 EB; Rietschle Thomas Inc., Puchheim, Germany). Adsorbent tubes consisted of a microvial (ChromatoProbe quartz microvials; Varian Inc., Palo Alto, CA, United States: length 15 mm, inner diameter 2 mm) filled with 3 mg of a 1:1 mixture of Tenax-TA (mesh 60–80) and Carbotrap B (mesh 20–40) (both Supelco, Bellefonte, PA, United States) fixed by glass wool plugs (Heiduk et al., 2015). Subsequently to sampling, the flowers were dissected to determine their sexual phase. To unambiguously identify compounds as floral volatiles, control samples of leaves, as well as ambient air, were sampled in a similar way. Samples were stored at 4°C during fieldwork and at –20°C in the laboratory before GC/MS analyses.
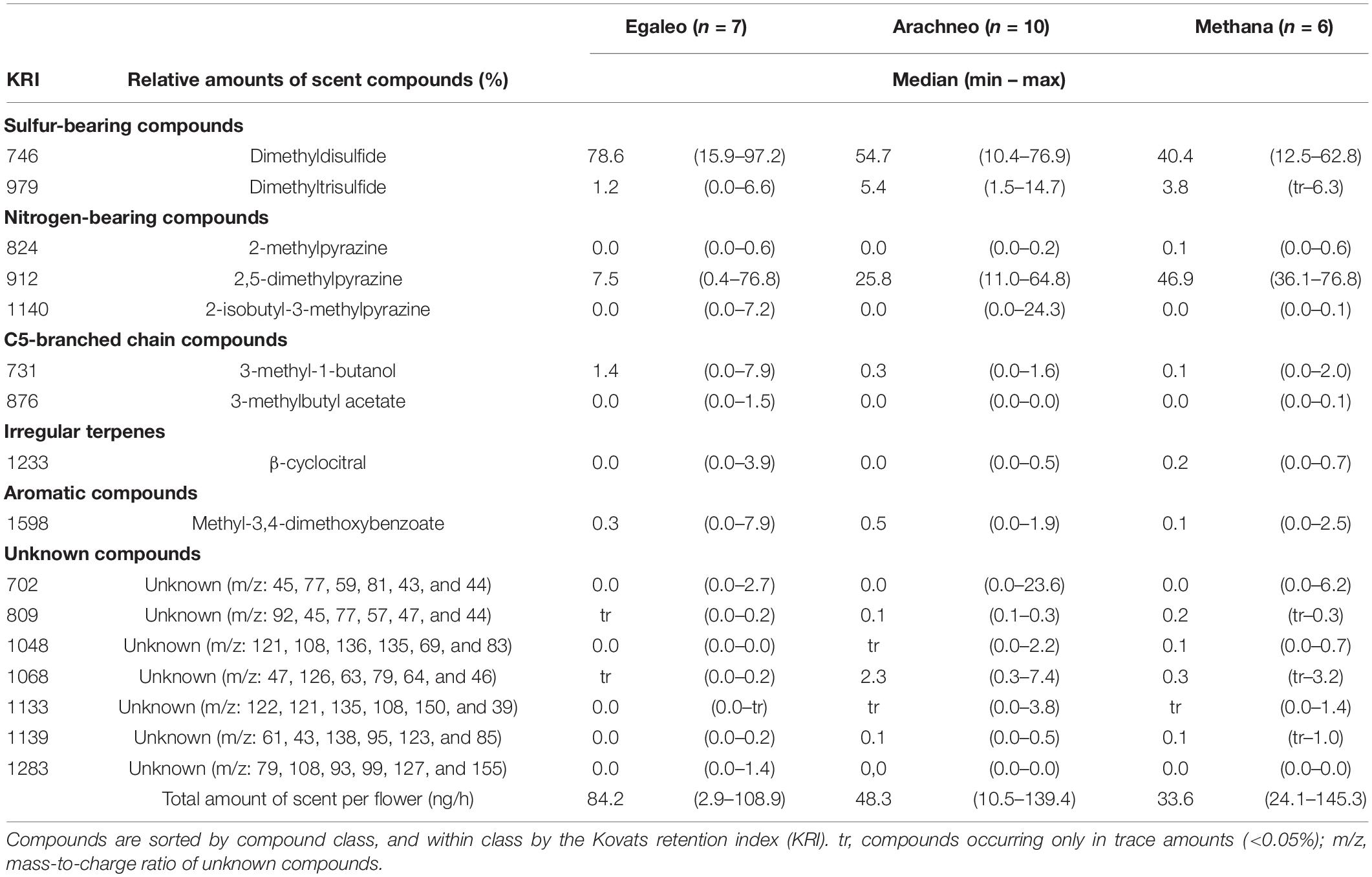
Table 1. Median relative (%) and total absolute (ng/h) amounts of scent (compounds) emitted by single female phase Aristolochia microstoma flowers, collected at three natural sites in Greece: Egaleo, Arachneo, and Methana.
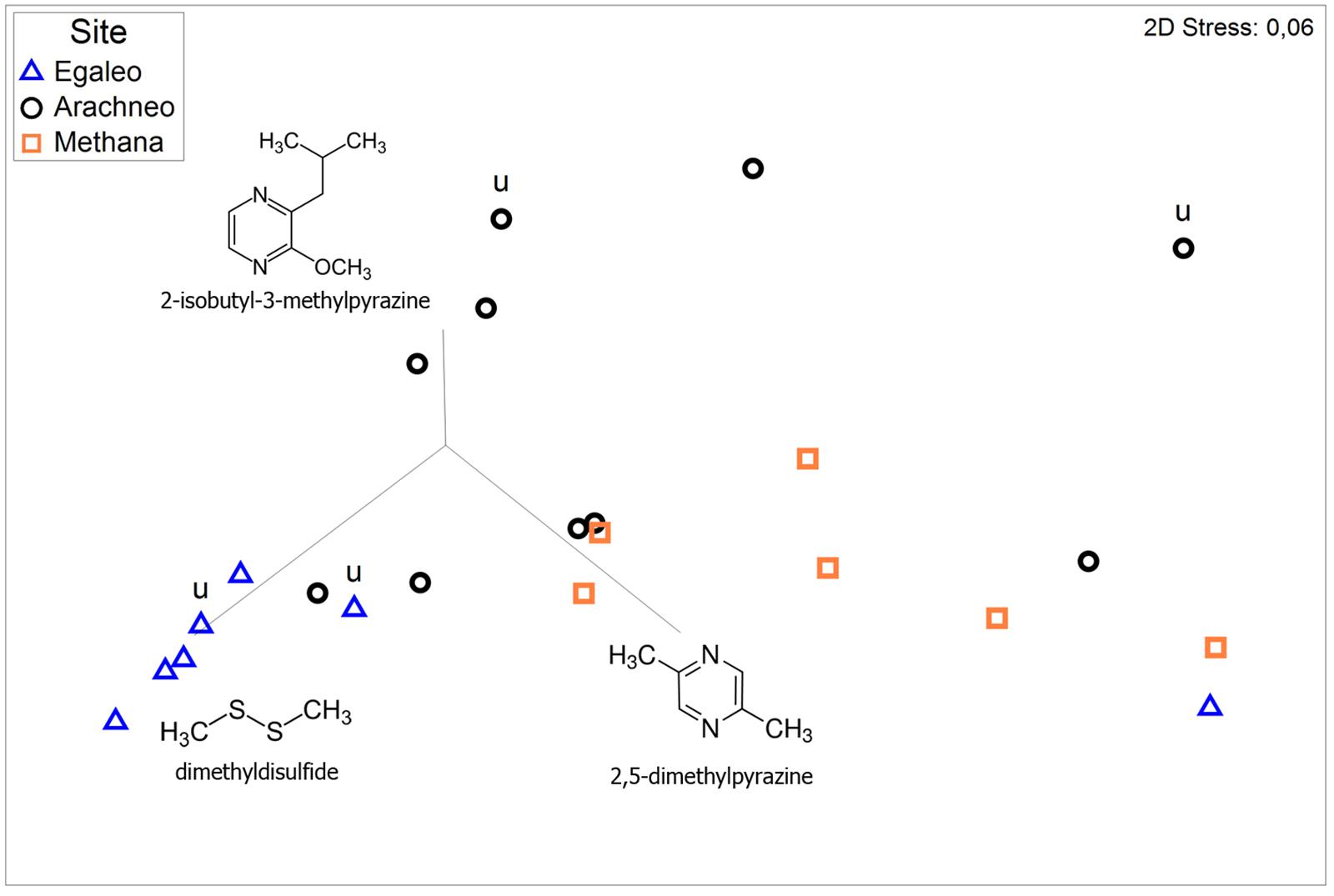
Figure 2. Non-metric multidimensional scaling (NMDS) to visualize semi-quantitative dissimilarities among individual floral scent samples from three Aristolochia microstoma populations in Greece: Egaleo (n = 7); Arachneo (n = 10); and Methana (n = 6). The ordination is based on pairwise Bray-Curtis similarities. The vectors depict the volatiles most correlating with the axes. “u” marks samples collected from uncut flowers, all other samples were collected from cut flowers.
Gas Chromatography Coupled to Mass Spectrometry (GC/MS)
The adsorbent tubes containing the trapped volatiles were analyzed by GC/MS on an automatic thermal desorption (TD) system (TD-20, Shimadzu, Japan) coupled to a Shimadzu GC/MS-QP2010 Ultra equipped with a ZB-5 fused silica column (5% phenyl polysiloxane; length = 60 m, inner diameter = 0.25 mm, film thickness = 0.25 μm, Phenomenex), as described by Heiduk et al. (2015). At a consistent helium carrier gas flow of 1.5 ml/min, the samples were processed at a split ratio of 1:1. The GC oven temperature started at 40°C, then increased by 6°C/min to 250°C and was held for 1 min. The MS interface worked at 250°C. Mass spectra were taken at 70 eV (EI mode) from m/z 30 to 350. GC/MS data were analyzed using the GCMSolution package, Version 4.41 (Shimadzu Corporation 1999-2015). Compounds were tentatively identified by comparison of Kovats retention indices (KRI, based on a series of n-alkanes) and mass spectra to data available in the databases ADAMS, ESSENTIALOILS-23P, FFNSC 2, and W9N11. All compound identities were confirmed by authentic reference standards available at the Plant Ecology lab of the University of Salzburg. Compounds also detected in leaf and ambient air controls were excluded from the analyses. Total scent emission was estimated by injecting known amounts of alkane standards.
Statistical Analyses
We used chi-square tests to compare sex-ratios in Phoridae and Sciaridae among populations. Similarities and dissimilarities in scent bouquets among the samples were visualized by non-metric multidimensional scaling (NMDS), based on pairwise Bray-Curtis dissimilarities calculated on the relative amounts of compounds. We performed analyses of similarities (ANOSIM; 10,000 permutations) to test for differences in floral scent among study sites, and PERMDISP (Anderson et al., 2008) to test for differences in dispersion among populations (10,000 permutations). All multivariate statistical analyses were performed with the software PRIMER 6.1.0.5 (Clarke and Gorley, 2006).
Results
Flower Visitors and Pollinators
The 248 flower visitors recorded in this study (Table 2) originated from 11 to 17% of investigated female-phase and 13–20% of male-phase flowers, respectively, depending on the study site. The majority of flowers had no visitors. Females and males of the dipteran families Phoridae (99 individuals in total) and Sciaridae (52) were the most abundant flower visitors at all study sites (Table 2 and Figure 3). In flower-visiting Phoridae, sex ratios differed between sites (chi-square2 = 10.3; P = 0.006). They were female-biased at Arachneo and Methana and balanced at Egaleo. In contrast, Sciaridae were male-biased at all sites (chi-square2 = 2.65; P = 0.265). Further visitors included other Diptera (Sphaeroceridae, Drosophilidae), and a range of other arthropods, such as Collembola, Acari, Myriapoda, Isopoda, and Coleoptera. The only visitors that carried pollen in female- and/or male-phase flowers were Diptera. In all flies, pollen was attached dorsally to the thorax, mainly around the wing-base, only occasionally on the front and central parts (see Figure 3B). Only Phoridae (25 individuals) carried pollen in female-phase flowers (Figures 3A,B), and thus classified as the exclusive pollinators at all three study sites (Table 2). At the sites Egaleo and Arachneo, Phoridae of both sexes were recorded as pollinators, at Methana only females. Additional pollen-carrying Phoridae of both sexes (10 females and 4 males in total) were found in male-phase flowers at each site. Morphological identification showed that all but one pollinator belonged to the genus Megaselia, mostly the Megaselia angusta/longicostalis complex, and Megaselia scalaris (LOEW, 1866). The BLAST hits on NCBI and BOLD confirmed the presence of species of the Megaselia angusta/longicostalis complex [three individuals, each up to >99.7% identity with GenBank accessions of Megaselia longicostalis (WOOD, 1912)], of M. scalaris (one individual; 99.7% identity to GenBank accession HM399356), and also suggests the presence of Conicera similis (HALIDAY, 1833) (one individual) as pollinator (95 to 97% identity with GenBank accessions). COI sequences of the pollinators are provided as a Supplementary Data Sheet 1.
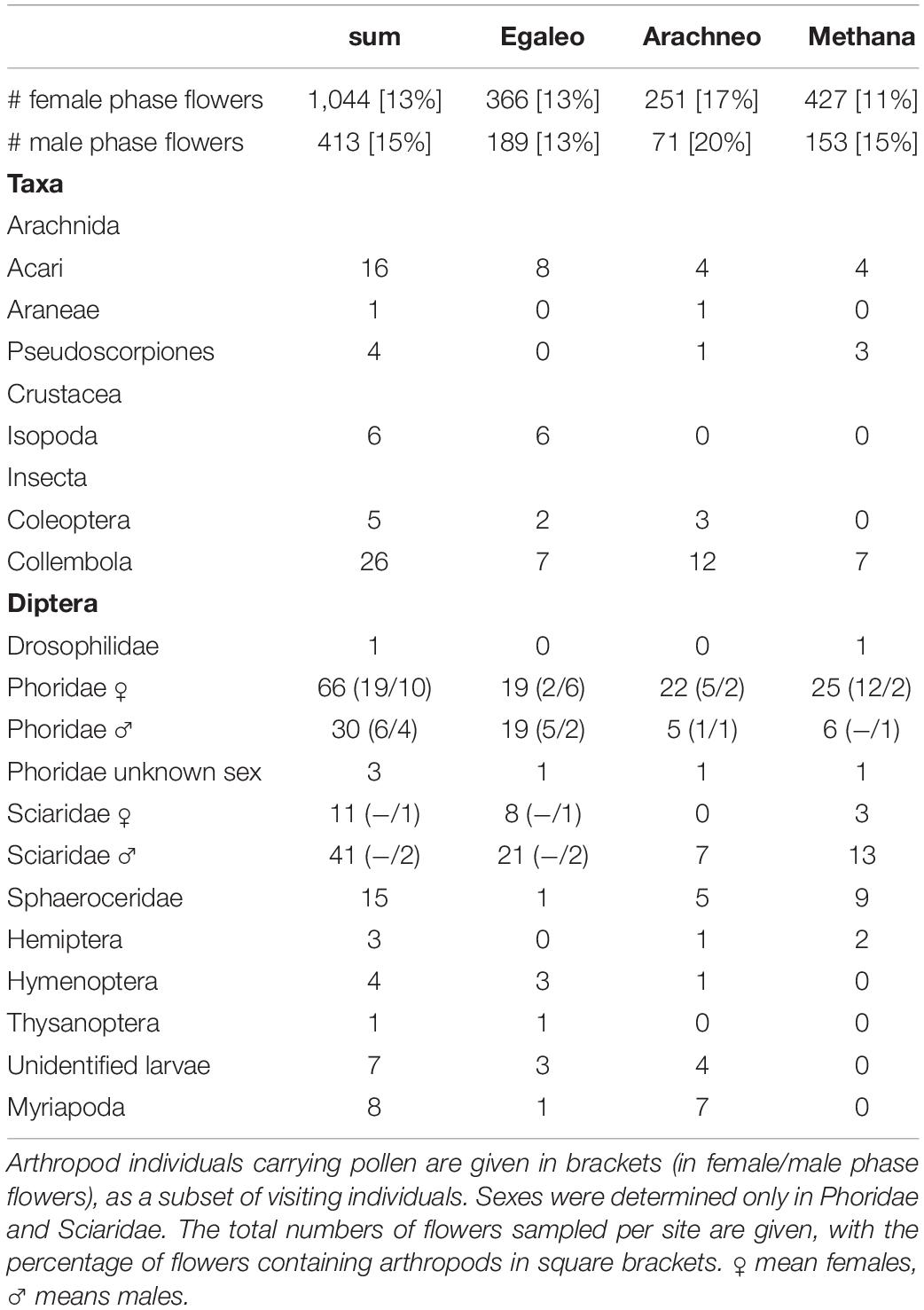
Table 2. Flower visitors of Aristolochia microstoma collected at the three study sites in Greece (Egaleo, Arachneo, and Methana), shown overall (sum) and per site.
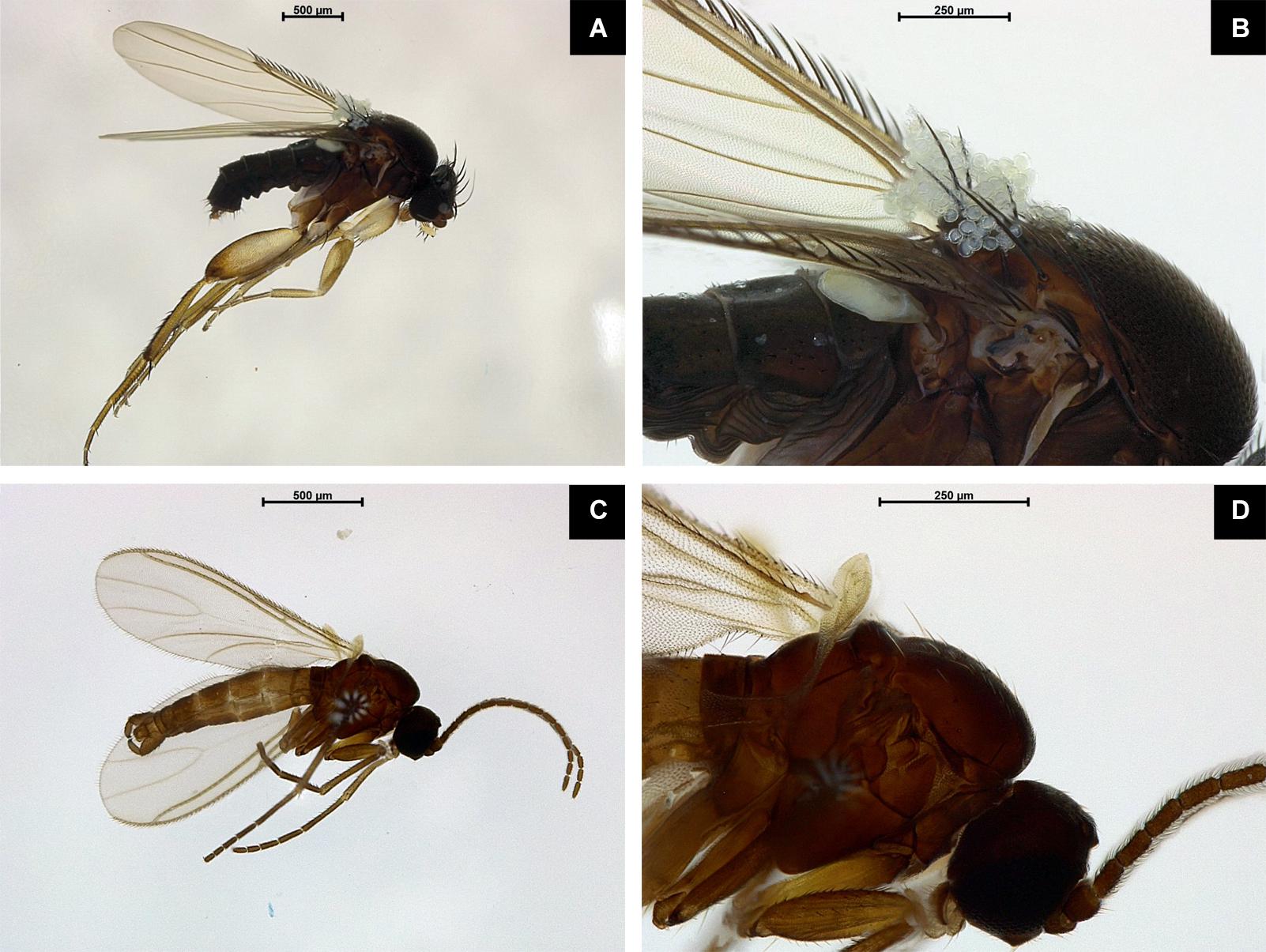
Figure 3. Two specimens of the two most frequent Diptera families visiting flowers of Aristolochia microstoma: (A) a pollinating female Megaselia sp. (Phoridae) carrying pollen on its thorax (B); and (C) a male of an unidentified species of Sciaridae not carrying pollen (D).
Although frequent visitors, Sciaridae never carried pollen in female phase flowers (Figures 3C,D), however, pollen grains were found on three individuals collected from male phase flowers at Egaleo.
Floral Scent
Aristolochia microstoma flowers emitted an unpleasant, carrion-like scent, which was typically well noticeable by the human nose from a few centimeters distance to the flowers. The total scent emission per flower varied considerably among flowers (2.9–145.3 ng/h), with a median between 34 and 84 ng/h, depending on the study site. A total of 16 compounds was found (Table 1), including nitrogen-bearing (3 compounds), sulfur-bearing (2) and C5-branched chain compounds (2), one aromatic compound, one irregular terpene, and seven unknown substances. The main compounds were dimethyldisulfide, with a median relative amount between 40 and 79%, and 2,5-dimethylpyrazine (8–47%), followed by dimethyltrisulfide (1–5%). Those three compounds were present in all samples, except dimethyltrisulfide, which was not detected in one sample. All further compounds, such as 3-methyl-1-butanol and methyl-3,4-dimethoxybenzoate, were minor. Interestingly, the nitrogen-bearing compound 2-isobutyl-3-methylpyrazine, as well as an unknown compound, were particularly strong in some flowers (both up to 24%), although absent in the majority of samples. The relative amount of compounds differed among sites (Figure 2) (ANOSIM: R = 0.826; P = 0.004) and cannot be explained by differences in dispersion among populations (PERMDISP: F2,20 = 0.443; P = 0.830). While there were no significant differences between the sites Arachneo and Methana, the site Egaleo differed from both other sites (ANOSIM: R > 0.265; P < 0.019), due to a higher relative amount of dimethyldisulfide (see Figure 2).
Discussion
Flower Visitors and Pollinators
Aristolochia microstoma was mainly visited by the dipteran families Phoridae and Sciaridae, and less frequently by Sphaeroceridae and Drosophilidae. Further flower visitors included a range of other arthropods, most frequently members of Collembola, Acari, Myriapoda, Isopoda, and Coleoptera. Among flower visitors, Phoridae were the exclusive pollinators at all study sites. The carrion-like floral scent comprised 16 compounds, and was dominated by the oligosulphides dimethyldisulfide and dimethyltrisulfide, and the nitrogen-bearing compound 2,5-dimethylpyrazine. Absolute and relative amounts of the main compounds were variable, and flowers from the site Egaleo differed in scent patterns from Arachneo and Methana.
Our findings show that A. microstoma is not pollinated by non-dipteran ground- or litter-dwelling arthropods, as Wanke (2006) hypothesized, but by flies, as all other Aristolochia species studied so far (Berjano et al., 2009). To which extent the pollinating phorid flies are ground-associated could not be determined. Of the 25 pollinating phorid flies, 24 belong to the megadiverse genus Megaselia, and the remaining individual to the genus Conicera (C. similis), but determination to species level remained difficult. While several COI sequences of the pollinating Megaselia specimens showed high accordances to GenBank accessions of the M. angusta/longicostalis complex, as well as M. scalaris, others did not match any identified accessions. Unfortunately, most individuals of Megaselia in BOLD and Genbank are identified to genus level only (if at all). This is due to the difficult identification and the large number of species in the genus Megaselia, with the majority of species still undescribed or known from one sex only (Disney, 1994). Therefore, the species mentioned here have to be viewed as provisional, and demand further investigations (ongoing research).
Phoridae are well-documented pollinators and flower visitors in Aristolochia. Numerous species in this genus are preferentially or exclusively pollinated by members of this family, including tropical and Mediterranean species, some of them with male or female sex bias (Hime and Costa, 1985; Hall and Brown, 1993; Bänziger and Disney, 2006; Rulik et al., 2008; Berjano et al., 2009; Hipólito et al., 2012; Martin et al., 2017). In our study, the observed sex-ratio in Phoridae could be the result of differing abundances of sexes in the respective fly populations during the collection period. However, a balanced sex-ratio in flower-visiting Phoridae was only found at the site Egaleo, where the floral scent bouquet differed significantly from the other two sites, in which the flower visitors of this family were female-biased. Therefore, it might be possible that these differences in scent lead to sex-biased attractiveness in phorid visitors. In contrast, the flower-visiting Sciaridae were male-biased at all sites, suggesting that the observed differences in floral volatiles did not affect sex-specific attractiveness in this family. Although Sciaridae, and to a lesser extent Sphaeroceridae, were frequently found in the flowers of A. microstoma, they were not classified as pollinators. The occurrence of significant numbers of non-pollinating Diptera families is not unusual in Aristolochia, since several species attract and trap different Diptera, with only a subset of taxa actually pollinating them (e.g., Cammerloher, 1933; Brantjes, 1980; Hilje, 1984; Burgess et al., 2004; Berjano et al., 2009). The spectrum of flower visitors of A. microstoma is remarkably similar to that of A. pallida (Rulik et al., 2008), another Mediteranean species. Apart from the pollinating male Phoridae (Megaselia longicostalis, M. pumila, M. superciliata), flowers of A. pallida are visited – but not pollinated – predominantly by Sciaridae of both sexes, and occasionally by other visitors also found in A. microstoma flowers, including Sphaeroceridae, Acari, Coleoptera, and Collembola (Rulik et al., 2008; Disney and Rulik, 2012). Preliminary morphometric measures of the narrowest part of the floral tube, and the distance between gynostemium and utricle wall of A. microstoma suggest that they are similar to those of A. pallida (mean = 1.37 mm and 1.68 mm, respectively; see Rulik et al., 2008). These two major morphological floral filters in Aristolochia assure that only visitors sharing a specific body size range – small enough to enter the flower, but big enough to physically interact with the gynostemium – can act as pollinators (Brantjes, 1980; Rulik et al., 2008).
In addition to body size, differences in thoracic bristles could contribute to pollinator specialization, as suggested by Cammerloher (1933). As in other Aristolochia species (Bänziger and Disney, 2006; Rulik et al., 2008; Oelschlägel et al., 2015), A. microstoma pollen was generally deposited dorsally on the thorax. The majority of the pollen was concentrated around the wing base, where the pollinating Phoridae are covered by pronounced, stiff bristles (Figure 3B) that probably facilitate pollen adherence. On the front and central parts of the thorax, where bristles are usually very short, pollen grains were hardly found. The lack of such bristles (Figure 3D) might exclude Sciaridae as pollinators of A. microstoma, or at least make them less efficient, as three pollen-carrying specimens collected from male flowers indicate. The less frequent dipteran flower visitors of the families Sphaeroceridae and Drosophilidae, which possess thoracic bristles similar to those of Phoridae, were never found with attached pollen. Whether this was due to their low abundance in our samples, or other reasons, i.e., different body size or non-recurrent visitation of flowers, remains unanswered. Anyhow, the importance of thoracic bristles for pollination of Aristolochia should be experimentally tested in the future. Non-dipteran arthropods were most likely accidental flower visitors, as reported in other Aristolochia species (Cammerloher, 1923, 1933; Trujillo and Séric, 2006; Rulik et al., 2008). Generally, the number of flowers containing visitors was strikingly low across all sites, which could be the result of low pollinator availability, of a low attractiveness of the floral signals, or of a small proportion of attracted animals that entered the flowers through the small pore. This pore might have evolved as a morphological filter, i.e., to limit the number of ground-dwelling animals not appropriate as pollinators, that accidentally fall or crawl into the flower, potentially blocking the flower’s reproductive organs.
Floral Scent and Possible Deceptive Strategies
The floral scent of A. microstoma was strongly dominated by oligosulphides, which are widespread among plants pollinated by carrion-flies and bats, and alkylpyrazines, which are rare floral volatiles (Knudsen et al., 2006). Especially the high amounts of oligosulphides (dimethyldisulfide and dimethyltrisulfide), suggest a sapromyiophilous pollination strategy, as those compounds are the two most common and characteristic volatiles in carrion and carnivorous dung-mimicking flowers, across several plant families (Jürgens et al., 2006, 2013). In contrast, the second main compound of A. microstoma, the alkylpyrazine 2,5-dimethylpyrazine, was rarely found in saprophilous flowers. In lower relative amounts than in the present study, it is emitted by the sapromyiophilous South African stapeliads Orbea variegata (11%) and Stapelia leendertzia (1%), which also emit high amounts of dimethyldisulfide and dimethyltrisulfide, among other compounds, most prominently indole (Johnson and Jürgens, 2010; Jürgens et al., 2013). Both stapeliads, however, were observed to be visited by flies of the families Calliphoridae and Sarcophagidae, and not by Phoridae (Meve and Liede, 1994; Johnson and Jürgens, 2010). Other pyrazines (3-isopentyl 2,5-dimethylpyrazine and 2,6-dimethyl-3-(2-methyl-1-butyl)-pyrazine) are the main compounds in another stapeliad, Echidnopsis montana, the biological function of which remains unclear (Jürgens et al., 2006). Sapromyiophily was proposed for several Aristolochia species (Cammerloher, 1923, 1933; Vogel, 1978; Johnson and Jürgens, 2010), but chemical analyses of floral scent remain scarce, limiting comparisons within the genus. Nevertheless, A. microstoma shares dimethyldisulfide and dimethyltrisulfide with the sapromyiophilous A. cymbifera, which, in cultivation, attracts carrion flies (Johnson and Jürgens, 2010). However, the scent of this species is overall dominated by benzenoids. Dimethyldisulfide is also found in smaller amounts in the neotropical phorid-pollinated A. gigantea, the odor of which is dominated by sweet lemon-scented citronella-like compounds (Martin et al., 2017). Compared to those and other Aristolochia species, which comprise between 63 and 168 floral scent compounds (Johnson and Jürgens, 2010; Oelschlägel et al., 2015; Martin et al., 2017), the odor of A. microstoma with only 16 compounds is strikingly less complex. Although the main floral scent compounds of A. microstoma were present throughout all samples, their absolute amounts were variable among individuals, and their relative amounts at the sites Arachnea and Methana differed from the site Egaleo. Such intraspecific variation in floral scent is a widespread phenomenon in both deceptive and rewarding plant species, and can be caused by multiple factors, such as local adaptation and genetic drift (Delle-Vedove et al., 2017). In dichogamous plants or plants with unisexual flowers, floral scents sometimes vary between the sexual phases/flower sexes. Preliminary data of A. microstoma, however, suggest that the scent of male-phase flowers is similar in both total amount and composition to that of female-phase flowers (Supplementary Table 1), and thus might also attract insects, likely to increase pollen export. In A. gigantea, the only Aristolochia species with such data available, the scent emission is strongly reduced in the male compared to the female phase, with strong differences in composition between the sexual phases (Martin et al., 2017).
Dimethyldisulfide and dimethyltrisulfide are common volatiles in degrading meat (carcasses and carnivore/omnivore feces), that, however, do not emit 2,5-dimethylpyrazine (Jürgens et al., 2006, 2013). Instead, 2,5-dimethylpyrazine was found in the scent of dead bark beetles (Ips typographus), alongside dimethyldisulfide, 3-methyl-1-butanol, and other compounds (Zhang et al., 2003). Future studies have to show whether those compounds are also released from other invertebrate carrion, e.g., other arthropods, and why 2,5-dimethylpyrazine is obviously not released by decomposing vertebrate carrion (Stutz et al., 1991; Johnson and Jürgens, 2010; Jürgens et al., 2013).
Various pyrazines are important volatiles in animal pheromones, such as urinal pheromones in mammals like mice, voles, and hamsters (Novotny et al., 1986; Boyer et al., 1989; Soini et al., 2005), and sex pheromones of fruit flies (Robacker et al., 2009) and thynnine wasps, the latter being exploited by the sexually deceptive orchid Drakaea glyptodon (Bohman et al., 2014). Best known, however, is the role of alkylpyrazines as key volatiles in alarm- and trail pheromones in several genera of ants, including 2,5-dimethylpyrazine (Attygalle and Morgan, 1984; Jackson et al., 1990; Morgan et al., 1992; Hölldobler et al., 2001). Pyrazines are key volatiles in host-localization in specialized myrmecophilous Phoridae (Pseudacteon spp.), so called ant-decapitating flies (Sharma et al., 2011; Sharma and Fadamiro, 2013; Ngumbi and Fadamiro, 2014). However, to the best of our knowledge, dimethyldisulfide and dimethyltrisulfide were never reported in context with ant pheromones, and no typical ant-associated (myrmecophilous) phorid genera were found among the pollinators of A. microstoma. Although there are also cases of myrmecophilous behavior found in Megaselia (Disney, 1994), often described as “one of the largest, most biologically diverse and taxonomically difficult genera in the entire animal kingdom” (Marshall, 2012), the pollinators recorded in the present study are probably unspecifically saprophagous. Larvae and adults of Conicera similis and members of the M. angusta/longicostalis complex (i.e., M. longicostalis) are known to feed on vertebrate (e.g., rabbit) and invertebrate (snail) carrion, decomposing plants, but also fungi (Disney, 1994, 1999; Buck, 1997, 2001). The cosmopolitan M. scalaris even utilizes the broadest spectrum of larval substrates known in all insects, including numerous dead and living animals, fungi and plants (reviewed in Disney, 2008). Larvae of Sciaridae, which were frequent flower visitors but not pollinators in A. microstoma, are usually feeding on living or decomposing plants and fungi, as well as on herbivore excrements, and are frequently found among detritus and forest litter (Menzel and Mohrig, 2000).
Conclusion and Outlook
The spatial position of A. microstoma flowers suggests that the pollinating Phoridae probably search for breeding sites or food close to the ground, in leaf litter, or between rocks. This hidden presentation of the flowers also points toward scent as the attractive cue to lure the pollinators. Our data on pollinators and floral scent indicate that A. microstoma deceives its phorid pollinators by employing a sapromyiophilous strategy, as proposed for other Aristolochia species. The co-occurrence of high amounts of oligosulphides and 2,5-dimethylpyrazine is novel among plants and suggests a so far undescribed type of sapromyiophilous mimicry. Due to the high similarity to carrion scents of dead beetles, and the absence of either 2,5-dimethylpyrazine or dimethyldisulfide in vertebrate carcasses and carnivorous feces, or ant pheromones, we hypothesize that brood-site mimicry of invertebrate carrion is the most likely deceptive strategy. Studies testing the attractiveness of the scent compounds of A. microstoma flowers and different potential substrates to the pollinators are currently carried out to test this hypothesis.
Data Availability Statement
All data used for the study are presented in the manuscript.
Author Contributions
BO, CN, SW, and SD designed and planned the study. TR, SW, and BO conducted the field work and collected the samples. TR, KR, BO, and HM processed the flower visitors. KR and TR identified the arthropods to order/family level. HM, BO, TW, and SW performed the molecular lab work and characterization of phorid flies. RD morphologically identified phorids flies. TR performed and SD supported the chemical and statistical analyses. TR drafted the manuscript. All authors contributed to the final manuscript and approved the submitted version.
Funding
This research has been funded by the Austrian Science Fund (FWF, I 3722-B29) and the German Research Foundation (DFG, WA 2461/9-1). RD’s studies of Phoridae are currently funded by the Balfour-Browne Trust (University of Cambridge).
Conflict of Interest
The authors declare that the research was conducted in the absence of any commercial or financial relationships that could be construed as a potential conflict of interest.
Acknowledgments
The collection permit was issued by the Greek General Directorate for Forest and Agriculture (66435/811). We are grateful to Ioanna Oikonomou (Athens) for assistance in field work and for her hospitality, Bernardo Cañiza (TU Dresden) for help in searching for flower visitors, Roman Fuchs (University of Salzburg) for lab support, and Enio Nardi (Florence) for valuable information on finding suitable A. microstoma sites. We thank Herbert Braunschmid, Eva Gfrerer, Karin Gross, and Danae Laina (all University of Salzburg) for valuable comments on an earlier version of the manuscript.
Supplementary Material
The Supplementary Material for this article can be found online at: https://www.frontiersin.org/articles/10.3389/fevo.2021.658441/full#supplementary-material
Supplementary Table 1 | It contains the floral scent dataset.
Supplementary Data Sheet 1 | It contains the COI sequence data of the pollinators.
Footnotes
References
Anderson, M. J., Gorley, R. N., and Clarke, K. R. (2008). PERMANOVA+ for PRIMER: Guide to Software and Statistical Methods. Plymouth: PRIMER-E.
Attygalle, A. B., and Morgan, E. D. (1984). Identification of trail pheromone of the ant Tetramorium caespitum L. (Hymenoptera: Myrmicinae). J. Chem. Ecol. 10, 1453–1468. doi: 10.1007/BF00990315
Bänziger, H., and Disney, R. H. L. (2006). Scuttle flies (Diptera: Phoridae) imprisoned by Aristolochia baenzigeri (Aristolochiaceae) in Thailand. Mitt. Schweiz. Entomol. Gesellschaft 79, 29–61.
Berjano, R., Ortiz, P. L., Arista, M., and Talavera, S. (2009). Pollinators, flowering phenology and floral longevity in two Mediterranean Aristolochia species, with a review of flower visitor records for the genus. Plant Biol. 11, 6–16. doi: 10.1111/j.1438-8677.2008.00131.x
Bohman, B., Phillips, R. D., Menz, M. H. M., Berntsson, B. W., Flematti, G. R., Barrow, R. A., et al. (2014). Discovery of pyrazines as pollinator sex pheromones and orchid semiochemicals: implications for the evolution of sexual deception. New Phytol. 203, 939–952. doi: 10.1111/nph.12800
Boyer, M. L., Jemiolo, B., Andreolini, F., Wiesler, D., and Novotny, M. V. (1989). Urinary volatile profiles of pine vole, Microtus pinetorum, and their endocrine dependency. J. Chem. Ecol. 15, 649–662. doi: 10.1007/BF01014708
Brantjes, N. B. M. (1980). Flower morphology of Aristolochia species and the consequences for pollination. Acta Bot. Neerl. 29, 212–213. doi: 10.1111/j.1438-8677.1986.tb00491.x
Brodmann, J., Twele, R., Francke, W., Hölzler, G., Zhang, Q.-H., and Ayasse, M. (2008). Orchids mimic Green-Leaf volatiles to attract prey-hunting wasps for pollination. Curr. Biol. 18, 740–744. doi: 10.1016/j.cub.2008.04.040
Brodmann, J., Twele, R., Francke, W., Yi-Bo, L., Xi-Qiang, S., and Ayasse, M. (2009). Orchid mimics honey bee alarm pheromone in order to attract hornets for pollination. Curr. Biol. 19, 1368–1372. doi: 10.1016/j.cub.2009.06.067
Buck, M. (1997). Untersuchungen zur Ökologischen Einnischung Saprophager Dipteren unter Besonderer Berücksichtigung der Phoridae und Sphaeroceridae (Brachycera/Cyclorrhapha). Ph.D. dissertation. Ulm: Universität Ulm.
Buck, M. (2001). Identification of females of European Conicera Meigen, with a discussion of certain features of the reproductive anatomy of female Phoridae (Diptera). Dtsch. Entomol. Z. 48, 69–81.
Burgess, K. S., Singfield, J., Melendez, V., and Kevan, P. G. (2004). Pollination biology of Aristolochia grandiflora (Aristolochiaceae) in Veracruz, Mexico. Ann. Mo. Bot. Gard. 91, 346–356.
Cammerloher, H. (1923). Zur Biologie der Blüte von Aristolochia grandiflora Swartz. Österr. Bot. Ztg. 72, 180–198. doi: 10.1007/BF01660049
Cammerloher, H. (1933). Die Bestäubungseinrichtungen der Blüten von Aristolochia lindneri Berger. Planta 19, 351–365. doi: 10.1007/BF01920951
Delle-Vedove, R., Schatz, B., and Dufay, M. (2017). Understanding intraspecific variation of floral scent in light of evolutionary ecology. Ann. Bot. 120, 1–20. doi: 10.1093/aob/mcx055
Disney, R. H. L. (1994). Scuttle Flies: The Phoridae. London: Chapman & Hall. doi: 10.1007/978-94-011-1288-8
Disney, R. H. L. (1999). A troublesome sibling species complex of scuttle flies (Diptera: Phoridae) revisited. J. Nat. Hist. 33, 1159–1216. doi: 10.1080/002229399299987
Disney, R. H. L. (2008). Natural history of the scuttle fly, Megaselia scalaris. Annu. Rev. Entomol. 53, 39–60. doi: 10.1146/annurev.ento.53.103106.093415
Disney, R. H. L., and Rulik, B. (2012). Scuttle flies (Diptera: Phoridae) trapped by flowers of Aristolochia L. (Aristolochiaceae) in Italy. Entomol. Mon. Mag. 148, 234–236.
Dötterl, S., Wolfe, L. M., and Jürgens, A. (2005). Qualitative and quantitative analyses of flower scent in Silene latifolia. Phytochemistry 66, 203–213. doi: 10.1016/j.phytochem.2004.12.002
Hall, D. W., and Brown, B. V. (1993). Pollination of Aristolochia littoralis (Aristolochiales: Aristolochiaceae) by males of Megaselia spp. (Diptera: Phoridae). Ann. Entomol. Soc. Am. 86, 609–613.
Heiduk, A., Brake, I., von Tschirnhaus, M., Göhl, M., Jürgens, A., Johnson, S. D., et al. (2016). Ceropegia sandersonii mimics attacked honeybees to attract kleptoparasitic flies for pollination. Curr. Biol. 26, 2787–2793. doi: 10.1016/j.cub.2016.07.085
Heiduk, A., Kong, H., Brake, I., Von Tschirnhaus, M., Tolasch, T., Tröger, A. G., et al. (2015). Deceptive Ceropegia dolichophylla fools its kleptoparasitic fly pollinators with exceptional floral scent. Front. Ecol. Evol. 3:66. doi: 10.3389/fevo.2015.00066
Hilje, L. (1984). Fenología y ecología floral de Aristolochia grandiflora Swartz (Aristolochiaceae) en Costa Rica. Brenesia 22, 1–44.
Hime, N. C., and Costa, E. L. (1985). Sobre Megaselia (M.) aristolochiae n. sp. (Diptera, Phoridae) cujas larvas se criam nas flores de Aristolochia labiata willd. (Aristolochiaceae). Rev. Bras. Biol. 45, 621–625.
Hipólito, J., Viana, B. F., Selbach-Schnadelbach, A., Galetto, L., and Kevan, P. G. (2012). Pollination biology and genetic variability of a giant perfumed flower (Aristolochia gigantea Mart. and Zucc., Aristolochiaceae) visited mainly by small Diptera. Botany 90, 815–829. doi: 10.1139/B2012-047
Hölldobler, B., Morgan, E. D., Oldham, N. J., and Liebig, J. (2001). Recruitment pheromone in the harvester ant genus Pogonomyrmex. J. Insect Physiol. 47, 369–374. doi: 10.1016/S0022-1910(00)00143-8
Jackson, B. D., Keegans, S. J., Morgan, E. D., Cammaerts, M. C., and Cammaerts, R. (1990). Trail pheromone of the ant Tetramorium meridionale. Naturwissenschaften 77, 294–296. doi: 10.1007/BF01131231
Johnson, S. D., and Jürgens, A. (2010). Convergent evolution of carrion and faecal scent mimicry in fly-pollinated angiosperm flowers and a stinkhorn fungus. S. Afr. J. Bot. 76, 796–807. doi: 10.1016/j.sajb.2010.07.012
Jürgens, A., Dötterl, S., and Meve, U. (2006). The chemical nature of fetid floral odours in stapeliads (Apocynaceae-Asclepiadoideae-Ceropegieae). New Phytol. 172, 452–468. doi: 10.1111/j.1469-8137.2006.01845.x
Jürgens, A., Wee, S.-L., Shuttleworth, A., and Johnson, S. D. (2013). Chemical mimicry of insect oviposition sites: a global analysis of convergence in angiosperms. Ecol. Lett. 16, 1157–1167. doi: 10.1111/ele.12152
Knudsen, J. T., Eriksson, R., Gershenzon, J., and Ståhl, B. (2006). Diversity and distribution of floral scent. Bot. Rev. 72, 1–120.
Marshall, S. A. (2012). Flies: The Natural History and Diversity of Diptera. Richmond Hill, Ont: Firefly Books.
Martel, C., Cairampoma, L., Stauffer, F. W., and Ayasse, M. (2016). Telipogon peruvianus (Orchidaceae) flowers elicit pre-mating behaviour in Eudejeania (Tachinidae) males for pollination. PLoS One 11:e0165896. doi: 10.1371/journal.pone.0165896
Martin, K. R., Moré, M., Hipólito, J., Charlemagne, S., Schlumpberger, B. O., and Raguso, R. A. (2017). Spatial and temporal variation in volatile composition suggests olfactory division of labor within the trap flowers of Aristolochia gigantea. Flora Morphol. Distrib. Funct. Ecol. Plants 232, 153–168. doi: 10.1016/j.flora.2016.09.005
Menzel, F., and Mohrig, W. (2000). Revision der paläarktischen Trauermücken (Diptera: Sciaridae). Stud. Dipterologica Suppl. 6, 1–761.
Meve, U., and Liede, S. (1994). Floral biology and pollination in stapeliads – new results and a literature review. Plant Syst. Evol. 192, 99–116. doi: 10.1007/BF00985911
Morgan, E. D., Hölldobler, B., Vaisar, T., and Jackson, B. D. (1992). Contents of poison apparatus and their relation to trail-following in the ant Daceton armigerum. J. Chem. Ecol. 18, 2161–2168.
Neinhuis, C., Wanke, S., Hilu, K. W., Müller, K., and Borsch, T. (2005). Phylogeny of Aristolochiaceae based on parsimony, likelihood, and Bayesian analyses of trnL-trnF sequences. Plant Syst. Evol. 250, 7–26. doi: 10.1007/s00606-004-0217-0
Ngumbi, E., and Fadamiro, H. Y. (2014). Comparative responses of four Pseudacteon phorid fly species to host fire ant alarm pheromone and analogs. Chemoecology 25, 85–92. doi: 10.1007/s00049-014-0178-x
Novotny, M., Jemiolo, B., Harvey, S., Wiesler, D., and Marchlewska-Koj, A. (1986). Adrenal-mediated endogenous metabolites inhibit puberty in female mice. Science 231, 722–725. doi: 10.1126/science.3945805
Oelschlägel, B., Gorb, S., Wanke, S., and Neinhuis, C. (2009). Structure and biomechanics of trapping flower trichomes and their role in the pollination biology of Aristolochia plants (Aristolochiaceae). New Phytol. 184, 988–1002.
Oelschlägel, B., Nuss, M., von Tschirnhaus, M., Pätzold, C., Neinhuis, C., Dötterl, S., et al. (2015). The betrayed thief – the extraordinary strategy of Aristolochia rotunda to deceive its pollinators. New Phytol. 206, 324–351. doi: 10.1111/nph.13210
Oosterbroek, P. (2006). The European Families of the Diptera. Utrecht: KNNV Publishing. doi: 10.1163/9789004278066
Proctor, M., Yeo, P., and Lack, A. (1996). The Natural History of Pollination. London: Harper Collins Publishers.
Renner, S. S. (2006). “Rewardless flowers in the angiosperms and the role of insect cognition in their evolution,” in Plant-Pollinator Interactions: From Specialization to Generalization, eds N. M. Waser and J. Olerton (Chicago, IL: University of Chicago Press), 123–144.
Robacker, D. C., Aluja, M., Bartelt, R. J., and Patt, J. (2009). Identification of chemicals emitted by calling males of the Sapote fruit fly, Anastrepha serpentina. J. Chem. Ecol. 35, 601–609. doi: 10.1007/s10886-009-9631-7
Rulik, B., Wanke, S., Nuss, M., and Neinhuis, C. (2008). Pollination of Aristolochia pallida willd. (Aristolochiaceae) in the Mediterranean. Flora 203, 175–184. doi: 10.1016/j.flora.2007.02.006
Schiestl, F. P. (2005). On the success of a swindle: pollination by deception in orchids. Naturwissenschaften 92, 255–264. doi: 10.1007/s00114-005-0636-y
Schiestl, F. P., Peakall, R., Mant, J. G., Ibarra, F., Schulz, C., Franke, S., et al. (2003). The chemistry of sexual deception in an orchid-wasp pollination system. Science 302, 437–438. doi: 10.1126/science.1087835
Sharma, K. R., and Fadamiro, H. Y. (2013). Fire ant alarm pheromone and venom alkaloids act in concert to attract parasitic phorid flies, Pseudacteon spp. J. Insect Physiol. 59, 1119–1124. doi: 10.1016/j.jinsphys.2013.08.010
Sharma, K. R., Vander Meer, R. K., and Fadamiro, H. Y. (2011). Phorid fly, Pseudacteon tricuspis, response to alkylpyrazine analogs of a fire ant, Solenopsis invicta, alarm pheromone. J. Insect Physiol. 57, 939–944. doi: 10.1016/j.jinsphys.2011.04.007
Soini, H. A., Wiesler, D., Apfelbach, R., König, P., Vasilieva, N. Y., and Novotny, M. V. (2005). Comparative investigation of the volatile urinary profiles in different Phodopus hamster species. J. Chem. Ecol. 31, 1125–1143. doi: 10.1007/s10886-005-4252-2
Stashenko, E. E., Ordóñez, S. A., Marín, N. A., and Martínez, J. R. (2009). Determination of the volatile and semi-volatile secondary metabolites, and aristolochic acids in Aristolochia ringens Vahl. J. Chromatogr. Sci. 47, 817–821. doi: 10.1093/chromsci/47.9.817
Stensmyr, M. C., Urru, I., Collu, I., Celander, M., Hansson, B. S., and Angioy, A.-M. (2002). Rotting smell of dead-horse arum florets. Nature 420, 625–626. doi: 10.1038/420625a
Stökl, J., Strutz, A., Dafni, A., Svatos, A., Doubsky, J., Knaden, M., et al. (2010). A deceptive pollination system targeting drosophilids through olfactory mimicry of yeast. Curr. Biol. 20, 1846–1852. doi: 10.1016/j.cub.2010.09.033
Stutz, H. K., Silverman, G. J., Angelini, P., and Levin, R. E. (1991). Bacteria and volatile compounds associated with ground beef spoilage. J. Food Sci. 56, 1147–1153. doi: 10.1111/j.1365-2621.1991.tb04721.x
Trujillo, C. G., and Séric, A. N. (2006). Floral biology of Aristolochia argentina (Aristolochiaceae). Flora 201, 374–382. doi: 10.1016/j.flora.2005.07.013
Urru, I., Stensmyr, M. C., and Hansson, B. S. (2011). Pollination by brood-site deception. Phytochemistry 72, 1655–1666. doi: 10.1016/j.phytochem.2011.02.014
Vogel, S. (1978). Pilzmückenblumen als Pilzmimeten. Erster Teil. Flora 167, 329–366. doi: 10.1016/s0367-2530(17)31124-6
Wanke, S. (2006). Evolution of the Genus Aristolochia – Systematics, Molecular Evolution and Ecology. Ph.D. dissertation. Dresden: Universität Dresden.
Wanke, S., González, F., and Neinhuis, C. (2006). Systematics of Pipevines: combining morphological and fast-evolving molecular characters to investigate the relationships within subfamily Aristolochioideae (Aristolochiaceae). Int. J. Plant Sci. 167, 1215–1227. doi: 10.1086/508024
Wolda, H., and Sabrosky, C. W. (1986). Insect visitors to two forms of Aristolochia pilosa in Las Cumbres, Panama. Biotropica 18, 295–299.
Woodcock, T. S., Larson, B. M. H., Kevan, P. G., Inouye, D. W., and Lunau, K. (2014). Flies and flowers II: floral attractants and rewards. J. Pollinat. Ecol. 12, 63–94. doi: 10.2307/2992015
Keywords: Aristolochiaceae, deceptive pollination, dimethyldisulfide, 2, 5-dimethylpyrazine, floral scent, Phoridae, sapromyiophily, Megaselia
Citation: Rupp T, Oelschlägel B, Rabitsch K, Mahfoud H, Wenke T, Disney RHL, Neinhuis C, Wanke S and Dötterl S (2021) Flowers of Deceptive Aristolochia microstoma Are Pollinated by Phorid Flies and Emit Volatiles Known From Invertebrate Carrion. Front. Ecol. Evol. 9:658441. doi: 10.3389/fevo.2021.658441
Received: 25 January 2021; Accepted: 26 April 2021;
Published: 21 May 2021.
Edited by:
Sara Diana Leonhardt, Technical University of Munich, GermanyReviewed by:
Manfred Ayasse, University of Ulm, GermanyRenate A. Wesselingh, Catholic University of Louvain, Belgium
Copyright © 2021 Rupp, Oelschlägel, Rabitsch, Mahfoud, Wenke, Disney, Neinhuis, Wanke and Dötterl. This is an open-access article distributed under the terms of the Creative Commons Attribution License (CC BY). The use, distribution or reproduction in other forums is permitted, provided the original author(s) and the copyright owner(s) are credited and that the original publication in this journal is cited, in accordance with accepted academic practice. No use, distribution or reproduction is permitted which does not comply with these terms.
*Correspondence: Stefan Wanke, stefan.wanke@tu-dresden.de; Stefan Dötterl, Stefan.Doetterl@sbg.ac.at