- 1Schiermeier Olentangy River Wetland Research Park, School of Environment and Natural Resources, The Ohio State University, Columbus, OH, United States
- 2Department of Biology, University of Nebraska at Omaha, Omaha, NE, United States
Larval aquatic insects are used to assess water quality, but less attention is paid to their adult, terrestrial life stage, which is an important food resource for declining aerial insectivorous birds. We used open-access water-quality, aquatic-invertebrate, and bird-survey data to study how impaired water quality can emanate from streams and lakes through changes in aquatic insect communities across the contiguous United States. Emergent insect relative abundance was highest across the West, in northern New England, and the Carolinas in streams, and highest near the Great Lakes, parts of the Southwest, and northern New England for lakes. Emergent insects declined with sedimentation, roads, and elevated ammonium concentrations in streams, but not lakes. The odds that a given taxon would be non-emergent increased by up to 2.0× as a function of pollution tolerance, underscoring the sensitivity of emergent aquatic insects to water-quality impairment. However, relationships between bird populations and emergent insects were generally weak for both streams and lakes. For streams, we observed the strongest positive relationships for a mixture of upland and riparian aerial insectivorous birds such as Western Wood-Pewee, Olive-sided Flycatcher, and Acadian Flycatcher and the strongest negative association for Purple Martin. Different avian insectivores responded to emergent insect abundances in lakes (e.g., Barn Swallow, Chimney Swift, Eastern Wood-Pewee, Common Nighthawk). In both streams and lakes, we observed stronger, but opposing, relationships between several aerial insectivores and the relative abundance of sensitive insect orders (E)phemeroptera, (P)lecoptera, and (T)richoptera (positive), and pollution tolerant individuals (negative). Overall, our findings indicate that emergent insects are negatively correlated with pollution tolerance, suggesting a large-scale loss of this nutritional subsidy to terrestrial environments from impaired aquatic ecosystems. While some bird populations tracked scarcities of emergent aquatic insects, especially EPT taxa, responses varied among species, suggesting that unique habitat and foraging behaviors likely complicated these relationships. Strengthening spatial and temporal concordance between emergent-insect and bird-survey data will improve our ability to interpret species-level responses over time. Thus, our analysis highlights the need for developing conservation and biomonitoring strategies that consider the cross-ecosystem effects of water quality declines for threatened insectivorous avifauna and other terrestrial wildlife.
Introduction
The health of aquatic ecosystems is strongly influenced by their surrounding landscape—a foundational idea for our current understanding of streams and lakes that is often applied to the conservation and management of these ecosystems in the United States (e.g., Hynes, 1975; Wohl, 2017; Sullivan et al., 2019). The influences that act at the scale of entire watersheds are important for explaining patterns of the physical and chemical characteristics that set the template for biological interactions that occur within lakes and streams (King et al., 2005). Widespread human activities within watersheds, such as urbanization and industrial-scale agriculture, are associated with a suite of undesirable effects in receiving waters, such as increased point and non-point source pollution, altered hydrology, and ultimately, reduced aquatic biodiversity (Wenger et al., 2009; Dubrovsky et al., 2010; Brown and Froemke, 2012).
Indeed, recent biological assessments of wadeable streams and lakes conducted by the US Environmental Protection Agency (EPA; National Aquatic Resource Surveys) suggest that 46% of streams surveyed were in “poor” condition (US EPA, 2016), and 57% of lakes surveyed were considered “moderately” to “most” disturbed (US EPA, 2017). These condition assessments were based on well established, multimetric indices of benthic macroinvertebrates living within the stream or in the littoral (near-shore) zone of lakes; such indices are widely recognized for their utility as integrative measures of detrimental human activities that affect the health of receiving waters in the land-to-water direction. Yet, the reciprocal, water-to-land effects of poor water quality on adjacent terrestrial (riparian) habitats has only recently begun to gain traction as an important conservation paradigm that recognizes how aquatic-to-terrestrial ecological linkages are critical for the functioning of both aquatic and adjacent riparian systems (Walters et al., 2008; Kraus et al., 2014; Sullivan and Manning, 2019).
Aerial insectivorous birds—including swallows, swifts, nightjars, and flycatchers—may be especially susceptible to the effects of poor water quality that prompt reduced or altered abundances of biphasic emergent insects. This guild of birds is experiencing widespread population declines (reviewed in Nebel et al., 2010; Sauer et al., 2017a), but identifying the mechanisms underpinning these declines remains a difficult task. Numerous explanations for aerial insectivore declines have been proposed, including nesting and over-wintering habitat loss, airborne pollutants, widespread pesticide use associated with insect-population collapse, climatic shifts, and land-use change (Hallmann et al., 2014, 2017; Sullivan et al., 2021); many of these causes are likely to be acting in concert (Spiller and Dettmers, 2019). Whereas the precise causes for declines in several aerial insectivorous birds remain unknown, a shared reliance on flying insects and population declines across species within the foraging guild implicates decreasing or fluctuating insect food quantity or quality (Paquette et al., 2013; Twining et al., 2018; Schilke et al., 2020; but see Imlay et al., 2017). Although aerial insectivores feed on a combination of terrestrial and aquatic insects, many species exhibit a nutritional reliance on subsidies of aquatic emergent insect prey (Gray, 1993; Iwata et al., 2003). Emergent insects may also confer a substantial energetic benefit over terrestrial insects (Twining et al., 2018, 2019), and aerial insectivores may time breeding to coincide with pulses of emergent insects (Twining et al., 2018). Thus, considering relationships between aerial insectivore ecology and emergent-insect subsidies could be a critical component of insectivorous bird conservation strategies.
Insect facilitated aquatic-to-terrestrial subsidies have been investigated in both streams (Baxter et al., 2005; Kautza and Sullivan, 2015) and lakes (Gratton et al., 2008; Gratton and Vander Zanden, 2009). Although benthic invertebrates in streams are generally more productive than in lakes, flux estimates are on the order of 2.5× greater for lakes than for streams because stream width is smaller on average than lake radius (Gratton and Vander Zanden, 2009). Nonetheless, both lakes and streams are important for aerial insectivorous birds given variable life-history and foraging strategies across the guild (e.g., Dreelin et al., 2018). For instance, riparian swallows such as Tree Swallows (Tachycineta bicolor) typically prefer to forage over open water habitats of lakes or rivers vs. smaller, canopied streams (Alberts et al., 2013).
Physical and chemical water quality and its landscape-scale drivers may be predictive of the relationship between aerial insectivores and emergent-insect subsidies. Larval macroinvertebrates are widely used to assess and monitor aquatic ecosystem integrity (e.g., Hill et al., 2017), which can be impaired by human perturbations such as land-use change (Sterling et al., 2016). Changes in land use commonly lead to altered hydrology, increased sediment and contaminant loads, and loss of biological integrity (Allan, 2004; Walsh et al., 2005). Benthic macroinvertebrate taxa with flying adult stages can be especially sensitive to stressors associated with human activities including sedimentation, heavy-metal pollution, and increased salinity (Greig et al., 2012; Kraus et al., 2014; Schröder et al., 2015), potentially disrupting aquatic-to-terrestrial trophic linkages (Greenwood and Booker, 2016). However, our understanding of the ways in which human-induced stressors can affect aquatic subsidies—and the consequences of alterations in these subsidies to avifauna and other terrestrial wildlife—remain in the initial stages (Kautza and Sullivan, 2015; Greenwood and Booker, 2016). Likewise, the combined effects of multiple stressors and their relationships to important functional emergent-insect traits (e.g., pollution sensitivity) that mediate cross-boundary fluxes of energy and nutrients are unresolved.
Benthic invertebrates are widely used to develop multimetric indicators of water quality because these organisms are relatively long-lived, immobile, and display varying responses to the suite of stressors that can impair water quality; thus, aquatic invertebrates integrate physical and chemical stressors within streams, lakes, and rivers (Poff et al., 2006; Qian et al., 2012; Hill et al., 2017). Certain life-history characteristics may make these taxa more or less susceptible to poor water quality (Yuan, 2004) with the underlying assumption that specific traits of benthic invertebrates confer reduced survival or reproductive ability in response to environmental stressors. Key traits include the number of development stages [i.e., 3–4 life stages (hemi- vs. holometabolous)], breathing apparati, trophic relationships, armoring, and others. Among benthic invertebrates, several taxa that emerge as adults tend to have specialized habitat requirements (e.g., larger cobbles and/or fast moving, turbulent water), delicate wingpads and gills, and prolonged pupation (Merritt et al., 2008). Together, these traits suggest that emergent insect taxa could be more susceptible to environmental stressors associated with impaired water quality (Greenwood and Booker, 2016).
In this analysis, we test the hypothesis that conservation paradigms would benefit from considering the reciprocal cross-boundary relationships among watershed land use, water quality, and the abundance of aquatic insects with an emergent adult stage that are important for aerial insectivorous birds and potentially other terrestrial wildlife populations. First, we predicted that impaired water quality, integrating multiple stressors of the surrounding landscape, would disproportionately affect emergent insects (Greenwood and Booker, 2016). In turn, we predicted that variability in the distribution and relative abundance of emergent insects (Paquette et al., 2013; Carlson et al., 2016) would track variability in the abundance of aerial insectivorous birds, especially for riparian obligate species. We also expected that aerial insectivores would respond more to insects emerging from lakes vs. streams owing to greater biomass of fluxes from open-water habitats.
To test these predictions, we used publicly available benthic invertebrate data sets that also contained corresponding watershed-scale predictors and stressor variables. We then linked patterns of physical and chemical water quality and benthic invertebrate communities to aerial insectivorous birds using the U.S. Geological Survey Breeding Bird Survey data set (as synthesized by Smith et al., 2015 and Sauer et al., 2017b). We focused on 21 aerial insectivorous bird species with previously documented annual population abundance estimates (e.g., Smith et al., 2015). To establish points of reference, we also compared aerial insectivore patterns with two granivorous and two gleaner/bark-probing (i.e., primarily consume non-flying terrestrial insects) species with the expectation that these species would not reflect changes in water quality and emergent insects.
Materials and Methods
Benthic Macroinvertebrates
We accessed publicly available benthic macroinvertebrate data from the contiguous United States collected by the US EPA as part of the National Aquatic Resource Surveys Wadeable Streams Assessment conducted in 2000–2004 (US EPA, 2006), which included data from 1,325 sites within 18 hydrologic units (HUC4). Benthic macroinvertebrates were sampled semi-quantitatively within 11, 0.09-m2 locations at each stream site using standard sampling equipment such as 500-μm D-frame nets. Sub-samples (up to 300 individuals) of macroinvertebrates from each sample were enumerated and identified to the lowest possible taxonomic unit (typically genus; US EPA, 2006).
We assigned aquatic macroinvertebrate taxa to emergence/non-emergence groups based on family-level categorizations (see Supplementary Material for full list of families). All emergent taxa were from the Class Insecta; non-emergent families included non-insects (e.g., orders Gastropoda and Amphipoda), and some Hemipterans and Coleopterans (e.g., true bugs of the family Gerridae). We calculated the relative abundance of emergent insects and non-emergent insects, and examined relative abundance of the sensitive insect orders Ephemeroptera, Plecoptera, and Trichoptera; (EPA variable name = “EPT_PIND”), and the relative abundance of pollution tolerant insect taxa [as defined by the EPA in the data sets; variable name = “TOLRPIND” or the percentage of individuals in the sample classified as tolerant to poor water quality (US EPA, 2006)]. We averaged these response variables by state to coincide with available spatial information in the Breeding Bird Survey data (see section “Aerial Insectivorous Birds”).
We also accessed benthic macroinvertebrate data collected as part of the National Lakes Assessment (NLA; US EPA, 2010). Briefly, we gathered available benthic macroinvertebrate community, basin, and water chemistry data from 1,210 lake sites across the contiguous United States surveyed in 2007. Benthic macroinvertebrates were sampled from 1 linear meter within the dominant habitat of the littoral zone of each lake using 500-μm D-frame nets (US EPA, 2007). We assigned lake macroinvertebrate taxa to emergence/non-emergence groups based on the same family-level categorizations we used with stream macroinvertebrates. We calculated the relative abundance of emergent and non-emergent insects, and examined reported relative abundances of the sensitive insect orders (EPT), and the relative abundances of pollution tolerant insect taxa (as defined by the EPA). We also averaged these response variables by state to coincide with available spatial information in the Breeding Bird Survey data (see section “Aerial Insectivorous Birds”). All data, metadata, and details about methods for EPA stream and lake macroinvertebrate sampling efforts can be found here: https://www.epa.gov/national-aquatic-resource-surveys/data-national-aquatic-resource-surveys.
Water Quality and Land Use
As part of the stream benthic macroinvertebrate sampling, site characteristics were recorded, and several physical and chemical water-quality metrics were measured. Land use was estimated from the National Land Cover Dataset according to EPA methods (US EPA, 2006). We assigned watershed-level impervious surface cover data to EPA site locations using the StreamCat dataset (Hill et al., 2016). Basin and water chemistry data included in the NLA (lake) datasets were different than for the Wadeable Streams Assessment (e.g., variables not applicable to streams are included—pelagic chlorophyll a, Secchi depth), but also included similar variables (e.g., % basin agriculture, total phosphorus concentrations). Several of these variables were highly skewed; we log-transformed variables as appropriate according to visual inspection of normal quantile-quantile (Q-Q) plots (provided in the Supplementary Material). As with invertebrate response variables, we averaged water quality and watershed metrics by state to coincide with aerial insectivorous bird data.
Aerial Insectivorous Birds
We used published data sets of modeled annual indices of abundance for aerial insectivorous bird species from Sauer et al. (2017b) (see Supplementary Material for a full list of the species considered in this analysis) that were analyzed from data collected as part of the US Geological Survey Breeding Bird Survey program1. Modeled annual indices were based on hierarchical models as described by Sauer et al. (2017b), and defined in Sauer and Link (2011). The annual indices of abundance can be interpreted as an indicator of the number of birds per survey route in a given region during a given year. We note that they are non-integers and are not zero inflated, thus Poisson or negative binomial models that are typically used for count data were not applicable in this context. We analyzed annual indices of abundance for 21 aerial insectivore species (see Supplementary Table 2) and a set of four non-aerial insectivore species as points of reference: two granivorous bird species [American Goldfinch (Spinus tristis); Mourning Dove (Zenaida macroura)] and two gleaner/bark-probing species [i.e., more likely to consume terrestrial insects; Gray Catbird (Dumetella carolinensis), White-breasted Nuthatch (Sitta carolinensis)]. These 4 species were chosen because of their non-aerial-insect based diets and their broad distributions, with ranges that encompass all or a majority of the contiguous US. We examined relationships between annual indices of abundance made available by the BBS for the years that coincided with the final years of the Wadeable Streams Assessment survey (2004) and National Lakes Assessment (2007) (see section “Statistical Analysis”).
Statistical Analysis
We used logistic regression to test the relationship between pollution tolerance and the emergence trait. Specifically, we categorized each invertebrate taxon observed in the EPA data set according to a binary response (emergent/non-emergent), and then related this binary variable to assigned pollution tolerance values that ranged from 0 to 10, with 0 representing an extremely sensitive organism, and 10 representing the greatest pollution tolerance (Yuan, 2004). We interpreted the coefficient of the binomial regression as the increase in the log-odds that a given taxon has the emergence trait per unit increase in pollution tolerance value.
We used multiple linear regression and multi-model selection within an information-theoretic approach to identify best-supported, state-level predictors of the relative abundance of emergent insects (Feld et al., 2016). Given the number of responses and predictors tested, we opted for maximum likelihood approaches at this stage of the analysis because they are more efficient from a computational standpoint, but useful inferences can still be drawn. For streams and lakes, the responses considered were relative abundances of emergent and non-emergent insects. For water-quality variables, we identified 27 (stream) and 12 (lake) watershed/basin- and site-level predictors of benthic macroinvertebrate taxonomic and functional trait abundance. Our predictors included land-use metrics such as urban or agricultural land cover (%), which we considered to encompass drivers of multiple stressors as well as act as stressors in their own right (Feld et al., 2016). We also included chemical/nutrient (e.g., conductivity, chloride, total nitrogen, total phosphorus), and physical water-quality data [turbidity and habitat variables related to benthic substrate (% sand and fines)]. A similar subset of variables was identified a priori for lakes.
We accounted for multicollinearity among the 27 stream predictors using correlation analysis; all predictors with correlation coefficients <0.75 were retained (vifcor function in R, e.g., Feld et al., 2016). We used the package MuMIn version 1.43.17 (Bartoń, 2020) and associated function dredge in R to generate subsets of models with all possible combinations of predictors selected from a global model. We retained models with the strongest support based on the differences in Akaike’s Information Criterion corrected for small sample size (ΔAICc) values <2 (Burnham and Anderson, 2002). We used model averaging and present weighted averages for parameter estimates for this subset of best-supported models (Burnham and Anderson, 2002). Global models for stream emergent insects included chloride (Cl–), total phosphorus (TP), turbidity, ammonium (NH4-N), sulfate (SO42–), nitrate (NO3-N),% sand and fines, watershed area (km2), road density, elevation (m), and precipitation (mm). Predictors were standardized using z-scores to compare among variables measured with different scales and units, and log-transformed if necessary to meet assumptions of normality and homogeneity of variance (see Supplementary Material). For lakes, we used a different global model, based on a priori selection of common measures of lake trophic state [Secchi depth, pelagic chlorophyll a, total nitrogen (TN), TP], basin land use and characteristics (% developed, crop, basin area), and indicators of lake acidification or salinization (pH, acid neutralizing capacity, sulfate [SO42–], conductivity, chloride). See Supplementary Material for further details about this approach and a full list of variables considered in this part of the analysis.
We log-transformed the annual indices of bird abundances for the 25 bird species in 2004 and 2007 (n = 837 for both years) because assumptions of normality were not met (Kolmogorov-Smirnov test, D = 0.545, 0.542, respectively, P < 0.001 in both cases; inspection of Q-Q plots showed right-skewness; see Supplementary Figures 3A,B), and we excluded one zero annual index value in each year [i.e., Common Nighthawk (Chordeiles minor), annual index was 0 in Massachusetts in both years]. We then used a Bayesian model to estimate posterior probabilities for US state-level bird species abundance in 2004 and 2007 using the Hamiltonian Monte Carlo algorithm in rstan version 2.19.3 (Stan Development Team, 2020) via the package brms version 2.12.0 in R (Bürkner, 2017). We recognize that our use of state-level annual indices of bird abundance as our primary spatial scale for this analysis has certain drawbacks (i.e., political boundaries have no significance for birds and insects)—however, more ecologically appropriate watershed-level bird population data that can be matched to watershed-level benthic invertebrate data are currently lacking. Further, using state boundaries has some advantages, including engendering stronger connections to broader societal conservation actions and quickly identifying spatial patterns that warrant closer investigation using watershed-level data. We used Bayesian inference at this stage of the analysis as opposed to maximum likelihood methods to enable more direct, but nuanced, interpretations of the probability of water-quality effects on bird population trends (e.g., a 90% credible interval that marginally overlaps zero still implies close to 90% probability of an effect). Models included random effects for each species/state combination to generate a posterior probability distribution for mean abundance for each bird species within a given state [n = 25 bird species, minimum number of states = 7 (see Supplementary Table 2)]. All models were run for 2,000 iterations (after 1,000 warmup iterations), with 4 chains and a thinning rate of 1.
We related the relative abundance (streams and lakes) of both emergent and non-emergent insects to aerial insectivore annual indices of their abundance in the year matching the Wadeable Streams Assessment survey (2004) using univariate Bayesian linear models (again with brms). We found that annual indices were not significantly different among years from 2000 to 2009, thus, we limited this analysis to 1 year for both streams (2004) and lakes (2007). Predictor variables were standardized using z-scores to allow for direct comparison of their effects on bird population trends. We generated models with group-level parameter estimates for all 25 species that included a random effect for a categorical variable, which separated them into habitat groups (riparian obligate, riparian facultative, upland, and non-aerial insectivore) that allowed us to test for differences in responses to emergent insects among bird species that used different habitats or feeding behaviors. In all cases, we examined the 90% credible intervals (CI) for the posterior probability distributions of species-level coefficient estimates for random effects [i.e., coefficients presented here were the sum of the global fixed effect for a given predictor (e.g., relative abundance emergent insects), and the species-specific parameter estimate (i.e., random effect)]; parameter CIs that did not contain zero were considered evidence of a trend.
Results
Emergent Insects Highly Susceptible to Poor Water Quality
The relative abundance of emergent insects was negatively correlated with the proportion of tolerant macroinvertebrates in streams (Pearson’s r = −0.798) and lakes (Pearson’s r = −0.352). In addition, the relative abundance of emergent insects was positively related to the relative abundance of the pollution sensitive insect orders Ephemeroptera, Plecoptera, and Trichoptera in both streams (Pearson’s r = 0.704) and lakes (Pearson’s r = 0.305). We found that the odds that a given aquatic invertebrate taxon would be non-emergent vs. emergent in streams increased as a function of assigned pollution tolerance values. As pollution tolerance value increased, the odds of a taxon exhibiting non-emergence traits increased by 1.75× (logistic regression, ecoef = e0.560 = 1.75 [95% CI = 1.70–1.77], P < 0.001). We found a similar pattern for invertebrates sampled from lakes, where the odds of a given taxa exhibiting non-emergence increased by 2.01× per unit increase in pollution tolerance value (logistic regression, ecoef = e0.702 = 2.01 [95% CI = 1.99–2.03).
Spatial Distribution and Drivers of Emergent Insect Relative Abundance
The relative abundance of stream emergent insects was generally lower in the Midwest, Great Lakes, and South-Central U.S. (Figure 1A). For lakes, emergent-insect relative abundance appeared to be generally higher around the Great Lakes, as well as in parts of the Southwest and northern New England (Figure 1B). In some cases, stream and lake relative abundances exhibited strong contrasts at the state level. For instance, we observed high stream emergent-insect relative abundance in Maine and Washington, but among the lowest relative abundances in lakes. On the other hand, states like Florida, Louisiana, and North Dakota supported among the lowest relative abundances of emergent insects from both lakes and streams.
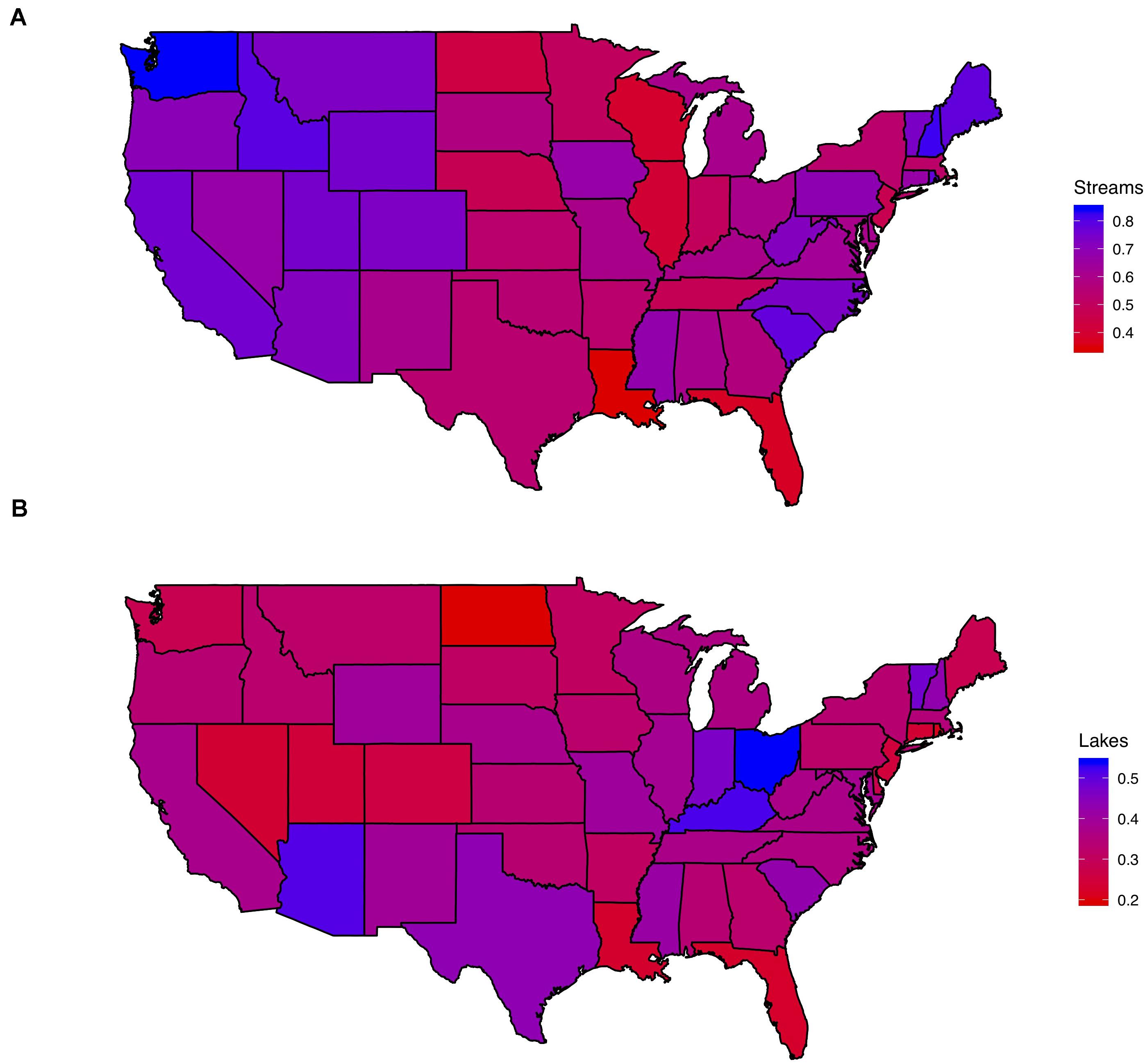
Figure 1. (A,B) Chloropleth maps indicating the location of higher or lower relative abundances of emergent insects in streams (A), and lakes (B). Red indicates lower relative abundances, and blue indicates higher relative abundances in each map.
Stream Emergent Insects
Based on our multi-model inference approach (Feld et al., 2016), best-supported models indicated that the relative abundance of emergent insects from streams decreased as a function of % sand and fines (P < 0.001), ammonium concentration (NH4-N; P = 0.005), and road density (P = 0.072) (Figures 2A–C and Table 1). With a one standard deviation (SD) increase in these variables, the relative abundance of emergent insects decreased by 4.9% (road density) to 14.9% (% sand and fines), with intermediate effects of ammonium concentration (decreased 8.8%). These three variables were the most important for predicting relative abundance of emergent insects based on the sum of AICc weights (relative importance index = 1, 1, 0.80, for NH4-N,% sand and fines, and road density, respectively, Table 1). The best-supported models for the relative abundance of non-emergent individuals showed inverse responses to the same predictors of the stream emergent insect model. The relative abundance of non-emergent individuals increased in response to % sand and fines (P < 0.001), ammonium concentration (P = 0.004), and road density (P = 0.067), respectively (Table 1).
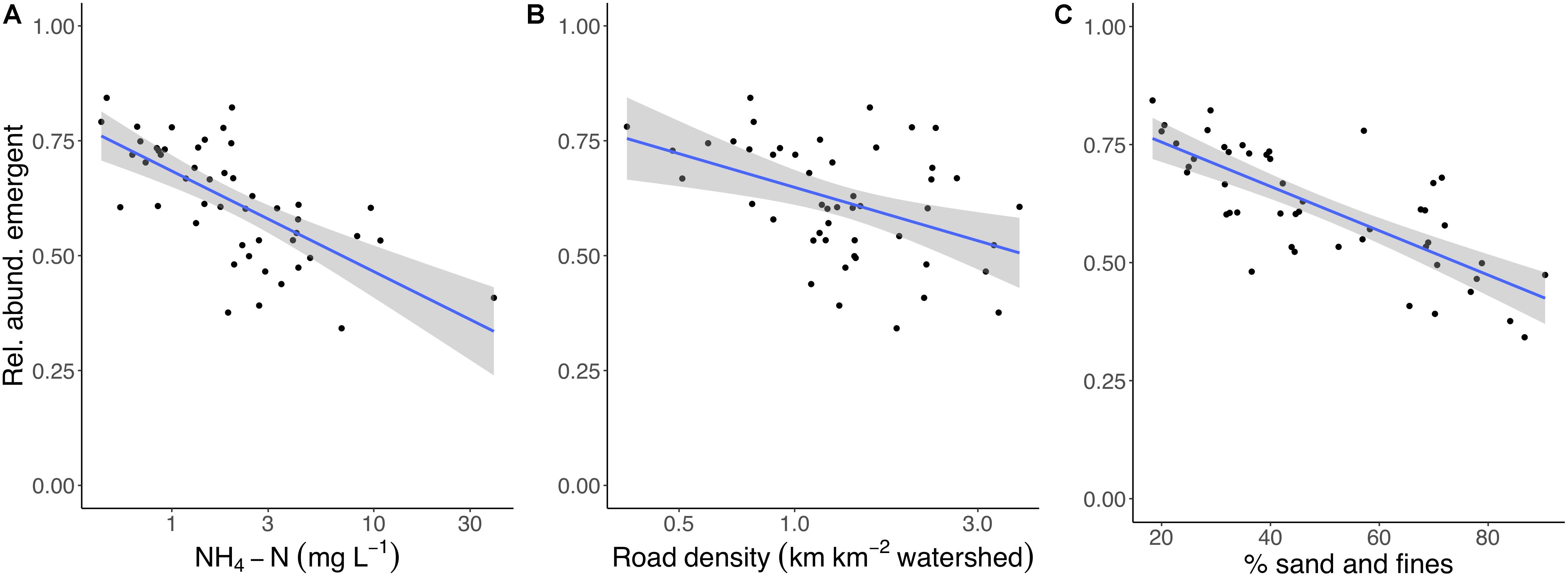
Figure 2. (A–C) Average, state-level relative abundance of emergent insects in streams as a function of ammonium (NH4-N) concentration (A), road density (km roads km–2 watershed) (B), and % sand and fines (C). These three predictors were the most important (according to AICc weights) in the subset of best-supported models (ΔAICc < 2; n = 4 models). Blue lines indicate simple linear regressions between a given predictor and emergent insect relative abundance and gray areas indicate 95% confidence bands for predictions from these models. Note: x-axis scales are logarithmic in (A,B).
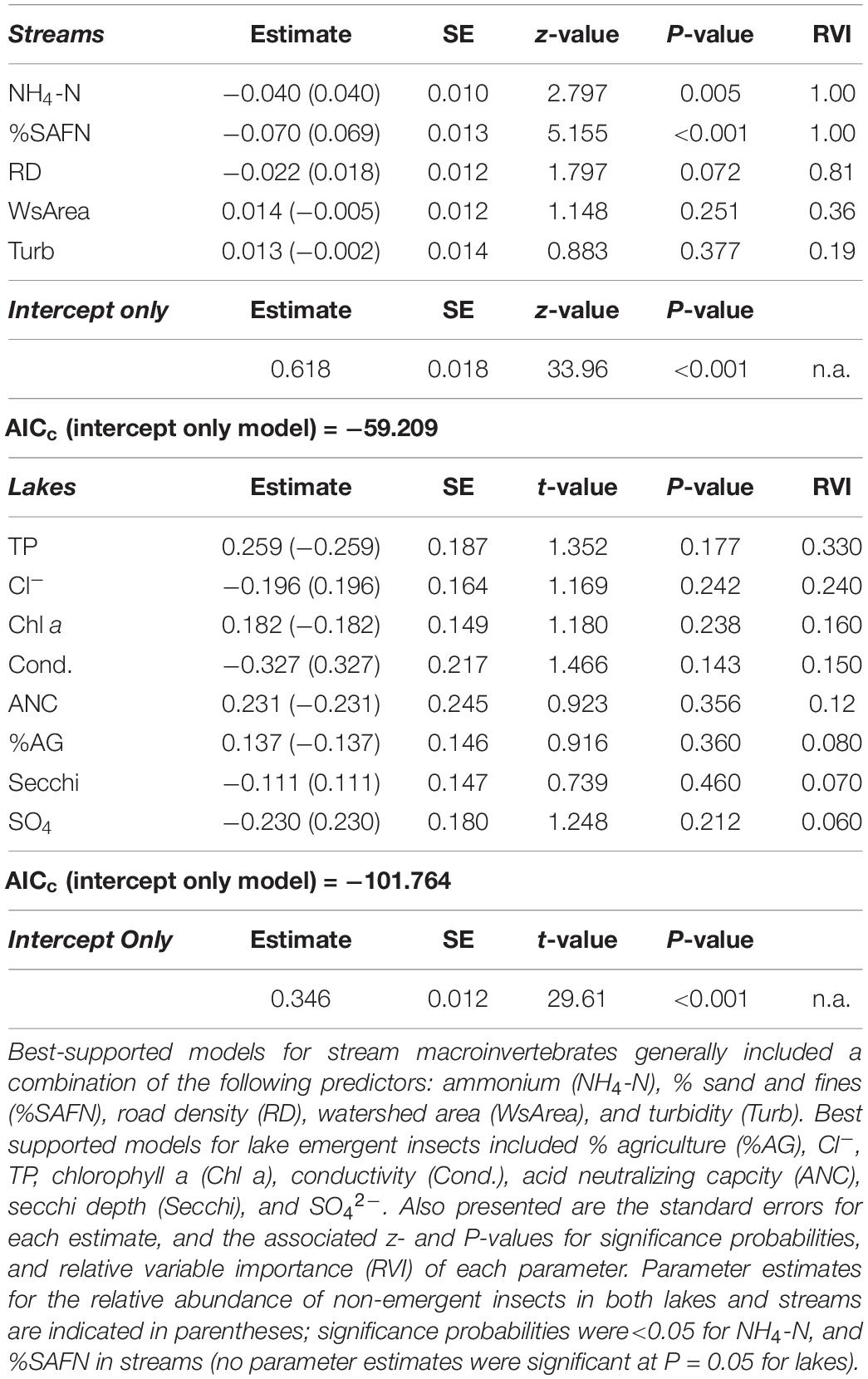
Table 1. Weighted averages of standardized parameter estimates (and SE) from the subset of best-supported models (all models with ΔAIC ≤ 2) predicting the relative abundance of emergent insects (n models = 9) in streams and lakes of the contiguous United States.
Lake Emergent Insects
Clear relationships between water quality and emergent insect relative abundance in best-supported models for lakes were scarce (Table 1). The strongest effect was conductivity (coefficient = −0.33; P = 0.143), followed by comparable negative effects of indicators of high solute concentrations such as sulfate and chloride (P = 0.212, 0.242, respectively, Table 1). Increased relative abundance of emergent insects also weakly associated with chlorophyll a (coefficient = 0.18, P = 0.238), and TP (coefficient = 0.26, P = 0.177). As with streams, non-emergent individuals showed the inverse responses to the same predictors (Table 1).
Aerial Insectivorous Bird Abundances and Stream Emergent Insects
Upland aerial insectivorous bird species responded most markedly to stream emergent insects (fixed + random effect = 1.19 90% CI = 0.108–2.23), followed by riparian obligate species [fixed + random effect = 0.42 (−0.44 to 1.36)]. We found weak (i.e., all 90% CI contained zero) but positive associations between emergent insect relative abundance and some aerial insectivorous bird species. The three strongest species-level responses were for Western Wood-Pewee (Contopus sordidulus), Olive-sided Flycatcher (Contopus cooperi), and Acadian flycatcher (Empidonax virescens), representing upland, riparian facultative, and riparian obligate species, respectively (Figure 3A). In contrast, Purple Martin (Progne subis), Barn Swallow (Hirundo rustica), and Eastern Kingbird (Tyrannus tyrannus) abundances showed the strongest negative associations with stream emergent insects (Figure 3A). However, Mourning Dove (Zenaida macroura) abundance—representing a non-aerial insectivore—also exhibited a negative association with stream emergent insects (Figure 3A).
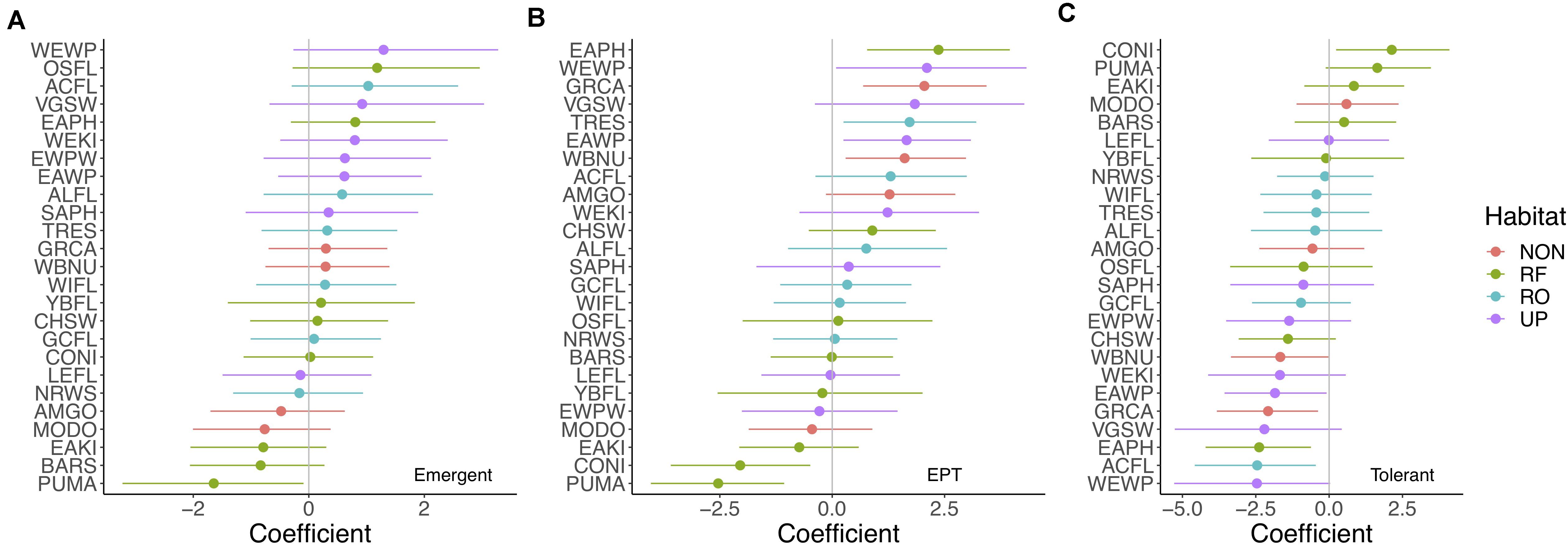
Figure 3. (A–C) Coefficient estimates (fixed effect + species-level random effects) for the effects of the relative abundance of emergent insects (A) individuals in the orders Ephemeroptera, Plecoptera, and Trichoptera (EPT) (B), and pollution tolerant individuals (C) in streams, as predictors of bird species abundances [including riparian obligate (blue circles), riparian facultative (green circles), upland (purple circles), and non-aerial insectivore species (red circles)] based on posterior probability distributions from Bayesian linear models. Ninety percent credible intervals are indicated by horizontal lines. Zero is emphasized by a vertical gray line. Bird species abbreviations can be found in Supplementary Material. Note: x-axis scales differ among (A–C).
We found stronger responses of aerial insectivores to the relative abundance of individuals in the sensitive EPT orders, with the strongest positive coefficients observed for Eastern Phoebe (Sayornis phoebe), Western Wood-Pewee, Violet-Green Swallow (Tachycineta thalassina), Tree Swallow (Tachycineta bicolor), and Eastern Wood-Pewee (Contopus virens) (Figure 3B). Some non-aerial insectivores also responded to the relative abundance of EPT, such as Gray Catbird (Dumetella carolinensis), and White-breasted Nuthatch (Sitta carolinensis). Notably, Common Nighthawk and Purple Martin abundances showed negative associations with EPT (Figure 3B).
Consistent with patterns for emergent insects and EPT, several of the same aerial insectivore species (and Gray Catbird) showed negative responses to the relative abundance of tolerant individuals, including Western Wood-Pewee, Acadian Flycatcher, Eastern Phoebe, Violet-Green Swallow, and Eastern Wood-Pewee (Figure 3C). Common Nighthawk and Purple Martin abundances tended to increase with the relative abundance of tolerant individuals.
Aerial Insectivorous Bird Abundances and Lake Emergent Insects
Non-aerial insectivores responded most strongly to the relative abundance of lake emergent insects (fixed + random effect = 0.938, 90% CI = −0.04 to 1.91), followed by riparian facultative aerial insectivorous species [fixed + random effect = 0.219 (−0.636 to 1.00)]. We found weak (i.e., all 90% CI contained zero) but positive associations between emergent insect relative abundance and some aerial insectivorous bird species (Figure 4A). These coefficients were generally weaker than for streams, and the strongest observed responses involved different species: Barn Swallow (Hirundo rustica), Chimney Swift (Chaetura pelagica), and Eastern Wood-Pewee (Figure 4A). The ground-forager, Mourning Dove (Zenaida macroura) showed the strongest response to emergent insects from lakes (Figure 4A).
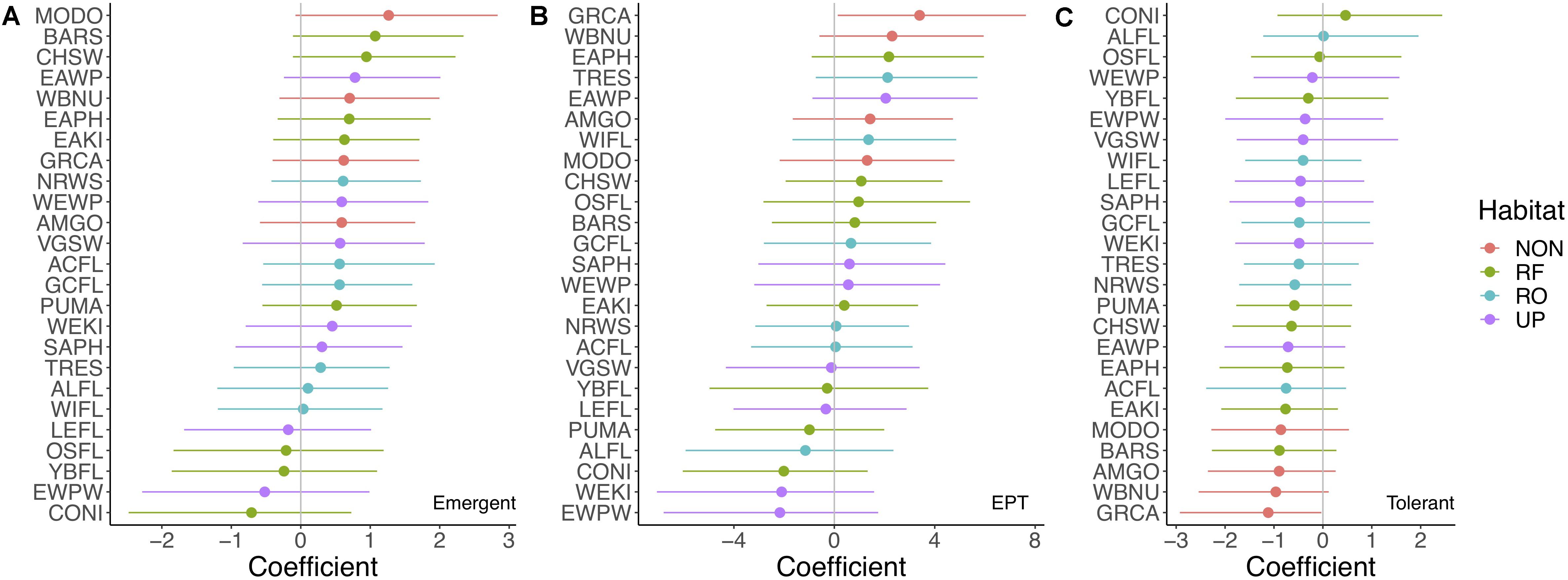
Figure 4. (A–C) Coefficient estimates (fixed effect + species-level random effect) for the effects of the relative abundance of emergent insects (A) individuals in the orders Ephemeroptera, Plecoptera, and Trichoptera (EPT) (B), and pollution tolerant individuals (C) in lakes, as predictors of bird species abundances [including riparian obligate (blue circles), riparian facultative (green circles), upland (purple circles), and non-aerial insectivore species (red circles)] based on posterior probability distributions from Bayesian linear models. Ninety percent credible intervals are indicated by horizontal lines. Zero is emphasized by a vertical gray line. Bird species abbreviations can be found in Supplementary Material. Note: x-axis scales differ among (A–C).
We found relatively stronger responses of bird abundances to the relative abundance of individuals in the EPT orders in lakes (Figure 4A). The three aerial insectivore species that responded most strongly to EPT were Eastern Phoebe, Tree Swallow, and Eastern Wood-Pewee (Figure 4B). Gray Catbird and White-breasted Nuthatch abundances increased to a greater degree than aerial insectivore species with EPT, and also showed the strongest negative responses to the proportion tolerant insects in lakes (Figures 4B,C).
Discussion
Subsidies of energy, nutrients, and organic matter are important drivers of ecological interactions across ecosystem boundaries (Polis et al., 1997; Nakano and Murakami, 2001). We show at the scale of the contiguous US, the impacts of land use and water quality were related to reduced relative abundances of emergent insects. In particular, we illustrate that insects with adult stages that disperse in flight from water to land are positively related to the relative abundance of pollution sensitive insect orders (EPT), and negatively correlated with pollution tolerance, suggesting a potential large-scale loss of this nutritional subsidy to terrestrial environments from impaired aquatic ecosystems (Wesner et al., 2020). For context, Bartrons et al. (2013) estimated that total insect emergence from lakes and streams in Wisconsin alone was ∼6,800 metric tons of carbon year–1, with 79% of the total from lentic systems. Conventional analysis of macroinverterbrate biomonitoring data based on immature insect stages has likely overlooked the implications of the functional roles that the adult life stages of aquatic insects play in conserving biotic interactions and biodiversity at watershed to continental scales.
We provide initial evidence that suggests that aerial insectivorous birds respond in complex ways to shifts in water quality and emergent insect abundance from streams and lakes. We also found evidence of habitat-specific effects, whereby stream emergent insect abundance, and especially sensitive insect orders, exhibited stronger relationships with upland aerial insectivore species compared to emergent insect abundance in lakes. Further, this analysis is an initial step that shows how the ecological impacts of water-quality variability are not necessarily constrained to providing information about the condition of aquatic habitats, but can be extended to provide useful information about terrestrial species as well, with implications for a suite of wildlife that depend on aquatic energy and nutrient subsidies including birds, spiders, bats, and reptiles (Baxter et al., 2005; Kautza and Sullivan, 2016).
Water Quality and Emergent Aquatic Insects
Our analysis underscores the threats of multiple environmental stressors that lead to changes in water quality on aquatic-insect species that provide important functions, such as aquatic-terrestrial prey subsidies (Carlson et al., 2016; Greenwood and Booker, 2016). Several lines of evidence from our analysis of large-scale surveys of benthic invertebrates suggest that a biphasic life cycle and pollution intolerance are linked traits in aquatic insect communities. For example, we observed that the relative abundance of emergent insects declined across environmental gradients related to human activities such as agriculture, urbanization, and other perturbations. These impacts increase sedimentation and impair chemical water quality, pervasively increasing nutrients and toxic pollutants (e.g., Walsh et al., 2005).
Impaired water quality, especially contamination by heavy metals, has been associated with reduced success of metamorphosis for some emergent insect taxa (Kraus et al., 2014; Wesner et al., 2014). Consistent with this, we found evidence that pollution tolerance and emergence are inversely related characteristics of aquatic invertebrates. This finding implies that across disturbed watersheds, completion of aquatic-insect life cycles could be disrupted, with disproportionate consequences for the reproductive success of emergent insects. For example, increased fine sediments and excess phosphorus have been shown to either smother or cause egg mortality of emergent insects in their larval or nymphal stages (Everall et al., 2018), and ammonium can become acutely toxic when unionized. Many emergent insects are typically univoltine or bi-voltine (i.e., with one or two reproductive cycles annually, respectively); thus, human-caused disturbances coinciding with their emergence and reproduction could be catastrophic for inter-annual population dynamics. Collectively, this large-scale analysis of emergent-insect abundance emphasizes the potential for insect emergence to be an integrative, functional metric of freshwater ecosystem health with cross-boundary implications.
Comparing Bird Species Responses to Stream vs. Lake Emergent Insects
Contrary to our hypothesis, upland aerial insectivore species responded more strongly to the relative abundance of emergent insects from streams than riparian obligate species. Similarly, non-aerial insectivores, especially Mourning Dove, Gray Catbird, and White-breasted Nuthatch abundances, showed surprisingly large responses to the relative abundances of emergent insects and EPT taxa from lakes, respectively. Further, relationships between bird abundance and lake emergent insects were generally less pronounced than for streams, also contrary to our hypothesis. However, the relative abundance of lake emergent insects was generally unrelated to lake water-quality predictors, whereas stream emergent insects decreased significantly with streamwater ammonium concentrations, and sedimentation of the stream bottom. This higher sensitivity of stream emergent insects to watershed stressors, along with the stronger response of upland and non-aerial insectivores like Gray Catbird and White-breasted Nuthatch, may suggest that the positive relationship observed for these bird species could simply signal that there are shared drivers of both declining water quality and the prevalence of upland aerial-insectivore and non-aerial insectivore species (e.g., deforestation, urbanization, agriculture). Nonetheless, we observed a clear response of several aerial insectivore species, including Tree Swallows, to the abundance of pollution sensitive, fatty acid-rich EPT taxa from streams, suggesting that there may be a dietary signal within these patterns as well. Importantly, the data sets we used necessitated a focus on insect abundance, rather than biomass; we expect that insect biomass may be a more appropriate metric to model and explore in future continental-scale analyses, especially because there are generally greater masses of insects deposited on land from lakes as compared to streams (e.g., Bartrons et al., 2013).
Detecting Cross-Boundary Effects of Poor Water Quality Remains a Challenge
While our analysis of emergent aquatic insects indicated strong influences of land use and water quality in streams, the relationships between bird abundances and emergent insects were more nuanced and species-specific, especially with respect to the emergent insect metric we generated in this analysis and the majority of bird species (Figures 3A, 4A). This finding is in contrast to evidence that indicates many aerial insectivorous birds, and to some degree, other insectivorous birds, are highly reliant on aquatic-to-terrestrial subsidies (e.g., Kautza and Sullivan, 2016; Schilke et al., 2020; Sullivan et al., 2021). Our ability to detect this reliance with publicly available biomonitoring data was potentially hampered in several ways. For example, the relationships between emergent insects and aerial insectivorous birds are diffuse in both time and space, such that the timing of the Breeding Bird Survey (early summer) and the precise locations of monitoring routes may have occurred outside the direct influence of emerging insects from nearby streams or lakes, i.e., the majority (>70%) of emergent insect deposition from streams and lakes generally falls within 100 m of the waterbody (Gratton and Vander Zanden, 2009; Muehlbauer et al., 2014; Schilke et al., 2020). Beyond these mismatches inherent to the monitoring data sets, differential access to water and habitat use among riparian-to-upland obligate species, detrimental effects carried over from non-breeding habitats, or landscape topography (e.g., ravines vs. low-elevation streams) are all expected to affect the foraging ecology of aerial insectivores.
The complexity of local food webs could also mediate aerial insectivore-emergent insectivore relationships. Sullivan et al. (2021) found that adult Tree Swallows at urban sites fed at significantly higher trophic positions than those at protected sites, implicating a suite of complex factors including local climate, water quality, and insect body-size preferences. Similarly, many riparian swallow species tend to forage over open water vs. heavily canopied areas (Alberts et al., 2013). Consistent with this, we found some bird species responded positively to the relative abundance of lake emergent insects. The greater surface area in lakes compared to streams leads to lentic systems contributing more insect production to terrestrial habitats (Bartrons et al., 2013).
There were some aerial insectivorous species that showed clearer relationships with emergent insect abundance, and stronger positive responses to the relative abundance of sensitive EPT taxa, consistent with our hypotheses. However, we also observed unexpected negative relationships between emergent insect abundance (and/or EPT) from streams and some aerial insectivores such as Purple Martin and Common Nighthawk. We cannot rule out inherent biases of the BBS sampling protocols (e.g., sampling during the day, survey routes occur along roads) that may have influenced these patterns, especially for Common Nighthawk. Variability in aerial insectivorous bird foraging strategies and diet also may explain some of these relationships. For example, Purple Martins, North America’s largest swallow, forage at high altitudes relative to many aerial insectivores and have been documented to predominantly feed on terrestrial flying social insects such as ants, honey bees, and termites (Helms et al., 2016).
Plasticity in aerial insectivore diet both in terms of prey type and habitat may also aid in the interpretation of contrasting results. For instance, Tree Swallow-emergent insect relationships were generally positive for both streams and lakes (Figures 3A, 4A), thus generally following our expectations given the riparian-obligate nature of Tree Swallows and their high dietary reliance on aquatic emergent insects (Beck et al., 2013). McCarty and Winkler (1999) observed that adult Tree Swallows actively selected for larger-bodied prey (e.g., Odonata) vs. smaller-bodied insects (0–3 mm in length), supporting the positive associations we observed between Tree Swallows and typically larger-bodied EPT taxa (Figures 3B, 4B). However, Barn Swallow responses were more variable, exhibiting a negative relationship with emergent insect relative abundance for streams (Figure 3A) but a positive association with emergent insects for lakes (Figure 4A). Barn Swallows are a non-riparian obligate species most commonly feeding on terrestrial flying insects such as flies, beetles, bees, wasps, flying ants, butterflies, and moths (Brown and Brown, 1999; Law et al., 2017). However, Barn Swallows are adaptable and will also forage over open water and feed on available aquatic insects. Thus, the positive relationship between emergent insects and Barn Swallows in open lake environments vs. the negative relationship observed for canopied stream environments is not surprising. Further, open, non-forested streams are commonly agricultural streams that exhibit poor water quality and support aquatic insect assemblages dominated by smaller-bodied, tolerant taxa such as midge flies in the family Chironomidae with limited landward dispersal, making aerial insectivorous bird foraging responses in these altered ecosystems difficult to predict (Carlson et al., 2016; McKie et al., 2018). Therefore, the negative relationships between some aerial insectivores and the relative abundance of stream emergent insects might also reflect changes in the availability or accessibility of preferred prey (potentially in favor of terrestrial insects vs. aquatic insects) in agricultural and other modified landscapes. Plasticity in diet and foraging strategy may also contribute to explaining patterns between non-aerial insectivores and emergent insects, such as Gray Catbird, that has a broad diet and is common in riparian areas (e.g., Rodewald and Kearns, 2011).
Consequences of Losing Aquatic-Terrestrial Connections Via Insect Emergence and the Need for Conservation Across Ecosystem Boundaries
Traditional biomonitoring efforts, including those focused on aquatic insects, continue to be essential tools for establishing baseline conditions and detecting aquatic ecosystem degradation (Hill et al., 2017). Our analysis emphasizes that their utility can extend beyond within-stream or within-lake measures of ecosystem condition to include crucial ecosystem functions that have implications for terrestrial ecosystems and linked aquatic-terrestrial food-web interactions (e.g., via potential adult insect production). Novel benthic invertebrate monitoring metrics could build on these ideas to emphasize the proportion or abundance of individuals with flying adult stages in future monitoring efforts. Measures that directly quantify the timing and amount of insect-mediated energetic subsidies from streams and lakes across a spectrum of watershed-scale stressors—while giving appropriate weight to the importance of insects that provision higher levels of nutritional macromolecules (such as fatty acids; Whorley et al., 2019) to riparian consumers—could also be useful in predicting potential consequences of poor water quality for terrestrial insectivores.
A recent analysis found a global increase of freshwater insect abundance by ∼11% per decade (van Klink et al., 2020), largely driven by North American patterns (although, consistent with our analysis, this meta-analysis also suggests that freshwater insects have declined ∼2.3% per year in the Midwest US). However, the studies included in this analysis were skewed toward larval vs. adult aquatic insects, potentially overlooked increases driven by pollution-tolerant aquatic insect taxa, and did not fully account for the fact that the scope and magnitude of these changes is spatially heterogeneous, illustrating the inherent challenges in comparing population trends across aquatic-terrestrial boundaries; all of these factors are potentially relevant to aerial insectivorous bird populations. Interannual variability in insect abundances can also be significant, with multiple implications for aerial insectivorous birds including breeding success and post-fledgling survival (Paquette et al., 2013; Twining et al., 2018). Available open-access macroinvertebrate data with broad spatial coverage are limited in temporal scope; additional years of emergent insect data could improve future analyses. Additionally, future work that makes concerted efforts to achieve tighter spatial and temporal synchronization between aerial insectivorous birds and their insect prey at watershed (and larger) scales (e.g., Schilke et al., 2020) will be an important step in further exploring the links between broad-scale water quality, emergent insects, and bird diets, fitness, and reproductive success (e.g., Twining et al., 2018).
Although aquatic-terrestrial ecological linkages are increasingly recognized, conservation that explicitly considers water-land boundaries is still uncommon. Here, we highlight the potential consequences of water quality on both emergent aquatic insects and species of aerial insectivorous birds—a guild that has experienced alarming population trends in North America, contributing to a massive decline in North American avifauna (Rosenberg et al., 2019). Although larval life stages are the target of biomonitoring programs (US EPA, 2015), explicitly monitoring the adult life stage of aquatic insects may be instructive in terms of further understanding the impacts of land-use change on aquatic systems. Furthermore, the abundance and distribution of aquatic insects that emerge from aquatic habitats as flying adults could be an important predictor of the survival and population dynamics of many species of aerial insectivorous birds, including both upland and riparian obligates that rely on energetic pulses of emerging aquatic insects for multiple aspects of their life cycles, i.e., during reproduction and migration (McCarty, 1997; MacDade et al., 2011). Although our results highlight that predicting the effects of broad-scale effects of variability in emergent insects on aerial insectivores can be challenging, they have intriguing implications for the future conservation of aerial insectivorous birds, as well as other aerial insectivorous wildlife (e.g., bats), which provide a suite of ecosystem services (e.g., Kelly et al., 2013). Accounting for the reciprocal, water-to-land consequences of impaired water quality could represent an important conservation paradigm, both in North America and globally, where water quality concerns persist (Vörösmarty et al., 2010; Brown and Froemke, 2012).
Data Availability Statement
The datasets presented in this study can be found in online repositories. The names of the repositories can be found below: https://www.epa.gov/national-aquatic-resource-surveys/data-national-aquatic-resource-surveys, https://www.pwrc.usgs.gov/bbs/rawdata/. Data files and R code used for this analysis can be found here: https://github.com/dmanningUNO/emergent_insects-aerial_insectivores.
Ethics Statement
Although data from vertebrate animals was analyzed as part of this study, ethical review and approval was not required because we used publicly available bird population data sets generated by a government agency (USGS Breeding Bird Survey).
Author Contributions
DWPM conceived of the analysis, collated and analyzed the data, and wrote the manuscript. SMPS conceived of the analysis, secured funding to support the analysis, contributed to analyzing the data sets, and wrote the manuscript. Both authors contributed to the article and approved the submitted version.
Funding
SMPS wishes to acknowledge support from NSF DEB-1341215, and the Ohio Department of Natural Resources, Division of Wildlife through the State Wildlife Grants Program and the Ohio Biodiversity Conservation Partnership.
Conflict of Interest
The authors declare that the research was conducted in the absence of any commercial or financial relationships that could be construed as a potential conflict of interest.
Acknowledgments
We thank Drs. Daniel Cristol and Kerri Vierling for constructive feedback on early versions of the manuscript, Dr. Ryan Hill for advice about the StreamCat dataset, and Dr. Adam Smith for guidance with modeling annual indices of bird abundances. This analysis was made possible by the availability of the USGS Breeding Bird and National Aquatic Resource Surveys data sets, which were a result of the collective efforts of dedicated citizen scientists, field crews, laboratory staff, data management and quality-control staff, analysts, and many others from USGS, EPA, states, tribes, federal agencies, universities, and other organizations.
Supplementary Material
The Supplementary Material for this article can be found online at: https://www.frontiersin.org/articles/10.3389/fevo.2021.633160/full#supplementary-material
List of benthic macroinvertebrate families (listed alphabetically by family) observed in the EPA wadeable streams assessment and their designation as emergent/non-emergent (Supplementary Table 1). Common and scientific names and conventional 4-letter abbreviations for the 25 bird species considered in this analysis and the number of states where annual indices of abundance were summarized for each species (Supplementary Table 2). Full list of predictors, and watershed/basin and site-level stressors that could affect water quality and benthic macroinvertebrates, their associated units/descriptions, and how these data were transformed to meet assumptions of normality (Supplementary Table 3). Additional results: normal quantile-quantile plots for lake water quality metrics (Supplementary Figure 1), stream water quality metrics (Supplementary Figure 2), and annual indices of bird abundance in 2004 and 2007 (Supplementary Figures 3, 4).
Footnotes
References
Alberts, J. M., Sullivan, S. M. P., and Kautza, A. (2013). Riparian swallows as integrators of landscape change in a multiuse river system: Implications for aquatic-to-terrestrial transfers of contaminants. Sci. Total Environ. 463–464, 42–50. doi: 10.1016/j.scitotenv.2013.05.065
Allan, J. D. (2004). Landscapes and riverscapes: the influence of land use on stream ecosystems. Ann. Rev. Ecol. Evol. Syst. 35, 257–284. doi: 10.1146/annurev.ecolsys.35.120202.110122
Bartoń, K. (2020). MuMIn: Multi-Model Inference. R package version 1.43.17. Available online at: https://CRAN.R-project.org/package=MuMIn
Bartrons, M., Papeş, M., Diebel, M. W., Gratton, C., and Vander Zanden, M. J. (2013). Regional-level inputs of emergent aquatic insects from water to land. Ecosystems 16, 1353–1363. doi: 10.1007/s10021-013-9688-6
Baxter, C. V., Fausch, K. D., and Saunders, W. C. (2005). Tangled webs: reciprocal flows of invertebrate prey link streams and riparian zones. Freshw. Biol. 50, 201–220. doi: 10.1111/j.1365-2427.2004.01328.x
Beck, M. L., Hopkins, W. A., and Jackson, B. P. (2013). Spatial and temporal variation in the diet of tree swallows: implications for trace-element exposure after habitat remediation. Arch. Environ. Contam. Toxicol. 65, 575–587. doi: 10.1007/s00244-013-9913-5
Brown, C. R., and Brown, M. B. (1999). “Barn Swallow (Hirundo rustica), version 2.0,” in The Birds of North America, ed. P. G. Rodewald, (Ithaca, New York, USA: Cornell Lab of Ornithology).
Brown, T. C., and Froemke, P. (2012). Nationwide assessment of non-point source threats to water quality. BioScience 62, 136–146. doi: 10.1525/bio.2012.62.2.7
Bürkner, P.-C. (2017). brms: an R package for bayesian multilevel models using stan. J. Stat. Softw. 80, 1–28. doi: 10.18637/jss.v080.i01
Burnham, K. P., and Anderson, D. R. (2002). Model selection and multi-model inference: A practical information-theoretic approach. New York, NY: Springer-Verlag.
Carlson, P. E., McKie, B. G., Sandin, L., and Johnson, R. K. (2016). Strong land-use effects on the dispersal patterns of adult stream insects: implications for transfers of aquatic subsidies to terrestrial consumers. Freshwater Biol. 61, 848–861. doi: 10.1111/fwb.12745
Dreelin, R. A., Shipley, J. R., and Winkler, D. W. (2018). Flight behavior of individual aerial insectivores revealed by novel altitudinal dataloggers. Front. Ecol. Evol. 6:182. doi: 10.3389/fevo.2018.00182
Dubrovsky, N. M., Burow, K. R., Clark, G. M., Gronberg, J. M., Hamilton, P. A., Hitt, K. J., et al. (2010). The Quality of Our Nation’s Waters–Nutrients in the Nation’s Streams and Groundwater, 1992–2004. Reston, VA: U.S. Geological Survey Circular 1350, 174.
Everall, N. C., Johnson, M. F., Wood, P., and Mattingley, L. (2018). Sensitivity of the early life stages of a mayfly to fine sediment and orthophosphate levels. Environ. Pollu. 237, 792–802. doi: 10.1016/j.envpol.2017.10.131
Feld, C. K., Segurado, P., and Gutiérrez-Cánovas, C. (2016). Analysing the impact of multiple stressors in aquatic biomonitoring data: A ‘cookbook’ with applications in R. Sci. Total Environ. 573, 1320–1339. doi: 10.1016/j.scitotenv.2016.06.243
Gratton, C., Donaldson, J., and Zanden, M. J. V. (2008). Ecosystem linkages between lakes and the surrounding terrestrial landscape in northeast Iceland. Ecosystems 11, 764–774. doi: 10.1007/s10021-008-9158-8
Gratton, C., and Vander Zanden, M. J. (2009). Flux of aquatic insect productivity to land: comparison of lentic and lotic ecosystems. Ecology 90, 2689–2699. doi: 10.1890/08-1546.1
Gray, L. J. (1993). Response of insectivorous birds to emerging aquatic insects in riparian habitats of a tallgrass prairie stream. Am. Midland Nat. 129, 288–300. doi: 10.2307/2426510
Greenwood, M. J., and Booker, D. J. (2016). Influence of hydrological regime and land cover on traits and potential export capacity of adult aquatic insects from river channels. Oecologia 180, 551–566. doi: 10.1007/s00442-015-3462-8
Greig, H. S., Kratina, P., Thompson, P. L., Palen, W. J., Richardson, J. S., Shurin, J. B., et al. (2012). Warming, eutrophication, and predator loss amplify subsidies between aquatic and terrestrial ecosystems. Global Change Biol. 18, 504–514. doi: 10.1111/j.1365-2486.2011.02540.x
Hallmann, C. A., Foppen, R. P. B., van Turnhout, C. A. M., de Kroon, H., and Jongejans, E. (2014). Declines in insectivorous birds are associated with high neonicotinoid concentrations. Nature 511, 341–343. doi: 10.1038/nature13531
Hallmann, C. A., Sorg, M., Jongejans, E., Siepel, H., Hofland, N., Schwan, H., et al. (2017). More than 75 percent decline over 27 years in total flying insect biomass in protected areas. PLoS One 12:e0185809. doi: 10.1371/journal.pone.0185809
Helms, J. A., Godfrey, A. P., Ames, T., and Bridge, E. S. (2016). Predator foraging altitudes reveal the structure of aerial insect communities. Sci. Rep. 6:10.
Hill, R. A., Fox, E. W., Leibowitz, S. G., Olsen, A. R., Thornbrugh, D. J., Weber, M. H., et al. (2017). Predictive mapping of the biotic condition of conterminous U.S. rivers and streams. Ecol. Appl. 27, 2397–2415. doi: 10.1002/eap.1617
Hill, R. A., Weber, M. H., Leibowitz, S. G., Olsen, A. R., and Thornbrugh, D. J. (2016). The stream-catchment (StreamCat) dataset: a database of watershed metrics for the conterminous united states. JAWRA J. Am. Water Res. Assoc. 52, 120–128. doi: 10.1111/1752-1688.12372
Hynes, H. B. N. (1975). The stream and its valley. SIL Proceedings, 1922-2010 19:1-15. Florida, NY: Taylor & Francis.
Imlay, T. L., Mann, H. A. R., and Leonard, M. L. (2017). No effect of insect abundance on nestling survival or mass for three aerial insectivores. Avian Conser. Ecol. 12:19.
Iwata, T., Nakano, S., and Murakami, M. (2003). Stream meanders increase insectivorous bird abundance in riparian deciduous forests. Ecography 26, 325–337. doi: 10.1034/j.1600-0587.2003.03355.x
Kautza, A., and Sullivan, S. M. P. (2015). Shifts in reciprocal river-riparian arthropod fluxes along an urban-rural landscape gradient. Freshwater Biol. 60, 2156–2168. doi: 10.1111/fwb.12642
Kautza, A., and Sullivan, S. M. P. (2016). The energetic contributions of aquatic primary producers to terrestrial food webs in a mid-size river system. Ecology 97, 694–705. doi: 10.1890/15-1095.1
Kelly, J. F., Bridge, E. S., Frick, W. F., and Chilson, P. B. (2013). Ecological energetics of an abundant aerial insectivore, the purple martin. PLoS One 8:e76616. doi: 10.1371/journal.pone.0076616
King, R. S., Baker, M. E., Whigham, D. F., Weller, D. E., Jordan, T. E., Kazyak, P. F., et al. (2005). Spatial considerations for linking watershed land cover to ecological indicators in streams. Ecol. Appl. 15, 137–153. doi: 10.1890/04-0481
Kraus, J. M., Schmidt, T. S., Walters, D. M., Wanty, R. B., Zuellig, R. E., Wolf, R. E., et al. (2014). Cross-ecosystem impacts of stream pollution reduce resource and contaminant flux to riparian food webs. Ecol. Appl. 24, 235–243. doi: 10.1890/13-0252.1
Law, A. A., Threlfall, M. E., Tijman, B. A., Anderson, E. M., McCann, S., Searing, G., et al. (2017). Diet and prey selection of barn swallows (Hirundo rustica) at Vancouver international airport. Can. Field Nat. 131:26. doi: 10.22621/cfn.v131i1.1777
MacDade, L. S., Rodewald, P. G., and Hatch, K. A. (2011). Contribution of emergent aquatic insects to refueling in spring migrant songbirds. The Auk 128, 127–137. doi: 10.1525/auk.2011.10186
McCarty, J. P. (1997). Aquatic community characteristics influence the foraging patterns of tree swallows. The Condor 99, 210–213. doi: 10.2307/1370241
McCarty, J. P., and Winkler, D. W. (1999). Foraging ecology and diet selectivity of tree swallows feeding nestlings. The Condor 101, 246–254. doi: 10.2307/1369987
McKie, B. G., Sandin, L., Carlson, P. E., and Johnson, R. K. (2018). Species traits reveal effects of land use, season and habitat on the potential subsidy of stream invertebrates to terrestrial food webs. Aquatic Sci. 80:15.
Merritt, R. W., Cummins, K. W., and Berg, M. B. (eds). (2008). An Introduction to the Aquatic Insects of North America, 4th edn. Kendall Hunt Publishing.
Muehlbauer, J. D., Collins, S. F., Doyle, M. W., and Tockner, K. (2014). How wide is a stream? spatial extent of the potential “stream signature” in terrestrial food webs using meta-analysis. Ecology 95, 44–55. doi: 10.1890/12-1628.1
Nakano, S., and Murakami, M. (2001). Reciprocal subsidies: dynamic interdependence between terrestrial and aquatic food webs. Proc. Natl. Acad. Sci. 98, 166–170. doi: 10.1073/pnas.98.1.166
Nebel, S., Mills, A., McCracken, J., and Taylor, P. (2010). Declines of aerial insectivores in north america follow a geographic gradient. Avian Conser. Ecol. 5:1.
Paquette, S. R., Garant, D., Pelletier, F., and Bélisle, M. (2013). Seasonal patterns in tree swallow prey (Diptera) abundance are affected by agricultural intensification. Ecol. Appl. 23, 122–133. doi: 10.1890/12-0068.1
Poff, N. L., Olden, J. D., Vieira, N. K. M., Finn, D. S., Simmons, M. P., Kondratieff, B. C., et al. (2006). Functional trait niches of north american lotic insects: traits-based ecological applications in light of phylogenetic relationships. J. N. Am. Benthol. Society 25, 730–755. doi: 10.1899/0887-3593(2006)025[0730:ftnona]2.0.co;2
Polis, G. A., Anderson, W. B., and Holt, R. D. (1997). Toward an integration of landscape and food web ecology: the dynamics of spatially subsidized food webs. Ann. Rev. Ecol. Syst. 28, 289–316. doi: 10.1146/annurev.ecolsys.28.1.289
Qian, S. S., Cuffney, T. F., and McMahon, G. (2012). Multinomial regression for analyzing macroinvertebrate assemblage composition data. Freshwater Sci. 31, 681–694. doi: 10.1899/11-026.1
Rodewald, A. D., and Kearns, L. J. (2011). Shifts in dominant nest predators along a rural-to-urban landscape gradient. The Condor 113, 899–906. doi: 10.1525/cond.2011.100132
Rosenberg, K. V., Dokter, A. M., Blancher, P. J., Sauer, J. R., Smith, A. C., Smith, P. A., et al. (2019). Decline of the north american avifauna. Science 366, 120–124. doi: 10.1126/science.aaw1313
Sauer, J. R., and Link, W. A. (2011). Analysis of the north american breeding bird survey using hierarchical models. The Auk 128, 87–98. doi: 10.1525/auk.2010.09220
Sauer, J. R., Pardieck, K. L., Ziolkowski, D. J., Smith, A. C., Hudson, M.-A. R., Rodriguez, V., et al. (2017a). The first 50 years of the north american breeding bird survey. The Condor 119, 576–593. doi: 10.1650/condor-17-83.1
Sauer, J. R., Niven, D. K., Hines, J. E., Ziolkowski, D. J. Jr., Pardieck, K. L., Fallon, J. E., et al. (2017b). The North American breeding bird survey, results and analysis 1966-2015. Laurel, MD, U.S.A: USGS Patuxent Wildlife Research Center.
Schilke, P. R., Bartrons, M., Gorzo, J. M., Vander Zanden, M. J., Gratton, C., Howe, R. W., et al. (2020). Modeling a cross-ecosystem subsidy: forest songbird resposne to emergent aquatic insects. Landscape Ecol. 35, 1587–1604. doi: 10.1007/s10980-020-01038-0
Schröder, M., Sondermann, M., Sures, B., and Hering, D. (2015). Effects of salinity gradients on benthic invertebrate and diatom communities in a german lowland river. Ecol. Indic. 57, 236–248. doi: 10.1016/j.ecolind.2015.04.038
Smith, A. C., Hudson, M.-A. R., Downes, C. M., and Francis, C. M. (2015). Change points in the population trends of aerial-insectivorous birds in north america: synchronized in time across species and regions. PLoS One 10:e0130768. doi: 10.1371/journal.pone.0130768
Spiller, K. J., and Dettmers, R. (2019). Evidence for multiple drivers of aerial insectivore declines in north america. The Condor 121:duz100.
Stan Development Team (2020). RStan: The R Interface to Stan. R Package Version 2.19.3. Available online at: http://mc-stan.org/
Sterling, J. L., Rosemond, A. D., and Wenger, S. J. (2016). Watershed urbanization affects macroinvertebrate community structure and reduces biomass through similar pathways in Piedmont streams, Georgia, USA. Freshwater Sci. 35, 676–688. doi: 10.1086/686614
Sullivan, S. M. P., Corra, J. W., and Hayes, J. T. (2021). Urbanization mediates the effects of water quality and climate on a model aerial insectivorous bird. Ecol. Monog. doi: 10.1002/ecm.1442
Sullivan, S. M. P., and Manning, D. W. P. (2019). Aquatic-terrestrial linkages as complex systems: insights and advances from network models. Freshw. Sci. 38, 936–945. doi: 10.1086/706071
Sullivan, S. M. P., Rains, M. C., and Rodewald, A. D. (2019). Opinion: the proposed change to the definition of “waters of the united states” flouts sound science. Proc. Natl. Acad. Sci. 116, 11558–11561. doi: 10.1073/pnas.1907489116
Twining, C. W., Brenna, J. T., Lawrence, P., Winkler, D. W., Flecker, A. S., and Hairston, N. G. (2019). Aquatic and terrestrial resources are not nutritionally reciprocal for consumers. Func. Ecol. 33, 2042–2052. doi: 10.1111/1365-2435.13401
Twining, C. W., Shipley, J. R., and Winkler, D. W. (2018). Aquatic insects rich in omega-3 fatty acids drive breeding success in a widespread bird. Ecol. Lett. 21, 1812–1820. doi: 10.1111/ele.13156
US EPA (2006). National Aquatic Resource Surveys. Wadeable Streams Assessment 2004-2005 (data and metadata files). Washington, DC: US Environmental Protection Agency.
US EPA (2007). Survey of the Nation’s Lakes. Field Operations Manual. EPA 841-B-07-004. U.S. Environmental Protection Agency. Washington, DC: US Environmental Protection Agency.
US EPA (2010). National Aquatic Resource Surveys. National Lakes Assessment 2007 (data and metadata files). Washington, DC: US Environmental Protection Agency.
US EPA (2015). Rapid bioassessment protocols for use in streams and wadeable rivers: periphyton, benthic macroinvertebrates, and fish, Second Edn. Washington, DC: US Environmental Protection Agency.
US EPA (2016). Office of Water and Office of Research and Development. National Rivers and Streams Assessment 2008-2009 Technical Report (EPA/841/R-16/008). Washington, DC: US Environmental Protection Agency.
US EPA (2017). National Lakes Assessment 2012: Technical Report. Washington, DC: US Environmental Protection Agency.
van Klink, R., Bowler, D. E., Gongalsky, K. B., Swengel, A. B., Gentile, A., and Chase, J. M. (2020). Meta-analysis reveals declines in terrestrial but increases in freshwater insect abundances. Science 368, 417–420. doi: 10.1126/science.aax9931
Vörösmarty, C. J., McIntyre, P. B., Gessner, M. O., Dudgeon, D., Prusevich, A., Green, P., et al. (2010). Global threats to human water security and river biodiversity. Nature 467, 555–561. doi: 10.1038/nature09440
Walsh, C. J., Roy, A. H., Feminella, J. W., Cottingham, P. D., Groffman, P. M., Morgan, R. P., et al. (2005). The urban stream syndrome: current knowledge and the search for a cure. J.N. Am. Benthol. Society 24, 706–723. doi: 10.1899/04-028.1
Walters, D. M., Fritz, K. M., and Otter, R. R. (2008). The dark side of subsidies: adult stream insects export organic contaminants to riparian predators. Ecol. Appl. 18, 1835–1841. doi: 10.1890/08-0354.1
Wenger, S. J., Roy, A. H., Jackson, C. R., Bernhardt, E. S., Carter, T. L., Filoso, S., et al. (2009). Twenty-six key research questions in urban stream ecology: an assessment of the state of the science. J. N. Am. Benthol. Soc. 28, 1080–1098. doi: 10.1899/08-186.1
Wesner, J. S., Kraus, J. M., Schmidt, T. S., Walters, D. M., and Clements, W. H. (2014). Metamorphosis enhances the effects of metal exposure on the mayfly, Centroptilum triangulifer. Environ. Sci. Technol. 48, 10415–10422. doi: 10.1021/es501914y
Wesner, J. S., Swanson, D. L., Dixon, M. D., Soluk, D. A., Quist, D. J., Yager, L. A., et al. (2020). Loss of potential aquatic-terrestrial subsidies along the Missouri River floodplain. Ecosystems 23, 111–123. doi: 10.1007/s10021-019-00391-9
Whorley, S. B., Smucker, N. J., Kuhn, A., and Wehr, J. D. (2019). Urbanisation alters fatty acids in stream food webs. Freshwater Biol. 64, 984–996. doi: 10.1111/fwb.13279
Wohl, E. (2017). The significance of small streams. Front. Earth Sci. 11:447–456. doi: 10.1007/s11707-017-0647-y
Keywords: aquatic ecosystems, aquatic-terrestrial linkages, bioassessment, land use, multiple stressors
Citation: Manning DWP and Sullivan SMP (2021) Conservation Across Aquatic-Terrestrial Boundaries: Linking Continental-Scale Water Quality to Emergent Aquatic Insects and Declining Aerial Insectivorous Birds. Front. Ecol. Evol. 9:633160. doi: 10.3389/fevo.2021.633160
Received: 24 November 2020; Accepted: 27 January 2021;
Published: 26 February 2021.
Edited by:
Natalia Ocampo-Peñuela, ETH Zürich, SwitzerlandReviewed by:
Christy Morrissey, University of Saskatchewan, CanadaJoseph Nocera, University of New Brunswick Fredericton, Canada
Copyright © 2021 Manning and Sullivan. This is an open-access article distributed under the terms of the Creative Commons Attribution License (CC BY). The use, distribution or reproduction in other forums is permitted, provided the original author(s) and the copyright owner(s) are credited and that the original publication in this journal is cited, in accordance with accepted academic practice. No use, distribution or reproduction is permitted which does not comply with these terms.
*Correspondence: David W. P. Manning, ZGF2aWRtYW5uaW5nQHVub21haGEuZWR1