- 1Primate Research Institute, Kyoto University, Inuyama, Japan
- 2Amis des Bonobos du Congo, Lola ya Bonobo Sanctuary, Kinshasa, Democratic Republic of the Congo
Intense selection pressure from parasites on free-living animals has resulted in behavioral adaptations that help potential hosts avoid sources of infection. In primates, such “behavioral immunity” is expressed in different contexts and may vary according to the ecology of the host, the nature of the infectious agent, and the individual itself. In this study, we investigated whether avoidance of contaminated food was associated with reduced parasite infection in sanctuary-housed bonobos. To do this, we used bonobos’ responses to soil- and fecally-contaminated food in behavioral experiments, and then compared the results with an estimate of protozoan infection across individuals. We found that avoidance of contaminated food correlated negatively with Balantioides coli infection, a potentially pathogenic protozoan transmitted through the fecal-oral route. The association between avoidance responses and parasitism were most evident in experiments in which subjects were offered a choice of food items falling along a gradient of fecal contamination. In the case of experiments with more limited options and a high degree of contamination, most subjects were averse to the presented food item and this may have mitigated any relationship between feeding decisions and infection. In experiments with low perceived levels of contamination, most subjects consumed previously contaminated food items, which may also have obscured such a relationship. The behavioral immunity observed may be a consequence of the direct effects of parasites (infection), reflecting the first scale of a landscape of disgust: individual responses. Indirect effects of parasites, such as modulation of feeding decisions and reduced social interactions—and their potential trade-offs with physiological immunity—are also discussed in light of individual fitness and primate evolution. This study builds on previous work by showing that avoidance behaviors may be effective in limiting exposure to a wide diversity of oro-fecally transmitted parasites.
Introduction
Intense selection pressure from parasites on free-living animals has driven behavioral adaptations in potential hosts to avoid sources of infection (Curtis, 2014; Sarabian et al., 2018b). The “behavioral immune system” (Schaller and Park, 2011)—also known as the “adaptive system of disgust” (Curtis, 2013; Lieberman and Patrick, 2014)—orchestrates avoidance of parasites through: (1) detection of signals that co-occur with infectious agents; (2) activation of emotional and cognitive responses; and (3) elicitation of behaviors that reduce the risk of disease (Curtis, 2014). Such behavioral immunity arises as a non-consumptive effect (NCE) of parasitism (Buck et al., 2018) and can have significant impacts on an individual, e.g., in its overall patterns of foraging and even in its choice of social partners. In primates, behavioral immunity is evidenced by avoidance of fecally-contaminated food and water (Sarabian and MacIntosh, 2015; Amoroso et al., 2017, 2019; Sarabian et al., 2018a; Poirotte and Kappeler, 2019; Poirotte et al., 2019), reductions in time spent grooming infected conspecifics (Poirotte et al., 2017), avoidance of risky sex with ulcerated mates with a sexually-transmitted disease (Paciência et al., 2019), and recursion to specific sites within a home range according to the intensity of infection within the group and contamination of the environment (Poirotte et al., 2017).
Although studies describing the mechanisms and strategies of parasite avoidance in primates and other animals are increasing, investigation of the consequences of parasite avoidance, its behavioral plasticity and the trade-offs involved remain scarce (Buck et al., 2018; Sarabian et al., 2018b). In non-human primates, to our knowledge, evidence of fitness consequences of behavioral immunity at an individual level is limited at present to two studies showing that hygienic tendencies and personalities (i.e., the persistence of such strategies across time and space) correlate with low levels of geohelminth infection in free-ranging Japanese macaques (Macaca fuscata; Sarabian and MacIntosh, 2015) and low oro-fecally transmitted parasite richness in wild gray mouse lemurs (Microcebus murinus; Poirotte and Kappeler, 2019). At a social level, a recent framework based on empirical studies illustrates how a change in the network structure, through a decrease in contact rates after a pathogen has been detected, reduces pathogen transmission (Romano et al., 2020). At a higher level of ecological organization, Weinstein et al. (2018) propose a rethink of host behavior through a “landscape of disgust,” in which animals would have evolved to navigate through peaks of parasite abundance and valleys of safety—all avoided or reached via different compromises—provoking cascading effects at lower (i.e., individual and social) and higher (ecosystem) levels.
In this article, we focus on the first level of avoidance: individuals and their immediate environment. To do so, we investigated how sanctuary-housed bonobos (Pan paniscus) fit into a “micro landscape of disgust,” i.e., whether their sensitivity toward surrounding contaminated food is associated with levels of parasite infection. Little is known about the gastrointestinal parasites of these highly social endangered primates in the wild, with only four published studies revealing a similar range of parasite taxa as that described in chimpanzees (Pan troglodytes) (Hasegawa et al., 1983; Dupain et al., 2009; Narat et al., 2015; Medkour et al., 2021). Typical protozoa and helminths in Pan species include Troglodytella, Balantioides, Entamoeba, Capillaria, Strongyloides, Ascaris, Trichuris, Enterobius, Oesophagostomum spp., and Dicrocoelids (Petrželková and Huffman, 2018). Many of these parasites have a direct life cycle and are transmitted through the fecal-oral route. Through a series of experiments, our previous study revealed a positive correlation between food/surface contamination and bonobo aversion toward potential food items (Sarabian et al., 2018a). Specifically, we observed a lower likelihood of feeding on contaminated food, as well as lower interaction rates and fewer instances of tool use with/through contaminated surfaces. Because we expected to observe variation in oro-fecally/soil transmitted parasite infection in bonobos, we considered results from three of our earlier experiments that focused specifically on the avoidance of feces and soil contamination. These experiments involved food that was either covered with soil/feces, in contact with or near feces, or previously in contact with feces. The logical next step was to investigate the potential correlation between aversion and infection phenotypes in bonobos.
We thus coupled experimental data previously published in Sarabian et al. (2018a) with coproscopic analyses to test the infection-avoidance hypothesis in bonobos. Based on the assumption that the adaptive system of disgust has evolved to procure health benefits (Curtis, 2014), we predicted that higher levels of avoidance would reflect lower levels of parasite infection. Then, given previous reports of variation in behavioral immunity among primates (Moya et al., 2004; Sarabian and MacIntosh, 2015; Sarabian et al., 2018a; Tybur et al., 2018; Paciência et al., 2019; Poirotte and Kappeler, 2019), we also predicted that the patterns of infection would vary according to the sex and age of each individual—with males and immatures being more prone to infection (i.e., higher parasite infection intensity and/or richness) than females and mature individuals, respectively.
Materials and Methods
Study Site and Bonobos
The experiments were conducted between May and July 2016 with semi-free-ranging bonobos at Lola ya Bonobo Sanctuary1 in Kinshasa, Democratic Republic of the Congo (for further details see Sarabian et al., 2018a). During the day, bonobos live in four separate enclosures including high canopy forest areas with palm oil trees, swamps or water ponds (E1–E3), and an outdoor forested playground (“nursery”). At night, they are socially housed in dormitories composed of different compartments with several hammocks. To facilitate maintenance, each outdoor enclosure and dormitory has doors with a grid of metal bars through which bonobos can pass an arm and be examined by caretakers and veterinarians. In addition to the fruits, leaves and palm oil nuts (Elaeis guineensis) that naturally occur in their enclosures, bonobos were fed twice daily with 6.5 kg of seasonal fruits and vegetables, as well as sugarcane, soy milk, boiled eggs and peanuts.
Behavioral Experiments
In this study, we considered three experiments for which experimental results were previously published in Sarabian et al. (2018a), and which involved feeding decisions on soil- and/or fecally-contaminated food under different contamination contexts (i.e., high, gradient, past). We classified the bonobos into two age groups for both males and females: immature (infants and juveniles; 2–7 years) and mature (adolescents and adults; 8–22 years; see Supplementary Table 1 for details). To match with fecal sampling data (below), we considered a total of 39 individuals with 34 (22 matures; 17 females) in experiment 1, 33 (21 matures; 15 females) in experiment 2, and 32 (19 matures; 14 females) in experiment 3 (Supplementary Table 1). The first experiment aimed to test for bonobos’ avoidance of highly contaminated food by presenting three slices of apple—one of their favorite food items at the sanctuary (Rosati and Hare, 2011, 2013)—simultaneously: one slice covered with soil, one slice covered with feces, and one slice clean (control). The second experiment explored bonobos’ sensitivity to a gradient of contamination risk by presenting a “chain of contagion”; a single row of six banana slices with one of the slices at the extremity placed atop feces from a conspecific (Figure 1A). The third experiment examined whether bonobos are sensitive to previous contamination (with conspecific feces), potentially reflecting a lower perceived level of contamination. The experimenter presented two slices of banana, one put in contact with feces, the other not. The feces were then removed from view using a cardboard cover and the two slices of banana were presented to the subject. For each of these experiments, items were presented on a table behind enclosures’ doors from where we recorded each subject’s feeding decision.
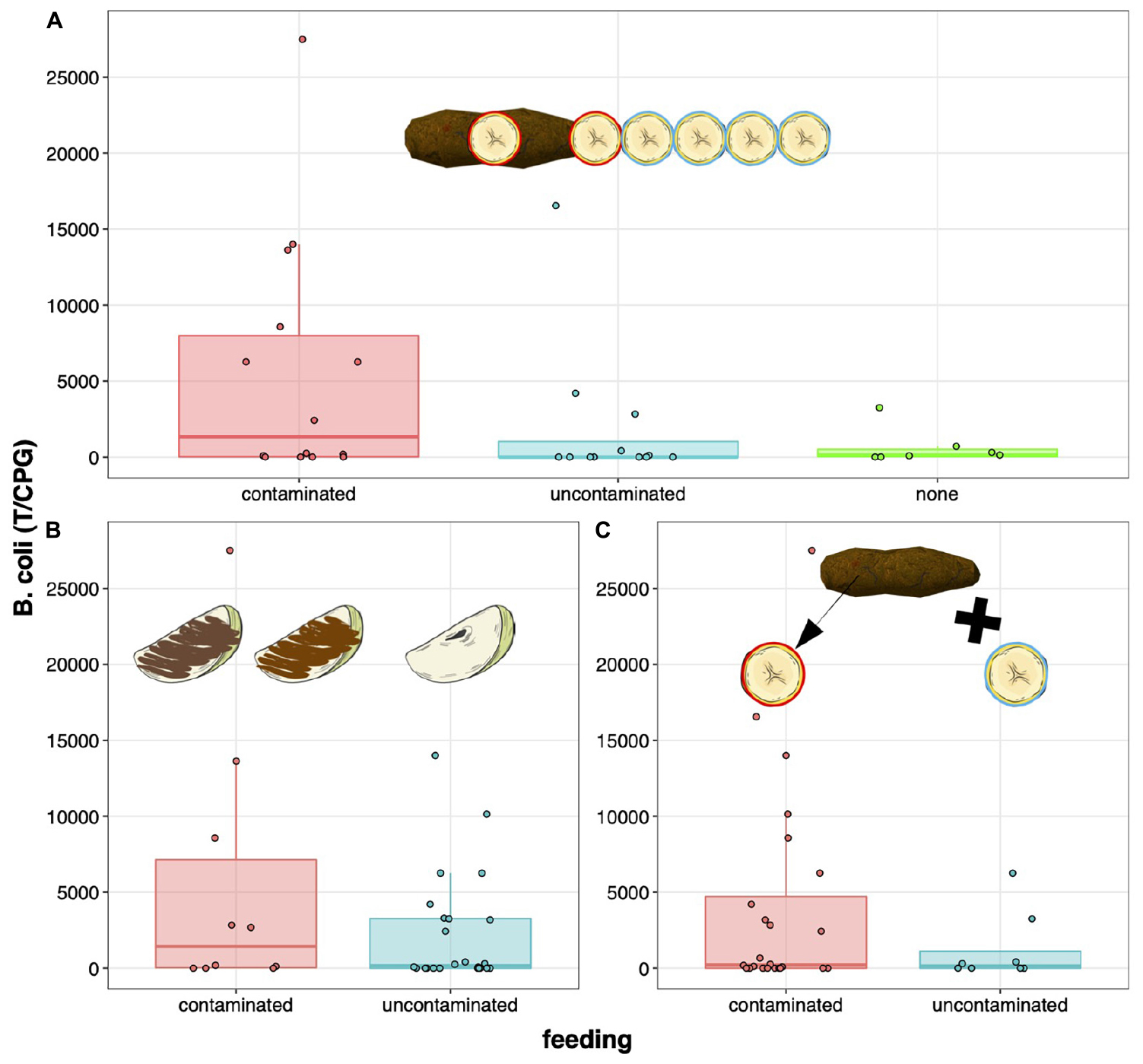
Figure 1. Levels of Balantioides coli infection (average number of trophozoites/cysts per gram of feces) according to feeding decisions in bonobos exposed to (A) a chain of contagion in exp. #2; (B) soil- and fecally-contaminated apple slices in exp. #1; and (C) previous contamination in exp. #3. Individuals (dots) feeding on contaminated food (red boxplots) tended to be significantly more infected with B. coli than individuals feeding on control food (blue boxplots) or not feeding at all (green boxplot in exp. #2).
In exp. 1, we considered the feeding response to contaminated apple slices (1 if they fed on soil- and/or feces-covered slices—see Results for details; 0 if they only fed on control items). In exp. 2, since our previous study (Sarabian et al., 2018a) reported feeding on contaminated (slices #1–2; atop and adjacent to feces, respectively) and uncontaminated slices of banana (#3–6), as well as not feeding at all despite approaching the experimental area, we considered three “feeding” categories (“contaminated”; “uncontaminated”; and “none”) instead of a binary response. The category “none” reflects a subject who approached to within 1 m of the experimental set-up—sometimes engaging with it (by e.g., touching the table/a food item or using a stick to reach the table)—but did not feed on any slice of banana. In exp. 3, we considered the feeding response to previously contaminated bananas (1 if they fed on the contaminated piece; 0 if they fed on the control only). Subjects were all tested in the morning/afternoon before feeding occurred to minimize the effect of potential satiety. All experiments and measured variables are described in greater detail in Sarabian et al. (2018a).
Fecal Sample Collection and Parasitological Analyses
Fecal samples were collected over the second half of June 2016 after bonobos awoke from sleep. The last anthelmintic treatment (Albendazole 400 mg) took place at the beginning of March 2016 (Mungongo et al., unpublished data) and no antiprotozoal treatments were conducted in the months preceding our study. Approximately 2 g of fecal matter was put in a 15 mL tube filled with sodium acetate-acetic acid-formaldehyde (SAF) solution. We processed 93 samples from 39 identified individuals (2.4 ± 1.3 samples individual–1; Supplementary Table 1) using fecal sedimentation and flotation (for a subset only; see below) procedures to identify and quantify parasite stages in feces (see Pomajbíková and Huzová, 2018). We used fecal egg/trophozoite/cyst counts (EPG: number of eggs gram–1 fecal sediment for metazoan parasites; T/CPG: number of trophozoites/cysts gram–1 fecal sediment for protozoan parasites) determined via microscopy using a McMaster slide as a proxy for parasite infection across subjects. The fecal sediment of each sample was screened 3 times (3 single McMaster chamber replicates; 3 chambers in total) and the average was used to calculate values of EPG or T/CPG. To test whether these counts were reliable, a second observer screened 11% (N = 10) of the samples using the same method and count procedures. In addition, the second observer processed the same 10 samples through different flotation solutions (Sheather’s sugar, Zinc sulfate, Sodium nitrate). Floated samples were screened twice (2 double McMaster chamber replicates; 4 chambers in total) and the average EPG or T/CPG was computed. We used Spearman’s rank correlation tests to compare for each parasite species: (1) inter-observer reliability examined after fecal sedimentation; and (2) inter-method reliability with the same observer. To maximize analytical efficiency in relating behavior with infection, we retained only parasites that reached a threshold of 30% prevalence in our samples. If results from our reliability tests across concentration methods differed, we used only the most sensitive method in our analyses.
Statistical Analyses
We built generalized linear mixed-effects models (GLMMs) to analyze how infection levels may covary with feeding decisions across experiments. Three models were constructed—one for each experiment—with the average EPG or T/CPG (across counts and samples) of each individual used as a count response. Each model used a negative binomial error structure to reflect the distribution of the EPG or T/CPG data. For predictor variables, we included feeding decision (#1: feed on contaminated apple slices or not; #2: feed on contaminated/uncontaminated banana slices or not; #3: feed on previously contaminated banana or not), and a combined age-sex category (mature female, mature male, immature female, immature male). Because we did not aim to explicitly test the mean difference between bonobos in different enclosures, we placed enclosure identity (E1, E2, E3, nursery) as a random effect in the models. Moreover, our study did not involve multiple trials per individual in the same experiment, so a repeated design was not necessary. All three models were fit using the package glmmTMB (Magnusson et al., 2020). We used the package lmtest (Hothorn et al., 2020) to compare full (fitted) models with all predictor variables included vs. null models with only variables to control for, i.e., “age-sex category” as a fixed effect and “enclosure” as a random effect, using likelihood ratio tests (LRT). When possible, we also compared full (fitted) models with potential interactions between terms of interest (i.e., feeding decisions and age-sex category; Sarabian et al., 2018a) vs. full models without interactions using LRT. Finally, we used the DHARMa package (Hartig, 2021) to test for homogeneity of residuals, homoscedasticity across variables, and the absence of zero-inflation in our data and no violations of model assumptions were found. All data were analyzed in R v.3.6.3 (R Core Team, 2020) and are accessible in the Supplementary Material.
Results
Which Parasites Infect Bonobos at Lola ya Bonobo?
The most common parasite observed in our subjects with a prevalence of 64% was Balantioides coli (formerly known as Balantidium coli; Pomajbíková and Modrý, 2018); a protozoan acquired through the ingestion of fecally-contaminated food and/or water. The prevalence of the other protozoan found in the samples, Troglodytella sp., was 23%. Apart from these two protozoans, we did not detect any other gastrointestinal parasites during our examinations. Moreover, out of the 14 fecal samples in which T. sp. was detected, B. coli was detected only twice. T/CPG of the observed organisms derived from fecal sedimentation were highly correlated across observers (Spearman’s rank correlation; Balantioides coli obs1 vs. obs2: r = 0.92; p < 0.001; Troglodytella sp. CPG obs1 vs. obs2: r = 1; p < 0.001; see Supplementary Material). However, a correlation matrix of CPG counts for the 10 samples analyzed across all methods (sedimentation vs. flotation) did not always show high reliability for B. coli (Spearman’s rank correlation; sedimentation vs. sugar: r = 0.41; p = 0.073; vs. zinc sulfate: r = 0.66; p = 0.002; vs. sodium nitrate: r = 0.38; p = 0.096). Correlations were better for the detection of Troglodytella sp. (Spearman’s rank correlation; sedimentation vs. sugar: r = 0.86; p < 0.001; vs. zinc sulfate: r = 0.86; p < 0.001; vs. sodium nitrate: r = 0.74; p < 0.001). Fecal sedimentation provided the highest sensitivity for diagnostics of B. coli, and the largest counts, so we used these data to calculate T/CPG.
Does Avoidance of Contaminated Food Relate to Infection?
The proportions of individuals feeding on contaminated food in experiments #1–3 were 0.29, 0.42 and 0.75, respectively. Note that only 2 out of 34 individuals fed on fecally-contaminated apple slices in exp. 1, hence the regrouping of soil- and fecally-contaminated apple slices as “contaminated food” (Figure 1B). Only model #2 significantly outperformed its respective null model (LRT; #1: ΔLogLik = 0.37, Δd.f. = 1, p = 0.389; #2: ΔLogLik = 3.67, Δd.f. = 2, p = 0.026; #3: ΔLogLik = 0.79, Δd.f. = 1, p = 0.208; see Supplementary Table 2 for details). We thus retained the full model (#2) without interaction between the age-sex category and feeding because the latter was not outperformed by the model with interaction (LRT; ΔLogLik = 5.17, Δd.f. = 6, p = 0.112). We found that B. coli infection (T/CPG) was significantly lower in subjects who fed on uncontaminated banana slices and in others who did not feed compared to those who fed on contaminated slices in exp. 2 (GLM#2; uncontaminated vs. contaminated: z = −2.73, p = 0.006; none vs. contaminated: z = −3.13, p = 0.002; Figure 1A and Table 1). Moreover, subjects who did not feed and those who fed on uncontaminated banana slices did not significantly differ in levels of B. coli infection (z = −0.10, p = 0.923; Table 1). We also found that mature males were infected to a higher degree with B. coli than other age-sex categories (all 0.006 < p ≤ 0.07; see Table 1 for details)—a pattern we did not find in null models #1 and #3.
Discussion
This study revealed a negative correlation between bonobos’ aversion to contaminated food and infection with an oro-fecally transmitted and potentially pathogenic gastrointestinal parasite (Balantioides coli). We previously showed that bonobo feeding decisions were influenced by contamination risk, with individuals avoiding food associated with sensory cues of soil and/or conspecific feces (Sarabian et al., 2018a). Here, we show that bonobos who are sensitive to gradients of risk (exp. 2) may benefit from reduced parasite burdens. As was shown in previous studies of Japanese macaques (Sarabian and MacIntosh, 2015) and gray mouse lemurs (Poirotte and Kappeler, 2019), our results indicate that risk-averse bonobos may therefore have a fitness advantage over risk-prone individuals in their ability to minimize oro-fecally transmitted parasite acquisition. If so, our study supports the infection-avoidance hypothesis in a new host-parasite system, and one with close phylogenetic ties to our own human lineage.
Yet, the detection of a significant correlation between aversion and infection could only be highlighted in a certain context. In exp. 1, the level of contamination was quite high for two-thirds of the food items (slices of apple) presented—with about the same weight of contaminant (soil or feces) as the slice. Consequently, 71% of subjects only fed on control (clean) apples. This “high contamination—high aversion” context may not have allowed enough behavioral variation to reflect a difference in the magnitude of infection among “avoiders” and “risk takers” (Figure 1B). In comparison, contamination in exp. 2 followed a gradient as one slice of banana (on 6) was deposited atop feces, a second next to it and others in contact with the previous one. In this condition, 58% of subjects restricted themselves to uncontaminated slices (#3–6) or did not feed at all. Comparatively, contamination in exp. 3 only refers to a previous contamination event: one slice of banana was put in contact with conspecific feces for 5 s (see Sarabian et al., 2018a) before being presented to the tested subject along the control slice. Thus, only 25% of subjects restricted their feeding to the control item, which may reflect lower perceived levels of contamination (Figure 1C). The validity of the model and significance of the negative correlation between feeding decisions and infection in exp. 2 may be explained by the gradient of avoidance that could be expressed along that gradient of contamination. Indeed, B. coli T/CPG were not only significantly different between feeders of contaminated banana slices and non-feeders, but also between feeders of contaminated slices and feeders of non-contaminated slices (Figure 1A). However, non-feeders and feeders of non-contaminated slices did not significantly differ in their levels of infection. These results support the hypothesis that caution (e.g., opting for non-contaminated food or refraining altogether from feeding in potentially risky contexts) reduces infection risk, while also highlighting potential NCEs related to such feeding trade-offs.
Intestinal protozoa such as Balantioides coli (primarily known for colonizing the intestine of suids) are common parasites in non-human primates and can also infect humans (Petrželková and Huffman, 2018; Pomajbíková and Modrý, 2018; Poirotte and Charpentier, 2020). In African great apes, though, B. coli is more commonly found in captive groups than in their wild counterparts (Pomajbíková et al., 2010). One hypothesis for this difference may be the higher exposure to synanthropic rats in captivity, which have been pointed out as potential reservoirs of infection (Bogdanovich, 1955; Knezevich, 1998). Previous studies have also found an association between a starch-rich diet in captive chimpanzees and crop-raiding baboons (Papio anubis) and high intensities of B. coli infection (Schovancová et al., 2013; Weyher et al., 2006). Bonobos at Lola ya Bonobo did not rely especially on starchy food—although tubers, bananas and peanuts made up to 30% of their diet at the time of the study. Although Balantioides infections are usually asymptomatic in captive great apes, they can cause balantidiasis, with symptoms ranging from mild diarrhea to fulminating dysentery (see Pomajbíková and Modrý, 2018). Some individuals did have high infection intensities, with the most heavily infected individual being a 4-years-old female, born at the sanctuary and living in enclosure 3 (Supplementary Table 1). In comparison, Troglodytella sp. had a much lower prevalence (23%), which would not allow statistical modeling. Moreover, T. sp. was negatively associated with B. coli—a trait also observed in chimpanzees, potentially because the latter taxon makes the large intestine less suitable for T. abrassarti (McLennan et al., 2017). Most importantly, while B. coli is transmitted via the fecal-oral route and considered to be mildly pathogenic in chimpanzees (Pomajbíková et al., 2010), T. sp. is not, and is rather viewed as a symbiont with the function of participating in food digestion (Profousová et al., 2011).
Regardless of its pathogenicity, one important aspect of B. coli regarding our experiments is its transmission pathway. Infections are usually acquired by ingesting cysts in contaminated food or water (Schuster and Ramirez-Avila, 2008), or otherwise from contaminated substrata. Thus, if the parasite is less prevalent in bonobos that are more averse to contaminated foods, as our study found, this would suggest that avoidance is effective in reducing transmission via the fecal-oral route. These results build on previous findings in other primate species demonstrating a negative correlation between avoidance behaviors and infection with oro-fecally transmitted parasites (Sarabian and MacIntosh, 2015; Poirotte and Kappeler, 2019). To our knowledge, this is the first study to display a link between feces avoidance and the intensity of B. coli infection. Our previous work with Japanese macaques focused on intensity of infection with nematode parasites (Sarabian and MacIntosh, 2015), and Poirotte and Kappeler (2019) used parasite status/richness (i.e., number of nematode species present) as a proxy of infection in gray mouse lemurs. Thus, our results add to the growing body of literature showing that sensitivity to contamination can drive feeding decisions in primates, as they do in other taxa (Ezenwa, 2004; Anderson and McMullan, 2018; Coulson et al., 2018; Zélé et al., 2019).
Also in line with previous studies of primates, and according to our prediction, we also observed a significant male bias toward infection. Mature males exhibited significantly higher infection intensities than mature females and immature males. Recent analyses of fecal samples from wild bonobos also found a higher prevalence of gastrointestinal helminths in males compared to females, although no significant differences in estimated infection intensities between the sexes (Dardel, 2020). On the whole, therefore, results in bonobos appear in line with general patterns of male-biased parasitism in mammals (Zuk and McKean, 1996; Klein, 2000, 2004). From a behavioral perspective—in our experiments, at least—this is not intuitive as immatures fed on contaminated food and interacted with contaminated surfaces more often than mature bonobos, including males, and we observed little difference in the risk sensitivity of adult males and females (Sarabian et al., 2018a). Thus, while we cannot rule out behavior as a mechanism underlying male biases in infection among bonobos, other mechanisms may be at play. In wild male chimpanzees, testosterone and cortisol are associated with higher helminth and protozoa richness (Muehlenbein, 2006), which may be explained by the immunosuppressive activity of both hormones (reviewed in Sapolsky et al., 2000; Muehlenbein and Bribiescas, 2005). In turn, levels of fecal testosterone vary with the dominance rank of the individual, so that higher ranking individuals express higher testosterone levels and greater helminth (but not protozoan) richness (Muehlenbein and Watts, 2010). Further studies should thus investigate whether there is a link between dominance rank and levels of gastrointestinal parasite infection in bonobos, and whether their behavioral immunity has a role to play in this equation.
Related to an individual’s sex and age, its physiological resistance to or tolerance of infection may also have played a role in shaping patterns of infection observed here. For protozoa such as B. coli—which can fully replicate inside their hosts and cause chronic infections (Ponce-Gordo and García-Rodríguez, 2020)—the physiological immune response is particularly relevant in minimizing the load during or after establishment of the parasite. Hosts can handle parasitism in three ways, known as: avoidance, resistance and tolerance (Rivas et al., 2014). A previous study in salmonids (Salmo salar and Salmo trutta) showed the potential interplay between these defensive strategies, with i.e., the most resistant populations being the less avoidant and tolerant toward eye fluke (Diplostomum pseudospathaceum) infection (Klemme et al., 2020). We acknowledge that focusing on one mechanism could have ignored how other defense strategies may impact the “avoidance-infection” relationship. There is evidence supporting the hypothesis that behavioral and physiological responses are correlated across species (Hawley et al., 2011). For example, promiscuous primates appear to invest more in the production of immune cells such as white blood cells (Nunn et al., 2000; Nunn, 2002), while social insects express reduced immunity to parasites compared to asocial insects (e.g., Barribeau et al., 2015; López-Uribe et al., 2016). Both examples suggest an interplay between behavior and immune physiology. Whether such interactions also exist within species like bonobos, expressed through variable behavioral and immune phenotypes across individuals, has not yet been tested and would be a great start to have a more comprehensive view of parasite defense strategies in these social and endangered primates.
In conclusion, the behavioral immunity observed in bonobos may be a consequence of the direct effects of parasites (infection), reflecting on the first scale of a landscape of disgust: individual responses. However, indirect effects of parasites (i.e., NCEs) such as modulation of feeding decisions and social interactions also exist, and are often accompanied by trade-offs. As such, Japanese macaques would avoid feeding atop conspecific feces for a grain of wheat, but not for half a peanut (Sarabian and MacIntosh, 2015). Similarly, mandrills (Mandrillus sphinx) would avoid grooming group members highly infected with oro-fecally transmitted protozoa, except if they are maternal kin (Poirotte and Charpentier, 2020). Although the parasites in question here (i.e., oro-fecally transmitted helminth and protozoa) are not highly pathogenic, the fact that their main contaminant (i.e., feces) can harbor a wide diversity of pathogens, may have led primates to evolve a general avoidance response toward unknown fecal material (see Poirotte et al., 2019). The latter would provide increased fitness in “hygienic” individuals and may have precluded the need to develop cognitively demanding and costly detection and discrimination mechanisms specifically toward helminths and protozoa. Such a strategy may reflect innate tendencies to avoid feces, but could also be acquired through associative learning, which would explain why immatures are less cautious around risky contaminants. Mechanisms for such conditioning may include the association of feces sensory cues with digestive discomfort due to parasite infections acquired from contact with feces (Amoroso, 2021), and/or observational learning from adults making their own foraging decisions (e.g., Huffman and Hirata, 2004; Tarnaud and Yamagiwa, 2008). In sum, we highlight that the micro-landscape of disgust in a social primate can be dynamic and affected by multiple factors, behavior being among them. We thus encourage future studies to explore other correlates of the micro-landscape of disgust in animals.
Data Availability Statement
The original contributions presented in the study are included in the article/Supplementary Material, further inquiries can be directed to the corresponding author/s.
Ethics Statement
The animal study was reviewed and approved by the Animal Welfare and Animal Care Committee of Kyoto University Primate Research Institute (#2016-138).
Author Contributions
CS and AM designed the study and wrote the manuscript. CS collected and analyzed the data. RB supported data collection at Lola ya Bonobo Sanctuary. All authors approved the final manuscript.
Funding
CS was supported by the Leading Graduate Program in Primatology and Wildlife Science of Kyoto University, the Ministry of Education, Culture, Sports, Science and Technology of Japan (MEXT), and is currently funded by the Japan Society for the Promotion of Science (#P19088). This study was supported in part by the Wild and Wise Collaborative Learning Program (“Short-term Intern Program in Primatology and Wildlife Science”) of the Kyoto University Primate Research Institute (KUPRI).
Conflict of Interest
The authors declare that the research was conducted in the absence of any commercial or financial relationships that could be construed as a potential conflict of interest.
Acknowledgments
We thank Fanny Minesi, Pierrot Mbonzo, Claudine André, and all the staff at Lola ya Bonobo for facilitating data collection; the Ministry of Research and the Ministry of Environment in the Democratic Republic of the Congo for providing permits; and Ashutosh Khandual for performing part of the parasitological analyses. We also thank two reviewers and Marie Sigaud for commenting on an earlier draft of this manuscript, which considerably improved it, as well as Prof. Val Curtis, who inspired the “chain of contagion” experiments and much more in this field of research.
Supplementary Material
The Supplementary Material for this article can be found online at: https://www.frontiersin.org/articles/10.3389/fevo.2021.651159/full#supplementary-material
Footnotes
References
Amoroso, C. R. (2021). Integrating concepts of physiological and behavioral resistance to parasites. Front. Ecol. Evol. 9:635607. doi: 10.3389/fevo.2021.635607
Amoroso, C. R., Frink, A. G., and Nunn, C. L. (2017). Water as a counterstrategy to faecally transmitted disease: an experimental study in captive lemurs. Behaviour 154, 1239–1258. doi: 10.1163/1568539X-00003466
Amoroso, C. R., Kappeler, P. M., Fichtel, C., and Nunn, C. L. (2019). Fecal contamination, parasite risk, and waterhole use by wild animals in a dry deciduous forest. Behav. Ecol. Sociobiol. 73:153. doi: 10.1007/s00265-019-2769-6
Anderson, A., and McMullan, R. (2018). Neuronal and non-neuronal signals regulate Caenorhabditis elegans avoidance of contaminated food. Phil. Trans. R. Soc. B 373: 20170255. doi: 10.1098/rstb.2017.0255
Barribeau, S. M., Sadd, B. M., du Plessis, L., Brown, M. J. F., Buechel, S. D., Cappelle, K., et al. (2015). A depauperate immune repertoire precedes evolution of sociality in bees. Gen. Biol. 16:83. doi: 10.1186/s13059-015-0628-y
Bogdanovich, V. V. (1955). Spontaneous balantidiosis in rats. Meditsinskaya Parazitologiya I Parazitarnye Bolezni 24, 326–329.
Buck, J. C., Weinstein, S. B., and Young, H. S. (2018). Ecological and evolutionary consequences of parasite avoidance. Trends Ecol. Evol. 33, 619–632. doi: 10.1016/j.tree.2018.05.001
Coulson, G., Cripps, J. K., Garnick, S., Bristow, V., and Beveridge, I. (2018). Parasite insight: assessing fitness costs, infection risks and foraging benefits relating to gastrointestinal nematodes in wild mammalian herbivores. Phil. Trans. R. Soc. B 373:20170197. doi: 10.1098/rstb.2017.0197
Curtis, V. A. (2013). Don’t Look, Don’t Touch: The Science Behind Revulsion. Oxford: Oxford University Press.
Curtis, V. A. (2014). Infection-avoidance behaviour in humans and other animals. Trends Immunol. 35, 457–464. doi: 10.1016/j.it.2014.08.006
Dardel, R. (2020). Suivi Longitudinal des Helminthes Intestinaux D’une Communauté de Bonobos (Pan paniscus) en Mosaïque Forêt-Savane. Toulouse: Université Paul-Sabatier de Toulouse, 71–81. DVM thesis.
Dupain, J., Nell, C., Petrželková, K. J., Garcia, P., Modrý, D., and Gordo, F. P. (2009). “Gastrointestinal parasites of bonobos in the Lomako Forest, Democratic Republic of Congo,” in Primate Parasite Ecology: The Dynamics of Host-Parasite Relationships, eds M. A. Huffman and C. A. Chapman, (Cambridge: Cambridge University Press), 297–310.
Ezenwa, V. (2004). Selective defecation and selective foraging: antiparasite behavior in wild ungulates? Ethology 110, 851–862. doi: 10.1111/j.1439-0310.2004.01013.x
Hartig, F. (2021). DHARMa: Residual Diagnostics for Hierarchical (Multi-Level/Mixed) Regression Models. R Package Version 0.4.0. Available online at: https://cran.r-project.org/web/packages/DHARMa/index.html (accessed April 8, 2021).
Hasegawa, H., Kano, T., and Mulawa, M. (1983). A parasitological survey on the feces of pygmy chimpanzees, Pan paniscus, at Wamba, Zaire. Primates 24, 419–423.
Hawley, D., Etienne, R. S., Ezenwa, V. O., and Jolles, A. E. (2011). Does animal behavior underlie covariation between hosts’ exposure to infectious agents and susceptibility to infection? Implications for disease dynamics. Int. Comp. Biol. 51, 528–539. doi: 10.1093/icb/icr062
Hothorn, T., Zeileis, A., Farebrother, R. W., Cummins, C., Millo, G., and Mitchell, D. (2020). Lmtest: Testing Linear Regression Models. R Package Version 0.9-38.
Huffman, M., and Hirata, S. (2004). An experimental study of leaf swallowing in captive chimpanzees: insights into the origin of a self-medicative behavior and the role of social learning. Primates 45, 113–118. doi: 10.1007/s10329-003-0065-5
Klein, S. L. (2000). The effects of hormones on sex differences in infection: from genes to behavior. Neurosci. Biobehav. Rev. 24, 627–638. doi: 10.1016/S0149-7634(00)00027-0
Klein, S. L. (2004). Hormonal and immunological mechanisms mediating sex differences in parasite infection. Parasite Immunol. 26, 247–264. doi: 10.1111/j.0141-9838.2004.00710.x
Klemme, I., Hyvärinen, P., and Karvonen, A. (2020). Negative associations between parasite avoidance, resistance and tolerance predict host health in salmonid fish populations. Proc. R. Soc. B 287:20200388. doi: 10.1098/rspb.2020.0388
Knezevich, M. (1998). Geophagy as a therapeutic mediator of endoparasitism in a free-ranging group of Rhesus Macaques (Macaca mulatta). Am. J. Primatol. 44, 71–82. doi: 10.1002/(SICI)1098-2345199844:1<71::AID-AJP6<3.0.CO;2-U
Lieberman, D., and Patrick, C. (2014). Are the behavioral immune system and pathogen disgust identical? Evol. Behav. Sci. 8, 244–250. doi: 10.1037/ebs0000018
López-Uribe, M. M., Sconiers, W. B., Frank, S. D., Dunn, R. R., and Tarpy, D. R. (2016). Reduced cellular immune response in social insect lineages. Biol. Lett. 12:20150984. doi: 10.1098/rsbl.2015.0984
Magnusson, A., Skaug, H., Nielsen, A., Berg, C., Kristensen, K., Maechler, M., et al. (2020). Package ‘glmmTMB’. R package version 1.0.2.1.
McLennan, M. R., Hasegawa, H., Bardi, M., and Huffman, M. A. (2017). Gastrointestinal parasite infections and self-medication in wild chimpanzees surviving in degraded forest fragments within an agricultural landscape mosaic in Uganda. PLoS One 12:e0180431. doi: 10.1371/journal.pone.0180431
Medkour, H., Castaneda, S., Amona, I., Fenollar, F., André, C., Belais, R., et al. (2021). Potential zoonotic pathogens hosted by endangered bonobos. Sci. Rep. 11:6331. doi: 10.1038/s41598-021-85849-4
Moya, J., Bearer, C. F., and Etzel, R. A. (2004). Children’s behavior and physiology and how it affects exposure to environmental contaminants. Pediatrics 113, 996–1006.
Muehlenbein, M. P. (2006). Intestinal parasite infections and fecal steroid levels in wild chimpanzees. Am. J. Phys. Anthropol. 130, 546–550. doi: 10.1002/ajpa.20391
Muehlenbein, M. P., and Bribiescas, R. G. (2005). Testosterone-mediated immune functions and male life histories. Am. J. Hum. Biol. 17, 527–558. doi: 10.1002/ajhb.20419
Muehlenbein, M. P., and Watts, D. P. (2010). The costs of dominance: testosterone, cortisol, and intestinal parasites in wild male chimpanzees. BioPsychoSoc. Med. 4:21. doi: 10.1186/1751-0759-4-21
Narat, V., Guillot, J., Pennec, F., Lafosse, S., Grüner, A. C., Simmen, B., et al. (2015). Intestinal helminths of wild bonobos in forest-savanna mosaic: risk assessment of cross-species transmission with local people in the Democratic Republic of the Congo. EcoHealth 12, 621–633. doi: 10.1007/s10393-015-1058-8
Nunn, C. L. (2002). A comparative study of leukocyte counts and disease risk in primates. Evolution 56, 177–190. doi: 10.1111/j.0014-3820.2002.tb00859.x
Nunn, C. L., Gittleman, J. L., and Antonovics, J. (2000). Promiscuity and the primate immune system. Science 290, 1168–1170. doi: 10.1126/science.290.5494.1168
Paciência, F. M. D., Rushmore, J., Chuma, I. S., Lipende, I. F., Caillaud, D., Knauf, S., et al. (2019). Mating avoidance in female olive baboons (Papio anubis) infected by Treponema pallidum. Sci. Adv. 5:eaaw9724. doi: 10.1126/sciadv.aaw9724
Petrželková, K. J., and Huffman, M. A. (2018). “Parasites of chimpanzees and bonobos,” in Parasites of Apes: An Atlas of Coproscopic Diagnostics, eds D. Modrý, B. Pafèo, K. J. Petrželková, and H. Hasegawa, (Frankfurt: Edition Chimaira), 56–63.
Poirotte, C., and Charpentier, M. (2020). Unconditional care from close maternal kin in the face of parasites. Biol. Lett. 16:20190869. doi: 10.1098/rsbl.2019.0869
Poirotte, C., and Kappeler, P. (2019). Hygienic personalities in wild grey mouse lemurs vary adaptively with sex. Proc. R. Soc. B 286:20190863. doi: 10.1098/rspb.2019.0863
Poirotte, C., Massol, F., Herbert, A., Willaume, E., Bomo, P. M., Kappeler, P. M., et al. (2017). Mandrills use olfaction to socially avoid parasitized conspecifics. Sci. Adv. 3:e1601721. doi: 10.1126/sciadv.1601721
Poirotte, C., Sarabian, C., Ngoubangoye, B., MacIntosh, A. J. J., and Charpentier, M. (2019). Faecal avoidance differs between the sexes but not with nematode infection risk in mandrills. Anim. Behav. 149, 97–106. doi: 10.1016/j.anbehav.2019.01.013
Pomajbíková, K., and Huzová, Z. (2018). “Coproscopic examination techniques,” in Parasites of Apes: An Atlas of Coproscopic Diagnostics, eds D. Modrý, B. Pafčo, K. J. Petrželková, and H. Hasegawa, (Frankfurt: Edition Chimaira), 112–115.
Pomajbíková, K., and Modrý, D. (2018). “Balantioides coli and related cyst-forming ciliates,” in Parasites of Apes: An Atlas of Coproscopic Diagnostics, eds D. Modrı, B. Pafčo, K. J. Petrželková, and H. Hasegawa, (Frankfurt: Edition Chimaira), 112–115.
Pomajbíková, K., Petrželková, K. J., Profousová, I., and Modrý, D. (2010). Discrepancies in the occurrence of Balantidium coli between wild and captive African great apes. J. Parasitol. 96, 1139–1144. doi: 10.1645/GE-2433.1
Ponce-Gordo, F., and García-Rodríguez, J. J. (2020). Balantioides coli. Res. Vet. Sci. 135, 424–431. doi: 10.1016/j.rvsc.2020.10.028
Profousová, I., Mihaliková, K., Laho, T., Váradyová, Z., Petrželková, K. J., Modrý, D., et al. (2011). The ciliate, Troglodytella abrassarti, contributes to polysaccharide hydrolytic activities in the chimpanzee colon. Folia Microbiol. 56, 339–343. doi: 10.1007/s12223-011-0053-x
R Core Team, (2020). R: a Language and Environment for Statistical Computing. Vienna: R Foundation for Statistical Computing.
Rivas, F. V., Chervonsky, A. V., and Medzhitov, R. (2014). ART and immunology. Trends Imm. 35:451. doi: 10.1016/j.it.2014.09.002
Romano, V., MacIntosh, A. J. J., and Sueur, C. (2020). Stemming the flow: information, infection and social evolution. Trends Ecol. Evol. 35, 849–853. doi: 10.1016/j.tree.2020.07.004
Rosati, A. G., and Hare, B. (2011). Chimpanzees and bonobos distinguish between risk and ambiguity. Biol. Lett. 7, 15–18. doi: 10.1098/rsbl.2010.0927
Rosati, A. G., and Hare, B. (2013). Chimpanzees and bonobos exhibit emotional responses to decision outcomes. PLoS One 8:e63058. doi: 10.1371/journal.pone.0063058
Sapolsky, R. M., Romero, M., and Munck, A. U. (2000). How do glucocorticoids influence stress responses? Integrating permissive, suppressive, stimulatory, and preparative actions. Endoc. Rev. 21, 55–89. doi: 10.1210/edrv.21.1.0389
Sarabian, C., Curtis, V., and McMullan, R. (2018b). Evolution of pathogen and parasite avoidance behaviours. Philos. Trans. R. Soc. B Biol. Sci. 373:20170256. doi: 10.1098/rstb.2017.0256
Sarabian, C., Belais, R., and Maclntosh, A. J. J. (2018a). Feeding decisions under contamination risk in bonobos. Philos. Trans. R. Soc. B Biol. Sci. 373:20170195. doi: 10.1098/rstb.2017.0195
Sarabian, C., and MacIntosh, A. J. J. (2015). Hygienic tendencies correlate with low geohelminth infection in free-ranging macaques. Biol. Lett. 11:20150757. doi: 10.1098/rsbl.2015.0757
Schaller, M., and Park, J. H. (2011). The behavioral immune system (and why it matters). Curr. Dir. Psych. Sci. 20, 99–103. doi: 10.1177/0963721411402596
Schovancová, K., Pomajbíková, K., Procházka, P., Modrý, D., Bolechová, P., and Petrželková, K. J. (2013). Preliminary insights into the impact of dietary starch on the ciliate, Neobalantidium coli, in captive chimpanzees. PLoS One 8:e81374. doi: 10.1371/journal.pone.0081374
Schuster, F. L., and Ramirez-Avila, L. (2008). Current world status of Balantidium coli. Clin. Microbiol. Rev. 21, 626–638. doi: 10.1128/CMR.00021-08
Tarnaud, L., and Yamagiwa, J. (2008). Age-dependent patterns of intensive observation on elders by free-ranging juvenile Japanese macaques (Macaca fuscata yakui) within foraging context on Yakushima. Am. J. Primatol. 70, 1103–1113. doi: 10.1002/ajp.20603
Tybur, J. M., C1nar, C., Karinen, A. K., and Perone, P. (2018). Why do people vary in disgust? Phil. Trans. R. Soc. B 373:20170204. doi: 10.1098/rstb.2017.0204
Weinstein, S. B., Buck, J. C., and Young, H. S. (2018). A landscape of disgust. Science 359, 1213–1214. doi: 10.1126/science.aas8694
Weyher, A. H., Ross, C., and Semple, S. (2006). Gastrointestinal parasites in crop raiding and wild foraging Papio anubis in Nigeria. Int. J. Primatol. 27, 1519–1534. doi: 10.1007/s10764-006-9089-1
Zélé, F., Santos-Matos, G., Figueiredo, A. R. T., Eira, C., Pinto, C., Laurentino, T. G., et al. (2019). Spider mites escape bacterial infection by avoiding contaminated food. Oecologia 189, 111–122. doi: 10.1007/s00442-018-4316-y
Keywords: parasite avoidance, behavioral immunity, Pan paniscus, Balantioides coli, micro-landscape of disgust
Citation: Sarabian C, Belais R and MacIntosh AJJ (2021) Avoidance of Contaminated Food Correlates With Low Protozoan Infection in Bonobos. Front. Ecol. Evol. 9:651159. doi: 10.3389/fevo.2021.651159
Received: 08 January 2021; Accepted: 19 April 2021;
Published: 25 May 2021.
Edited by:
Sara B. Weinstein, The University of Utah, United StatesReviewed by:
Caroline R. Amoroso, University of Virginia, United StatesCollin James Horn, University of Alberta, Canada
Copyright © 2021 Sarabian, Belais and MacIntosh. This is an open-access article distributed under the terms of the Creative Commons Attribution License (CC BY). The use, distribution or reproduction in other forums is permitted, provided the original author(s) and the copyright owner(s) are credited and that the original publication in this journal is cited, in accordance with accepted academic practice. No use, distribution or reproduction is permitted which does not comply with these terms.
*Correspondence: Cécile Sarabian, c2FyYWJpYW5jZWNpbGVAZ21haWwuY29t