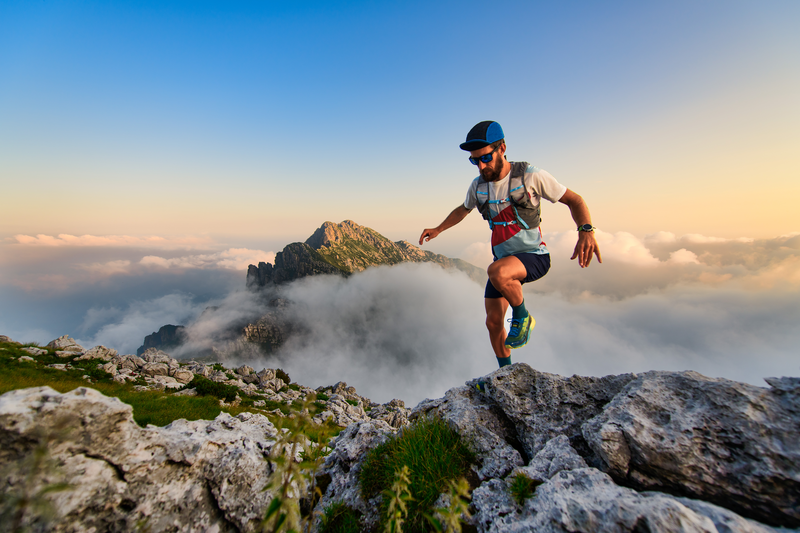
94% of researchers rate our articles as excellent or good
Learn more about the work of our research integrity team to safeguard the quality of each article we publish.
Find out more
MINI REVIEW article
Front. Ecol. Evol. , 19 March 2021
Sec. Biogeography and Macroecology
Volume 9 - 2021 | https://doi.org/10.3389/fevo.2021.634711
This article is part of the Research Topic Roles and Implications of Functional Traits and Phylogenies to Characterize Refugia Under Increasing Climate Variability View all 8 articles
The plight of Southeast Asia’s animals, plants and ecosystems in the face of unsustainable exploitation and habitat destruction has been illustrated in several recent studies, despite often falling outside the global discourse on global conservation priorities. Here, we collate biogeographic and phylogenetic information to argue that this beleaguered region is one of world’s primary macrorefugia, and possibly its best chance of regaining its natural biodiversity distribution patterns after the current Anthropocene upheaval. The region uniquely combines top diversity values in (a) ancient lineage diversity and (b) cosmopolitan lineage diversity, suggesting that it has acted in the past as a biodiversity museum and source of global colonization. This is at least partly due to the interplay between latitudinal diversity gradients and continental connectivity patterns. However, the peak values in South China/North Indochina for cosmopolitan tetrapods and their sister lineages suggest that a key feature is also the availability of diverse climatic conditions. In particular, the north-south orientation of the mountain ranges here has allowed for rapid recolonization within the region following past climatic changes, resulting in high survival values and overall exceptional relict lineage diversity. From this starting point, global colonization occurred on multiple occasions. It is hoped that, with urgent action, the region can once again fulfill this function.
Most plants and animals alive today belong to lineages that have reached widespread status during the Cenozoic, with small numbers of ancient lineages with relictual distributions (Donoghue and Sanderson, 2015). The latter may be concentrated in isolated areas which have seen more stable climates, and a reduced chance of extinction during global extinction events (Procheş et al., 2015). Spread and diversification have followed each of the major mass extinctions in the past, generally with lineages diversifying on each occasion and most of them going extinct in the next. Which lineages have survived, where, and how soon and how widely they were able to spread has no doubt been influenced by continental arrangement (Donoghue, 2008). This has been the case especially with poorly dispersed groups, but to some extent even with well-dispersed ones. Besides dispersal abilities, which are group-specific, a factor relevant to overall biodiversity loss in mass extinction events has been the distribution of biodiversity at the time of each event, the ideal scenario being one where maximum biodiversity regions were least affected by the cosmic, geological, atmospheric, or ecological processes responsible for the mass extinction itself (see Johnson, 1993).
There is little doubt that human impacts on the environment are bound to leave a long-lasting mark on the global distribution of biotic lineages, and the Anthropocene has already been described as a mass extinction event, with current rates of extinction up to 1,000 times the background extinction rate (Barnosky et al., 2011; De Vos et al., 2015). Given rapid rates of habitat loss and degradation combined with direct species exploitation, there is no reason to assume that this will change without decisive action to prevent the numerous drivers of native diversity loss (Hughes, 2017). Various timeframes have been proposed as to how long anthropogenic activities will continue at the current pace, but irrespective of this, it is likely that, at some point, they will either cease or substantially relent (Watson and Watson, 2020). If measured as number of species, the proportion of biodiversity lost will be severe, but if viewing biodiversity as a number of phylogenetically or ecologically distinctive lineages, chances are that a few species from most of these will survive. This should be the case even in the most pessimistic biodiversity loss scenarios, albeit perhaps in only a fraction of their original ranges.
It is important to understand what the best sources for recolonization under such circumstances may be, by identifying refugia providing resilience to climate change (Keppel et al., 2012, 2015) as well as to other anthropogenic changes, and thus prioritize areas likely to maintain the greatest proportion of phylogenetic diversity (Keppel et al., 2018). Although the comparison is not perfect, a good place to start would be mapping regions that may have acted as refugia during past mass extinctions, or regions that conserve ancient lineages today, whether their survival of past upheavals happened in those precise areas or elsewhere (while keeping in mind the potential mismatch between past and present pressures, and even between diverse concurrent pressures; Mokany et al., 2017; Keppel et al., 2018). A second approach could be to map the greatest present-day concentrations of competitive lineages, such as those that have achieved worldwide distribution, and as such would have global recolonization potential, and try to gather information on their origination. To achieve this globally, it is necessary to collate distribution patterns for distinctive plant and animal lineages following diverse criteria, such as age, range size and ecological niches. Here we present evidence that at this point Southeast Asia appears to be the region conserving the greatest diversity of widespread as well as ancient lineages, and contend that it will consequently represent one of the most likely areas of origination for subsequent global colonization. This is, however, conditional to the relevant biotas surviving in the region, which is questionable given the disproportionate biodiversity loss currently taking place there (Hughes, 2017). Therefore, here we seek to understand the potential role of the Asian region in both acting as a refugium for ancient lineages, in addition to a source of cosmopolitan lineages, and discuss its role in acting as a cradle and museum for global biodiversity into the future.
In terms of species diversity, Southeast Asia is largely congruent with one of world’s three major tropical rainforest regions, and as such has top biodiversity values by numerous criteria, although in most groups it comes second to the Neotropics (Richards, 1973; Barthlott et al., 1996; Roll et al., 2017). When it comes to measures of phylogenetic and functional diversity, the information is fragmentary, and better mapped globally in vertebrates compared to plants – not to mention invertebrates. Patterns in tetrapod vertebrates differ substantially from one group to another, and depend on the precise metrics used, but Southeast Asia seldom stands out on its own. In amphibians, it is tied at the top with the tropical/warm parts of the Americas (Fritz and Rahbek, 2012). In birds and mammals, the region appears sub-average for a tropical rainforest region with most measures used (Davies and Buckley, 2012; Hawkins et al., 2012; Daru et al., 2019), albeit Borneo and Sulawesi show exceptional phylogenetically standardized trait beta diversity, and high levels of regional endemism may be reflected by small range sizes in some parts of the region (Mazel et al., 2017). None of these measures, however, are particularly relevant to colonization potential per se. High phylogenetic diversity values may reflect a diversity of ancient lineages,.
The distribution of plant and animal lineages that had already diverged prior to the last two mass extinctions has been analyzed by Procheş et al. (2015), and is re-mapped here in the case of plants, where the Southeast Asia peak is particularly strong (Figure 1). In plants, which are overall better dispersed than vertebrates (with the exception of birds), Southeast Asia represents the center of a continuum between the East Asian flora, itself with many North American links, and the Australasian one, harboring numerous Gondwanan relict lineages. For the deepest levels of divergence (201 Mya), with Southeast Asia, and Australia are the only regions of the planet with 5–6 major seed plant lineages, compared to 1–3 in the Afrotropics and Latin America. The high Southeast Asian plant lineage diversity is not strictly localized, but connected to high values that extend from Japan, through China, all the way to Queensland and New Caledonia. It can be argued that such small numbers, where angiosperms are represented as a single lineage, are not particularly meaningful when looking at the conservation of present-day plant diversity. However, this is the case both when looking at all seed plant lineages delimited at a cut-off value of 201 Mya, coinciding with the Triassic-Jurassic extinction event, and when looking at those that only went through the last mass extinction event, the end-Cretaceous event (66 Mya), in a subset of seed plants (subclass Campanulidae) which has the highest richness in the world with over 14 lineages throughout Southeast Asia.
Figure 1. Global patterns of plant diversity in ancient plant lineages that survived (A) the last, and (B) the last two mass extinctions. In (B), the map comprehensively covers all seed plants; in (A) it is restricted to one selected group, the Campanulidae. Redrawn from Procheş et al. (2015).
By definition, these lineages have widespread distributions, which may appear to limit the usefulness of analyzing their diversity patterns. However, if setting cut-off values that allow such lineages to be absent in up to 25–50% of world’s ecoregion-scale units, these absences are sufficient to illustrate what regions hold good potential in retaining a full inventory (Procheş and Ramdhani, 2013). This is particularly important considering that their near-worldwide coexistence has carved characteristic niches that these lineages fill through all or most of their ranges, meaning key roles in maintaining ecosystem function. Sadly, no study has summarized geographic patterns in cosmopolitan plants globally. Multi-regional studies (Stohlgren et al., 2011) indicate that homogenization in plant assemblages is happening far more rapidly compared to animals, and the uncertain status of some species (indigenous or alien) is indeed a hurdle in completing a list of plant lineages that are naturally cosmopolitan, particularly in monocots. In animals, most widespread lineages co-occur through vast areas of Eurasia and Africa, but many are absent in large portions of the Americas, and more than half are not found in Australia, New Zealand, and Madagascar. The greatest concentration, with almost complete inventories, is in South China and North Indochina. The remainder of Southeast Asia also hosts high numbers, but not necessarily higher than elsewhere in Eurasia. Possibly even more importantly, the same region – South China and North Indochina – is also topping the charts when looking at the distribution of lineages sister to cosmopolitan lineages, possibly indicative of origination in this region (Figure 2). Broad geographic range also has the potential of being indicative of specific traits, such as dispersal, fecundity, and niche breadth (Laube et al., 2013; Procheş et al., 2019). This needs to be weighed against the age of lineages – even in the case of species, but more so with multi-specific lineages, as even less competitive lineages may be able to attain cosmopolitan status if given enough time. However, if accounting for age in the mapping of cosmopolitan lineages, there should be good reasons to hope that, in future studies of such lineages, a functional component can be considered.
Figure 2. Global diversity maps for (A) widespread tetrapod lineages and (B) their sister lineages. Redrawn from Procheş and Ramdhani (2013).
The patterns described here in detail are focused on two rather specific contrasting methods of measuring biodiversity, and in one group of organisms each, albeit with some replication within that group – in the case of plants ancient lineage diversity across two mass extinction events, and in the case of cosmopolitan tetrapod lineages between the lineages themselves and their sister lineages. As the use of these measures has not been widely explored, additional evidence along either of the two lines would be most welcome. Even so, although Southeast Asia is the most diverse overall in both ancient and cosmopolitan lineages, patterns are somewhat different in the two cases, with the whole region having high values in one case, and only parts of it in the other. The differences suggest that, although the entire region has exceptional conservation value in a present-day context, in the long term, the highest value is to be placed on the northern parts of the region, particularly North Indochina and South China. This is in agreement with studies of evolutionary patterns within the region that are informed by paleoclimatic analyses (e.g., de Bruyn et al., 2014).
The South China/North Indochina hotspot in tetrapod lineage diversity is probably linked to the spectacular topographic heterogeneity found in this part of the region (Lei et al., 2015). This presumably influences biodiversity both directly, and via climatic effects. The latter could happen in two different ways. On a fine scale, topographic heterogeneity means climatic heterogeneity (Copeland and Harrison, 2015). In a broader context, the massive mountain chains at the eastern end of the Tibetan Plateau have also exerted an effect on the development of monsoon systems regionally (Ding et al., 2020). The convergence of multiple such systems here is likely to provide some buffering and to ensure consistently high precipitation (Spicer, 2017), although the precise future trajectories of the monsoon systems involved would need further investigation. It is nevertheless encouraging to see that changes in rainfall over the key Indochinese section of the region are unlikely to decrease due to anthropogenic climate change, and may in fact increase (Tangang et al., 2020).
The presence in this part of the region of deeply dissected landscapes such as the Hengduan Mountains is probably another key to the survival of moisture-reliant biota (e.g., Feng et al., 2020), which represent a large proportion of biodiversity in general, and of ancient lineages in particular. This has been clearly illustrated for the Chinese flora, where this area is no doubt the highest in terms of plant phylogenetic diversity (Lu et al., 2018). The mainly north-south orientation of the valleys and mountain ranges is also important (Li et al., 2011; Lei et al., 2015), meaning that climatic fluctuations can be weathered better by mobile but climate-sensitive biotas by migrating either altitudinally or lengthwise along the valleys (c.f. Médail and Diadema, 2009). This will presumably mitigate the impacts of oncoming climatic changes.
Beyond species and lineages, it would also be interesting to understand how recolonizing biotas may be able to reconstitute biotic communities (c.f. White et al., 2019) comparable to pre-Anthropocene ones. With cosmopolitan lineages being the backbone of most tetrapod vertebrate assemblages worldwide, community composition in this group is likely to be restorable in the post-Anthropocene on the basis of Southeast Asian survival, or conversely disproportionately disadvantaged by its loss. The combination of both high concentrations of regionally endemic and cosmopolitan lineages means that the continued loss of viable habitat in Southeast Asia may drive a disproportionate loss of phylogenetic diversity, which would consequently require millions of years to recover equivalent values should current rates of loss across much of the region continue.
Southeast Asia also has one of world’s most complete inventories of ancient plant lineages (Procheş et al., 2019), but the global mapping of more recent, mainly angiosperm, lineages is far from complete. There is also no information on how cosmopolitan plant lineages are represented there or elsewhere, which could help build an understanding of future plant community dynamics (Harrison et al., 2020), especially in the context of a changing climate. It is worth questioning to what extent the diversity of sister tetrapod lineages is indeed indicative of a Southeast Asian origination for currently cosmopolitan lineages. In terms of ancestral area reconstruction, a single sister lineage occurring in one area is not conclusive evidence of origination in that region, although multiple cases with the same area of sister lineage occurrence are starting to build a fairly strong case in that direction. However, to build a fully compelling case, ancestral area reconstruction analyses (Ree and Smith, 2008) would need to be conducted, and whilst analysis has been conducted within some lineages, it is still missing for the majority of taxa.
Although the information that forms the basis of this conclusion is fragmentary, it appears at this stage that Southeast Asia would indeed represent the highest-ranking region toward world’s recovery of pre-Anthropocene Cenozoic biodiversity distribution patterns. This is not good news. Multiple studies have already shown this region to be severely affected by recent human impacts, including habitat destruction and degradation, hunting and other forms of unsustainable resource uses (Sodhi et al., 2004; Wilcove et al., 2013; Harrison et al., 2016; Hughes, 2017). While some issues, such as the increasing area under oil palm cultivation and the plight of orangutans, are well known across the global conservation community, most other aspects of this environmental disaster remain poorly reflected in the media. Furthermore, it should be noted that whilst diversity of well-studied mammals such as primates may not be comparable to other tropical regions, bats make up over half diversity in many Asian forest systems (Hughes et al., 2012), and yet up to 70% of some taxa in this group may not have been described to date (Francis et al., 2010). Additionally, in terms of higher taxa and broader lineages, relative diversity and turnover have rarely been assessed, and it remains imperative to understand the phylogenetic diversity which could potentially be lost by the continued habitat destruction of this area. In terms of overall conservation research, Southeast Asia is clearly below global standards (Di Marco et al., 2017). All is not lost however; awareness of the dire situation the region finds itself in is improving, and a comprehensive list for research priorities (Coleman et al., 2019) has been compiled. In the context of the current study, we would additionally recommend testing the patterns presented here with data from additional taxa and metrics, to cement the assertion made here that Southeast Asia likely represents one of world’s primary macrorefugia [as defined by Keppel et al. (2012)].
Another key follow-up would be to assemble a list of endemic ancient lineages, including taxa which show relictual and restricted distributions for the region and map them across finer scales (Rossetto and Kooyman, 2005; Mokany et al., 2017), and explore how turnover within the region fits in with global lineage turnover (Kooyman et al., 2011). This, in combination with an improved trait-based approach (Copeland and Harrison, 2015; Ottaviani et al., 2020), would allow building an understanding of how various threats, and in particular climate change would affect them individually, and how effective the current protected area network and various practices may be in preserving them to maximize the conservation of phylogenetic diversity, especially keeping in mind the oncoming climatic change implications, particularly for coastal and montane diversity (Harrison and Noss, 2017). Furthermore, we highlight the need for these alternate metrics of richness to be a more frequent consideration in developing conservation priorities, as they provide the best hope of targeting areas with maximum phylogenetic richness, and also underscores the importance of Southeast Asia as a global priority under threat.
ŞP formulated the concept of this article and led the writing. SR did the mapping and assisted with writing. AH and LK assisted with conceptualization of the article and writing. All authors contributed to the article and approved the submitted version.
Research support was provided through the University of KwaZulu-Natal.
The authors declare that the research was conducted in the absence of any commercial or financial relationships that could be construed as a potential conflict of interest.
Sreekar Rachakonda and David Taylor are thanked for discussions.
Barnosky, A. D., Matzke, N., Tomiya, S., Wogan, G. O. U., Swartz, B., Quental, T. B., et al. (2011). Has the Earth’s sixth mass extinction already arrived? Nature 471, 51–57. doi: 10.1038/nature09678
Barthlott, W., Lauer, W., and Placke, A. (1996). Global distribution of species diversity in vascular plants: towards a world map of phytodiversity. Erdkunde 50, 317–327. doi: 10.3112/erdkunde.1996.04.03
Coleman, J. L., Ascher, J. S., Bickford, D., Buchori, D., Cabanban, A., Chrisholm, R. A., et al. (2019). Top 100 research questions for biodiversity conservation in Southeast Asia. Biol. Conserv. 234, 211–220. doi: 10.1016/j.biocon.2019.03.028
Copeland, S. M., and Harrison, S. P. (2015). Identifying plant traits associated with topographic contrasts in a rugged and diverse region (Klamath-Siskiyou Mts, OR, USA). Ecography 38, 569–577. doi: 10.1111/ecog.00802
Daru, B. H., le Roux, P. C., Gopalraj, J., Park, D. S., Holt, B. G., and Greve, M. (2019). Spatial overlaps between the global protected areas network and terrestrial hotspots of evolutionary diversity. Glob. Ecol. Biogeogr. 28, 757–766. doi: 10.1111/geb.12888
Davies, T. J., and Buckley, L. B. (2012). Exploring the phylogenetic history of mammal species richness. Glob. Ecol. Biogeogr. 21, 1096–1105. doi: 10.1111/j.1466-8238.2012.00759.x
de Bruyn, M., Stelbrink, B., Morley, R. J., Hall, R., Carvalho, G. R., Cannon, C. H., et al. (2014). Borneo and Indochina are major evolutionary hotspots for Southeast Asian biodiversity. Syst. Biol. 63, 879–901. doi: 10.1093/sysbio/syu047
De Vos, J. M., Joppa, L. N., Gittleman, J. L., Stephens, P. R., and Pimm, S. L. (2015). Estimating the normal background rate of species extinction. Conserv. Biol. 29, 452–462. doi: 10.1111/cobi.12380
Di Marco, M., Chapman, S., Althor, G., Kearney, S., Besancon, C., Butt, N., et al. (2017). Changing trends and persisting biases in three decades of conservation science. Glob. Ecol. Conserv. 10, 32–42. doi: 10.1016/j.gecco.2017.01.008
Ding, W.-N., Ree, R. H., Spicer, R. A., and Xing, Y.-W. (2020). Ancient orogenic and monsoon-driven assembly of the world’s richest temperate alpine flora. Science 369, 578–581. doi: 10.1126/science.abb4484
Donoghue, M. J. (2008). A phylogenetic perspective on the distribution of plant diversity. Proc. Natl. Acad. Sci. U.S.A. 105, 11549–11555. doi: 10.1073/pnas.0801962105
Donoghue, M. J., and Sanderson, M. J. (2015). Confluence, synnovation, and depauperons in plant diversification. New Phytol. 207, 260–274. doi: 10.1111/nph.13367
Feng, G., Huang, X., Mao, L., Wang, N., Yang, X., and Wang, Y. (2020). More endemic birds occur in regions with stable climate, more plant species and high altitudinal range in China. Avian Res. 11:17. doi: 10.1186/s40657-020-00203-y
Francis, C. M., Borisenko, A. V., Ivanova, N. V., Eger, J. L., Lim, B. K., Guillén-Servent, A., et al. (2010). The role of DNA barcodes in understanding and conservation of mammal diversity in Southeast Asia. PLoS One 5:e12575. doi: 10.1371/journal.pone.0012575
Fritz, S. A., and Rahbek, C. (2012). Global patterns of amphibian phylogenetic diversity. J. Biogeogr. 39, 1373–1382. doi: 10.1111/j.1365-2699.2012.02757.x
Harrison, R. D., Sreekar, R., Brodie, J. F., Brook, S., Luskin, M., O’Kelly, H., et al. (2016). Impacts of hunting on tropical forests in Southeast Asia. Conserv. Biol. 30, 972–981. doi: 10.1111/cobi.12785
Harrison, S., and Noss, R. (2017). Endemism hotspots are linked to stable climatic refugia. Ann. Bot. 119, 207–214. doi: 10.1093/aob/mcw248
Harrison, S., Spasojevic, M. J., and Li, D. (2020). Climate and plant community diversity in space and time. Proc. Natl. Acad. Sci. U.S.A. 117, 4464–4470. doi: 10.1073/pnas.1921724117
Hawkins, B. A., McCain, C. M., Davies, T. J., Buckley, L. B., Anacker, B. L., Cornell, H. V., et al. (2012). Different evolutionary histories underlie congruent species richness gradients of birds and mammals. J. Biogeogr. 39, 825–841. doi: 10.1111/j.1365-2699.2011.02655.x
Hughes, A. C. (2017). Understanding the drivers of Southeast Asian biodiversity loss. Ecosphere 8:e01624. doi: 10.1002/ecs2.1624
Hughes, A. C., Satasook, A., Bates, P. J. J., Bumrungsri, S., and Jones, G. (2012). The projected effects of climatic and vegetation changes on the distribution and diversity of Southeast Asian bats. Glob. Change Biol. 18, 1854–1865. doi: 10.1111/j.1365-2486.2012.02641.x
Keppel, G., Mokany, K., Wardell-Johnson, G., Phillips, B. L., Welbergen, J. A., and Reside, A. E. (2015). The capacity of refugia for conservation planning under climate change. Front. Ecol. Environ. 13:106–112. doi: 10.1890/140055
Keppel, G., Ottaviani, G., Harrison, S., Wardell-Johnson, G. W., Marcantonio, M., and Mucina, L. (2018). Towards an eco-evolutionary understanding of endemism hotspots and refugia. Ann. Bot. 122, 927–934. doi: 10.1093/aob/mcy173
Keppel, G., Van Niel, K. P., Wardell−Johnson, G. W., Yates, C. Y., Byrne, M., Mucina, L., et al. (2012). Refugia: identifying and understanding safe havens for biodiversity under climate change. Glob. Ecol. Biogeogr. 21, 393–404. doi: 10.1111/j.1466-8238.2011.00686.x
Kooyman, R. M., Rossetto, M., Cornwell, W., and Westoby, M. (2011). Phylogenetic tests of community assembly across regional to continental scales in tropical and subtropical rain forests. Glob. Ecol. Biogeogr. 20, 707–716. doi: 10.1111/j.1466-8238.2010.00641.x
Laube, I., Korntheuer, H., Schwager, M., Trautmann, S., Rahbek, C., and Böhning-Gaese, K. (2013). Towards a more mechanistic understanding of traits and range sizes. Glob. Ecol. Biogeogr. 22, 233–241. doi: 10.1111/j.1466-8238.2012.00798.x
Lei, F., Qu, Y., Song, G., Alström, P., and Fjeldså, J. (2015). The potential drivers in forming avian diversity hotspots in the East Himalaya Mountains of Southwest China. Integr. Zool. 10, 171–181. doi: 10.1111/1749-4877.12121
Li, Y., Zhai, S.-N., Qui, Y., Guo, Y.-P., Ge, X.-J., and Comes, H. P. (2011). Glacial survival east and west of the ‘Mekong–Salween Divide’ in the Himalaya–Hengduan Mountains region as revealed by AFLPs and cpDNA sequence variation in Sinopodophyllum hexandrum (Berberidaceae). Mol. Phylogenet. Evol. 59, 412–424. doi: 10.1016/j.ympev.2011.01.009
Lu, L.-M., Mao, L.-F., Yang, T., Ye, J.-F., Liu, B., Li, H.-L., et al. (2018). Evolutionary history of the angiosperm flora of China. Nature 554, 234–238. doi: 10.1038/nature25485
Mazel, F., Wüest, R. O., Gueguen, M., Renaud, J., Ficetola, G. F., Lavergne, S., et al. (2017). The geography of ecological niche evolution in mammals. Curr. Biol. 27, 1369–1374. doi: 10.1016/j.cub.2017.03.046
Médail, F., and Diadema, K. (2009). Glacial refugia influence plant diversity patterns in the Mediterranean Basin. J. Biogeogr. 36, 1333–1345. doi: 10.1111/j.1365-2699.2008.02051.x
Mokany, K., Jordan, G. J., Harwood, T. D., Harrison, P. A., Keppel, G., Gilfedder, L., et al. (2017). Past, present and future refugia for tasmania’s palaeoendemic flora. J. Biogeogr. 44, 1537–1546. doi: 10.1111/jbi.12927
Ottaviani, G., Keppel, G., GÖtzenberger, L., Harrison, S., Opedal, Ø. H., Conti, L., et al. (2020). Linking plant functional ecology to island biogeography. Trends Plant Sci. 25, 329–339. doi: 10.1016/j.tplants.2019.12.022
Procheş, Ş, and Ramdhani, S. (2013). Eighty three lineages that took over the world: a first review of terrestrial cosmopolitan tetrapods. J. Biogeogr. 40, 1819–1831. doi: 10.1111/jbi.12125
Procheş, Ş, Ramdhani, S., Ali, J. R., Perera, S. J., and Gairola, S. (2015). Global hotspots in the present-day distribution of ancient animal and plant lineages. Sci. Rep. 5:15457. doi: 10.1038/srep15457
Procheş, Ş, Sukri, R. S., Jaafar, S. M., Sieben, E. J. J., Zaini, N. H., Abas, N., et al. (2019). Soil niche of rain forest plant lineages: implications for dominance on a global scale. J. Biogeogr. 46, 2378–wor2387. doi: 10.1111/jbi.13656
Ree, R. H., and Smith, S. A. (2008). Maximum likelihood inference of geographic range evolution by dispersal, local extinction, and cladogenesis. Syst. Biol. 57, 4–14. doi: 10.1080/10635150701883881
Richards, P. W. (1973). “Africa, the ‘odd man out’,” in Tropical Forest Ecosystems in Africa and South America: A Comparative Review, eds B. J. Meggers, E. S. Ayensu, and W. D. Duckworth (Washington, DC: Smithsonian Institute Press), 21–26.
Roll, U., Feldman, A., Novosolov, M., Allison, A., Bauer, A. M., Bernard, R., et al. (2017). The global distribution of tetrapods reveals a need for targeted reptile conservation. Nat. Ecol. Evol. 1, 1677–1682. doi: 10.1038/s41559-017-0332-2
Rossetto, M., and Kooyman, R. M. (2005). The tension between dispersal and persistence regulates the current distribution of rare palaeo-endemic rain forest flora: a case study. J. Ecol. 93, 906–917. doi: 10.1111/j.1365-2745.2005.01046.x
Sodhi, N. S., Koh, L. P., Brook, B. W., and Ng, P. K. L. (2004). Southeast Asian biodiversity: an impending disaster. Trends Ecol. Evol. 19, 654–660. doi: 10.1016/j.tree.2004.09.006
Spicer, R. A. (2017). Tibet, the Himalaya, Asian monsoons and biodiversity? In what ways are they related? Plant Divers. 39, 233–244. doi: 10.1016/j.pld.2017.09.001
Stohlgren, T. J., Pyšek, P., Kartesz, J., Nishino, M., Pauchard, A., Winter, M., et al. (2011). Widespread plant species: natives versus aliens in our changing world. Biol. Invasions 13, 1931–1944. doi: 10.1007/s10530-011-0024-9
Tangang, F., Chung, J. X., Juneng, L., Supari Salimun, E., Ngai, S. T., Jamaluddin, A. F., et al. (2020). Projected future changes in rainfall in Southeast Asia based on CORDEX–SEA multi-model simulations. Clim. Dyn. 55, 1247–1267. doi: 10.1007/s00382-020-05322-2
Watson, M. J., and Watson, D. M. (2020). Post-Anthropocene conservation. Trends Ecol. Evol. 35, 1–3. doi: 10.1016/j.tree.2019.10.006
White, A. E., Dey, K. K., Mohan, D., Stephens, M., and Price, T. D. (2019). Regional influences on community structure across the tropical-temperate divide. Nat. Commun. 10:2646. doi: 10.1038/s41467-019-10253-6
Keywords: ancient lineages, Anthropocene extinction, Southeast Asia, widespread lineages, recolonization
Citation: Procheş Ş, Ramdhani S, Hughes AC and Koh LP (2021) Southeast Asia as One of World’s Primary Sources of Biotic Recolonization Following Anthropocene Extinctions. Front. Ecol. Evol. 9:634711. doi: 10.3389/fevo.2021.634711
Received: 28 November 2020; Accepted: 11 February 2021;
Published: 19 March 2021.
Edited by:
Gianluigi Ottaviani, Institute of Botany (ASCR), CzechiaReviewed by:
Milan Chytry, Masaryk University, CzechiaCopyright © 2021 Procheş, Ramdhani, Hughes and Koh. This is an open-access article distributed under the terms of the Creative Commons Attribution License (CC BY). The use, distribution or reproduction in other forums is permitted, provided the original author(s) and the copyright owner(s) are credited and that the original publication in this journal is cited, in accordance with accepted academic practice. No use, distribution or reproduction is permitted which does not comply with these terms.
*Correspondence: Şerban Procheş, c2V0YXBpb25AZ21haWwuY29t
Disclaimer: All claims expressed in this article are solely those of the authors and do not necessarily represent those of their affiliated organizations, or those of the publisher, the editors and the reviewers. Any product that may be evaluated in this article or claim that may be made by its manufacturer is not guaranteed or endorsed by the publisher.
Research integrity at Frontiers
Learn more about the work of our research integrity team to safeguard the quality of each article we publish.