- 1Department of Biological Sciences, National University of Singapore, Singapore, Singapore
- 2Nature Society (Singapore), Singapore, Singapore
Increasing urbanization in the tropics has led to the loss of natural habitats and local extirpations and the introduction of non-native plants in urban centers. Non-native plants can have widespread positive and negative ecological implications on native fauna including butterflies. In the small tropical urbanized city-state of Singapore, Aristolochia jackii (Aristolochiaceae), a native host plant of the nationally threatened Common Birdwing (Troides helena) and Common Rose (Pachliopta aristolochiae), is considered extirpated, but their shared non-native host plant Aristolochia acuminata is a cultivated ornamental in urban habitat. We conducted systematic surveys from years 2010 to 2014 and collated sighting records from 1999 to 2019 to map the distribution of T. helena and P. aristolochiae, and their host plant A. acuminata. We utilized machine learning models (i.e., random forest algorithms) to establish the relationships between various habitat (managed and natural tree cover, waterbody and impervious surface cover) and life-history parameters (minimum distance from the nearest larval host plant and population source derived from expert knowledge) that are associated with the butterfly distributions. Response curves were generated for each species and projected spatially across Singapore’s landscape to estimate occupancy. We found that both butterflies had clustered distributions with a greatly reduced probability of occurrence further away from identified population sources and non-native A. acuminata. Both study species had similar spatial niche and similar species occurrence responses though there were differences in habitat preferences and temporal niche. Both species showed positive dependence on managed tree cover (Rose more than Birdwing) but the Birdwing also had high positive dependence on natural tree cover, unlike the Rose. We report novel findings that a non-native host plant can provide positive ecological benefits and critically sustain tropical butterfly populations. While there will be a need to evaluate the full ecological impacts of non-native plantings, we suggest using them as a secondary strategy when re-establishment of the native plants has failed, particularly in highly urbanized tropical landscapes.
Introduction
Most conservation efforts for tropical butterflies are directed toward habitat protection (New et al., 1995; Bonebrake et al., 2010; Scriven et al., 2019), often due to the lack of species-level data sets that are necessary in formulating species-specific conservation strategies. Yet, as habitat loss continues, many tropical butterflies continue to face a precarious future (Jain et al., 2018; Theng et al., 2020). Many rare tropical insects cannot maintain their populations and need intervention for the species to recover their numbers—just maintaining their native habitats or resources is not sufficient (Schultz and Chang, 1998; Schultz and Dlugosch, 1999; Xing et al., 2019).
Lessons from management strategies devised for temperate butterflies are usually of limited relevance as they are specific to a particular habitat type or ecosystem such as a grassland (Crone and Schultz, 2003; Baguette et al., 2011). Population recovery studies in the tropics have rarely looked beyond documenting the loss and recovery of butterflies due to natural cataclysmic events like forest fires and droughts (Cleary and Mooers, 2004; Dunn, 2004). Therefore, there is a need to design and test strategies for tropical butterflies to restore populations for conservation management. Designing such a strategy is complex because a priori knowledge of the species is needed; such as knowledge of species biology, habitat range and dispersal, habitat requirements and an understanding of species survival in potential enrichment locations (Schultz et al., 2008). Species distribution modeling is increasingly being applied to aid such efforts, however, studies tend to lack clear links to ecological interactions that affect the model’s translatability to specific conservation management strategies.
Another dimension to consider is the increasing global urbanization that has not only led to the loss of natural habitats but has introduced a large number of non-native plants with mixed impacts on native fauna (Reichard et al., 2001; McKinney, 2006; Schlaepfer et al., 2011; Trentanovi et al., 2013; van Kleunen et al., 2015; Jain et al., 2016). This phenomenon may increase the reliance of native insects including butterflies on non-native host plants (Graves and Shapiro, 2003) and nectar plants (Jain et al., 2016). With rapid urbanization bringing non-native plants to tropical areas, the reliance of butterflies on non-native host plants can be expected. The positive benefits of non-native plants are seldom discussed and remain poorly understood particularly for the restoration of tropical butterfly populations.
In this study, we evaluated the dependence of two threatened but phylogenetically related butterflies in Singapore (both in family Papilionidae)—Common Birdwing (Troides helena, hereafter “BW”) and Common Rose (Pachliopta aristolochiae, hereafter “CR”) on their non-native host plant Aristolochia acuminata. To do this, we mapped the butterfly and host plant distributions and estimated the occupancy of the butterfly species in Singapore. We also identified the species habitat dependence (forests, urban parks and private gardens), establish relationships between various habitat and life-history parameters (such as distance from larval host plant, % natural tree cover, % managed tree cover, % impervious surface cover) and investigated spatial and temporal niche overlap between the two species. The BW and CR are listed as nationally “vulnerable” (Singapore Red Data book version 2: Davison et al., 2008). CR was voted as the people’s choice of the national butterfly of Singapore in 2015 (Zengkun, 2015).
Methods
Host Plants of the Study Species
BW and CR butterflies use the non-native Aristolochia acuminata (Figure 1) as their host plant in Singapore (Tan and Khew, 2012; A. J. pers. obs.) as Aristolochia jackii (the only known Aristolochia species native to Singapore) is considered nationally extirpated. Its last known wild habitat, i.e., Jurong swamp in Singapore was destroyed in the 1930s. A. acuminata is believed to be introduced for its ornamental value with earliest records from the Botanic Gardens in 1918 (Chong et al., 2009; Singapore Herbarium Online, 2020) and in the past decades has been mainly planted by butterfly enthusiasts. BW and CR are believed to have utilized A. jackii as their native host plant in Singapore in the past and are hypothesized to have survived extirpation by relying on the introduced A. acuminata post extirpation of their native host plant (Jain et al., 2019).
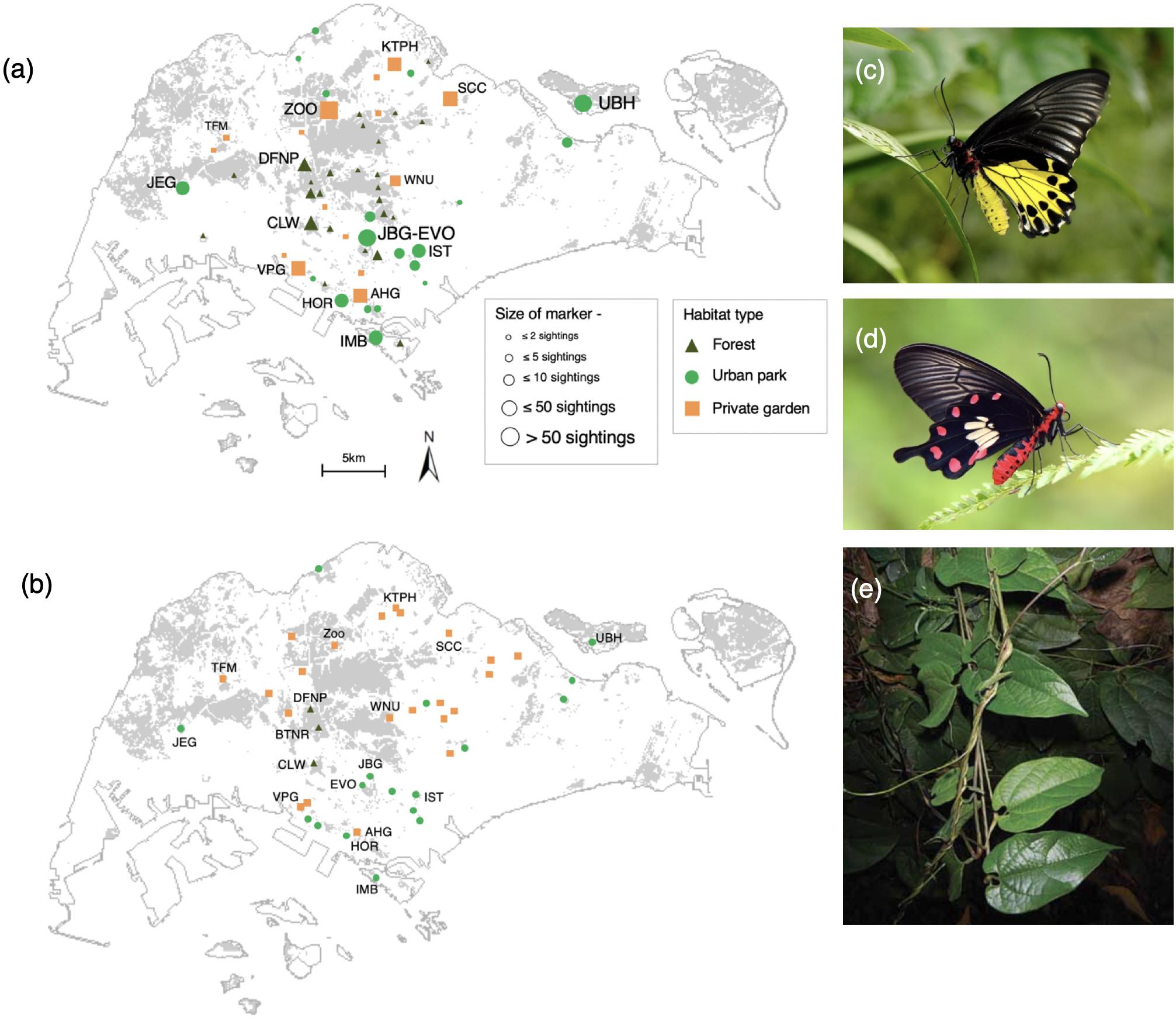
Figure 1. (a) Combined sightings of Common Birdwing (BW) and Common Rose (CR) butterflies, (b) Larval host plant locations across habitat types in Singapore from years 1999 to 2019. Gray areas in the map refer to forested areas. Abbreviations refer to source locations identified by experts (see Supplementary Table 1 for details). (c) Common Birdwing, (d) Common Rose, (e) Aristolochia acuminata larval host plant. Photos (c,d) by Tea Yi Kai, (e) by Amy Tsang.
Data Collection for Habitat Mapping
We consulted 30 local butterfly experts (including A. J.) about areas where the BW and CR have been sighted in Singapore since the year 1999. These consultations yielded 61 locations between the years 1999 and 2009 that were mapped to a 20 m × 20 m resolution. The survey effort at each location as estimated by the experts was also recorded. Because BW and CR are large and conspicuous butterflies, we assumed that local experts could identify them reliably in the wild.
Butterfly surveys were conducted across 125 locations between the years 2010 and 2014 (Figure 1a) ranging from forested areas to urban parks and private gardens. Our survey locations included areas where the two species have been previously sighted, or locations with a high perceived likelihood of BW or CR sightings (Supplementary Figure 1), i.e., proximity to known host plants and/or perceived appropriate habitat conditions (e.g., medium to high canopy cover, multi-tiered urban plantings or a complex habitat structure). The western catchment forest and Pulau Tekong (in the east) could not be sampled because they are restricted military areas. However, experts were asked to include sightings from these locations.
All locations were visited at least five times, with most locations visited more than 10 times on days of clear weather (no cloud or rain conditions); each visit lasted at least an hour or more. Visits were spaced out over time with each location visited typically once every 3–6 months. The minimum distance between two locations was 400 m. Existing trails were walked in forests and urban park locations. Additionally, transects of 100 m length and 2.5 m width on both sides of transect locations were established in forested sites in the Bukit Timah and Central Catchment Nature Reserves, which are the largest forest patches in Singapore. The number of transects per patch varied depending on the patch size, with larger forest patches (>100 ha) having 8–10 off-trail transect locations. Private gardens were searched as exhaustively as possible each visit, in lieu of establishing transects.
The abundance of BW and CR was recorded during each visit at every location. One hundred and nineteen locations with a total of 6,294 h of survey effort were retained in the analysis (Table 1). Locations with less than 5 h spent were deemed insufficient to detect the study species and were discarded. The 2010–2014 surveys included 51 locations that overlapped with locations surveyed between 1999 and 2009. Overall, 72% of survey effort and 84% of butterfly sightings occurred between the years 2010 and 2014. Additionally, sightings of BW or CR and locations of their host plants were checked between the years 2015 and 2019 on www.inaturalist.org for all of Singapore. Three new sighting locations were discovered during this time that were not raised by the experts or recorded between the years 1999 and 2014.
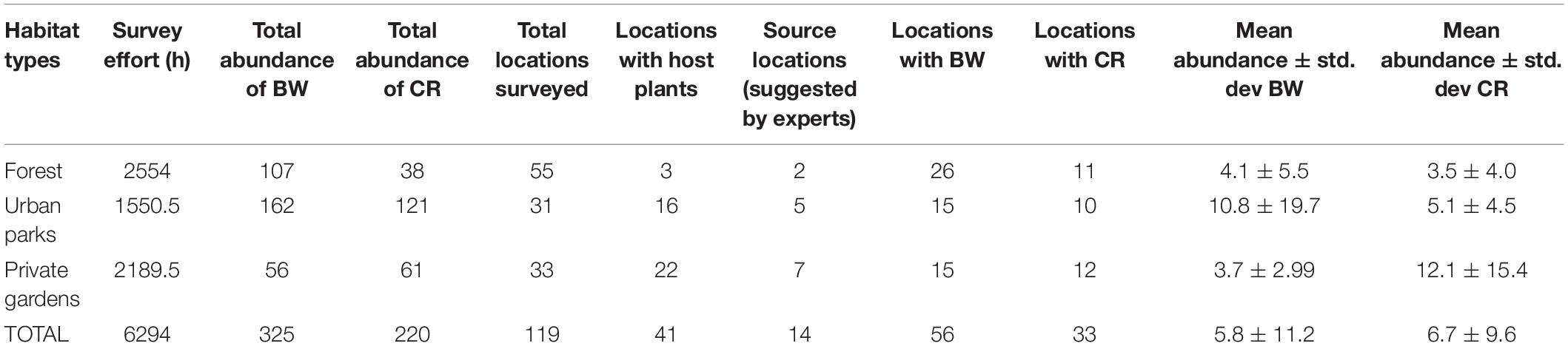
Table 1. Abundance (number of sightings) of Common Birdwing (BW) and Common Rose (CR), presence of host plants and source locations across habitat types between years 1999 and 2019.
Known locations of the host plant A. acuminata (n = 41) between years 1999 and 2019 were compiled with the help of local butterfly and plant experts, relevant personnel from the National Parks Board (Singapore) and Singapore Botanic Gardens Herbarium records (Singapore Herbarium Online, 2020). These locations were mapped to a 20 m × 20 m resolution. The majority (>80%) of locations were ground-truthed between the years 2013 and 2014, with additional locations (where adults were sighted between 2015 and 2019) ground-truthed in year 2019–2020 to confirm if A. acuminata was still found there (Figure 1b). All except four sites were found to (still) have the host plant.
Source Population Consultations
A.J. consulted 30 experts who have monitored and studied BW and CR over the years (some since the year 1999) to identify likely (or certain) source populations for BW and CR based on the consistency of butterfly observations during their field visits. They identified 14 potential source population locations (see Supplementary Table 1).
Models to Examine Habitat Preference and Spatial Occurrence
To establish the relationships between various land-use and life-history factors that can contribute to the distributions of the two butterfly species, we took a geospatial approach utilizing random forest models. Specifically, we implemented conditional random forests, which is a machine learning technique that relies upon a series of conditional inference trees (n = 999) and the permutation of variables (mtry = 7) to assess the relative contribution of each variable (Hothorn et al., 2006; Strobl et al., 2007). Conditional random forest models were chosen owing to their ability to handle and reduce the effects of overfitting, selection bias, and collinearity (Hothorn et al., 2006). Besides being able to provide accurate predictions, random forest models are also able to generate variable importance values that denote the degree of influence each variable had on improving its performance (indicated as variable importance in Figure 3), by covering the impact of each explanatory variable individually as well as in multivariate interactions with other explanatory variables. The variable importance values in random forest models do not represent the directionality between explanatory and response variables and instead represent the degree of importance. Positive variable importance means that the model performance improves with the inclusion of the explanatory variable in the model. Negative variable importance means that removing a given variable from the model improves the performance. It can be inferred that such variables do not have a role in the prediction and are not important.
These models were constructed based on each butterfly species’ distribution data (presence or absence), and the associated land-use and life-history conditions within the 100 m surrounding that species locality (i.e., data from each 100 m raster cell resolution). Land-use variables considered here were obtained from Gaw et al. (2019), which is based on land-cover from 2003–2018 and included classifications that reflected managed and natural tree cover, managed and natural shrub/grassland cover, bare ground cover, building cover, waterbody and impervious surface cover (see Gaw et al., 2019 for details). Time period was based on the month and year of data collection. Dependence on life-history variables was estimated by utilizing sampling results and constructing maps representing minimum distance from the nearest larval host plant and potential population source in meters (as identified by experts).
Data was randomly split into testing (75%) and training (25%), and bootstrapped 50 times to account for potential uncertainties (reported as 95% confidence intervals in Figure 3). We then projected the averaged model across Singapore’s landscape to predict the potential occurrence of both butterfly species. Owing to the complexities of random forest models, and the inability of variable importance values to denote directionality between explanatory and response variables, we secondarily generated response curves by using the random forest model (formed by the “party” package) to predict the effect of increasing values of the five most important explanatory variable for each species (e.g., distance from source, managed tree cover) on the response variable (probability of occurrence) to elucidate more precise relationships.
All analyses were performed in R version 3.6.0 (R Core Team, 2019), utilizing the package “party” (Hothorn et al., 2006; Strobl et al., 2007) and “raster” (Hijmans et al., 2019).
Seasonality
Seasonal patterns for BW and CR were inferred based on sighting records across months that were aggregated over the study period. We also collated records of BW and CR larvae in consultation with experts and during surveys and ground-truthing of the host plants. However, larval records were not included in the species distribution models.
Results
Abundance Across Habitat Types
Overall, ∼55% of locations surveyed had the presence of BW or CR (Table 1). Urban parks had the highest abundance of both butterfly species. Private gardens had the highest proportion (22/33 locations) of host plant locations but the lowest abundance of BW among the three habitats (Table 1 and Figure 1). A greater percentage (29%) of BW sightings were in forest habitats than CR which had only 8% of sightings in forest habitats. CR abundance was higher in private gardens than forested areas.
There was considerable variation in survey effort (average = 52.9 ± 35.5 h) per location. However, correlations between survey effort and BW presence (Pearson’s R = 0.035, p = 0.71, n = 119) as well as survey effort and CR presence (Pearson’s R = 0.16, p = 0.095, n = 119) were not significant.
Spatial Occurrence
Based on the area under the receiver operating characteristic curve (AUC) value, the accuracy of models for BW and CR was 0.809 ± 0.006 and 0.669 ± 0.02 (95% confidence interval), respectively. Overall, BW tended to possess more areas with a higher probability of occurrence compared to CR. There was a relatively high degree of spatial niche overlap between the two species (Schoener’s D = 0.960; I-statistic = 0.999; Figure 2) suggesting that they possess similar spatial distributions.
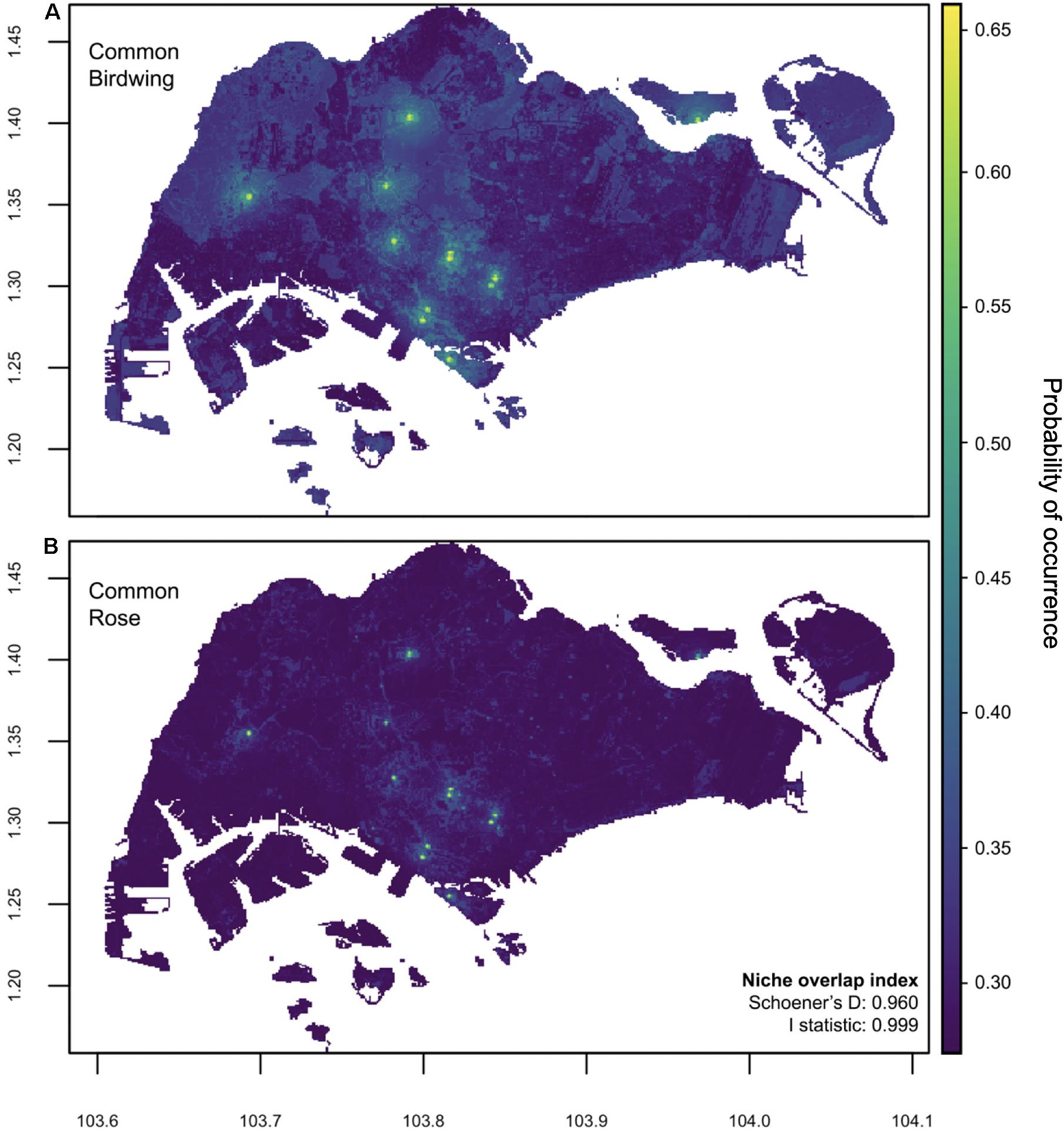
Figure 2. Prediction maps showing the probability of occurrence of (A) BW and (B) CR butterfly species in Singapore. Both species possess similar predicted occurrence patterns and possess a relatively high degree of spatial overlap, as indicated by the Schoener’s D and I-statistic. The latitude and longitude values are shown on the vertical and horizontal axis, respectively.
Source Populations
We found that the source population locations identified by experts corresponded to a minimum of 0.3 predicted probability of occurrence for BW, and a minimum of 0.14 predicted probability of occurrence for CR (Figure 2). Only 8 of 14 source locations had >0.3 predicted probability of occurrence for CR. Ten of 14 locations reported a minimum of 5 BW individuals whereas only 8 of 14 locations reported a minimum of 5 CR individuals. Our results support the expert opinion and indicate that the presumed 14 locations are likely source populations for BW. However, if the minimum of 0.3 predicted probability of occurrence (as observed for BW) is applied to CR, only 8 locations qualify as CR source populations. Additionally, the importance of locations where BW or CR abundance was low (<5 individuals) may be debated as being a source. The presence of larval host plant was confirmed at all source populations by ground truthing. No additional source populations (not identified by experts) were detected by the species distribution models.
Relationship Between Butterfly Occurrence and Habitat and Life-History Parameters
The distance from the nearest source population had the highest influence on BW occurrence and this and distance from larval host plant had the highest influence on CR occurrence (Figure 3). The probability of occurrence for both species greatly reduced further away from the population source and larval host plant (Figure 4). Distance from larval host plant, impervious surface cover, managed tree cover and natural tree cover were also important for the occurrence of BW (Figures 3, 4). Managed tree cover and managed shrub/grassland cover were important and had a positive influence on CR occurrence (Figures 3, 4). Impervious surface cover had negative influence on BW and CR occurrence (Figure 4).
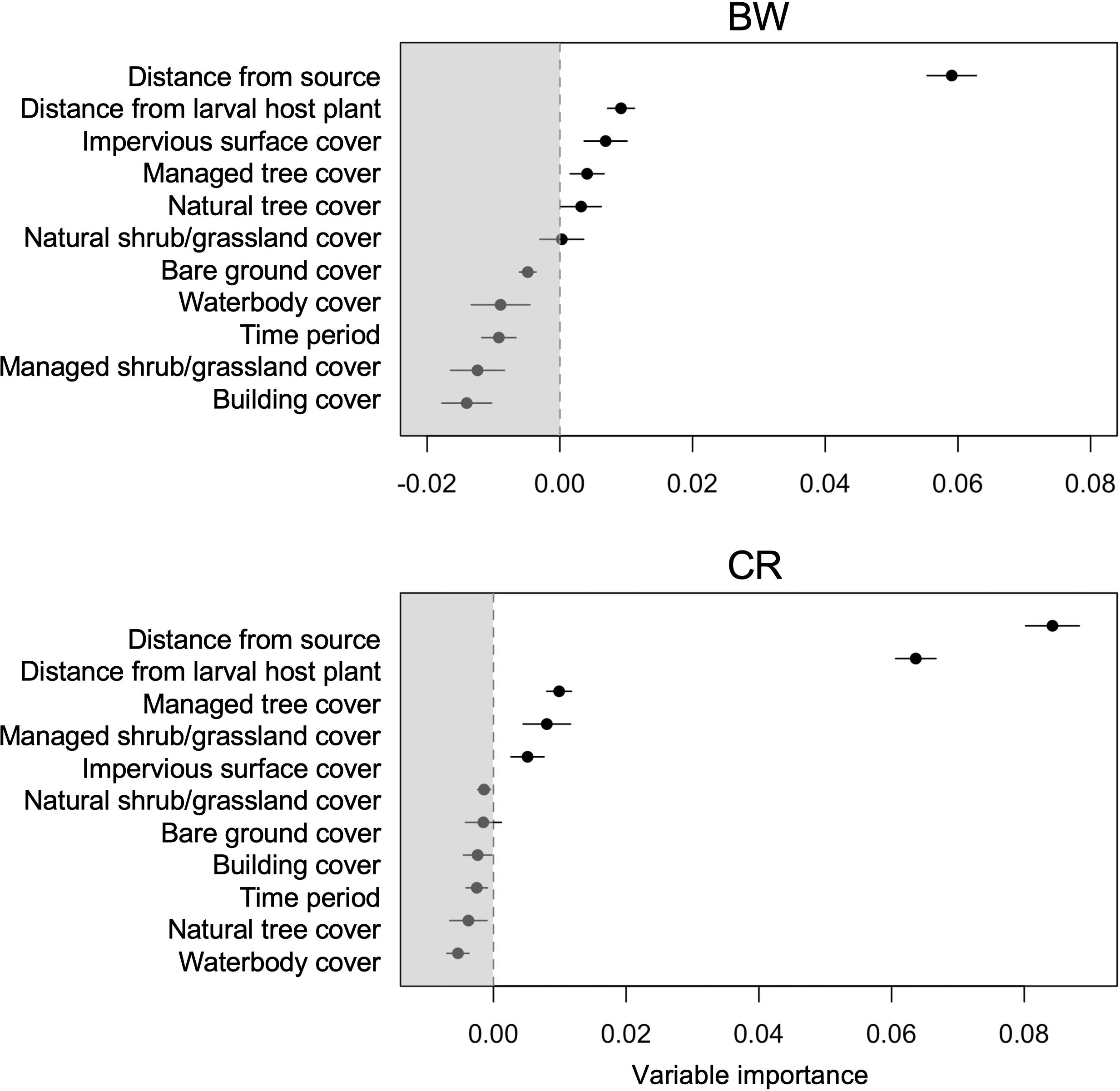
Figure 3. Relative importance of habitat and life-history parameter values on the occurrence of BW and CR butterfly species in the random forest model. Error bars are 95% confidence intervals.
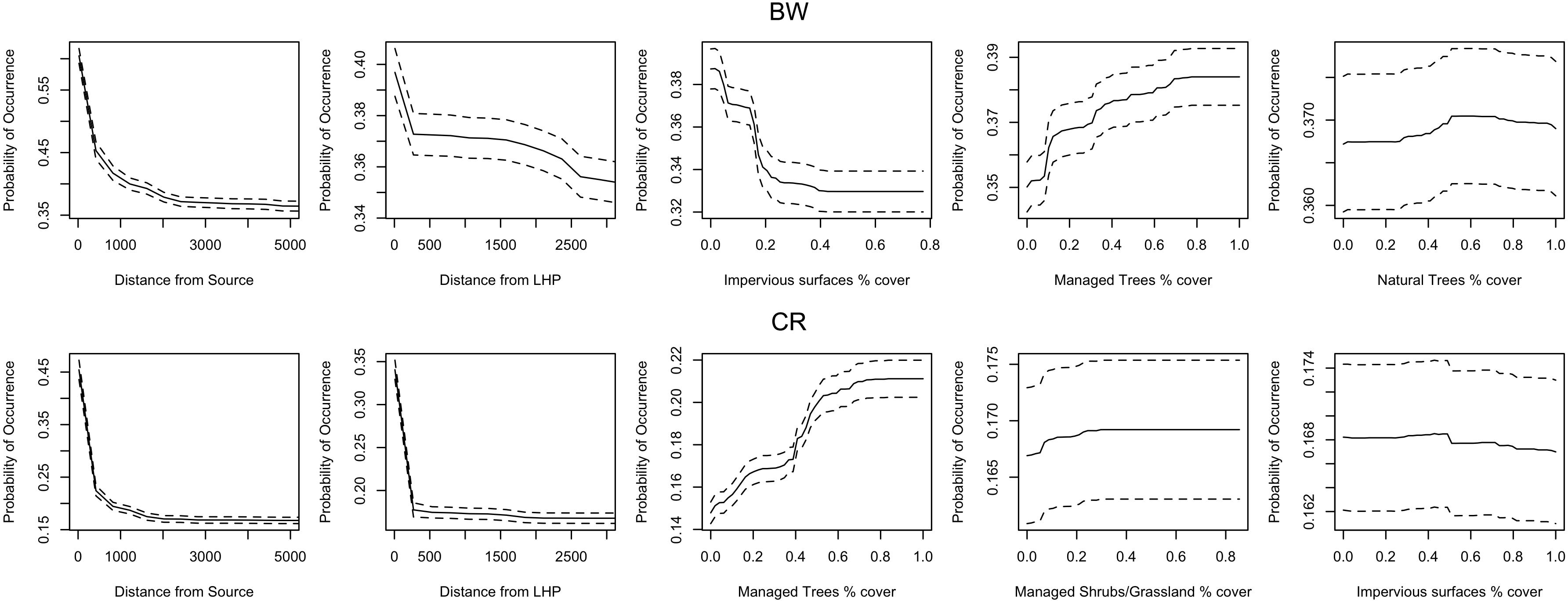
Figure 4. Response curves showing the relationship between the probability of occurrence of BW and CR various habitat and life-history parameters. The order of the response curves is by the importance of the variable. Distances are shown in meters. Habitat variables (e.g., managed tree cover) are shown as percentage values. LHP refers to the larval host plant. The dotted lines are 95% confidence intervals.
Seasonality
Aggregated over the study period, BW and CR adults were recorded in all 12 months of the year across survey locations. CR larvae were recorded in all 12 months but BW larvae were recorded in 9 months of the year with no records in March, May and August. The relative abundance of BW peaked in December with smaller peaks in February, May and July. The relative abundance of CR peaked in June, August and January (Figure 5). Typically, CR populations peaked a month after BW populations (Figure 5).
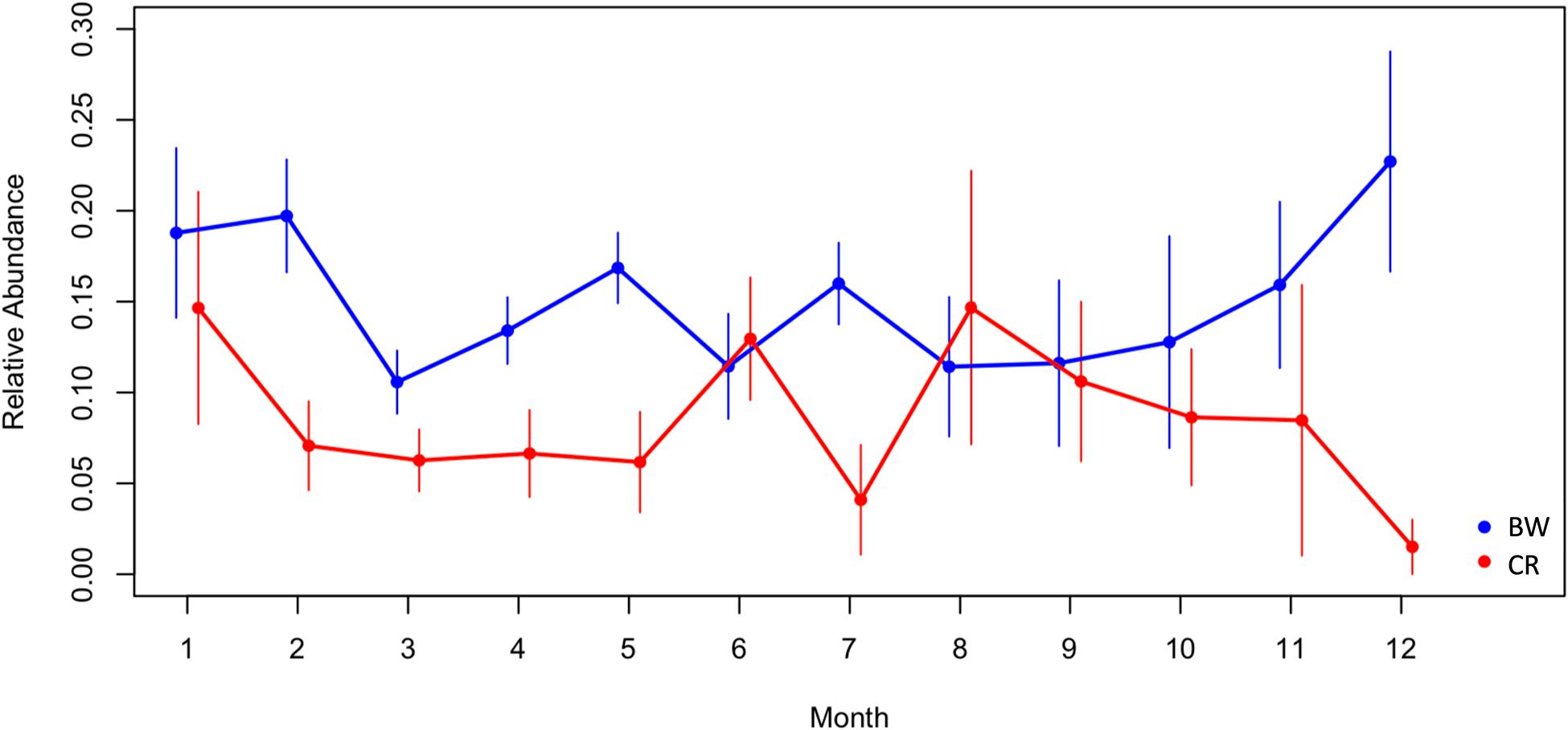
Figure 5. Relative abundance and seasonality BW and CR across months aggregated over the study period. Relative abundance = log(abundance + 1)/survey effort. Error bars indicate standard errors of the mean.
Discussion
Critical Dependence on Non-native Urban Plantings
Our results show that the highest abundance and the majority of source populations of BW and CR are found in urban parks and private gardens. Therefore, urban plantings are critical for the survival of these butterflies. This is an unusual situation because BW and CR are forest species that can be found in primary forest and secondary forest habitats throughout Southeast Asia (Hsieh et al., 2010; Corbet and Pendlebury, 2020). The current situation, therefore, presents an unusual case of two forest butterflies that have switched to a non-native host plant and now rely completely on urban plantings after the extirpation of their native host plant.
With a greater prevalence of non-native plants (particularly introduced as ornamentals) across urban centers globally (McKinney, 2006; Trentanovi et al., 2013), the reliance of native butterflies on non-native host plants seems poised to become an increasingly common phenomenon. At least a third of butterfly species in California are known to utilize non-native plant taxa (Graves and Shapiro, 2003) with geographic range and native diet breadth being significant predictors of non-native host use (Jahner et al., 2011). As many native plants face extirpation with loss and fragmentation of natural habitats in urban centers, non-native plants may provide alternative hosts and refuge for butterflies and other native fauna. While some non-native species cause much ecological damage, the potential conservation and ecosystem service value of non-native species is increasingly being recognized in areas where climate and land use are changing rapidly (Schlaepfer et al., 2011).
Habitat Preference and Potential Natal Affinity
BW and CR appear to have similar spatial niches and similar species occurrence responses as they share the same larval host plant and have a preference toward managed tree cover. However, the main difference between the two species appears to be in their preference toward forested habitats. The spatial model confirmed that BW has a higher habitat preference toward natural tree cover than CR, which corroborates with higher BW sightings in forest habitats than CR. Studies on other species of Birdwings (e.g., Troides aeacus formasanus in Taiwan, Hsieh et al., 2010) suggest that they prefer inner forest compared with forest trails and road edges.
In contrast, CR occurrence was positively influenced by the managed tree cover and managed shrub/grassland cover in the spatial model. One explanation for this result could be that CR has a stronger natal affinity than BW which limits CR’s home range around the planted larval host plants in urban parks and private gardens in Singapore. However, further research on the role of CR’s natal affinity in determining its habitat preference is needed. In its natural habitat in western China, Byasa impediens, a species with similar life-history characteristics as CR, was observed to prefer open areas and sparse forests, and did not utilize host plants in forests with dense vegetation (Li et al., 2010). CR also exhibits a greater degree of flower specialization compared with BW (Jain et al., 2016). Finally, it should be noted that our results showing model performance for BW, at AUC > 0.8, is considered acceptable whereas the model performance for CR, at AUC = 0.669, is considered relatively poor and has limited predictive power. For this reason, it may be premature to generalize model results for CR. More field surveys are suggested to improve the predictive power and generalizability of the CR model.
The Persistence and Connectivity of Source Populations
Meta-population theory suggests that a butterfly should have a minimum of 20 suitable habitat patches for the long-term survival of the meta-populations (Hanski et al., 1995; Li et al., 2010). The identified BW and CR source population locations in Singapore do not appear to meet the stable meta-population criterion of 20 patches and may be under a high risk of inbreeding and potential extinction. During the study, we observed that BW and CR were sometimes missing in smaller population sources (when ranked by BW or CR abundance) such as SCC and KTPH. This observation agrees with the literature where only large habitat patches were found to be significant predictors of year on year occupancy for the Golden Birdwing (Troides aeacus) and local extinctions in small patches were common (Li et al., 2010). It may also be possible that population sources of BW and CR exist in southern Malaysia within the species dispersal range and may be contributing to the persistence of the species in Singapore. Systematic long-term population monitoring at each source population is necessary to determine local extinction rates and better understand long-term metapopulation persistence in Singapore and potential interactions with Malaysian populations.
Connectivity between source populations identified through our study can be understood based on dispersal estimates from the Golden Birdwing (Troies aeacus) and Byasa impediens as they are species phylogenetically related to and with similar life-history characteristics as BW and CR, respectively. B. impediens has been found to disperse up to 5 km in mark-recapture studies (Li et al., 2012). T. aeacus was recorded to disperse to 4.3 km in telemetry studies in China (Wang et al., 2019). However, it likely disperses to more than 5 km as it is bigger in size than B. impediens. BW and CR can be assumed to disperse to 5 km over suitable habitat as a conservative estimate, however, their dispersal over impervious surfaces and unsuitable habitat remains unknown. Further research is needed to understand the connectivity between various previously identified source populations in Singapore.
Temporal Niche Separation
Our findings that the relative abundance of BW and CR peaks at different months suggests that the two species may have some temporal niche separation. This may be adaptive as the two butterflies share the same larval host plant. The emergence of early instar caterpillars may also be timed with the presence of young leaves on the host plant. Our field observations suggest that the BW tends to use larval host plants with more leaves (i.e., older plants) as BW larvae are bigger and have a more voracious appetite than CR. The temporal niche separation may also be related to BW and CR caterpillar’s ability to digest Aristolochic acids in A. acuminata leaves which vary with flowering and fruiting cycles (Brown et al., 1980). Additionally, BW caterpillars are sometimes observed to ring-bark their host plant vines, similar to other Birdwing species (Ornithoptera alexandrae and O. goliath larvae; Parsons, 1992) which leads to withering of the host plant and thereby, rending it unsuitable for a few weeks until plant recovery. The role of ring barking in the context of temporal niche separation of the two species should be investigated in the future.
Implications for Habitat Enrichment
We show that clustered populations of BW and CR have emerged in Singapore shaped by the locations of larval host plantings by butterfly enthusiasts in Singapore. Long-term survival of these threatened butterflies would require a planned habitat enrichment strategy that can increase the size of existing source populations, increase the number of source populations to at least 20 patches for stable metapopulations and increase the connectivity between existing source populations to reduce local extinction risk. The observed habitat preferences of the species can provide important cues in site selection for habitat enrichment.
The planting of host plants in urban parks adjacent to forest habitats may be the most suitable locations for BW enrichment because BW’s probability of occurrence increased with natural and managed tree cover. Isolated urban parks and gardens can be utilized for CR enrichment because natural tree cover was not important for CR’s probability of occurrence. Habitats can also be enriched with nectar species preferred by BW and CR (Jain et al., 2016) to achieve synergistic effects.
Though urban plantings are currently the only available option for BW and CR management in Singapore (planting of the non-native host plant is not permissible in forest habitats), it should be no surprise that the longevity of urban plantings is limited as they are prone to frequent landscape changes. There have been at least 4 documented locations of breeding populations of BW and CR in Singapore that were wiped out in the past (e.g., Asimont Lane) as a result of a change in garden/park management (van Heezik et al., 2012). In fact, this dependence on a planted resource continues to make the BW and CR particularly vulnerable to extinction. Future studies should also aim to identify if any identified population sources may be ecological traps.
A longer-term strategy with less risk would be to reintroduce Aristolochia jackii as the native host plant for the BW and CR. At least three of California’s butterfly species have been documented to have lower rates of survival on non-native host plants compared to their native counterpart (Graves and Shapiro, 2003). If A. jackii turns out to be an effective host plant for BW and CR, restoration efforts could be united to systematically bring back these butterflies to forest habitats in Singapore.
A coordinated planting effort of (native and non-native) host plants in forests, urban parks and private gardens to expand the size, quantity and connectivity of source populations could be explored in Singapore. In particular, source populations may need to be established in the central and eastern parts of Singapore to connect the western and easternmost (i.e., Ubin) populations. Important lessons can be drawn from the community engagement program for the Richmond Birdwing (Ornithoptera richmondia) in Australia in which schools planted the rare native host plant (Pararistolochia praevenosa) to restore historical connectivity between the fragmented butterfly populations (Sands, 2008). The success of such an effort also depends on an improved understanding of the study species’ micro-habitat preference during the juvenile (egg/caterpillar/pupa) stages across forested and urban areas.
Broadly speaking, the enrichment strategy identified here that introduced (non-native) host plants could be used for butterfly conservation is novel in the context of tropical urban habitats. Caveats involved include possibly lower rates of larval survival on non-native host plants compared to their native counterpart (see Graves and Shapiro, 2003). For this season, we recommend that non-native plants be planted as a secondary strategy only when re-establishment of the native plants has failed. The wider ecological consequences of non-native plantings will also have to be carefully studied before such a strategy can be widely adopted for conservation.
Data Availability Statement
The original contributions presented in the study are included in the article/Supplementary Material, further inquiries can be directed to the corresponding author/s.
Author Contributions
AJ designed the study and was responsible for data collection. AJ and YZ analyzed the data. AJ, YZ and EW contributed ideas and drafted the manuscript. All authors contributed to the article and approved the submitted version.
Funding
This research was supported under Wildlife Reserves Singapore Conservation Grant (2013), Joan Mosenthal DeWind Award by Xerces Society USA (2014), and National Parks Board (Singapore) permits NP/RP12-072 and NP/RP13-003.
Conflict of Interest
The authors declare that the research was conducted in the absence of any commercial or financial relationships that could be construed as a potential conflict of interest.
Acknowledgments
We thank the following people for contributing butterfly sighting records—Amy Tsang, Andrew Tay, Angie Ng, Ariel Chong, Clayton Low, Cheong Weei Gan, Craig Williams, Crystalyn K. L. Leo, Cuifen Pui, Howming Tian, Kwek Yan Chong, Lena Chow, Margaret Hall, Maggie Lim, Richard Ong, Shawn K. Y. Lum, Simon K. M. Chan, Sin Khoon Khew, Stella L. L. Tan, Steven S. H. Neo, Steven Chong, K. F. Yap, Subaraj Rajathurai, Yi Kai Tea, and Zakil Jalil. We also thank Maxel Ng, Sharon Chan, and Samantha Lai from National Parks Board Singapore and plant experts—Ali Ibrahim, Angie Ng, Hugh T. Tan, Shawn K. Y. Lum, and Y. C. Wee for advice on Aristolochia acuminata locations. We thank the numerous contributors to citizen science project “Butterflies of Singapore” and “Aristolochia in Singapore” hosted on iNaturalist. Finally, we also thank the reviewers for their constructive comments which helped improve the manuscript.
Supplementary Material
The Supplementary Material for this article can be found online at: https://www.frontiersin.org/articles/10.3389/fevo.2021.655012/full#supplementary-material
Supplementary Figure 1 | Survey locations for BW and CR butterflies divided by habitat types.
Supplementary Table 1 | Source populations for Common Birdwing (BW) and Common Rose (CR) in Singapore as identified by experts, survey effort and BW and CR abundance recorded as part of our study. BW and CR prediction values represent the probability of occurrence in Singapore based on the random forest models.
References
Baguette, M., Clobert, J., and Schtickzelle, N. (2011). Metapopulation dynamics of the bog fritillary butterfly: experimental changes in habitat quality induced negative density-dependent dispersal. Ecography 34, 170–176. doi: 10.1111/j.1600-0587.2010.06212.x
Bonebrake, T. C., Ponisio, L. C., Boggs, C. L., and Ehrlich, P. R. (2010). More than just indicators: a review of tropical butterfly ecology conservation. Biol. Conserv. 143, 1831–1841. doi: 10.1016/j.biocon.2010.04.044
Brown, K. S., Damman, A. J., and Feeny, P. (1980). Troidine Swallowtails (Lepidoptera: Papilionidae) in southeastern Brazil: natural history and foodplant relationships. J. Res. Lepid. 19, 199–226.
Chong, K. Y., Tan, H. W. T., and Corlett, R. T. (2009). A Checklist of the Total Vascular Plant Flora of Singapore. Singapore: Raffles Museum of Biodiversity Research.
Cleary, D. F. R., and Mooers, A. O. (2004). Butterfly species richness and community composition in forests affected by ENSO-induced burning and habitat isolation in Borneo. Trop. Ecol. 20, 359–367. doi: 10.1017/s0266467404001312
Corbet, A. S., and Pendlebury, H. M. (2020). The Butterflies of the Malay Peninsula, Fifth edition. Malaysia: Malaysian Nature Society.
Crone, E., and Schultz, C. (2003). “Movement behavior and minimum patch size for butterfly population persistence,” in Butterflies as Model Systems: Ecology and Evolution Taking Flight, eds C. Boggs, P. Ehrlich, and W. Watt (Chicago: University of Chicago Press), 561–576.
Davison, G. W. H., Ng, P. K. L., and Chew, H. H. (2008). Singapore Red Data Book: Threatened Plants and Animals of Singapore, Second Edn. Malaysia: Nature Society Singapore.
Dunn, R. R. (2004). Recovery of faunal communities during tropical forest regeneration. Conserv. Biol. 18, 302–309. doi: 10.1111/j.1523-1739.2004.00151.x
Gaw, L. Y.-F., Yee, A. T. K., and Richards, D. R. (2019). A high-resolution map of Singapore’s Terrestrial ecosystems. Data 4:116. doi: 10.3390/data4030116
Graves, S. D., and Shapiro, A. M. (2003). Exotics as host plants of the California butterfly fauna. Biol. Conserv. 110, 413–433. doi: 10.1016/s0006-3207(02)00233-1
Hanski, I., Pakkala, T., Kuussaari, M., and Lei, G. C. (1995). Metapopulation persistence of an endangered butterfly in a fragmented landscape. Oikos 72, 21–28. doi: 10.2307/3546033
Hijmans, R. J., van Etten, J., Sumner, M., Cheng, J., Bevan, A., Bivand, R., et al. (2019). raster: Geographic Data Analysis and Modeling. Version 3.0-7.
Hothorn, T., Hornik, K., and Zeileis, A. (2006). Unbiased recursive partitioning: a conditional inference framework. J. Comput. Graph. Stat. 15, 651–674. doi: 10.1198/106186006x133933
Hsieh, K. J., Kuo, Y. L., Perng, J. J., Lee, T. C., and Lee, H. L. (2010). Oviposition preference and larval survival of Troides aeaceus formasanus (Lepidoptera: Papilionidae) on Aristolochia zollingeriana in different environments in the Kenting area. Taiwan J. For. Sci. 25, 353–368.
Jahner, J. P., Bonilla, M. M., Badik, K. J., Shapiro, A. M., and Forister, M. L. (2011). Use of exotic hosts by lepidoptera: widespread species colonize more novel hosts. Evolution 65, 2719–2724. doi: 10.1111/j.1558-5646.2011.01310.x
Jain, A., Gan, C. W., Tsang, A., and Tsang, K. C. (2019). The Common Rose and Common Birdwing: a Race Against Time? Nature Watch Oct – Dec 2019: 9 – 11. Singapore: Nature Society Singapore.
Jain, A., Khew, S. K., Gan, C. W., and Webb, E. L. (2018). Butterfly extirpations, discoveries and rediscoveries in Singapore in 28 years. Raffles Bull. Zool. 66, 217–257.
Jain, A., Kunte, K., and Webb, E. L. (2016). Flower specialization of butterflies and impacts of non-native flower use in a transformed tropical landscape. Biol. Conserv. 201, 184–191. doi: 10.1016/j.biocon.2016.06.034
Li, X. S., Luo, Y. Q., Zhang, Y. L., Schweiger, O., Settele, J., and Yang, Q. S. (2010). On the conservation biology of a Chinese population of the birdwing Troides aeacus (Lepidoptera: Papilionidae). J. Insect Conserv. 14, 257–268. doi: 10.1007/s10841-009-9254-x
Li, X. S., Zhang, Y. L., Settele, J., Franzen, M., and Schweiger, O. (2012). Long-distance dispersal and habitat use of the butterfly Byasa impediens in a fragmented subtropical forest. Insect Conserv. Divers. 6, 170–178. doi: 10.1111/j.1752-4598.2012.00199.x
McKinney, M. L. (2006). Urbanization as a major cause of biotic homogenization. Biol. Conserv. 127, 247–260. doi: 10.1016/j.biocon.2005.09.005
New, T. R., Pyle, R. M., Thomas, J. A., Thomas, C. D., and Hammond, P. C. (1995). Butterfly conservation management. Annu. Rev. Entomol. 40, 57–83.
Parsons, M. J. (1992). The world’s largest butterfly endangered: the ecology, status and conservation of Ornithoptera alexandrae (Lepidoptera: Papilionidae). Trop. Lepid. 3(Suppl. 1), 33–60.
R Core Team (2019). R: a Language and Environment for Statistical Computing. Vienna: R Foundation for Statistical Computing.
Reichard, S. H., Chalker-Scott, L., and Buchanan, S. (2001). “Interactions among non-native plants and birds,” in Avian Ecology and Conservation in an Urbanizing World, eds J. M. Marzluff, R. Bowman, and R. Donnelly (Boston, MA: Springer).
Sands, D. (2008). Conserving the richmond birdwing butterfly for over two decades: where to next? Ecol. Manag. Restoration 9, 4–16. doi: 10.1111/j.1442-8903.2008.00382.x
Schlaepfer, M. A., Sax, D. F., and Olden, J. D. (2011). The potential conservation value of non-native species. Conserv. Biol. 25, 428–437. doi: 10.1111/j.1523-1739.2010.01646.x
Schultz, C. B., and Chang, G. C. (1998). “Challenges in insect conservation: managing fluctuating populations in disturbed environments,” in Conservation Biology for the Coming Decade, eds P. Fiedler and P. Kareiva (New York, NY: Chapman & Hall), 228–254. doi: 10.1007/978-1-4615-6051-7_9
Schultz, C. B., and Dlugosch, K. M. (1999). Nectar and hostplant scarcity limit populations of an endangered Oregon butterfly. Oecologia 129, 231–238. doi: 10.1007/s004420050781
Schultz, C. B., Russell, C., and Wynn, L. (2008). Restoration, reintroduction, and captive propagation for at-risk butterflies: a review of British and American conservation efforts. Israel J. Ecol. Evol. 54, 41–61. doi: 10.1560/ijee.54.1.41
Scriven, S. A., Williams, S. H., Ghani, M. A., Agama, A. L., Benedick, S., Brodie, J. F., et al. (2019). Assessing the effectivenss of protected areas for conserving range-restricted rain forest butterflies in Sabah. Borneo. Biotropica 52, 380–391. doi: 10.1111/btp.12708
Singapore Herbarium Online (2020). Available online at: http://herbaria.plants.ox.ac.uk/bol/sing (accessed December. 29, 2020).
Strobl, C., Boulesteix, A.-L., Zeileis, A., and Hothorn, T. (2007). Bias in random forest variable importance measures: illustrations, sources and a solution. BMC Bioinform. 8:25.
Tan, H., and Khew, S. K. (2012). Caterpillars of Singapore’s Butterflies. Singapore: National Parks Board.
Theng, M., Jusoh, W. F. A., Jain, A., Huertas, B., Tan, D. J. X., Tan, H. Z., et al. (2020). A comprehensive assessment of diversity loss in a well-documented tropical insect fauna: almost half of Singapore’s butterfly species extirpated in 160 years. Biol. Conserv. 242:108401. doi: 10.1016/j.biocon.2019.108401
Trentanovi, G., Lippe, M. D., Sitzia, T., Ziechmann, U., Kowarik, I., and Cierjacks, A. (2013). Biotic homogenization at the community scale: disentangling the roles of urbanization and plant invasion. Divers. Distrib. 19, 738–748. doi: 10.1111/ddi.12028
van Heezik, Y. M., Dickinson, K. J. M., and Freeman, C. (2012). Closing the gap: communicating to change gardening practices in support of native biodiversity in urban private gardens. Ecol. Soc. 17:34.
van Kleunen, M., Dawson, W., Essl, F., Pergl, J., Winter, M., and Weber, E. (2015). Global exchange and accumulation of non-native plants. Nature 525, 100–103.
Wang, Z., Huang, Y., and Pierce, N. E. (2019). Radio telemetry helps record the dispersal patterns of birdwing butterflies in mountainous habitats: golden Birdwing (Troides aeacus) as an example. J. Insect Conserv. 23, 729–738. doi: 10.1007/s10841-019-00167-5
Xing, S., Au, T. F., Dufour, P. C., Cheng, W., Yuan, F. L., Jia, F., et al. (2019). Conservation of data deficient species under multiple threats: lessons from an iconic tropical butterfly (Teinopalpus aureus). Biol. Conserv. 234, 154–164. doi: 10.1016/j.biocon.2019.03.029
Zengkun, F. (2015). Common Rose Crowned S’pore’s National Butterfly. Straits Times. 6 Jun 2015. Home. Available online at: B4. https://www.asiaone.com/singapore/common-rose-crowned-spores-national-butterfly (Last accessed 29 December 2020).
Keywords: Lepidoptera, species distribution model, Singapore, Asia, urbanization, exotic species, introduced species
Citation: Jain A, Zeng Y and Webb EL (2021) Critical Dependence of Butterflies on a Non-native Host Plant in the Urban Tropics. Front. Ecol. Evol. 9:655012. doi: 10.3389/fevo.2021.655012
Received: 18 January 2021; Accepted: 16 April 2021;
Published: 11 May 2021.
Edited by:
Eben Goodale, Guangxi University, ChinaReviewed by:
Toby Tsang, The University of Hong Kong, Hong KongKonrad Kalarus, Institute for Agricultural and Forest Environment (PAS), Poland
Copyright © 2021 Jain, Zeng and Webb. This is an open-access article distributed under the terms of the Creative Commons Attribution License (CC BY). The use, distribution or reproduction in other forums is permitted, provided the original author(s) and the copyright owner(s) are credited and that the original publication in this journal is cited, in accordance with accepted academic practice. No use, distribution or reproduction is permitted which does not comply with these terms.
*Correspondence: Anuj Jain, YW51akB1Lm51cy5lZHU=; Edward L. Webb, dGVkLndlYmJAbnVzLmVkdS5zZw==
†Present Address: Anuj Jain, BirdLife International, Singapore, Singapore