- 1Laboratorio de Biología Marina, Facultad de Biología, Universidad de Sevilla, Seville, Spain
- 2Área de Investigación I+D+i del Acuario de Sevilla, Seville, Spain
- 3Estación de Biología Marina del Estrecho, Ceuta, Spain
Plastic debris constitutes up to 87% of marine litter and represents one of the most frequently studied vectors for marine alien species with invasive potential in the last 15 years. This review addresses an integrated analysis of the different factors involved in the impact of plastic as a vector for the dispersal of marine species. The sources of entry of plastic materials into the ocean are identified as well as how they move between different habitats affecting each trophic level and producing hot spots of plastic accumulation in the ocean. The characterization of plastic as a dispersal vector for marine species has provided information about the inherent properties of plastics which have led to its impact on the ocean: persistence, buoyancy, and variety in terms of chemical composition, all of which facilitate colonization by macro and microscopic species along with its dispersion throughout different oceans and ecosystems. The study of the differences in the biocolonization of plastic debris according to its chemical composition provided fundamental information regarding the invasion process mediated by plastic, and highlighted gaps of knowledge about this process. A wide range of species attached to plastic materials has been documented and the most recurrent phyla found on plastic have been identified from potentially invasive macrofauna to toxic microorganisms, which are capable of causing great damage in places far away from their origin. Plastic seems to be more efficient than the natural oceanic rafts carrying taxa such as Arthropoda, Annelida, and Mollusca. Although the differential colonization of different plastic polymers is not clear, the chemical composition might determine the community of microorganisms, where we can find both pathogens and virulent and antibiotic resistance genes. The properties of plastic allow it to be widely dispersed in practically all ocean compartments, making this material an effective means of transport for many species that could become invasive.
Introduction
Marine ecosystems around the world are threatened by several factors related to human activity (Ibabe et al., 2020), such as biological invasions (Ojaveer et al., 2015). Invasive species generally have a strong influence on the invaded environment, altering the structure of the community and the functions of the ecosystem, through competition with native species (Bertness, 1984), introduction of pathogens (Rilov and Crooks, 2009b), or indirect changes in habitat conditions (Crooks, 2002).
The exponential increase in the rate of invasive alien species (IAS) over the last decades has stimulated the study of biological invasions (Seebens et al., 2017), especially in the marine environment, which has received less attention compared to the terrestrial one (Davis, 2000; Katsanevakis et al., 2014). In addition to traditional marine vectors (biofouling and ballast water), which gained importance with the development of commercial shipping (Carlton, 1987; Clarke Murray et al., 2014), new challenges were added to the study of dispersion factors, such as plastic materials (see Audrézet et al., 2020; previous and complementary article of this review, mainly focused on the biosecurity of marine plastic debris and the knowledge gaps and research priorities that exist on this topic), other materials derived from aquaculture or aquarium hobbies (Rilov and Crooks, 2009b; Walters et al., 2011).
Plastic is a potential dispersal vector of marine species (Rech et al., 2016). It is the most common marine debris, constituting 61–87% of all types of marine debris (Eriksen et al., 2014; Serra-Gonçalves et al., 2019), and is considered as one of the major threats to marine biodiversity (Avio et al., 2017). Plastic production has increased exponentially in the last 60 years, from 0.5 million tons in 1960, to almost 300 million tons in 2013 (PlasticsEurope, 2014) and 360 million tons in 2018 (PlasticsEurope, 2018). About 10% of plastic production has been introduced into marine ecosystems (Thompson, 2006) through land-based sources such as rivers, storm drains (Moore et al., 2011), urban runoff, sewage discharge, effluents from plastic manufacturing factories (Eerkes-Medrano et al., 2015), landfills or recycling points (Alomar et al., 2016), coastal areas due to the action of the wind, illegal dumping, fishing, and other human activities (Derraik, 2002). van Sebille et al. (2015) estimated that microplastics (MPs) in the oceans have reached 52.2 × 1012 particles, 236,000 metric tons, mainly distributed in the centers of the subtropical gyres.
The global distribution, buoyancy, and high levels of colonization of plastic debris greatly facilitate the transport of microbial communities (Carson et al., 2013), algae, invertebrates, and fish (Goldstein et al., 2014) to non-native regions (Barnes, 2002). Marine plastic debris is not only a threat to marine wildlife, but also causes significant economic and ecological damage (Keswani et al., 2016) acting both as a vector for the primary introduction of alien species into remote regions, and as a secondary vector for the regional expansion of marine species (Rech et al., 2016; Audrézet et al., 2020).
Several gaps remain to be filled regarding the potential of plastic as a species vector. For example, the harmonization of methodological approaches to study marine litter in different environmental compartments (Galgani et al., 2019) or the impact caused by the secondary propagation, which is not yet sufficiently documented (National Oceanic and Atmospheric Administration Marine Debris Program, 2017). Also, understanding the biosecurity implications associated with plastics could be a vital step toward understanding, monitoring, and eventually mitigating its impacts on a global scale (Audrézet et al., 2020).
This work aims to identify the dispersal potential of plastic as a vector for alien species introductions and to compare it with other vectors, as well as to expose the qualitative composition of the communities that inhabit plastic debris. On the other hand, we attempt to synthesize the methodological aspects of the detection of AIS introduced through plastic debris and the prevention of their negative impacts.
Methodology
Scientific literature published in the last 30 years (1990–2020) was collected from Science Direct, Scopus, Web of Science, and Google Scholar scientific databases, and the most widely consulted publishers and/or scientific internet networks were Elsevier, Springer, ResearchGate, Wiley Online Library, Dialnet, and Academia. The keywords related to invasive species in the ocean, especially those carried by plastic debris, were used in the title and keywords field: “Alien Species,” “Ballast Water,” “Biofouling,” “Ecology,” “Ecosystem,” “Impact,” “Invasive Species,” “Marine,” “Management,” “Microplastics,” “Ocean,” “Plastic Debris,” “Rafting,” “Sea,” “Threat,” “Transport,” “Vector,” and “Waste.” The searches were conducted mostly in March 2020 on the full range of articles or reviews available at that time. The last search was made on April 20, 2020. This initial search yielded a total of 447 articles which included information on invasive and potentially invasive species in the ocean and different dispersal vectors. In this preliminary library, a pre-selection step was carried out according to the presence of at least one of three criteria: (1) articles focused on the impact caused by one or more invasive marine species; (2) articles focused on the management of the invasion of one or more marine species; or (3) articles that include both concepts. After applying these selection criteria, 228 articles were obtained, of which 48 were discarded after analysis because they were not directly related to the topic with respect to the sections considered in the manuscript. Therefore, most of the information presented in this paper was extracted from 180 scientific publications. In addition, other articles named in the literature and previously known to the authors due to their high topic relevance were used for the review.
Selected articles were classified according to the dispersion vector(s) (Plastic Debris, Boat hulls (biofouling), Climatic Events, Ballast Water, Aquaculture, or General), their publication date (1990–2005 or 2006–2020), and the aspect addressed: Impact (I), Management (M) or Impact+Management (I+M). Impacts included articles focused on describing the impacts produced by alien species, and Management included articles focused on the management of these impacts. We separated the last 30 years into two bands to appreciate the differences in the efforts made by scientists regarding different topics in the near past and at present. On the other hand, the label “General” was included for those papers that covered more than one vector.
For the invasive or potentially invasive species listed in Table 1, it was specified whether they were sessile or no sessile, in order to draw conclusions about the biology of the species inhabiting plastic. Also, it was specified the transport vector for which they were identified (Plastic Debris, Boat hulls (biofouling), Climatic Events, Ballast Water, Aquaculture, Aquariums, or Transoceanic Channels/Swimming). The native and non-native locations of the invasive and potentially invasive species were indicated.

Table 1. Compilation of invasive and non-native species which have been introduced or transported into areas far from their origin by the following dispersal vectors: Plastic, boat hulls (biofouling), ballast water, aquaculture, aquarium, and transoceanic channel/swimming.
Moreover, other relevant information was extracted from the selected and related articles such as plastic as a vector, different types of plastic and how their characteristics affect the colonization of macro and microscopic marine species, recurrent species transported by plastics, associated microorganisms dispersion and species and dispersal patterns of plastic in the ocean and how they can influence horizontal and vertical transport.
Plastic Debris Compared to Other Dispersal Vectors
With respect to the total number of articles, the labels that yielded the highest number of selected articles were “Boat hulls (biofouling) and Ballast Water” including I, M, and I + M, with 40 and 31 papers, respectively, followed by “Plastic Debris, Aquaculture and Climate Events” (21, 21, 15, respectively) (Figure 1).
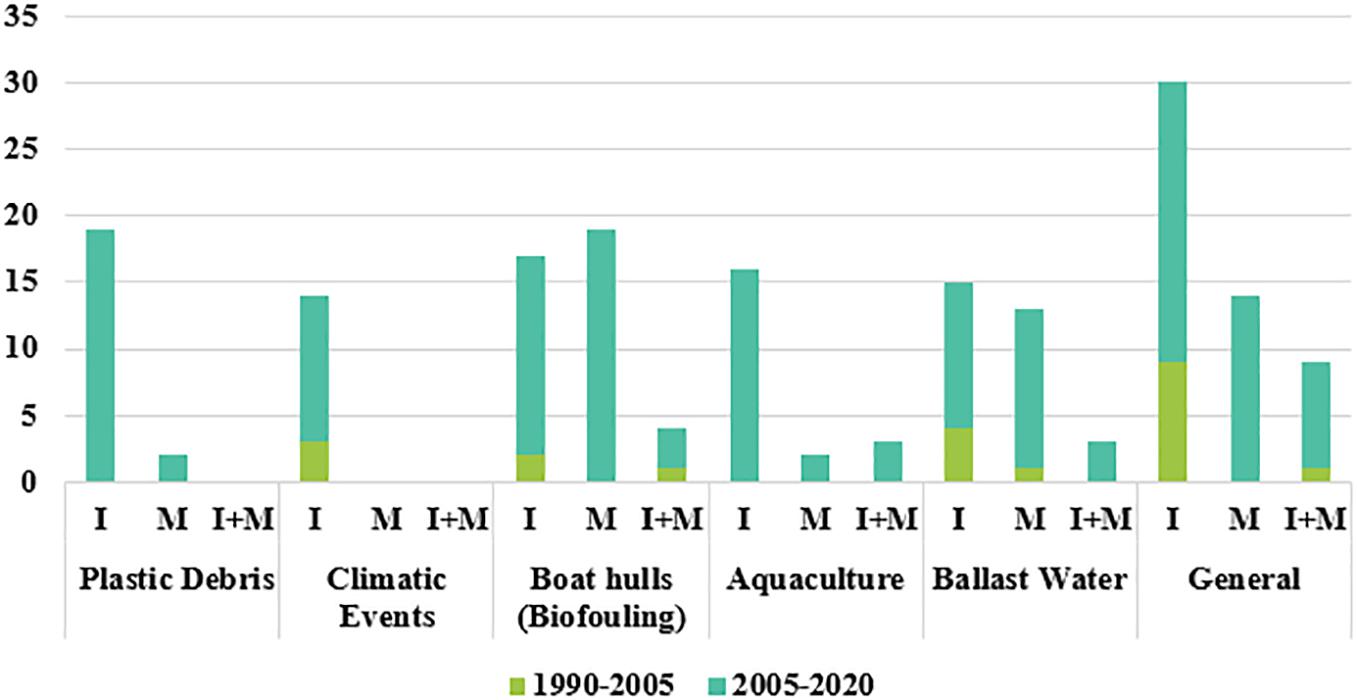
Figure 1. Percentage of alien marine species introduced by different dispersal vectors on a global scale (see Table 1 for details).
In the last 15 years, the most frequent labels were “Plastic Debris-Impact,” and “Biofouling – Management” with 19 articles each. No articles were selected between 1990 and 2005 for the labels “Plastic Debris” and “Aquaculture.” There was also a great difference in the number of research papers on the management of invasive species from the dispersal vectors “Boat hulls (biofouling)” and “Ballast Water,” and the vectors “Plastic Debris,” “Aquaculture,” and “Climatic Events.”
Of the 216 exotic species identified in the present study (Table 1), 68% were considered to have been introduced through maritime transport, divided into the categories “Boat hulls (biofouling)” and “Ballast Water,” followed by dispersal as a consequence of the “Aquaculture” (16%), and “Plastic debris” (5%) (Figure 2).
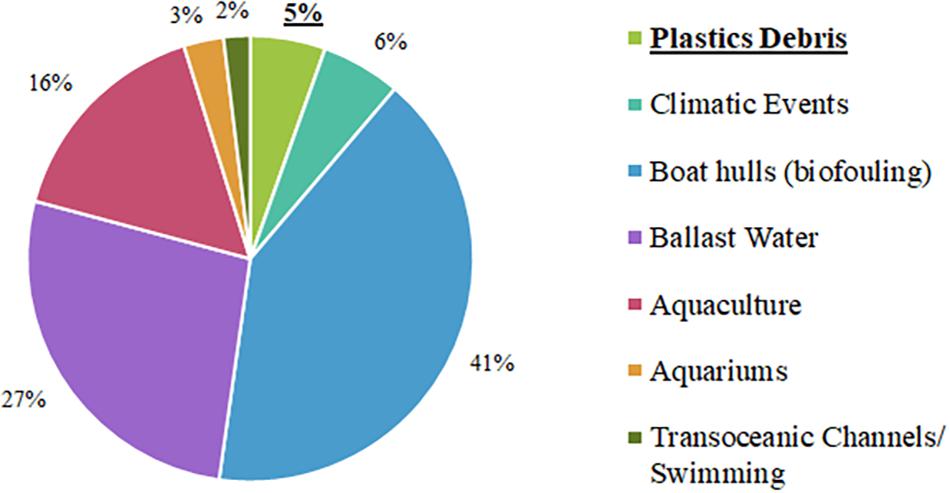
Figure 2. Scientific publications on the vectors for the introduction of alien marine species (plastic, rafting, biofouling aquaculture, ballast water and general) over the last 30 years (1990-2020) and classified by Impact (I), Management (M) and Impact and Management (I+M).
This result is to be expected, as commercial shipping as a cause of IAS dispersal has been cited long before other vectors such as plastic (e.g., Carlton, 1987). Although it is a more recent problem, we consider that the studies on plastics as an IAS vector were quite important between 2005 and 2020. Furthermore, it is expected that the number of papers on plastic as a vector of species will increase in the coming years, as its production increases every year and it is currently an emergent topic.
Characterization of Plastic as a Vector
Plastic debris abundance (Winston et al., 1997), artificial origin (Glasby et al., 2007; Pinochet et al., 2020), and properties can affect its potential to act as a vector of IAS: durability, buoyancy (Schoener and Rowe, 1970), size, and structural complexity of the surface determine colonization by marine organisms and the succession of the community associated with plastic debris, with differences in the sessile and mobile organisms (Kiessling et al., 2015).
The increasing introduction of plastic pollution into the ocean increases the chances for alien species to become invasive. For example, the bryozoan Electra tenella [Hickins, 1880; this name is currently not accepted and it is Arbopercula tenella (Hickins, 1880)] previously identified on natural rafts, may be increasing in abundance and distribution due to the increasing amounts of plastic entering the Caribbean currents and the Gulf Stream (Winston et al., 1997). Natural rafts (eg, wood, pumice, and marine vegetation) are generally characterized by low or patchy abundance, limited longevity, and relatively high habitability, due to high surface roughness, structural complexity, and biodegradability (Gil and Pfaller, 2016). Compared to natural rafts, the abundance of plastic debris is increasing (Ebbesmeyer and Ingraham, 1992), and its longevity generally exceeds that of natural debris, taking decades or even centuries to be degraded (Gregory, 1999). The durability of plastic along with its buoyancy in comparison to organic materials (Schoener and Rowe, 1970) allows a greater dispersal potential for organisms that colonize plastic debris (Barnes, 2002; Barnes and Milner, 2004), by increasing their potential travel distance (Thiel and Gutow, 2005).
Wasson et al. (2005) suggested that alien species preferred hard artificial materials (rip rap, gravel bars, pilings, and docks), while native species were found mainly on soft substrates. Pinochet et al. (2020) affirmed that native species are more commonly found on natural surfaces; for example, native algae such as Sargassum sp. and Corallina sp. are prevalent on natural reefs but not on artificial structures (Glasby et al., 2007). Pinochet et al. (2020) found that the settlement of the larvae of two invasive species of the genus Bugula on plastic surfaces was 70% higher than in cement or wood. Furthermore, settlement on plastic substrates was extremely rapid, with 50% of the larvae settling only after 5 min. For some species of invasive bryozoans, it has been suggested that their prevalence in artificial structures and settlement on plastic panels is explained by their ecology, since they are early successional species (Vail and Tranter, 1981), they show a faster growth, an early initiation of reproductive stages and have higher metabolic rates, allowing them to outgrow their competitors in the early successional stages of the developing community (Pettersen et al., 2016; Lagos et al., 2017). Astudillo et al. (2009) reported that approximately 60% of the fauna found on plastic buoys in Coquimbo Bay, a temperate zone of the Southeast Pacific Ocean, had direct development or short larval durations, so they were capable of maintaining persistent populations in floating elements, suggesting a high potential for long-distance dispersal of fauna on buoys.
Recent data suggest that larger pieces of plastic debris support greater biological diversity, which is consistent with the classic species-area relationships inherent in the biogeography of islands (Simberloff, 1976; Gil and Pfaller, 2016; Garcia-Vazquez et al., 2018). Debroas et al. (2017) observed a higher bacterial and eukaryotic richness in polyethylene (PE) of mesoplastic size (5 mm–20 cm) compared to MPs of 300 μm–5 mm, mainly PE. However, it is necessary to consider the complexity of the debris materials, since those with greater structural complexity (for example, groups of tangled ropes) support greater diversity (Goldstein et al., 2014). Plastic debris of all sizes often has limited structural complexity and smooth, rigid surfaces (e.g., buoys, containers, balls, liners). These characteristics can limit the habitability of plastic waste for many species, since a wide variety of organisms require shelter to persist (Gil and Pfaller, 2016). Even floating harbor pontoons, which carry well-established biofouling communities, can be an important vector for the massive expansion of native species in the face of extreme events that destroy them, such as tsunamis (Wang et al., 2016), displacing them thousands of kilometers away (Figures 3A–D).
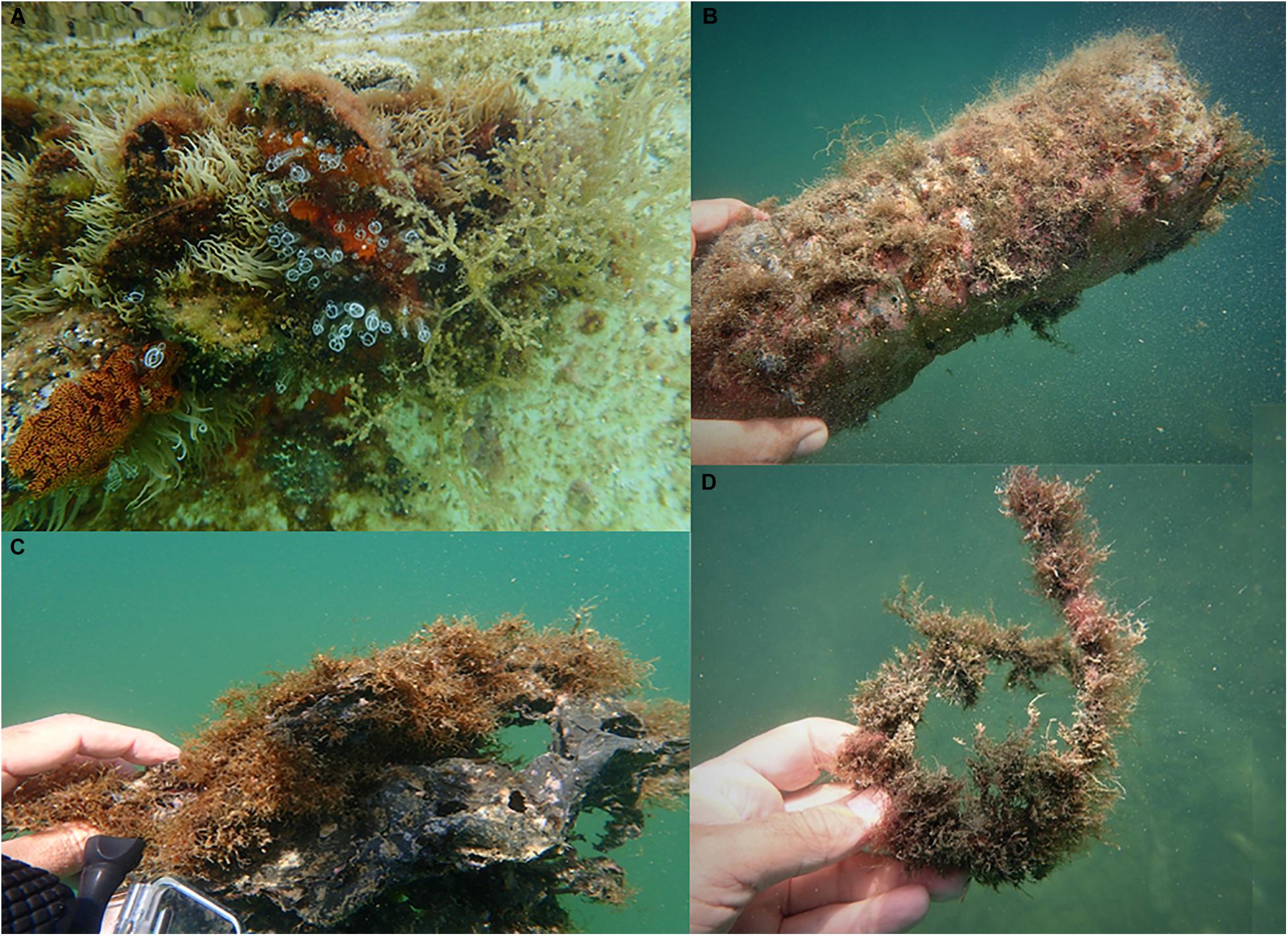
Figure 3. Plastic fouling examples: (A) in floating boxes of polystyrene docks covered with pvc carrying fauna such us the invasive species Amathia verticillata (their breakage, due to a storm or tsunami (see text) can lead to their dispersion in the sea over great distances); (B,C) plastic bottle and plastic bag taken from the bottom of a port, with incipient cauloids of the possible fine morphotype of the Asian invasive algae, Rugulopteryx okamurae; (D) loose end nylon net, extracted from the bottom of a port, completely covered with biofouling. Pictures were taken at Leisure port La Alcaidesa (La Línea), Bay of Algeciras.
Gil and Pfaller (2016) studied the relationship between the area and the structural complexity of marine plastic debris and the colonization of species. The study revealed contrasting patterns for the richness of sessile and mobile taxa. Regarding the number of sessile taxa on debris, the increase in surface had a significant positive effect, while the cover of barnacles of the genus Lepas had a significant negative effect. However, regarding the number of mobile taxa on the debris, the increase in surface area had a trivial positive effect, while the number of barnacles had a significant positive effect. These results suggest that barnacles of the genus Lepas act as base species in communities on plastic debris, providing a complex structural habitat on otherwise structurally limited plastic debris. In agreement with these data, Astudillo et al. (2009) carried out a study on biota inhabiting buoys in the sea and observed that the number of mobile species on buoys was positively related to the number and biomass of sessile species. Thus, benthic species which colonize plastic surfaces are considered eco-engineers, since they provide a habitat for mobile species that otherwise would not be able to colonize these surfaces (Astudillo et al., 2009).
Differential Colonization in the Different Types of Plastic Polymers
The five main classes of plastic polymers, which comprise about 90% of polymer production, are polyethylene (PE), polypropylene (PP), polyvinyl chloride (PVC), polystyrene (PS), and polyethylene terephthalate (PET), with the latter being the most abundant in the ocean (Andrady and Neal, 2009).
While many authors have observed no evidence that the type of polymer is relevant for the composition of the macrobiota associated with plastics, Gündoǧdu (2017) have found that the type of plastic (PE, PET, and PP) shows significant differences with respect to the diversity and abundance of species. On the other hand, it is commonly accepted that the difference in structural and/or chemical properties (plasticizers and colorants) observed among polymer families influences bacterial communities and dynamics (De Tender et al., 2015). Pinochet et al. (2020) observed that the bryozoan larvae of two invasive species of the genus Bugula showed preferences for colonizing PS and polycarbonate (PC) substrates within the polymer possibilities (PP, PVC, PET, and PC). Furthermore, antifouling treatments applied to different plastic materials, such as nylon fishing nets, could influence the community of organisms adhering to them (Núñez et al., 2006).
Although the reason for association with certain polymers is not clear, some authors have indicated that it might be due to the biofilm that develops on each polymer (Shin et al., 2013; Lagos et al., 2016; Morohoshi et al., 2018). According to Oberbeckmann et al. (2014) the composition of the polymer not only influences the abundance of microorganisms associated with polymers, but also shapes the structure of the biofilm community, which could play a role in the establishment of other species associated with the biofilm (Shin et al., 2013; Lagos et al., 2016; Morohoshi et al., 2018).
Macrobiota Transported by Plastics
Barnes (2002) highlighted the importance of marine debris as a distribution vector for marine species and estimated that it doubled the probability of transport of the species. After analyzing more than 200 pieces of debris from 30 different islands, he concluded that the most abundant groups were bryozoans, barnacles, polychaetes, hydroids and molluscs. Astudillo et al. (2009) found in the Southeastern Pacific 134 species in a total of 40 sampled buoys, mostly belonging to the Arthropoda, Annelida, and Mollusca phyla, 4 of them classified as invasive on the Chilean coast (Castilla et al., 2005): Ciona intestinalis (Linnaeus, 1767), Bugula neritina (Linnaeus, 1758), B. flabellata [Thompson in Gray, 1848; this name is currently not accepted and it is Bugulina flabellata (Thompson in Gray, 1848)] and the macroalgae Codium fragile (Suringar) Hariot, 1889 (frequencies of 73, 82, 59 and 9%, respectively). Later, in 2014, Goldstein et al. (2014) found 95 taxa in 242 pieces of plastic debris, most of them from the phylum Arthropoda, followed by Mollusca and Cnidaria. These data are consistent with the results obtained in our study (Table 1), as the phylum observed on plastic were Arthropoda (6), Bryozoa (4), Mollusca (4), Annelida (1) and Cnidaria (1), and the group Algae (1).
Some recurrent characteristics have been noted in the biology and ecology of species associated with plastic debris in the sea, such as cosmopolitan distributions, suspensivorous feeding (Astudillo et al., 2009) and sessile with short-lived larval development without natural potential means of dispersal (Barnes, 2002).
Kiessling et al. (2015) found 335 taxa associated with plastic garbage items in the ocean and stranded on the coast. In a study in the Atlantic Ocean, Barnes and Milner (2004) found several species of barnacles with a high incidence; the balanomorph Semibalanus balanoides (Linnaeus, 1767) were present in marine debris at all arctic and subarctic study sites; the invasive species Elminius modestus Darwin, 1854 [this name is currently not accepted and it is Austrominius modestus (Darwin, 1854)] was also found on plastic items in the Shetland Islands. The genus Lepas (one of the most common colonizers of plastic litter) (Miralles et al., 2018) shows a wide distribution associated with debris: from high latitudes in the Shetland Islands [Lepas (Anatifa) anatifera Linnaeus, 1758] to the Malvinas Islands [L. (Anatifa) australis Linnaeus, 1758], including locations closer to the equator such as the Ligurian Sea [L. (Anatifa) pectinata Spengler, 1793] (Aliani and Molcard, 2003). Other plastic colonizers include several species of hydroids and bryozoans (Aliani and Molcard, 2003; Barnes and Milner, 2004). The suspensivorous bivalve family Mytilidae form dense aggregations (Mikkelsen and Bieler, 2008) in specific vectors (e.g., marine debris, artificial substrates, ship hulls, and ballast water). This family includes invasive species carried by plastic debris such as Perna viridis (Linnaeus, 1758) (Gracia and Rangel-Buitrago, 2020).
Nikula et al. (2013) documented the transport of algae in debris, mostly plastic, between islands separated by more than 500 km. After a 30-year examination of the impact of the invasion of Undaria pinnatifida (Harvey) Suringar, 1873 in Australasia, South et al. (2017) indicated that its ability to settle and develop on any hard substrate until it reaches reproductive maturity, among those who frequented plastic products such as buoys, could be a key factor toin the initial success of its invasion. Recently, a study of the distribution and impact of Rugulopteryx okamurae in the Strait of Gibraltar also showed the highly competitive capacity of the algae to settle onto hard substrates, describing its ability to adhere to nets and ropes (made of nylon), and hooks of nets, constituting a problem for the fishing sector, and showing the potential of polyamides for the dispersal of species (García-Gómez et al., 2018).
Plastic as a Vector for the Dispersal of Microorganisms and Associated Diseases
Plastics, including MPs and NPs, adsorb organic and inorganic nutrients from water (Frère et al., 2018), which, along with its physical properties and widespread distribution provides a unique and stable habitat (Zettler et al., 2013; Oberbeckmann et al., 2015; Keswani et al., 2016), thus attracting bacteria, viruses, plankton, and other microorganisms which adhere to its surface (Frère et al., 2018), and enhancing their dispersion to different oceanic regions (Zettler et al., 2013; Oberbeckmann et al., 2015; Keswani et al., 2016). This adhesion is facilitated by the complexity of plastic surfaces, such as roughness and braiding (Núñez et al., 2006).
Zettler et al. (2013) introduced the term “plastisphere” to define a community of microorganisms associated with marine plastic debris found on the surface of seawater. “Plastisphere” differs from the bacterial populations found in other marine ecosystems, both in the water column and in other natural substrates (Zettler et al., 2013; Harrison et al., 2014; Dussud et al., 2018; Curren and Leong, 2019) and host a diverse community, including heterotrophs, autotrophs, predators, and symbionts, which generally begin with microbial colonization and biofilm conformation, which at the same time facilitate the settlement of other species, for example bryozoans (Bryant et al., 2016). Oberbeckmann et al. (2014) show that microbial communities in plastic change in structure and composition with respect to geographic location, season and type of polymer, but that there are also similarities between these plastic communities, such as the predominance of the phyla Proteobacteria and Bacteroidetes (Zettler et al., 2013; Oberbeckmann et al., 2014; Frère et al., 2018; Curren and Leong, 2019) and some microalgal species such as diatoms and dinoflagellates (Carson et al., 2013).
Different cases of dissemination of potentially toxic species have been documented, including pathogens and invasive algae, which can invade new habitats and modify their structure, becoming a threat to the ecosystem (Zettler et al., 2013; Kirstein et al., 2016). The toxic bacterial genus Vibrio has been commonly detected in MPs (Zettler et al., 2013; Frère et al., 2018; Curren and Leong, 2019); Kirstein et al. (2016) confirmed the presence of Vibrio spp. in 13% of all MP particles collected in the sea, identifying the potentially pathogenic species V. parahaemolyticus (Fujino et al., 1974) Sakazaki et al., 1963 and V. fluvialis Lee et al., 1981. Masó et al. (2003) detected members of the potentially harmful dinoflagellate genera Ostreopsis, Coolia, and Alexandrium in plastic debris floating in Mediterranean coastal waters. These infectious organisms can reach their hosts through the ingestion of plastic (Harrison et al., 2011; Zettler et al., 2013).
Several authors found antibiotic resistance genes (ARG; Miller et al., 2009; Laganà et al., 2019), metal resistance genes (MRG; Yang et al., 2019) and virulence genes (Radisic et al., 2020) in different species of bacteria in marine environments. Radisic et al. (2020) findings of virulence genes and new ARG variants in the fish pathogen Aeromonas salmonicida (Lehmann and Neumann, 1896; Griffin et al. 1953) isolated from plastic debris in Norway showed their potential for causing infections.
Audrézet et al. (2020) highlight the importance of the study of the succession of plastiphere communities and the different factors that influence the transmission of microorganisms mediated by plastic through the combination of molecular and microscopic approaches, and the use of genetic markers.
Therefore, there is concern that MP pollution, which is increasing in the marine environment, may cause serious marine ecological effects, influence the dynamics of its population and, ultimately, the emergence of pathogens (Frère et al., 2018; Shen et al., 2019). The introduction of MPs colonized by non-native microbial communities is likely to alter microbial communities and genetic exchange in natural water and consequently affect the ecological function of microbial communities (Miao et al., 2019).
Spatiotemporal Dispersion Patterns of Plastic Debris in the Marine Environment and Vulnerable Areas
Plastic horizontal dispersion in the ocean is driven by different large-scale processes, such as the action of ocean currents, wind, tides (Figure 4; Law et al., 2010; Kim et al., 2015) and extreme meteorological events, such as hurricanes (Wang et al., 2019; Lo et al., 2020) and tsunamis (Wang et al., 2016). Sea state, wind (Astudillo et al., 2009; Thiel et al., 2011), and particle size and type (Reisser et al., 2015) influence the duration of transport. A Plastic particle from the east coast of the United States may reach the subtropical gyre of the Atlantic North in less than 60 days (Law et al., 2010). Six years after the 2011 tsunami in Japan, Carlton et al. (2017) documented 289 living species on the coasts of North America and Hawaii in marine debris originating after the catastrophe, among which plastic debris was abundant. On the ocean surface, downwind and slow current habitats are potential sinks for plastic debris (Browne et al., 2010). Currently, 5 ocean gyres have been identified, located in the North Atlantic, South Atlantic, South Indian, South Pacific, and North Pacific (Eriksen et al., 2014), which accumulate on their surfaces at least 79 thousand tons of plastic (Lebreton et al., 2018). Moreover, the appearance of another patch in the Barents Sea has been predicted (van Sebille et al., 2012). These areas can accommodate quantities of up to 21,290 tons of plastic in the North Pacific gyre (Law et al., 2010). In addition, in the convergence regions, surface water is pumped down to depths of a few hundred meters (van Sebille et al., 2020).
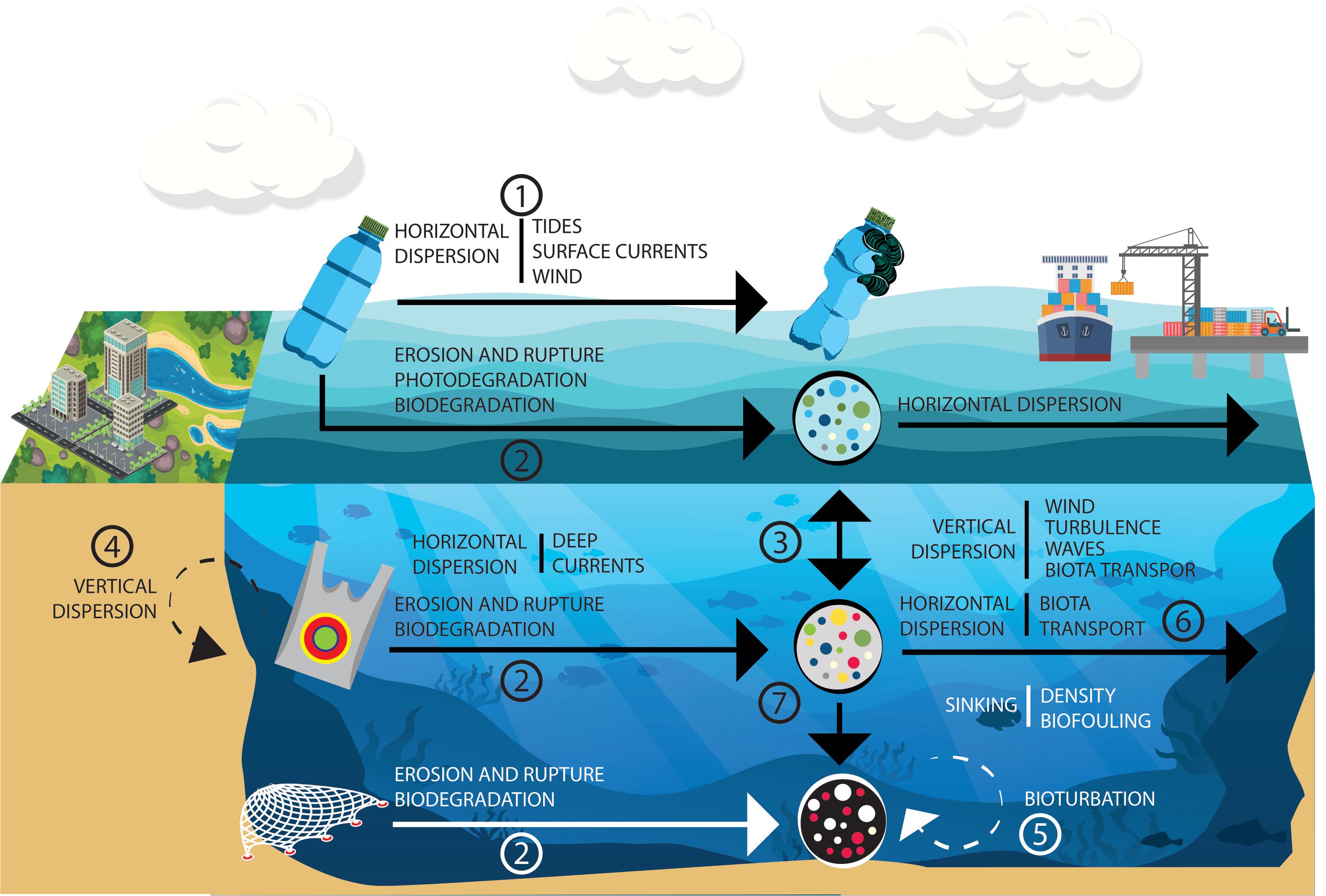
Figure 4. Debris made of high-density polyethylene (HDPE), such as plastic bottles which could be transported through large areas and be vectors for potentially invasive marine species (1) or result in micro and nanoplastic material through physical degradation, photodegradation, or biodegradation (2). Plastic bags composed of low-density polyethylene (LDPE) which usually settle in the water column, while plastic fishing nets, in many cases constituted by nylon, tend to sink and settle on the seabed. Both plastic bags and fishing nets are susceptible to physical degradation and biodegradation. Plastic particles which could succumb to bioturbation (5), describe vertical movements (3,4) and be simultaneously ingested by animals from all oceanic stratum being dispersed by them (6), as well as sink and stay at the seafloor due to their original density or to increase by processes such as biofouling (7). Steps 1, 2, and 3 of “Horizontal Dispersion,” which are the most frequently involved in biological invasions. To a lesser degree, steps 3 and 4 involve “Vertical Dispersion.”
The vertical transport of plastic is both size- and density-dependent. MPs are more abundant than larger plastic debris, both on the sea’s surface and in the water column (Kooi et al., 2017). On the other hand, plastic materials with a density lower than water (LD) (1.02 g/cm3) are usually found on the surface and in neustonic environment (Moore et al., 2011), while those with a higher density (HD) reach the marine benthic environments (Moret-Ferguson et al., 2010; Ballent et al., 2012).
During plastic debris stay in the marine environment, their density can change over time due to the physical/chemical/biological degradation or biofouling attachment Figure 4) (Moret-Ferguson et al., 2010) of suspended matter, contamination by epiphytes or the formation of microbial biofilms (Lobelle and Cunliffe, 2011; Collignon et al., 2014; Bagaev et al., 2017). Increased density could cause the debris to sink, to be transported by underlying currents (Engler, 2012), trapped by turbulent currents of the benthic boundary layer, resuspended by deep currents, or finally to settle onto the seafloor (Bagaev et al., 2017; Figure 4). In many cases, sedimentation is facilitated by oceanographic processes (Wang et al., 2016) such as dense shelf water cascading (Canals et al., 2006), severe coastal storms (Sanchez-Vidal et al., 2012), offshore convection (Durrieu de Madron et al., 2013), and saline subduction (Talley, 2002). Predicting this vertical mixing could be essential, as it affects the horizontal drifting patterns and ecological impacts of plastic pollution (Reisser et al., 2015). Plastic concentrations have been shown to decrease exponentially with depth (Reisser et al., 2015). However, Woodall et al. (2014) reported an abundance of MPs on the seafloor four orders of magnitude greater than in surface water gyres, while Peng et al. (2018) reported abundant MP particles in the Mariana Trench, the deepest part of the world’s ocean.
Plastic debris is widely distributed throughout our oceans and colonize from latitudes near the equator to the poles (Obbard et al., 2014), with the tropical regions being the areas where it is most frequent and predominant (Barboza et al., 2019). Regardless of the geographical region, the most vulnerable areas with respect to the colonization of exotic species transported by this debris are those where endemisms abound and endangered species are present (Gregory, 2009; Thevenon et al., 2014).
Therefore, given the spatial “cosmopolitanism” of plastic materials and their increasing abundance in the marine environment, generalist invasive species (or with invasive potential) in the surface waters of all oceans which can be transported by this vector, constitute an increasing threat—within the bathymetric range to which they are adaptive—especially to pristine and highly biodiverse ecosystems, with particular relevance to Marine Protected Areas.
Early Detection and Surveillance of AIS in Marine Plastic Debris
Rech et al. (2018a) found that the frequency of a specific taxon attached to plastic litter in a coastal area can be predicted based on the characteristics of biological communities associated with each litter material and the composition of beach litter. This approach, after being tested in other regions, may contribute as a simple and cost-effective tool for risk assessment in the future (Rech et al., 2018a). On the other hand, Fazey and Ryan (2016a,b) showed that small samples of plastic litter lost buoyancy due to biofouling much faster than larger ones, providing the first estimates of the longevity of different sizes of plastic debris at the surface of the ocean. This finding could be used to improve model predictions of the distribution and abundance of floating plastic debris globally.
Ports are often export areas for native generalist species and entry areas for alien species (Mineur et al., 2006; Keller et al., 2010; Airoldi et al., 2015; López-Legentil et al., 2015; Ferrario et al., 2017). A sport or recreational vessel whose hull is made of fiberglass-reinforced polyester can import or export native and alien species. But also, by accumulation and subsequent sinking, ports and marinas can import and export plastic trash with alien species. In many cases the plastic sinks (especially bags), because of the weight of the biofouling, remain at shallow depths (especially in ports and marinas, which tend to accumulate plastic garbage on their bottoms). For their control and environmental monitoring, a modification of the SBPQ (Sessile Bioindicators Permanent Quadrats) method could be applied, as recently proposed by García-Gómez (2015) and García-Gómez et al. (2020a) for the early detection of alien species and environmental impacts of a local nature (e.g., urban discharges) or global (climate change) in rocky natural habitats. It is a non-invasive method focuses on the monitoring of preselected sensitive (indicators) sessile target species associated with rocky coralligenous habitats using permanent quadrats in underwater sentinel stations. It could be adapted to plastic panels (completed with other types of non-plastic panels) which are susceptible to colonization by opportunistic sessile species that could become invasive, and act as “traps” for the early detection of alien species. In this regard, the installation of underwater sentinel stations should be tested at various inland points of ports, with plastic panels of at least five panels per point, of 25 cm × 25 cm, with different roughness and nature (e.g., polyamide or nylon, polyester, polyethylene, high density polyethylene and polypropylene), which serve to recognize the species which establish easily on this type of material and those which are more frequent and with a structural and adaptive profile of higher risk for invasion. This method could contribute to the early detection of alien species with invasive potential, and to the implementation of immediate mitigation and/or eradication measures.
Additional Considerations
From the foregoing, it can be deduced that plastic debris represents a ubiquitous vector with great potential for transporting both sessile and mobile species associated with it, capable of traveling long distances because plastic, due to its composition, is not biodegradable and, therefore, very durable over time.
We could ask ourselves which species of those transported by plastic (or that could be transported by this type of substrata) may have a greater risk of invasion. They would be sessile generalist arborescent species (e.g., seaweeds, hydrozoans, bryozoans) that, according to Bradshaw et al. (2003), are common components of fouling communities. So, they can provide food, shelter or hiding conditions for other mobile species that can travel with them (both non-native and native). About this, Marchini et al. (2015a) reported three mobile NIS associated with the introduced sessile species Amathia verticillata (= Zoobotrion verticillatum) (Bryozoa) and suggested this species as substrate for transport between ports, facilitating its distribution. Also, Gavira-O’Neill et al. (2018) found 19 species associated with the invasive bryozoan Tricellaria inopinata—in list of “100 Worst Invasives” in the Mediterranean (Streftaris and Zenetos, 2006)—between them the three mobile introduced species Caprella scaura, Monocorophium sextonae (Amphipoda), and the Paracerceis sculpta, adding that these species represented over half of the quantified individuals and discussing the possibility of a potential case of “invasional meltdown”—expression by Simberloff and Von Holle (1999)—during which introduced species provide suitable habitat for other non-native species, favoring their establishment. Other studies also support this hypothesis for T. inopinata as a host for other mobile species introduced from other zoological groups, such as isopods and nudibranchs (Keppel et al., 2012; Hobbs et al., 2015). So, such arborescent sessile species (hosts of mobile fauna) are those that need to occupy the substrate surfaces of the bottom (even as epibionts) and, therefore, those that can generate the greatest environmental impact on the native sessile biota.
In order to improve biosecurity, the best mechanism is prevention and, in this sense, it is important to start acting against this ongoing problem; for example, through protocols for the sighting (from small boats and large ships) of accumulations of plastic adrift within 20 miles off the coasts, where the presence of accumulations of floating with well-established biofouling is detected. In the same way, ports and marinas must be involved in environmental surveillance for the early detection of alien species before they can become widespread.
Actions to manage the problem should be put into place, such as the collection of floating plastic by cleaning boats employed in coastal areas at risk of the entry of plastic accumulations due to winds and/or currents. International regulations or legal provisions must be implemented in this regard. Collaboration on the part of society must also be encouraged. Environmental education and the emerging “Citizen Science” movement (Wiggins and Crowston, 2011) should be stimulated and coordinated from public administrations, as well as large industries, companies or institutions that have large coastal infrastructures. In addition, large industries and companies should also participate in mitigating the problem under the influence of the emerging philosophy of “Working with nature” (PIANC, 2014; Martin et al., 2017; Nebot et al., 2017). which has generated an awareness of respect for nature, by which it is intended to act with it and not against it, collaborating in environmental monitoring and surveillance studies of threatened species naturally established in port breakwaters (García-Gómez et al., 2010, 2014) and, in the present case, for the early detection of alien species with invasive potential.
Conclusion
1. The number of articles published of plastic debris as a vector for the introduction of alien species has increased enormously in recent years. This increase could be related to the increase in annual plastic production, which results in a greater threat, in addition to a growing interest in the problem on the part of the scientific community and, therefore, the greater number of research papers related to it.
2. Several of the biological characteristics of marine species commonly associated with plastic, such as the short life cycle and larval development, are also characteristics of a large portion of the known invasive species; so these species that travel on plastic debris across the ocean could generally be perceived as a major threat to their destination.
3. A wide variety of organisms colonize plastic materials, both microorganisms (e.g., species of the genus Vibrio or different species carrying virulent and antibiotic resistance genes) and macrofauna species (e.g., algae or bryozoan species). This fact increases the threat to ocean life caused by plastic and turns it into a means for spreading disease.
4. There are large gaps in knowledge about the functioning of plastic objects as vectors and the lack of studies on colonization processes on different plastic polymers by marine species generate contradictions between different authors. Despite the great advances produced today in the knowledge of plastic debris in the ocean, greater research are necessary to mitigate the threat of biological invasions linked to this type of pollutant.
Author Contributions
MG and JG made Figures 1, 2, and 4. JG-G provided the photographs of Figure 3. All authors decided the consensus on the structure of the manuscript and accomplished the literature search, and participated for the conclusion and formal aspects.
Conflict of Interest
The authors declare that the research was conducted in the absence of any commercial or financial relationships that could be construed as a potential conflict of interest.
Acknowledgments
We are deeply grateful to the CEPSA Foundation and Red Eléctrica from Spain (REE) for its initiative to support and finance scientific research. Secondly, we are grateful to OBIMASA, ACERINOX, ENDESA, OPP-51 (Almadrabas) and Diputación de Cádiz. Additionally, this work has benefitted from the scientific projects financed by the Port Authority of Seville. We express our gratitude for the collaboration provided to the Seville Aquarium, the Town Halls of Tarifa and La Línea as well as the Puerto Deportivo La Alcaidesa, Club Marítimo and Real Club Náutico (RCN) de La Línea. From the Marine Biology Station of the Strait (Ceuta) we would also like to thank Watergames Gran Azul diving club, Marina Hércules S.A. and Consejería de Medio Ambiente y Sostenibilidad, Autonomous City of Ceuta.
References
Airoldi, L., Turon, X., Perkol-Finkel, S., and Rius, M. (2015). Corridors for aliens but not for natives: effects of marine urban sprawl at a regional scale. Diver. Distrib. 21, 755–768. doi: 10.1111/ddi.12301
Aliani, S., and Molcard, A. (2003). “Hitch-hiking on floating marine debris: macrobenthic species in the Western Mediterranean Sea,” in Migrations And Dispersal Of Marine Organisms, eds A. Ingólfsson, E. Ólafsson, G. V. Helgason, J. Svavarsson, K. Gunnarsson, and M. B. Jones (Dordrecht: Springer Netherlands), 59–67. doi: 10.1023/b:hydr.0000008480.95045.26
Alomar, C., Estarellas, F., and Deudero, S. (2016). Microplastics in the Mediterranean Sea: deposition in coastal shallow sediments, spatial variation and preferential grain size. Mar. Environ. Res. 115, 1–10. doi: 10.1016/j.marenvres.2016.01.005
Andrady, A., and Neal, M. (2009). Applications and societal benefits of plastics. Philos. Trans. R. Soc. B Biol. Sci. 364, 1977–1984. doi: 10.1098/rstb.2008.0304
Anil, A., Venkat, K., Sawant, S., Dileepkumar, M., Dhargalkar, V., Ramaiah, N., et al. (2002). Marine bioinvasion: concern for ecology and shipping. Curr. Sci. 83, 214–218.
Astudillo, J., Bravo, M., Dumont, C., and Thiel, M. (2009). Detached aquaculture buoys in the SE Pacific: potential dispersal vehicles for associated organisms. Aquat. Biol. 5, 219–231. doi: 10.3354/ab00151
Audrézet, F., Zaiko, A., Lear, G., Wood, S., Tremblay, L., and Pochon, X. (2020). Biosecurity implications of drifting marine plastic debris: current knowledge and future research. Mar. Pollut. Bull. 162:111835. doi: 10.1016/j.marpolbul.2020.111835
Avio, C., Gorbi, S., and Regoli, F. (2017). Plastics and microplastics in the oceans: from emerging pollutants to emerged threat. Mar. Environ. Res. 128, 2–11. doi: 10.1016/j.marenvres.2016.05.012
Bagaev, A., Mizyuk, A., Khatmullina, L., Isachenko, I., and Chubarenko, I. (2017). Anthropogenic fibres in the Baltic Sea water column: field data, laboratory and numerical testing of their motion. Sci. Total Environ. 59, 560–571. doi: 10.1016/j.scitotenv.2017.04.185
Ballent, A., Purser, A., de Jesus Mendes, P., Pando, S., and Thomsen, L. (2012). Physical transport properties of marine microplastic pollution. Biogeosci. Discuss. 9, 18755–18798.
Barboza, L., Cózar, A., Gimenez, B., Barros, T., Kershaw, P., and Guilhermino, L. (2019). “Macroplastics pollution in the marine environment,” in World Seas: An Environmental Evaluation. Volume 3: Ecological Issues and Environmental Impacts, ed. C. Sheppard (Coventry: University of Warwick), 305–328. doi: 10.1016/b978-0-12-805052-1.00019-x
Barnes, D. (2002). Invasions by marine life on plastic debris. Nature 416, 808–809. doi: 10.1038/416808a
Barnes, D., and Milner, P. (2004). Drifting plastic and its consequences for sessile organism dispersal in the Atlantic Ocean. Mar. Biol. 146, 815–825. doi: 10.1007/s00227-004-1474-8
Bertness, M. (1984). Habitat and community modification by an introduced herbivorous snail. Ecology 65, 370–381. doi: 10.2307/1941400
Bonanno, G., and Orlando-Bonaca, M. (2019). Non-indigenous marine species in the Mediterranean Sea—Myth and reality. Environ. Sci. Policy 96, 123–131. doi: 10.1016/j.envsci.2019.03.014
Bradshaw, C., Collins, P., and Brand, A. (2003). To what extent does upright sessile epifauna affect benthic biodiversity and community composition? Mar. Biol. 143, 783–791. doi: 10.1007/s00227-003-1115-7
Braga, A., Lage, S., Pacheco, M., Rydberg, S., and Costa, P. (2017). Native (Ruditapes decussatus) and non-indigenous (R. philippinarum) shellfish species living in sympatry: comparison of regulated and non-regulated biotoxins accumulation. Mar. Environ. Res. 129, 147–155. doi: 10.1016/j.marenvres.2017.05.002
Brine, O., Hunt, L., and Costello, M. (2013). Marine biofouling on recreational boats on swing moorings and berths. Manag. Biol. Invasions 4, 327–341. doi: 10.3391/mbi.2013.4.4.07
Browne, M., Galloway, T., and Thompson, R. (2010). Spatial patterns of plastic debris along estuarine shorelines. Environ. Sci. Technol. 44, 3404–3409. doi: 10.1021/es903784e
Bryan, S., Cook, A., Evans, J., Colls, P., Wells, M., Lawrence, M., et al. (2004). Pumice rafting and faunal dispersion during 2001–2002 in the Southwest Pacific: record of a dacitic submarine explosive eruption from Tonga. Earth Planetary Sci. Lett. 227, 135–154. doi: 10.1016/j.epsl.2004.08.009
Bryant, J., Clemente, T., Viviani, D., Fong, A., Thomas, K., Kemp, P., et al. (2016). Diversity and activity of communities inhabiting plastic debris in the north pacific gyre. Msystems 1, e00024–16. doi: 10.1128/msystems.00024-16
Campbell, M., King, S., Heppenstall, L., van Gool, E., Martin, R., and Hewitt, C. (2017). Aquaculture and urban marine structures facilitate native and non-indigenous species transfer through generation and accumulation of marine debris. Mar. Pollut. Bull. 123, 304–312. doi: 10.1016/j.marpolbul.2017.08.040
Canals, M., Puig, P., de Madron, X., Heussner, S., Palanques, A., and Fabres, J. (2006). Flushing submarine canyons. Nature 444, 354–357. doi: 10.1038/nature05271
Carlton, J. (1987). Patterns of transoceanic marine biological invasions in the Pacific Ocean. Bull. Mar. Sci. 41, 452–465.
Carlton, J. (1996). Pattern, process, and prediction in marine invasion ecology. Biol. Conserv. 78, 97–106. doi: 10.1016/0006-3207(96)00020-1
Carlton, J., Chapman, J., Geller, J., Miller, J., Carlton, D., McCuller, M., et al. (2017). Tsunami-driven rafting: transoceanic species dispersal and implications for marine biogeography. Science 357, 1402–1406. doi: 10.1126/science.aao1498
Carson, H., Nerheim, M., Carroll, K., and Eriksen, M. (2013). The plastic-associated microorganisms of the North Pacific Gyre. Mar. Pollut. Bull. 75, 126–132. doi: 10.1016/j.marpolbul.2013.07.054
Castilla, J., Uribe, M., Bahamonde, N., Clarke, M., Desqueyroux-Faúndez, R., Kong, I., et al. (2005). Down under the southeastern Pacific: marine non-indigenous species in Chile. Biol. Invasions 7, 213–232. doi: 10.1007/s10530-004-0198-5
Castilla, J. C., and Neill, P. E. (2009). “Marine bioinvasions in the southeastern pacific: status, ecology, economic impacts, conservation and management,” in Biological Invasions in Marine Ecosystems. Ecological Studies (Analysis and Synthesis), Vol. 204, eds G. Rilov and J. A. Crooks (Heidelberg, BE: Springer). doi: 10.1353/psc.2002.0016
Chainho, P., Fernandes, A., Amorim, A., Ávila, S., Canning-Clode, J., Castro, J., et al. (2015). Non-indigenous species in Portuguese coastal areas, coastal lagoons, estuaries and islands. Estuarine Coastal Shelf Sci. 167, 199–211. doi: 10.1016/j.ecss.2015.06.019
Clarke Murray, C., Gartner, H., Gregr, E., Chan, K., Pakhomov, E., and Therriault, T. (2014). Spatial distribution of marine invasive species: environmental, demographic and vector drivers. Diver. Distrib. 20, 824–836. doi: 10.1111/ddi.12215
Collignon, A., Hecq, J., Galgani, F., Collard, F., and Goffart, A. (2014). Annual variation in neustonic micro- and meso-plastic particles and zooplankton in the Bay of Calvi (Mediterranean–Corsica). Mar. Pollut. Bull. 79, 293–298. doi: 10.1016/j.marpolbul.2013.11.023
Cook, E., Jahnke, M., Kerckhof, F., Minchin, D., Faasse, M., Boos, K., et al. (2007). European expansion of the introduced amphipod Caprella mutica Schurin, 1935. Aquat. Invasions 2, 411–421. doi: 10.3391/ai.2007.2.4.11
Creed, J., Junqueira, A., Fleury, B., Mantelatto, M., and Oigman-Pszczol, S. (2017). The Sun-Coral Project: the first social-environmental initiative to manage the biological invasion of Tubastraea spp. in Brazil. Manag. Biol. Invasions 8, 181–195. doi: 10.3391/mbi.2017.8.2.06
Crooks, J. (2002). Characterizing ecosystem-level consequences of biological invasions: the role of ecosystem engineers. Oikos 97, 153–166. doi: 10.1034/j.1600-0706.2002.970201.x
Curren, E., and Leong, S. (2019). Profiles of bacterial assemblages from microplastics of tropical coastal environments. Sci. Total Environ. 655, 313–320. doi: 10.1016/j.scitotenv.2018.11.250
Davis, M., and Davis, M. (2007). The distribution of styela clava (Tunicata, Ascidiacea) in European waters. J. Exp. Mar. Biol. Ecol. 342, 182–184. doi: 10.1016/j.jembe.2006.10.039
De Tender, C., Devriese, L., Haegeman, A., Maes, S., Ruttink, T., and Dawyndt, P. (2015). Bacterial Community Profiling of Plastic Litter in the Belgian Part of the North Sea. Environ. Sci. Technol. 49, 9629–9638. doi: 10.1021/acs.est.5b01093
Debroas, D., Mone, A., and Ter Halle, A. (2017). Plastics in the North Atlantic garbage patch: a boat-microbe for hitchhikers and plastic degraders. Sci. Total Environ. 59, 1222–1232. doi: 10.1016/j.scitotenv.2017.05.059
Derraik, J. (2002). The pollution of the marine environment by plastic debris: a review. Mar. Pollut. Bull. 44, 842–852. doi: 10.1016/s0025-326x(02)00220-5
do Amaral, V., Simone, L., de Souza Tâmega, F., Barbieri, E., Calazans, S., Coutinho, R., et al. (2020). New records of the non-indigenous oyster Saccostrea cucullata (Bivalvia: Ostreidae) from the southeast and south Brazilian coast. Reg. Stud. Mar. Sci. 33:100924. doi: 10.1016/j.rsma.2019.100924
Durrieu de Madron, X., Houpert, L., Puig, P., Sanchez-Vidal, A., Testor, P., Bosse, A., et al. (2013). Interaction of dense shelf water cascading and open-sea convection in the northwestern Mediterranean during winter 2012. Geophys. Res. Lett. 40, 1379–1385. doi: 10.1002/grl.50331
Dussud, C., Meistertzheim, A., Conan, P., Pujo-Pay, M., George, M., Fabre, P., et al. (2018). Evidence of niche partitioning among bacteria living on plastics, organic particles and surrounding seawaters. Environ. Pollut. 236, 807–816. doi: 10.1016/j.envpol.2017.12.027
Ebbesmeyer, C., and Ingraham, W. (1992). Shoe spill in the North Pacific. Eos Trans. Am. Geophys. Union 73, 361–361. doi: 10.1029/91eo10273
Eerkes-Medrano, D., Thompson, R., and Aldridge, D. (2015). Microplastics in freshwater systems: a review of the emerging threats, identification of knowledge gaps and prioritisation of research needs. Water Res. 75, 63–82. doi: 10.1016/j.watres.2015.02.012
Engler, R. (2012). The complex interaction between marine debris and Toxic chemicals in the Ocean. Environ. Sci. Technol. 46, 12302–12315. doi: 10.1021/es3027105
Eno, N. (1996). Non-native marine species in British waters: effects and controls. Aquat. Conserv. Mar. Freshw. Ecosyst. 6, 215–228. doi: 10.1002/(sici)1099-0755(199612)6:4<215::aid-aqc191>3.0.co;2-q
Eno, N. C., Clark, R. A., and Sanderson, W. G. (1997). Non-Native Marine Species in British Waters: A Review and Directory. Peterborough: JNCC.
Epstein, G., and Smale, D. (2017). Undaria pinnatifida: a case study to highlight challenges in marine invasion ecology and management. Ecol. Evol. 7, 8624–8642. doi: 10.1002/ece3.3430
Eriksen, M., Lebreton, L., Carson, H., Thiel, M., Moore, C., Borerro, J., et al. (2014). Plastic pollution in the world’s oceans: more than 5 trillion plastic pieces weighing over 250,000 Tons Afloat at Sea. PLoS One 9:e111913. doi: 10.1371/journal.pone.0111913
Fazey, F., and Ryan, P. (2016a). Biofouling on buoyant marine plastics: an experimental study into the effect of size on surface longevity. Environ. Pollut. 210, 354–360. doi: 10.1016/j.envpol.2016.01.026
Fazey, F., and Ryan, P. (2016b). Debris size and buoyancy influence the dispersal distance of stranded litter. Mar. Pollut. Bull. 110, 371–377. doi: 10.1016/j.marpolbul.2016.06.039
Ferrario, J., Caronni, S., Anna Occhipinti-Ambrogi, A., and Marchini, A. (2017). Role of commercial harbours and recreational marinas in the spread of non-indigenous fouling species. Biofouling 33 (N8), 651–660. doi: 10.1080/08927014.2017.1351958
Fine, M., Zibrowius, H., and Loya, Y. (2001). Oculina patagonica: a non-lessepsian scleractinian coral invading the Mediterranean Sea. Mar. Biol. 138, 1195–1203. doi: 10.1007/s002270100539
Fraser, C., Morrison, A., Hogg, A., Macaya, E., van Sebille, E., Ryan, P., et al. (2018). Antarctica’s ecological isolation will be broken by storm-driven dispersal and warming. Nat. Climate Change 8, 704–708. doi: 10.1038/s41558-018-0209-7
Frère, L., Maignien, L., Chalopin, M., Huvet, A., Rinnert, E., Morrison, H., et al. (2018). Microplastic bacterial communities in the Bay of Brest: influence of polymer type and size. Environ. Pollut. 242, 614–625. doi: 10.1016/j.envpol.2018.07.023
Fujino, T., Sakazaki, R., and Tamura, K. (1974). Designation of the type strain of vibrio parahaemolyticus and Description of 200 strains of the species. Int. J. Syst. Bacteriol. 24, 447–449. doi: 10.1099/00207713-24-4-447
Galgani, L., Beiras, R., Galgani, F., Panti, C., and Borja, A. (2019). Editorial: impacts of marine litter. Front. Mar. Sci. 6:208. doi: 10.3389/fmars.2019.00208
García-Gómez, J. (2015). A Guide on Environmental Monitoring of Rocky Seabeds in Mediterranean Marine Protected Areas and Surrounding Zones. Tunis: RAC/SPA-MedMPAnet Project, 1–491.
García-Gómez, J., González, A., Maestre, M., and Espinosa, F. (2020a). Detect coastal disturbances and climate change effects in coralligenous community through sentinel stations. PLoS One 15:e0231641. doi: 10.1371/journal
García-Gómez, J., Guerra-García, J., Espinosa, F., Maestre, M., Rivera-Ingraham, G., Fa, D., et al. (2014). Artificial Marine Micro-Reserves Networks (AMMRNs): an innovative approach to conserve marine littoral biodiversity and protect endangered species. Mar. Ecol. 36, 259–277. doi: 10.1111/maec.12167
García-Gómez, J., López-Fé, C., Espinosa, F., Guerra-García, J., and Rivera-Ingraham, G. (2010). Marine artificial micro-reserves: a possibility for the conservation of endangered species living on artificial substrata. Mar. Ecol. 32, 6–14. doi: 10.1111/j.1439-0485.2010.00409.x
García-Gómez, J., Sempere-Valverde, J., González, A., Martínez-Chacón, M., Olaya-Ponzone, L., Sánchez-Moyano, E., et al. (2020b). De exótico a invasivo en tiempo récord: el impacto extremo de Rugulopteryx okamurae (Dictyotales, Ochrophyta) en el estrecho de Gibraltar. Sci. Total Environ. 704:135408.
García-Gómez, J., Sempere-Valverde, J., Ostalé-Valriberas, E., Martínez, M., Olaya-Ponzone, L., González, A., et al. (2018). Rugulopteryx okamurae (E.Y. Dawson) I.K. Hwang, W.J. Lee & H.S. Kim (Dictyotales, Ochrophyta), alga exótica “explosiva” en el estrecho de Gibraltar. Observaciones preliminares de su distribución e impacto. Almoraima 48, 97–113.
Garcia-Vazquez, E., Cani, A., Diem, A., Ferreira, C., Geldhof, R., Marquez, L., et al. (2018). Leave no traces – Beached marine litter shelters both invasive and native species. Mar. Poll. Pollut. Bull. 131, 314–322. doi: 10.1016/j.marpolbul.2018.04.037
Gartner, H., Murray, C., Frey, M., Nelson, J., Larson, K., Ruiz, G., et al. (2016). Non-indigenous invertebrate species in the marine fouling communities of British Columbia, Canada. Bioinvasions Rec. 5, 205–212. doi: 10.3391/bir.2016.5.4.03
Gavira-O’Neill, K., Guerra-García, J., Moreira, J., and Ros, M. (2018). Mobile epifauna of the invasive bryozoan Tricellaria inopinata: is there a potential invasional meltdown? Mar. Biodivers. 48, 1169–1178. doi: 10.1007/s12526-016-0563-5
Gil, M., and Pfaller, J. (2016). Oceanic barnacles act as foundation species on plastic debris: implications for marine dispersal. Sci. Rep. 6:19987. doi: 10.1038/srep19987
Glasby, T., Connell, S., Holloway, M., and Hewitt, C. (2007). Nonindigenous biota on artificial structures: could habitat creation facilitate biological invasions? Mar. Biol. 151, 887–895. doi: 10.1007/s00227-006-0552-5
Godwin, L. (2003). Hull fouling of maritime vessels as a pathway for marine species invasions to the Hawaiian Islands. Biofouling 19(sup1), 123–131. doi: 10.1080/0892701031000061750
Goldstein, M., Carson, H., and Eriksen, M. (2014). Relationship of diversity and habitat area in North Pacific plastic-associated rafting communities. Mar. Biol. 161, 1441–1453. doi: 10.1007/s00227-014-2432-8
Gollasch, S. (2002). The importance of ship hull fouling as a vector of species introductions into the North Sea. Biofouling 18, 105–121. doi: 10.1080/08927010290011361
Gracia, C. A., and Rangel-Buitrago, N. (2020). The invasive species Perna viridis (Linnaeus, 1758 - Bivalvia: Mytilidae) on artificial substrates: a baseline assessment for the Colombian Caribbean Sea. Mar. Pollut. Bull. 152:110926. doi: 10.1016/j.marpolbul.2020.110926
Gregory, M. (1999). Plastics and South Pacific Island shores: environmental implications. Ocean Coastal Manag. 42, 603–615. doi: 10.1016/s0964-5691(99)00036-8
Gregory, M. (2009). Environmental implications of plastic debris in marine settings—entanglement, ingestion, smothering, hangers-on, hitch-hiking and alien invasions. Philos. Trans. R. Soc. B Biol. Sci. 364, 2013–2025. doi: 10.1098/rstb.2008.0265
Grosholz, E., Crafton, R., Fontana, R., Pasari, J., Williams, S., and Zabin, C. (2015). Aquaculture as a vector for marine invasions in California. Biol. Invasions 17, 1471–1484. doi: 10.1007/s10530-014-0808-9
Grosholz, E., and Ruiz, G. (1995). Spread and potential impact of the recently introduced European green crab, Carcinus maenas, in central California. Mar. Biol. 122, 239–247.
Gündoǧdu, S. (2017). Fouling assemblage of benthic plastic debris collected from Mersin Bay, NE Levantine coast of Turkey. Mar. Pollut. Bull. 124, 147–154. doi: 10.1016/j.marpolbul.2017.07.023
Harrison, J., Sapp, M., Schratzberger, M., and Osborn, A. (2011). Interactions between microorganisms and marine microplastics: a call for research. Mar. Technol. Soc. J. 45, 12–20. doi: 10.4031/mtsj.45.2.2
Harrison, J., Schratzberger, M., Sapp, M., and Osborn, A. (2014). Rapid bacterial colonization of low-density polyethylene microplastics in coastal sediment microcosms. BMC Microbiol. 14:232. doi: 10.1186/s12866-014-0232-4
Hebert, P., Wilson, C., Murdoch, M., and Lazar, R. (1991). Demography and ecological impacts of the invading mollusc Dreissena polymorpha. Can. J. Zool. 69, 405–409. doi: 10.1139/z91-063
Hernández-Delgado, E., Toledo-Hernández, C., Ruíz-Díaz, C., Gómez-Andújar, N., Medina-Muñiz, J., Canals-Silander, M., et al. (2020). Hurricane impacts and the resilience of the invasive Sea Vine, Halophila stipulacea: a case study from Puerto Rico. Estuaries Coast. 43, 1263–1283. doi: 10.1007/s12237-019-00673-4
Hobbs, N.-V., Lazo-Wasem, E., Faasse, M., Cordell, J., Chapman, J., Smith, C., et al. (2015). Going global: the introduction of the Asian isopod Laniropsis serricaudis Gurjanova (Crustacea: Peracarida) to North America and Europe. Aquat. Invasions 10, 177–187. doi: 10.3391/ai.2015.10.2.06
Holmes, M., Kotta, J., Persson, A., and Sahlin, U. (2019). Marine protected areas modulate habitat suitability of the invasive round goby (Neogobius melanostomus) in the Baltic Sea. Estuarine Coast. Shelf Sci. 229:106380. doi: 10.1016/j.ecss.2019.106380
Horta, P., and Oliveira, E. (2000). Morphology and reproduction of Anotrichium yagii (Ceramiales, Rhodophyta) – a new invader seaweed in the American Atlantic? Phycologia 39, 390–394. doi: 10.2216/i0031-8884-39-5-390.1
Huang, Z. G. (1994). Marine Species and Their Distributions in China’s Seas. Beijing: China Ocean Press.
Ibabe, A., Rayón, F., Martinez, J., and Garcia-Vazquez, E. (2020). Environmental DNA from plastic and textile marine litter detects exotic and nuisance species nearby ports. PLoS One 15:e0228811. doi: 10.1371/journal.pone.0228811
Katsanevakis, S., Wallentinus, I., Zenetos, A., Leppäkoski, E., Çinar, M., Oztürk, B., et al. (2014). Impacts of invasive alien marine species on ecosystem services and biodiversity: a pan-European review. Aquat. Invasions 9, 391–423. doi: 10.3391/ai.2014.9.4.01
Kauano, R., Roper, J., and Rocha, R. (2016). Small boats as vectors of marine invasion: experimental test of velocity and desiccation as limits. Mar. Biol. 164:27. doi: 10.1007/s00227-016-3057-x
Keller, R., Drake, J., and Drew, J. (2010). Linking environmental conditions and ship movements to estimate invasive species transport across the global shipping network. Diver. Distrib. 17, 93–102. doi: 10.1111/j.1472-4642.2010.00696.x
Keppel, E., Sigovini, M., and Tagliapietra, D. (2012). A new geographical record of Polycera hedgpethi Er. Marcus, 1964 (Nudibranchia: Polyceridae) and evidence of its established presence in the Mediterranean Sea, with a review of its geographical distribution. Mar. Biol. Res. 8, 969–981. doi: 10.1080/17451000.2012.706306
Kerckhof, F., Haelters, J., and Degraer, S. (2010). The barnaclesChirona (Striatobalanus) amaryllis (Darwin 1854) andMegabalanus coccopoma(Darwin 1854) (Crustacea, Cirripedia): two invasive species new to tropical West African waters. African J. Mar. Sci. 32, 265–268. doi: 10.2989/1814232x.2010.501573
Keswani, A., Oliver, D., Gutierrez, T., and Quilliam, R. (2016). Microbial hitchhikers on marine plastic debris: human exposure risks at bathing waters and beach environments. Mar. Environ. Res. 118, 10–19. doi: 10.1016/j.marenvres.2016.04.006
Kiessling, T., Gutow, L., and Thiel, M. (2015). “Marine litter as habitat and dispersal vector,” in Marine Anthropogenic Litter, eds M. Bergmann, L. Gutow, and M. Thiel (Cham: Springer). doi: 10.1016/j.marpolbul.2018.03.038
Kim, I., Chae, D., Kim, S., Choi, S., and Woo, S. (2015). Factors influencing the spatial variation of microplastics on high-tidal coastal Beaches in Korea. Arch. Environ. Contam. Toxicol. 69, 299–309. doi: 10.1007/s00244-015-0155-6
Kirstein, I., Kirmizi, S., Wichels, A., Garin-Fernandez, A., Erler, R., Löder, M., et al. (2016). Dangerous hitchhikers? Evidence for potentially pathogenic Vibrio spp. on microplastic particles. Mar. Environ. Res. 120, 1–8. doi: 10.1016/j.marenvres.2016.07.004
Kooi, M., Nes, E., Scheffer, M., and Koelmans, A. (2017). Ups and Downs in the Ocean: effects of biofouling on vertical transport of microplastics. Environ. Sci. Technol. 51, 7963–7971. doi: 10.1021/acs.est.6b04702
Korsun, S., Fahrni, J., and Pawlowski, J. (2012). Invading Aurelia aurita has established scyphistoma populations in the Caspian Sea. Mar. Biol. 159, 1061–1069. doi: 10.1007/s00227-012-1886-9
Laganà, P., Caruso, G., Corsi, I., Bergami, E., Venuti, V., Majolino, D., et al. (2019). Do plastics serve as a possible vector for the spread of antibiotic resistance? First insights from bacteria associated to a polystyrene piece from King George Island (Antarctica). Int. J. Hygiene Environ. Health 222, 89–100. doi: 10.1016/j.ijheh.2018.08.009
Lagos, M., White, C., and Marshall, D. (2016). Biofilm history and oxygen availability interact to affect habitat selection in a marine invertebrate. Biofouling 32, 645–655. doi: 10.1080/08927014.2016.1178725
Lagos, M., White, C., and Marshall, D. (2017). Do invasive species live faster? Mass-specific metabolic rate depends on growth form and invasion status. Funct. Ecol. 31, 2080–2086. doi: 10.1111/1365-2435.12913
Law, K., Morét-Ferguson, S., Maximenko, N., Proskurowski, G., Peacock, E., Hafner, J., et al. (2010). Plastic accumulation in the North Atlantic subtropical Gyre. Science 329, 1185–1188. doi: 10.1126/science.1192321
Lebreton, L., Slat, B., Ferrari, F., Sainte-Rose, B., Aitken, J., Marthouse, R., et al. (2018). Evidence that the Great Pacific Garbage Patch is rapidly accumulating plastic. Sci. Rep. 8:4666. doi: 10.1038/s41598-018-22939-w
Leppäkoski, E., and Olenin, S. (2000). Non-native species and rates of spread: lessons from the brackish Baltic Sea. Biol. Invasions. 2, 151–163.
Lewis, J., and Coutts, A. (2010). Biofouling Invasions. Biofouling 348–365. doi: 10.1002/9781444315462.ch24
Lewis, J., Watson, C., and ten Hove, H. (2006). Establishment of the Caribbean Serpulid Tubeworm Hydroides sanctaecrucis Krøyer [in] Mörch, 1863, in Northern Australia. Biol. Invasions 8, 665–671. doi: 10.1007/s10530-005-2062-7
Lo, H., Lee, Y., Po, B., Wong, L., Xu, X., Wong, C., et al. (2020). Impacts of Typhoon Mangkhut in 2018 on the deposition of marine debris and microplastics on beaches in Hong Kong. Sci. Total Environ. 716:137172. doi: 10.1016/j.scitotenv.2020.137172
Lobelle, D., and Cunliffe, M. (2011). Early microbial biofilm formation on marine plastic debris. Mar. Pollut. Bull. 62, 197–200. doi: 10.1016/j.marpolbul.2010.10.013
López-Legentil, S., Legentil, M., Erwin, P., and Turon, X. (2015). Harbor networks as introduction gateways: contrasting distribution patterns of native and introduced ascidians. Biol. Invasions. 17, 1623–1638. doi: 10.1007/s10530-014-0821-z
Lynch, S., Darmody, G., O’Dwyer, K., Gallagher, M., Nolan, S., McAllen, R., et al. (2016). Biology of the invasive ascidian Ascidiella aspersa in its native habitat: Reproductive patterns and parasite load. Estuarine Coast. Shelf Sci. 181, 249–255. doi: 10.1016/j.ecss.2016.08.048
Mantelatto, M., Silva, A., Louzada, T., McFadden, C., and Creed, J. (2018). Invasion of aquarium origin soft corals on a tropical rocky reef in the southwest Atlantic, Brazil. Mar. Pollut. Bull. 130, 84–94. doi: 10.1016/j.marpolbul.2018.03.014
Marchini, A., Ferrario, J., and Minchin, D. (2015a). Marinas may act as hubs for the spread of the pseudo-indigenous bryozoan Amathia verticillata (Delle Chiaje, 1822) and its associates. Sci. Mar. 79, 355–365. doi: 10.3989/scimar.04238.03a
Marchini, A., Ferrario, J., Sfriso, A., and Occhipinti-Ambrogi, A. (2015b). Current status and trends of biological invasions in the Lagoon of Venice, a hotspot of marine NIS introductions in the Mediterranean Sea. Biol. Invasions 17, 2943–2962. doi: 10.1007/s10530-015-0922-3
Martin, J., Baptist, E. E., van Eekelen, P. J. T., Dankers, B., Grasmeijer, T., van Kessel, D. S., et al. (2017). “Working with nature in wadden sea ports,” in Proceedingsof the Coasts & Ports 2017 Conference, (Cairns), 7.
Martínez, J., and Adarraga, I. (2008). First record of invasive caprellid Caprella scaura Templeton, 1836 sensu lato (Crustacea: Amphipoda: Caprellidae) from the Iberian Peninsula. Aquat. Invasions 3, 165–171. doi: 10.3391/ai.2008.3.2.6
Masó, M., Garcés, E., Pagès, F., and Camp, J. (2003). Drifting plastic debris as a potential vector for dispersing Harmful Algal Bloom (HAB) species. Sci. Mar. 67, 107–111. doi: 10.3989/scimar.2003.67n1107
Mathieson, A., Pederson, J., and Dawes, C. (2008). Rapid assessment surveys of fouling and introduced seaweeds in the Northwest Atlantic. Rhodora 110, 406–478. doi: 10.3119/07-4.1
McCuller, M., and Carlton, J. (2018). Transoceanic rafting of Bryozoa (Cyclostomata, Cheilostomata, and Ctenostomata) across the North Pacific Ocean on Japanese tsunami marine debris. Aquat. Invasions 13, 137–162. doi: 10.3391/ai.2018.13.1.11
Miao, L., Wang, P., Hou, J., Yao, Y., Liu, Z., Liu, S., et al. (2019). Distinct community structure and microbial functions of biofilms colonizing microplastics. Sci. Total Environ. 650, 2395–2402. doi: 10.1016/j.scitotenv.2018.09.378
Micael, J., Rodrigues, P., Halldórsson, H., and Gíslason, S. (2020). Distribution and abundance of the invasive tunicate Ciona intestinalis (Linnaeus, 1767) in Icelandic harbours. Reg. Stud. Mar. Sci. 34:101039. doi: 10.1016/j.rsma.2020.101039
Mikkelsen, P., and Bieler, R. (2008). Seashells of Southern Florida. Living Marine Mollusks of the Florida Keys and Adjacent Regions. Princeton: Princeton University Press.
Miller, J., Gillman, R., Carlton, J., Murray, C., Nelson, J., Otani, M., et al. (2018). Trait-based characterization of species transported on Japanese tsunami marine debris: effect of prior invasion history on trait distribution. Mar. Pollut. Bull. 132, 90–101. doi: 10.1016/j.marpolbul.2017.12.064
Miller, K., Engle, J., Uwai, S., and Kawai, H. (2006). First report of the Asian seaweed Sargassum filicinum Harvey (Fucales) in California, USA. Biol. Invasions 9, 609–613. doi: 10.1007/s10530-006-9060-2
Miller, R., Gammon, K., and Day, M. (2009). Antibiotic resistance among bacteria isolated from seawater and penguin fecal samples collected near Palmer Station, Antarctica. This article is one of a selection of papers in the Special Issue on Polar and Alpine Microbiology. Can. J. Microbiol. 55, 37–45. doi: 10.1139/w08-119
Minchin, D., and Gollasch, S. (2005). Vector pathways and the spread of exotic species in the sea. ICES Coop. Res. Rep. 271:25.
Mineur, F., Johnson, M., Maggs, C., and Stegenga, H. (2006). Hull fouling on comercial ships as a vector of macroalgal introduction. Mar. Biol. 151, 1299–1307. doi: 10.1007/s00227-006-0567-y
Miralles, L., Gomez-Agenjo, M., Rayon-Viña, F., Gyraitë, G., and Garcia-Vazquez, E. (2018). Alert calling in port areas: marine litter as possible secondary dispersal vector for hitchhiking invasive species. J. Nat. Conserv. 42, 12–18. doi: 10.1016/j.jnc.2018.01.005
Moore, C., Lattin, G., and Zellers, A. (2011). Quantity and type of plastic debris flowing from two urban rivers to coastal waters and beaches of Southern California. Rev. Gestão Cost. Integrada 11, 65–73. doi: 10.5894/rgci194
Moret-Ferguson, S., Law, K., Proskurowski, G., Murphy, E., Peacock, E., and Reddy, C. (2010). The size, mass, and composition of plastic debris in the western North Atlantic Ocean. Mar. Pollut. Bull. 60, 1873–1878. doi: 10.1016/j.marpolbul.2010.07.020
Morohoshi, T., Oi, T., Aiso, H., Suzuki, T., Okura, T., and Sato, S. (2018). Biofilm formation and degradation of commercially available biodegradable plastic films by bacterial consortiums in freshwater environments. Microb. Environ. 33, 332–335. doi: 10.1264/jsme2.me18033
National Oceanic and Atmospheric Administration Marine Debris Program (2017). Report on Marine Debris as a Potential Pathway for Invasive Species. Silver Spring, MD: National Oceanic and Atmospheric Administration Marine Debris Program.
Nebot, N., Rosa-Jimenez, C., Pie Ninot, R., and Perea-Medina, P. (2017). Challenges for the future of ports. What can be learnt from the Spanish Mediterranean ports? Ocean Coast. Manag. 137, 165–174. doi: 10.1016/j.ocecoaman.2016.12.016
Nikula, R., Spencer, H., and Waters, J. (2013). Passive rafting is a powerful driver of transoceanic gene flow. Biol. Lett. 9:20120821. doi: 10.1098/rsbl.2012.0821
Núñez, J., Molleda, F., and de Lara, J. (2006). El Control del Biofouling en Las Instalaciones Offshore de Acuicultura Marina. XVL Sesiones Técnicas de Ingeniería Naval. Escuela Técnica Superior de Ingenieros Navales. Madrid: Universidad Politécnica de Madrid.
Obbard, R., Sadri, S., Wong, Y., Khitun, A., Baker, I., and Thompson, R. (2014). Global warming releases microplastic legacy frozen in Arctic Sea ice. Earth. Future 2, 315–320. doi: 10.1002/2014ef000240
Oberbeckmann, S., Löder, M., and Labrenz, M. (2015). Marine microplastic-associated biofilms – a review. Environ. Chem. 12:551. doi: 10.1071/en15069
Oberbeckmann, S., Loeder, M., Gerdts, G., and Osborn, A. (2014). Spatial and seasonal variation in diversity and structure of microbial biofilms on marine plastics in Northern European waters. FEMS Microbiol. Ecol. 90, 478–492. doi: 10.1111/1574-6941.12409
Occhipinti-Ambrogi, A., and Savini, D. (2003). Biological invasions as a component of global change in stressed marine ecosystems. Mar. Pollut. Bull. 46, 542–551. doi: 10.1016/s0025-326x(02)00363-6
Ojaveer, H., Galil, B., Campbell, M., Carlton, J., Canning-Clode, J., Cook, E., et al. (2015). Classification of non-indigenous species based on their impacts: considerations for application in marine management. PLoS Biol. 13:e1002130. doi: 10.1371/journal.pbio.1002130
Padilla, D., and Williams, S. (2004). Beyond ballast water: aquarium and ornamental trades as sources of invasive species in aquatic ecosystems. Front. Ecol. Environ., 2, 131–138. doi: 10.1890/1540-9295(2004)002[0131:bbwaao]2.0.co;2
Peng, X., Chen, M., Chen, S., Dasgupta, S., Xu, H., Ta, K., et al. (2018). Microplastics contaminate the deepest part of the world’s ocean. Geochem. Perspect. Lett. 9, 1–5. doi: 10.7185/geochemlet.1829
Pettersen, A., White, C., and Marshall, D. (2016). Metabolic rate covaries with fitness and the pace of the life history in the field. Proc. R. Soc. B Biol. Sci. 283:20160323. doi: 10.1098/rspb.2016.0323
PIANC (2014). Sustainable Ports’ a Guide for Port Authorities. Report n. 150. Available online at: http://www.pianc.org/edits/articleshop.php?id1/42014150 (accessed 30 September, 2016).
Pinochet, J., Leclerc, J., Brante, A., Daguin-Thiébaut, C., Díaz, C., Tellier, F., et al. (2017). Presence of the tunicate Asterocarpa humilis on ship hulls and aquaculture facilities in the coast of the Biobío Region, south central Chile. PeerJ 5:e3672. doi: 10.7717/peerj.3672
Pinochet, J., Urbina, M., and Lagos, M. (2020). Marine invertebrate larvae love plastics: habitat selection and settlement on artificial substrates. Environ. Pollut. 257:113571. doi: 10.1016/j.envpol.2019.113571
Pinteus, S., Lemos, M., Alves, C., Neugebauer, A., Silva, J., Thomas, O., et al. (2018). Marine invasive macroalgae: turning a real threat into a major opportunity - the biotechnological potential of Sargassum muticum and Asparagopsis armata. Algal Res. 34, 217–234. doi: 10.1016/j.algal.2018.06.018
PlasticsEurope (2014). Datos de Mercado: Plastics - the Facts 2014. Available online at: https://www.plasticseurope.org/es/resources/market-data (accessed January 16, 2020).
PlasticsEurope (2018). Datos de Mercado: Plastics - the Facts 2018. Available online at: fhttps://www.plasticseurope.org/es/resources/market-data (accessed March 19, 2018).
Radashevsky, V., and Selifonova, Z. (2013). Records of Polydora cornuta and Streblospio gynobranchiata (Annelida, Spionidae) from the Black Sea. Mediterr. Mar. Sci. 14:261. doi: 10.12681/mms.415
Radisic, V., Nimje, P., Bienfait, A., and Marathe, N. (2020). Marine plastics from Norwegian West Coast Carry Potentially virulent fish pathogens and opportunistic human pathogens harboring new variants of antibiotic resistance genes. Microorganisms 8:1200. doi: 10.3390/microorganisms8081200
Rech, S., Borrell, Y., and García-Vazquez, E. (2016). Marine litter as a vector for non-native species: what we need to know. Mar. Pollut. Bull. 113, 40–43. doi: 10.1016/j.marpolbul.2016.08.032
Rech, S., Borrell Pichs, Y., and García-Vazquez, E. (2018a). Anthropogenic marine litter composition in coastal areas may be a predictor of potentially invasive rafting fauna. PLoS One 13:e0191859. doi: 10.1371/journal.pone.0191859
Rech, S., Salmina, S., Borrell Pichs, Y., and García-Vazquez, E. (2018b). Dispersal of alien invasive species on anthropogenic litter from European mariculture areas. Mar. Pollut. Bull. 131, 10–16.
Reisser, J., Slat, B., Noble, K., du Plessis, K., Epp, M., Proietti, M., et al. (2015). The vertical distribution of buoyant plastics at sea: an observational study in the North Atlantic Gyre. Biogeosciences 12, 1249–1256. doi: 10.5194/bg-12-1249-2015
Rilov, G., and Crooks, J. (2009a). “European enclosed and semienclosed Seas,” in Biological Invasions in Marine Ecosystems, eds G. Rilov and J. Crooks (Berlín: Springer), 529–548.
Rilov, G., and Crooks, J. (2009b). “Marine bioinvasions: conservation hazards and vehicles for ecological understanding,” in Biological Invasions in Marine Ecosystems, eds G. Rilov and J. Crooks (Berlín: Springer), 3–11. doi: 10.1007/978-3-540-79236-9_1
Ros, M., Vázquez-Luis, M., and Guerra-García, J. (2013). The tropical caprellid amphipod Paracaprella pusilla: a new alien crustacean in the Mediterranean Sea. Helgoland Mar. Res. 67, 675–685. doi: 10.1007/s10152-013-0353-4
Sanchez-Vidal, A., Canals, M., Calafat, A., Lastras, G., Pedrosa-Pàmies, R., Menéndez, M., et al. (2012). Impacts on the deep-sea ecosystem by a severe coastal storm. PLoS One 7:e30395. doi: 10.1371/journal.pone.0030395
Schoener, A., and Rowe, G. (1970). Pelagic Sargassum and its presence among the deep-sea benthos. Deep Sea Res. Oceanogr. Abstr. 17, 923–925. doi: 10.1016/0011-7471(70)90010-0
Schwindt, E., López Gappa, J., Raffo, M., Tatián, M., Bortolus, A., Orensanz, J., et al. (2014). Marine fouling invasions in ports of Patagonia (Argentina) with implications for legislation and monitoring programs. Mar. Environ. Res. 99, 60–68. doi: 10.1016/j.marenvres.2014.06.006
Seebens, H., Blackburn, T., Dyer, E., Genovesi, P., Hulme, P., Jeschke, J., et al. (2017). No saturation in the accumulation of alien species worldwide. Nat. Commun. 8:14435. doi: 10.1038/ncomms14435
Serra-Gonçalves, C., Lavers, J., and Bond, A. (2019). Global review of beach debris monitoring and future recommendations. Environ. Sci. Technol. 53, 12158–12167. doi: 10.1021/acs.est.9b01424
Shen, M., Zhu, Y., Zhang, Y., Zeng, G., Wen, X., Yi, H., et al. (2019). Micro(nano)plastics: unignorable vectors for organisms. Mar. Pollut. Bull. 139, 328–331. doi: 10.1016/j.marpolbul.2019.01.004
Shiganova, T., Riisgård, H., Ghabooli, S., and Tendal, O. (2014). First report on Beroe ovata in an unusual mixture of ctenophores in the Great Belt (Denmark). Aquat. Invasions 9, 111–116. doi: 10.3391/ai.2014.9.1.10
Shiganova, T., Sommer, U., Javidpour, J., Molinero, J., Malej, A., Kazmin, A., et al. (2019). Patterns of invasive ctenophore Mnemiopsis leidyi distribution and variability in different recipient environments of the Eurasian seas: A review. Marine Environmental Research 152, 104791. doi: 10.1016/j.marenvres.2019.104791
Shin, P., Leung, J., Qiu, J., Ang, P., Chiu, J., Thiyagarajan, V., et al. (2013). Hypoxia induces abnormal larval development and affects biofilm–larval interaction in the serpulid polychaete -Hydroides elegans. Mar. Pollut. Bull. 76, 291–297. doi: 10.1016/j.marpolbul.2013.08.022
Simberloff, D. (1976). Experimental zoogeography of islands: effects of island size. Ecology 57, 629–648. doi: 10.2307/1936179
Simberloff, D., and Von Holle, B. (1999). Positive interactions of nonindigenous species: invasional meltdown? Biol. Invasions 1, 21–32.
Soares, M., Campos, C., Santos, N., Barroso, H., Mota, E., Menezes, M., et al. (2018). Marine bioinvasions: differences in tropical copepod communities between inside and outside a port. J. Sea Res. 134, 42–48. doi: 10.1016/j.seares.2018.01.002
Sobral, P. (1985). Distribuicao de acartia tonsa dana no estuario do tejo e sua relação com acartia clausi giesbrecht. Bol. Inst. Nacional Invest. Pescas 13, 61–75.
South, P., Floerl, O., Forrest, B., and Thomsen, M. (2017). A review of three decades of research on the invasive kelp Undaria pinnatifida in Australasia: an assessment of its success, impacts and status as one of the world’s worst invaders. Mar. Environ. Res. 131, 243–257. doi: 10.1016/j.marenvres.2017.09.015
Spinuzzi, S., Schneider, K., Walters, L., Yuan, W., and Hoffman, E. (2013). Tracking the distribution of non-native marine invertebrates (Mytella charruana, Perna viridis and Megabalanus coccopoma) along the south-eastern USA. Mar. Biodivers. Rec. 6:e55.
Streftaris, N., and Zenetos, A. (2006). Alien marine species in the Mediterranean-the 100 ‘Worst Invasives’ and their impact. Mediterr. Mar. Sci. 7, 87–118. doi: 10.12681/mms.180
Talley, L. (2002). “Salinity patterns in the Ocean,” in Encyclopedia of Global Change, Volume: the Earth System: Physical and Chemical Dimensions of Global Environmental Change, eds M. C. MacCracken and J. S. Perry (Hoboken, NJ: Wiley), 629–640.
Tavares, M., and De Melo, G. (2004). Discovery of the first known benthic invasive species in the Southern Ocean: the North Atlantic spider crab Hyas araneus found in the Antarctic Peninsula. Antarct. Sci. 16, 129–131. doi: 10.1017/s0954102004001877
Therriault, T., Nelson, J., Carlton, J., Liggan, L., Otani, M., Kawai, H., et al. (2018). The invasion risk of species associated with Japanese Tsunami Marine Debris in Pacific North America and Hawaii. Mar. Pollut. Bull. 132, 82–89. doi: 10.1016/j.marpolbul.2017.12.063
Thevenon, F., Carroll, C., and Sousa, J. (2014). Plastic Debris in the Ocean: The Characterization of Marine Plastics and Their Environmental Impacts, Situation Analysis Report. Gland: IUCN.
Thiel, M., and Gutow, L. (2005). The ecology of rafting in the marine environment. I. the floating substrata. Oceanogr. Mar. Biol. 42, 181–263. doi: 10.1201/9780203507810.ch6
Thiel, M., Hinojosa, I., Joschko, T., and Gutow, L. (2011). Spatio-temporal distribution of floating objects in the German Bight (North Sea). J. Sea Res. 65, 368–379. doi: 10.1016/j.seares.2011.03.002
Thompson, R. (2006). Plastic debris in the marine environment: consequences and solutions. Mar. Nat. Conserv. Eur. 193, 107–115.
Tutman, P., Kapiris, K., Kirinčič, M., and Pallaoro, A. (2017). Floating marine litter as a raft for drifting voyages for Planes minutus (Crustacea: Decapoda: Grapsidae) and Liocarcinus navigator (Crustacea: Decapoda: Polybiidae). Mar. Pollut. Bull. 120, 217–221. doi: 10.1016/j.marpolbul.2017.04.063
Vail, L., and Tranter, D. (1981). Experimental studies on the settlement and growth of bryozoa in the natural environment. Mar. Freshw. Res. 32:639. doi: 10.1071/mf9810639
van Sebille, E., Aliani, S., Law, K., Maximenko, N., Alsina, J., Bagaev, A., et al. (2020). The physical oceanography of the transport of floating marine debris. Environ. Res. Lett. 15:023003. doi: 10.1088/1748-9326/ab6d7d
van Sebille, E., England, M., and Froyland, G. (2012). Origin, dynamics and evolution of ocean garbage patches from observed surface drifters. Environ. Res. Lett. 7:044040. doi: 10.1088/1748-9326/7/4/044040
van Sebille, E., Wilcox, C., Lebreton, L., Maximenko, N., Hardesty, B., van Franeker, J., et al. (2015). A global inventory of small floating plastic debris. Environ. Res. Lett. 10:124006. doi: 10.1088/1748-9326/10/12/124006
Verlaque, M., Steen, F., and De Clerck, O. (2009). Rugulopteryx (Dictyotales, Phaeophyceae), a genus recently introduced to the Mediterranean. Phycologia 48, 536–542. doi: 10.2216/08-103.1
Walters, L., Odom, R., and Zaleski, S. (2011). The aquarium hobby industry and invasive species: Has anything changed? Front. Ecol. Environ. 9:206–207. doi: 10.2307/41149766
Wang, J., Lu, L., Wang, M., Jiang, T., Liu, X., and Ru, S. (2019). Typhoons increase the abundance of microplastics in the marine environment and cultured organisms: a case study in Sanggou Bay, China. Sci. Total Environ. 667, 1–8. doi: 10.1016/j.scitotenv.2019.02.367
Wang, J., Tan, Z., Peng, J., Qiu, Q., and Li, M. (2016). The behaviors of microplastics in the marine environment. Mar. Environ. Res. 113, 7–17. doi: 10.1016/j.marenvres.2015.10.014
Wasson, K., Fenn, K., and Pearse, J. (2005). Habitat differences in marine invasions of central California. Biol. Invasions 7, 935–948. doi: 10.1007/s10530-004-2995-2
Wiggins, A., and Crowston, K. (2011). “From conservation to crowdsourcing: a typology of fcitizen science,” in Proceedings of the Annual Hawaii International Conference on System Sciences, (Kauai, HI).
Williams, S. (2007). Introduced species in seagrass ecosystems: Status and concerns. J. Exp. Mar. Biol. Ecol. 350, 89–110. doi: 10.1016/j.jembe.2007.05.032
Winston, J., Gregory, M., and Stevens, L. (1997). “Encrusters, epibionts, and other biota associated with pelagic plastics: a review of biogeographical, environmental, and conservation issues,” in Marine Debris. Springer Series on Environmental Management, eds J. M. Coe and D. B. Rogers (New York, NY: Springer), 81–97. doi: 10.1007/978-1-4613-8486-1_9
Woodall, L., Sanchez-Vidal, A., Canals, M., Paterson, G., Coppock, R., Sleight, V., et al. (2014). The deep sea is a major sink for microplastic debris. R. Soc. Open Sci. 1:140317. doi: 10.1098/rsos.140317
Yang, Y., Liu, G., Song, W., Ye, C., Lin, H., Li, Z., et al. (2019). Plastics in the marine environment are reservoirs for antibiotic and metal resistance genes. Environ. Int. 123, 79–86. doi: 10.1016/j.envint.2018.11.061
Keywords: plastic debris, alien species, marine exotic species, plastic dispersion, marine ecosystem, non-indigenous species
Citation: García-Gómez JC, Garrigós M and Garrigós J (2021) Plastic as a Vector of Dispersion for Marine Species With Invasive Potential. A Review. Front. Ecol. Evol. 9:629756. doi: 10.3389/fevo.2021.629756
Received: 15 November 2020; Accepted: 18 March 2021;
Published: 26 May 2021.
Edited by:
Ana Novoa, Institute of Botany (ASCR), CzechiaReviewed by:
Jonathan Tempesti, University of Pisa, ItalyGiuseppe Suaria, National Research Council (CNR), Italy
Copyright © 2021 García-Gómez, Garrigós and Garrigós. This is an open-access article distributed under the terms of the Creative Commons Attribution License (CC BY). The use, distribution or reproduction in other forums is permitted, provided the original author(s) and the copyright owner(s) are credited and that the original publication in this journal is cited, in accordance with accepted academic practice. No use, distribution or reproduction is permitted which does not comply with these terms.
*Correspondence: José Carlos García-Gómez, amNnYXJjaWFAdXMuZXM=; Marta Garrigós, bWdhcmxvcGJAZ21haWwuY29t; Javier Garrigós, ZmphdmdhcmxvcEBnbWFpbC5jb20=