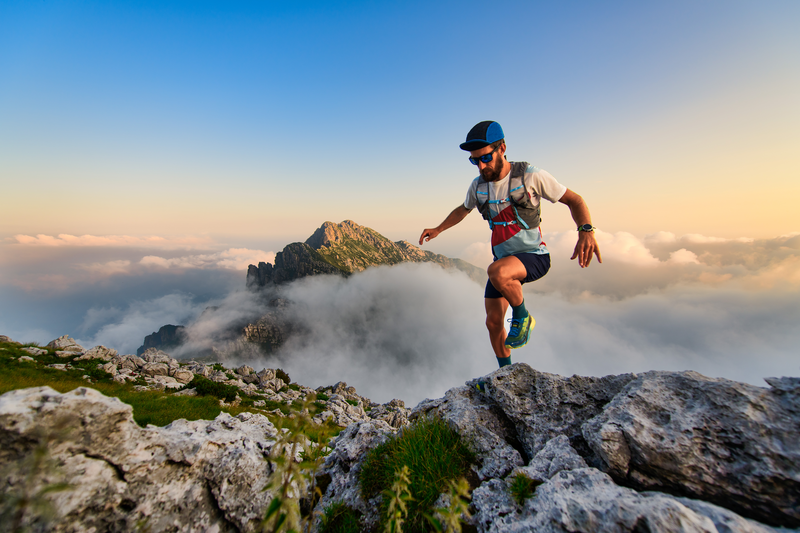
94% of researchers rate our articles as excellent or good
Learn more about the work of our research integrity team to safeguard the quality of each article we publish.
Find out more
BRIEF RESEARCH REPORT article
Front. Ecol. Evol. , 27 January 2022
Sec. Models in Ecology and Evolution
Volume 9 - 2021 | https://doi.org/10.3389/fevo.2021.630151
This article is part of the Research Topic Can the Trees Save the Crops? Predicting the Services Provided by Traditional and Novel Agroforests in Changing Mediterranean Landscapes View all 6 articles
Considering the present ecological crisis, land use-biodiversity relationships have become a major topic in landscape planning, ecosystem management and ecological restoration. In this scope, consistent patterns of outstanding biodiversity have been identified in agroforestry systems within diverse biogeographic regions and types of management. Empirical work has revealed that agroforestry higher structural complexity, when compared with current simplified agricultural systems, might be partially responsible for the observed patterns. The recently developed Habitat Amount Hypothesis predicts diversity for a local habitat patch, from the amount of the same habitat within the local landscape. We have expanded the previous hypothesis to the landscape level, computing the influence of the dominant land uses on the diversity of coexisting guilds. As a case study, we have considered archetypal landscapes dominated (or co-dominated) by crops or trees, which were compared using normalized diversities. The results obtained show that agroforestry systems substantially increase functional diversity and overall biodiversity within landscapes. We highlight that the normalized values should be parametrized to real conditions where the type of crop, tree and agroecological management will make a difference. Most importantly, our findings provide additional evidence that agroforestry has a critical role in enhancing biodiversity in agricultural landscapes and, in this way, should be regarded as a priority measure in European Agri-environmental funding schemes.
Farming systems should be recognized not only from the crops’ production perspective, but also from the regulating and cultural services standpoint (Plieninger et al., 2019). In fact, several works highlight their contribution to biodiversity conservation, soil enrichment, landscape beauty and carbon sequestration (e.g., Santos M. et al., 2019; Liu et al., 2020). However, the adoption of intensive management techniques, new crops, large-scale machinery and the massive use of fertilizers and pesticides triggered obvious changes in farming systems and agricultural landscapes, with significant drawbacks (Kanter et al., 2018; Bakış et al., 2021). In Europe, the Common Agricultural Policy (CAP) encouraged the specialization of agriculture and forestry systems in the most productive areas and abandonment of marginal (less productive) regions (De Roest et al., 2018; Santos et al., 2018; Abson, 2019). This was especially noticed in southern Europe, where CAP supporting schemes (including Agri-environmental measures) promoted relevant socio-ecological change but were unable to halt biodiversity loss and stop ecosystem services’ degradation (Harlio et al., 2019; Pardo et al., 2020).
Agroforestry, a jeopardized land use system in which trees are grown in combination with crops and/or livestock systems, is considered as one of the fundamental strategies to tackle the increasing need for high quality productions while maximizing ecosystem services and reducing environmental impacts (Torralba et al., 2016; Arosa et al., 2017; Den Herder et al., 2017; de Jalón et al., 2018; Moreno et al., 2018). In fact, wood pastures and grazed orchards, which are still common landscape features in some parts of Mediterranean Europe (De Roest et al., 2018), were highlighted as win-win multi-functional systems, responding to consumers’ worries while embracing the united nations sustainable development goals (van Noordwijk et al., 2018). Furthermore, multifunctional benefits of introducing trees in arable lands, novel silvopastoralism techniques and the maintenance and/or improvement of already existing agroforestry practices were recently discussed and added to the European Union Green Deal1.
Agroforestry has been identified as important for reducing species loss in agricultural landscapes, but also for endangered species conservation (e.g., Mosquera-Losada et al., 2009; Torralba et al., 2016; Udawatta et al., 2019). Biodiversity conservation is one of Europe 2030 objectives for a ‘‘resource efficient’’ Europe, contributing to a range of services that benefit human wellbeing, including food and fiber production and regulating and cultural services (EU Biodiversity Strategy for 2030)2. Nevertheless, species loss has been particularly dramatic in the Mediterranean (namely in agroecosystems), considered a biodiversity hotspot (Palacín and Alonso, 2018; Rosas-Ramos et al., 2019). In this way, understanding how agroforestry influences patterns of biodiversity is of utmost importance, given its significance for future landscape planning and for funding the most effective Agri-environment measures (Ansell et al., 2016; Pavlis et al., 2016). Agroforestry has the potential to contribute to biodiversity conservation in agricultural landscapes (and forest landscapes) by increasing structural complexity, and enhancing habitat and landscape heterogeneity (Torralba et al., 2016; Boinot et al., 2019; Haggar et al., 2019). Nevertheless, limited understanding of the interrelated effects of agroforestry micro-habitats on diversity patterns reduces our ability to project how a possible expansion of agroforestry will impact biodiversity and ecosystem services (Boinot et al., 2019; Santos P.Z.F. et al., 2019; Richard et al., 2020).
Biodiversity patterns and strategies for biodiversity conservation in agricultural landscapes have been increasingly enlightened by the recently developed Habitat Amount Hypothesis (HAH) (e.g., Fahrig, 2013, 2021; Melo et al., 2017; Watling et al., 2020). The HAH suggests that species richness, occurrence and abundance (from here referred to as “diversity”) in a given habitat site depend exclusively on the amount of that habitat in the “local landscape”, defined for an area surrounding the site (effect of scale) (Watling et al., 2020). Even though some authors challenged the HAH, considering “Spatial Configuration” or “Island Effect” theories more important to describe and manage biodiversity in agricultural landscapes (Hanski, 2015; Evju and Sverdrup-Thygeson, 2016; Haddad et al., 2017), new research shows that HAH is actually complementary and might integrate the previous concepts (Bueno and Peres, 2019; Watling et al., 2020; Fahrig, 2021; Saura, 2021). Indeed, Saura (2021) suggested that most discussion might have aroused from a misinterpretation of the HAH, i.e., habitat/landscape configuration is actually fundamental for HAH biodiversity patterns. Also, and as noticed in the original paper, HAH is not the only determinant of diversity: other factors should be considered, such as the type of species vs the type of habitat, the appropriate scale to analyze and the quality of the habitat matrix (Fahrig, 2013).
The practical implementation of the HAH was explicitly depicted by Saura (2021), assuming a formula that relates diversity in the habitat site with the amount of the same habitat within the local landscape. Saura (2021) does not investigates the different types of “diversities” that are associated with the different types of habitats encompassed by a landscape. This is especially pertinent when estimating diversity in complex landscapes or within the intermingled micro-habitats of agroforestry (Riginos et al., 2009; Arosa et al., 2017; Simonson et al., 2018). To decode this gap, a gradient of landscapes should be tested (e.g., dominated by agroforestry, agriculture, forest), estimating the potential diversity provided by each land-use type. Also, as the type of species (e.g., guilds such as habitat specialists vs edge species) is of utmost importance when applying the HAH, this was also simulated in our investigation, since the interaction between type of species and their habitat might change in accordance with the environmental conditions (Watling et al., 2020).
A promising way to gain insight of how HAH predicts diversity patterns in agricultural landscapes is to create virtual landscapes using spatial models (Mas et al., 2014), which enable the integration of habitat specific information and estimation of emergence outcomes (e.g., Prevedello et al., 2016; Santos et al., 2016). Also, simple spatial explicit models can improve our understanding of diversity within ecological systems and support the identification of landscape thresholds and successful conservation practices (Sequeira et al., 2018; Bakış et al., 2021). In the present work, a simple and flexible two-dimensional spatial model was developed, focusing on the interaction between diversity and landscapes, easily adaptable to other regions and case studies. In fact, identifying how different agricultural landscapes are related with biodiversity (and concomitant ecosystem services) is fundamental to assess whether agroforestry provides a biodiverse (and productive) alternative to contemporary Mediterranean agricultural landscapes (Kay et al., 2019a).
The following hypotheses were tested: (1) HAH should predict diversity at each site using the landscape matrix; (2) HAH discriminates the different guilds associated with each site; (3) HAH landscape diversity emerges from the combined effects of the size and isolation of all patches in the landscape; (4) HAH anticipates the creation of highly diverse agricultural landscapes when agroforestry is the dominant land use.
The virtual landscape mimics characteristic agricultural landscapes of Mediterranean Europe (Debussche et al., 1999; Van Doorn and Bakker, 2007; Geri et al., 2010): agricultural landscapes dominated by crops (from here referred to as “Agriculture”); agricultural landscapes dominated by trees (from here referred to as “Forest”); agricultural landscapes integrating in separate areas crops and trees (from here referred to as “Clumped”); agricultural landscapes alternating rows of crops with rows of trees (modern agroforestry, from here referred to as “Linear”); agricultural landscapes integrating random patches of crops and trees (traditional agroforestry, from here referred to as “Random”) (Figure 1). The conceptual description of the model follows a simplified version of the specifications of the standard protocol ODD (Overview, Design Concepts and Details) (Grimm et al., 2010). The software Netlogo 6.1.1 (Wilensky, 1999) was chosen to create virtual landscapes and to calculate the biodiversity outcomes.
Figure 1. Interface of the model used to simulate Agricultural landscapes (Al): (A) agriculture (crop dominated landscape); (B) Forest (tree dominated landscape); (C) clumped (separated areas of crop and tree dominated landscapes); (D) linear (lines of crop alternating with lines of trees); (E) Random (random distribution of crop patches and tree patches). Linear and Random Al represent modern (mostly mechanized) and traditional Agroforests, respectively.
The model investigates how agricultural landscapes biodiversity emerges from the interplay between patch-level land uses, discriminated within tree or crop dominated patches. By implementing the HAH (Watling et al., 2020) to the simulated landscapes, we intend to understand agroforestry biodiversity patterns, compare with other agricultural landscapes related to agricultural intensification and/or abandonment/afforestation (Santos et al., 2016).
The model includes 10,000 patches (unit cells) that make up the landscape extent of the study area (Figure 1 and Table 1 for details). Each patch was characterized by its dominant land use (tree or crop) and the normalized biodiversity was gauged accordingly with the formulas of Saura (2021), applied to all patches of the landscape.
Simple agricultural landscapes presented only crop patches (Agriculture) or tree patches (Forest) while in the case of Linear, Random and Clumped agricultural landscapes, an equivalent number of patches is associated with crops and trees (Figure 1).
Diversity predictions (Di) per patch were partitioned within crop species biodiversity (C_B), tree species biodiversity (T_B), edge species biodiversity (E_B) and overall species biodiversity (B, sums the contributions of the previous guilds) (e.g., Morrison et al., 2017; Wilson et al., 2017; Jackson et al., 2019). C_B and T_B were assessed for crop and tree patches, respectively. E_B was gauged only when the number of dissimilar bordering patches was equal or greater than 1. The power function was used to relate Diversity (Di) in a specific patch, considering the habitat amount surrounding it within a radius of i (Ai) (total area of habitat matching the central patch), as defined by Saura (2021) (Eq. 1). The power function associated with the HAH was calibrated to follow an average slope (z) of 0.45, supported on previous studies (Haddad et al., 2017; Rabelo et al., 2017; Bueno and Peres, 2019; Saura, 2021). In addition, k is a constant which depends on the unit used for area measurement, and equals the diversity that would exist if the habitat area was confined to one square unit (Whittaker et al., 2017) (details in Supplementary Appendix 5).
All metrics of diversity (C_B, T_B, E_B, B) were normalized (Dnorm,i, Eq. 2) using Dmax, the maximum value of Di possible. Dnorm,i (N_C_B, N_T_B, N_E_B, N_B) values, ranging from 0 to 1, are independent from k and, in this way, from the type of diversity, thus allowing direct comparisons between the different metrics and the different landscapes (Geneletti et al., 2018; Saura, 2021) (details in Supplementary Appendix 5).
Cohen’s effect size was used to reveal the magnitude of the differences in diversity between the agricultural landscapes simulated (Sullivan and Feinn, 2012; White et al., 2014). The rules of thumb for standardized mean differences effect sizes are defined as: d = 0, none; d ≈ 0.01, very small; d ≈ 0.2, small; d ≈ 0.5, medium; d ≈ 0.8, large; d ≈ 1.2, very large; d ≈ (>) 2, huge (Sawilowsky, 2009). To complement the previous results, the Kruskal-Wallis test and the Steel-Dwass multi-comparisons test were applied to determine the significance of pairwise differences (Sokal and Rohlf, 1995; Zar, 1996). Statistics were done using R 4.0.3 (R Core Team, 2020), through the effectsize (v 0.4.0; Ben-Shachar et al., 2020) and the ezr packages (Kanda, 2013).
Habitat Amount Hypothesis biodiversity predictions were uneven, contingent on the guild composition and agricultural landscape (Figure 2). In fact, while Normalized crop biodiversity (N_C_B) and Normalized tree biodiversity (N_T_B) attained higher medians in Agriculture and Forest dominated landscapes, respectively, Normalized Edge Biodiversity (N_E_B) and Normalized Biodiversity (N_B) reached higher medians within Agroforestry landscapes (Linear and Random) (Figure 2). On the other hand, the lower medians for N_C_B, N_T_B and N_E_B were simulated for Forest, Agriculture and both Agricultural landscapes, respectively (Figure 2). Additionally, Clumped landscapes presented the higher dispersion (interquartile range) for all guilds (N_C_B, N_T_B, N_E_B, N_B) (Figure 2). Moreover, all Biodiversity was condensed within a single partition in Agriculture and Forest landscapes, N_C_B and N_F_B, respectively, and spread within all guilds (N_C_B, N_T_B, N_E_B) for Clumped, Linear and Random landscapes (Figures 2A–C).
Figure 2. Box and Whisker plots expressing the differences in the Biodiversity metrics associated with the different Agricultural landscapes simulated: (A) normalized Crop Biodiversity (N_C_B); (B) normalized Tree Biodiversity (N_T_B); (C) normalized Edge Biodiversity (N_E_B); (D) normalized Biodiversity (N_B). Medians for N_C_B: Agriculture, 1.000; Clumped, 0.728; Forest, 0.000; Linear, 0.360; Random, 0.702. Medians for N_T_B: Agriculture, 0.000; Clumped, 0.000; Forest, 1.000; Linear, 0.360; Random, 0.000. Medians for N_E_B: Agriculture, 0.000; Clumped, 0.000; Forest, 0.000; Linear, 0.735; Random, 0.735. Medians for N_B: Agriculture, 0.645; Clumped, 0.609; Forest, 0.645; Linear, 0.938; Random, 0.945. Associated Steel-Dwass multiple comparisons tests in Supplementary Appendixes 1–4.
Pairwise differences using effect sizes (d) corroborate the previous results, depicting that even though Clumped landscapes, incorporating both uses (crop and tree dominated patches) in separate areas, are expected to be associated with more biodiversity (N_B) than single Agriculture or Forest (Very small differences, d < 0.2), only Agroforestry (Linear and Random landscapes) differences (from Agriculture and Forest) were considered Huge (d > 2.0) (Table 2). Additionally, both types of Agroforestry depict none to small differences in the biodiversity metrics (N_C_B, N_T_B, N_E_B), although with a small advantage in overall biodiversity for linear Agroforestry (N_B, d = 0.249) (Table 2).
Table 2. Pairwise comparisons (Cohen’s d) for the Normalized diversity predictions between all Agriculture landscapes (Agricultural landscapes details in Table 1): interpretation of Cohen’s d values and average d values in parenthesis. Positive values indicate higher diversity for the first landscape in comparison, negative values indicate higher diversity for the second landscape in comparison, and 0 indicates equivalent diversity values. N_C_B, normalized crop biodiversity; N_T_B, normalized tree biodiversity; N_E_B, normalized edge biodiversity; N_B, normalized biodiversity. Complementary Kruskal-Wallis and Stell-Dwass multiple comparisons test in Supplementary Appendixes 1–4.
Complementary comparisons using non-parametric statistics, depicted in the Supplementary Material (Supplementary Appendixes 1–4), corroborate the previous results: N_C_B higher t-values were attained when comparing Agriculture with all other agricultural landscapes (Supplementary Appendix 1); N_T_B higher t-values were attained when comparing Forest with all other agricultural landscapes (Supplementary Appendix 2); N_E_B higher t-values were attained when comparing Agroforestry landscapes (Linear and Random) with all other agricultural landscapes (Supplementary Appendix 3); N_B higher t-values were attained when comparing Agroforestry landscapes (Linear and Random) with all other agricultural landscapes, particularly Linear vs Agriculture, Forestry and Clumped (Supplementary Appendix 4).
As expected (e.g., Fahrig, 2013; Torralba et al., 2016), higher Normalized crop biodiversity (N_C_B) and Normalized tree biodiversity (N_T_B) values were attained within monospecific landscapes, Agriculture and Forest correspondingly, but also both landscapes low overall biodiversity’ predictions (Normalized biodiversity, N_B) (Figure 2 and Table 2). The corresponding higher dispersion for all guilds in the case of Clumped landscapes [N_C_B, N_T_B, N_E_B (Normalized edge biodiversity)] was also anticipated (Figure 2): depending of the fractal structure of the landscape associated with the type of species and grain size, detached patches with very low biodiversity could occur close to biodiverse ones (e.g., Benton et al., 2003; Toderi et al., 2017; Bakış et al., 2021). Nevertheless, even if Agroforestry landscapes (Linear and Random) were associated with comparable N_C_B and N_T_B to Clumped landscapes, their maximization of ecotones had an overwhelming impact on the N_E_B and in the N_B (Figure 2C and Table 2) as suspected from previous works (Jose, 2012; Moreno et al., 2018; Marconi and Armengot, 2020). This last comparison was surprising as, from the HAH theory predictions, equivalent amounts of habitat (Agroforestry – Linear and Random – had equivalent areas per habitat to Clumped) should have resulted in equivalent diversity (Fahrig, 2013, 2021; Saura, 2021).
The results obtained when applying the HAH to our virtual agricultural landscapes contribute to pinpoint the general mechanisms underlying biodiversity enhancement by agroforestry systems (Udawatta et al., 2019). In particular, agroforestry “boosting” effect was captured by the HAH implementation, since HAH implicitly reflects isolation/linkage of habitat patches and complexity within landscapes which is inherently associated with the biodiversity predictions (Bueno and Peres, 2019; Balzan et al., 2020; Saura, 2021). Also, the outcomes we got agree with Torralba et al. (2016) meta-analysis of European agroforestry: the authors found that land-use systems that are structurally and functionally more complex than crop-dominated or tree-dominated monospecific systems result in higher levels of biodiversity and ecosystem goods and services, without compromising productivity (see also Jose, 2012; Haggar et al., 2019).
Our results support the hypothesis that agroforestry impacts biodiversity (Torralba et al., 2016). However, our simple model does not consider mechanisms operating at larger scales, neither management issues or crop/tree species’ combinations (Hanane et al., 2019; Kay et al., 2019b; Varah et al., 2020). Habitat heterogeneity at different scales – from individual farms to whole landscapes – is commonly recognized as the core factor to reverse declines in farmland biodiversity (Benton et al., 2003). Albeit current Agri–environment measures to sustain biodiversity being implemented at the farm level, their ultimate objective is to impact landscapes (Toderi et al., 2017). Yet, the impact of the resulting landscape-scale distribution of habitat patches and their effectiveness remains largely unknown (Ansell et al., 2016; Marja et al., 2019). Ultimately, agroforestry benefits to the society depend on upscaling the different practices to generate add-value at landscape scale, particularly if applied in the right locations and with the correct management options (Plieninger et al., 2020).
Management plays an essential role shaping agroforestry biodiversity: positive effects of unproductive and reduced input areas and negative effects of intensive management were noticed for diverse taxonomic groups and systems (Lüscher et al., 2016). As an example, the shift to organic farming combined with agroforestry can increase biodiversity further, not only agrodiversity (e.g., traditional varieties) but also wild species associated with the complex microenvironments created (e.g., Marconi and Armengot, 2020; Rosati et al., 2020). Also, environmentally friendly agroforestry management might improve valuable ecosystem services such as habitat for pollinators and natural enemies of pests (Rosati et al., 2020). However, despite the diverse Agri-environmental measures of the Common Agricultural Policy (CAP), European agriculture is ruled by productivity, aggravating biodiversity loss (Concepción et al., 2020). Actually, agroforestry benefits for biodiversity – and agroforestry long term sustainability – might be jeopardized when these systems are oversimplified (e.g., Steffan-Dewenter et al., 2007; Arosa et al., 2017; Avilés, 2019).
The crop(s) and tree(s) chosen have an enormous effect on biodiversity enhancement in agroforestry, namely when native and/or traditional crops are combined with native tree species (Campos et al., 2007; Torralba et al., 2016). Native trees, apart from the structural complexity gain associated with agroforestry, support higher diversity of resources for wildlife, are usually adapted to local conditions and might even contribute to reduce fire risk in Mediterranean ecosystems (Damianidis et al., 2020). Conversely, alien species, especially potentially invasive trees, might threaten biodiversity and should be totally avoided (McNeely, 2004). Nevertheless, most productive non-invasive alien tree species could be considered, if applying adaptive management and monitoring, namely in harsh environmental conditions (e.g., eroded soils) (Guillerme et al., 2020).
Additionally, even if the HAH predictions seem to integrate the metapopulation dynamics theory (Semper-Pascual et al., 2021), by holistically considering that extinction probability decreases as the size of patches of habitat increase (assuming that expected population size is positively correlated with overall habitat area), the distributions of organisms do not always reflect the distribution of suitable habitats (Merckx et al., 2019). Population dynamics were not explicitly considered in our simple model, nor changes in quality of the micro-habitats present (Gardiner et al., 2018). Nevertheless, several authors consider that more attention should be given to spatially explicit methods for hierarchically defining patches based on the dynamics of the focal species (e.g., Cavanaugh et al., 2014). Moreover, patch occupancy, colonization and extinction result from combination of processes at both local and regional scales, especially difficult to be understood and simulated for multispecies responses to landscape dynamics (Dallas et al., 2020).
Biodiversity predictions from HAH should be analyzed not only as a whole but considering the different partitions (e.g., guilds), assuming that mechanisms of biodiversity emerge from patch-scale changes, such as edge effects, changes in behavior and local interactions (Fletcher et al., 2018). The underlying mechanistic pathway occurs via expanding connectivity and dispersal, while suitability for each species might vary spatially within patches and in relation to configuration variables (e.g., distance from edge) (Fletcher et al., 2018). The approach used in this work was also able to highlight that even though agroforestry should increase overall landscape biodiversity, part of this diversity – associated with crops and or trees – is only attained when the previous land uses dominate the landscape (Sokos et al., 2013; Concepción and Díaz, 2019). That is to say that agroforestry may not be a panacea for halting biodiversity loss in agroecosystems but, within an increasingly anthropogenic ecosystems and novel landscapes’ world, one of the win-win strategies (Montagnini, 2017). In fact, the relevance for overall biodiversity of tree patches (and isolated trees) in agroecosystems was highlighted by Concepción et al. (2020) but also the detrimental effects on agroecosystem specialists (e.g., threaten pseudo-steppe species).
The HAH challenges the assumption that small patches have little biodiversity value, by predicting that the size of the patch in which a plot is located has little additional effect on diversity beyond its contribution to the habitat amount in the local landscape (Saura, 2021). Also, evidence shows that the effect of habitat amount around a sample plot is stronger than either the individual or combined effects of patch size and isolation (Watling et al., 2020). In our case study, landscape biodiversity emerges from the several patches specific contributions to habitat amount. When the local landscape is composed by a single use, overall landscape biodiversity decreases, even though with increasing values for specific guilds (e.g., N_C_B or N_T_B). We have assumed that the larger the area occupied by a single land use, more important is its contribution to increase habitat amount, but not more than a collection of multiple patches summing an equivalent area. Nevertheless, higher biodiversity is expected to occur in more complex landscapes (e.g., comparing Clumped vs Random or Linear landscapes), suggesting agroforestry as a welcomed strategy in conservation.
Higher crop yields and food production needed for an increasingly population, originated an intense debate whether the most assertive strategy to biodiversity conservation is land-sharing (low-yield, environmentally friendly agriculture occupying larger areas, represented here by agroforestry) or land-sparing (high-yield, conventionally intensified agriculture occupying smaller areas, represented by Clumped) (e.g., Grass et al., 2019). Our conceptual model seems to support the idea that intensification may foster biodiversity declines at the landscape scale, as well as at the farm site through the direct effect of homogenization (e.g., Phalan et al., 2011). Thus, land-sharing might be the winning strategy for biodiversity enhancement, without disregarding the importance of land-sparing for specialists and endangered species conservation (Marull et al., 2018). In fact, multi-scale perspectives are fundamental and should consider land use history, local values, soil characteristics, climate but also socio-economic factors influence on land use at the regional scale and external drivers such as agricultural policies, trade treaties or climate agreements (Karner et al., 2019).
Mediterranean agriculture urges alternatives and agroforestry could be a key element amongst the tools to fight contemporary environmental challenges, such as climate change, water scarcity and food security (Malek et al., 2018; Yves et al., 2020). Concerning biodiversity, trees in agricultural landscapes appear particularly efficient in contributing to biodiversity conservation, while environmentally valuable and economically profitable (Barrios et al., 2018; Kay et al., 2019b). In the European Union, the “green architecture” of the new CAP framework (2021–2028) includes the safeguarding of small landscape elements and connectivity features, as well as regionally-adapted and specific voluntary environmental measures (such as agroforestry) (Nilsson et al., 2017; Ustaoglu and Collier, 2018; Balzan et al., 2020; Hristov et al., 2020). Ultimately, agroforestry systems address several sustainable development goals, offer countless ecosystem services and (hopefully) are expected to get more attention and importance in the future of world’s agriculture (Kanter et al., 2018).
Our framework complements previous approaches relating agroforestry and biodiversity conservation in agricultural landscapes. The simple modeling demonstration, supported on a robust theory, could be considered a stepping-stone to support future quantification of biodiversity patterns within agricultural landscapes. Further unraveling of how habitat amount influences biodiversity in agricultural landscapes should consider other variables such as type of species (e.g., guilds), management practices and multiple spatial and temporal scales. Our findings also reinforce the idea that agroforestry is critical to halt biodiversity loss in agricultural landscapes and, despite the limitations inherent to a preliminary demonstration, the methodology developed provides a starting point to anticipate the changes in landscape biodiversity induced by land use change and management options, guiding pertinent strategies to integrate crop production with biodiversity conservation.
The original contributions presented in the study are included in the article/Supplementary Material, further inquiries can be directed to the corresponding author.
MS: conceptualization, formal analysis, investigation, software, writing – original draft, and writing – review and editing. RC: conceptualization and writing – review and editing. RB, DG, A-LP, PL, WB and JC: writing – review and editing. DG: writing – review and editing. BG and MM-L; conceptualization and writing – review and editing. All authors contributed to the article and approved the submitted version.
The following entities that contributed to this work: European Investment Funds by FEDER/COMPETE/POCI– Operational Competitiveness and Internationalization Programme, under Project POCI-01-0145-FEDER-006958, National Funds by FCT – Portuguese Foundation for Science and Technology, under the project UIDB/04033/2020 and INTERACT, Integrative Research in Environment, Agro-Chains and Technology, Project Norte 2020 45/2015/02.
The authors declare that the research was conducted in the absence of any commercial or financial relationships that could be construed as a potential conflict of interest.
All claims expressed in this article are solely those of the authors and do not necessarily represent those of their affiliated organizations, or those of the publisher, the editors and the reviewers. Any product that may be evaluated in this article, or claim that may be made by its manufacturer, is not guaranteed or endorsed by the publisher.
We would like to acknowledge the valuable reflections, ideas and theories of the scientists Leonor Farhig and Santiago Saura which inspired us to prepare the present work. MM-L was supported by the Spanish Ministry of Economy and Competitivity through “Sistemas agroforestales para la producción de cereal como estrategia de adaptación y mitigación al cambio climático en el ámbito de la península ibérica” (AFCLIMA) (CTM2016-80176-C2-1-R) from the Retos Investigación Programme.
The Supplementary Material for this article can be found online at: https://www.frontiersin.org/articles/10.3389/fevo.2021.630151/full#supplementary-material
Abson, D. J. (2019). “The economic drivers and consequences of agricultural specialization,” in Agroecosystem Diversity, eds G. Lemaire, P. C. de Carvalho, S. Kronberg, and S. Recous (Cambridge, MA: Academic Press), 301–315. doi: 10.1016/b978-0-12-811050-8.00019-4
Ansell, D., Freudenberger, D., Munro, N., and Gibbons, P. (2016). The cost-effectiveness of agri-environment schemes for biodiversity conservation: a quantitative review. Agric. Ecosyst. Environ. 225, 184–191.
Arosa, M. L., Bastos, R., Cabral, J. A., Freitas, H., Costa, S. R., and Santos, M. (2017). Long-term sustainability of cork oak agro-forests in the Iberian Peninsula: a model-based approach aimed at supporting the best management options for the montado conservation. Ecol. Model. 343, 68–79.
Avilés, J. M. (2019). Pruning promotes the formation of an insufficient number of cavities for hollow-dependent birds in Iberian Holm-oak dehesas. For. Ecol. Manag. 453:117627. doi: 10.1016/j.foreco.2019.117627
Bakış, A. L. P., Macovei, I., Barros, P., Gomes, C., Carvalho, D., Cabral, J. A., et al. (2021). Is biodiversity linked with farm management options in vineyard landscapes? A case study combining ecological indicators within a hybrid modelling framework. Ecol. Indic. 121:107012.
Balzan, M. V., Sadula, R., and Scalvenzi, L. (2020). Assessing ecosystem services supplied by agroecosystems in Mediterranean Europe: a literature review. Land 9:245. doi: 10.3390/land9080245
Barrios, E., Valencia, V., Jonsson, M., Brauman, A., Hairiah, K., Mortimer, P. E., et al. (2018). Contribution of trees to the conservation of biodiversity and ecosystem services in agricultural landscapes. Int. J. Biodivers. Sci. Ecosyst. Serv. Manag. 14, 1–16. doi: 10.1080/21513732.2017.1399167
Ben-Shachar, M. S. Lüdecke, D., and Makowski, D. (2020). effectsize: estimation of effect size indices and standardized parameters. J. Open Sour. Softw. 5:2815. doi: 10.21105/joss.02815
Benton, T. G., Vickery, J. A., and Wilson, J. D. (2003). Farmland biodiversity: is habitat heterogeneity the key? Trends Ecol. Evol. 18, 182–188. doi: 10.1016/s0169-5347(03)00011-9
Boinot, S., Poulmarc’h, J., Mézière, D., and Lauri, P. É, and Sarthou, J. P. (2019). Distribution of overwintering invertebrates in temperate agroforestry systems: implications for biodiversity conservation and biological control of crop pests. Agric. Ecosyst. Environ. 285:106630.
Bueno, A. S., and Peres, C. A. (2019). Patch-scale biodiversity retention in fragmented landscapes: reconciling the habitat amount hypothesis with the island biogeography theory. J. Biogeogr. 46, 621–632.
Campos, P. Caparrós, A. Cerdá, E., Huntsinger, L., and Standiford, R. B. (2007). “Modeling multifunctional agroforestry systems with environmental values: dehesa in Spain and woodland ranches in California,” in Handbook of Operations Research in Natural Resources, eds A. Weintraub, C. Romero, T. Bjørndal, R. Epstein, and J. Miranda (Boston, MA: Springer), 33–52.
Cavanaugh, K. C., Siegel, D. A., Raimondi, P. T., and Alberto, F. (2014). Patch definition in metapopulation analysis: a graph theory approach to solve the mega-patch problem. Ecology 95, 316–328. doi: 10.1890/13-0221.1
Concepción, E. D., Aneva, I., Jay, M., Lukanov, S., Marsden, K., Moreno, G., et al. (2020). Optimizing biodiversity gain of European agriculture through regional targeting and adaptive management of conservation tools. Biol. Conserv. 241:108384. doi: 10.1016/j.biocon.2019.108384
Concepción, E. D., and Díaz, M. (2019). Varying potential of conservation tools of the Common Agricultural Policy for farmland bird preservation. Sci. Total Environ. 694:133618. doi: 10.1016/j.scitotenv.2019.133618
Dallas, T. A., Saastamoinen, M., Schulz, T., and Ovaskainen, O. (2020). The relative importance of local and regional processes to metapopulation dynamics. J. Anim. Ecol. 89, 884–896. doi: 10.1111/1365-2656.13141
Damianidis, C., Santiago-Freijanes, J. J., den Herder, M., Burgess, P., Mosquera-Losada, M. R., Graves, A., et al. (2020). Agroforestry as a sustainable land use option to reduce wildfires risk in European Mediterranean areas. Agrofor. Syst. 95, 919–929.
de Jalón, S. G., Graves, A., Palma, J. H., Williams, A., Upson, M., and Burgess, P. J. (2018). Modelling and valuing the environmental impacts of arable, forestry and agroforestry systems: a case study. Agrofor. Syst. 92, 1059–1073. doi: 10.1007/s10457-017-0128-z
De Roest, K., Ferrari, P., and Knickel, K. (2018). Specialisation and economies of scale or diversification and economies of scope? Assessing different agricultural development pathways. J. Rural Stud. 59, 222–231. doi: 10.1016/j.jrurstud.2017.04.013
Debussche, M., Lepart, J., and Dervieux, A. (1999). Mediterranean landscape changes: evidence from old postcards. Glob. Ecol. Biogeogr. 8, 3–15.
Den Herder, M., Moreno, G., Mosquera-Losada, R. M., Palma, J. H., Sidiropoulou, A., Freijanes, J. J. S., et al. (2017). Current extent and stratification of agroforestry in the European Union. Agric. Ecosyst. Environ. 241, 121–132.
Evju, M., and Sverdrup-Thygeson, A. (2016). Spatial configuration matters: a test of the habitat amount hypothesis for plants in calcareous grasslands. Landsc. Ecol. 31, 1891–1902.
Fahrig, L. (2013). Rethinking patch size and isolation effects: the habitat amount hypothesis. J. Biogeogr. 40, 1649–1663.
Fahrig, L. (2021). What the habitat amount hypothesis does and does not predict: a reply to Saura. J. Biogeogr. 48, 1530–1535. doi: 10.1111/jbi.14061
Fletcher, R. J. Jr., Didham, R. K., Banks-Leite, C., Barlow, J., Ewers, R. M., Rosindell, J., et al. (2018). Is habitat fragmentation good for biodiversity? Biol. Conserv. 226, 9–15.
Gardiner, R., Bain, G., Hamer, R., Jones, M. E., and Johnson, C. N. (2018). Habitat amount and quality, not patch size, determine persistence of a woodland-dependent mammal in an agricultural landscape. Landsc. Ecol. 33, 1837–1849. doi: 10.1007/s10980-018-0722-0
Geneletti, D., Scolozzi, R., and Adem Esmail, B. (2018). Assessing ecosystem services and biodiversity tradeoffs across agricultural landscapes in a mountain region. Int. J. Biodivers. Sci. Ecosyst. Serv. Manag. 14, 188–208.
Geri, F., Amici, V., and Rocchini, D. (2010). Human activity impact on the heterogeneity of a Mediterranean landscape. Appl. Geogr. 30, 370–379. doi: 10.1016/j.apgeog.2009.10.006
Grass, I., Loos, J., Baensch, S., Batáry, P., Librán-Embid, F., Ficiciyan, A., et al. (2019). Land-sharing/-sparing connectivity landscapes for ecosystem services and biodiversity conservation. People Nat. 1, 262–272.
Grimm, V., Berger, U., DeAngelis, D. L., Polhill, J. G., Giske, J., and Railsback, S. F. (2010). The ODD protocol: a review and first update. Ecol. Model. 221, 2760–2768. doi: 10.1016/j.ecolmodel.2010.08.019
Guillerme, S., Barcet, H., de Munnik, N., Maire, E., and Marais-Sicre, C. (2020). Evolution of traditional agroforestry landscapes and development of invasive species: lessons from the Pyrenees (France). Sustain. Sci. 15, 1285–1299. doi: 10.1007/s11625-020-00847-1
Haddad, N. M., Gonzalez, A., Brudvig, L. A., Burt, M. A., Levey, D. J., and Damschen, E. I. (2017). Experimental evidence does not support the habitat amount hypothesis. Ecography 40, 48–55. doi: 10.1111/ecog.02535
Haggar, J., Pons, D., Saenz, L., and Vides, M. (2019). Contribution of agroforestry systems to sustaining biodiversity in fragmented forest landscapes. Agric. Ecosyst. Environ. 283:106567. doi: 10.1016/j.jenvman.2019.109504
Hanane, S., Cherkaoui, S. I., Magri, N., and Yassin, M. (2019). Bird species richness in artificial plantations and natural forests in a North African agroforestry system: assessment and implications. Agrofor. Syst. 93, 1755–1764. doi: 10.1007/s10457-018-0281-z
Hanski, I. (2015). Habitat fragmentation and species richness. J. Biogeogr. 42, 989–993. doi: 10.1111/jbi.12478
Harlio, A., Kuussaari, M., Heikkinen, R. K., and Arponen, A. (2019). Incorporating landscape heterogeneity into multi-objective spatial planning improves biodiversity conservation of semi-natural grasslands. J. Nat. Conserv. 49, 37–44.
Hristov, J., Clough, Y., Sahlin, U., Smith, H. G., Stjernman, M., Olsson, O., et al. (2020). Impacts of the EU’s common agricultural policy “Greening” reform on agricultural development, biodiversity, and ecosystem services. Appl. Econ. Perspect. Policy 42, 716–738.
Jackson, L. E., Bowles, T. M., Ferris, H., Margenot, A. J., Hollander, A., Garcia-Palacios, P., et al. (2019). Plant and soil microfaunal biodiversity across the borders between arable and forest ecosystems in a Mediterranean landscape. Appl. Soil Ecol. 136, 122–138. doi: 10.1016/j.apsoil.2018.11.015
Kanda, Y. (2013). Investigation of the freely available easy-to-use software ‘EZR’for medical statistics. Bone Marrow Transplant. 48, 452–458. doi: 10.1038/bmt.2012.244
Kanter, D. R., Musumba, M., Wood, S. L., Palm, C., Antle, J., Balvanera, P., et al. (2018). Evaluating agricultural trade-offs in the age of sustainable development. Agric. Syst. 163, 73–88. doi: 10.1016/j.agsy.2016.09.010
Karner, K., Cord, A. F., Hagemann, N., Hernandez-Mora, N., Holzkämper, A., Jeangros, B., et al. (2019). Developing stakeholder-driven scenarios on land sharing and land sparing–Insights from five European case studies. J. Environ. Manag. 241, 488–500. doi: 10.1016/j.jenvman.2019.03.050
Kay, S., Graves, A., Palma, J. H., Moreno, G., Roces-Díaz, J. V., Aviron, S., et al. (2019a). Agroforestry is paying off–Economic evaluation of ecosystem services in European landscapes with and without agroforestry systems. Ecosyst. Serv. 36:100896. doi: 10.1016/j.ecoser.2019.100896
Kay, S., Rega, C., Moreno, G., den Herder, M., Palma, J. H., Borek, R., et al. (2019b). Agroforestry creates carbon sinks whilst enhancing the environment in agricultural landscapes in Europe. Land Use Policy 83, 581–593. doi: 10.1016/j.landusepol.2019.02.025
Liu, X., Bakshi, B. R., Rugani, B., de Souza, D. M., Bare, J., Johnston, J. M., et al. (2020). Quantification and valuation of ecosystem services in life cycle assessment: application of the cascade framework to rice farming systems. Sci. Total Environ. 747:141278. doi: 10.1016/j.scitotenv.2020.141278
Lüscher, G., Whittington, A. E., and Gillingham, P. K. (2016). Farmland biodiversity and agricultural management on 237 farms in 13 European and 2 African regions. Ecology 97:1625. doi: 10.1890/15-1985.1
Malek, Ž, Verburg, P. H., Geijzendorffer, I. R., Bondeau, A., and Cramer, W. (2018). Global change effects on land management in the Mediterranean region. Glob. Environ. Change 50, 238–254. doi: 10.1016/j.gloenvcha.2018.04.007
Marconi, L., and Armengot, L. (2020). Complex agroforestry systems against biotic homogenization: the case of plants in the herbaceous stratum of cocoa production systems. Agric. Ecosyst. Environ. 287:106664. doi: 10.1016/j.agee.2019.106664
Marja, R., Kleijn, D., Tscharntke, T., Klein, A. M., Frank, T., and Batáry, P. (2019). Effectiveness of agri-environmental management on pollinators is moderated more by ecological contrast than by landscape structure or land-use intensity. Ecol. Lett. 22, 1493–1500. doi: 10.1111/ele.13339
Marull, J., Tello, E., Bagaria, G., Font, X., Cattaneo, C., and Pino, J. (2018). Exploring the links between social metabolism and biodiversity distribution across landscape gradients: a regional-scale contribution to the land-sharing versus land-sparing debate. Sci. Total Environ. 619, 1272–1285. doi: 10.1016/j.scitotenv.2017.11.196
Mas, J. F., Kolb, M., Paegelow, M., Olmedo, M. T. C., and Houet, T. (2014). Inductive pattern-based land use/cover change models: a comparison of four software packages. Environ. Model. Softw. 51, 94–111.
McNeely, J. A. (2004). “Nature vs. nurture: managing relationships between forests, agroforestry and wild biodiversity,” in New Vistas in Agroforestry, eds P. K. R. Nair, M.R. Rao, and L.E. Buck, (Dordrecht: Springer), 155–165. doi: 10.1023/b:agfo.0000028996.92553.ea
Melo, G. L., Sponchiado, J., Cáceres, N. C., and Fahrig, L. (2017). Testing the habitat amount hypothesis for South American small mammals. Biol. Conserv. 209, 304–314. doi: 10.1016/j.biocon.2017.02.031
Merckx, T., Dantas de Miranda, M., and Pereira, H. M. (2019). Habitat amount, not patch size and isolation, drives species richness of macro-moth communities in countryside landscapes. J. Biogeogr. 46, 956–967. doi: 10.1111/jbi.13544
Montagnini, F. (ed.) (2017). Integrating Landscapes: Agroforestry for Biodiversity Conservation and Food Sovereignty, Vol. 494. Berlin: Springer.
Moreno, G., Aviron, S., Berg, S., Crous-Duran, J., Franca, A., de Jalón, S. G., et al. (2018). Agroforestry systems of high nature and cultural value in Europe: provision of commercial goods and other ecosystem services. Agrofor. Syst. 92, 877–891. doi: 10.1007/s10457-017-0126-1
Morrison, J., Izquierdo, J., Plaza, E. H., and González-Andújar, J. L. (2017). The role of field margins in supporting wild bees in Mediterranean cereal agroecosystems: which biotic and abiotic factors are important? Agric. Ecosyst. Environ. 247, 216–224.
Mosquera-Losada, M. R., McAdam, J. H., Romero-Franco, R., Santiago-Freijanes, J. J., and Rigueiro-Rodróguez, A. (2009). “Definitions and components of agroforestry practices in Europe,” in Agroforestry in Europe, eds A. Rigueiro-Rodróguez., J. McAdam and M.R. Mosquera-Losada (Dordrecht: Springer), 3–19. doi: 10.1007/978-1-4020-8272-6_1
Nilsson, L., Andersson, G. K., Birkhofer, K., and Smith, H. G. (2017). Ignoring ecosystem-service cascades undermines policy for multifunctional agricultural landscapes. Front. Ecol. Evol. 5:109. doi: 10.3389/fevo.2017.00109
Palacín, C., and Alonso, J. C. (2018). Failure of EU Biodiversity Strategy in Mediterranean farmland protected areas. J. Nat. Conserv. 42, 62–66.
Pardo, A., Rolo, V., Concepción, E. D., Diaz, M., Kazakova, Y., Stefanova, V., et al. (2020). To what extent does the European common agricultural policy affect key landscape determinants of biodiversity? Environ. Sci. Policy 114, 595–605.
Pavlis, E. S., Terkenli, T. S., Kristensen, S. B., Busck, A. G., and Cosor, G. L. (2016). Patterns of agri-environmental scheme participation in Europe: indicative trends from selected case studies. Land Use Policy 57, 800–812. doi: 10.1016/j.landusepol.2015.09.024
Phalan, B., Onial, M., Balmford, A., and Green, R. E. (2011). Reconciling food production and biodiversity conservation: land sharing and land sparing compared. Science 333, 1289–1291. doi: 10.1126/science.1208742
Plieninger, T., Munoz-Rojas, J., Buck, L. E., and Scherr, S. J. (2020). Agroforestry for sustainable landscape management. Sustain. Sci. 15, 1255–1266. doi: 10.1007/s11356-021-17605-0
Plieninger, T., Torralba, M., Hartel, T., and Fagerholm, N. (2019). Perceived ecosystem services synergies, trade-offs, and bundles in European high nature value farming landscapes. Landsc. Ecol. 34, 1565–1581. doi: 10.1007/s10980-019-00775-1
Prevedello, J. A., Gotelli, N. J., and Metzger, J. P. (2016). A stochastic model for landscape patterns of biodiversity. Ecol. Monogr. 86, 462–479.
R Core Team (2020). R: A Language and Environment for Statistical Computing. Vienna: R Foundation for Statistical Computing. Available online at: https://www.R-project.org/
Rabelo, R. M., Bicca-Marques, J. C., Aragón, S., and Nelson, B. W. (2017). Are fluvial islands “real” islands for arboreal mammals? Uncovering the effect of patch size under the species–area relationship. J. Biogeogr. 44, 1802–1812.
Richard, R., Cahon, T., Llandres, A. L., Le Levier, L., Proudhom, G., and Casas, J. (2020). Alley cropping agroforestry mediates carabid beetle distribution at a micro-habitat scale. Agrofor. Syst. 94, 309–317. doi: 10.1007/s10457-019-00390-8
Riginos, C., Grace, J. B., Augustine, D. J., and Young, T. P. (2009). Local versus landscape-scale effects of savanna trees on grasses. J. Ecol. 97, 1337–1345.
Rosas-Ramos, N., Baños-Picón, L., Trivellone, V., Moretti, M., Tormos, J., and Asís, J. D. (2019). Ecological infrastructures across Mediterranean agroecosystems: towards an effective tool for evaluating their ecological quality. Agric. Syst. 173, 355–363. doi: 10.1016/j.agsy.2019.03.017
Rosati, A., Borek, R., and Canali, S. (2020). Agroforestry and organic agriculture. Agrofor. Syst. 95, 805–821. doi: 10.1007/s13280-020-01353-5
Santos, M., Carvalho, D., Luis, A., Bastos, R., Hughes, S. J., and Cabral, J. A. (2019). Can recreational ecosystem services be inferred by integrating non-parametric scale estimators within a modelling framework? The birdwatching potential index as a case study. Ecol. Indic. 103, 395–409. doi: 10.1016/j.ecolind.2019.04.026
Santos, M., Ferreira, D., Bastos, R., Vicente, J., Honrado, J., Kueffer, C., et al. (2016). Linking landscape futures with biodiversity conservation strategies in northwest Iberia—A simulation study combining surrogates with a spatio-temporal modelling approach. Ecol. Inform. 33, 85–100. doi: 10.1016/j.ecoinf.2016.04.008
Santos, M., Silva, S., Bastos, R., Carvalho, D., and Cabral, J. A. (2018). How good are the ecological assumptions and predictions made in the past? Insights from a dynamic modelling approach applied to changing landscapes. Ecol. Indic. 90, 226–230. doi: 10.1016/j.ecolind.2018.03.023
Santos, P. Z. F., Crouzeilles, R., and Sansevero, J. B. B. (2019). Can agroforestry systems enhance biodiversity and ecosystem service provision in agricultural landscapes? A meta-analysis for the Brazilian Atlantic Forest. For. Ecol. Manag. 433, 140–145.
Saura, S. (2021). The habitat amount hypothesis implies negative effects of habitat fragmentation on species richness. J. Biogeogr. 48, 11–22. doi: 10.1111/jbi.13958
Semper-Pascual, A., Burton, C., Baumann, M., Decarre, J., Gavier-Pizarro, G., Gómez-Valencia, B., et al. (2021). How do habitat amount and habitat fragmentation drive time-delayed responses of biodiversity to land-use change? Proc. R. Soc. B Biol. Sci. 288:20202466. doi: 10.1098/rspb.2020.2466
Sequeira, A. M., Bouchet, P. J., Yates, K. L., Mengersen, K., and Caley, M. J. (2018). Transferring biodiversity models for conservation: opportunities and challenges. Methods Ecol. Evol. 9, 1250–1264.
Simonson, W. D., Allen, H. D., Parham, E., e Santos, E. D. B., and Hotham, P. (2018). Modelling biodiversity trends in the montado (wood pasture) landscapes of the Alentejo, Portugal. Landsc. Ecol. 33, 811–827. doi: 10.1007/s10980-018-0627-y
Sokos, C. K., Mamolos, A. P., Kalburtji, K. L., and Birtsas, P. K. (2013). Farming and wildlife in Mediterranean agroecosystems. J. Nat. Conserv. 21, 81–92.
Steffan-Dewenter, I., Kessler, M., Barkmann, J., Bos, M. M., Buchori, D., Erasmi, S., et al. (2007). Tradeoffs between income, biodiversity, and ecosystem functioning during tropical rainforest conversion and agroforestry intensification. Proc. Natl. Acad. Sci. U. S. A. 104, 4973–4978. doi: 10.1073/pnas.0608409104
Sullivan, G. M., and Feinn, R. (2012). Using effect size—or why the P value is not enough. J. Grad. Med. Educ. 4, 279–282. doi: 10.4300/JGME-D-12-00156.1
Toderi, M., Francioni, M., Seddaiu, G., Roggero, P. P., Trozzo, L., and D’Ottavio, P. (2017). Bottom-up design process of agri-environmental measures at a landscape scale: evidence from case studies on biodiversity conservation and water protection. Land Use Policy 68, 295–305. doi: 10.1016/j.landusepol.2017.08.002
Torralba, M., Fagerholm, N., Burgess, P. J., Moreno, G., and Plieninger, T. (2016). Do European agroforestry systems enhance biodiversity and ecosystem services? A meta-analysis. Agric. Ecosyst. Environ. 230, 150–161.
Udawatta, P. R., Rankoth, L., and Jose, S. (2019). Agroforestry and biodiversity. Sustainability 11:2879.
Ustaoglu, E., and Collier, M. J. (2018). Farmland abandonment in Europe: an overview of drivers, consequences, and assessment of the sustainability implications. Environ. Rev. 26, 396–416.
Van Doorn, A. M., and Bakker, M. M. (2007). The destination of arable land in a marginal agricultural landscape in South Portugal: an exploration of land use change determinants. Landsc. Ecol. 22, 1073–1087.
van Noordwijk, M., Duguma, L. A., Dewi, S., Leimona, B., Catacutan, D. C., Lusiana, B., et al. (2018). SDG synergy between agriculture and forestry in the food, energy, water and income nexus: reinventing agroforestry? Curr. Opin. Environ. Sustain. 34, 33–42.
Varah, A., Jones, H., Smith, J., and Potts, S. G. (2020). Temperate agroforestry systems provide greater pollination service than monoculture. Agric. Ecosyst. Environ. 301:107031. doi: 10.1016/j.agee.2020.107031
Watling, J. I., Arroyo-Rodríguez, V., Pfeifer, M., Baeten, L., Banks-Leite, C., Cisneros, L. M., et al. (2020). Support for the habitat amount hypothesis from a global synthesis of species density studies. Ecol. Lett. 23, 674–681. doi: 10.1111/ele.13471
White, J. W., Rassweiler, A., Samhouri, J. F., Stier, A. C., and White, C. (2014). Ecologists should not use statistical significance tests to interpret simulation model results. Oikos 123, 385–388. doi: 10.1111/j.1600-0706.2013.01073.x
Whittaker, R. J., Fernández-Palacios, J. M., Matthews, T. J., Borregaard, M. K., and Triantis, K. A. (2017). Island biogeography: taking the long view of nature’s laboratories. Science 357:eaam8326. doi: 10.1126/science.aam8326
Wilensky, U. (1999). NetLogo. Evanston, IL: Center for Connected Learning and Computer-Based Modeling, Northwestern University.
Wilson, S., Mitchell, G. W., Pasher, J., McGovern, M., Hudson, M. A. R., and Fahrig, L. (2017). Influence of crop type, heterogeneity and woody structure on avian biodiversity in agricultural landscapes. Ecol. Indic. 83, 218–226. doi: 10.1016/j.ecolind.2017.07.059
Yves, T., Koutroulis, A., Samaniego, L., Vicente-Serrano, S. M., Volaire, F., Boone, A., et al. (2020). Challenges for drought assessment in the Mediterranean region under future climate scenarios. Earth-Sci. Rev. 210:103348.
Keywords: land-sharing, land-sparing, agroecosystems, trade-offs, landscape heterogeneity, fragmentation, biodiversity management
Citation: Santos M, Cajaiba RL, Bastos R, Gonzalez D, Petrescu Bakış A-L, Ferreira D, Leote P, Barreto da Silva W, Cabral JA, Gonçalves B and Mosquera-Losada MR (2022) Why Do Agroforestry Systems Enhance Biodiversity? Evidence From Habitat Amount Hypothesis Predictions. Front. Ecol. Evol. 9:630151. doi: 10.3389/fevo.2021.630151
Received: 16 November 2020; Accepted: 20 December 2021;
Published: 27 January 2022.
Edited by:
Bradley S. Case, Auckland University of Technology, New ZealandReviewed by:
Sergio Noce, Fondazione Centro Euro-Mediterraneo sui Cambiamenti Climatici (CMCC), ItalyCopyright © 2022 Santos, Cajaiba, Bastos, Gonzalez, Petrescu Bakış, Ferreira, Leote, Barreto da Silva, Cabral, Gonçalves and Mosquera-Losada. This is an open-access article distributed under the terms of the Creative Commons Attribution License (CC BY). The use, distribution or reproduction in other forums is permitted, provided the original author(s) and the copyright owner(s) are credited and that the original publication in this journal is cited, in accordance with accepted academic practice. No use, distribution or reproduction is permitted which does not comply with these terms.
*Correspondence: Mário Santos, bWdzYW50b3NAdXRhZC5wdA==, bWdzYW50b3NzQGdtYWlsLmNvbQ==
Disclaimer: All claims expressed in this article are solely those of the authors and do not necessarily represent those of their affiliated organizations, or those of the publisher, the editors and the reviewers. Any product that may be evaluated in this article or claim that may be made by its manufacturer is not guaranteed or endorsed by the publisher.
Research integrity at Frontiers
Learn more about the work of our research integrity team to safeguard the quality of each article we publish.