- 1Faculty of Sciences and Technology, University of the Azores, Ponta Delgada, Portugal
- 2CIBIO – Research Centre in Biodiversity and Genetic Resources, InBio Associate Laboratory, University of the Azores, Ponta Delgada, Portugal
Freshwater systems on remote oceanic islands are particularly vulnerable to biological invasions. The case of freshwater ecosystems in the Azores Archipelago is especially relevant considering the islands’ youth and remoteness, and low natural connectivity. This study presents a review of the introduction and presence of non-indigenous freshwater species in the Azores, retrieved from various historical records, paleoenvironmental reconstructions, published records, and field data from two decades of the Water Framework Directive (WFD) monitoring programs. At least 132 non-indigenous freshwater species have successfully established in the Azores, belonging to several taxonomic groups: cyanobacteria (10), synurophytes (1), desmids (1), diatoms (20), plants (41), invertebrates (45), amphibia (2), and fishes (12). Intentional and accidental introductions have been occurring since the establishment of the first human settlers on the archipelago, impacting freshwater ecosystems. The first reported introductions in the Azores were intentional fish stocking in some lakes. Non-deliberate introductions have recently increased through transport-contaminants (51%) associated with the aquarium trade or agricultural products. In the Azores, the highest number of non-indigenous species occur on the largest and most populated island, São Miguel Island (116), followed by Flores (68). Plants constitute the most representative group of introduced species on all islands, but invertebrates, diatoms, and fishes are also well established on most islands. Among invertebrates, non-indigenous arthropods are the most well-established group on all islands except on the smallest Corvo Island. Many non-indigenous species will likely benefit from climate change and magnified by globalization that increases the probability of the movement of tropical and subtropical species to the Azores. Present trends in international trade, importations, and enhanced connectivity of the archipelago by increasing flights and shipping will probably promote the arrival of new species. Augmented connectivity among islands is likely to improve non-indigenous species dispersal within the archipelago as accidental transportation seems to be an essential pathway for non-indigenous freshwater species already present in the Azores.
Introduction
Human activities have for centuries promoted the transport of multiple species across huge biogeographical barriers (Gippoliti and Amori, 2006; Clavero and Villero, 2014), and this has accelerated exponentially since the beginning of the twentieth century (Vander Zanden and Olden, 2008; Clavero and Villero, 2014). The increasing introduction rate and spread of non-indigenous species are among the most critical threats to biodiversity and ecosystem services (Vitousek et al., 1996; Sala et al., 2000). Even though biological introductions may cause no detectable or long-term impact (Williamson and Fitter, 1996), some non-indigenous species, especially the invasive ones, usually display high spreading rates in the introduced environment causing significant impacts, from the alteration of habitats, replacement of native species through predation and competition, the transmission of diseases, and effects on human health and economy (Pimentel et al., 2000; Cowie, 2001; Blackburn et al., 2014; Gallardo et al., 2016). Identifying these invasive species is crucial for prioritizing management efforts (Ricciardi and Atkinson, 2004). Their control is often more practical, cheaper, and more effective soon after detection, and for some, eradication might be possible (Simberloff, 2009; Simberloff et al., 2011).
Freshwater ecosystems provide many benefits to humankind, and the induced changes (e.g., by invasive species) in the goods and services they provide can have a substantial impact on the human well-fare (Gherardi, 2007). Nevertheless, freshwater ecosystems are among the systems most heavily affected by non-indigenous species introduction (Amat-Trigo et al., 2019). The degradation of these ecosystems has caused non-indigenous species to establish and become invasive more easily than in other environments (Sala et al., 2000). Many of these species are effective colonizers that exhibit rapid adaptation in degraded aquatic or riparian habitats characterized by communities with a reduced competition that facilitate such adaptation (Conlan, 1994; MacNeil et al., 2004). The disturbance is commonly assumed to release resources and provide opportunities for invaders (Lozon and MacIsaac, 1997; Davis et al., 2000). Moreover, some invaders that inhabit human-disturbed environments in their native range might have a greater ability to adapt to human-disturbed environments than resident species (Niemelä and Mattson, 1996), giving them the advantages for successful invasion (Shea and Chesson, 2002). Also, systems already impaired by non-indigenous species are susceptible to additional disturbance, as non-indigenous species often facilitate each other’s establishment and/or their continued existence, increasing the likelihood and the magnitude of the global environmental impact inflicted by biological invasions (Gherardi, 2007).
Freshwater ecosystems are highly vulnerable to anthropogenic and natural introductions of species and their subsequent spread (Gherardi et al., 2008b; Strayer, 2010; Havel et al., 2015; Tricarico et al., 2016). The effects of intensive human usage and hydromorphological changes to aquatic systems, such as the impoundment of rivers (e.g., dams and weirs, water removal), water quality deterioration (e.g., pollution, eutrophication, acidification), habitat degradation and fragmentation (e.g., channelization and land-use change), resources overexploitation (Ricciardi, 2001), as well as climate change (Rahel and Olden, 2008) have been enhancing the dispersal of aquatic organisms (Gherardi et al., 2008a; Oscoz et al., 2010). Both anthropogenic habitat disturbance and the introduction of non-indigenous species are today the main drivers of biodiversity change in these ecosystems (Didham et al., 2005).
Aquatic non-indigenous organisms encompass a great variety of taxonomic groups, including microorganisms, plants, sponges, cnidaria, flatworms, molluscs, crustaceans, fishes, birds, amphibians, reptiles, and mammals (see García-Berthou et al., 2007; Nunes et al., 2015). However, most of the freshwater non-indigenous species that have been deliberately introduced are fishes and plants (Pimentel et al., 2005). Most freshwater species introduced in Europe are native to northern America and arrived in France, United Kingdom, or Germany, and spread from there to southern Europe, e.g., Portugal and Spain (García-Berthou et al., 2005; Boix et al., 2007). For example, the pumpkinseed [Lepomis gibbosus (Linnaeus, 1758)] and mosquito fishes (Gambusia spp.), which are American fish species (García-Berthou et al., 2005), are very well-established in Europe. One of the major pathways of freshwater species introduction is aquarium species trade, which accounts for 21% of world freshwater fish introductions (Andrews, 1990; Gozlan, 2008; Maceda-Veiga, 2013; Ishikawa and Tachihara, 2014; Nunes et al., 2015) and secondarily, aquaculture (Gherardi et al., 2008b; Nunes et al., 2015). In Europe, introductions related to ornamental trade were mostly freshwater plants (Keller et al., 2011). More than 400 non-indigenous aquatic and semi-aquatic species of plants are currently traded in Europe, and many are considered potentially invasive in European freshwater habitats (Hussner, 2012). Most of those introductions arrived in Portugal via Spain (García-Berthou et al., 2005). Nonetheless, some accidental freshwater introductions in Portugal seem to be related to escapes from cultivation sites and disseminated along watercourses. For example, red swamp crayfish [Procambarus clarkii (Girard, 1852)] might have arrived in Portugal from escapes in aquaculture facilities in Spain to the Guadiana river (Gherardi et al., 2002). Most imports to the Azores came from mainland Portugal, through commercial shipping and flights to the archipelago, mainly arriving on São Miguel island (Calado et al., 2014).
Oceanic islands are highly vulnerable to invasive species due to the low levels of diversity, lack of competitors and predators, and the resulting availability of ecological niches (Simberloff, 1995; Sax, 2001; Covich, 2010), offered by the existence of non-saturated assemblages (see Cornell and Lawton, 1992). Moreover, species on islands have small populations with restricted genetic diversity, and this, coupled with limited habitat availability, increases their vulnerability to environmental stresses, including the introduction of non-indigenous species (Russell et al., 2017). Therefore, insular freshwaters are among the most vulnerable ecosystems to invasive species (Raposeiro et al., 2009; Costa et al., 2013; Raposeiro et al., 2017).
The geographic setting of oceanic islands and the Azores archipelago presents a considerable distance across open ocean from a continental source which hampers natural dispersal and species colonization (Bilton et al., 2001; MacArthur and Wilson, 2001). In the Azores, isolation, small island area, young geology, and numerous volcanic eruptions have acted as strong biogeographical filters, resulting in low diversity of native biotic assemblages and a high percentage of freshwater faunal endemism (11% – Raposeiro et al., 2012), when compared to continental systems (Hughes, 2006). Since the establishment of the first human settlements in the islands (official Portuguese colonization in 1432 CE), pressure on the ecosystems has increased exponentially, mainly associated with landscape disturbance due to changes in land use which have resulted in habitat degradation and fragmentation and the introduction of non-indigenous species (Triantis et al., 2010; Connor et al., 2012; Ferreira et al., 2017; Raposeiro et al., 2017; Rull et al., 2017; Vázquez-Loureiro et al., 2019).
The consequences of introduced species have been an object of discussion in several studies (Mooney and Hobbs, 2000; Ricciardi, 2003; Bohlen et al., 2004), including in island ecosystems (Reaser et al., 2007), but the introduction of non-indigenous species and the impact of these in the highly vulnerable insular freshwater systems of the Azores has not yet been thoroughly addressed (Raposeiro et al., 2011a). The inventory of non-indigenous species in the archipelago and knowledge of their introduction pathways, establishment, and spread potential is vital to predicting threats to freshwater native biodiversity and to developing management strategies for local freshwater environments (Raposeiro et al., 2009; Lamelas-López et al., 2017).
This paper updates the knowledge on all non-indigenous freshwater species in the Azores through a broad taxonomic approach from unicellular phytoplankton to vertebrates, presenting what is known of their introduction history, pathways, origins, and ecological and socioeconomic impacts in the archipelago. Furthermore, species’ invasion risk and management actions are discussed.
Study Area
The Azores archipelago comprises nine islands of volcanic origin located in the middle of the northern Atlantic Ocean (Figure 1), between the latitudes, 36° 45′ N and 39° 43′ N and the longitudes 24° 45′ W and 31° 17′ W, about 1500 km off mainland Portugal (Santos et al., 2004). This archipelago is particularly rich in freshwater ecosystems due to volcanic geomorphology and climatic conditions that prevail in altitude with a total land surface area of 2,325 km2 drainage corresponding to 763 hydrographic basins (Cruz and Soares, 2018) and many small (maximum length of 29 km) streams (Raposeiro et al., 2013). Following its discovery in 1439, extensive deforestation began in coastal areas of hydrographic basins to establish human settlements that extended in the early 20th century. The intensification of agricultural activity, the excessive application of agrochemicals, building roads, effluent discharge of livestock farms, and the release or non-deliberate introduction of non-indigenous species are some of the main factors currently affecting the water quality and ecosystems services provided by these islands’ freshwater habitats (Pereira et al., 2014; Raposeiro et al., 2014).
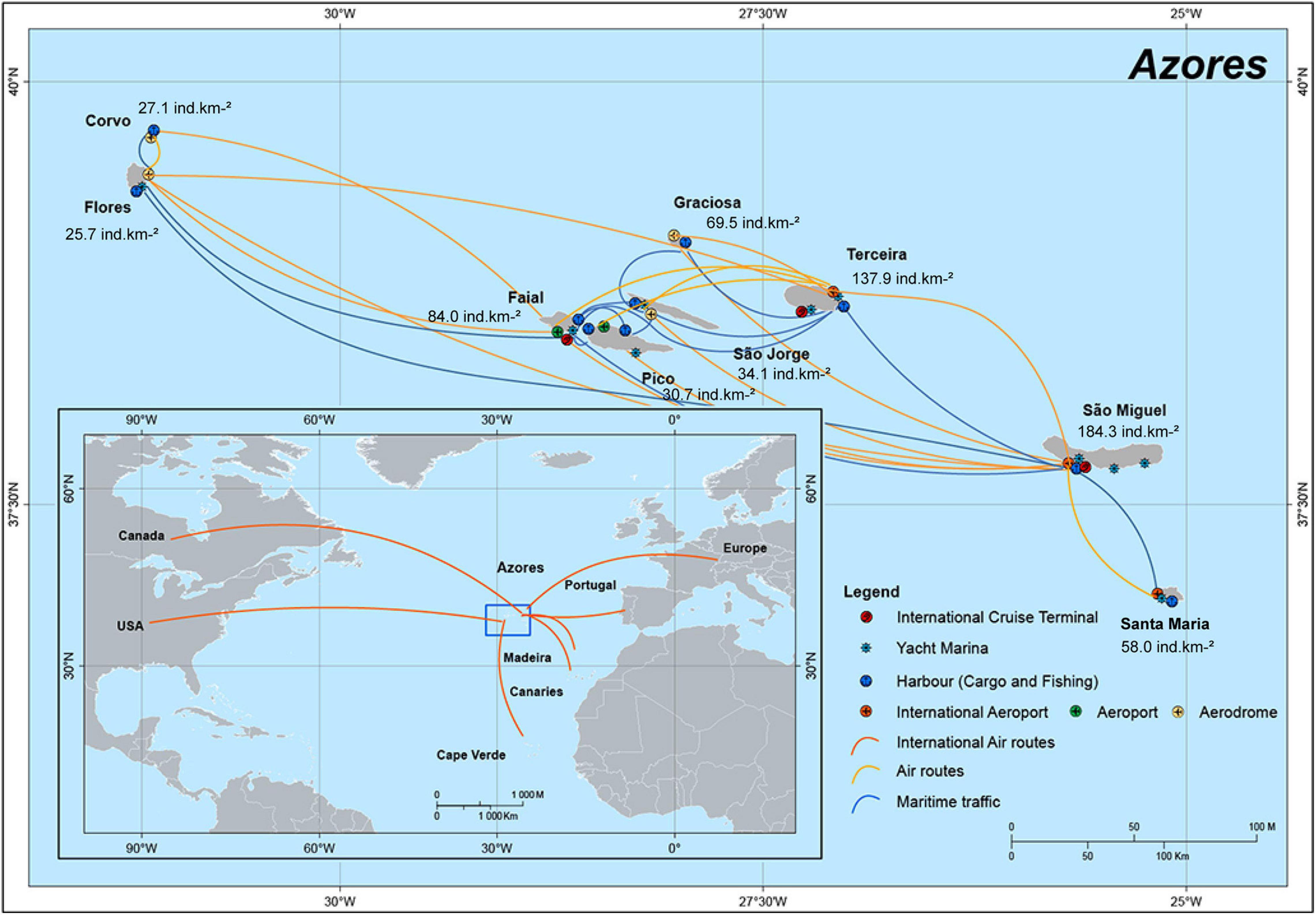
Figure 1. Location of Azores Archipelago on North Atlantic Ocean. Principal routes of entry for non-indigenous species onto the islands are presented.
Materials and Methods
The GBIF Backbone Taxonomy was used to harmonize the taxonomy of the studied groups (GBIF Secretariat, 2019). For the present paper, freshwater species were considered those organisms that complete their entire life cycle in the water [e.g., diatoms (Bacillariophyceae), molluscs (Mollusca), crustacea (Crustacea), fishes (Actinopterygii), cyanobacteria (Cyanobacteria), synurophytes (Synurophyceae) and desmids (Desmidiaceae)], animals that live in aquatic habitats at some point in their life cycle [e.g., some arthropods (Arthropoda), amphibia (Amphibia) and floating, submerged and helophyte plants (Tracheophyta) (Thorp et al., 2014; Bellinger and Sigee, 2015; Dodds and Whiles, 2019)].
Indigenous and non-indigenous species were categorized following criteria in Essl et al. (2018); indigenous species from the Azores were considered those not related to human-modified habitats or activities, occurring in native habitats, and also present in other Macaronesian archipelagos. Species that have human-mediated movement across biogeographic barriers from their presumed native/indigenous origin are considered non-indigenous. In the Azores, this biogeographic filter is evident due to its distance to the mainland (around 1,500 km) and the Atlantic Ocean’s physical barrier. Possible pathway analysis and their absence from pristine habitats had to be put into the equation to establish the species as non-indigenous. The review of the introduction and presence of non-indigenous freshwater species in the Azores considered all available records to date (see Supplementary Material 1), retrieved from various sources as historical and contemporary records, paleoenvironmental reconstructions, and field data from WFD monitoring programs in place for the last two decades.
According to the Convention on Biological Diversity (Convention on Biological Diversity, 2014), each species’ introduction pathway was determined, distinguishing intentional and/or non-deliberate introductions. The species introduction mechanism as importation, transport vector or dispersal corridor association and natural spread from a previously invaded region were considered and addressed to one of five groups (as the CBD 6th pathway is not locally applicable): Release – an intentional introduction for human use in the natural environment (e.g., biological control, fishery, hunting); Escape – the movement of non-indigenous species from confinement (e.g., aquaria, botanic gardens, zoos) to the wild; Transport-contaminants – the non-deliberate movement of organisms transferred through intentional trade (e.g., pets, food diseases, seeds); Transport-stowaway – the movement of organisms attached to transporting vessels and associated equipment and media (e.g., ballast water, boats, fishing equipment’s); and Unaided – secondary natural dispersal of organisms that have been introduced elsewhere through pathways 1–5.
Some of the non-indigenous species established in Azorean freshwater habitats were categorized as invasive species following the definitions of the International Union for Conservation of Nature (IUCN), US National Aquatic Invasive Species Act (NAISA), and the Convention on Biological Diversity (CBD). Thus, invasive species are herein considered as non-indigenous species whose introduction and/or spread outside their natural past or present distribution has led to their establishment and spread in an ecosystem or a natural or semi-natural habitat, being an agent of a change and threat to native biodiversity, economy, environment, human health, recreation, or public welfare.
We used information on the invasive potential of non-indigenous species from Delivering Alien Invasive Species Inventories for Europe (DAISIE), European Alien Species Information Network (EASIN), Global Invasive Species Database (GISD) databases to established them as invasive or non-invasive species. Moreover, field and published data about their spread capacity and proliferation, feeding habits, predation and competition behavior, and preferred habitat were also used as complementary information to define the invasive status of those species and determine their possible negative impact on archipelago’s ecosystems.
Literature Review and Historical Records
Historical data can provide important insight to understand biological invasions (Willis and Birks, 2006). The assignment of introduced status to the Azores islands species has also been inferred using historical records, including those from the first 17th century chronicles and reports from naturalist and scientific expeditions of the 19th century. Data on species were extracted from these sources and completed with information retrieved from different peer-reviewed published papers, gray literature, reports, and studies carried out in Azorean freshwater systems. Species introductions associated with developing human economic activities in the archipelago and clear evidence for direct human mediation were considered deliberate introductions, releases, or escapes. The more obvious cases are related to agricultural and ornamental plants introductions (Williamson, 1996; Heywood, 2012). Ornamental plants and fishes deliberately introduced in Azorean lakes for leisure, stocking, and fishing purposes, were the most prevalent, especially during the late 18th century (see Raposeiro et al., 2017 and references associated). Species that might have arrived in the archipelago due to indirect consequences of human actions, such as accidental transport related to habitat modification, eliminating indigenous competitors or predators, were also considered. The date of introduction was considered the date of the first record reported in the literature or the publication date when the introduction date was not mentioned.
Paleolimnology Records
Long-term data are essential to assign indigenous and non-indigenous species status and detect the exact date of a species introduction (Moser, 2004; Willis and Birks, 2006; Smol, 2014). To overcome the scarcity and incomplete historical records in the Azorean islands, environmental reconstructions based on long continuous sequences of natural archives (sediments older than 1450 AD) were used to establish species presence in pristine conditions before human settlement. Data from recent paleolimnological studies in the Azores (São Miguel, Pico, and Flores islands) that have been focused on climatic and environmental reconstruction using biological proxies, e.g., pollen, plant remains, frustules of diatoms, water fleas, and chironomids (van Leeuwen et al., 2005; Connor et al., 2012; Raposeiro et al., 2017; Rull et al., 2017; Vázquez-Loureiro et al., 2019) were considered for this new approach. For example, van Leeuwen et al. (2005) unequivocally confirm the status of Selaginella kraussiana (Kunze) A. Braun as native on the Azores islands. As a consequence, species only collected in sediments post-1450 AD were considered non-indigenous. Despite the usefulness of paleolimnological records as an essential tool to understand native biota, the preservation of organisms in the sediments depends on the type of organism and the environmental conditions at the time of sedimentation (Heiri et al., 2009; Smol, 2014). So, the absence of species in the paleolimnological registry does not, per se, qualifies them as non-native. To overcome this, paleolimnological data was only used to access the status of diatoms, which are well preserved in lake sediments. Only the species that were consistently present in the sediment after human arrival was considered non-native, while all species that occurred sporadically were excluded.
Field Records
Field data from WFD monitoring programs carried out between 2000 and 2020 covering several water masses on all islands were also used to compile plants, macroinvertebrates, microalgae, and cyanobacteria data. Due to the different distribution of water masses among islands, the number of sampling events is not the same for every island: 4 on Faial, 12 on Terceira, 55 on Corvo, 60 on Santa Maria, 212 on Pico, 421 on Flores, and 1101 on São Miguel. Records of recent fish releases on different islands were obtained by net-fishing field data for various projects (authors’ unpublished data).
Results and Discussion
Taxonomic Groups and Area of Origin of Non-indigenous Species
At least 132 non-indigenous species are established in Azorean freshwater ecosystems (see Supplementary Material 1), belonging to different taxonomic groups (Table 1). Plants (41), invertebrates (45), diatoms (20), and fishes (12) presented the highest number of records, followed by cyanobacteria (10), amphibia (2), synurophytes (1), and desmids (1). Plants and arthropods are the most representative contributing with 31.1 and 19.7%, respectively, of the total number of non-indigenous species, followed by diatoms (15.2%) and fishes (9.1%) (Table 1). Freshwater non-indigenous fauna represents a total of 59 species, of which 23.7% are vertebrates. Invasive species account for 51.5% (68 species) of Azorean non-indigenous freshwater species (Table 1). All amphibians and fishes, except for the native eel Anguilla anguilla (Linnaeus, 1758) are considered invasive species in the archipelago. Plants and mollusks also presented a higher percentage of invasive species (82.9 and 66.7%, respectively) than the other groups (Table 1). More than 60% of vascular plant flora in the terrestrial realm, all the mammals (except the Azorean bat) and reptiles are reported to be non-indigenous (Silva et al., 2008). As for marine species, the number of non-indigenous species reported for the Azores by Tsiamis et al. (2019) is 53 species, most of them macroalgae and invertebrates.
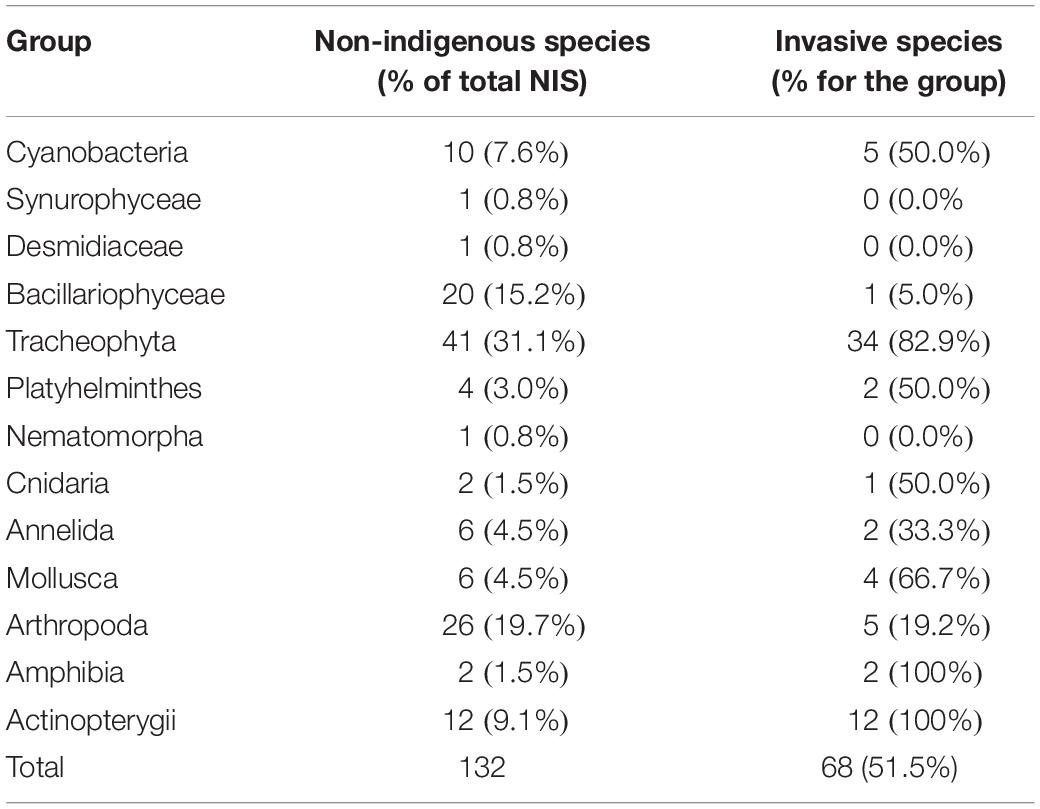
Table 1. Number and percentage (in brackets) of freshwater non-indigenous (NIS) and invasive species in different taxonomic groups.
The Azorean non-indigenous species are predominantly Palearctic (mostly invertebrates, amphibians, and fishes) and unknown (mostly cyanobacteria, diatoms, and desmids) in origin. Neotropic and Nearctic regions are also represented in non-indigenous species in the Azores in plants, Platyhelminthes, Mollusca, and fishes (Figure 2). According to historical records, some non-indigenous species might have been transported inadvertently by the Portuguese during the initial human colonization of the islands (archeophytes, i.e., introduced before 1500; Mandak and Pysek, 1998). However, the latest introductions in the late 19th century were deliberate releases with economic purposes for fish stocking or as ornamental plants for gardens. The first record of a species introduction is the goldfish [Carassius auratus (Linnaeus, 1758)] in 1792 (Ribeiro et al., 2009) (Figure 3), which is also the first record for freshwater species’ introduction in Portugal (Kottelat and Freyhof, 2007). It is often difficult to be sure whether humans have introduced insular species or whether they are, in fact, native. Paleolimnology records helped solve this question, especially in well-preserved groups as diatoms, plants, and some invertebrates. In the Azores, the significant impacts revealed by the sedimentary record are concurrent with the Portuguese colonization, providing evidence for strong anthropogenic landscape transformations leading to eutrophication (Connor et al., 2012; Raposeiro et al., 2017; Rull et al., 2017). Eighteen diatom taxa only appear in the records after the Portuguese settlement providing evidence that these species are non-indigenous [e.g., Gomphonema minutum (C.Agardh) C.Agardh, Fragilaria crotonensis Kitton, Asterionella formosa Hassall].
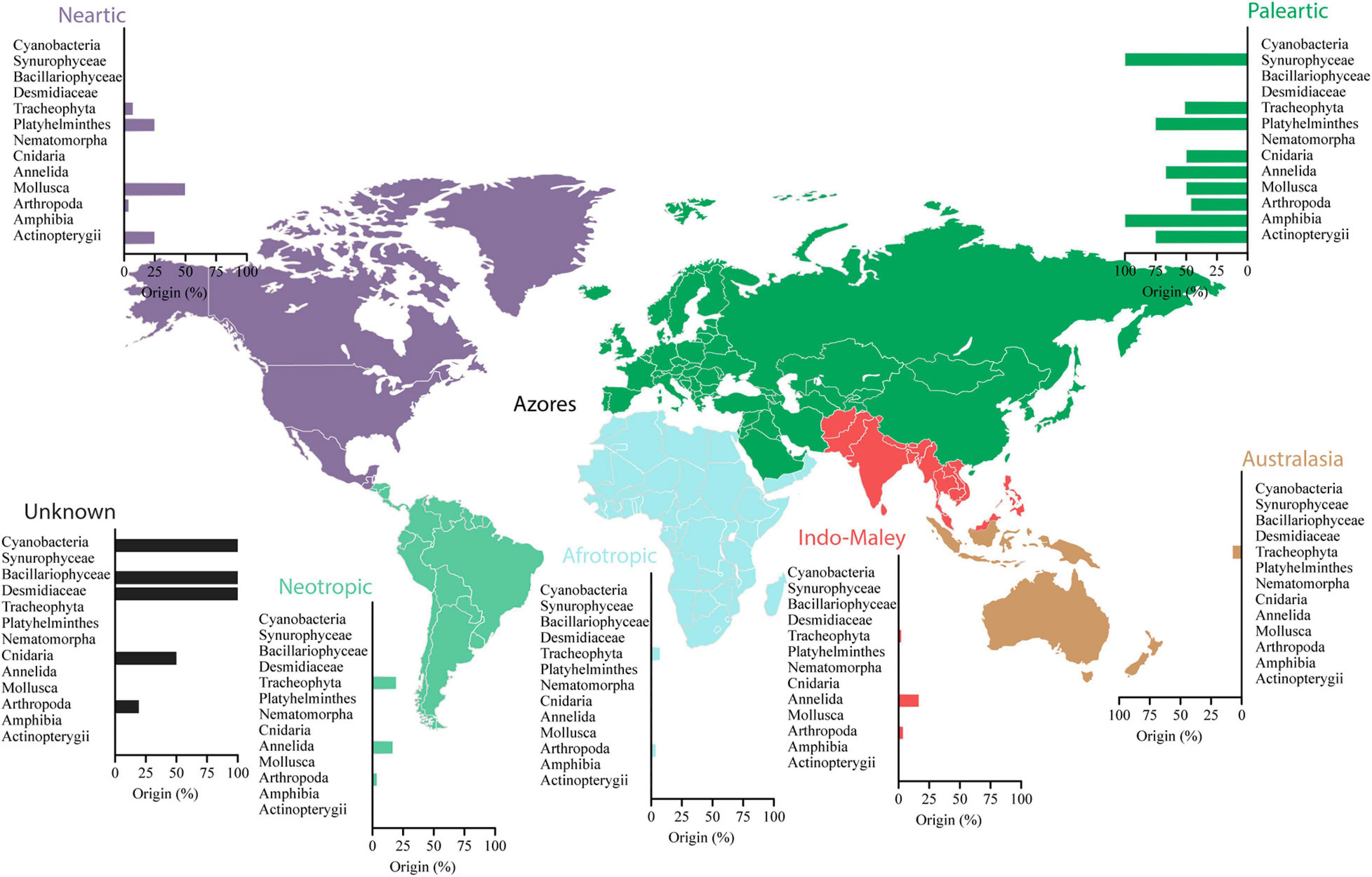
Figure 2. Percentage of freshwater non-indigenous species from different native distribution areas recorded in the Azores.
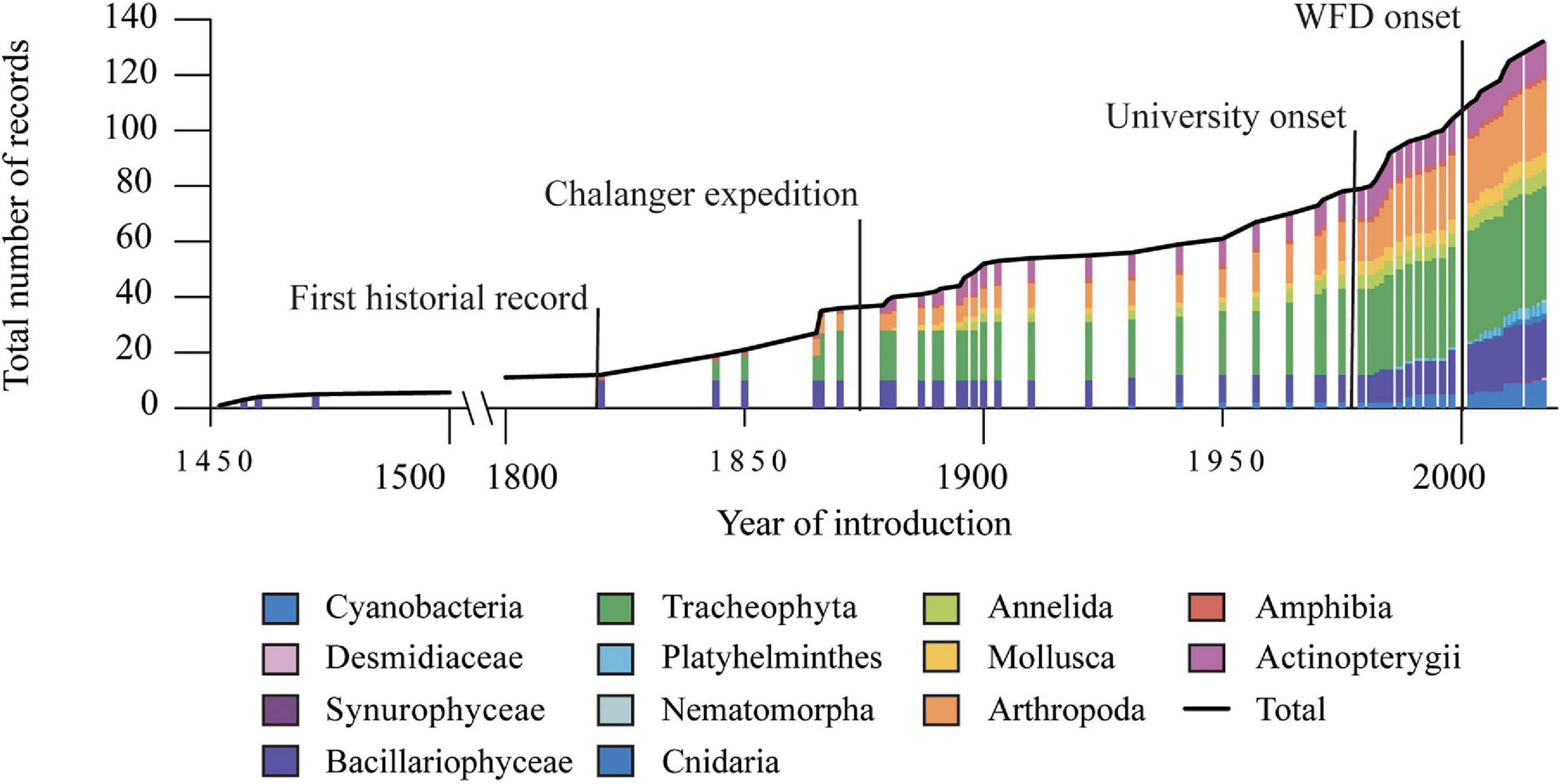
Figure 3. Records of non-indigenous species introduced in Azorean freshwaters from 1450 to 2020 over 50 years intervals.
Fishes and plants represented the groups for which first introductions have been reported (Figure 3) since the 19th century. The onset of the 19th-century naturalist expeditions to the archipelago, e.g., Challenger, encompass a growing number of studies on freshwater biota, increasing the number of non-indigenous species recorded. The number rose again in the second half of the 20th century when more studies on the lakes were carried out (Figure 3) due to the local university’s establishment and when the international airport in São Miguel started its regular operation. The number of freshwater species reported vastly increased in the last two decades, with new records of non-indigenous species resulting from the extensive sampling efforts in freshwater ecosystems due to WFD monitoring programs’ implementation. During this period, the records for cyanobacteria also started to become relevant.
Non-indigenous Species Distribution in the Archipelago
In the Azores archipelago, the highest number of non-indigenous species occurs on São Miguel Island (116), followed by Flores (68), Pico (65), and Terceira (56) (Figure 4). Although plants are the most represented group of introduced species on all islands, from 31.0% in São Miguel to 69.0% in São Jorge, invertebrates, diatoms, and fishes are also well established in São Miguel, Santa Maria, Pico, Flores, and Corvo islands. Non-indigenous species do not present a clear distribution pattern linked to human factors such as population density and/or the number of ports. Taxonomic distribution differences among islands are also not apparent (Figure 4). These results seem to be related to the sampling efforts associated with WFD monitoring programs during the last two decades in several islands (see field records in the section “Materials and Methods”). However, Terceira, Graciosa, São Jorge, and Faial showed higher percentages of non-indigenous plants (60.7, 63.0, 69.0, and 58.5%, respectively) than the rest of the groups (Figure 4). São Miguel and Flores are also the islands with higher habitat availability for freshwater species. Therefore, a higher number of successful introductions can be expectable as mentioned by several authors (Raposeiro et al., 2011a, 2013; Gonçalves et al., 2015). Habitat availability could also explain the distribution of specific groups, such as cyanobacteria and diatoms. Most non-indigenous cyanobacteria and several diatoms are planktonic and therefore restricted to islands with lakes. A similar situation is observed in the fish group, where most of the species were introduced in the lakes of São Miguel island during several decades, from where they were more recently spread to the rest of the archipelago.
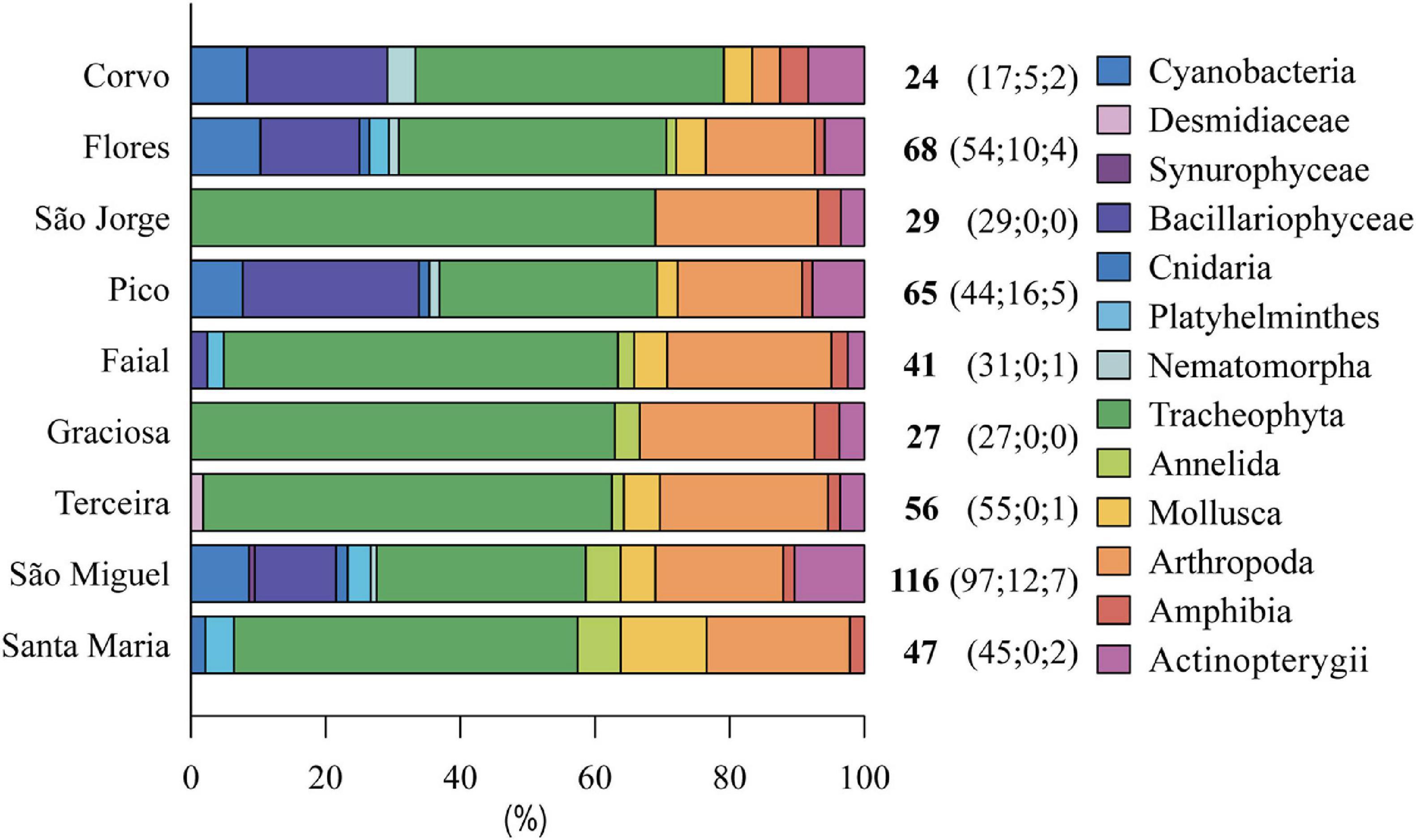
Figure 4. Percentage and total number (number of literature records; the number of paleolimnology records; field records) of non-indigenous species included in different taxonomic groups registered in freshwaters habitats for each Azorean island.
Among invertebrates, non-indigenous arthropods are the most well-established group on all islands (from 18.5% in Pico to 26% in Graciosa) except for Corvo where arthropods only represent 4.2% of introductions (Figure 4). The poor records on this island may be related to a lower number of studies, limiting the data available for this group.
Most invasive species are restricted to just one archipelago island (Figure 5), mainly on São Miguel, Flores, and Pico islands (Raposeiro et al., 2011b; Gonçalves et al., 2015). Half of invasive fish and amphibia and all invasive Annelida and Platyhelminthes are only present on São Miguel island (Figure 5). Being the largest and most populated island, São Miguel has greater connectivity with mainland Portugal, Europe, and America (Figure 1), becoming the main entrance of invasive species in the archipelago. This island is the main entry point of traded goods in the archipelago, functioning as a distribution hub for other islands. São Miguel is also the island with the most significant contribution to the archipelagos economy reflected in the economic indicators as importation/exportation of agriculture and fisheries’ products or tourism (FMS, 2013). The increased human pressure in São Miguel island leads to a higher habitat degradation posing more opportunities for opportunistic non-indigenous species to establish (Silva and Smith, 2004; Cardoso et al., 2013). This island has also hosted a higher number of studies (Johansson, 1976; Borges et al., 2010), and it has thus been more intensively sampled, which might also be reflected in the present results. Invasive plants, fishes, and arthropods present a larger dispersion in Azorean freshwater habitats, with several species established in eight islands (Figure 5). Amphibians occur on nine islands; however, this extended distribution is represented only by the green frog, Pelophylax perezi (López-Seoane, 1885). Other invasive species, Mollusca, Cnidaria, and Cyanobacteria, are scattered on three or four islands (Figure 5).
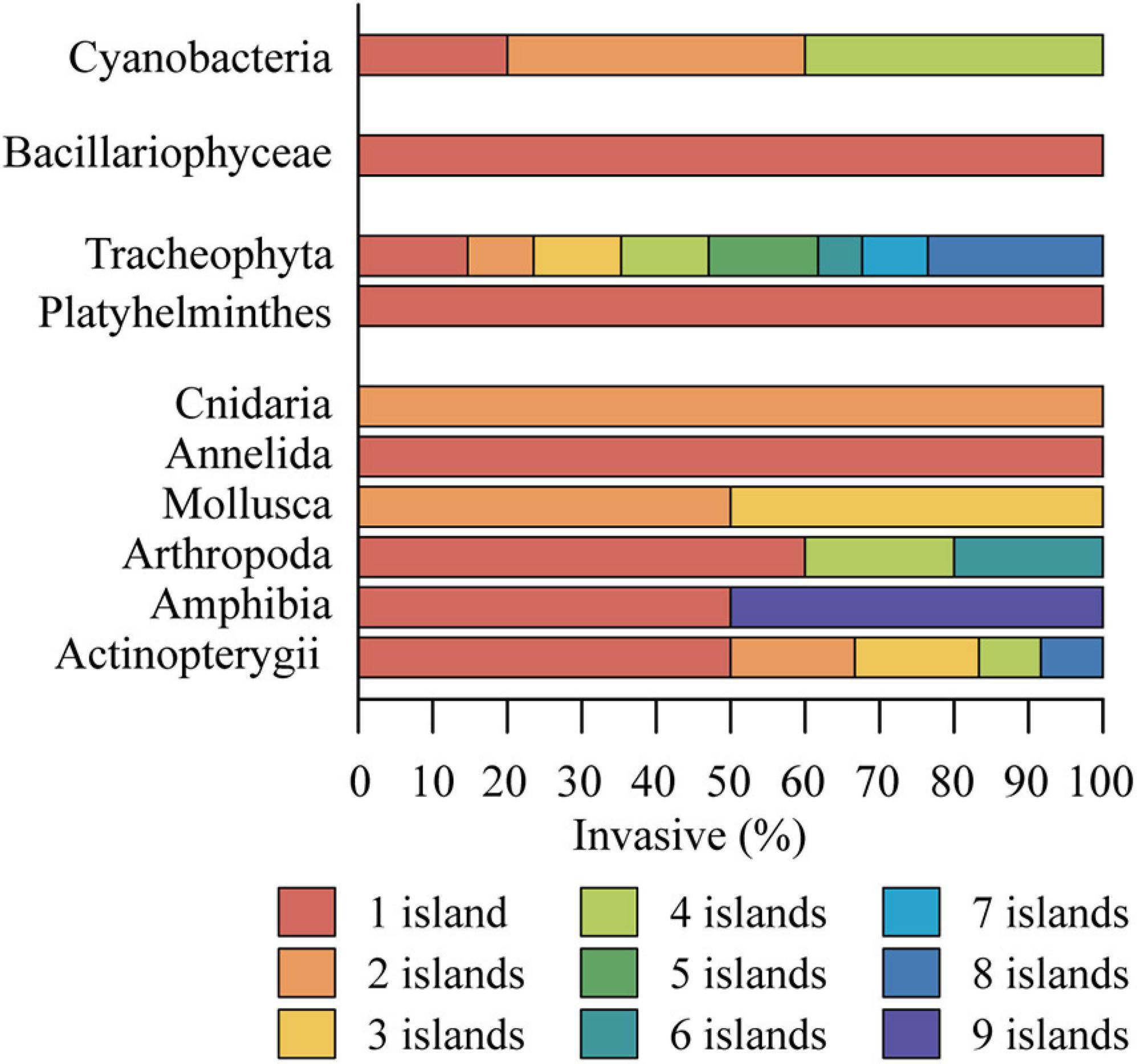
Figure 5. Percentage of invasive species of the different taxonomic groups present in one to nine islands. Synurophytes, Desmids, and Nematomorpha were not included due to the absence of invasive species in these groups.
Principal Introduction Pathways
Most non-indigenous species were probably accidentally introduced to the Azores archipelago, mainly inadvertently by the aquarium trade, attached to plants, as eggs, or in resistant life stages (“Transport-contaminant” pathway), such as Mollusca [e.g., Ferrissia fragilis (Tryon, 1863), Physella acuta (Draparnaud, 1805) and Galba truncatula (O. F. Müller 1774)], Platyhelminthes (e.g., Dugesia sp.) and Cnidaria (e.g., Hydra vulgaris Pallas, 1766 and Craspedacusta sowerbii Lankester, 1880). Arthropods may have been accidentally transported with agricultural products, including food, or packing material, including Coleoptera (mainly species of Hydrophilidae) and Diptera. Agricultural trade is also involved in the introduction of associated pests, such as the turfgrass pest Tipula oleracea Linnaeus, 1758 (“Transport-contaminant” pathway) (Figure 6).
Ornamental trade was inadvertently involved in the release and spread of many aquatic plant species that might have been imported for use in private or botanical gardens, an important leisure activity of the 19th century in islands as São Miguel, Terceira, and Faial. These aquatic species such as Zantedeschia aethiopica (L.) Spreng, Nymphaea alba L. or Eichhornia crassipes Mart. (Solms) escaped, invading large extensions of freshwater habitats (“Escape” pathway) (Figure 6). Another example of species that might have been introduced via domestic aquarium trade, probably by releases in private tanks and later escapes, eventually ending up in native aquatic ecosystems, is the crayfish, P. clarkii.
Some species might have been transported accidentally on fishing equipment, airplane wheels, and shoes, among others (“Transport-stowaway” pathway), but this introduction pathway is not easy to determine. The introduction pathway for most non-indigenous species in Azorean freshwaters was categorized as transport-contaminant movement (51.0%) (Figure 6). In addition to these accidental introductions, other species have been intentionally introduced. Since the late 18th century, intentional release is the main introduction pathway for fishes such as Cyprinus carpio Linnaeus, 1758, Perca fluviatilis Linnaeus, 1758, and Esox lucius Linnaeus, 1758 among others, attributed to angling activities in Azorean lakes. A well-known example of deliberate introduction is the release of Gambusia holbroki Girard, 1859 for biological control of mosquito populations on the mainland and Cabo Verde, another Macaronesian archipelago (Cabral and Marques, 1999; Salgueiro et al., 2019). However, in the Azores, the introduction of G. holbrooki was probably related to escapes from private aquaria. After the last known Rutilus rutilus (Linnaeus, 1758) introduction in 1983 in Lake Azul (Raposeiro et al., 2017), deliberate releases into the wild stopped. Annelids introduction pathway seems to be escapes related to leeches for medical purposes in the late 19th century (Chaves, 1949). Nevertheless, releases (10.0%) and escapes (15%) are less significant introduction pathways for non-indigenous freshwater species in the Azores (Figure 6) when compared with Transport/contamination pathway.
As for Cyanobacteria, Synurophytes, Diatoms, and Desmids, the introduction pathways remain uncertain. Natural dispersion of freshwater microalgae and cyanobacteria is mainly related to transportation by migratory waterbirds (Proctor, 1959) or other organisms (e.g., insects, mammals) (Kristiansen, 1996; Coste and Ector, 2000), water or wind (Sharma et al., 2007). Especially on remote oceanic islands, like the Azores, migratory waterbirds, which can transport microorganisms to very distant places, are probably the most important natural vectors of microalgae and cyanobacteria (Guerne, 1887; Proctor, 1959). Although the presence of non-native microalgae and cyanobacteria could be explained by the interplay of these natural dispersion mechanisms with ecological changes in target areas that enable their establishment (Cellamare et al., 2010), human facilitated transport (e.g., introduction of non-indigenous aquatic plants and fishes) is the most probable pathway of their invasion into new areas across the globe (Cellamare et al., 2010; Kaštovský et al., 2010) and in the Azores.
The international airport in Ponta Delgada (São Miguel) was inaugurated in 1969, and in the following three decades, escapes and transport/contaminant pathways were more critical than introductions. After 2000, escapes became a less clear pathway, which might be related to law enforcement related to living imports. Other pathways such as escapes and transport/contaminant, the first related to ornamental and aquariology trade, and the second with agriculture/livestock importations, are still very relevant and primary pathway in recent years (Supplementary Material 1).
Ecological and Economic Impacts
It is widely accepted that invasive species affect biodiversity and ecosystems and have socio-economic impacts (Pimentel et al., 2000). Traditionally, ecologists and invasion biologists have solely focused on the impacts of invasive species on biodiversity and ecosystem functions (Sala et al., 2000; Courchamp et al., 2017). However, the adoption of ecosystem services where biodiversity is considered within the context of human well-being has emphasized the need to consider people’s health, wildlife, and domestic animals (Pejchar and Mooney, 2009). Most of the time, it is challenging to quantify long-term ecological impacts because freshwater ecosystems adjust and evolve in response to invasions. However herein we present some examples on ecological and economical effects of species introductions with special emphasis in the Azores.
The introduction of predators (fishes) on the Azores lakes had marked effects on the trophic dynamics and ecological state of formerly fishless lakes. Particularly evident was the negative effect of C. auratus and C. carpio both of which have altered the trophic dynamics and the relative importance of benthic and pelagic production, contributing to the increase of turbidity, leading to a change in oxygen conditions of the hypolimnion of the deep lakes, and increasing the eutrophication processes in the lakes (Skov et al., 2010; Raposeiro et al., 2017). Additionally, direct predation has caused the disappearance of several native macroinvertebrate and zooplankton species (Skov et al., 2010; Buchaca et al., 2011; Raposeiro et al., 2017).
In the Azores, the introduction of Lymnaeidae snails [e.g., G. truncatula and Radix peregra (Müller, 1774) vectors of Fasciola hepatica Linnaeus, 1758] have contributed to significant revenue losses in livestock farms in São Miguel (Frias Martins, 1991), which highlights the importance to consider health and ecosystem services aspects in locally accessing introductions’ impacts.
Economic impacts are also associated with landscape deterioration due to the decline and impoverishment of ecosystem services (Fei et al., 2014). The introduction of non-indigenous cyanobacteria and plants such as Egeria densa Planch. has decreased water quality and increased turbidity of Azorean lakes, negatively impacting leisure recreational activities undertaken both by tourists and the local population (Santos et al., 2012; Cruz et al., 2015; Cordeiro et al., 2020). Moreover, climate changes may promote the spread of non-indigenous species that act as hosts of parasites and transmit human illness.
Despite the high number of non-indigenous species that may negatively affect ecology and economy, the introduction of some species may also have had socio-economic benefits like enhanced recreational fishing in Azorean lakes by fish stocking (Hunt et al., 2017).
Cyanobacteria, Diatoms, Desmids, and Synurophytes
Little is known about the distribution of freshwater cyanobacteria, desmids, synurophytes, and diatom species in the Azores (Jorgen Kristiansen, 1996; Foissner and Hawksworth, 2009). Nevertheless, cyanobacterial blooms were recorded for the first time in Azorean lakes, namely Sete Cidades and Furnas (e.g., Santos et al., 2005; Gonçalves, 2008; Cruz et al., 2015) during the late 1980s. Several well-known bloom-forming species associated with eutrophication, such as D. scheremetieviae, M. aeruginosa, M. flosaquae were not only recorded for the Azores between 1989 and 1991, but became dominant in several lakes. When these microorganisms become invasive, they contribute to the eutrophication of aquatic ecosystems (Hillebrand and Sommer, 2000), altering the structure of native communities significantly and leading to loss of biodiversity (Chapin et al., 2000; Korneva, 2014).
In general, invasions by microorganisms are poorly understood and, until recently, their impact on the environment has been underestimated (Foissner and Hawksworth, 2009; Kaštovský et al., 2010). Many species of cyanobacteria can produce toxins harmful to the ecosystem, and when present in drinking or recreational waters might lead to human health problems (Christoffersen and Kaas, 2000; Funari and Testai, 2008; Oscoz et al., 2010) that can vary from psychological effects, discomfort, nuisance, and phobias, to skin irritations, allergies, poisoning, disease and even death (Bayliss et al., 2017; Peyton et al., 2019). Examples of the latter can be posed by the invasive species Microcystis aeruginosa (Kützing) Kützing, Microcystis flosaquae (Wittrock) Kirchner, Aphanizomenon flos-aquae Ralfs ex Bornet & Flahault, Aphanizomenon gracile Lemmermann and Dolichospermum scheremetieviae (Elenkin) Wacklin, L. Hoffmann & Komárek, found in many lakes in the archipelago, often with high abundance (Santos et al., 2005; Cordeiro et al., 2020; Luz et al., 2020).
In addition to the predominance of cyanobacteria in most eutrophic lakes, which manifests the most significant degradation of these ecosystems’ biological quality, non-indigenous diatoms can also cause considerable damage to the environment. The invasive A. formosa and non-indigenous F. crotonensis, are widely distributed species whose populations have been increasing in numerous lakes worldwide (Gonçalves, 2008; Sivarajah et al., 2016). The species A. formosa has at times been the most abundant diatom in several Azorean lakes (Gonçalves, 2008). The synurophyte Synura petersenii Korshikov and the desmid Micrasterias papilifera Brébisson ex Ralfs are also considered non-indigenous and were recently found in one lake in São Miguel and Terceira islands, respectively.
Plants
Among the 41 plant species (see Supplementary Material 1), free-floating macrophytes such as the water hyacinth E. crassipes interfere with water utilization resources (Cook, 1990; Vereecken et al., 2006). Water hyacinth has invaded freshwater systems on five continents, and it is expected to expand in consequence of global warming (Kriticos and Brunel, 2016). In Portugal, the possession and sale of water hyacinth were prohibited by Law Decree no 165/74 (1974). Its mats with complex radicular structures prevent light penetration and water oxygenation with severe consequences to the autochthonous fauna and flora. Despite its presence on several islands of the archipelago (currently present in five of the nine islands), it has only been found in water tanks and ponds as ornamental, or on temporally waterlogged areas in Sete Cidades lakes (São Miguel Island). The local containment of this species is essential as it may constitute a threat for many small lakes in the Azores (Kriticos and Brunel, 2016).
The non-indigenous duckweeds Landoltia punctata (G. Meyer) D. H. Les & D. and Lemna minor L. can be aggressive invaders in aquatic ecosystems, whose colonies cover the surface of the water, causing oxygen depletion (Landolt, 1986). These plants should be controlled before they cover the entire water surface. Although they have a high dispersal capacity, populations in the archipelago are small and sparse.
Two species of Hydrocharitaceae recently introduced to the archipelago also stand out, Egeria densa and Elodea canadensis Michx. The first is disseminated worldwide and is capable of high-speed growth (Oliveira et al., 2005) due to fragmentation and posterior vegetative reproduction. E. densa is a very resistant species and tolerates an extensive range of nutrient, oxygen, carbon dioxide, and pH conditions (Matthews et al., 2014). In the Azores, it is present in three islands, but problems have been mainly reported in Sete Cidades and Furnas lakes (São Miguel Island) where the eutrophic conditions have been enhancing its growth. E. canadensis was first reported in 1970 for São Miguel island (Santos et al., 2005). These species introductions have been related to escapes from aquaria or deliberate ornamental plantations in natural ponds and subsequent dispersion by fragments through water flow or associated with human activities. Their impacts include restrictions on recreational activities, rising flooding risk, and interfering with some infrastructure such as turbines (Oliveira et al., 2005; Matthews et al., 2014).
A number of non-indigenous dicotyledonous helophytes develop on temporarily flooded soils, such as Apium nodiflorum (L.) Rchb.fil., Nasturtium officinale W.T.Aiton, Persicaria hydropiper (L.) Spach and Poaceae Glyceria fluitans R.Br. and are common species inhabiting small watercourses. Similarly, the emergent and submergent macrophytes (helophytes) can flourish in fens and temporarily or permanently flooded areas (Rivas-Martínez et al., 2001). In the Azores, these macrophyte communities, mainly composed of non-indigenous species, are favored by removing forest cover and the abusive use of water resources for both humans and livestock (Rodrigues et al., 2004). This ecotone has been extended in many aquatic margins where livestock access creates disturbed areas that facilitate invasive species spreading, such as Cyperus spp. and Typha spp.
Certain herbaceous invasive species, non-strictly aquatic, prefer wetland habitats, such as Ranunculus spp., Mentha spp. and Tradescantia fluminensis Vell., and form dense populations in moist and shady areas. With a short reproductive maturation and high levels of seed dispersal, these species are common on most islands. These reproductive strategies constitute a competitive advantage over native flora that inhabit similar environments.
Invertebrates
Non-indigenous invertebrate species that may cause ecological and socioeconomic impacts in Azorean freshwaters are represented by Platyhelminthes, Cnidaria, Annelida, Mollusca, and Arthropoda (Table 1). The species that have the most serious negative effects in freshwater ecosystems are described in detail.
Craspedacusta sowerbii Lankester, 1880 (Cnidaria, Hydrozoa) native from China (Jankowski, 2001), shows a cosmopolitan distribution due to its introduction in many freshwater habitats (Jankowski et al., 2008) on all continents except Antarctica (Rayner, 1988). Most of these introductions have been accidental and associated with fauna trade or ornamental plants (Jakovčev-Todorović et al., 2010). In 2010 some individuals were found in Congro lake, São Miguel Island (Raposeiro et al., 2011c). Recently, C. sowerbii has been discovered on other islands. C. sowerbii feeds on zooplankton (Smith and Alexander, 2008; Moreno-Leon and Ortega-Rubio, 2009) and may impact aquatic food webs by removal of a significant part of the zooplankton population, leading to changes in the structure of plankton community (Smith and Alexander, 2008) and cascading effects on primary producers (Brooks and Dodson, 1965; Carpenter and Kitchell, 1984; Williamson et al., 1989). Moreover, blooms of C. sowerbii, that often occur under eutrophic conditions and high temperatures, may trigger severe impact on the ecosystem (Davis, 1955; Jakovčev-Todorović et al., 2010).
Ferrissia fragilis (Mollusca, Gastropoda) is a native from North America and was probably introduced to the Azores by aquarists and/or associated with ornamental plants (“Transport-contaminant” pathway). This species was first observed on São Miguel Island in Sete Cidades Lake in 2004 (Raposeiro et al., 2011b). Since then, it has been found on Santa Maria, Graciosa, and Pico islands. Freshwater habitats where F. fragilis are present are usually characterized by high nutrient concentrations where these snails are typically found on submerged leaves of the introduced plant E. densa in shallow littoral shores. F. fragilis can also be found in submersed periphyton-covered stones or on plant detritus in weak flow areas (Raposeiro et al., 2013). The impact of this species is not clear (Raposeiro et al., 2011b). The impact in the Azores is probably masked by other introduced freshwater gastropods (Backhuys, 1975; Frias Martins, 1991; Raposeiro et al., 2012).
Galba truncatula (Mollusca, Gastropoda) introduction to the archipelago is possibly associated with imported sheep from mainland Portugal or aquarium trade (Duggan, 2010). Its introduction may have also been mediated by Nearctic birds or insects (Raposeiro et al., 2011a). The invasive potential of Lymnaeids species may be related to marked resistance to desiccation which increases their survival probability (Chapuis and Ferdy, 2012). When released in new environments, even a single individuals can develop a population as Lymnaeids can be self-fertilized (Escobar et al., 2011; Lounnas et al., 2018). Snails belonging to the Lymnaeidae family (Hurtrez-Boussès et al., 2001; Mas-Coma et al., 2005), such as G. truncatula and R. peregra, both introduced in the archipelago, are intermediary hosts for the Trematoda F. hepatica that contaminates part of São Miguel, Flores, and Santa Maria islands causing significant socioeconomic losses associated with damage to dairy livestock (Barbosa et al., 2019).
Procambarus clarkii, a crayfish native from North America and Mexico, is considered globally one of the most invasive aquatic species (Meineri et al., 2014; Arce and Diéguez-Uribeondo, 2015). In Portugal, it is forbidden under the Law Decree no 565/99 (1999) to keep and rear this species. P. clarkii was reported for the first time in São Miguel Island in 1994 (Correia and Costa, 1994; Costa et al., 1997), and it has since spread to Terceira Island. Its introduction in the Azores may be related to the aquarium trade, with an escape or release from a private aquarium or tank. This crayfish can actively disperse by land (Gherardi et al., 2000; Ramalho and Anastácio, 2014) and spread by passive vectors such as vehicles and animals (Águas et al., 2014; Anastácio et al., 2014). Its short life cycle, rapid growth, digging activity, and high populational densities facilitate its establishment (Gherardi and Acquistapace, 2007). In lakes in São Miguel island, P. clarkii is associated with the invasive species E. densa contributing actively to this plant fragmentation and dispersion. The presence of the red swamp crayfish in the archipelago is restricted to highly impacted systems, making its impact evaluation difficult, but it is probably lower than on the mainland. Interestingly, P. clarkii is now absent from Lagoa do Peixe, the small lake where its presence was first detected in São Miguel island, which seems to be a first record for a population extinction of this species.
Other non-indigenous species categorized as invasive in Azores freshwaters are the platyhelminth Dugesia tigrina (Girard, 1850); the annelids B. sowerbyi and Eiseniella tetraedra (Savigny, 1826); the gastropod P. acuta; the crustacean Argulus foliaceus (Linnaeus, 1758); and diptera considered urban and agricultural pests, as Culex pipiens Linnaeus, 1758 and T. oleracea.
Vertebrates
Non-indigenous amphibians in the Azores include, the frog P. perezi, introduced in 1820 and scattered on all the islands and by the crested newt Triturus cristatus carnifex (Laurenti, 1768) already present in 1922 on São Miguel (Svenberg, 1975), and both considered as invasive in the region. Due to the Azores’ oceanic geography, fishless lakes were common before human settlement, and the only native freshwater fish is A. anguilla, a common inhabitant of Azorean streams. In Azorean lakes, fishes’ introduction had a strong effect on macroinvertebrates communities, mainly due to the absence of native predators, with direct impacts on the diversity and abundance of prey populations (e.g., native invertebrates) (Skov et al., 2010; Raposeiro et al., 2017). Among the non-indigenous fishes introduced in the Azores (Supplementary Material 1), we mention the most abundant species with a negative impact on Azorean freshwaters.
Cyprinus carpio is the most abundant fish species in Azorean lakes, and is present on Flores, Pico and São Miguel islands (Gonçalves et al., 2006; Bio et al., 2008; Raposeiro et al., 2017). The first record of introduction dates from 1890 in São Miguel Island (Chaves, 1911). Recent introductions of fishes in the Azores have been for recreational fishing purposes (Anastácio et al., 2019), and dispersion among aquatic systems and islands is due to human action either by introductions from breeding facilities (e.g., trout) or by anglers’ translocations. C. carpio has a detritivorous bottom-feeding strategy consuming benthic invertebrates, removing sediments from the bottom, uprooting the macrophytes leading to the increase of water turbidity (Parkos et al., 2003; Miller and Crowl, 2006) and consequently, contributing to decrease water quality and habitat degradation (Lougheed et al., 1998; Buchaca et al., 2011). Moreover, C. carpio has a substantial impact on benthic communities by releasing nutrients from sediments thus and indirectly promoting algae blooms (Ribeiro et al., 2009; Raposeiro et al., 2017). C. carpio may also have socio-economic impacts through decreasing high value fish species by outcompeting with them and reducing recreational activities’ attractiveness. Increasing nutrient availability through sediment disturbance and excretion by fish may directly affect primary producers (quantity and diversity) and subsequent bottom–up consequences on food webs (Skov et al., 2010; Buchaca et al., 2011; Raposeiro et al., 2017).
Micropterus salmoides is a piscivorous species introduced in 1898 for fishing purposes. Nowadays, it is well distributed in Pico, São Miguel, Flores, and Corvo Island (unpublished data). This species may impact the ichthyofauna and amphibians, causing top-down consequences in trophic webs (Takamura, 2007).
Perca fluviatilis is planktivorous fish introduced for angling in São Miguel’s lakes in 1898, an activity long fomented by Forestry Services. Thus, anglers can act as dispersion vectors for some non-indigenous species, fishes, and accompanying species. P. fluviatilis, as a zooplanktivorous fish, may promote the decrease of native zooplankton densities, lowering grazing pressure on phytoplankton with resulting increased chlorophyll-a concentration, reduced water clarity, and enhanced eutrophication in Azorean lakes (Skov et al., 2010; Buchaca et al., 2011).
Management and Conservation Actions
Climate change and the increasing magnitude and frequency of introductions of species across geographic barriers resulting from international trade (see Hulme, 2009) are likely to change their establishment, spread, abundance, physiology, or phenology, potentially altering humans’ health impacts (Bayliss et al., 2017). Many non-indigenous species will likely benefit from climate change as some of their ecological traits provide high plasticity and adaptation abilities to cope with changing conditions (Hellmann et al., 2008). Global change may lead to new public health concerns as globalization increases the likelihood of disease vectors’ movement and facilitates the transmission of tropical and subtropical pathogens to higher latitudes and other Atlantic archipelagos (Seixas et al., 2019). Trade plays a vital role in the spread of invasive species and has arguably contributed to the recent acceleration of biological invasions (Seebens et al., 2015). The magnitude of merchandise imports is a determinant of the number of invasive species (Hulme, 2009), evidenced by correlations between invasive species richness and economic indicators (Dalmazzone, 2013). This has been proved stronger for islands than for continents, reflecting the more significant proportion of imports and higher establishment of invasive species (Hulme, 2009). Considering the observed trends in international trading, importations, and enhanced connectivity of the Azores archipelago as a result of the increasing number of flights and shipping (Vieira et al., 2019), unintentional introductions may accelerate beyond control if some preventive measures are not enforced (Howeth et al., 2016) due to enhanced propagule pressure. Higher connectivity among islands (Figure 1) is likely to improve non-indigenous freshwater species’ dispersal within the archipelago as accidental transportation seems to be an essential pathway for the non-indigenous freshwater species present in the Azores.
An important focus should be developing a biosecurity program addressing different sorts of species importation, improving the existing legislation and customs controls, and public campaigns to ensure good practices for watersheds users. For example, the “Clean Drain” movements, applied in the United States, New Zealand, and the United Kingdom, empowered recreational and other water users to promote biosecurity best practice to reduce the risk of accidental introduction and spread of aquatic IAS, encouraging people to check, clean and dry all equipment and clothing thoroughly (Beyer et al., 2011; Anderson et al., 2015). A relevant problem to address is the introduction of ornamental species. The ornamental plant industry needs “new” varieties of plants to offer to the public. Still, at the same time, there must be mechanisms to prevent non-indigenous species’ entry with high invasive potential. The large number of species coming from aquariums is worrying, and strict control of importation for business purposes must be put in place, and information and prevention campaigns should be carried out among the general public and anglers. The awareness-raising campaign could also include citizen science initiatives directed at key species that should be included in an early warning monitoring program. It is widely accepted that citizen science can play a significant role in public engagement. Their observations are crucial to informing policy on biodiversity conservation from government decisions to those made by local conservation managers (Theobald et al., 2015; Anderson et al., 2017). This is particularly relevant in restricting and preventing biological invasions (Theobald et al., 2015; Anderson et al., 2017; Chandler et al., 2017). A wealth of species occurrence data generated by citizen scientists enables surveillance of emerging and established invaders at larger spatial extents. Strictly regulating – or even prohibiting – the trade of species highly likely to become invasive, together with early alert and rapid response systems, is generally considered the most successful management strategy (Leung et al., 2002).
In parallel, monitoring of aquatic ecosystems would allow early detection of new species. To do this effectively, it is necessary to provide high quality and up-to-date information. Monitoring staff must be well trained in species identification to avoid confusion as occurred in the past, such as the case of clover fern Marsilea azorica Launert & J. Paiva (Schaefer et al., 2011). This species was described in 1983 and included as ‘critically endangered’ on the IUCN red list and as ‘strictly protected’ species by the Bern Convention and the European Union’s habitats directive, but was later identified by Schaefer et al. (2011) as Marsilea hirsuta R.Br., a non-indigenous species in the archipelago. A similar problem arose with Potamogeton lucens Nolte, subsequently determined by Kaplan and Zdenek (2005) as Potamogeton schweinfurthii A.Benn.
In the Azores case, the moderate climate without large annual fluctuations makes it possible for many species to potentially acclimatize (Walther et al., 2009; March-Salas and Pertierra, 2020), which would be more difficult elsewhere. Despite its oceanic isolation, the archipelago is halfway between the Americas and Europe, doubling the possible routes of entry for invasive species that can rapidly spread in the islands. Therefore, regarding the flora, most non-indigenous aquatic plants in the Azores should have monitoring plans due to the risk of rapid dispersal in lakes and water courses, and eradication should be advised for newly established species with limited distribution (Sharov, 2004). This should be considered, for instance, for controlling the water hyacinth E. crassipes in the Azores. The percentage of non-indigenous plants (69%) in the Azorean flora is relatively high (Silva and Smith, 2004). Some of these such as the terrestrial Hedychium gardnerianum Ker Gawl., Clethra arborea Vent., or Hydrangea macrophylla Thunb. although not strictly aquatic plants, strongly influence the Azorean freshwater ecosystems’ ecotone zones. Their presence at the margins of water courses, transport of rhizome fragments, deliberate introduction (hedges) allied with their aggressive nature, and strong presence in the archipelago will create severe problems in the structure, native status, and succession of these ecosystems. This complete alteration of riparian phytocenosis is of paramount importance since it provides the habitat and food for many organisms and influences aquatic microbial community composition (Ferreira et al., 2017).
Eutrophication is a slow natural process that has been dramatically accelerated by human activities, such as the runoff of excess fertilizer or sewage effluent in the Azores. Anthropogenic eutrophication is typically associated with higher primary productivity, higher oxygen demand, lower species richness, and species abundance changes (Engelhardt, 2011). One of the leading causes of this eutrophication in the Azores is the agricultural and livestock use of the territory, mainly focused on cattle. The alterations caused by livestock in the riparian areas are mostly three: physical destruction of the habitat by trampling, selective grazing of the vegetation, and excess supply of nutrients and seeds of non-indigenous species by excrements. This complex interaction of factors can lead over time to the complete alteration of delicate aquatic ecosystems by favoring invasion of non-indigenous plants in the riparian galleries and their proliferation in the water, as is the case of E. densa and non-indigenous cyanobacteria blooms.
Management actions against freshwater weeds were applied in some Azorean lakes (e.g., Sete Cidades lakes) using mechanical harvesting. Although large amounts of weed biomass were removed from the lakes (Santos et al., 2012) no ecological improvement was detected in these lakes. Mechanical approaches to control E. densa are known to be ineffective and can even enhance its dispersal and spread (Pennington, 2009). Massive removal of lake macrophytes can also resuspend lake sediments and release sediment-sequestered nutrients, favoring phytoplankton growth (Sayer et al., 2010; Quilliam et al., 2015) and enhancing eutrophication. Therefore, the removal of large stands of weeds in water bodies with massive proliferation is not recommended. However, removing non-indigenous macrophytes should be prioritized in recently invaded water bodies to contain or even eliminate them in the early invasion stages.
Another important factor contributing to eutrophication is the internal recycling of nutrients (Sondergaard et al., 2001). Fishes play an essential role in this process (Horppila et al., 1998; Buchaca et al., 2011), especially in ecosystems where they were naturally absent, as is the case of Azorean lakes (Skov et al., 2010; Raposeiro et al., 2017). Efforts were made in one lake (Lake Furnas, São Miguel Island) to reduce fish abundance to minimize eutrophication, and increase water quality (Bio et al., 2008). Despite the promising results of the first attempt to control fish populations in Azorean lakes, this approach was only applied for a short period (Bio et al., 2008). However, habitat restoration and implementation of land-use changes and more sustainable livestock and agriculture practices within some watersheds can not only fight the eutrophication problem but could also help manage the invasive species that benefit from nutrient-enriched conditions and impaired habitats. Some efforts have been put in place, e.g., on São Miguel, through watershed management plans, to tackle this problem (Cruz et al., 2015), but more robust measures have to be enforced to control of the freshwater invasive species spread.
A very positive point of Portuguese legislation in force on invasive species (Law Decree no 92/2019, 2019) is that investment is made in preventive culture. Monitoring, early detection, and rapid reaction mechanisms are put in place to contain the spread of invasive species. Despite including more invasive species than the previous law (Law Decree no 565/99, 1999), many species appear in this document as invasive only for the autonomous region of Madeira, leaving out the Azores, as it is the case of Adiantum raddianum C.Presl, Adiantum hispidulum Sw., Colocasia esculenta (L.) Schott, Holcus lanatus L., Z. aethiopica and Crocosmia x crocosmiiflora. The Regional Legislative Decree n.o 15/2012/A (2012) aims to regulate the import, holding, and introduction into the territory of the Autonomous Region of the Azores of non-indigenous species, in annex XI (Animal specimens whose introduction is permitted in the Autonomous Region of the Azores). However this decree concerning pets allows the entry into the islands of two groups of animals whose dispersion in the aquatic systems of the archipelago is problematic: “(e) Turtles (reptiles of the Chelidae family) and (f) Freshwater aquarium fish when produced in captivity.” Another point to note is the absence in Annex IX (List of species of fauna and flora invasive or at known ecological risk) of a fish list, which does appear in national law (Regional Legislative Decree n.o 15/2012/A, 2012). Moreover, in recent years, efforts have been made to reinforce controls at border levels by customs in local airports and ports.
River basin management plans implemented in the framework of WFD should also be an instrument for invasive species control. Even though non-indigenous species are not explicitly referred in the WFD, the directive aims to restore pristine communities in European water bodies, which implies that invasive species must be removed from or controlled within these ecosystems. Unfortunately, most European countries do not include invasive species in WFD assessments, and many do not take them into account in the status classification (Boon et al., 2020). Although in the Azores invasive species are not used for the ecological status classification, they negatively impact water bodies, and measures to control invasive plants in transitional waters were proposed in the Azores river basin management plan (AHA-DRA, 2015). Due to their impact on native communities and ecological quality, invasive species can preclude the achievement of WFD environmental objectives of good water status in water bodies. Therefore, more actions to control invasive species must be considered in future river basin management plans.
Final Remarks
In island freshwater ecosystems, it is challenging to predict vulnerability to non-indigenous species. Still, it is crucial to compile a detailed list of species, distribution, and associated environmental and historical information. Using this information combined with alternative approaches, like paleolimnological reconstruction, a comparative analysis of past introductions could be a significant step to understand the consequence of invasive species on insular freshwater systems. Detailed and general knowledge of the principal pathways and efforts to enhance environmental conditions would minimize invaders’ success within fragile insular ecosystems. Also, control measures such as checking the consignment both by air and sea for non-indigenous species would prevent new species’ entrance. In contrast, a more conscious and educated behavior from all users (e.g., tourists, anglers, and aquariologists) and management measures to improve water quality will prevent the spread of present invasions. Furthermore, systematic monitoring of these habitats and biota should be carried out to provide additional information that is essential for improving freshwater management, sustainable development, and ensuring the functioning and services of freshwater ecosystems.
Managing non-indigenous species on remote oceanic islands requires a well-coordinated strategy among all stakeholders. In the future, we must take into account that expanding international trade, tourism, transport, and climate change will probably facilitate the entry, spread, and establishment of non-native species through new pathways. Future actions such as political awareness and compromise and public awareness and education concerning species introductions are needed to tackle this problem.
Data Availability Statement
The original contributions presented in the study are included in the article/Supplementary Material, further inquiries can be directed to the corresponding author/s.
Author Contributions
AC and PR contributed to the conception and design of this study. All the authors contributed to the manuscript with essential inputs from AB (fauna species lists and data compilation), PR (data treatment), and SS (microalgae and cyanobacteria data compilation), largely contributed to the manuscript writing, and approved the submitted version. VG contributed microalgae, cyanobacteria and management, and ecosystem data analysis. MS contributed plants and management sections.
Funding
Part of this study was financed by Portuguese National Funds, through FCT - Fundação para a Ciência e a Tecnologia, the European Union, QREN, FEDER, COMPETE, by funding the CIBIO/InBIO (project UID/BIA/50027/2020 and POCI-01-0145-FEDER-006821), DISCOVERAZORES Project (PTDC/CTA-AMB/28511/2017P), P. M. Raposeiro and M. S. Souto (DL57/2016/ICETA/EEC2018/25 and ICETA 2019/56). This study was also financed by PRIBES project (Direcção Regional do Ambiente – LIFE17 IPE/PT/000010).
Conflict of Interest
The authors declare that the research was conducted in the absence of any commercial or financial relationships that could be construed as a potential conflict of interest.
Acknowledgments
The surveys were performed to comply with the current laws of Portugal. We want to thank all the collaborators from FRESCO – Freshwater Ecology research group from CIBIO-A (University of the Azores), which somehow helped gather data for this paper. Thanks are also due to Erik Zettler for his comments and language revision. We also thank the three reviewers and the editor whose comments/suggestions helped to improve and clarify this manuscript.
Supplementary Material
The Supplementary Material for this article can be found online at: https://www.frontiersin.org/articles/10.3389/fevo.2021.631214/full#supplementary-material
References
Águas, M., Banha, F., Marques, M., and Anastácio, P. M. (2014). Can recently-hatched crayfish cling to moving ducks and be transported during flight? Limnologica 48, 65–70. doi: 10.1016/j.limno.2014.07.001
AHA-DRA (2015). Plano de Gestão da Região Hidrográfica dos Açores – RH9. Versão para consulta pública (Azores River Basin Management Plan). Ponta Delgada: AHA-DRA.
Amat-Trigo, F., Forero, M. T., Ruiz-Navarro, A., and Oliva-Paterna, F. J. (2019). Colonization and plasticity in population traits of the invasive Alburnus alburnus along a longitudinal river gradient in a Mediterranean river basin. Aquat. Invasions 14, 310–331. doi: 10.3391/ai.2019.14.2.10
Anastácio, P. M., Ferreira, M. P., Banha, F., Capinha, C., and Rabaça, J. E. (2014). Waterbird-mediated passive dispersal is a viable process for crayfish (Procambarus clarkii). Aquat. Ecol. 48, 1–10. doi: 10.1007/s10452-013-9461-0
Anastácio, P. M., Ribeiro, F., Capinha, C., Banha, F., Gama, M., Filipe, A. F., et al. (2019). Non-native freshwater fauna in Portugal: a review. Sci. Total Environ. 650, 1923–1934. doi: 10.1016/j.scitotenv.2018.09.251
Anderson, L. G., Chapman, J. K., Escontrela, D., and Gough, C. L. A. (2017). The role of conservation volunteers in the detection, monitoring and management of invasive alien lionfish. Manag. Biol. Invasions 8, 589–598. doi: 10.3391/mbi.2017.8.4.14
Anderson, L. G., Dunn, A. M., Rosewarne, P. J., and Stebbing, P. D. (2015). Invaders in hot water: a simple decontamination method to prevent the accidental spread of aquatic invasive non-native species. Biol. Invasions 17, 2287–2297. doi: 10.1007/s10530-015-0875-6
Andrews, C. (1990). The ornamental fish trade and fish conservation. J. Fish Biol. 37, 53–59. doi: 10.1111/j.1095-8649.1990.tb05020.x
Arce, J. A., and Diéguez-Uribeondo, J. (2015). Structural damage caused by the invasive crayfish Procambarus clarkii (Girard, 1852) in rice fields of the Iberian Peninsula: a study case. Fund. Appl. Limnol. 186, 259–269. doi: 10.1127/fal/2015/0715
Backhuys, W. (1975). Zoogeography and Taxonomy of the Land and Freshwater Molluscs of the Azores. Amsterdam: Backhuys & Meesters.
Barbosa, R., Pinto, C., Garcia, P., and Rodrigues, A. (2019). Prevalence of fasciolosis in slaughtered dairy cattle from São Miguel Island, Azores, Portugal. Vet. Parasitol. Reg. Stud. Rep. 17:100319. doi: 10.1016/j.vprsr.2019.100319
Bayliss, H. R., Schindler, S., Adam, M., Essl, F., and Rabitsch, W. (2017). Evidence for changes in the occurrence, frequency or severity of human health impacts resulting from exposure to alien species in Europe: a systematic map. Environ. Evid. 6:21. doi: 10.1186/s13750-017-0100-4
Bellinger, E. G., and Sigee, D. C. (2015). Freshwater Algae: Identification, Enumeration and Use as Bioindicators, Second Edn. Hoboken, NJ: Wiley-Blackwell.
Beyer, J., Moy, P., and De Stasio, B. (2011). Acute upper thermal limits of three aquatic invasive invertebrates: hot water treatment to prevent upstream transport of invasive species. Environ. Manage. 47, 67–76. doi: 10.1007/s00267-010-9573-4
Bilton, D. T., Freeland, J. R., and Okamura, B. (2001). Dispersal in freshwater invertebrates. Annu. Rev. Ecol. Syst. 32, 159–181. doi: 10.1146/annurev.ecolsys.32.081501.114016
Bio, A., Prestes, A., Vieira, N., Valente, A., and Azevedo, J. (2008). Effects of fish removal in the Furnas Lake, Azores. Arquipélago Life Mar. Sci. 25, 77–87.
Blackburn, T. M., Essl, F., Evans, T., Hulme, P. E., Jeschke, J. M., Kühn, I., et al. (2014). A unified classification of alien species based on the magnitude of their environmental impacts. PLoS Biol. 12:e1001850. doi: 10.1371/journal.pbio.1001850
Bohlen, P. J., Groffman, P. M., Fahey, T. J., Fisk, M. C., Suárez, E., Pelletier, D. M., et al. (2004). Ecosystem consequences of exotic earthworm invasion of north temperate forests. Ecosystems 7, 1–12. doi: 10.1007/s10021-003-0126-z
Boix, D., Clavero, M., and Garcı, E. (2007). Biological Invaders in Inland Waters: Profiles, Distribution, and Threats. Cham: Springer.
Boon, P. J., Clarke, S. A., and Copp, G. H. (2020). Alien species and the EU Water Framework Directive: a comparative assessment of European approaches. Biol. Invasions 22, 1497–1512. doi: 10.1007/s10530-020-02201-z
Borges, P. A. V., Costa, A., Cunha, R., Gabriel, R., Gonçalves, V., Martins, A. F., et al. (2010). Listagem dos Organismos Terrestres e Marinhos dos Açores, 1st Edn. Princípia.
Brooks, J. L., and Dodson, S. I. (1965). Predation, body size, and composition of plankton 12:43. New Ser. 150, 28–35.
Buchaca, T., Skov, T., Amsinck, S. L., Gonçalves, V., Azevedo, J. M. N., Andersen, T. J., et al. (2011). Rapid ecological shift following piscivorous fish introduction to increasingly eutrophic and Warmer Lake Furnas (Azores Archipelago, Portugal): a paleoecological approach. Ecosystems 14, 458–477. doi: 10.1007/s10021-011-9423-0
Cabral, J. A., and Marques, J. C. (1999). Life history, population dynamics and production of eastern mosquitofish, Gambusia holbrooki (Pisces, Poeciliidae), in rice fields of the lower Mondego River Valley, Western Portugal. Acta Oecol. 20, 607–620. doi: 10.1016/S1146-609X(99)00102-2
Calado, H., Bragagnolo, C., Silva, S. F., and Pereira, M. (2014). A multi-scale analysis to support the implementation of a regional conservation policy in a small-island archipelago – the Azores, Portugal. J. Coast. Res. 70, 485–489. doi: 10.2112/SI70-082.1
Cardoso, P., Rigal, F., Fattorini, S., Terzopoulou, S., and Borges, P. A. V. (2013). Integrating landscape disturbance and indicator species in conservation studies. PLoS One 8:e63294. doi: 10.1371/journal.pone.0063294
Carpenter, S. R., and Kitchell, J. F. (1984). Plankton community structure and limnetic primary production. Am. Nat. 124, 159–172. doi: 10.1086/284261
Cellamare, M., Leitão, M., Coste, M., Dutartre, A., and Haury, J. (2010). Tropical phytoplankton taxa in Aquitaine lakes (France). Hydrobiologia 639, 129–145. doi: 10.1007/s10750-009-0029-x
Chandler, M., See, L., Copas, K., Bonde, A. M. Z., López, B. C., Danielsen, F., et al. (2017). Contribution of citizen science towards international biodiversity monitoring. Biol. Conserv. 213, 280–294. doi: 10.1016/j.biocon.2016.09.004
Chapin, F. S., Zavaleta, E. S., Eviner, V. T., Naylor, R. L., Vitousek, P. M., Reynolds, H. L., et al. (2000). Consequences of changing biodiversity. Nature 405, 234–242. doi: 10.1038/35012241
Chapuis, E., and Ferdy, J.-B. (2012). Life history traits variation in heterogeneous environment: the case of a freshwater snail resistance to pond drying. Ecol. Evol. 2, 218–226. doi: 10.1002/ece3.68
Chaves, A. (1949). Introdução de algumas espécies zoológicas na Ilha de São Miguel depois da sua descoberta. Açoreana 4, 325–342.
Chaves, F. A. (1911). “Introdução de algumas espécies zoológicas na ilha de S. Miguel depois da dus descoberta,” in Proceedings of the Conferência Realizada no Ateneau Comercial no dia 14 de Janeiro de 1909 - Ponta Delgada: Tipografia “Diário dos Açores, Portugal.
Christoffersen, K., and Kaas, H. (2000). Toxic cyanobacteria in water. A guide to their public health consequences, monitoring, and management. Limnol. Oceanogr. 45, 1212–1212. doi: 10.4319/lo.2000.45.5.1212
Clavero, M., and Villero, D. (2014). Historical ecology and invasion biology: long-term distribution changes of introduced freshwater species. Bioscience 64, 145–153. doi: 10.1093/biosci/bit014
Conlan, K. E. (1994). Amphipod crustaceans and environmental disturbance: a review. J. Nat. Hist. 28, 519–554. doi: 10.1080/00222939400770241
Connor, S. E., van Leeuwen, J. F. N., Rittenour, T. M., van der Knaap, W. O., Ammann, B., and Björck, S. (2012). The ecological impact of oceanic island colonization - a palaeoecological perspective from the Azores. J. Biogeogr. 39, 1007–1023. doi: 10.1111/j.1365-2699.2011.02671.x
Convention on Biological Diversity (2014). Pathways of Introduction of Invasive Species, their Prioritization and Management. Available online at: https://www.cbd.int/doc/meetings/sbstta/sbstta-18/official/sbstta-18-09-add1-en.pdf
Cook, C. D. K. (1990). “Origin, autecology, and sptread of troublesome aquatic weeds,” in Aquatic Weeds: The Ecology and Management of Nuisance Aquatic Vegetation, eds K. Pieterse and A. H. Murphy (Oxford: Oxford University), 31–38.
Cordeiro, R., Luz, R., Vasconcelos, V., Fonseca, A., and Gonçalves, V. (2020). A critical review of cyanobacteria distribution and cyanotoxins occurrence in Atlantic Ocean Islands. Cryptogam. Algol. 41, 73–89. doi: 10.5252/cryptogamie-algologie2020v41a9
Cornell, H. V., and Lawton, J. H. (1992). Species interactions, local and regional processes, and limits to the richness of ecological communities: a theoretical perspective. J. Anim. Ecol. 61:1. doi: 10.2307/5503
Correia, A. M., and Costa, A. C. (1994). Introduction of the red swamp crayfish Procambarus clarkii (Crustacea, Decapoda) in São Miguel, Azores, Portugal. Arquipélago - Life Mar. Sci. 12A, 67–73.
Costa, A. C., Correia, A. M., Rodrigues, M. L., Am Correia, A., Rodrigues, M. L., Correia, A. M., et al. (1997). Monitoring a population of Procambarus clarkii (Decapoda, Cambaridae) in Sao Miguel (Azores, Portugal). Freshw. Crayfish J. Astacol. 11, 203–212.
Costa, H., Bettencourt, M. J., Silva, C. M. N., Teodósio, J., Gil, A., and Silva, L. (2013). “Invasive alien plants in the azorean protected areas: invasion status and mitigation actions,” in Plant Invasions in Protected Areas: Patterns, Problems and Challenges, eds P. Pyšek, L. C. Foxcroft, P. Genovesi, and D. M. Richardson (Netherlands: Springer), 375–394.
Coste, M., and Ector, L. (2000). Diatomées invasives exotiques ou rares en France: principales observations effectuées au cours des dernières décennies. Syst. Geogr. Plants 373–400. doi: 10.2307/3668651
Courchamp, F., Fournier, A., Bellard, C., Bertelsmeier, C., Bonnaud, E., Jeschke, J. M., et al. (2017). Invasion biology: specific problems and possible solutions. Trends Ecol. Evol. 32, 13–22. doi: 10.1016/j.tree.2016.11.001
Covich, A. P. (2010). Winning the biodiversity arms race among freshwater gastropods: competition and coexistence through shell variability and predator avoidance. Hydrobiologia 653, 191–215. doi: 10.1007/s10750-010-0354-0
Cowie, R. H. (2001). Invertebrate invasions on pacific islands and the replacement of unique native faunas: a synthesis of the land and freshwater snails. Biol. Invasions 3, 119–136. doi: 10.1023/A:1014529019000
Cruz, J. V., Pacheco, D., Porteiro, J., Cymbron, R., Mendes, S., Malcata, A., et al. (2015). Sete Cidades and Furnas lake eutrophication (São Miguel, Azores): analysis of long-term monitoring data and remediation measures. Sci. Total Environ. 520, 168–186. doi: 10.1016/j.scitotenv.2015.03.052
Cruz, J. V., and Soares, N. (2018). Groundwater governance in the Azores archipelago (Portugal): valuing and protecting a strategic resource in small islands. Water 10:408. doi: 10.3390/w10040408
Dalmazzone, S. (2013). “Economic factors affecting vulnerability to biological invasions,” in Econ. Biol. Invasions, eds C. Perrings, M. Williamson, and S. Dalmazzone (Cheltenham: Edward Elgar).
Davis, C. C. (1955). Notes on the food of Craspedacusta sowerbii in Crystal Lake, Ravenna, Ohio. Ecology 36, 364–366. doi: 10.2307/1933253
Davis, M. A., Grime, J. P., and Thompson, K. (2000). Fluctuating resources in plant communities: a general theory of invasibility. J. Ecol. 88, 528–534. doi: 10.1046/j.1365-2745.2000.00473.x
Didham, R. K., Tylianakis, J. M., Hutchison, M. A., Ewers, R. M., and Gemmell, N. J. (2005). Are invasive species the drivers of ecological change? Trends Ecol. Evol. 20, 470–474. doi: 10.1016/j.tree.2005.07.006
Duggan, I. C. (2010). The freshwater aquarium trade as a vector for incidental invertebrate fauna. Biol. Invasions 12, 3757–3770. doi: 10.1007/s10530-010-9768-x
Engelhardt, K. A. M. (2011). “Eutrophication – aquatic,” in Encyclopedia of Biological Invasions, eds D. Simberloff and M. Rejmanek (Los Angeles: University of California Press), 209–213.
Escobar, J. S., Auld, J. R., Correa, A. C., Alonso, J. M., Bony, Y. K., Coutellec, M. A., et al. (2011). Patterns of mating-system evolution in hermaphroditic animals: correlations among selfing rate, inbreeding depression, and the timing of reproduction. Evolution 65, 1233–1253. doi: 10.1111/j.1558-5646.2011.01218.x
Essl, F., Bacher, S., Genovesi, P., Hulme, P. E., Jeschke, J. M., Katsanevakis, S., et al. (2018). Which taxa are alien? criteria, applications, and uncertainties. Bioscience 68, 496–509. doi: 10.1093/biosci/biy057
Fei, S., Phillips, J., and Shouse, M. (2014). Biogeomorphic impacts of invasive species. Annu. Rev. Ecol. Evol. Syst. 45, 69–87. doi: 10.1146/annurev-ecolsys-120213-091928
Ferreira, V., Faustino, H., Raposeiro, P. M., and Gonçalves, V. (2017). Replacement of native forests by conifer plantations affects fungal decomposer community structure but not litter decomposition in Atlantic island streams. For. Ecol. Manage. 389, 323–330. doi: 10.1016/j.foreco.2017.01.004
Foissner, W., and Hawksworth, D. L. (2009). Protist Diversity and Geographical Distribution. Cham: Springer.
Frias Martins, A. (1991). Distribuição dos moluscos de água doce em São Miguel e na Terceira. Açoreana 7, 257–276.
Funari, E., and Testai, E. (2008). Human health risk assessment related to cyanotoxins exposure. Crit. Rev. Toxicol. 38, 97–125. doi: 10.1080/10408440701749454
Gallardo, B., Clavero, M., Sánchez, M. I., and Vilà, M. (2016). Global ecological impacts of invasive species in aquatic ecosystems. Glob. Chang. Biol. 22, 151–163. doi: 10.1111/gcb.13004
García-Berthou, E., Alcaraz, C., Pou-Rovira, Q., Zamora, L., Coenders, G., and Feo, C. (2005). Introduction pathways and establishment rates of invasive aquatic species in Europe. Can. J. Fish. Aquat. Sci. 62, 453–463. doi: 10.1139/f05-017
García-Berthou, E., Boix, D., and Clavero, M. (2007). “Biological invaders in Iberian inland waters: Profiles, distribution, and threats,” in Biological Invaders in inland Waters: Profiles, Distribution, and Threats, ed. F. Gherardi (Springer), doi: 10.1007/978-1-4020-6029-8
Gherardi, F. (2007). “Biological invasions in inland waters: an overview,” in Biological Invaders in Inland Waters: Profiles, Distribution, and Threats, ed. F. Gherardi (Dordrecht: Springer), 3–25.
Gherardi, F., and Acquistapace, P. (2007). Invasive crayfish in Europe: the impact of Procambarus clarkii on the littoral community of a Mediterranean lake. Freshw. Biol. 52, 1249–1259. doi: 10.1111/j.1365-2427.2007.01760.x
Gherardi, F., Barbaresi, S., and Salvi, G. (2000). Spatial and temporal patterns in the movement of Procambarus clarkii, an invasive crayfish. Aquat. Sci. 62, 179–193. doi: 10.1007/pl00001330
Gherardi, F., Bertolino, S., Bodon, M., Casellato, S., Cianfanelli, S., Ferraguti, M., et al. (2008a). Animal xenodiversity in Italian inland waters: distribution, modes of arrival, and pathways. Biol. Invasions 10, 435–454. doi: 10.1007/s10530-007-9142-9
Gherardi, F., Gollasch, S., Minchin, D., Olenin, S., and Panov, V. E. (eds) (2008b). “Alien Invertebrates and Fish in European Inland Waters,” in Handbook of Alien Species in Europe (Netherlands: Springer), 81–92.
Gherardi, F., Tricarico, E., and Ilhéu, M. (2002). Movement patterns of an invasive crayfish, Procambarus clarkii, in a temporary stream of southern Portugal. Ethol. Ecol. Evol. 14, 183–197. doi: 10.1080/08927014.2002.9522739
Gippoliti, S., and Amori, G. (2006). Ancient introductions of mammals in the Mediterranean Basin and their implications for conservation. Mamm. Rev. 36, 37–48. doi: 10.1111/j.1365-2907.2006.00081.x
Gonçalves, V. (2008). Contribuição do Estudo das Microalgas Para a Avaliação da Qualidade Ecológica das Lagoas dos Açores: Fitoplâncton e Diatomáceas Bentónicas. Nagoya: NOVA.
Gonçalves, V., Marques, H. S., and Raposeiro, P. M. (2015). Diatom assemblages and their associated environmental drivers in isolated oceanic island streams (Azores archipelago as case study). Hydrobiologia 751, 89–103. doi: 10.1007/s10750-015-2174-8
Gonçalves, V., Raposeiro, P., Couto, A. I., Costa, R. M., Rocha, F., Wattiez, X., et al. (2006). Contribuição para a caracterização das águas interiores de superficie da ilha do Pico. Relatórios e Comun. do Dep. Biol. da Univ. dos Açores 34, 93–103.
Gozlan, R. E. (2008). Introduction of non-native freshwater fish: is it all bad? Fish Fish. 9, 106–115. doi: 10.1111/j.1467-2979.2007.00267.x
Guerne, M. J. (1887). La faune des eaux douce des Açores et le transport des animaux a grande distance par l’intermediaire des oiseaux. Du Comptes Rendu la Société Biol. 8, 580–584.
Havel, J. E., Kovalenko, K. E., Thomaz, S. M., Amalfitano, S., and Kats, L. B. (2015). Aquatic invasive species: challenges for the future. Hydrobiologia 750, 147–170. doi: 10.1007/s10750-014-2166-0
Heiri, O., Wooller, M., van Hardenbroek, M., and Wang, Y. (2009). Stable isotopes in chitinous fossils of aquatic invertebrates. PAGES News 17, 100–102. doi: 10.22498/pages.17.3.100
Hellmann, J. J., Byers, J. E., Bierwagen, B. G., and Dukes, J. S. (2008). Five potential consequences of climate change for invasive species. Conserv. Biol. 22, 534–543. doi: 10.1111/j.1523-1739.2008.00951.x
Heywood, V. (2012). The role of New World biodiversity in the transformation of Mediterranean landscapes and culture. Bocconea 24, 69–93.
Hillebrand, H., and Sommer, U. (2000). Diversity of benthic microalgae in response to colonization time and eutrophication. Aquat. Bot. 67, 221–236. doi: 10.1016/S0304-3770(00)00088-7
Horppila, J., Peltonen, H., Malinen, T., Luokkanen, E., and Kairesalo, T. (1998). Top-down or bottom-up effects by fish: issues of concern in biomanipulation of Lakes. Restor. Ecol. 6, 20–28.
Howeth, J. G., Gantz, C. A., Angermeier, P. L., Frimpong, E. A., Hoff, M. H., Keller, R. P., et al. (2016). Predicting invasiveness of species in trade: climate match, trophic guild and fecundity influence establishment and impact of non-native freshwater fishes. Divers. Distrib. 22, 148–160. doi: 10.1111/ddi.12391
Hughes, S. J. (2006). Temporal and spatial distribution patterns of larval trichoptera in Madeiran streams. Hydrobiologia 553, 27–41. doi: 10.1007/s10750-005-0627-1
Hulme, P. E. (2009). Trade, transport and trouble: managing invasive species pathways in an era of globalization. J. Appl. Ecol. 46, 10–18. doi: 10.1111/j.1365-2664.2008.01600.x
Hunt, T. L., Scarborough, H., Giri, K., Douglas, J. W., and Jones, P. (2017). Assessing the cost-effectiveness of a fish stocking program in a culture-based recreational fishery. Fish. Res. 186, 468–477. doi: 10.1016/j.fishres.2016.09.003
Hurtrez-Boussès, S., Meunier, C., Durand, P., and Renaud, F. (2001). Dynamics of host-parasite interactions: the example of population biology of the liver fluke (Fasciola hepatica). Microbes Infect. 3, 841–849. doi: 10.1016/S1286-4579(01)01442-3
Hussner, A. (2012). Alien aquatic plant species in European countries. Weed Res. 52, 297–306. doi: 10.1111/j.1365-3180.2012.00926.x
Ishikawa, T., and Tachihara, K. (2014). Introduction history of non-native freshwater fish in Okinawa-jima Island: ornamental aquarium fish pose the greatest risk for future invasions. Ichthyol. Res. 61, 17–26. doi: 10.1007/s10228-013-0367-6
Jakovčev-Todorović, D., Dikanović, V., Skorić, S., and Cakić, P. (2010). Freshwater jellyfish Craspedacusta sowerbyi Lankester, 1880 (Hydrozoa, Olindiidae) - 50 years’ observations in Serbia. Arch. Biol. Sci. 62, 123–128. doi: 10.2298/ABS1001123J
Jankowski, T. (2001). The freshwater medusae of the world - A taxonomic and systematic literature study with some remarks on other inland water jellyfish. Hydrobiologia 462, 91–113. doi: 10.1023/A:1013126015171
Jankowski, T., Collins, A. G., and Campbell, R. (2008). Global diversity of inland water cnidarians. Hydrobiologia 595, 35–40. doi: 10.1007/s10750-007-9001-9
Kaplan, Z., and Zdenek, K. (2005). Potamogeton schweinfurthii A. Benn., a new species for Europe. Preslia 77, 419–431.
Kaštovský, J., Hauer, T., Mareš, J., Krautová, M., Bešta, T., Komárek, J., et al. (2010). A review of the alien and expansive species of freshwater cyanobacteria and algae in the Czech Republic. Biol. Invasions 12, 3599–3625. doi: 10.1007/s10530-010-9754-3
Keller, R. P., Geist, J., Jeschke, J. M., and Kühn, L. (2011). Invasive species in Europe: ecology, status, and policy. Environ. Sci. Eur. 23, 1–17. doi: 10.1186/2190-4715-23-23
Korneva, L. G. (2014). Invasions of alien species of planktonic microalgae into the fresh waters of Holarctic (Review). Russ. J. Biol. Invasions 5, 65–81. doi: 10.1134/S2075111714020052
Kottelat, M., and Freyhof, J. (2007). Handbook of European Freshwater Fishes. Delémont: Publications Kottelat.
Kristiansen, J. (1996). 16. Dispersal of freshwater algae — a review. Hydrobiologia 336, 151–157. doi: 10.1007/BF00010829
Kriticos, D. J., and Brunel, S. (2016). Assessing and managing the current and future pest risk from water hyacinth, (Eichhornia crassipes), an invasive aquatic plant threatening the environment and water security. PLoS One 11:e0120054. doi: 10.1371/journal.pone.0120054
Lamelas-López, L., Raposeiro, P. M., Borges, P. A. V. V., Florencio, M., Lamelas-Lopez, L., Raposeiro, P. M., et al. (2017). Annotated checklist of aquatic beetles (Coleoptera) and true bugs (Heteroptera) in the Azores Islands: new records and corrections of colonization status. Zootaxa 4353, 117–132. doi: 10.11646/zootaxa.4353.1.7
Landolt E. (ed.) (1986). “The family of Lemnaceae—A monographic study (vol. 2),” in Biosystematic Investigations in the Family of Duckweeds (Lemnaceae) (Cham: Springer), 309–310.
Law Decree no 165/74 (1974). Decreto-Lei 165/74. Diário do Governo n.o 94/1974, Série I de 22 de abril de 1974. New Delhi: Government Gazette.
Law Decree no 565/99 (1999). Decreto-Lei n.o 565/99 Diário da República n.o 295/1999, Série I-A de 21 de dezembro de 1999. Available online at: https://dre.pt/application/conteudo/661769 (accessed November 4, 2020).
Law Decree no 92/2019 (2019). Decreto-Lei n.o 92/2019. Diário da República, Série I N.o 130 10 de julho de 2019. Available online at: https://dre.pt/application/file/a/123025530 (accessed November 4, 2020).
Leung, B., Lodge, D. M., Finnoff, D., Shogren, J. F., Lewis, M. A., and Lamberti, G. (2002). An ounce of prevention or a pound of cure: bioeconomic risk analysis of invasive species. Proc. R. Soc. London. Ser. B Biol. Sci. 269, 2407–2413. doi: 10.1098/rspb.2002.2179
Lougheed, V. L., Crosbie, B., and Chow-Fraser, P. (1998). Predictions on the effect of common carp (Cyprinus carpio) exclusion on water quality, zooplankton, and submergent macrophytes in a Great Lakes wetland. Can. J. Fish. Aquat. Sci. 55, 1189–1197. doi: 10.1139/f97-315
Lounnas, M., Correa, A. C., Alda, P., David, P., Dubois, M. P., Calvopiña, M., et al. (2018). Population structure and genetic diversity in the invasive freshwater snail Galba schirazensis (Lymnaeidae). Can. J. Zool. 96, 425–435. doi: 10.1139/cjz-2016-0319
Lozon, J. D., and MacIsaac, H. J. (1997). Biological invasions: are they dependent on disturbance? Environ. Rev. 5, 131–144. doi: 10.1139/a97-007
Luz, R., Cordeiro, R., Vilaverde, J., Raposeiro, P. M., Fonseca, A., and Gonçalves, V. (2020). Cyanobacteria from freshwater lakes in the Azores archipelago, Portugal: data from long term phytoplankton monitoring. Biodivers. Data J. 8:51928. doi: 10.3897/BDJ.8.E51928
MacArthur, R. H., and Wilson, E. O. (2001). The Theory of Island Biogeography. Princeton, NJ: Princeton University Press.
Maceda-Veiga, A. (2013). Towards the conservation of freshwater fish: iberian Rivers as an example of threats and management practices. Rev. Fish Biol. Fish. 23, 1–22. doi: 10.1007/s11160-012-9275-5
MacNeil, C., Dick, J. T. A., Johnson, M. P., Hatcher, M. J., and Dunn, A. M. (2004). A species invasion mediated through habitat structure, intraguild predation, and parasitism. Limnol. Oceanogr. 49, 1848–1856. doi: 10.4319/lo.2004.49.5.1848
Mandak, B., and Pysek, P. (1998). “History of the spread and habitat preferences of Atriplex sagittata (Chenopodiaceae) in the Czech Republic,” in Plant invasions Ecol. Mech. Hum. Responses, eds U. Starfinger, K. Kowarik, and Edwards I. M. Williamson (Leiden: Backhuys Publishers), 209–224.
March-Salas, M., and Pertierra, L. R. (2020). Warmer and less variable temperatures favour an accelerated plant phenology of two invasive weeds across sub-Antarctic Macquarie Island. Austral Ecol. 45, 572–585. doi: 10.1111/aec.12872
Mas-Coma, S., Bargues, M. D., and Valero, M. A. (2005). Fascioliasis and other plant-borne trematode zoonoses. Int. J. Parasitol. 35, 1255–1278. doi: 10.1016/j.ijpara.2005.07.010
Matthews, J., Koopman, K. R., Beringen, R., Odé, B., Pot, R., van der Velde, G., et al. (2014). Knowledge document for risk analysis of the non-native Brazilian waterweed (Egeria densa) in the Netherlands. Rep. Environ. Sci. 468, 1–58.
Meineri, E., Rodriguez-Perez, H., Hilaire, S., and Mesleard, F. (2014). Distribution and reproduction of Procambarus clarkii in relation to water management, salinity and habitat type in the Camargue. Aquat. Conserv. Mar. Freshw. Ecosyst. 24, 312–323. doi: 10.1002/aqc.2410
Miller, S. A., and Crowl, T. A. (2006). Effects of common carp (Cyprinus carpio) on macrophytes and invertebrate communities in a shallow lake. Freshw. Biol. 51, 85–94. doi: 10.1111/j.1365-2427.2005.01477.x
Mooney, H. A., and Hobbs, R. J. (2000). “Global change and invasive species: where do we go from here?,” in Invasive Species in a Changing World, eds H. A. Mooney and R. J. Hobbs (Washington, DC: Island Press), 425–434.
Moreno-Leon, M. A., and Ortega-Rubio, A. (2009). First record of Craspedacusta sowerbyi Lankester, 1880 (Cnidaria: Limnomedusae: Olindiidae) in Mexico (Adolfo Lopez Mateos reservoir), with notes on their feeding habits and limnological dates. Biol. Invasions 11, 1827–1834. doi: 10.1007/s10530-008-9361-8
Moser, K. A. (2004). Paleolimnology and the frontiers of biogeography. Phys. Geogr. 25, 453–480. doi: 10.2747/0272-3646.25.6.453
Niemelä, P., and Mattson, W. J. (1996). Invasion of North American forests by European phytophagous insects: legacy of the European crucible? Bioscience 46, 741–753. doi: 10.2307/1312850
Nunes, A. L., Tricarico, E., Panov, V. E., Cardoso, A. C., and Katsanevakis, S. (2015). Pathways and gateways of freshwater invasions in Europe. Aquat. Invasions 10, 359–370. doi: 10.3391/ai.2015.10.4.01
Oliveira, N. M. B., Sampaio, E. V. S. B., Pereira, S. M. B., and Moura Junior, A. M. (2005). Capacidade de regeneração de Egeria densa nos reservatórios de Paulo Afonso, BA. Planta Daninha 23, 363–369.
Oscoz, J., Tomás, P., and Durán, C. (2010). Review and new records of non-indigenous freshwater invertebrates in the Ebro River basin (Northeast Spain). Aquat. Invasions 5, 263–284. doi: 10.3391/ai.2010.5.3.04
Parkos, J. J., Santucci, V. J., and Wahl, D. H. (2003). Effects of adult common carp (Cyprinus carpio) on multiple trophic levels in shallow mesocosms. Can. J. Fish. Aquat. Sci. 60, 182–192. doi: 10.1139/f03-011
Pejchar, L., and Mooney, H. A. (2009). Invasive species, ecosystem services and human well-being. Trends Ecol. Evol. 24, 497–504. doi: 10.1016/j.tree.2009.03.016
Pennington, T. (2009). “Egeria,” in Biology and Control of Aquatic Plants: A Best Management Practices Handbook, eds L. A. Gettys, W. T. Haller, and M. Bellaud (Marietta: Aquatic Ecosystem Restoration Foundation), 129–133.
Pereira, C. L., Raposeiro, P. M., Costa, A. C., Bao, R., Giralt, S., and Gonçalves, V. (2014). Biogeography and lake morphometry drive diatom and chironomid assemblages’ composition in lacustrine surface sediments of oceanic islands. Hydrobiologia 730, 93–112. doi: 10.1007/s10750-014-1824-6
Peyton, J., Martinou, A. F., Pescott, O. L., Demetriou, M., Adriaens, T., Arianoutsou, M., et al. (2019). Horizon scanning for invasive alien species with the potential to threaten biodiversity and human health on a Mediterranean island. Biol. Invasions 21, 2107–2125. doi: 10.1007/s10530-019-01961-7
Pimentel, D., Lach, L., Zuniga, R., and Morrison, D. (2000). Environmental and economic costs of nonindigenous species in the United States. Bioscience 50, 53–65.
Pimentel, D., Zuniga, R., and Morrison, D. (2005). Update on the environmental and economic costs associated with alien-invasive species in the United States. Ecol. Econ. 52, 273–288. doi: 10.1016/j.ecolecon.2004.10.002
Proctor, V. W. (1959). Dispersal of fresh-water algae by migratory water birds. Science 130, 623–624. doi: 10.1126/science.130.3376.623
Quilliam, R. S., van Niekerk, M. A., Chadwick, D. R., Cross, P., Hanley, N., Jones, D. L., et al. (2015). Can macrophyte harvesting from eutrophic water close the loop on nutrient loss from agricultural land? J. Environ. Manage. 152, 210–217. doi: 10.1016/j.jenvman.2015.01.046
Rahel, F. J., and Olden, J. D. (2008). Assessing the effects of climate change on aquatic invasive species. Conserv. Biol. 22, 521–533. doi: 10.1111/j.1523-1739.2008.00950.x
Ramalho, R. O., and Anastácio, P. M. (2014). Factors inducing overland movement of invasive crayfish (Procambarus clarkii) in a ricefield habitat. Hydrobiologia 746, 135–146. doi: 10.1007/s10750-014-2052-9
Raposeiro, P. M., Costa, A. C., and Hughes, S. J. (2011a). Environmental factors-spatial and temporal variation of chironomid communities in oceanic island streams (Azores archipelago). Ann. Limnol. 47, 325–338. doi: 10.1051/limn/2011048
Raposeiro, P. M., Costa, A. C., and Martins, A. F. (2011b). On the presence, distribution and habitat of the alien freshwater snail Ferrissia fragilis (Tryon, 1863) (Gastropoda: Planorbidae) in the oceanic islands of the Azores. Aquat. Invasions 6:3. doi: 10.3391/ai.2011.6.S1.003
Raposeiro, P. M., Ramos, J. C., and Costa, A. C. (2011c). First record of Craspedacusta sowerbii Lankester, 1880 (Cnidaria: Limnomedusae) in the Azores. Arquipélago Life Mar. Sci. 1880, 11–13.
Raposeiro, P. M., Cruz, A. M., Hughes, S. J., and Costa, A. C. (2012). Azorean freshwater invertebrates: status, threats and biogeographic notes. Limnetica 31, 13–22.
Raposeiro, P. M., Hughes, S. J., and Costa, A. C. (2013). Environmental drivers – spatial and temporal variation of macroinvertebrate communities in island streams: the case of the Azores Archipelago. Fundam. Appl. Limnol. Arch. Hydrobiol. 182, 337–350. doi: 10.1127/1863-9135/2013/0384
Raposeiro, P. M., Martins, G. M., Moniz, I., Cunha, A., Costa, A. C., and Gonçalves, V. (2014). Leaf litter decomposition in remote oceanic islands: the role of macroinvertebrates vs. microbial decomposition of native vs. exotic plant species. Limnol. Ecol. Manag. Inl. Waters 45, 80–87. doi: 10.1016/j.limno.2013.10.006
Raposeiro, P. M., Ramos, J. C., and Costa, A. C. (2009). First record of Branchiura sowerbyi Beddard, 1892 (Oligochaeta: Tubificidae) in Azores. Aquat. Invasions 4, 487–490. doi: 10.3391/ai.2009.4.3.8
Raposeiro, P. M., Rubio, M. J., González, A., Hernández, A., Sánchez-López, G., Vázquez-Loureiro, D., et al. (2017). Impact of the historical introduction of exotic fishes on the chironomid community of Lake Azul (Azores Islands). Palaeogeogr. Palaeoclimatol. Palaeoecol. 466, 77–88. doi: 10.1016/j.palaeo.2016.11.015
Rayner, N. A. (1988). First record of Craspedacusta sowerbyi Lankester (Cnidaria: Limnomedusae) from Africa. Hydrobiologia 162, 73–77. doi: 10.1007/BF00014334
Reaser, J. K., Meyerson, L. A., Cronk, Q., De Poorter, M., Eldrege, L. G., Green, E., et al. (2007). Ecological and socioeconomic impacts of invasive alien species in island ecosystems. Environ. Conserv. 34, 98–111. doi: 10.1017/S0376892907003815
Regional Legislative Decree n.o 15/2012/A (2012). Decreto Legislativo Regional n.o 15/2012/A. Diário da República n.o 66/2012, Série I de 02 de Abril de 2012. Available online at: https://dre.pt/application/file/a/553827 (accessed November 4, 2020).
Ribeiro, F., Collares-Pereira, M. J., and Moyle, P. B. (2009). Non-native fish in the fresh waters of Portugal, Azores and Madeira Islands: a growing threat to aquatic biodiversity. Fish. Manag. Ecol. 16, 255–264. doi: 10.1111/j.1365-2400.2009.00659.x
Ricciardi, A. (2001). Facilitative interactions among aquatic invaders: is an “invasional meltdown” occurring in the Great Lakes? Can. J. Fish. Aquat. Sci. 58, 2513–2525. doi: 10.1139/f01-178
Ricciardi, A. (2003). Predicting the impacts of an introduced species from its invasion history: an empirical approach applied to zebra mussel invasions. Freshw. Biol. 48, 972–981. doi: 10.1046/j.1365-2427.2003.01071.x
Ricciardi, A., and Atkinson, S. K. (2004). Distinctiveness magnifies the impact of biological invaders in aquatic ecosystems. Ecol. Lett. 7, 781–784. doi: 10.1111/j.1461-0248.2004.00642.x
Rivas-Martínez, S., Díaz, T., Fernández-González, F., Izco, J., Loidi, J., Lousã, M., et al. (2001). Vascular plant communities of Spain and Portugal: addenda to the syntaxonomical checklist of 2001. Part II. Itinera Geobot. 15, 433–922.
Rodrigues, A. C., Pacheco, D., Romanets, Y., Bruns, S., Nogueira, R., Coutinho, R., et al. (2004). “Modelação da Qualidade da Água da Lagoa das Furnas e da Lagoa Verde das Sete Cidades,” in Actas da 8.a Conferência Nacional de Ambiente (Lisboa: Universidade Nova (Lisboa)).
Rull, V., Lara, A., Rubio-Inglés, M. J., Giralt, S., Gonçalves, V., Raposeiro, P., et al. (2017). Vegetation and landscape dynamics under natural and anthropogenic forcing on the Azores Islands: a 700-year pollen record from the São Miguel Island. Quat. Sci. Rev. 159, 155–168. doi: 10.1016/j.quascirev.2017.01.021
Russell, J. C., Meyer, J. Y., Holmes, N. D., and Pagad, S. (2017). Invasive alien species on islands: impacts, distribution, interactions and management. Environ. Conserv. 44, 359–370. doi: 10.1017/S0376892917000297
Sala, O. E., Chapin, F. S., Armesto, J. J., Berlow, E., Bloomfield, J., Dirzo, R., et al. (2000). Global biodiversity scenarios for the year 2100. Science 287, 1770–1774. doi: 10.1126/science.287.5459.1770
Salgueiro, P., Serrano, C., Gomes, B., Alves, J., Sousa, C. A., Abecasis, A., et al. (2019). Phylogeography and invasion history of Aedes aegypti, the Dengue and Zika mosquito vector in Cape Verde islands (West Africa). Evol. Appl. 12, 1797–1811. doi: 10.1111/eva.12834
Santos, F. D., Valente, M. A., Miranda, P. M., Aguiar, A., Azevedo, E. B., Tome, A., et al. (2004). Climate change scenarios in the Azores and Madeira Islands. World Resour. Rev. 16, 473–491.
Santos, M., da, C. R., Pacheco, D. M. M., Santana, F., and Muelle, H. (2005). Cyanobacteria blooms in Sete-Cidades lake (São Miguel Island - Azores). Algol. Stud. für Hydrobiol. 117, 393–406. doi: 10.1127/1864-1318/2005/0117-0393
Santos, M. C. R. R., Muelle, H., and Pacheco, D. M. D. D. (2012). Cyanobacteria and microcystins in lake Furnas (S. Miguel island-Azores). Limnetica 31, 107–118.
Sax, D. F. (2001). Latitudinal gradients and geographic ranges of exotic species: implications for biogeography. J. Biogeogr. 28, 139–150. doi: 10.1046/j.1365-2699.2001.00536.x
Sayer, C. D., Davidson, T. A., and Jones, J. I. (2010). Seasonal dynamics of macrophytes and phytoplankton in shallow lakes: a eutrophication-driven pathway from plants to plankton? Freshw. Biol. 55, 500–513. doi: 10.1111/j.1365-2427.2009.02365.x
Schaefer, H., Carine, M. A., and Rumsey, F. J. (2011). From european priority species to invasive weed: Marsilea azorica (Marsileaceae) is a misidentified Alien. Syst. Bot. 36, 845–853. doi: 10.1600/036364411X604868
Seebens, H., Essl, F., Dawson, W., Fuentes, N., Moser, D., Pergl, J., et al. (2015). Global trade will accelerate plant invasions in emerging economies under climate change. Glob. Chang. Biol. 21, 4128–4140. doi: 10.1111/gcb.13021
Seixas, G., Salgueiro, P., Bronzato-Badial, A., Gonçalves, Y., Reyes-Lugo, M., Gordicho, V., et al. (2019). Origin and expansion of the mosquito Aedes aegypti in Madeira Island (Portugal). Sci. Rep. 9, 1–13. doi: 10.1038/s41598-018-38373-x
Sharma, N. K., Rai, A. K., Singh, S., and Brown, R. M. (2007). Airborne algae: their present status and relevance. J. Phycol. 43, 615–627. doi: 10.1111/j.1529-8817.2007.00373.x
Sharov, A. A. (2004). Bioeconomics of managing the spread of exotic pest species with barrier zones. Risk Anal. 24, 879–892. doi: 10.1111/j.0272-4332.2004.00486.x
Shea, K., and Chesson, P. (2002). Community ecology theory as a framework for biological invasions. Trends Ecol. Evol. 17, 170–176.
Silva, L., Ojeda Land, E., and Rodríguez Luengo, J. (2008). Invasive Terrestial Flora & Fauna of Macaronesia. Azores: Madeira y Canarias.
Silva, L., and Smith, C. W. (2004). A characterization of the non-indigenous flora of the Azores Archipelago. Biol. Invasions 6, 193–204. doi: 10.1023/B:BINV.0000022138.75673.8c
Simberloff, D. (1995). Why do introduced species appear to devastate islands more than mainland areas? Pacific Sci. 49, 87–97.
Simberloff, D. (2009). We can eliminate invasions or live with them. Successful management projects. Biol. Invasions 11, 149–157. doi: 10.1007/s10530-008-9317-z
Simberloff, D., Alexander, J., Allendorf, F., Aronson, J., Antunes, P. M., Bacher, S., et al. (2011). Non-natives: 141 scientists object. Nature 475:36. doi: 10.1038/475036a
Sivarajah, B., Rühland, K. M., Labaj, A. L., Paterson, A. M., and Smol, J. P. (2016). Why is the relative abundance of Asterionella formosa increasing in a Boreal Shield lake as nutrient levels decline? J. Paleolimnol. 55, 357–367. doi: 10.1007/s10933-016-9886-2
Skov, T., Buchaca, T., Amsinck, S. L., Landkildehus, F., Odgaard, B. V., Azevedo, J., et al. (2010). Using invertebrate remains and pigments in the sediment to infer changes in trophic structure after fish introduction in Lake Fogo: a crater lake in the Azores. Hydrobiologia 654, 13–25. doi: 10.1007/s10750-010-0325-5
Smith, A. S., and Alexander, J. E. (2008). Potential effects of the freshwater jellyfish Craspedacusta sowerbii on zooplankton community abundance. J. Plankton Res. 30, 1323–1327. doi: 10.1093/plankt/fbn093
Smol, J. P. (2014). Paleolimnology: an introduction to approaches used to track long-term environmental changes using lake sediments. Limnol. Oceanogr. E Lectures 1, 1–73. doi: 10.4319/lol.2009.jsmol.3
Sondergaard, M., Jensen, P. J., and Jeppesen, E. (2001). Retention and internal loading of phosphorus in shallow, eutrophic lakes. Sci. World J. 1, 427–442. doi: 10.1100/tsw.2001.72
Strayer, D. L. (2010). Alien species in fresh waters: ecological effects, interactions with other stressors, and prospects for the future. Freshw. Biol. 55, 152–174. doi: 10.1111/j.1365-2427.2009.02380.x
Takamura, K. (2007). Performance as a fish predator of largemouth bass [Micropterus salmoides (Lacepède)] invading Japanese freshwaters: a review. Ecol. Res. 22, 940–946. doi: 10.1007/s11284-007-0415-7
Theobald, E. J., Ettinger, A. K., Burgess, H. K., DeBey, L. B., Schmidt, N. R., Froehlich, H. E., et al. (2015). Global change and local solutions: tapping the unrealized potential of citizen science for biodiversity research. Biol. Conserv. 181, 236–244. doi: 10.1016/j.biocon.2014.10.021
Thorp, J. H., Rogers, D. C., and Dimmick, W. W. (2014). Thorp and Covich’s Freshwater Invertebrates: Ecology and General Biology: Fourth Edition. Amsterdam: Elsevier.
Triantis, K. A., Borges, P. A. V., Ladle, R. J., Hortal, J., Cardoso, P., Gaspar, C., et al. (2010). Extinction debt on oceanic Islands. Ecography 33, 285–294. doi: 10.1111/j.1600-0587.2010.06203.x
Tricarico, E., Junqueira, A. O. R. R., and Dudgeon, D. (2016). Alien species in aquatic environments: a selective comparison of coastal and inland waters in tropical and temperate latitudes. Aquat. Conserv. Mar. Freshw. Ecosyst. 26, 872–891. doi: 10.1002/aqc.2711
Tsiamis, K., Palialexis, A., Stefanova, K., Gladan, ŽN., Skejiæ, S., Despalatoviæ, M., et al. (2019). Non-indigenous species refined national baseline inventories: a synthesis in the context of the European Union’s marine strategy framework directive. Mar. Pollut. Bull. 145, 429–435. doi: 10.1016/j.marpolbul.2019.06.012
van Leeuwen, J. F. N. N., Schäfer, H., van der Knaap, W. O. O., Rittenour, T., Björck, S., Ammann, B., et al. (2005). Native or introduced? Fossil pollen and spores may say. An example from the Azores Islands. Neobiota 6, 27–34.
Vander Zanden, M. J., and Olden, J. D. (2008). A management framework for preventing the secondary spread of aquatic invasive species. Can. J. Fish. Aquat. Sci. 65, 1512–1522. doi: 10.1139/F08-099
Vázquez-Loureiro, D., Gonçalves, V., Sáez, A., Hernández, A., Raposeiro, P. M., Giralt, S., et al. (2019). Diatom-inferred ecological responses of an oceanic lake system to volcanism and anthropogenic perturbations since 1290 CE. Palaeogeogr. Palaeoclimatol. Palaeoecol. 534:109285. doi: 10.1016/j.palaeo.2019.109285
Vereecken, H., Baetens, J., Viaene, P., Mostaert, F., and Meire, P. (2006). Ecological management of aquatic plants: effects in lowland streams. Hydrobiologia 570, 205–210. doi: 10.1007/s10750-006-0181-5
Vieira, J., Câmara, G., Silva, F., and Santos, C. (2019). Airline choice and tourism growth in the Azores. J. Air Transp. Manag. 77, 1–6. doi: 10.1016/j.jairtraman.2019.02.009
Vitousek, P. M., D’Antonio, C. M., Loope, L. L., and Westbrooks, R. (1996). Our mobile society is redistributing the species on the earth at a pace that challenges ecosystems I threatens human health and strains economies. Am. Sci. 84, 468–478.
Walther, G. R., Roques, A., Hulme, P. E., Sykes, M. T., Pyšek, P., Kühn, I., et al. (2009). Alien species in a warmer world: risks and opportunities. Trends Ecol. Evol. 24, 686–693. doi: 10.1016/j.tree.2009.06.008
Williamson, C. E., Stoeckel, M. E., and Schoeneck, L. J. (1989). Predation risk and the structure of freshwater zooplankton communities. Oecologia 79, 76–82.
Williamson, M., and Fitter, A. (1996). The varying success of invaders. Ecology 77, 1661–1666. doi: 10.2307/2265769
Keywords: non-indigenous species, invasive species, oceanic islands, freshwater ecosystems, Azores archipelago
Citation: Costa AC, Balibrea A, Raposeiro PM, Santos S, Souto M and Gonçalves V (2021) Non-indigenous and Invasive Freshwater Species on the Atlantic Islands of the Azores Archipelago. Front. Ecol. Evol. 9:631214. doi: 10.3389/fevo.2021.631214
Received: 19 November 2020; Accepted: 19 March 2021;
Published: 13 April 2021.
Edited by:
Ross Taylor Shackleton, University of Lausanne, SwitzerlandReviewed by:
Maria Leonor Fidalgo, University of Porto, PortugalEmily Rebecca Coridon Smith, Angling Trust, United Kingdom
M. Veronica Toral-Granda, Charles Darwin University, Australia
Copyright © 2021 Costa, Balibrea, Raposeiro, Santos, Souto and Gonçalves. This is an open-access article distributed under the terms of the Creative Commons Attribution License (CC BY). The use, distribution or reproduction in other forums is permitted, provided the original author(s) and the copyright owner(s) are credited and that the original publication in this journal is cited, in accordance with accepted academic practice. No use, distribution or reproduction is permitted which does not comply with these terms.
*Correspondence: Ana C. Costa, ana.cm.costa@uac.pt