- Ecology and Evolution, Research School of Biology, Australian National University, Acton, ACT, Australia
Theoretical studies predict that hosts of avian brood parasites should evolve defenses against parasitism in a matter of decades. However, opportunities to test these predictions are limited because brood parasites rarely switch to naïve hosts. Here, we capitalize on a recent host switch by the brood-parasitic Pacific Koel (Eudynamys orientalis) in eastern Australia, to investigate how quickly the Red Wattlebird (Anthochaera carunculata), a recent host that has been annexed by the koel within the last 90 years, can learn to recognize and mob adult cuckoos and evolve the ability to eject parasite eggs. Pacific Koel nestlings kill all host young, so there should be strong selection for hosts to evolve defenses. However, low parasitism rates and high egg recognition costs might slow the spread of egg ejection in our study populations, while adult parasite recognition should be able to spread more rapidly, as this defense has been shown to be a learned trait rather than a genetically inherited defense. We tested Red Wattlebirds at two sites where parasitism rate differed. As predicted, we found that the Red Wattlebird showed little or no ability to eject foreign model eggs at either site, whereas two historical hosts showed high levels of egg ejection at both sites. However, Red Wattlebirds responded significantly more aggressively to a koel mount than to mounts of a harmless control and nest predator at the site with the higher parasitism rate and gave significantly more alarm calls overall toward the koel mount. Our results support previous evidence that recognition and mobbing of a brood parasite are learned traits and may be especially beneficial to naïve hosts that have not had enough time or a high enough selection pressure to evolve egg rejection.
Introduction
Brood parasitic cuckoos lay eggs in the nests of other species and provide no parental care. Upon hatching, many cuckoo nestlings eject all host eggs and young from the nest, thereby completely eliminating the host’s reproductive success for that particular breeding attempt (Davies, 2000). This cost should select for the evolution of host defenses to circumvent brood parasitism. Mobbing is one type of host defense that has been shown to be highly effective in certain species at deterring parasitism (Feeney et al., 2013) and preventing the parasite from removing or damaging host eggs (Gloag et al., 2013). In rare cases, mobbing can result in the death of the parasite (e.g., Jackson and Kyne, 2010; Gloag et al., 2013; Abernathy and Langmore, 2016). If mobbing fails to prevent parasitism, egg ejection (removing parasitic eggs from the nest) can be an efficient defense for some hosts because it allows the host to keep its nest and most of its original clutch intact (reviewed in Soler, 2014).
There is evidence that the ability to discriminate and reject foreign eggs is a genetically inherited trait although female hosts may vary in their expression of this trait throughout their lives (Molina-Morales et al., 2014; Martínez et al., 2020). Multiple egg ejection studies have shown that host species tend to retain egg ejection ability even in the absence of parasitism (e.g., Avilés, 2004; Peer et al., 2005; Soler, 2014; Yang et al., 2014) and even through speciation events (Peer et al., 2013). Further, Martín-Gálvez et al. (2006) showed that genetic differences exist between individuals that ejected model parasitic eggs and those that accepted them in the European Magpie (Pica pica), although they did not control for age differences known to affect rejection (Martínez et al., 2020). Theoretical models predict that the evolution of egg rejection requires at least 30–100 years and will occur more quickly when parasitism rates and costs of parasitism are high (Rothstein, 1975a; Kelly, 1987; Takasu et al., 1993; Davies et al., 1996). According to one model, egg rejection could evolve within 97 years if parasitism rate is 50%, but would take 1,974 years if parasitism rate is 2% (Kelly, 1987). Similarly, Rothstein (1975a) calculated that under parasitism rates of 19% the proportion of egg rejecters in a population of Eastern Phoebes (Sayornis phoebe) would increase from 5 to 95% in about one hundred generations.
While egg ejection is likely to be a genetically inherited trait, the ability to recognize a brood parasite as a nest threat is likely to be a learned trait. Many studies have demonstrated that parasitized populations and individuals with prior experience of parasites respond more aggressively to a parasite near the nest than individuals that have not experienced parasitism (reviewed in Feeney et al., 2012). In one cuckoo host, the Superb Fairy-wren (Malurus cyaneus), first-year individuals that had never encountered a cuckoo did not mob a cuckoo mount, but learned to do so after witnessing mobbing of a cuckoo mount by their family members (Feeney and Langmore, 2013). Similar studies were conducted on captive European Blackbirds (Turdus merula) that were trained to mob a harmless species by watching other conspecifics mob it (Curio et al., 1978). If brood parasite recognition and mobbing is not dependent on genetic evolution and can be transmitted socially, then this trait has the potential to spread rapidly throughout a host population (Curio et al., 1978; Davies and Welbergen, 2009). This may be especially beneficial for naïve or recent hosts that have not had enough time or a high enough selection pressure to evolve other defenses such as egg ejection.
Because brood parasites rarely switch to naïve hosts, there are only a handful of studies that have quantified the types of defenses that naïve hosts use against brood parasites (Soler et al., 1994; Nakamura et al., 1998; Avilés et al., 2006; Spottiswoode and Stevens, 2012). Understanding what types of defenses are available to naïve hosts is important because it may help us understand how quickly hosts can evolve defenses and can aid us in making conservation decisions in regards to endangered potential hosts, as many brood parasites have been expanding their breeding ranges due to environmental changes (Rothstein, 1975a; Dinets et al., 2015).
The Pacific Koel (Eudynamys orientalis) provides a rare case of a cuckoo that has recently adopted a new host. Koels are found along the northern and eastern coasts of Australia and the nestlings are highly virulent, evicting all host young from the nest (Higgins, 1999). In the past, the koel’s primary hosts in New South Wales were the Magpie-lark (Grallina cyanoleuca) and Noisy Friarbird (Philemon corniculatus) (Brooker and Brooker, 1989, 2005). However, in recent decades they have switched to a new host, the Red Wattlebird (Anthochaera carunculata) (Abernathy and Langmore, 2017). Evidence suggests that the Red Wattlebird in the SE of Australia (where koel parasitism occurs) was likely to be naïve to brood parasitism, as range expansions of both the koel and wattlebird brought them into contact with one another around the early 1900s and the first time a koel was observed using the wattlebird as a host was in Sydney in 1978 (Abernathy and Langmore, 2017). Further, while wattlebirds in the SW of Australia are a host of the Pallid Cuckoo (Cuculus pallidus), they appear to be only rarely used as a host in the SE and there is no evidence that these two wattlebird populations interbreed (Abernathy and Langmore, 2017).
We conducted this study in both Sydney and Canberra. At the time of this study in Sydney (2016), wattlebirds may have been a host of the koel for around 38–86 years and experience a current parasitism rate of 24% (Abernathy and Langmore, 2017), which, as theory predicts, could be enough time and a high enough parasitism rate for egg ejection to have evolved (Rothstein, 1975a; Kelly, 1987; Takasu et al., 1993; Davies et al., 1996). However, parasitism rate in Canberra is very low (4%) and the length of time wattlebirds have been parasitized by koels is also less (around 8–33 years at the time of this study) (Abernathy and Langmore, 2017). Further, while successful parasitism by a koel means the wattlebird will lose its entire clutch (costs of parasitism are high), a previous study found that the impacts of koel parasitism on wattlebird breeding success in Sydney were low (Abernathy and Langmore, 2017). This was partly due to the koel’s poor timing of egg laying coupled with the wattlebird’s abandonment of koel eggs laid before the host had started laying (Abernathy and Langmore, 2017). Additionally, costs of recognition errors (rejecting a host egg rather than the parasitic egg) could be high, as wattlebird and koel eggs appear very similar in luminance and pattern and their ground colors were found to be nearly indistinguishable from a bird’s visual perspective (Abernathy et al., 2017, see also Takasu, 1998; Robert and Sorci, 1999). This high cost could be exacerbated by the fact that wattlebirds typically only lay 1–2 eggs per clutch, potentially making it more difficult to identify the parasitic egg as the “odd one out” in the nest. Because wattlebirds are a recent host, the close match in egg appearance between their eggs and koel eggs is unlikely to be the result of direct mimicry by the koel. Rather, it may be the result of mimicry by the koel of the wattlebird’s close relative and a historic host of the koel, the Noisy Friarbird, whose eggs also appear very similar in luminance and pattern and were indistinguishable in ground color from koel and wattlebird eggs (Abernathy et al., 2017). However, this would need to be tested with egg ejection experiments to determine if friarbirds and wattlebirds are capable of ejecting odd-looking eggs from their nests.
We hypothesize that the evolution of egg ejection in the wattlebird has been constrained due to all of the above factors, but wattlebirds are likely to have had enough time to learn to recognize adult koels as a nest threat, especially in Sydney where koel parasitism is higher (24%) than in Canberra (4%, Abernathy and Langmore, 2017). To determine whether wattlebirds exhibit mobbing defenses against adult koels, we exposed nesting pairs to freeze-dried mounts of a harmless species (Crimson Rosella, Platycercus elegans), a nest predator (Pied Currawong, Strepera graculina), and a female koel and quantified the wattlebirds’ behavioral responses toward each mount. Due to the higher parasitism rate in Sydney, we predicted that wattlebirds would be more aggressive and more likely to attack koel mounts in Sydney than in Canberra. Further, we predicted that wattlebirds would exhibit higher aggression toward koel mounts than currawong and rosella mounts in Sydney, but should show similar levels of aggression toward both koels and currawongs in Canberra. Wattlebirds should be able to recognize koels as a nest threat, but may not view them differently from a nest predator, as successful parasitism results in a complete loss of host young. Additionally, koels may depredate nests late in incubation or in the nestling phase in order to force the host to re-lay, as other brood parasites have been shown to do (Elliott, 1999; Davies, 2000; Granfors et al., 2001), though we have no direct evidence of Pacific Koels predating nests. Thus, this type of test was used to determine if wattlebirds viewed koels as a nest threat, but was insufficient to determine whether or not wattlebirds understand the kind of threat koels pose to their nests (brood parasitism, rather than nest predation). As some brood parasite hosts have been shown to exhibit passive nest defense (sitting longer on the nest to prevent the parasite from laying), we also tested wattlebirds for this behavior (Gill and Sealy, 2004; Canestrari et al., 2009; Medina and Langmore, 2016).
We used model eggs to determine the level of egg ejection ability in wattlebirds and compared this to the level of egg ejection in the two old hosts, the Magpie-lark and Noisy Friarbird. Not only did the comparison of the old hosts to the recent host aid us in understanding if our model eggs were useful indicators of egg ejection ability, but it also allowed us to better understand whether koels have evolved egg mimicry with the Noisy Friarbird and/or Red Wattlebird. We presented hosts with either a model egg that appeared somewhat similar to the hosts’ eggs (similar spotting pattern and similar ground color) or strikingly different (no spotting and dissimilar, blue ground color). Two different experimental egg morphs were used because discrimination abilities may vary according to the sensory perception of particular species (Lahti, 2015) and we wanted to test if hosts could eject both extremely non-mimetic and somewhat mimetic model eggs. If the evolution of egg ejection has been constrained in the Red Wattlebird, we predicted that the two old hosts would exhibit egg ejection at higher rates than wattlebirds even though they are rarely parasitized in the areas where we conducted our study. This is because many studies show that egg ejection, once evolved, is maintained in the absence of parasitism for most hosts (e.g., Avilés, 2004; Peer et al., 2005; Soler, 2014; Yang et al., 2014). We also predicted that the old hosts would eject the blue eggs at a higher rate than the spotted model eggs because the blue eggs should be easier to distinguish from the host eggs than the spotted model eggs.
Materials and Methods
Study Species and Sites
We tested the level of mobbing defenses in Red Wattlebirds and the level of egg ejection defenses in Red Wattlebirds, Magpie-larks and Noisy Friarbirds at two sites, Western Sydney and Canberra. At the time of this study (2016) in Sydney, koels had been parasitizing wattlebirds for 38–86 years with a current parasitism rate of 24%, while in Canberra wattlebirds had been a host of the koel for 8–33 years with a current parasitism rate of only 4% (Abernathy and Langmore, 2017). Abernathy and Langmore (2017) found no cases of parasitism of the Magpie-lark or Noisy Friarbird in Western Sydney or in Canberra. However, there has been one anecdotal report of a Noisy Friarbird raising a koel nestling in Canberra in 2014 (Abernathy and Langmore, 2016). Though no formal studies have been conducted on koel parasitism rates of the two old hosts, Brooker and Brooker (2005) found 20 records of koel parasitism of the Magpie-lark on the east coast of Australia from 1909–2008 and 29 records of koel parasitism of the Noisy Friarbird on the east coast from 1893–2002.
All experiments were conducted during the breeding season. The mobbing experiments on Red Wattlebirds were performed from September–October 2015 in Western Sydney and October–November 2015 in Canberra (Table 1). For the egg ejection experiments we searched for Red Wattlebird, Magpie-lark, and Noisy Friarbird nests at several different sites in Canberra from August 2013–January 2014 and August 2015–December 2016, and in Western Sydney from August 2014–January 2015 and August 2015–December 2016 (Table 1). In both Canberra and Sydney, wattlebird and Magpie-lark nests were mostly found in residential areas that included a wetland feature, while friarbird nests were only found in high numbers in nature parks or rural areas with native habitat. Koel parasitism was only found in residential areas or urban parks in both Western Sydney and Canberra and wattlebirds were the only host species for which parasitism was detected at our study sites (Abernathy and Langmore, 2016, 2017). For more information on the koel-wattlebird study system, a map of the study sites and evidence of duration of sympatry between wattlebirds and koels in Sydney and Canberra, see Abernathy and Langmore (2017). In addition to testing these three host species, we informally tested the egg ejection ability of a minor host, the Olive-backed Oriole (Oriolus sagittatus; Brooker and Brooker, 1989), as we occasionally found them nesting near Noisy Friarbirds and their egg ejection capability was unknown. Due to low sample sizes, we only tested orioles with the non-mimetic blue egg and we did not include these nests in any of our statistical analyses.
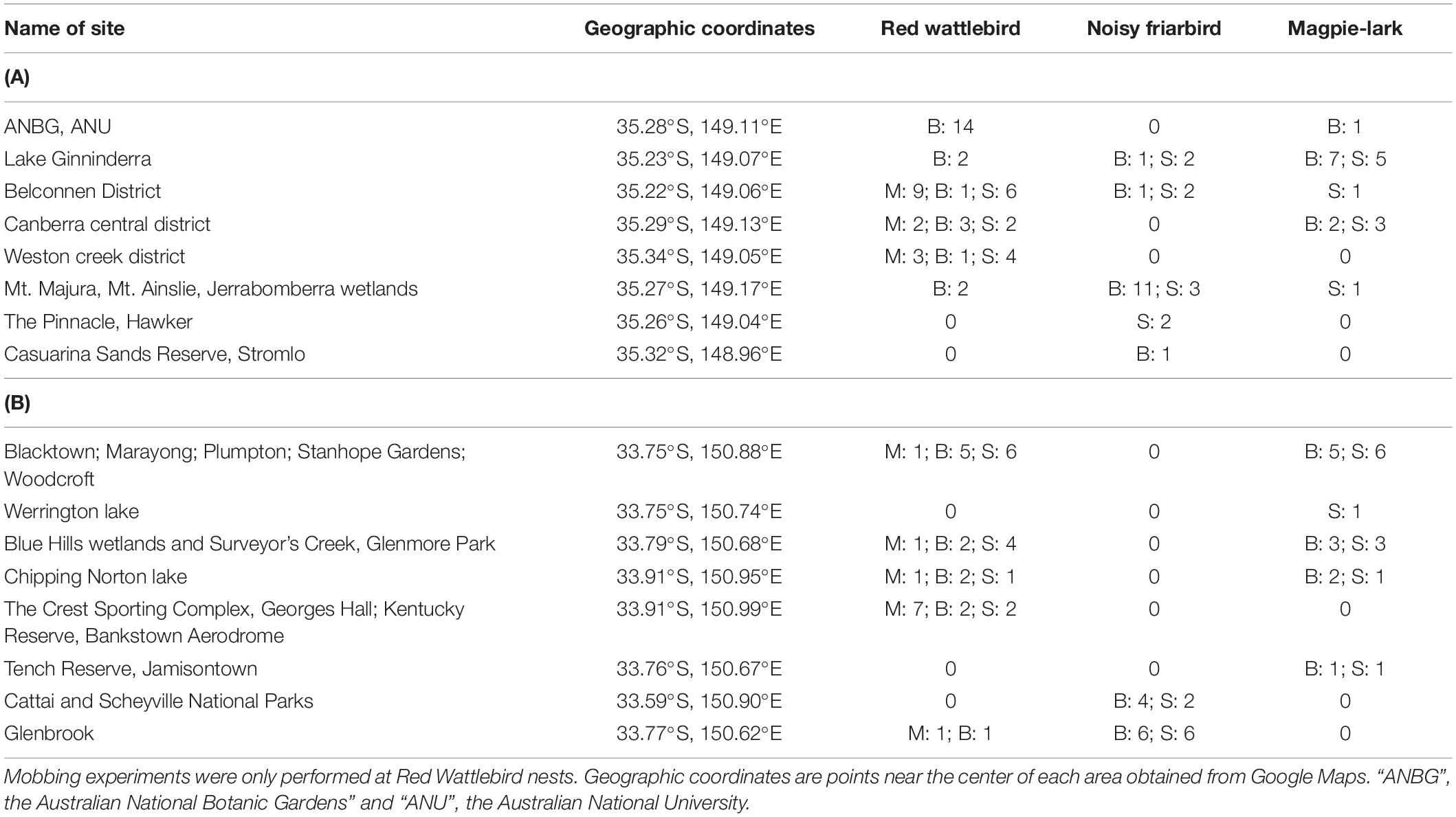
Table 1. Location and number of mobbing and egg ejection experiments performed for each host and egg treatment (“M” = mobbing experiment, “B” = blue egg ejection experiment, “S” = spotted egg ejection experiment) in (A) Canberra, ACT and (B) Western Sydney, NSW.
Mobbing Experiments
We compared the aggressive response of wattlebird pairs in the incubation stage of breeding to mounted specimens of a female koel, a common nest predator (Pied Currawong) and a common, harmless parrot (Crimson Rosella). Each of these species have vastly different plumages, making them easy to distinguish, but similar sizes. Crimson Rosellas are around 35–38 cm long and have mainly crimson and blue-colored plumage (Higgins, 1999). Pied Currawongs are slightly larger, about 48 cm long on average, and have mostly black plumage with some white patches (Higgins et al., 2006). Female koels are around 41 cm long and have a dark cap, off-white to buffy front with dark horizontal barring and a dark brown back with white spotting (Higgins, 1999). We used two rosella and currawong mounts, alternating them for each nest, but only had one available female koel mount. Mounts were placed 2–2.5 m from the nest. For low nests (1.5–3 m high), mounts were fastened to the top of a 2.3 m ladder. For higher nests (4–10 m), mounts were hauled up to the appropriate height using a rope hanging over a nearby branch. While this difference in mount presentation has the potential to cause a response bias, we found no effect on aggressive response based on how the mount was positioned (see section “Results”). Mounts were placed in a mesh cage to protect them from damage by the wattlebirds. Observations were made from a blind or car placed four or more meters from the base of the nest tree.
When possible, each pair was presented with all three mounts and mounts were presented in random order to control for order-effects. We only performed two trials per day at a nest to reduce the amount of disturbance and we waited at least 2 h between trials to reduce the chance of carry-over aggression. Before starting the trial, the wattlebirds were allowed at least 30 min to habituate to the cage and hide setup before the mount was placed in the cage. In all but two trials, if the female was on the nest when the mount was placed in the cage, we flushed her off the nest. Trials commenced when the male or female came within 2 m of the mount and continued for 5 min. Observations of the pair’s response to the mount and any vocalizations given by the pair were recorded into a Tascam DR-07 or DR-05 recorder and microphone. We estimated the distances (in meters) of both members of the pair from the mount each time they moved. We counted the number of times they swooped or attacked (physical contact with the cage), the number and type of vocalizations given and we noted whether the female was on the nest.
Male and female wattlebirds are distinguishable in the field by their vocalizations; females give the more musical whistle, while males give harsh clucks and cackles (Higgins et al., 2001, Abernathy personal observation); females give the more musical whistle, while males give harsh clucks and cackles (Higgins et al., 2001). Higgins et al. (2001) mention that males may incubate, but in our study whenever a bird flushed off the nest and vocalized, it gave whistles and never clucks or cackles. Therefore, a bird on the nest was assumed to be a female. For most trials it was possible to distinguish male from female based on these characteristics. We classified wattlebird calls into seven different types by ear and by visual inspection of structural differences in spectrograms generated using Audacity 2.0.5 and Raven Pro 1.4 (alarm calls, growls, cackles, clucks, whistles, contact calls, and single note calls; Higgins et al., 2001). We used Audacity to score the number of each vocalization type. We excluded vocalizations if they were given by a bird not in our view or far away (8–10 m away) or if the bird was clearly responding to another bird.
Egg Ejection Experiments
Studies have shown that many brood parasite hosts use a combination of cues to recognize brood parasite eggs, including ground color, pattern, luminance and size (e.g., Rothstein, 1982; Marchetti, 2000; Spottiswoode and Stevens, 2010; de la Colina et al., 2012). Therefore, to determine how well hosts could discriminate between their eggs and a foreign egg, we created two types of model eggs: a dark blue immaculate egg that differed in two parameters, pattern and ground color, and a spotted egg that was painted to resemble host eggs in pattern and ground color, at least according to the human eye (Longmore, 1991, Figure 1 and see Supplementary Materials). We chose not to test egg size as a variable, so all model eggs came from molds of the same size and were a similar size to all host eggs (see Supplementary Materials).
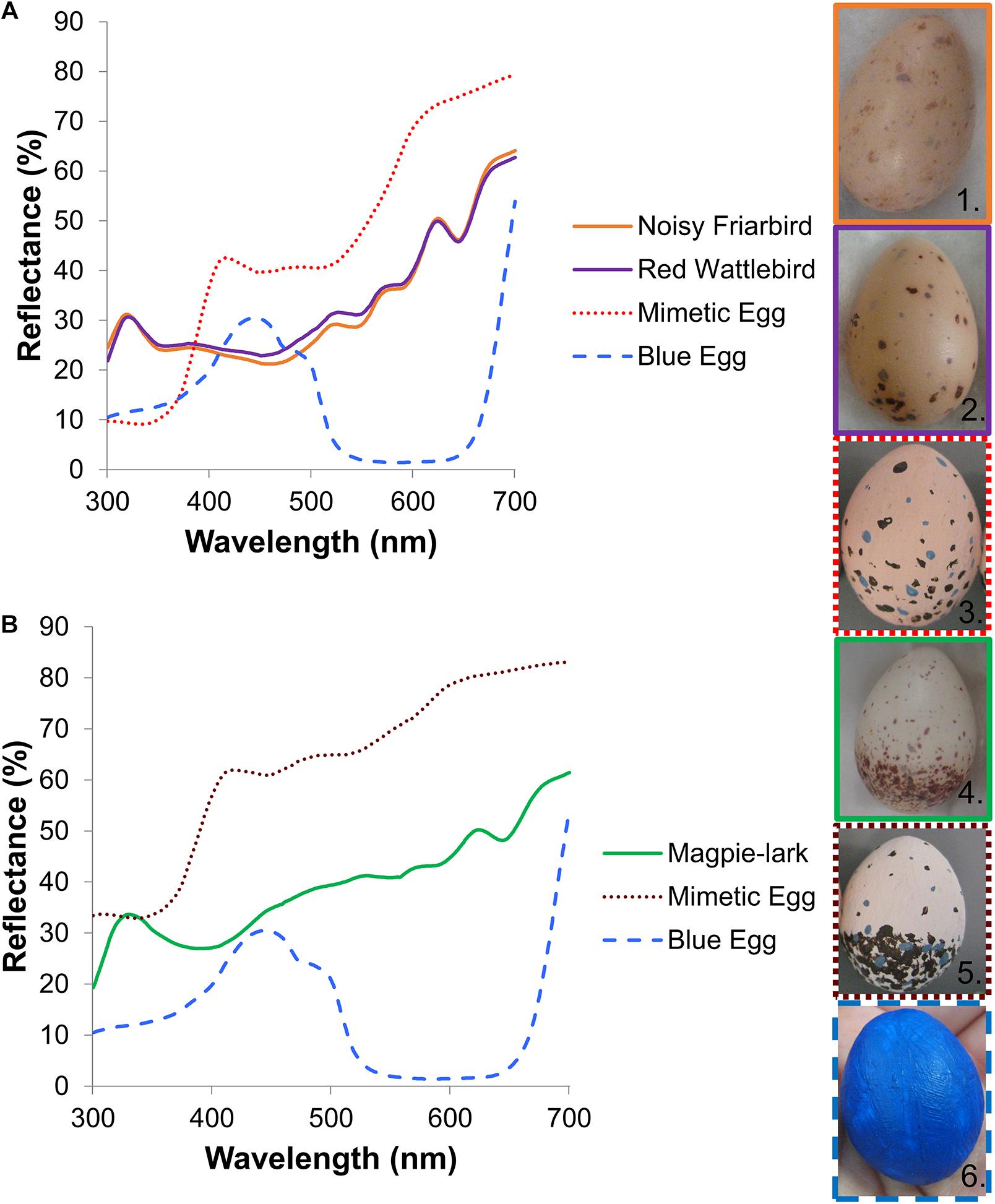
Figure 1. (A) Average spectral reflectance of fresh Noisy Friarbird (n = 13) and Red Wattlebird (n = 45) clutches and their corresponding spotted model eggs (n = 37) and blue eggs (n = 10). (B) Average spectral reflectance of fresh Magpie-lark clutches (n = 15), Magpie-lark spotted model eggs (n = 21) and blue eggs (n = 10). Images 1–6 are representative real host eggs and model eggs used in the egg ejection experiments: 1, Noisy Friarbird; 2, Red Wattlebird; 3, Wattlebird/friarbird spotted model; 4, Magpie-lark; 5, Magpie-lark spotted model; 6, Blue model. All photographs taken by V. E. Abernathy.
One of these egg types was placed into a host nest during the laying or incubation periods and the nest was monitored to determine if and when the host ejected the model egg. Nests were typically checked every 1–2 days. For high nests we used an extendable mirror pole to check contents. If nests were accessible with a ladder or by climbing the tree, model eggs were typically placed in the nest by hand. For nests that were inaccessible, we created a device to deposit the egg in the nest using an extendable mirror pole (see Supplementary Figure 1). Even though koels typically remove one host egg during parasitism (Brooker and Brooker, 1989), we did not remove host eggs from the nest during experimental parasitism, as this has not been found to alter host responses in other studies (Davies and Brooke, 1988; Moksnes and Røskaft, 1989).
In our study, 89% of ejections took place within the first 5 days of the experiment. Therefore, an egg was considered accepted if it remained in an active nest (eggs were warm or bird was observed incubating the clutch or defending the nest) for at least 5 days, though we continued checking after this point for 89% of nests to verify the egg was not ejected after this period (see also Rothstein, 1975b). An egg was considered ejected if the model egg was removed and the nest was clearly still active upon discovery of the ejection event. Of the nests where ejection took place, 75% of nests were checked again at least 1 day after ejection and found to still be active, which suggests the model egg was not taken by a predator. Wattlebird nests that were naturally parasitized during the first 5 days of the experiment were not included in the analyses. There were never any naturally parasitized Magpie-lark or Noisy Friarbird nests. We avoided any potential re-nests or territories of breeding pairs that had already been tested successfully in that particular breeding season or in a previous breeding season to minimize the risk of pseudo-replication. How territories in this study were estimated is explained in a previous study (Abernathy and Langmore, 2017, see “Estimating territories” in the “Materials and Methods” section). It was not possible to record data blind because our study involved focal animals in the field.
In our study, we did not include abandoned nests as rejection events for two reasons. First, all the hosts in our study are large (85–122 g; Higgins et al., 2001, 2006) and were capable of grasp-ejecting a foreign egg (see results in Rohwer and Spaw, 1988). Second, a previous study found nest abandonment by wattlebirds was only related to general nest disturbance by researchers and not to the presence of a koel egg in the nest or the study site (Abernathy and Langmore, 2017).
Model Eggs
Model eggs were made using a two-part silicone mold and polyurethane resin. After removal from the mold, eggs were smoothed with sand paper and painted with acrylic paint. Spotted eggs had a pinkish ground color with dark reddish-brown and violet-gray spots and were meant to appear similar to the host’s own eggs (Figure 1 and see Supplementary Materials). Even though koel eggs do appear similar to most of their host eggs in color, luminance and spotting, and there is no evidence they exhibit host egg races, koel eggs are significantly more similar in egg pattern and are closer in ground color to Noisy Friarbird and Red Wattlebird eggs than to Magpie-lark eggs (Abernathy et al., 2017). These differences in spotting pattern and ground color could create confounding variables, making our model eggs easier to distinguish for Magpie-larks than for the other hosts. So instead of making model eggs that resembled koel eggs, we attempted to create model eggs that mimicked each host species’ eggs to reduce this potential issue. Spots were created using the pointed end of a plastic dental floss pick, though it was difficult to create the smallest size of spots that occur naturally on the host eggs. Noisy Friarbird and Red Wattlebird eggs are very similar in ground color, luminance and egg pattern, with the majority of spotting at the larger end of the egg, while Magpie-larks typically have a lighter ground color and an obvious ring of spotting around the larger end of the egg (Longmore, 1991; Abernathy et al., 2017, Figure 1). Therefore, we created Magpie-lark spotted model eggs with a ring of spotting at the blunt end and with a lighter ground color (extra white paint mixed with pink) and for Noisy Friarbird and Red Wattlebird spotted model eggs, we created eggs without the distinct spotting ring and a darker ground color (Figure 1). Many passerine eggs have a spectral reflectance pattern with a peak in the UV range (e.g., Cherry and Bennett, 2001; Honza et al., 2007; Cassey et al., 2010; Abernathy and Peer, 2015), including host eggs in this study (Figure 1). In an effort to create more realistic spotted model eggs, we mixed white ultraviolet-reflecting paint (ReelWings) with pink acrylic paint to make the ground color. While this did create a UV-reflecting egg, the spectral pattern of the spotted model eggs did not perfectly match that of a real egg, as there was no peak in the UV range (Figure 1). However, the hosts are presumed to have VS rather than UVS opsins, which will have very low sensitivity to these ∼320 nm peaks (Ödeen and Håstad, 2010; Ödeen et al., 2011). To determine how similar our eggs appeared to host eggs from the host’s visual perspective, we took objective measurements in size, color, luminance and pattern for a subset of fresh host eggs and each model egg type (see Supplementary Materials). While our spotted model eggs did not mimic host eggs perfectly, their ground color was very similar from a bird’s visual perspective and their size and all pattern measurements did fall within the natural range found for each species (see Supplementary Tables 1, 2 and Supplementary Figure 2).
Statistical Analysis
Mobbing Experiments
In order to measure aggressive response during mobbing trials, we used three variables: the proportion of the trial that at least one parent was less than 2 m from the mount, vocalization rate and swoop or attack rate. We suspected that certain vocalizations, such as the alarm call, would be more important in predicting mount type than other vocalizations. However, vocalization function in Red Wattlebirds is poorly understood. Therefore, we first determined which vocalizations were important predictors of mount type by performing restricted maximum likelihood model (REML) analysis for each vocalization. We used the number of calls given during a trial for a particular call type as the response variable, the nest ID as the random effect, and the mount type as the independent variable. We were not able to analyze the growl call type because it was only given during one trial by one pair. These analyses indicated that only alarm calls were significant predictors of mount type (Table 2 and Figure 2; see more in section “Results”). Based on this result, we only used the number of alarm calls given when calculating vocalization rate in our aggressive response analysis.
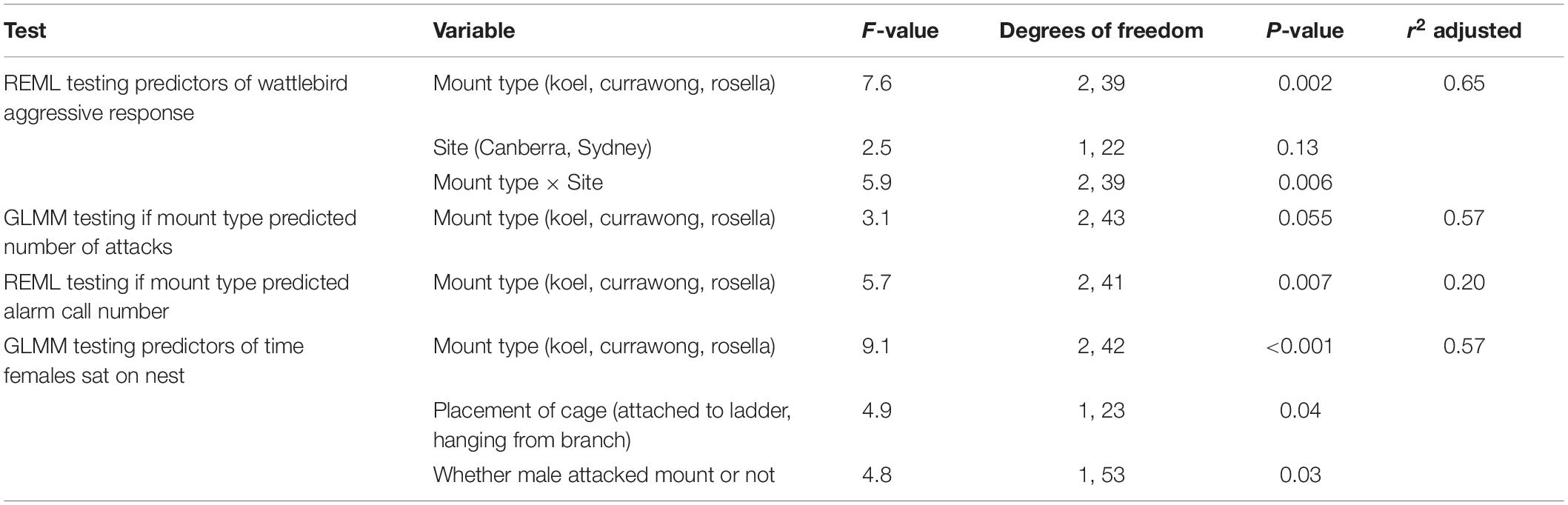
Table 2. Results from the best REML testing what predicted wattlebird aggressive response, the GLMM testing if mount type predicted number of attacks, the REML testing if alarm call number was predicted by mount type, and the GLMM testing if females exhibited passive defense during mobbing experiments.
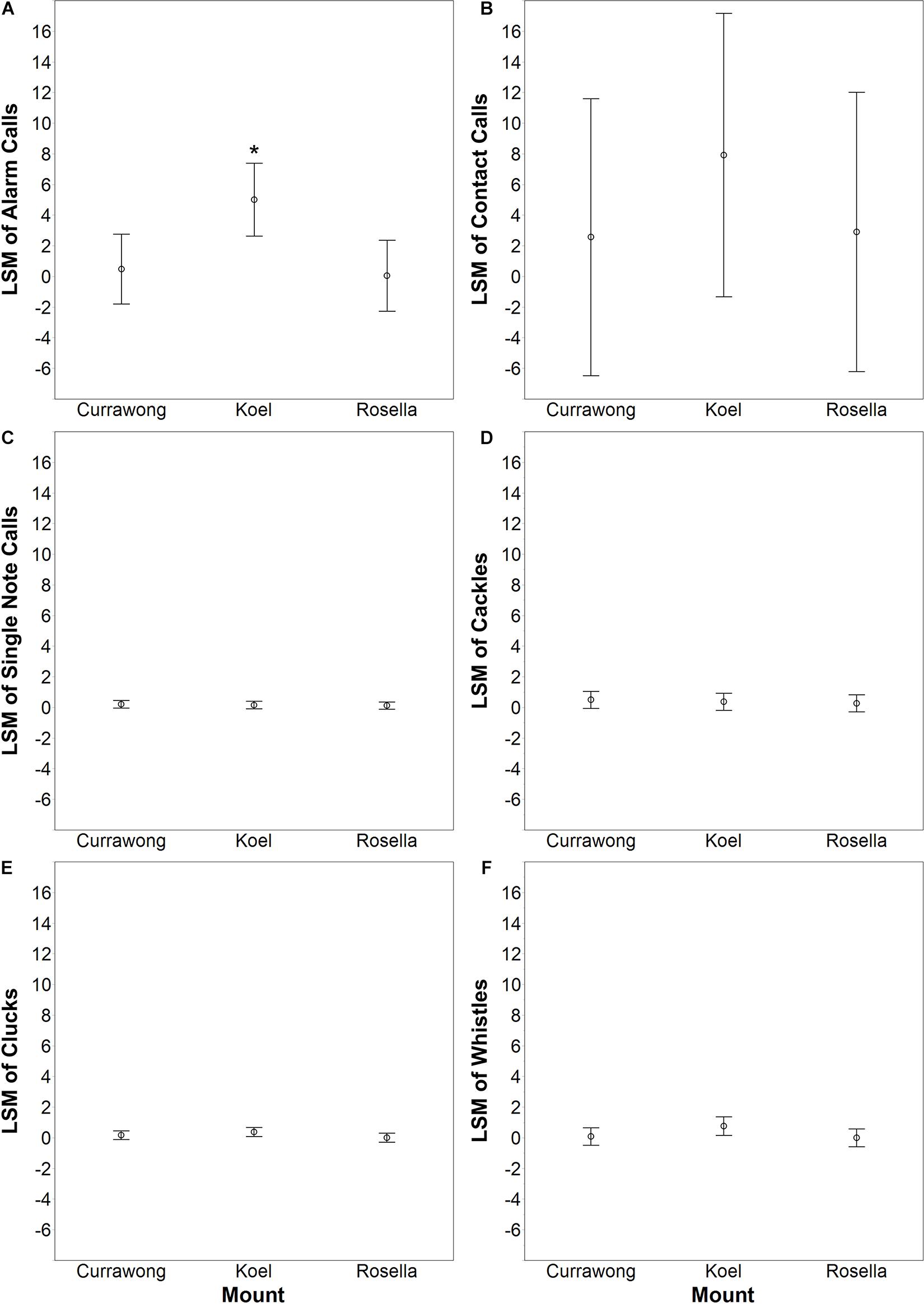
Figure 2. The least square means (LSM) ± 95% CI from six (A–F) REMLs where the number of calls given for each call type during a trial was the response variable, nest ID was the random effect and the type of mount (koel, currawong and rosella) was the independent variable. An asterisk indicates a significant difference between treatments (Tukey HSD, P < 0.05).
To determine if females used passive defense to protect their nests from koels we measured the proportion of time females sat on the nest during the trial. Females were present during every trial, while males were only present for some trials. In addition, sometimes one member of the pair was present for only part of a trial and distinguishing male from female for the entire duration of every trial was not always possible as the birds did not have colored bands. Therefore, to calculate rates of alarm calling and swooping, we first calculated the total minutes of observation of the two focal birds (e.g., 10 min if both members of the pair were present for the entire trial, 5 min if only one member of the pair was present for the entire trial), and then calculated the number of alarm calls or swoops given over that time period. To be counted as present (aware of the mount) once the trial had begun, the focal bird must have either landed within 5 m of the mount or have clearly shown aggression toward the mount.
In order to test if differences existed in the aggressive responses of wattlebirds based on mount type and other independent variables, we first combined the aggressive response variables using a Principle Components Analysis (PCA) to obtain a single aggressive response score. We checked for high correlation among our three aggressive response variables and found that none had a correlation value above 0.55. The PC1 was the only component with an Eigenvalue above one (1.998) and it explained 67% of the variation.
We treated the PC1 for each trial as our aggressive response score and used this as our response variable in another REML with nest ID as a random effect. Independent variables included mount (koel, currawong, and rosella), the site where the experiment took place (Canberra, Sydney), the Julian date of the trial, whether the cage was hanging from a branch or attached to the ladder and the interaction between site and mount. To determine if pairs physically attacked or swooped based on mount type or if they were equally likely to attack all mounts, we ran a binomial GLMM with a logit link function with nest ID as the random effect and whether the pair attacked (1) or not (0) as the response variable. We used all the same independent variables as in the REML, but were not able to include any interactions due to the few times pairs actually attacked a mount. We also used a Fisher’s Exact Test to compare the percentage of pairs that attacked or swooped koels at each site. This test was done in the R Statistical Package (R Core Team, 2014). To determine if females sat on the nest for longer in the presence of a koel mount, we ran a binomial GLMM with a logit link function with nest ID as the random effect and the proportion of time a female sat out of the total time she was present as the response variable. We used the same independent variables in this analysis as in the REML, and also included whether the male attacked or not.
We performed the PCA, REMLs, and GLMMs in JMP Pro 14.3.0. For all statistical models, the most non-significant terms were removed sequentially starting with the interactions until only significant or near-significant terms remained. We used an alpha of 0.05 and the model with the lowest AIC score was chosen. Least square means (LSM) ± 95% confidence intervals are reported for the REMLs and GLMMs.
Egg Ejection Experiments
We used a binomial generalized linear model (GLM) with a logit link function to determine which variables predicted ejection response (1 = ejection, 0 = acceptance). Five of the independent variables in the full model were host species (Red Wattlebird, Magpie-lark or Noisy Friarbird), egg type (blue or spotted), the site where the experiment took place (Canberra or Sydney), the breeding season year (first or second) and laying date based on a Julian calendar. If the actual laying date for a nest was unknown, we estimated laying date by counting back from hatch day (incubation is typically 16 days for wattlebirds and friarbirds, and 18 days for Magpie-larks; Higgins et al., 2001, 2006). We assumed wattlebirds and friarbirds always began incubating after laying the last egg in a clutch and Magpie-larks always began incubating immediately after laying the first egg (Higgins et al., 2001, 2006). For some nests, hatch day or laying date could only be estimated to a range of dates (e.g., August 02–04). In these cases, we estimated laying date by taking the median value of the range and only if the range was from 2 to 3 days.
A final variable in the full model was the number of days left until the clutch would be completed when the model egg was added to the nest (“days until clutch completion”). This variable is similar to the commonly used variable nesting phase (laying or incubating), but it is more precise, breaking down the phases by days. This variable is important in understanding ejection decisions because a host may be less likely to eject an egg if it is placed in the nest at the beginning of the laying period before it has seen its entire clutch, especially if the host is a first-time breeder (Lotem et al., 1995; Rodríguez-Gironés and Lotem, 1999, but see Soler et al., 2013). Days until clutch completion also controls for varying clutch sizes, as a host that lays fewer eggs may be more likely to eject than a host with a larger clutch simply because there is a higher chance of placing the model egg in the nest after the clutch has been completed. We did not include clutch size as an independent variable because each host species tended to lay different clutch sizes. In our study, wattlebirds typically laid 1–2 eggs, friarbirds 2–3, and Magpie-larks 3–4. Therefore, adding the variable “clutch size” might have confounded our results, as clutch size also related to host species.
Laying date was unknown or unable to be accurately estimated for 36 nests. Therefore, we first ran the GLM only including nests where laying date was known (n = 107 nests). However, this variable was not significant or near-significant independently or in interactions. We ran the GLM again, excluding the laying date variable and adding in the remaining 36 nests (n = 143 nests) and only report results for this second GLM.
Generalized linear models were performed using the rms (Harrell, 2016) and multcomp packages (Hothorn et al., 2008) in the R Statistical Package (R Core Team, 2014) with alpha < 0.05. GLMs were run including all terms and interactions between host and every other term, except for the breeding season year because in Canberra, Magpie-larks were only tested in the first year of the study. The model with the lowest AIC score was chosen, which always only included significant or near-significant variables.
Ethics Statement
This project was approved by and conducted in accordance with the Australian National University Animal Experimentation Ethics Committee: protocol number A2013/20. Permits from the Territory and Municipal Services of the ACT (license number: LT2013678) and the Office of Environment and Heritage of the NSW National Parks and Wildlife Service (license number: SL101349) were obtained to conduct scientific experiments in Canberra and Western Sydney.
Results
Do Wattlebirds Recognize Koels as a Threat?
Overall, we tested the behavioral response of 11 wattlebird breeding pairs in Sydney and 12 pairs in Canberra to the mounts, but two koel trials and one rosella trial conducted on three different pairs were not successfully completed and so these were excluded from the statistical analyses. The best REML for predicting wattlebird aggressive response included mount, site and the interaction between mount type and site (Table 2). Overall, wattlebirds responded significantly more aggressively to the koel mount (n = 21 trials, LSM = 0.70 ± 0.55) than to the currawong mount (n = 23 trials, LSM = −0.07 ± 0.53; Tukey HSD: P = 0.03) and the rosella mount (n = 22 trials, LSM = −0.45 ± 0.54; Tukey HSD: P = 0.001), but their response to currawong and rosella mounts was not significantly different (Tukey HSD: P = 0.40). Furthermore, the interaction between mount type and site was important, as wattlebirds in Sydney exhibited a significantly higher aggressive response toward koel mounts than any other group (Figure 3; Tukey HSD: P < 0.03 for all comparisons). More pairs attacked and/or swooped the koel mount in Sydney (60%, n = 10 pairs), than in Canberra (9%, n = 11 pairs; Fisher’s Exact Test, P = 0.02). The type of mount was a borderline significant predictor of whether a pair actually physically attacked the cage containing the mount (Table 2), with the koel mount eliciting a significantly higher number of attacks (LSM = −0.97 ± 1.38) than the rosella mounts (LSM = −2.91 ± 1.62; Tukey HSD, P = 0.045), but not the currawong mounts (LSM = −1.46 ± 1.38; Tukey HSD, P = 0.72). The number of attacks on currawongs was not significantly different from the number of attacks on rosellas (Tukey HSD, P = 0.16).
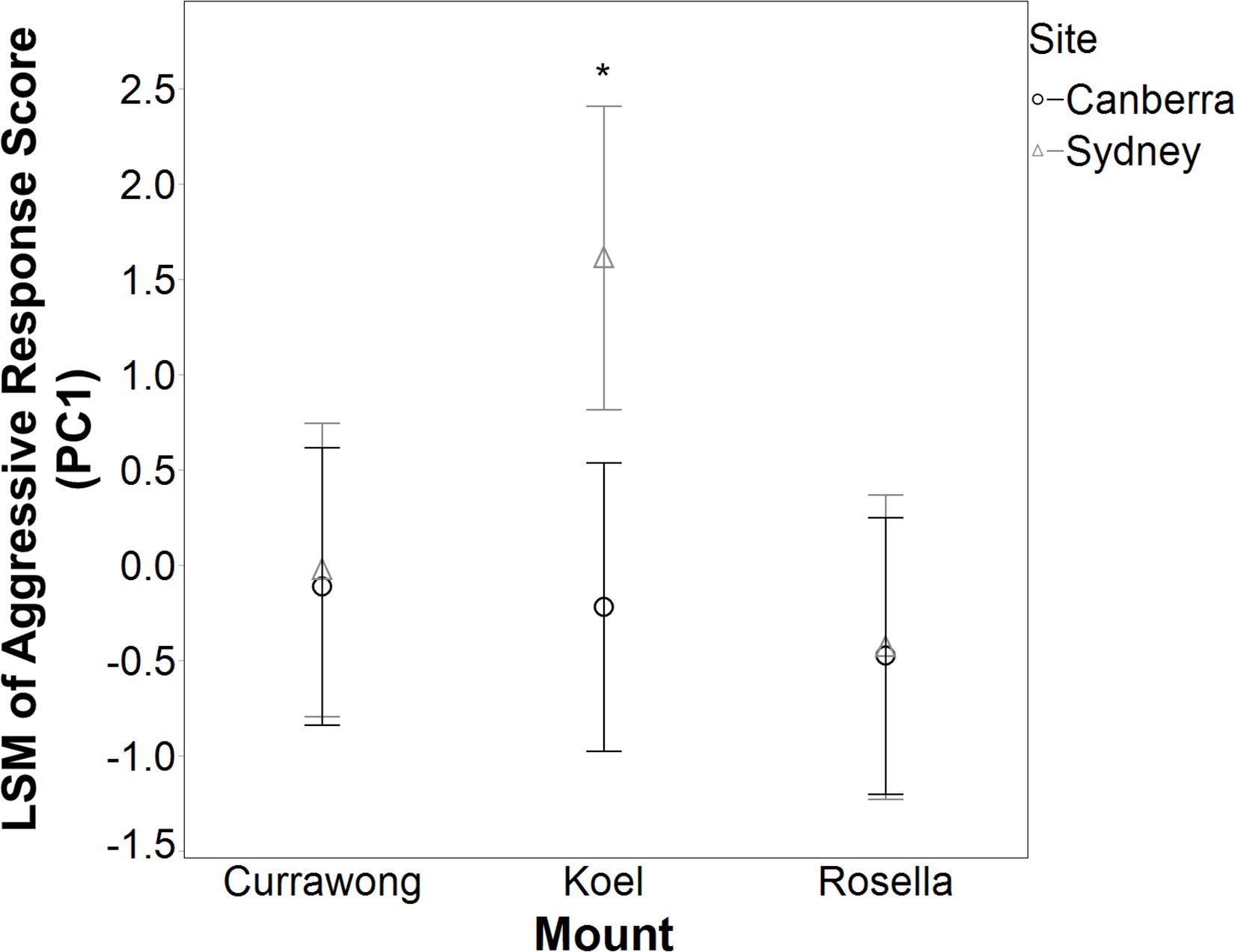
Figure 3. Least square means (LSM) ± 95% CI of the Red Wattlebird aggressive response score (PC1) for each mount type in Canberra and Western Sydney. Wattlebirds in Sydney showed a significantly higher aggressive response score toward koels than any other group, as indicated by the asterisk (REML, Tukey HSD, P < 0.03 for all comparisons).
Wattlebirds produced significantly more alarm calls in the presence of the koel (LSM = 5.01 ± 2.38) than the currawong (LSM = 0.48 ± 2.27; REML, Tukey HSD: P = 0.02) and the rosella mounts (LSM = 0.05 ± 2.33; REML, Tukey HSD: P = 0.01) and this was the only call type that was significantly predicted by mount type (Table 2 and Figure 2). There was no evidence of a cuckoo-specific vocalization in wattlebirds (e.g., Langmore et al., 2012). Only one call type, the growl call, was given exclusively during the koel trial by one pair, but this call type was also given by multiple other pairs toward researchers checking nests (Abernathy personal observation).
The best GLMM model for predicting how long female wattlebirds sat on the nest included mount type, whether the cage was hanging or attached to the ladder and whether the male attacked the mount or not (Table 2). The results suggest that females do not appear to use passive nest defense against brood parasitism, as females sat for a significantly longer period in the presence of the rosella mounts (LSM = 1.85 ± 1.14) than in the presence of the koel mount (LSM = −0.24 ± 1.01; Tukey HSD, P = 0.001) or currawong mounts (LSM = −0.27 ± 0.95; Tukey HSD, P < 0.001). There was no difference in how long females sat in the presence of the koel or currawong mounts (Tukey HSD, P = 1.00). Females also sat for longer on the nest when the cage was attached to the ladder (LSM = 1.28 ± 1.38) than when it was hanging from a branch (LSM = −0.39 ± 0.88) and when the male was not attacking the mount (male not attacking: LSM = 1.20 ± 0.83; male attacking: LSM = −0.31 ± 1.32).
Factors Influencing Egg Ejection
The three host species differed in their response to the different egg types (best model: host × egg type: χ2 = 7.4, df = 2, P = 0.02), and this was the only interaction that was significant. Magpie-larks ejected a similar number of blue eggs (91%, n = 22) and spotted eggs (86%, n = 21; Tukey HSD: z = −0.2, P = 1.00; Figure 4). Friarbirds ejected a similar number of spotted eggs to Magpie-larks (94%, n = 17; Tukey HSD: z = 0.4, P = 1.00), but they ejected significantly fewer blue eggs (38%, n = 24; Tukey HSD: z = −3.6, P = 0.003). Wattlebirds were consistently poor egg ejecters, ejecting significantly fewer blue (3%, n = 35) and spotted eggs (4%, n = 25) than Magpie-larks (blue: Tukey HSD: z = −4.9, P < 0.001; spotted: z = −4.4, P < 0.001) and friarbirds (blue: Tukey HSD: z = −2.9, P = 0.045; spotted: z = −4.3, P < 0.001). When all hosts are combined, ejection was more likely to occur when the model egg was added closer to or after clutch completion (best model: Wald’s test: SE = 0.39, z = −2.0, P = 0.04). In addition, we successfully tested 7 Olive-backed Oriole nests using the non-mimetic blue model egg (3 in Canberra and 4 in Sydney). Orioles showed 100% ejection of the blue egg and all ejected in less than 4 days.
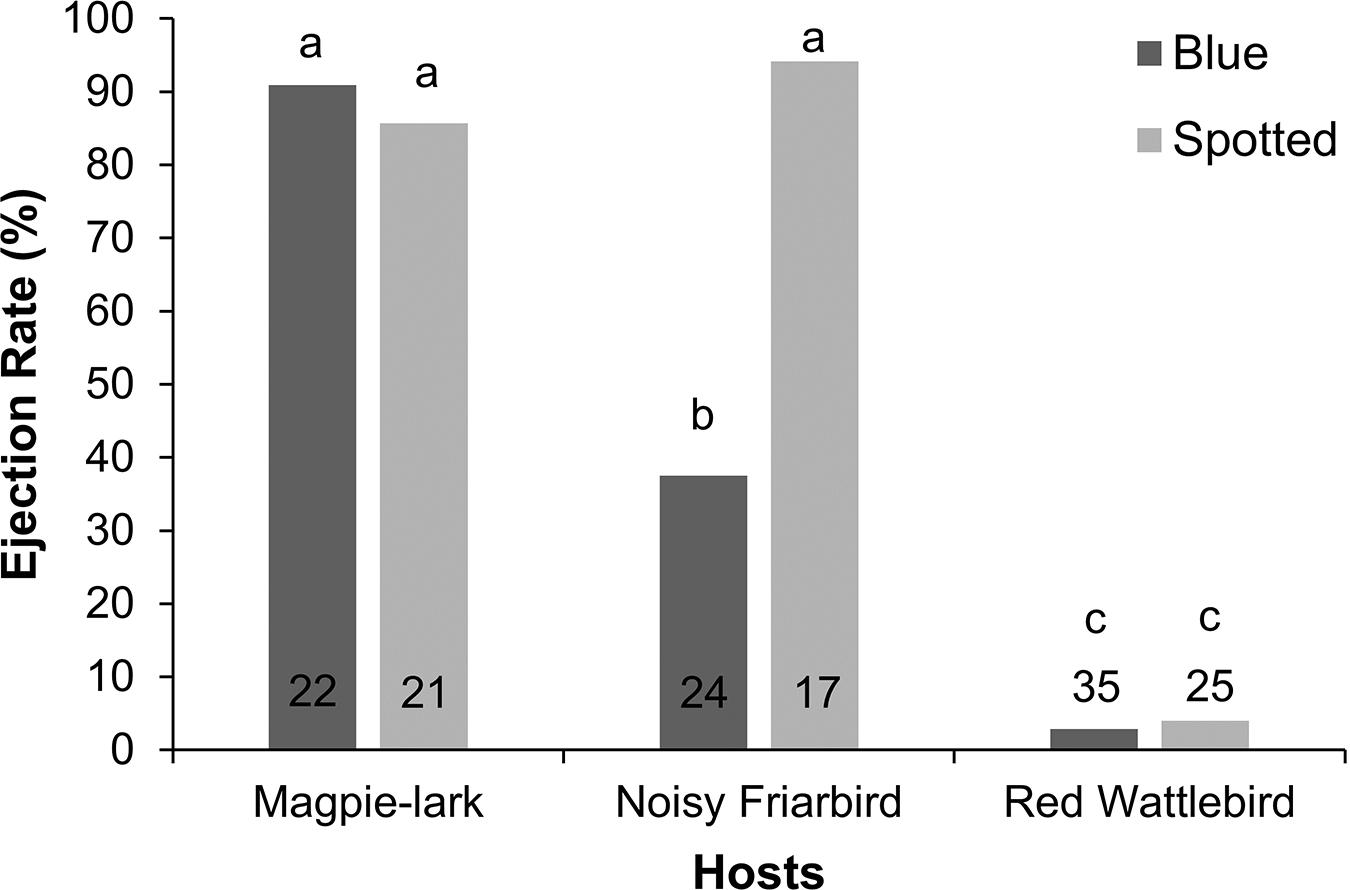
Figure 4. Ejection rates of three Pacific Koel hosts for two model egg treatments from 2013 to 2016 in Canberra, ACT and Western Sydney, NSW combined. Number of nests tested for each treatment are at the bottom of each column and letters indicate significant differences (GLM, Tukey HSD, P < 0.05).
Discussion
Mobbing Experiments
Wattlebirds recognized koels as a special nest threat, responding significantly more aggressively to the koel mount than to a harmless control and a nest predator, but their aggressive response did not differ between the harmless species and nest predator. Presentations of koel mounts elicited significantly more alarm calls than presentations of the other 2 mounts and this vocalization was the only one that was influenced by mount type. Wattlebirds were also more likely to physically attack koel mounts than rosella mounts. Furthermore, Sydney wattlebirds were more aggressive toward the koel, where parasitism rate is higher (24%) than in Canberra (4%) (Abernathy and Langmore, 2017). This might indicate that brood parasite recognition may take longer to spread throughout an entire population when parasitism rates are low. Overall, our results seem to suggest that wattlebirds in Sydney viewed the threat posed by koels as different from the threat posed by currawongs. Considering the extra energetic cost associated with raising a cuckoo nestling, these results do make sense and similar results have been found in other mount experiments where both an obligate brood parasite and nest predator mount were used (e.g., Gill and Sealy, 1996; Li et al., 2015; Noh et al., 2021).
Our results are consistent with a learned response because the behavior was acquired rapidly (within 38–86 years of exploitation by the parasite). Moreover, the lower aggressive response and attack rate in Canberra is consistent with learning because a learned response to a threat requires either personal experience of the threat or observation of other species or conspecifics responding to the threat (e.g., Feeney and Langmore, 2013), and this would be less common at the site with lower exposure to koels. Multiple studies have shown that animals can learn anti-predator behaviors toward novel predators over the course of their life through cultural transmission by watching how other individuals respond to those species (reviewed in Griffin et al., 2000; Davies and Welbergen, 2009; Feeney and Langmore, 2013). Anti-predator responses can be further generalized to other novel threatening species that are morphologically similar to the known predator, allowing individuals to respond quickly to potential threats from species they have not previously encountered (Griffin et al., 2001; Ferrari et al., 2007, 2009). The process of cultural transmission and the generalization of anti-predator responses could allow rapid acquisition of the ability to recognize and mob a brood parasite in a naïve host population. This process may be facilitated further by the resemblance of many brood parasitic cuckoos to hawks (Davies and Welbergen, 2008; Welbergen and Davies, 2011). The rapidity of the spread of this defense, however, is likely to be dependent upon how many individuals in the population are actually exposed to the threatening species and the perceived cost of engaging in an aggressive encounter with the threatening species (e.g., Forsman and Mönkkönen, 2001; Davies and Welbergen, 2008; Welbergen and Davies, 2008, 2011). While brood parasites are not a threat to their adult hosts, they will often remove or damage host eggs when laying their own (Sealy, 1992; Davies, 2000; Soler and Martínez, 2000; Gloag et al., 2013) and will sometimes depredate nests late in incubation or in the nestling phase in order to force the host to re-nest (Elliott, 1999; Davies, 2000; Granfors et al., 2001). Therefore, hosts may quickly learn that brood parasites are a threat to their nest and may even view them as nest predators (Mcleod, 1997). Interestingly, our results show wattlebirds may view koels as a greater threat to their nest than a common nest predator, the currawongs. A previous study conducted on wattlebirds and koels at our study sites indicated that predation rate of wattlebird nests does increase once koels have arrived to the breeding area (from 19%, n = 27 before koels arrive to 40%, n = 102 after koels arrive) (Abernathy and Langmore, 2017). This does not necessarily mean koels are the main predator of wattlebird nests, as the currawong is also nesting during this same time period, but it could mean wattlebirds are more vigilant during this time and more likely to mob koels if they view them as a greater threat to their nest.
Contrary to some other studies (Gill and Sealy, 2004; Canestrari et al., 2009; Medina and Langmore, 2016), we found no evidence that wattlebird females exhibit passive nest defense as they were more likely to sit on the nest in the presence of the harmless mount than in the presence of the koel and currawong mounts. They also seemed more disturbed and less likely to sit on the nest when the cage was hanging from a branch, possibly due to more movement of the cage, especially in windier conditions. And male behavior influenced how females responded to the mount, as they were less likely to sit if the male was attacking the mount, which was more likely to happen when the mount was either the koel or currawong.
Factors Influencing Egg Ejection
The two old primary hosts, the Noisy Friarbird and Magpie-lark, as well as the minor host, the Olive-backed Oriole, all ejected eggs at a higher rate than the recent host, the Red Wattlebird, demonstrating that the old hosts have retained egg ejection in the virtual absence of brood parasitism, while this defense has yet to spread throughout the wattlebird population. Indeed, wattlebird egg ejection rates did not differ significantly between Canberra (6%, a site with 8–33 years of parasitism) and Sydney (0%, a site with 38–86 years of parasitism) despite the longer length of sympatry between wattlebirds and koels in Sydney. Many other studies have found that host egg ejection is often maintained after parasitism has ceased (e.g., Avilés, 2004; Peer et al., 2005; Soler, 2014; Yang et al., 2014) and the adaptation may even be retained from a common ancestor after speciation events (Peer et al., 2013). This suggests that once egg ejection evolves and spreads throughout a population, it may pose little cost to the host to maintain it even in the absence of parasitism.
We did not find higher ejection rate of blue eggs compared to spotted model eggs in any of the host species. Surprisingly, friarbirds showed only intermediate ejection of blue model eggs, but ejected almost 100% of spotted model eggs. This could be a consequence of the extremely atypical appearance of the blue model eggs; friarbirds may have failed to associate them with the intended context (Lahti, 2015) and in some species egg recognition is specifically tuned to the natural gradient of eggshell colors such that artificial colors are not rejected in a predictable way (Hanley et al., 2017). Alternatively, friarbirds may have a pre-existing bias toward blue eggs, as several other studies have found that other brood parasite hosts tend to accept bluer eggs over browner eggs (Soler et al., 2012; Hanley et al., 2017; Abolins-Abols et al., 2019; Hanley et al., 2019; Manna et al., 2020). This suggests that, for Noisy Friarbirds, the spotted model eggs provided a better test of egg discrimination ability.
Hosts were more likely to eject model eggs that were added after their clutch was complete. This supports other findings that hosts are more likely to eject an odd egg once they have learned the appearance of their own eggs (Lotem et al., 1995; Rodríguez-Gironés and Lotem, 1999, but see Soler et al., 2013).
Defenses Available to a Recent Host
Even though wattlebirds are a fairly recent host and may be considered naïve to brood parasitism prior to being utilized by the koel as a host (Abernathy and Langmore, 2017), they were able to recognize koels as a nest threat and most pairs in Sydney (60%) exhibited a stronger aggressive response toward the koel than wattlebirds in Canberra. However, wattlebirds showed little to no egg ejection response to model eggs at either site and a previous study showed no evidence that wattlebirds reject naturally laid koel eggs or nestlings, unless the koel eggs are laid in the nest before the wattlebird has started laying (Abernathy and Langmore, 2017). This lack of egg ejection could be due to various factors constraining the evolution of this defense in the wattlebird, despite the high costs of parasitism. A previous study found that, while unparasitized wattlebird nests fledged significantly more young than parasitized wattlebird nests, fledging success rate of koels in parasitized wattlebird nests was relatively low (26%, n = 38) (Abernathy and Langmore, 2017). One reason was due to koels laying eggs at inopportune times (too early or too late to be successful), which may have been exacerbated by the fact that wattlebirds tend to have small clutch sizes compared to the older hosts (1–2 eggs, as opposed to 3–5 eggs) and their incubation period is similar to the koel’s, making it more difficult for the koel nestling to hatch out before the wattlebird nestling in order to evict it from the nest. The study also points out that at least 41% of wattlebird pairs in Sydney avoided parasitism of at least one of their nests by initiating nesting before koels arrived to the area. Thus, parasitism rate and the impacts of koel parasitism on wattlebird breeding success appear to be low (Abernathy and Langmore, 2017). This, coupled with the extreme similarity in egg appearance between wattlebird and koel eggs could make egg ejection more costly to evolve in wattlebirds (Davies et al., 1996; Abernathy et al., 2017). We found evidence that the koel’s egg phenotype may have evolved as a way to mimic the appearance of the Noisy Friarbird egg, but not likely the Red Wattlebird egg, as 94% of friarbirds ejected spotted model eggs, but only 4% of wattlebirds ejected this same egg type.
Conclusion
Our results indicate that 38–86 years of parasitism has been insufficient time for wattlebirds to evolve high egg ejection rates, possibly due to low parasitism rates (Abernathy and Langmore, 2017), the costs of egg recognition errors (Abernathy et al., 2017), and the utilization of generalized defenses that do not require evolution, such as learning the appearance of a koel and chasing it away from the nest (this study and Abernathy and Langmore, 2016) or abandoning nests that become parasitized before the host has started laying (Abernathy and Langmore, 2017). Our results combined with those from previous studies also indicate that naïve or recent hosts incapable of egg ejection are not completely defenseless. However, egg ejection is considered one of the most efficient defenses against parasitism and could reduce the risk of losing an entire breeding attempt. Thus, it would be interesting to conduct a similar study on wattlebirds after more time has passed to better determine how quickly a recent host population can evolve egg ejection. This knowledge could be helpful in a conservation setting, if a parasite switches to a naïve, endangered host.
Data Availability Statement
The datasets generated during the current study are deposited into the Dryad digital repository (An Experimental Test of Defenses in a Recent Host_Datasets; https://doi.org/10.5061/dryad.dfn2z351b).
Ethics Statement
The animal study was reviewed and approved by Australian National University Animal Experimentation Ethics Committee.
Author Contributions
VA, LJ, and NL conceived and designed the study. VA collected and analyzed the data with assistance from LJ, while NL supervised the research. LJ and NL designed the analysis for the mount experiment, while VA and NL designed the analysis for the egg ejection experiment. VA wrote the manuscript and NL contributed to revised versions and substantially edited the article. All authors contributed to the article and approved the submitted version.
Funding
This project was approved by and conducted in accordance with the Australian National University Animal Experimentation Ethics Committee: protocol number A2013/20. Permits from the Territory and Municipal Services of the ACT (license number: LT2013678) and of the Office of Environment and Heritage of the NSW National Parks and Wildlife Service (license number: SL101349) were obtained to conduct scientific experiments in Canberra and Western Sydney.
Conflict of Interest
The authors declare that the research was conducted in the absence of any commercial or financial relationships that could be construed as a potential conflict of interest.
Acknowledgments
We are grateful to the Sydney Museum for lending us two Crimson Rosella mounts for use in this study. We also acknowledge the tireless efforts of the field assistants on this project: M. Wright, who also assisted in designing the tube parasitizing method used in this study, L. McClean, who also assisted with the acquisition of the female koel mount used in this study, and A. Ye, R. Bigonneau, K. Leonard, and S. Levins. We are grateful to J. Adams who assisted with some work in the field and helped to design Supplementary Figure 1. We owe much thanks to the members of the Canberra Ornithologists’ Group and M. Fuller who aided in finding nests during the project, to S. Haslett with the Australian National University Statistical Consulting Unit, who assisted with the statistical analysis, and to Jolyon Troscianko, who assisted us in the use of his multispectral image calibration and analysis toolbox. We also thank the reviewers whose helpful comments improved the quality of this manuscript. This study is revised from two chapters in the Ph.D. thesis by VA published by the Australian National University in 2017.
Supplementary Material
The Supplementary Material for this article can be found online at: https://www.frontiersin.org/articles/10.3389/fevo.2021.651733/full#supplementary-material
References
Abernathy, V. E., and Langmore, N. E. (2016). Observations of the breeding behavior of the pacific koel in relation to its newest host. Canberra Bird Notes 41, 124–134.
Abernathy, V. E., and Langmore, N. E. (2017). The first stages of coevolution between a brood parasite and its new host: are naïve hosts defenceless? Emu 117, 114–129. doi: 10.1080/01584197.2017.1296330
Abernathy, V. E., and Peer, B. D. (2015). Mechanisms of egg recognition in brown-headed cowbird hosts: the role of ultraviolet reflectance. Anim. Behav. 109, 73–79. doi: 10.1016/j.anbehav.2015.08.006
Abernathy, V. E., Troscianko, J., and Langmore, N. E. (2017). Egg mimicry by the pacific koel: mimicry of one host facilitates exploitation of other hosts with similar egg types. J. Avian Biol. 48, 1414–1424. doi: 10.1111/jav.01530
Abolins-Abols, M., Hanley, D., Moskát, C., Grim, T., and Hauber, M. E. (2019). Anti-parasitic egg rejection by great reed warblers (Acrocephalus arundinaceus) tracks differences along an eggshell color gradient. Behav. Process. 166:103902. doi: 10.1016/j.beproc.2019.103902
Avilés, J. M. (2004). Egg rejection by Iberian azure-winged magpies Cyanopica cyanus in the absence of brood parasitism. J. Avian Biol. 35, 295–299. doi: 10.1111/j.0908-8857.2004.03327.x
Avilés, J. M., Stokke, B. G., Moksnes, A., Røskaft, E., Åsmul, M., and Møller, A. P. (2006). Rapid increase in cuckoo egg matching in a recently parasitized reed warbler population. J. Evol. Biol. 19, 1901–1910. doi: 10.1111/j.1420-9101.2006.01166.x
Brooker, L., and Brooker, M. (2005). Records of Parasitism by the Common Koel Eudynamys Scolopacea. Available online at: http://lesmikebrooker.com/KOELDB.HTM (accessed 9 April 2013).
Brooker, M. G., and Brooker, L. C. (1989). Cuckoo hosts in Australia. Aust. Zool. Rev. 2, 1–67. doi: 10.1093/beheco/10.1.1
Canestrari, D., Marcos, J. M., and Baglione, V. (2009). Cooperative breeding in carrion crows reduces the rate of brood parasitism by great spotted cuckoos. Anim. Behav. 77, 1337–1344. doi: 10.1016/j.anbehav.2009.02.009
Cassey, P., Portugal, S. J., Maurer, G., Ewen, J. G., Boulton, R. L., Hauber, M. E., et al. (2010). Variability in avian eggshell color: a comparative study of museum eggshells. PLoS One 5:e12054. doi: 10.1371/journal.pone.0012054
Cherry, M. I., and Bennett, A. T. D. (2001). Egg color matching in an African cuckoo, as revealed by ultraviolet-visible reflectance spectrophotometry. Proc. R. Soc. Lond. B 268, 565–571. doi: 10.1098/rspb.2000.1414
Curio, E., Ernst, U., and Vieth, W. (1978). The adaptive significance of avian mobbing II. Cultural transmission of enemy recognition in blackbirds: effectiveness and some constraints. Z. Tierpsychol. 48, 184–202. doi: 10.1111/j.1439-0310.1978.tb00255.x
Davies, N. B., and Brooke, M. L. (1988). Cuckoos versus reed warblers: adaptations and counteradaptations. Anim. Behav. 36, 262–284. doi: 10.1016/s0003-3472(88)80269-0
Davies, N. B., Brooke, M. L., and Kacelnik, A. (1996). Recognition errors and probability of parasitism determine whether reed warblers should accept or reject mimetic cuckoo eggs. Proc. R. Soc. Lond. B 263, 925–931. doi: 10.1098/rspb.1996.0137
Davies, N. B., and Welbergen, J. A. (2008). Cuckoo-hawk mimicry? An experimental test. Proc. R. Soc. Lond. B 275, 1817–1822. doi: 10.1098/rspb.2008.0331
Davies, N. B., and Welbergen, J. A. (2009). Social transmission of a host defence against cuckoo parasitism. Science 324, 1318–1320. doi: 10.1126/science.1172227
de la Colina, M. A., Pompilio, L., Hauber, M. E., Reboreda, J. C., and Mahler, B. (2012). Different recognition cues reveal the decision rules used for egg rejection by hosts of a variably mimetic avian brood parasite. Anim. Cogn. 15, 881–889. doi: 10.1007/s10071-012-0515-9
Dinets, V., Samaš, P., Croston, R., Grim, T., and Hauber, M. E. (2015). Predicting the responses of native birds to transoceanic invasions by avian brood parasites. J. Field Ornithol. 86, 244–251. doi: 10.1111/jofo.12111
Elliott, P. F. (1999). Killing of host nestlings by the brown-headed cowbird. J. Field Ornithol. 70, 55–57.
Feeney, W. E., and Langmore, N. E. (2013). Social learning of a brood parasite by its host. Biol. Lett. 9:20130443. doi: 10.1098/rsbl.2013.0443
Feeney, W. E., Medina, I., Somveille, M., Heinsohn, R., Hall, M. L., Mulder, R. A., et al. (2013). Brood parasitism and the evolution of cooperative breeding in birds. Science 342, 1506–1508.
Feeney, W. E., Welbergen, J. A., and Langmore, N. E. (2012). The frontline of avian brood parasite-host coevolution. Anim. Behav. 84, 3–12. doi: 10.1016/j.anbehav.2012.04.011
Ferrari, M. C., Brown, G. E., Messier, F., and Chivers, D. P. (2009). Threat-sensitive generalization of predator recognition by larval amphibians. Behav. Ecol. Sociobiol. 63, 1369–1375. doi: 10.1007/s00265-009-0779-5
Ferrari, M. C. O., Gonzalo, A., Messier, F., and Chivers, D. P. (2007). Generalization of learned predator recognition: an experimental test and framework for future studies. Proc. R. Soc. Lond. B 274, 1853–1859. doi: 10.1098/rspb.2007.0297
Forsman, J. T., and Mönkkönen, M. (2001). Responses by breeding birds to heterospecific song and mobbing call playbacks under varying predation risk. Anim. Behav. 62, 1067–1073. doi: 10.1006/anbe.2001.1856
Gill, S. A., and Sealy, S. G. (1996). Nest defence by yellow warblers: recognition of a brood parasite and an avian nest predator. Behaviour 133, 263–282. doi: 10.1163/156853996x00143
Gill, S. A., and Sealy, S. G. (2004). Functional reference in an alarm signal given during nest defence: seet calls of yellow warblers denote brood-parasitic brown-headed cowbirds. Behav. Ecol. Sociobiol. 56, 71–80. doi: 10.1007/s00265-003-0736-7
Gloag, R., Fiorini, V. D., Reboreda, J. C., and Kacelnik, A. (2013). The wages of violence: mobbing by mockingbirds as a frontline defence against brood-parasitic cowbirds. Anim. Behav. 86, 1023–1029. doi: 10.1016/j.anbehav.2013.09.007
Granfors, D. A., Pietz, P. J., and Joyal, L. A. (2001). Frequency of egg and nestling destruction by female brown-headed cowbirds at grassland nests. Auk 118, 765–769. doi: 10.1093/auk/118.3.765
Griffin, A. S., Blumstein, D. T., and Evans, C. S. (2000). Training captive-bred or translocated animals to avoid predators. Conserv. Biol. 14, 1317–1326. doi: 10.1046/j.1523-1739.2000.99326.x
Griffin, A. S., Evans, C. S., and Blumstein, D. T. (2001). Learning specificity in acquired predator recognition. Anim. Behav. 62, 577–589. doi: 10.1006/anbe.2001.1781
Hanley, D., Grim, T., Igic, B., Samas, P., López, A. V., Shawkey, M. D., et al. (2017). Egg discrimination along a gradient of natural variation in eggshell coloration. Proc. R. Soc. Lond. B 284:20162592. doi: 10.1098/rspb.2016.2592
Hanley, D., López, A. V., Fiorini, V. D., Reboreda, J. C., Grim, T., and Hauber, M. E. (2019). Variation in multicomponent recognition cues alters egg rejection decisions: a test of the optimal acceptance threshold hypothesis. Philos. Trans. R. Soc. of Lond. B 374:20180195. doi: 10.1098/rstb.2018.0195
Harrell, F. E. (2016). rms: Regression Modeling Strategies. R package version 4.5-0. Available online at: https://CRAN.R-project.org/package=rms.
Higgins, P. J. (ed.) (1999). “Handbook of Australian, New Zealand and Antarctic Birds,” in Parrots to Dollarbird, Vol. 4, (Melbourne, AU: Oxford University Press).
Higgins, P. J., Peter, J. M., and Cowling, S. J. (eds) (2006). “Handbook of Australian, New Zealand and Antarctic Birds,” in Boatbill to Starlings, Vol. 7, (Melbourne, AU: Oxford University Press).
Higgins, P. J., Peter, J. M., and Steele, W. K. (eds) (2001). “Handbook of Australian, New Zealand and Antarctic Birds,” in Tyrant-Flycatchers to Chats, Vol. 5, (Melbourne, AU: Oxford University Press).
Honza, M., Polačiková, L., and Procházka, P. (2007). Ultraviolet and green parts of the color spectrum affect egg rejection in the song thrush (Turdus philomelos). Biol. J. Linn. Soc. 92, 269–276. doi: 10.1111/j.1095-8312.2007.00848.x
Hothorn, T., Bretz, F., and Westfall, P. (2008). Simultaneous inference in general parametric models. Biom. J. 50, 346–363. doi: 10.1002/bimj.200810425
Jackson, M. V., and Kyne, P. M. (2010). Potential host species fatally attack female eastern koel Eudynamys orientalis, a brood parasite. Aust. Field Ornithol. 27, 133–136.
Kelly, C. (1987). A model to explore the rate of spread of mimicry and rejection in hypothetical populations of cuckoos and their hosts. J. Theor. Biol. 125, 283–299. doi: 10.1016/s0022-5193(87)80060-7
Lahti, D. C. (2015). The limits of artificial stimuli in behavioral research: the umwelt gamble. Ethology 120, 529–537. doi: 10.1111/eth.12361
Langmore, N. E., Feeney, W. E., Crowe-Riddell, J., Luan, H., Louwrens, K. M., and Cockburn, A. (2012). Learned recognition of brood parasitic cuckoos in the superb fairy-wren Malurus cyaneus. Behav. Ecol. 23, 798–805. doi: 10.1093/beheco/ars033
Li, D., Wei, H., Zhang, Z., Liang, W., and Stokke, B. G. (2015). Oriental reed warbler (Acrocephalus orientalis) nest defence behaviour towards brood parasites and nest predators. Behaviour 152, 1601–1621. doi: 10.1163/1568539x-00003295
Longmore, W. (1991). Honeyeaters and Their Allies of Australia. Auckland: William Collins Publishers Ltd, 403.
Lotem, A., Nakamura, H., and Zahavi, A. (1995). Constraints on egg discrimination and cuckoo-host co-evolution. Anim. Behav. 49, 1185–1209. doi: 10.1006/anbe.1995.0152
Manna, T. J., Hanley, D., Honza, M., Capek, M., Rutila, J., Samaš, P., et al. (2020). Fitting different visual models to behavioral patterns of parasitic egg rejection along a natural egg color gradient in a cavity-nesting host species. Vision Res. 167, 54–59. doi: 10.1016/j.visres.2019.12.007
Marchetti, K. (2000). Egg rejection in a passerine bird: size does matter. Anim. Behav. 59, 877–883. doi: 10.1006/anbe.1999.1388
Martínez, J. G., Molina-Morales, M., Precioso, M., and Avilés, J. M. (2020). Age-related brood parasitism and egg rejection in magpie hosts. Am. Nat. 195, 876–885. doi: 10.1086/708155
Martín-Gálvez, D., Soler, J. J., Martínez, J. G., Krupa, A. P., Richard, M., Soler, M., et al. (2006). A quantitative trait locus for recognition of foreign eggs in the host of a brood parasite. J. Evol. Biol. 19, 543–550. doi: 10.1111/j.1420-9101.2005.01002.x
Mcleod, K. M. (1997). Magpie-Larks Grallina cyanoleuca vs. common Koels Eudynamys Scolopacea: Brood Parasite or Predator? B.Sc. Honours thesis. Brisbane, AU: Griffith University.
Medina, I., and Langmore, N. E. (2016). Batten down the thatches: front-line defences in an apparently defenceless cuckoo host. Anim. Behav. 112, 195–201. doi: 10.1016/j.anbehav.2015.12.006
Moksnes, A., and Røskaft, E. (1989). Adaptations of meadow pipits to parasitism by the common cuckoo. Behav. Ecol. Sociobiol. 24, 25–30. doi: 10.1007/bf00300114
Molina-Morales, M., Martínez, J. G., Martín-Gálvez, D., Dawson, D. A., Burke, T., and Avilés, J. M. (2014). Cuckoo hosts shift from accepting to rejecting parasitic eggs across their lifetime. Evolution 68, 3020–3029. doi: 10.1111/evo.12471
Nakamura, H., Kubota, S., and Suzuki, R. (1998). Coevolution between the common cuckoo and its major hosts in Japan: stable versus dynamic specialization on hosts. Oxford Ornithol. Ser. 9, 94–112.
Noh, H., Jacomb, F., Gloag, R., and Langmore, N. E. (2021). Frontline defences against brood parasitism in large-billed gerygones. Anim. Behav. 174, 51–61. doi: 10.1016/j.anbehav.2021.01.021
Ödeen, A., and Håstad, O. (2010). Pollinating birds differ in spectral sensitivity. J. Comp. Physiol. 196, 91–96. doi: 10.1007/s00359-009-0474-z
Ödeen, A., Håstad, O., and Alström, P. (2011). Evolution of ultraviolet vision in the largest avian radiation-the passerines. BMC Evol. Biol. 11:313. doi: 10.1186/1471-2148-11-313
Peer, B. D., Rivers, J. W., and Rothstein, S. I. (2013). Cowbirds, conservation, and coevolution: potential misconceptions and directions for future research. Chin. Birds 4, 15–30. doi: 10.5122/cbirds.2013.0009
Peer, B. D., Rothstein, S. I., Kuehn, M. J., and Fleischer, R. C. (2005). Host defenses against cowbird (Molothrus spp.) parasitism: implications for cowbird management. Ornithol. Monogr. 57, 84–97. doi: 10.2307/40166816
R Core Team (2014). R: A Language and Environment for Statistical Computing. Vienna: R Foundation for Statistical Computing.
Robert, M., and Sorci, G. (1999). Rapid increase of host defense against brood parasites in a recently parasitized area: the case of village weavers in Hispaniola. Proc. R. Soc. Lond. B 266, 941–946. doi: 10.1098/rspb.1999.0727
Rodríguez-Gironés, M. A., and Lotem, A. (1999). How to detect a cuckoo egg: a signal-detection theory model for recognition and learning. Am. Nat. 153, 633–648. doi: 10.1086/303198
Rohwer, S., and Spaw, C. D. (1988). Evolutionary lag versus bill-size constraints: a comparative study of the acceptance of cowbird eggs by old hosts. Evol. Ecol. 2, 27–36. doi: 10.1007/bf02071586
Rothstein, S. I. (1975a). Evolutionary rates and host defenses against avian brood parasitism. Am. Nat. 109, 161–176. doi: 10.1086/282984
Rothstein, S. I. (1975b). An experimental and teleonomic investigation of avian brood parasitism. Condor 77, 250–271. doi: 10.2307/1366221
Rothstein, S. I. (1982). Mechanisms of avian egg recognition: which egg parameters elicit responses by rejecter species? Behav. Ecol. Sociobiol. 11, 229–239. doi: 10.1007/bf00299299
Sealy, S. G. (1992). Removal of yellow warbler eggs in association with cowbird parasitism. Condor 94, 40–54. doi: 10.2307/1368794
Soler, J. J., Avilés, J. M., Møller, A. P., and Moreno, J. (2012). Attractive blue-green egg coloration and cuckoo-host coevolution. Biol. J. Linn. Soc. 106, 154–168. doi: 10.1111/j.1095-8312.2012.01857.x
Soler, M. (2014). Long-term coevolution between avian brood parasites and their hosts. Biol. Rev. 89, 688–704. doi: 10.1111/brv.12075
Soler, M., and Martínez, J. G. (2000). Is egg-damaging behaviour by great spotted cuckoos an accident or an adaptation? Behav. Ecol. 11, 495–501. doi: 10.1093/beheco/11.5.495
Soler, M., Ruiz-Castellano, C., Carra, L. G., Ontanilla, J., and Martín-Galvez, D. (2013). Do first-time breeding females imprint on their own eggs? Proc. R. Soc. Lond. B 280:20122518. doi: 10.1098/rspb.2012.2518
Soler, M., Soler, J. J., Martinez, J. G., and Møller, A. P. (1994). Micro-evolutionary change in host response to a brood parasite. Behav. Ecol. Sociobiol. 35, 295–301. doi: 10.1007/s002650050100
Spottiswoode, C. N., and Stevens, M. (2010). Visual modeling shows that avian host parents use multiple visual cues in rejecting parasitic eggs. Proc. Natl. Acad. Sci. U.S.A. 107, 8672–8676. doi: 10.1073/pnas.0910486107
Spottiswoode, C. N., and Stevens, M. (2012). Host-parasite arms races and rapid changes in bird egg appearance. Am. Nat. 179, 633–648. doi: 10.1086/665031
Takasu, F. (1998). Modelling the arms race in avian brood parasitism. Evol. Ecol. 12, 969–987. doi: 10.1023/a:1006520323251
Takasu, F., Kawasaki, K., Nakamura, H., Cohen, J. E., and Shigesada, N. (1993). Modeling the population dynamics of a cuckoo-host association and the evolution of host defenses. Am. Nat. 142, 819–839. doi: 10.1086/285574
Welbergen, J. A., and Davies, N. B. (2008). Reed warblers discriminate cuckoos from sparrowhawks with graded alarm signals that attract mates and neighbours. Anim. Behav. 76, 811–822. doi: 10.1016/j.anbehav.2008.03.020
Welbergen, J. A., and Davies, N. B. (2011). A parasite in wolf’s clothing: hawk mimicry reduces mobbing of cuckoos by hosts. Behav. Ecol. 22, 574–479. doi: 10.1093/beheco/arr008
Keywords: Anthochaera carunculata, avian brood parasitism, egg ejection, Eudynamys orientalis, host defenses, mobbing, Pacific Koel, Red Wattlebird
Citation: Abernathy VE, Johnson LE and Langmore NE (2021) An Experimental Test of Defenses Against Avian Brood Parasitism in a Recent Host. Front. Ecol. Evol. 9:651733. doi: 10.3389/fevo.2021.651733
Received: 10 January 2021; Accepted: 31 March 2021;
Published: 27 April 2021.
Edited by:
Cynthia Ursino, Princeton University, United StatesReviewed by:
Mercedes Molina-Morales, University of Granada, SpainDanielle June Whittaker, Michigan State University, United States
Copyright © 2021 Abernathy, Johnson and Langmore. This is an open-access article distributed under the terms of the Creative Commons Attribution License (CC BY). The use, distribution or reproduction in other forums is permitted, provided the original author(s) and the copyright owner(s) are credited and that the original publication in this journal is cited, in accordance with accepted academic practice. No use, distribution or reproduction is permitted which does not comply with these terms.
*Correspondence: Virginia E. Abernathy, dmFiZXJuYUBjbGVtc29uLmVkdQ==