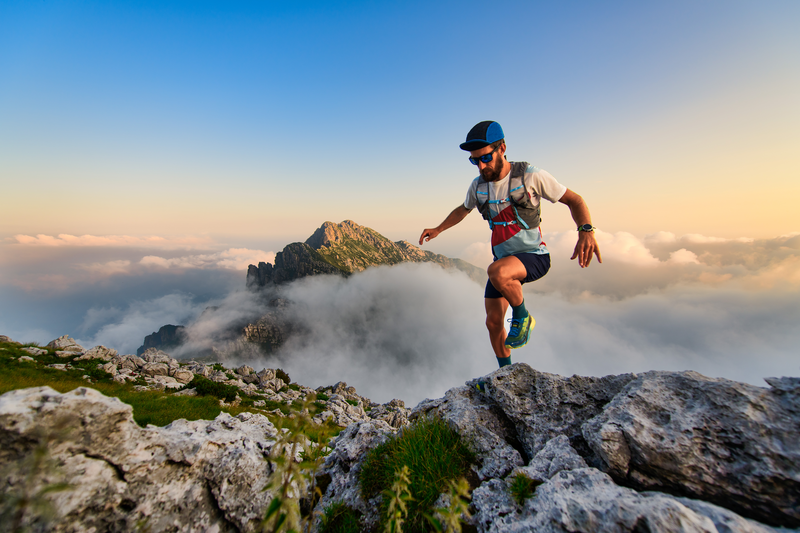
95% of researchers rate our articles as excellent or good
Learn more about the work of our research integrity team to safeguard the quality of each article we publish.
Find out more
MINI REVIEW article
Front. Ecol. Evol. , 12 April 2021
Sec. Models in Ecology and Evolution
Volume 9 - 2021 | https://doi.org/10.3389/fevo.2021.628658
This article is part of the Research Topic Looking Through the Mesh of a Net: The Challenge of Socio-Ecological Systems View all 8 articles
The study of ecosystem services requires the integration of different observational points. This is particularly true in Water, as this element continuously cycles, increasing chances of interaction among services originating in different ecosystems. However, aquatic scientists historically approached the study of inland/freshwater and open/marine waters in different ways and this cultural division potentially hampers integrative approaches. Herein, we explored the literature pertaining to ecosystem services across the last 23 years, analysing 4,590 aquatic papers. By aggregating and intersecting topics included in this papers’ collection using text-mining and topical network approaches, we saw that the study of local environmental conditions (e.g., river estuary management) and synergies and trade-offs between services (e.g., carbon sequestration and water purification) can display several potential conceptual links between freshwater and marine sciences. Our analyses suggest that to intersect ecosystem services across the aquatic continuum, the conceptual integration between marine and freshwater science must be reinforced, especially at the interface between different “salinity realms.” Such integration should adopt a “system thinking” perspective, in which the focus is on multiple socio-ecological processes giving rise to interactions that are (i) biologically mediated, (ii) potentially conflicting, and (iii) entangled within networks.
Covering 70% of Earth’s surface, Water is the foundation for our life, health, and wealth. Inland waters, mainly rivers and lakes (∼0.5% of the total), are reservoirs of drinking water and support agriculture and industrial activities. In contrast, open waters, like seas and oceans (∼97%), mainly represent a vast “territory” suitable for resources supply, transportation, and explorations (Van der Leeden, 1990). In its multiple manifestations, water is the fulcrum of “ecosystem services,” a socio-ecological concept formalising the strong bond between Nature and Society (Costanza et al., 1997; Liquete et al., 2013; Grizzetti et al., 2016; Aznar-Sánchez et al., 2019).
Ecosystem services (ES) have been defined as the multiple and diverse benefits to humans provided by healthy environments, and they were initially categorised into four groups. Supporting services, including nutrients’ cycling and primary production, are the ones that determine the best environmental conditions for ecosystems to guarantee the other three categories: Provisioning (of material goods, e.g., drinking water and food), Regulating (e.g., climate regulation, coastal protection), and Cultural services (e.g., education and aesthetics values; Millennium Ecosystem Assessment, 2005). Two policy milestones, the Economics of Ecosystems and Biodiversity (TEEB) in 2010 (Sukhdev et al., 2010), and The Common International Classification of Ecosystem Services (CICES) in 2013 (Haines-Young and Potschin-Young, 2018), led to the integration of Supporting services in the Regulating and Maintenance, remarking the intrinsic role of biological factors, collectively expressed by biodiversity and natural habitats, in guaranteeing all ES categories.
Physical, chemical, and biological factors connect ES within interaction networks, in which overlapping cause–effect relationships can be disentangled only by pursuing integration between different observational points, focusing on ecological and socio-economic drivers, within and across ecosystems (Dee et al., 2017). Such a “system thinking” perspective is particularly needed in aquatic ES-studies since water continuously cycles, increasing chances of interaction among services originating in different ecosystems (e.g., Hamilton, 2010). However, aquatic scientists historically approached the study of inland/freshwater and open/marine waters in different ways (e.g., see the well-known “salty-divide” between Limnology and Oceanography; Kavanaugh et al., 2013), and this cultural division potentially hampers integrative approaches to ES.
Building on the considerations above, in this paper we assess the present—and envision the potential for—conceptual intersections among the different approaches to ES across the aquatic continuum. To pursue this goal, we explored peer-reviewed papers published throughout 23 years, from January 1997 to June 2020, by employing an informatic approach to aggregate and synthesise the scientific information.
Our workflow included the following operational steps:
I. Extraction of the most frequent issues (e.g., concepts and practices) associated with aquatic ES;
II. Use of this information to analyse the semantic correlation between research in different aquatic systems;
III. Detection and discussion—through topical network analysis—of intersection pathways between approaches to ES across the aquatic continuum.
I. Literature was explored with an informatic approach run in the R language (R Core Team, 2017) using the tidytext package (Silge and Robinson, 2016) and following the rationale described in Hay Mele et al. (2019). Web of Science was queried for the simultaneous presence of the topic ecosystem services and the words sea, ocean, river, or lake (as representative of the main and most studied types of aquatic systems) in the title and/or abstract of published papers (1997–2020). The output was divided into four sets, one for each of the targeted systems (Figure 1A), plus two sets aggregating all papers pertaining to either freshwater (river + lake) or marine water (ocean + sea). We used an upSet plot (Gehlenborg, 2019) to visualise how papers were partitioned among systems (Figure 1B). This visualisation is composed of two parts: a bar chart and a dot and lines plot. The main bar chart shows the number of papers that belong to a combination of systems, with each bar reporting a different combination. The dot and lines plot below each bar show the mix that makes up each cluster.
II. For each paper, we derived a “bag of words” synthesising concepts therein; to eliminate conceptual redundancy in such bags, we derived “lemmas” (by reducing, e.g., change, changing to chang) to detect bigrams—more contextualised two-word sequences. We counted bigrams within each aquatic system and calculated, as an index of rarity, the “inverse document frequency” (IDF; the logarithm of the number of total documents, divided by the number of documents in which the bigram appears). Based on IDF, we identified the top fifteen bigrams in each aquatic system and then we assigned each bigram to an ES group, using the ES-classification by CICES (Liquete et al., 2013; Feeley et al., 2016; Haines-Young and Potschin-Young, 2018; classifications are summarised in Supplementary Table 1). To correlate ecosystems against each other based on ES, we calculated the Pearson correlation coefficient [cor.test() function in R] between bigrams’ frequencies for marine and fresh waters and estimated statistical significance (SS) and confidence intervals (CI) for the index using standard methods (t-test for SS, and Fisher transformation for CI; Figure 1C).
III. We used papers more involved in cross-system correlation to produce a topical network (Figure 2), using the text-mining web platform InfraNodus (Paranyushkin, 2019)1. In such a network, nodes are words/topics referring to ES-concepts characterising different papers, edges between nodes represent the association of topic pairs within and among papers, and edge-weights are proportional to association frequency. This kind of network allows the extraction of topical groups, the identification of those categories of topics facilitating the information flow, and the connotation of conceptual intersections amongst different aquatic contexts. Network display and analyses were performed within Gephi using the ForceAtlas2 algorithm (Bastian et al., 2009; Jacomy et al., 2014).
Figure 1. Correlation of aquatic systems based on sharing of ecosystem services (ES) concepts. (A) Publication trend of aquatic-ES papers (1997–2020). (B) upSet plot displaying the repartition of papers among and within systems. Bars are paper partitions; histograms display the size of the partition. Colours in (B) are as in (A). (C) Comparison of word frequencies between fresh- and marine waters. The plot is a 2d histogram with hexagonal bins, marking 2d range in frequencies; histogram colour represents the number of words with that 2d range; no hexagon is drawn in areas without points. The closer a hexagon is to the red line, the more the words within it are used with similar frequencies and the other way round. Words with a frequency higher than 0.0005% are explicitly shown as labels in each plot, with lines connecting each word to its bin. The x and y scales are in logs and mark the relative bigram frequency within fresh- and marine waters, respectively.
Figure 2. Topical network based on concepts relating with ecosystem services (ES) at the fresh–seawater interface. The network is based on papers including concepts shared between oceans, seas and rivers (n = 177). In the upper-left graph, the network structure is shown, with nodes being words/topics, edges between nodes representing the association of nodes in the text, and edge-weights being proportional to the frequency of these associations across the analysed dataset. Nodes’ size is proportional to their importance in the network. Nodes’ colour remarks their belonging to different topical groups, shown in the upper-right bars’ drawing. The larger graph shows the topical network with labels indicating ES-related concepts.
Our search retrieved 4,590 peer-reviewed papers pertaining to aquatic ES (Supplementary Data). The publication trend becomes seemingly exponential after the publication of MAE in 2005 (Figure 1A). Regulation and Maintenance services (RM) were the most prominent, with an overall limited presence of Provisioning and Cultural services (P and C; Supplementary Table 2). Most ES-papers (2782) dealt with freshwaters and papers crossing salinity boundaries were a mere 3.8% of the total (Figure 1B). Only two papers integrated all aquatic ecosystems, and the most prominent one of these addressed the impact of climate change on ES in tropical rivers and, in turn, on the associated enclosed, semi-enclosed and open water bodies (Hamilton, 2010).
“Water quality” was the most recurrent concept in riverine and lacustrine studies, followed by the relationship between land cover (e.g., expansion of productive zones and urbanisation) and aquatic resources. These observations remark the historically established perception of a strong dependence of terrestrial economies from the management of aquatic reservoirs (e.g., Meneses et al., 2015). As for sea/ocean papers, the management and protection of shallow areas were predominant (Supplementary Table 2): sea-level rising was a prominent issue, while the frequency of coastal-related words remarked a substantial scientific interest for the land-sea/ocean interface. Coral-reef-related issues, among the most prominent ones, indicate that ubiquitous, iconic, severely menaced (e.g., by climate change), and service-providing (e.g., coastal protection) systems attracted investigation efforts too (e.g., Beck et al., 2018).
Concepts relating with Cultural ES had a low prevalence among the papers retrieved, and they were unevenly represented in the different systems (Supplementary Table 2). For instance, whereas “human activity” was present in all systems, except for the oceans, “sustainable development” appeared in the sole ocean papers. This observation justifies international organisations and governments’ efforts to empower research around these issues (e.g., Rodrigues et al., 2017). Moreover, Cultural services were weakly associated with recreation, although this latter intercept wealthy economic sectors (Hay Mele et al., 2019). On this line, methods are being tested to assign economic values to C-services provided by Posidonia oceanica and coralligenous assemblages in support of recreational diving in the Mediterranean Sea (Zunino et al., 2019, 2020). However, efforts are required to integrate spiritual, inspirational, and identity experiences within Cultural ES, as suggested by Rodrigues et al. (2017).
When correlating aquatic systems against each other in terms of the relative frequency of ES bigrams, the most integrated pairs were “river–lake” (Pearson r = 0.77, p < 0.0001) and “sea–ocean” (Pearson r = 0.82, p < 0.0001), plausibly due to the physical proximity and connectance between contiguous systems. Climate change, the main topic in one-fourth of the sea–ocean papers and one-sixth of the river–lake papers, was a significant driver of the correlations mentioned above. This result is not surprising: climate-change research increased globally in the 2000s’ (Haunschild et al., 2016), as global warming can affect all “waters” and the services they provide (Downing, 2014; Callaghan et al., 2020), for instance by impacting primary production (e.g., O’Beirne et al., 2017; D’Alelio et al., 2020) and food webs (Murphy et al., 2020).
The correlation between fresh- and marine realms was not negligible (Pearson r = 0.4, p < 0.0001; Figure 1C) and driven by papers (27%) dealing with ES in estuaries, which represent both physical and conceptual interfaces (Xenopoulos et al., 2017). This correlation was supported by shared topics besides “climate change,” such as “ecosystem functioning” and “trade-offs.” This is also not surprising: ecosystem functioning (sensu Tilman et al., 2014)—i.e., the multiple and intertwined ecological processes at the base of ES—positively affected sharing of methods and concepts between Limnology and Oceanography, driving the adoption of the same theoretical background to, e.g., assess and predict water quality in lakes and productivity levels in the oceans (Xenopoulos et al., 2017; Spanbauer et al., 2020). Moreover, the prominent role played by trade-offs (sensu Rodríguez et al., 2006; Figure 1C), may plausibly stem from the rising interest, in both marine and freshwater contexts, in interactions between socio-ecological processes.
Somewhat surprisingly, “biodiversity” and related issues, like “habitat” and “conservation,” did not appear to contribute to the conceptual cross-salinity correlation (Figure 1C), even though these topics were present in roughly one-quarter of the papers that we analysed. On the one hand, the fact that a topic has not yet been focused on may reflect that it is not worth addressing: for instance, that topic could be assumed scientifically uninteresting or irrelevant for environmental management. Conversely, we might consider that biodiversity is tightly connected with ecosystem functioning (Tilman et al., 2014); since the relationship between the biological properties of aquatic ecosystems and the services they provide is still an emerging topic (Teixeira et al., 2019), research efforts across different “salinity realms” could be weakly present in the primary literature.
Nonetheless, inland and open waters exchange matter because water moves, or provides a medium within which organisms move, and these facts can affect biological processes, leading to interactions between ES. For instance, on the macroscopic side, the presence of migratory fishes undergoing freshwater-to-seawater transitions can call for conservation actions in reducing coastal urbanisation and river dams, which threaten those fishes’ survival (Lennox et al., 2019), with positive feedback on a number of ES. On the microscopic side, the convergence of freshwater and marine Harmful Algal Blooms can call for management actions to prevent noxious phytoplankton accumulation in estuaries, which might kill fish and contaminate mussels (Peacock et al., 2018), thus hampering provisioning services.
These considerations led us to imagine that the link between ES originating between different but contiguous aquatic systems (like transitional and coastal ones) may be more explicit (and direct) under the physical and chemical (say, environmental) points of view and more hidden (therefore, indirect) when considering biological factors—this fact stemming from the fact that marine and freshwater systems host different ecological communities, and scientists tend to study these independently. That is why we intersected the fragmented information present in the scientific literature using network theory, as we show in the next section.
We produced a topical network using papers about transitional and coastal systems (n = 177; Figure 2) extracted from those supporting the conceptual correlation between marine and freshwater contexts (Figure 1C). In that network, we found eleven topical groups, including words that are more frequently associated within papers (see, in Figure 2, the rightmost bar chart). Overall, topical groups were tightly linked, both directly and indirectly. The most relevant ones dealt with estuary management and observation of tidal systems (e.g., Stahl et al., 2018; Murray et al., 2019). These topics were entangled within a convoluted network spanning from the assessment of flood-related risks (Broekx et al., 2011) to the economic impact of sea-level rise (Shirazi et al., 2019). “Environmental” topics were numerically relevant and conceptually broad, albeit not setting at the centre of the information-flow (see pink, green and blue nodes in Figure 2), which was occupied by a topical group head by “coastal,” “wetland,” and “quantify” (orange nodes in Figure 2).
By exploring this “orange” group, we saw that biological concepts were central to convey the information about ES between marine and freshwater systems. For instance, going from inland to open waters, an apparently minor wetland like peatlands (orange nodes in Figure 2) can sustain freshwater biodiversity, provide safe drinking water (green nodes), minimise flood risk and sequester carbon (dark and bright green, respectively). They are also considered crucial for the coastal systems since mosses—the dominant life form of peatlands—can release biologically available iron (a limiting nutrient) that contribute to the sustenance of marine phytoplankton production (Krachler et al., 2016). Biologically driven integration pathways are also consistent in the reverse direction: going from open to inland waters, oyster reefs are reported to regulate water quality, protect the shoreline from erosion, and drive economic productivity in marine ecosystems (Grabowski et al., 2012). Simultaneously, they can be beneficial for inland water systems since they provide a barrier against landward penetration of seawater and can retain freshwater from rivers (Kaplan et al., 2016).
The topical network approach made it possible to explore interactions and biologically mediated trade-offs between ES. For instance, the landward encroachment observed in mangrove forests over the last decades at the global scale, flanking climate change and sea-level rise (see red and blue nodes in Figure 2), seemed to strongly enhance the global level of blue carbon storage (i.e., the sequestration of carbon performed by aquatic plants; bright-green nodes in Figure 2; Kelleway et al., 2016). This effect counteracts climate change and protects fisheries (with positive feedback on RM and P services, from the red to the bright green network nodes). Nonetheless, in their geographic expansion along the coasts (across orange nodes; Figure 2), mangroves (and the blue carbon storage) started to compete with salt marshes (light blue nodes; Figure 2), provoking a reduction in their coverage. This competition, in turn, induced habitat withdrawn (negative feedback on P and C services; e.g., Kelleway et al., 2017) and modified ecosystem functioning at a local scale (by changing the soil microbial-community, e.g., Barreto et al., 2018), making this an example of aquatic vs. terrestrial ES trade-off.
Topical network analysis also allowed us to identify those topics that, though poorly investigated, could be important to integrate ES studies. Indeed, the words “reef,” “quantify,” “matter,” “change,” and “risk” (belonging, respectively, to whitish-green, orange, orange, red, and dark-green nodes in Figure 2) bridged many thematic areas. Thus, research questions embracing them, like “quantifying the role of oyster reefs in reducing the risk associated with changes in riverine matter fluxes to the sea,” could represent important integrative initiatives. This kind of semantic effort also helps connect distant themes, such as those represented by the topical groups ecosystem-change-service and sediment-impact-marsh (see the red and light-blue nodes in Figure 2). It does so, for example, by intensifying the “study of the state of salt marshes as impacted by changes in sediment fluxes from rivers,” a rare but cross-cutting topic (Rutherford et al., 2018; Yang et al., 2020).
All these examples remark that sorting for the role of biological processes in connecting aquatic systems can help to assess the historical events bringing to the present state of ES, as well as to envision the potential outcomes of ES interactions. To this respect, the application of Long-Term Socio-Ecological Research (see “long + term” dark-green nodes in Figure 2) in both coastal (Zingone et al., 2019), freshwater (Salmaso et al., 2020), and transversal contexts (Morabito et al., 2018), may represent a necessary step toward conceptual integration in aquatic sciences across the aquatic continuum, going from land-based pollution regulation to fishery management.
Our analyses suggest that, in order to intersect ecosystem services across the aquatic continuum, the conceptual integration between marine and freshwater science must be reinforced, especially at the interface between different “salinity realms.” Such integration should adopt a “system thinking” perspective, in which the focus is on multiple and overlapping socio-ecological processes giving rise to interactions that are (i) biologically mediated, (ii) potentially conflicting, and (iii) entangled within networks.
Ecosystem services are provided by natural processes acting at hugely different scales (i.e., from the global to regional ones). However, the combined effects of global and local phenomena, such as climate change and anthropogenic stressors, respectively, is highly dependent on local conditions, including those characterising the land-sea interface (Gissi et al., 2020). While inland water-systems are characterised by concrete actions to manage socio-ecological systems using properly holistic approaches (e.g., Rathwell and Peterson, 2012), analogous attempts must be improved in coastal and transitional systems, as the so-called marine spatial planning, aiming to drive the use of ecosystems reducing socio-ecological/economic conflicts, still represents an emerging topic (Arkema et al., 2015),
Finally, interactions between socio-ecological processes introduce synergies and trade-offs, which can impact humans’ health and wealth either positively or negatively at different time-scales (Howe et al., 2014); to map the complex interaction between ES fully, it is fundamental to reveal the role of biodiversity and ecosystem functioning in ES regulation across aquatic systems. This need is even more pressing as the dependence of the human population from aquatic food resources (e.g., from aquaculture) is not only rising, but it is becoming severely menaced by multiple and synergistic stressors acting at both global and local scales (Hay Mele et al., 2020; Sarà et al., 2021).
DD’A conceived and supervised research. DD’A, LR, and BHM performed analyses and visualised data. LR drafted the first version of the manuscript. DD’A and FP wrote the final version of the manuscript, with the help of LR and BHM. All authors contributed to the article and approved the submitted version.
This study was supported by the project PO FEAMP 2014/2020 (Misura 2.51) funded by Regione Campania, Italy. BHM and LR acknowledge the projects DISCO, funded by the Gordon and Betty Moore Foundation (Grant Agreement #7978), and EuroSea, funded by the European Commission (Grant Agreement ID: 862626), respectively, for financial support.
The authors declare that the research was conducted in the absence of any commercial or financial relationships that could be construed as a potential conflict of interest.
We thank reviewers for their constructive comments that enhanced the quality of this manuscript.
The Supplementary Material for this article can be found online at: https://www.frontiersin.org/articles/10.3389/fevo.2021.628658/full#supplementary-material
Arkema, K. K., Verutes, G. M., Wood, S. A., Clarke-Samuels, C., Rosado, S., Canto, M., et al. (2015). Embedding ecosystem services in coastal planning leads to better outcomes for people and nature. Proc. Natl. Acad. Sci. U.S.A. 112, 7390–7395. doi: 10.1073/pnas.1406483112
Aznar-Sánchez, J. A., Velasco-Muñoz, J. F., Belmonte-Ureña, L. J., and Manzano-Agugliaro, F. (2019). The worldwide research trends on water ecosystem services. Ecol. Indic. 99, 310–323. doi: 10.1016/j.ecolind.2018.12.045
Barreto, C. R., Morrissey, E. M., Wykoff, D. D., and Chapman, S. K. (2018). Co-occurring mangroves and salt marshes differ in microbial community composition. Wetlands 38, 497–508. doi: 10.1007/s13157-018-0994-9
Bastian, M., Heymann, S., and Jacomy, M. (2009). “Gephi: an open-source software for exploring and manipulating networks,” in Proceedings of the 3rd International Conference on Weblogs and Social Media, ICWSM 2009, ICWSM 8, San Jose, CA, 361–362.
Beck, M. W., Losada, I. J., Menéndez, P., Reguero, B. G., Díaz-Simal, P., and Fernández, F. (2018). The global flood protection savings provided by coral reefs. Nat. Commun. 9:2186. doi: 10.1038/s41467-018-04568-z
Broekx, S., Smets, S., Liekens, I., Bulckaen, D., and de Nocker, L. (2011). Designing a long-term flood risk management plan for the Scheldt estuary using a risk-based approach. Nat. Hazards 57, 245–266. doi: 10.1007/s11069-010-9610-x
Callaghan, M. W., Minx, J. C., and Forster, P. M. (2020). A topography of climate change research. Nat. Clim. Chang. 10, 118–123. doi: 10.1038/s41558-019-0684-5
Costanza, R., Arge, R., Groot, R., De Farber, S., Hannon, B., Limburg, K., et al. (1997). The value of the world’s ecosystem services and natural capital. Nature 387, 253–260.
D’Alelio, D., Rampone, S., Cusano, L. M., Morfino, V., Russo, L., Sanseverino, N., et al. (2020). Machine learning identifies a strong association between warming and reduced primary productivity in an oligotrophic ocean gyre. Sci. Rep. 10:3287. doi: 10.1038/s41598-020-59989-y
Dee, L. E., Allesina, S., Bonn, A., Eklöf, A., Gaines, S. D., Hines, J., et al. (2017). Operationalizing network theory for ecosystem service assessments. Trends Ecol. Evol. 32, 118–130. doi: 10.1016/j.tree.2016.10.011
Downing, J. A. (2014). Limnology and oceanography: two estranged twins reuniting by global change. Inl. Waters 4, 215–232. doi: 10.5268/IW-4.2.753
Feeley, H. B., Bruen, M., Bullock, C., Christie, M., Kelly, F., Remoundou, K., et al. (2016). ESManage Literature Review Ecosystem Services in Freshwaters. Wexford: Environmental Protection Agency, doi: 10.13140/RG.2.2.10420.24968
Gehlenborg, N. (2019). UpSetR: A More Scalable Alternative to Venn and Euler Diagrams for Visualizing Intersecting Sets. R package version 1.4.0. doi: 10.1093/bioinformatics/btx364
Gissi, E., Manea, E., Mazaris, A. D., Fraschetti, S., Almpanidou, V., Bevilacqua, S., et al. (2020). A review of the combined effects of climate change and other local human stressors on the marine environment. Sci. Total Environ. 755:142564. doi: 10.1016/j.scitotenv.2020.142564
Grabowski, J. H., Brumbaugh, R. D., Conrad, R. F., Keeler, A. G., Opaluch, J. J., Peterson, C. H., et al. (2012). Economic valuation of ecosystem services provided by oyster reefs. Bioscience 62, 900–909. doi: 10.1525/bio.2012.62.10.10
Grizzetti, B., Lanzanova, D., Liquete, C., Reynaud, A., and Cardoso, A. C. (2016). Assessing water ecosystem services for water resource management. Environ. Sci. Policy 61, 194–203. doi: 10.1016/j.envsci.2016.04.008
Haines-Young, R., and Potschin-Young, M. B. (2018). Revision of the common international classification for ecosystem services (CICES V5.1): a policy brief. One Ecosyst. 3, e27108. doi: 10.3897/oneeco.3.e27108
Hamilton, S. K. (2010). Biogeochemical implications of climate change for tropical rivers and floodplains. Hydrobiologia 657, 19–35. doi: 10.1007/s10750-009-0086-1
Haunschild, R., Bornmann, L., and Marx, W. (2016). Climate change research in View of bibliometrics. PLoS One 11:e0160393. doi: 10.1371/journal.pone.0160393
Hay Mele, B., Russo, L., Crocetta, F., Gambi, C., Dell’Anno, A., Danovaro, R., et al. (2020). Ecological assessment of anthropogenic impact in marine ecosystems: the case of Bagnoli Bay. Mar. Environ. Res. 158, 104953. doi: 10.1016/j.marenvres.2020.104953
Hay Mele, B., Russo, L., and D’Alelio, D. (2019). Combining marine ecology and economy to roadmap the integrated coastal management: a systematic literature review. Sustainability 11:4393. doi: 10.3390/su11164393
Howe, C., Suich, H., Vira, B., and Mace, G. M. (2014). Creating win-wins from trade-offs? Ecosystem services for human well-being: a meta-analysis of ecosystem service trade-offs and synergies in the real world. Glob. Environ. Chang. 28, 263–275. doi: 10.1016/j.gloenvcha.2014.07.005
Jacomy, M., Venturini, T., Heymann, S., and Bastian, M. (2014). ForceAtlas2, a continuous graph layout algorithm for handy network visualization designed for the Gephi software. PLoS One 9:e98679. doi: 10.1371/journal.pone.0098679
Kaplan, D. A., Olabarrieta, M., Frederick, P., and Valle-Levinson, A. (2016). Freshwater detention by oyster reefs: Quantifying a keystone ecosystem service. PLoS One 11:e0167694. doi: 10.1371/journal.pone.0167694
Kavanaugh, M. T., Holtgrieve, G. W., Baulch, H., Brum, J. R., Cuvelier, M. L., Filstrup, C. T., et al. (2013). A salty divide within aslo? Limnol. Oceanogr. Bull. 22, 34–37. doi: 10.1002/lob.201322233
Kelleway, J. J., Cavanaugh, K., Rogers, K., Feller, I. C., Ens, E., Doughty, C., et al. (2017). Review of the ecosystem service implications of mangrove encroachment into salt marshes. Glob. Chang. Biol. 23, 3967–3983. doi: 10.1111/gcb.13727
Kelleway, J. J., Saintilan, N., Macreadie, P. I., Skilbeck, C. G., Zawadzki, A., and Ralph, P. J. (2016). Seventy years of continuous encroachment substantially increases “blue carbon” capacity as mangroves replace intertidal salt marshes. Glob. Chang. Biol. 22, 1097–1109. doi: 10.1111/gcb.13158
Krachler, R., Krachler, R. F., Wallner, G., Steier, P., El Abiead, Y., Wiesinger, H., et al. (2016). Sphagnum-dominated bog systems are highly effective yet variable sources of bio-available iron to marine waters. Sci. Total Environ. 556, 53–62. doi: 10.1016/j.scitotenv.2016.03.012
Lennox, R. J., Paukert, C. P., Aarestrup, K., Auger-Méthé, M., Baumgartner, L., Birnie-Gauvin, K., et al. (2019). One hundred pressing questions on the future of global fish migration science, conservation, and policy. Front. Ecol. Evol. 7:286. doi: 10.3389/fevo.2019.00286
Liquete, C., Piroddi, C., Drakou, E. G., Gurney, L., Katsanevakis, S., Charef, A., et al. (2013). Current status and future prospects for the assessment of marine and coastal ecosystem services: a systematic review. PLoS One 8:e67737. doi: 10.1371/journal.pone.0067737
Meneses, B. M., Reis, R., Vale, M. J., and Saraiva, R. (2015). Land use and land cover changes in Zêzere watershed (Portugal) - Water quality implications. Sci. Total Environ. 527–528, 439–447. doi: 10.1016/j.scitotenv.2015.04.092
Millennium Ecosystem Assessment. (2005). Ecosystems and Human Well-Being. Washington DC: Synthesis.Island Press.
Morabito, G., Mazzocchi, M. G., Salmaso, N., Zingone, A., Bergami, C., Flaim, G., et al. (2018). Plankton dynamics across the freshwater, transitional and marine research sites of the LTER-Italy network. patterns, fluctuations, drivers. Sci. Total Environ. 627, 373–387. doi: 10.1016/j.scitotenv.2018.01.153
Murphy, G. E. P., Romanuk, T. N., and Worm, B. (2020). Cascading effects of climate change on plankton community structure. Ecol. Evol. 10, 2170–2181. doi: 10.1002/ece3.6055
Murray, N. J., Phinn, S. R., DeWitt, M., Ferrari, R., Johnston, R., Lyons, M. B., et al. (2019). The global distribution and trajectory of tidal flats. Nature 565, 222–225. doi: 10.1038/s41586-018-0805-8
O’Beirne, M. D., Werne, J. P., Hecky, R. E., Johnson, T. C., Katsev, S., and Reavie, E. D. (2017). Anthropogenic climate change has altered primary productivity in Lake Superior. Nature communications 8:15713.
Paranyushkin, D. (2019). “InfraNodus: generating insight using text network analysis,” in Proceedings of the WWW 19 The World Wide Web Conference, eds L. Liu and R. White San Francisco CA: Association for Computing Machinery 3584–3589.
Peacock, M. B., Gibble, C. M., Senn, D. B., Cloern, J. E., and Kudela, R. M. (2018). Blurred lines: Multiple freshwater and marine algal toxins at the land-sea interface of San Francisco Bay. California. Harmful Algae 73, 138–147. doi: 10.1016/j.hal.2018.02.005
R Core Team (2017). R: A Language and Environment for Statistical Computing. R Foundation for Statistical Computing. Vienna: Austria.
Rathwell, K. J., and Peterson, G. D. (2012). Connecting social networks with ecosystem services for watershed governance: a social-ecological network perspective highlights the critical role of bridging organizations. Ecol. Soc. 17:24. doi: 10.5751/ES-04810-170224
Rodrigues, J. G., Conides, A. J., Rivero Rodriguez, S., Raicevich, S. S., Pita, P., Kleisner, K. M., et al. (2017). Marine and coastal cultural ecosystem services: knowledge gaps and research priorities. One Ecosyst. 2:e12290. doi: 10.3897/oneeco.2.e12290
Rodríguez, J. P., Beard, T. D., Bennett, E. M., Cumming, G. S., Cork, S. J., Agard, J., et al. (2006). Trade-offs across space, time, and ecosystem services. Ecol. Soc. 11:28. doi: 10.5751/ES-01667-110128
Rutherford, J. S., Day, J. W., D’Elia, C. F., Wiegman, A. R. H., Willson, C. S., Caffey, R. H., et al. (2018). Evaluating trade-offs of a large, infrequent sediment diversion for restoration of a forested wetland in the Mississippi delta. Estuar. Coast. Shelf Sci. 203, 80–89. doi: 10.1016/j.ecss.2018.01.016
Salmaso, N., Buzzi, F., Capelli, C., Cerasino, L., Leoni, B., Lepori, F., et al. (2020). Responses to local and global stressors in the large southern perialpine lakes: present status and challenges for research and management. J. Great Lakes Res 46, 752–766. doi: 10.1016/j.jglr.2020.01.017
Sarà, G., Mangano, M. C., Berlino, M., Corbari, L., Lucchese, M., Milisenda, G., et al. (2021). The Synergistic Impacts of Anthropogenic Stressors and COVID-19 on Aquaculture: A Current Global Perspective. Rev. Fish. Sci. Aquac. doi: 10.1080/23308249.2021.187663.3. [Epub ahead of print].
Shirazi, Y. A., Carr, E. W., Parsons, G. R., Hoagland, P., Ralston, D. K., and Chen, J. (2019). Increased operational costs of electricity generation in the delaware river and estuary from salinity increases due to sea-level rise and a deepened channel. J. Environ. Manage. 244, 228–234. doi: 10.1016/j.jenvman.2019.04.056
Silge, J., and Robinson, D. (2016). tidytext: text mining and analysis using tidy data principles in R. J. Open Source Softw. 1:37. doi: 10.21105/joss.00037
Spanbauer, T. L., Briseño-Avena, C., Pitz, K. J., and Suter, E. (2020). Salty sensors, fresh ideas: the use of molecular and imaging sensors in understanding plankton dynamics across marine and freshwater ecosystems. Limnol. Oceanogr. Lett. 5, 169–184. doi: 10.1002/lol2.10128
Stahl, M., Widney, S., and Craft, C. (2018). Tidal freshwater forests: sentinels for climate change. Ecol. Eng. 116, 104–109. doi: 10.1016/j.ecoleng.2018.03.002
Sukhdev, P., Wittmer, H., Schröter-Schlaack, C., Nesshöver, C., Bishop, J., Ten Brink, P., et al. (2010). The Economics of Ecosystems and Biodiversity: Mainstreaming the Economics of Nature: A Synthesis of the Approach, Conclusions and Recommendations of TEEB. Geneva: TEEB, doi: 10.1007/BF02331707
Teixeira, H., Lillebø, A. I., Culhane, F., Robinson, L., Trauner, D., Borgwardt, F., et al. (2019). Linking biodiversity to ecosystem services supply: patterns across aquatic ecosystems. Sci. Total Environ. 657, 517–534. doi: 10.1016/j.scitotenv.2018.11.440
Tilman, D., Isbell, F., and Cowles, J. M. (2014). Biodiversity and ecosystem functioning. Annu. Rev. Ecol. Evol. Syst. 45, 471–493. doi: 10.1146/annurev-ecolsys-120213-091917
Xenopoulos, M. A., Downing, J. A., Kumar, M. D., Menden-Deuer, S., and Voss, M. (2017). Headwaters to oceans: ecological and biogeochemical contrasts across the aquatic continuum. Limnol. Oceanogr. 62, S3–S14. doi: 10.1002/lno.10721
Yang, S. L., Luo, X., Temmerman, S., Kirwan, M., Bouma, T., Xu, K., et al. (2020). Role of delta-front erosion in sustaining salt marshes under sea-level rise and fluvial sediment decline. Limnol. Oceanogr. 65, 1990–2009. doi: 10.1002/lno.11432
Zingone, A., D’Alelio, D., Mazzocchi, M. G., Montresor, M., and Sarno, D. (2019). Time series and beyond: multifaceted plankton research at a marine Mediterranean LTER site. Nat. Conserv. 34:273. doi: 10.3897/natureconservation.34.30789
Zunino, S., Canu, D. M., Marangon, F., and Troiano, S. (2020). Cultural ecosystem services provided by coralligenous assemblages and posidonia oceanica in the Italian seas. Front. Mar. Sci. 6:823. doi: 10.3389/fmars.2019.00823
Keywords: ecosystem services, sea, ocean, river, lake, climate change, trade-offs, networks
Citation: D’Alelio D, Russo L, Hay Mele B and Pomati F (2021) Intersecting Ecosystem Services Across the Aquatic Continuum: From Global Change Impacts to Local, and Biologically Driven, Synergies and Trade-Offs. Front. Ecol. Evol. 9:628658. doi: 10.3389/fevo.2021.628658
Received: 12 November 2020; Accepted: 15 March 2021;
Published: 12 April 2021.
Edited by:
Jeffrey Mark Dambacher, CSIRO, AustraliaReviewed by:
Martin Drechsler, Helmholtz Centre for Environmental Research (UFZ), GermanyCopyright © 2021 D’Alelio, Russo, Hay Mele and Pomati. This is an open-access article distributed under the terms of the Creative Commons Attribution License (CC BY). The use, distribution or reproduction in other forums is permitted, provided the original author(s) and the copyright owner(s) are credited and that the original publication in this journal is cited, in accordance with accepted academic practice. No use, distribution or reproduction is permitted which does not comply with these terms.
*Correspondence: Domenico D’Alelio, ZG9tZW5pY28uZGFsZWxpb0Bzem4uaXQ=; ZG9tLmRhbGVsaW9AZ21haWwuY29t
Disclaimer: All claims expressed in this article are solely those of the authors and do not necessarily represent those of their affiliated organizations, or those of the publisher, the editors and the reviewers. Any product that may be evaluated in this article or claim that may be made by its manufacturer is not guaranteed or endorsed by the publisher.
Research integrity at Frontiers
Learn more about the work of our research integrity team to safeguard the quality of each article we publish.