- 1Laboratório de Mosquitos Transmissores de Hematozoários, Instituto Oswaldo Cruz, Fundação Oswaldo Cruz (FIOCRUZ), Rio de Janeiro, Brazil
- 2Programa de Computação Científica, Fundação Oswaldo Cruz (FIOCRUZ), Rio de Janeiro, Brazil
- 3Department of Thematic Studies—Environmental Change, Linköping University, Linköping, Sweden
- 4Multiuser Unit of Environmental Analysis, Universidade Federal do Rio de Janeiro, Rio de Janeiro, Brazil
Aedes aegypti is adapted to live in close association with human dwellings, where it lays eggs in several man-made container types with a broad range of size, shape, and material. Biotic and abiotic conditions of larval habitats determine the abundance and body size of emerging adult mosquitoes. Here, we estimated the predictive potential of physicochemical water variables for Culicidae immature abundance and Ae. aegypti adult body size in four neighborhoods with distinct urban landscapes in Rio de Janeiro, Brazil. Domestic water holding containers (N = 240) were inspected for the presence of Culicidae immatures and had several physiochemical parameters measured. Larvae and pupae were counted, and pupae were reared to the adult stage for taxonomic identification. Dry weight and wing size were measured for Ae. aegypti adult mosquitoes (N = 981). The association between larval habitat parameters with Culicidae abundance and Ae. aegypti body size data was estimated through linear mixed models and generalized linear mixed models, respectively, with the neighborhood as random effect. The abundance of immature Culicidae in larval habitats (from which >90% of adults emerging from field collected pupae were Ae. aegypti) was positively associated with container volume and the dissolved organic carbon concentration (DOC). Female average dry weight and male and female wing lengths were positively associated with larval habitat temperature whereas male average dry weight was positively related to water conductivity. Aedes aegypti originating from larval habitats with Ae. albopictus exhibited no differences in median wing length and dry body weight when compared with specimens collected in containers exclusively colonized by Ae. aegypti. These results demonstrate that container water volume (characteristic easily observed in the field) and DOC (often higher in unmanaged water holding recipients) is related to higher Ae. aegypti immature density. Estimating the effects of physicochemical water variables on immature abundance and adult body size can provide valuable information for predicting arbovirus transmission risk in endemic settings.
Introduction
The incidence of arboviral diseases has increased substantially in the last few decades, with up to half of the world population currently under the risk of infection by dengue, Zika and chikungunya viruses (Bhatt et al., 2013; Messina et al., 2016; Nsoesie et al., 2016). The spread of mosquito-borne diseases to urban settlements in tropical and subtropical regions worldwide is associated with the geographic expansion of the main vectors. In the Americas, the primary vector of the three aforementioned viruses is the container-breeding mosquito Aedes aegypti.
Aedes aegypti is adapted to live in close association with human dwellings and its geographic distribution is constrained by environmental conditions, especially temperature (Kraemer et al., 2015). Female mosquitoes preferentially blood feed on human hosts (Harrington et al., 2001), rest inside premises and lay their eggs mostly in man-made containers located in peridomestic areas (Clements, 1992). Several container types with a broad range of size, shape and material usually receive Ae. aegypti eggs, including tires, plant pots, plastic pots, drains, swimming pools and water tanks (Christophers, 1960; Lourenço-de-Oliveira et al., 2004; Maciel-de-Freitas et al., 2007b). The frequency in which Ae. aegypti colonizes each container type in a given area is influenced by manifold socio-economic and behavioral factors.
The quality of larval habitats is determined by several factors including the nutritional resources input (e.g., organic matter, microorganisms and algae), larval density (i.e., competition), presence of predators and water physicochemical properties (Clements, 1992; Juliano, 2009). Aedes aegypti exhibits a decrease in larval development time and body size as temperature increases but there is a reduction in survival when the larvae are kept at extreme temperatures, as 15°C or 35°C (Rueda et al., 1990; Tun-Lin et al., 2000). Other abiotic factors, such as salinity and pH, may also impact larval survival and growth rate, hindering the presence of this mosquito species in salted water or habitats with extreme pH values (Peryassú, 1908; Clark et al., 2004a,b; Medeiros-Sousa et al., 2020). Similarly, food availability or its limitation by intraspecific or interspecific competition has significant effects on larval development time and adult emergence (Yee et al., 2006).
Food availability and competition in domestic water holding containers are considered major determinants of adult Ae. aegypti development and body size. Physical and chemical characteristics of larval habitats have also been correlated with the occurrence and abundance of Ae. aegypti, including water volume, temperature, pH and salinity (Strickman and Kittayapong, 2003; Schneider et al., 2004; Barrera et al., 2006; Medeiros-Sousa et al., 2020). Among the traits affected by the water quality, some are directly related with mosquito vectorial capacity such as survival, body size and biting behavior, and thus have a direct effect on disease transmission intensity (Garrett-Jones, 1964; Nasci, 1991; Tun-Lin et al., 2000; Cohuet et al., 2010; Sasmita et al., 2019). Increasing temperatures of larval habitats are associated with shortening larval development time, which reflects on a faster adult emergence (Tun-Lin et al., 2000). The likelihood of dengue infection in mosquitoes increased with size, i.e., Ae. aegypti that experienced a less competitive larval habitat (Juliano et al., 2014). On the other hand, poor nutrition and/or competition during larval development resulted in reduced adult body size, longevity and fecundity (Briegel, 1990; Tun-Lin et al., 2000; Maciel-De-Freitas et al., 2007a; Reiskin and Lounibos, 2009), but were associated with higher susceptibility to dengue virus under laboratory conditions (Alto et al., 2008). In addition, smaller females usually blood-fed more frequently than larger females (Scott et al., 2000; Farjana and Tuno, 2013) as they might emerge with insufficient energy reserves to complete oogenesis from a single bloodmeal (Macdonald, 1956). Such behavior increases the contact rate with hosts and as a consequence the risk of pathogen transmission. Identifying container variables correlated with better habitat quality for Ae. aegypti in urban settings has the potential to direct control approaches toward the elimination of larval habitats that potentially produce high numbers of large sized adults with presumably higher survival and fecundity rates (Chadee and Focks, 1997).
In this context, we conducted an exploratory survey to estimate the predictive potential of physicochemical water variables for immature Culicidae abundance and Ae. aegypti adult body size in four neighborhoods with distinct urban landscapes in Rio de Janeiro. We also addressed the effects of the co-occurrence with Ae. albopictus on the weight and wing size of Ae. aegypti.
Materials and Methods
Study Areas
Rio de Janeiro city, Brazil, is a dengue and chikungunya endemic city with complex, heterogeneous and disordered urban structure, in which high-income areas and slums are in vicinity to each other. In this scenario, Ae. aegypti larval habitats have been shown to vary according to urban characteristics such as the availability of piped water, human density and housing type (Maciel-de-Freitas et al., 2007b; David et al., 2009). Field collections were conducted in four neighborhoods: Curicica (CUR), Prainha (PRA), Tubiacanga (TUB) and Vila Valqueire (VILA), representing the range of socio-demographic and economic conditions across Rio de Janeiro (Table 1). Therefore, it is expected that the containers sampled are representative of Ae. aegypti larval habitats in Rio. At each site, agents from the Rio de Janeiro Department of Health randomly visited and inspected the houses until they found approximately 10 negatives and 50 positives water-holding containers for Ae. aegypti immature forms (larvae and/or pupae). Dengue and chikungunya epidemic season in Rio usually goes from December-April. Data were collected April–August 2010.
Physicochemical Characterization of Mosquito Larval Habitats and Water/Sediment Analysis
Containers were classified according to type, location (indoors or outdoors) and the following parameters were registered in loco: dissolved O2, water temperature (oxymeter SevenGo Pro, Mettler Toledo, Ohio, EUA), pH (pHmeter model 826 pH Mobile, Metrohm, São Paulo, Brazil), conductivity (codutivimeter SevenGo, Mettler Toledo, Ohio, EUA), air temperature, wind speed, light incidence (CA810 Luxmeter, Chauvin Arnoux, Paris, France) and the presence of insect predators (e.g., Toxorhynchites and Odonata immature stages). Water volume was calculated from the length, width and height of the water column in large containers (>10 L) or directly measured by transferring it to a graduated beaker. The surface of larval habitats was calculated using container size measurements.
A sample of the water and sediment from each container was collected using an algae sampler, which consisted of a kitchen sink plunger with a brush inside connected to an plastic syringe for sample collection (Loeb, 1981). The sediment was sampled by pressing the plunger firmly to the substrate, which was scrubbed with the brush. The contents of the plunger cup were then collected with a syringe. Two sizes of algae samplers were used according to containers surface: one using an 20ml syringe (7 cm2 of sampling area) for containers with <50cm2 and one with an 80ml syringe (44.2 cm2 of sampling area) for containers >50cm2 of surface area. The water/sediment samples from containers were transferred to plastic bags, properly identified and transported in a cool box to the laboratory, where they remained frozen (–20°C) for about 2 months.
Unfiltered frozen samples (10 ml) of water/sediment were processed for Total Phosphorus via orthophosphate determination in a spectrophotometer according to Valderrama (1981) and Total Nitrogen through nitrate detection with ion chromatography with UV detection (Metrohm, São Paulo, Brazil).
Water samples were filtered with GF/F 47 mm Whatman filters, preserved with 15 μL of phosphoric acid and kept in the fridge at 4°C for no longer than 10 days. DOC was determined by an elemental carbon analyzer (model HiperToc, Thermo Scientific, Waltham, EUA) for oxidation with sodium persulfate and determination with UV light. Samples were analyzed in analytical triplicate.
The sediment was analyzed for total organic carbon (sediment organic carbon, SOC) levels after drying in porcelain crucibles in an oven with forced air circulation for 30 min at 60°C. The remaining dry material was weighed, and the organic matter was dosed by calcination in a muffle furnace at 400°C for 12 h.
Mosquito Collection, Taxonomic Identification, and Body Measurement
All larvae and pupae were collected with a strainer and plastic pipette, transferred to a plastic bags containing water from the larval site and stored in a cool box. For ethical issues, all the remaining water was discarded from containers. When larval site elimination was not possible (e.g., water for domestic use), the water holding container was covered or treated with larvicide by local health agents.
Samples were transported to the laboratory where immature mosquitoes were counted, and the pupae reared to adults in dechlorinated tap water at 28 ± 2°C and 70 ± 10% relative humidity. After taxonomic identification (Consoli and de Oliveira, 1994), adult Ae. aegypti mosquitoes were killed with ethyl acetate and dried in a heat oven at 50°C by 15 h. Subsequently, specimens were weighed in a precision scale (Denver Instrument, New York, EUA) and the wing length measured by taking the distance from the axillary incision to the apical margin excluding the fringe (Harbach and Knight, 1980).
Data Analysis
Statistical analysis was conducted with R environment (R Development Core Team, 2011). Container types were classified into five categories according to the criteria used by the Brazilian Ministry of Health (Ministério da Saúde, 2009; Table 1). A list of all positive and negative containers types sampled per neighborhood is available in Supplementary Tables S1, S2. Exploratory analyses were first performed to identify environmental differences between neighborhoods, as well as variations in physicochemical characteristics according to larval habitat categories.
Principal Component Analysis (PCA) and Random Forest (RF) classification (Liaw and Wiener, 2002) were employed to test whether larval habitats exhibited specific physicochemical profiles according to container category or geographical origin. Differences between positive and negative larval habitats for Ae. aegypti were also graphically evaluated through PCA. RF classifying models were trained with a random sample of ∼70% of the data (N = 139 larval habitats) and then validated with the remaining 30% (N = 60 larval habitats) using the “caret” R package. The overall model accuracy and the Kappa index, as well as the identification of most influencing parameters for classification, were obtained after applying the RF models to the validation dataset. PCA was applied on the correlation matrix after scaling variables to have unit variance since they were on different scales and therefore exhibit different variances. In this case, variables were standardized to mean equal to zero and variance equal to one. The environmental parameters “air temperature,” “container surface,” and “location (indoors or outdoors)” were removed from data analysis due to strong correlation (r > 0.7) with “water temperature,” “water volume,” and “light incidence,” respectively. The presence of predators was excluded from the analyses since only a single predatory larva of Toxorhynchites sp. was observed in one bromeliad from VILA. The environmental variables considered in RF and PCA analyses are listed in Table 3.
In order to elucidate possible associations between larval habitats characteristics and mosquito abundances, the total count of immature per recipient was included as dependent variable in generalized linear mixed models (GLMMs) using the “lme4” R package (Bates et al., 2015). We considered the total number of Culicidae immature forms collected, since only the pupae were reared to the adult stage for taxonomic identification, and also positive and negative containers for Ae. aegypti. The independent variables considered in the GLMMs are listed in Table 3. In order to improve the quality of model fit, the variables “volume,” “light incidence,” “conductivity,” “DOC,” and “SOC” were included in the models at the log scale. The count of Culicidae immature per larval habitat was analyzed with a GLMM with negative binomial distribution with the neighborhood as random effect in order to incorporate the variation between collection sites in the models. A regression with negative binomial distribution was preferred over the traditional Poisson distribution because data exhibited over-dispersion (i.e., variance was larger than the mean), confirmed by the Pearson Chi2 test and Dispersion Statistic >1, calculated using the “msme” R package (Hilbe and Robinson, 2013). The consistency of data with the negative binomial distribution was verified using the goodness of fit test “Minimum Chi-squared” (Pearson χ2 = 240.7, df = 233, p = 0.35) from the “goodfit” command, implemented in the “vcd” R package (Meyer et al., 2020). After adjusting independent variables to univariate models, those with significant effects (α = 0.1) on immature Culicidae per larval habitat were used to build GLMMs. The most informative and parcimonious model was selected through second-order Akaike’s information criterion scores (AICc) and level of support (AICcWt), calculated using the “AICcmodavg” package (Mazerolle, 2019). Collinearity between independent variables was checked in the best model through VIFs (Variance Inflation Factors) (Zuur et al., 2010). The assumptions of the best model were examined by checking heteroscedasticity, residuals dispersion and the presence of outliers with the R package DHARMa (Hartig, 2020). The conditional Pseudo-R-squared for GLMMs (R2c), calculated using the MuMIn R package (Bartón, 2014), was employed as goodness-of-fit metric and a measurement of variance explained by the entire model, including both fixed and random effects.
Regarding the body size analyses, Ae. aegypti average wing length and dry weight were calculated for each larval habitat according to mosquito sex. Since there was a significant, but moderate correlation between these two variables (Pearson’s correlation female: t = 11.7, p < 0.001, r = 0.69, r2 = 0.48; male: t = 10.4, p < 0.001, r = 0.60, r2 = 0.36; Supplementary Figure S1), both were fitted as dependent variables at the Linear Mixed Models (LLMs) with the neighborhood as random effect (Table 3). As data was considered normally distributed, a gaussian distribution was applied (One-sample Kolmogorov-Smirnov test p > 0.05). The independent variable “wind speed” was removed from these analyses once aquatic mosquito larvae and pupae are submerged, therefore would not be directly exposed to variations in this environmental condition. Model selection and validation followed the previously mentioned procedures.
The dry weight and wing size were compared between Ae. aegypti originating from larval habitats with and without Ae. albopictus (or any other mosquito species). Differences in mosquito body size were tested between groups using the Kruskal-Wallis test as male wing size was not considered normally distributed (One-sample Kolmogorov-Smirnov test p < 0.05).
Ethical Considerations
Larval surveys are routinely performed by health agents from the Rio de Janeiro City Health Department (Secretaria Municipal de Saúde do Rio de Janeiro, SMS-RJ). Access to containers inside dwellings were obtained after consent of householder approving the entrance of the research team and the health agent who routinely worked at that neighborhood.
Results
Larval Habitats and Mosquito Collection
In total, 240 water holding containers were selected at the four field sites, of which 204 were positive for Ae. aegypti immature presence. The number of sampled containers according to category and study site are shown in Table 4 and a total of 3,184 mosquito immatures were collected, of which 1,168 were pupae. Pupae survival to the adult stage allowed the taxonomic identification of 1,064 mosquitoes, 692 males and 372 females. Although most of adult mosquitoes were identified as Ae. aegypti (Table 5), Ae. albopictus, Aedes (Ochlerotatus) sp., Culex sp., and Wyeomyia sp. were also identified in the inspected containers (Table 5). Of the 981 adult Ae. aegypti, 638 were males and 343 were females which provided the measure of body weight and wing length as a proxy for body size.

Table 5. Taxonomic identification of adult mosquitoes collected during the pupal stage in larval habitat from four neighborhoods in Rio de Janeiro, Brazil.
Physicochemical Parameters of Water and Sediment According to Geographical Origin and Between Container Types
The accuracy and Kappa index of RF models fitted to classify larval habitats using physicochemical parameters of water and sediment were higher according to geographical location than container category (Table 6). Overall model accuracy for neighborhood was 78.3%, with Classification sensitivity (true positive rate) varying between 63 (VILA) to 92% (TUB). Water temperature, volume and light incidence were the most important predictors for sample discrimination into neighborhood of collection. Median water temperature in TUB containers was 25.7°C meanwhile it ranged between 21.4 and 21.6°C in the other field sites. Container volume tended to be higher in TUB and contrasted to the median volume of larval habitats sampled elsewhere (TUB: median of 7.2 L; other sites: median of 0.3–0.6 L). On the other hand, median light incidence was 63.5 lx in TUB larval habitats while it ranged between 113 and 154.1 lx in the other sampled neighborhoods. A less efficient classification was observed for container categories, with the container volume as the most important predictor and overall accuracy of 50%. Raw larval habitat data can be found in Supplementary Table S3.
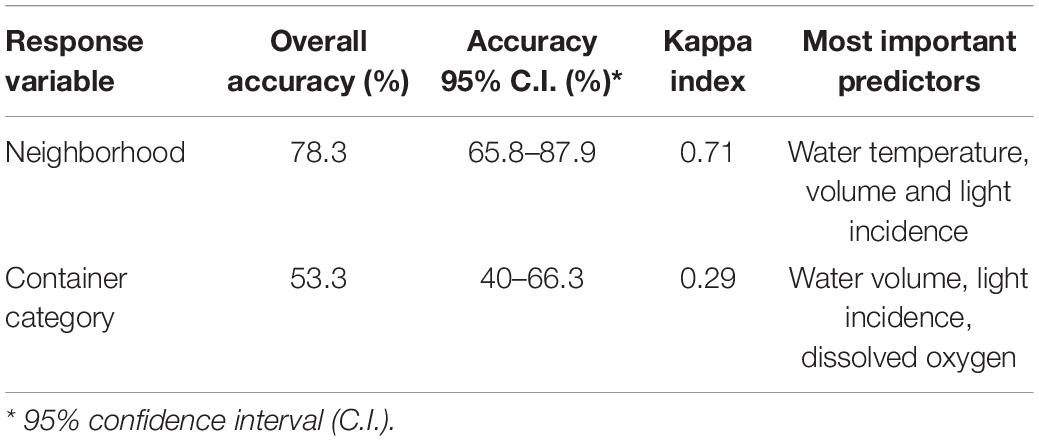
Table 6. Random forest classification of larval habitats into container types or neighborhoods using physicochemical characteristics of water and sediment as predictors.
According to the PCA, the first two axes explained 27.3% of total variation. The first axis accounted for 13.8% of data variance, with DOC, light incidence and water temperature as the variables that most contributed to explain the dataset variation (Supplementary Figure S2A). Dissolved O2, SOC and water temperature were the main variables explaining the second component (accounting for 13.5% of variance) (Supplementary Figure S2B). TUB larval habitat formed a more heterogeneous cluster, compared to CUR, PRA and VILA (Figure 1A). No discrimination was noted between container types (Figure 1B) nor between positive and negative Ae. aegypti larval habitats (Figure 1C), corroborating RF classification results. Considering the higher heterogeneity between TUB larval habitats in comparison to the other field sites, GLMMs and LMMs for abundance and body size investigations were adjusted with neighborhood as a random effect.
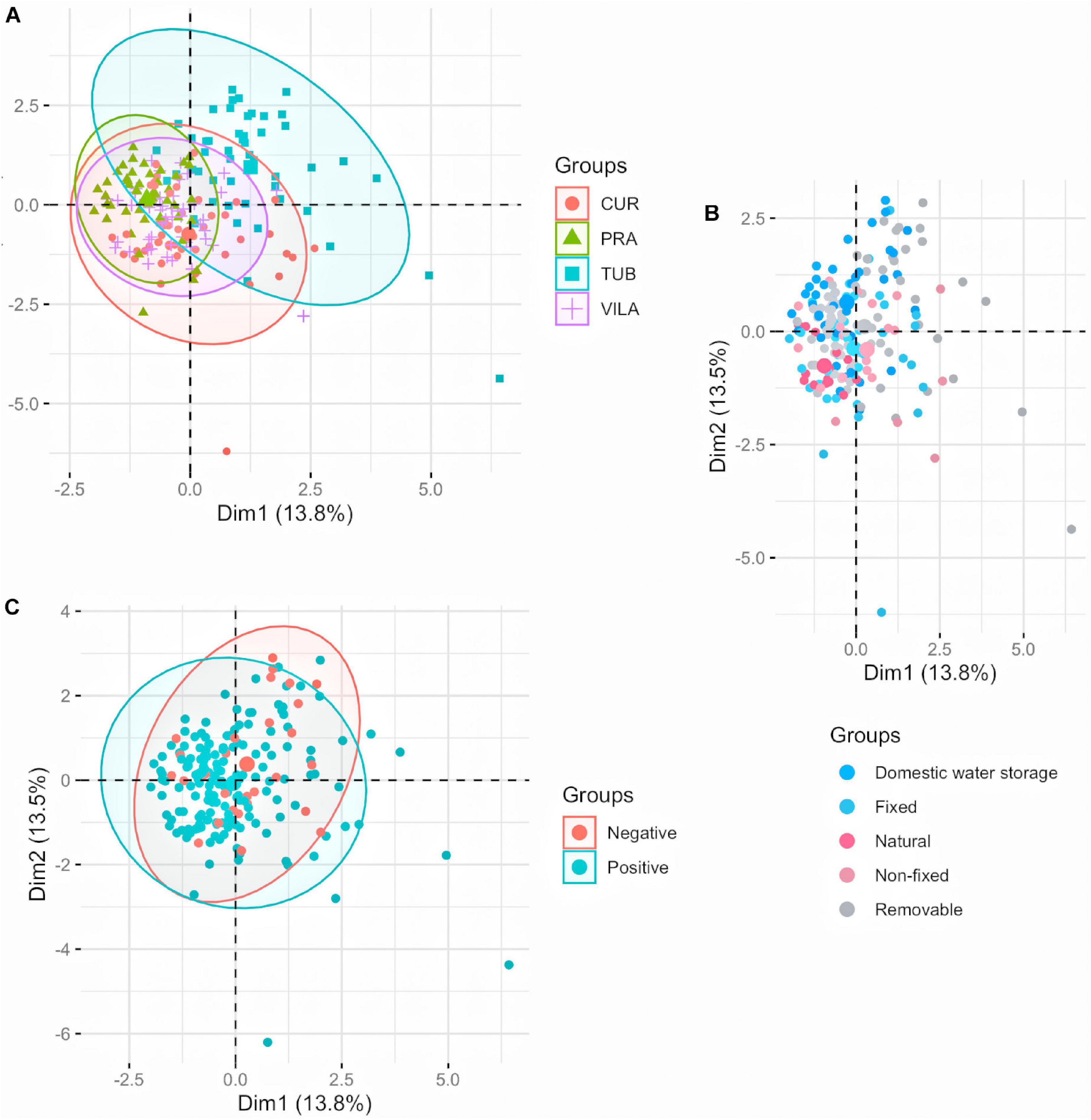
Figure 1. Principal component analysis of larval habitats. Each point corresponds to a larval habitat, colored according to the field site of collection (A), container category (B) and positive or negative for Ae. aegypti presence (C). Overlapped points are represented by bigger circles. Container categories are described in Table 2.
Larval Habitat Characteristics and Immature Abundance
Univariate GLMM analyses indicated a significant association of immature Culicidae abundance in larval habitats with volume, total nitrogen, total phosphorous and DOC concentrations (Supplementary Table S4). Subsequently, GLMMs were fitted using these four independent variables and compared to the null model. The most informative GLMM had volume, total nitrogen, total phosphorous and DOC as independent variables, but only volume and DOC exhibited significant effects on the number of immature Culicidae. Model R2c was 0.37. These results suggest that the number of immature Culicidae increased with larval habitat volume and DOC concentration (Table 7). For example, TUB, the neighborhood in which container volume tended to be higher, exhibited 31.3 immature per larval habitat in average, while it varied between 4.7 and 8.8 in the other field sites. Descriptive analysis of immature abundance data can be found in Supplementary Table S5.
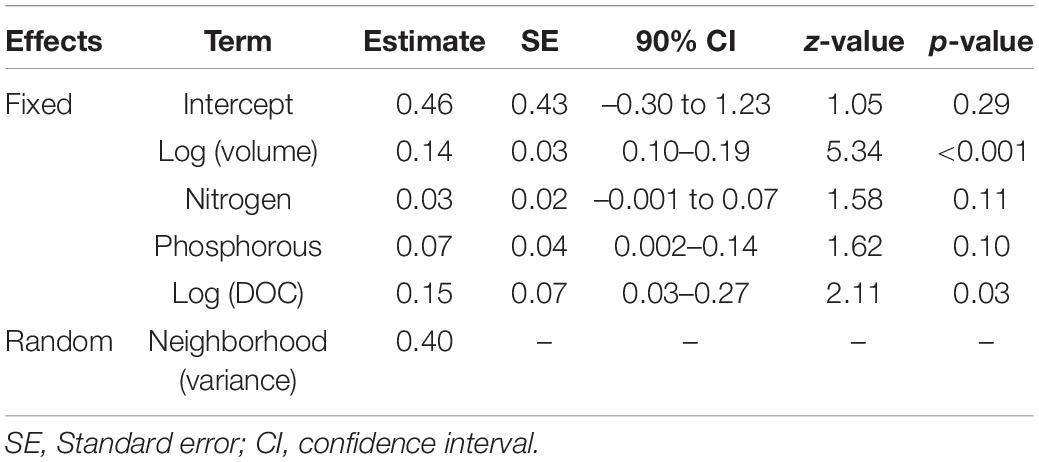
Table 7. Results of the generalized linear mixed model (negative binomial) of the number of immature Culicidae in larval habitats.
Larval Habitat Characteristics and Ae. aegypti Body Size
Female Ae. aegypti originated from 126 larval habitats. Regarding average dry weight and wing length per container, the univariate LMMs presented only water temperature as significant independent variable, with R2c of 0.15 and 0.06, respectively. The models output indicates that both female weight and wing length increased with water temperature (Table 8). Additionally, neighborhood variance estimates were near zero, suggesting there was virtually no among-neighborhood variance left to be explained by the random intercepts (Table 8). Indeed, in TUB, the neighborhood with higher water temperatures, female Ae. aegypti had slightly larger wings, with 2.56 mm in average, while it ranged between 2.39 and 2.51 in the other field sites. However, the same pattern could not be clearly seen for the dry weight, as PRA and TUB mosquitoes had in average 0.32 mg, while it was 0.38–0.39 mg in average in VILA and CUR, respectively (Supplementary Table S5).
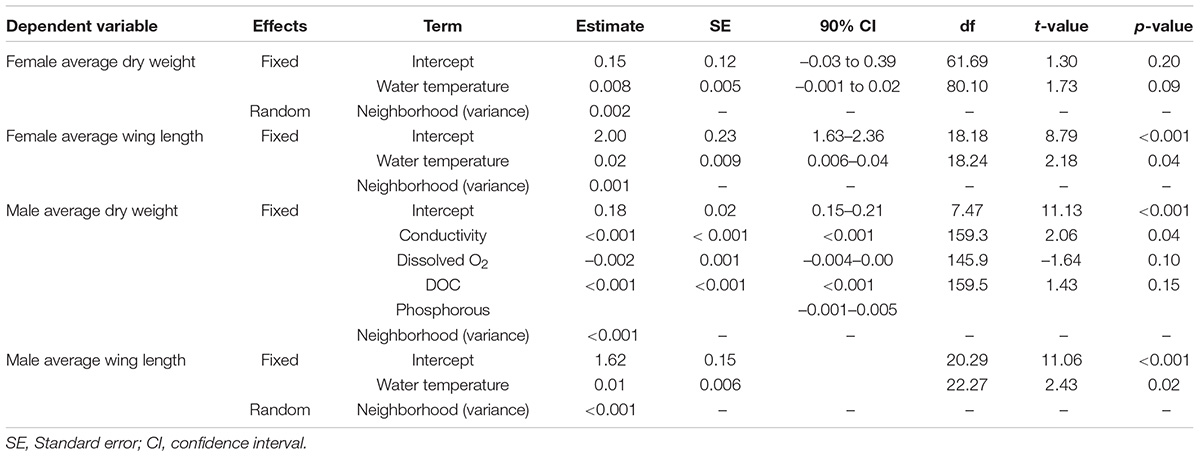
Table 8. Results of the linear mixed models of the average wing length and body weight per larval habitat of female and male Ae. aegypti.
Male Ae. aegypti originated from 160 larval habitats. The univariate LLMs indicated a significant association between average male dry weight per container with dissolved O2, conductivity, phosphorous and DOC (Supplementary Table S6). Therefore, GLMMs were fitted using these four independent variables and were compared to the null model. The full model was the one with the highest support, with male dry weight increasing with water conductivity (Table 8), which was in median 322.50 μS/cm (ranging from 2.25 to 1,374 μS/cm), with no variation between neighborhoods or container categories, as shown by RF and PCA analysis. The R2c was 0.09 for this LLM. For male wing length, only water temperature had a significant effect, suggesting an increase of wing length with temperature. As seen for female Ae. aegypti, male tended to have larger wings in TUB (2.06 mm in average against 1.90–1.98 mm in average in the other neighborhoods, Supplementary Table S5). However, the R2c was quite low (0.06), as shown for the other LMMs fitted for body size measurements. Neighborhood variance estimates were near zero, suggesting there was virtually no among-neighborhood variance left to be explained by the random intercepts (Table 8).
Co-occurrence With Ae. albopictus and Ae. aegypti Body Size
The two species were simultaneously observed in 12 out of 204 larval habitats (5.9%), six from TUB and in two containers from each of the other field sites. A total of 30 male and 22 female Ae. aegypti mosquitoes were collected from shared larval habitats with Ae. albopictus, while 545 males and 301 Ae. aegypti females originated from larval habitats where this species occurred exclusively. Due to disparities higher than 10-fold between the number of Ae. aegypti originating from shared and non-shared containers, a random sample of 30 female and 30 male mosquitoes was taken from the second group in order to balance sample sizes for statistical comparison. Median dry weight and wing length tended to be lower for both male and female when co-occurring with Ae. albopictus, although with no statistical significance (Figures 2A–D: Female wing length: KW χ2 = 1.88, df = 1, p = 0.17; Female dry weight: KW χ2 = 1.93, df = 1, p = 0.16; Male wing length: KW χ2 = 1.99, df = 1, p = 0.16; Male dry weight: KW χ2 = 2.76, df = 1, p = 0.10).
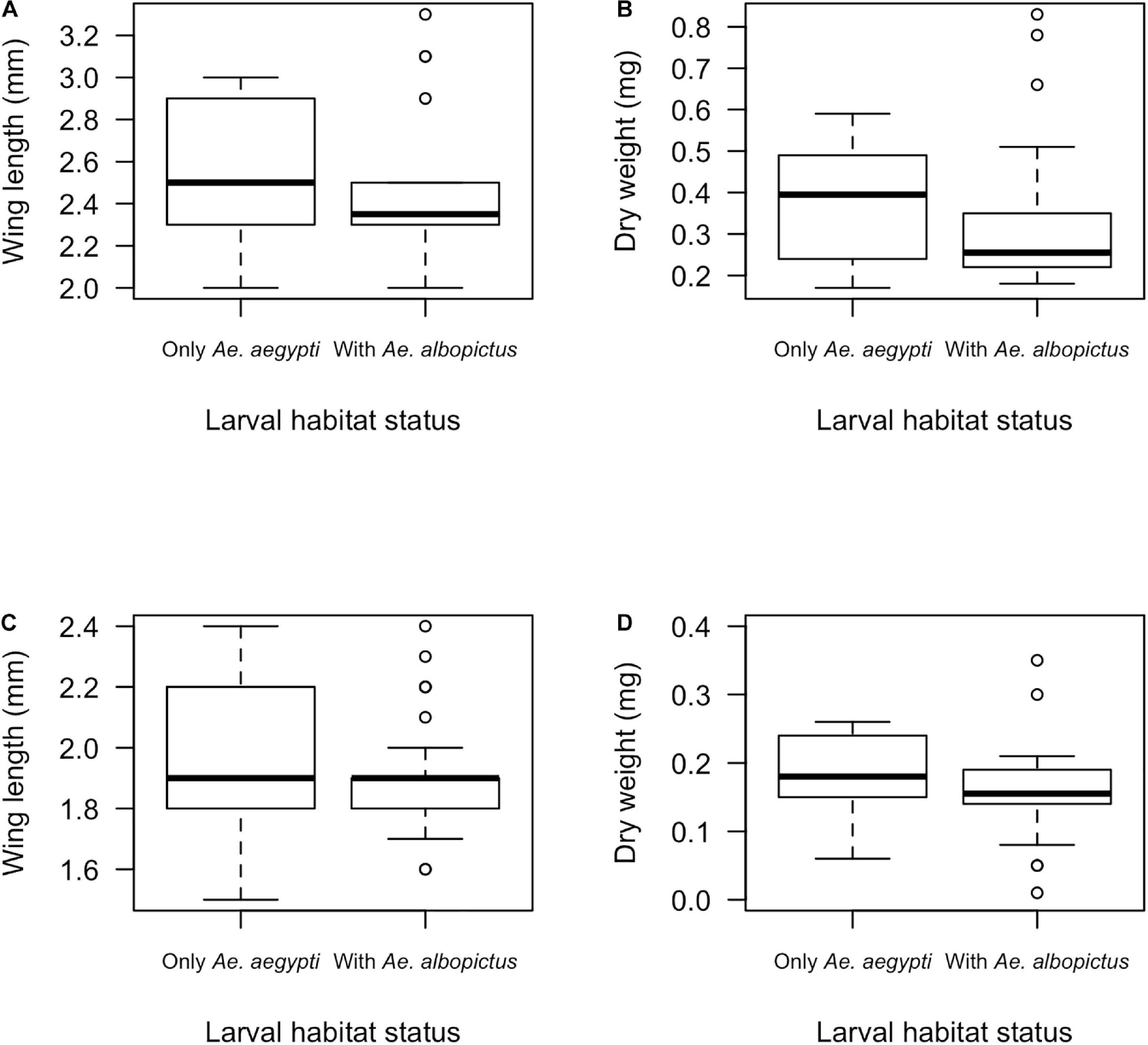
Figure 2. Wing length and dry weight of female (A,B) and male Ae. aegypti (C,D) according the presence of no other species or the co-occurrence with Ae. albopictus in the larval habitat. No significant differences in body measurements were observed.
Discussion
In the present study, we addressed the association between physicochemical properties of larval habitats and Culicidae immature abundance and Ae. aegypti adult body size. We showed that domestic containers from four distinct urban landscapes in Rio de Janeiro were massively colonized by Ae. aegypti, which accounted for >90% of adults emerging from field collected pupae. Moreover, the abundance of immature Culicidae was positively associated with container volume and the DOC concentration. Although the habitat parameters measured explained a small fraction (≤15%) of the data variation for Ae. aegypti body size, female average dry weight and male and female wing lengths were positively associated with larval habitat temperature whereas male average dry weight was positively related to water conductivity. Lastly, Ae. aegypti originating from larval habitats shared with Ae. albopictus exhibited no differences in median wing length and dry body weight when compared to specimens collected in containers exclusively colonized by Ae. aegypti.
Female Ae. aegypti has opportunistic egg-laying behavior, i.e., lay eggs in water-holding containers of varied characteristics. Here, we demonstrate that through RF classification and PCA that larval habitats differed in some physiochemical characteristics according to the geographical origin, but not according to container category and the presence of Ae. aegypti. Studies conducted in different environments showed a fuzzy association between physicochemical characteristics of larval habitats and container types. However, differences in some parameters such as total dissolved solids, water hardness, electrical conductivity, chemical oxygen demand and conductivity could be linked to container nature (i.e., natural or artificial) and water origin (Chatterjee et al., 2015; Gnanasoundari et al., 2017; Hery et al., 2020).
Potential differences in physiochemical parameters between positive and negative containers for Ae. aegypti could indicate oviposition preferences by female mosquitoes, egg hatching rate, immature survival and/or modifications of water following the Ae. aegypti colonization. Larvae development for several generations have been linked, for example, to the decrease of water turbidity, which would increase the activity of nitrifying bacteria and consequently the concentration of nitrate and nitrite ions (Darriet and Corbel, 2008). Nevertheless, any differentiation between positive and negative containers for Ae. aegypti immature was notice by the PCA. This result might be explained by sampling methods, since we had no control for how many generations these water containers were colonized by Ae. aegypti, i.e., whether it was long enough to cause any change in water and sediment parameters.
Containers exhibited differences in water physiochemical profile according to the neighborhood of location, with TUB larval habitats forming a more heterogenous cluster in relation to CUR, PRA, and VILA. Water temperature and volume, as well as the light incidence, were the most important variables distinguishing larval habitats according to its geographical location. This observation is in line with the high environmental temperatures (>35°C) usually registered in TUB in comparison to other locations in Rio de Janeiro (e.g., Dutra et al., 2015; Garcia et al., 2019). Sampling during April (autumn in Rio de Janeiro) might also have contributed to higher water temperatures in TUB comparison to the other field sites, which were sampled between June and August (autumn and winter). Median container volume was also higher in TUB, what might have been influenced by the 23.3% of domestic water storage containers sampled in TUB. Although PRA also had a high prevalence of this container category (35%), TUB houses were bigger than in PRA and as a consequence, residents usually stored water in larger containers (personal observation).
The abundance of Culicidae immature increased with container water volume and dissolved organic carbon (DOC) concentration. The container size has been recognized as an important predictor of the number of eggs laid by Ae. aegypti under lab and semi-field conditions, as day active mosquitoes would choose oviposition sites based on visual cues (Harrington et al., 2008; Panigrahi et al., 2014). Moreover, large containers are expected to provide more food (due to larger submerged surface and input of organic matter), as well as be more resistant to desiccation. A positive association between Ae. aegypti immature abundance and water volume have also been described in Puerto Rico, Dominican Republic and Bangladesh (Barrera et al., 2006; González et al., 2019; Islam et al., 2019).
Food availability is one of the main attributes that regulate Ae. aegypti development (Tun-Lin et al., 2000). Here, the number of immature Culicidae was positively associated to the dissolved organic carbon (DOC) concentration in the larval habitat. A higher availability of organic matter usually results in a higher flow of energy via the food web (Benavides-Gordillo et al., 2019) and could have acted as an oviposition attractant for mosquito female (together with container size) and/or food source enabling increased immature survival. In addition, it might also serve as substrate for bacterial growth (Benavides-Gordillo et al., 2019). In the urban area of Iquitos, Peru, female Ae. aegypti laid more eggs in larger unmanaged containers with more organic material, characteristics that were expected to maximize the progeny growth and survival. Nevertheless, those larval habitats produced mosquitoes with lower pupation probability and smaller body size when compared to specimens from smaller unmanaged containers, which received less eggs. Thus, although more attractive for mosquito oviposition, the larger containers were considered more competitive habitats for Ae. aegypti (Wong et al., 2012).
Considering the influence of physiochemical parameters on Culicidae immature density, we are aware that our findings should extrapolated carefully to Ae. aegypti, as only the pupae were reared to the adult stage for taxonomic identification. However, the sampled container types are well known to be historically colonized mainly by Ae. aegypti in Rio de Janeiro (Maciel-de-Freitas et al., 2007b; David et al., 2009), fact that was confirmed by the >90% of pupae identified of being of this species. Since we have no evidence of differential survival between the immature Culicidae species found, we are confident that this high proportion of Ae. aegypti found in emerging adults can be extrapolated to immature forms of mosquitoes present in larval habitats.
Unlike the density of immature mosquitoes, whose ∼37% of the data variance could be explained by the GLMM, a smaller fraction (≤15%) of the variation in body size measurements could be related to the addressed physiochemical characteristics of larval habitats. Such finding reinforces the complex and dynamic nature of larval habitat suitability for mosquito development (Hemme et al., 2009). Even so, the wing length of both male and female Ae. aegypti, as well as female dry weight, exhibited a positive significant association with water temperature of larval habitats. Water temperature is a determining factor for the development and survival of immature Ae. aegypti (Christophers, 1960; Couret and Benedict, 2014). In addition, water temperature also can influence the production and survival of algae, bacteria and fungi, which are part of the diet of mosquito larvae, thus altering food availability in the larval habitat (Hemme et al., 2009). In the laboratory, optimal survival of Ae. aegypti occurred between 20 and 30°C, with significant decrease in body size in temperatures >25°C (Tun-Lin et al., 2000). Considering the four field sites, water median temperature was 21.7°C (22.7°C in average), while it was in median 25.7°C in TUB. In all cases, the water temperature may not have been high enough to cause any development acceleration and reduction in adult body size as seen in other lab (Tun-Lin et al., 2000; Mohammed and Chadee, 2011) and field studies such as Barrera et al., 2006 (in which water temperature was 29.3°C in average).
Male average dry weight showed a positive significant association with water conductivity, a commonly used proxy for the amount of dissolved nutrients. The amount of solids dissolved in the water release ions as organic fractions of solids are consumed leading to an increase in conductivity. Thus, it could be interpreted as an indirect measure of food availability for larvae and/or substrate for microorganism growth (Hery et al., 2020). Conductivity has been linked to the presence of Ae. aegypti in artificial containers and to the larval density (Chatterjee et al., 2015; Garcia-Sánchez et al., 2017), but it effects on mosquito development, nutrition and body size are still unknown.
Competition has been associated to Ae. aegypti body size reduction (Strickman and Kittayapong, 2003; Barrera et al., 2006; Wong et al., 2012). Surprisingly, there was no evidence for density dependent effects on adult body size in our study, as the total number of immature Culicidae did not show any association with Ae. aegypti wing length or dry weight. In addition, a non-significant reduction in mosquito body measurements was noticed after comparing specimens originating from containers shared with Ae. albopictus or exclusively colonized by Ae. aegypti, what might be carefully interpreted due to the low frequency of co-occurrence registered here. The strength of density-dependent effects on mosquito fitness (e.g., body size) and population dynamics are heterogenous between water containers in the field, as they vary in accordance with larval densities and food availability (Walsh et al., 2013). We hypothesized that the range of immature densities in the sampled larval habitats (0.03 immature/ml in average, with a maximum of 0.66 immature/ml) were below the larval habitats carrying capacity (i.e., larval growth was not constrained by the nutritional resources saturation), thus, density-dependent intraspecific competition effect was not strong enough to produce smaller mosquitoes, as seen by others (Barrera et al., 2006; Walsh et al., 2013).
In summary, this study expands the currently knowledge about the relation between Ae. aegypti immature density, adult body size and larval habitat characteristics in the field. Our results indicate that container water volume (an easily observed characteristic in the field) is a predictor of Ae. aegypti immature density. Although the contribution of variations in larval habitats in adult populations was not investigated here, our results reinforces the recommendation that eliminating or preventing mosquito access to larger containers have the potential to effectively reduce Ae. aegypti field populations. Similarly, DOC concentration (often higher in unmanaged water holding recipients) can also be a predictor of Ae. aegypti productivity. Larval habitat characteristics have important implications population dynamics, as well as biology parameters, such as body size, fecundity and survival, which ultimately influence vector capacity and pathogen transmission to human populations.
Data Availability Statement
The original contributions presented in the study are included in the article/Supplementary Material, further inquiries can be directed to the corresponding author/s.
Author Contributions
MD analyzed the data and drafted the manuscript. ED conducted field collections and chemical analysis of larval habitat water. RM-d-F contributed to study design and field collections. CC contributed to data analysis. AP contributed to chemical analysis of larval habitat water. RL-d-O conceived the study. All authors edited the manuscript and approved the submitted version.
Funding
This work was supported by the US National Institutes of Health Fogarty International Center FIRCA (Grant No. R03TW007446-01A1), Conselho Nacional de Desenvolvimento Científico e Tecnológico (Grant No. 12446/2018), Preventing and Combating the Zika Virus MCTIC/FNDCT-CNPq/MEC-CAPES/MS-Decit. (Grant No. 440929/2016-4), Fundação Carlos Chagas Filho de Amparo à Pesquisa do Estado do Rio de Janeiro (Grants Nos. E-26/203.064/2016 and E-26/201.335/2016). ED received a scholarship of Coordenação de Aperfeiçoamento de Pessoal de Nível Superior—CAPES (Finance Code 001). We are grateful for the support of the Coordination for the Improvement of Higher Education Personnel—CAPES, and Fiocruz (Programa Institucional de Internacionalização da Fiocruz PrInt Fiocruz-CAPES). The funders had no role in study design, data collection and analysis, decision to publish, or preparation of the manuscript.
Conflict of Interest
The authors declare that the research was conducted in the absence of any commercial or financial relationships that could be construed as a potential conflict of interest.
Acknowledgments
We thank the Rio de Janeiro City Health Department (Secretaria Municipal de Saúde do Rio de Janeiro, SMS-RJ), Reginaldo Rego, Roberto Peres, Renato Carvalho and Marcelo Celestino for contributions to the fieldwork. We also thank L. Philip Lounibos, Márcio G. Pavan, and Steven A. Juliano for insightful comments and suggestions in statistical analyses.
Supplementary Material
The Supplementary Material for this article can be found online at: https://www.frontiersin.org/articles/10.3389/fevo.2021.626757/full#supplementary-material
Supplementary Figure 1 | Linear regressions of mean wing length and mean weight per larval habitat for Ae. aegypti female and male mosquitoes.
Supplementary Figure 2 | Contribution of each environmental variable to the first (A) and second (B) components of PCA. The red dashed line indicates the expected average contribution if the contribution of the variables was uniform, the expected value would be 1/11 variables, i.e., 9.09%.
Supplementary Table 1 | Aedes aegypti positive containers sampled per neighborhood.
Supplementary Table 2 | Aedes aegypti negative containers sampled per neighborhood.
Supplementary Table 3 | Raw larval habitat data.
Supplementary Table 4 | Univariate generalized linear mixed models with environmental variables to predict the number of immature Culicidae in larval habitats.
Supplementary Table 5 | Descriptive analysis of immature abundance and mosquito body size according to field sites.
Supplementary Table 6 | Univariate linear mixed models with environmental variables to predict the average male Ae. aegypti dry weight per larval habitat.
References
Alto, B. W., Lounibos, L. P., Mores, C. N., and Reiskind, M. H. (2008). Larval competition alters susceptibility of adult Aedes mosquitoes to dengue infection. Proc. R. Soc. B Biol. Sci. 275, 463–471. doi: 10.1098/rspb.2007.1497
Barrera, R., Amador, M., and Clark, G. G. (2006). Ecological Factors Influencing Aedes aegypti (Diptera: Culicidae) Productivity in Artificial Containers in Salinas, Puerto Rico. J. Med. Entomol. 43, 484–492. doi: 10.1603/0022-2585200643
Bates, D., Mächler, M., Bolker, B. M., and Walker, S. C. (2015). Fitting linear mixed-effects models using lme4. J. Stat. Softw. 2015:v067. doi: 10.18637/jss.v067.i01
Benavides-Gordillo, S., Farjalla, V. F., González, A. L., and Romero, G. Q. (2019). Changes in rainfall level and litter stoichiometry affect aquatic community and ecosystem processes in bromeliad phytotelmata. Freshw. Biol. 64, 1357–1368. doi: 10.1111/fwb.13310
Bhatt, S., Gething, P. W., Brady, O. J., Messina, J. P., Farlow, A. W., Moyes, C. L., et al. (2013). The global distribution and burden of dengue. Nature 496, 504–507. doi: 10.1038/nature12060
Briegel, H. (1990). Metabolic relationship between female body size, reserves, and fecundity of Aedes aegypti. J. Insect Physiol. 36, 165–172. doi: 10.1016/0022-1910(90)90118-Y
Chadee, D. D., and Focks, D. A. (1997). Pupal Survey: An Epidemiologically Significant Surveillance Method for Aedes aegypti: An Example Using Data from Trinidad. Am. J. Trop. Med. Hyg. 56, 159–167. doi: 10.4269/ajtmh.1997.56.159
Chatterjee, S., Chakraborty, A., and Sinha, S. K. (2015). Spatial distribution & physicochemical characterization of the breeding habitats of Aedes aegypti in & around Kolkata, West Bengal, India. Indian J. Med. Res. 142, S79–S86. doi: 10.4103/0971-5916.176631
Christophers, S. R. (1960). A{ë}des Aegypti (L.), the Yellow Fever Mosquito: Its Life History, Bionomics, and Structure. New York: University Press Available online at: https://books.google.com.br/books?id=rnJkjgEACAAJ.
Clark, T. M., Flis, B. J., and Remold, S. K. (2004a). Differences in the effects of salinity on larval growth and developmental programs of a freshwater and a euryhaline mosquito species (Insecta: Diptera, Culicidae). J. Exp. Biol. 207, 2289–2295. doi: 10.1242/jeb.01018
Clark, T. M., Flis, B. J., and Remold, S. K. (2004b). pH tolerances and regulatory abilities of freshwater and euryhaline Aedine mosquito larvae. J. Exp. Biol. 207, 2289–2295. doi: 10.1242/jeb.01021
Clements, A. N. (1992). The Biology of Mosquitoes: Development, nutrition, and reproduction. London: Chapman and Hall.
Cohuet, A., Harris, C., Robert, V., and Fontenille, D. (2010). Evolutionary forces on Anopheles: what makes a malaria vector? Trends Parasitol. 26, 130–136. doi: 10.1016/j.pt.2009.12.001
Consoli, R. A. G. B., and de Oliveira, R. L. (1994). Principais mosquitos de importância sanitária no Brasil. 1st ed., ed. E. Fiocruz Rio de Janeiro: Editora FIOCRUZ doi: 10.7476/9788575412909
Couret, J., and Benedict, M. Q. (2014). A meta-analysis of the factors influencing development rate variation in Aedes aegypti (Diptera: Culicidae). BMC Ecol. 14:3. doi: 10.1186/1472-6785-14-3
da Saúde, M. (2009). Diretrizes Nacionais para a Prevenção e Controle de Epidemias de Dengue. Brasília: Ministério da saúde
Darriet, F., and Corbel, V. (2008). Attractive properties and physicochemical modifications of water following colonization by Aedes aegypti larvae (Diptera: Culicidae). Compt. Ren. Biol. 331, 617–622. doi: 10.1016/j.crvi.2008.05.003
David, M. R., Lourenço-de-Oliveira, R., Freitas, R. M., and De (2009). Container productivity, daily survival rates and dispersal of Aedes aegypti mosquitoes in a high income dengue epidemic neighbourhood of Rio de Janeiro: presumed influence of differential urban structure on mosquito biology. Mem. Inst. Oswaldo Cruz 104, 927–932.
Dutra, H. L. C., dos Santos, L. M. B., Caragata, E. P., Silva, J. B. L., Villela, D. A. M., Maciel-de-Freitas, R., et al. (2015). From Lab to Field: The Influence of Urban Landscapes on the Invasive Potential of Wolbachia in Brazilian Aedes aegypti Mosquitoes. PLoS Negl. Trop. Dis. 9:e0003689. doi: 10.1371/journal.pntd.0003689
Farjana, T., and Tuno, N. (2013). Multiple Blood Feeding and Host-Seeking Behavior in Aedes aegypti and Aedes albopictus (Diptera: Culicidae). J. Med. Entomol. 50, 838–846. doi: 10.1603/ME12146
Garcia, G., de, A., Sylvestre, G., Aguiar, R., da Costa, G. B., Martins, A. J., Lima, J. B. P., et al. (2019). Matching the genetics of released and local Aedes aegypti populations is critical to assure Wolbachia invasion. PLoS Negl. Trop. Dis. 13(1):e0007023. doi: 10.1371/journal.pntd.0007023
Garcia-Sánchez, D. C., Pinilla, G. A., and Quintero, J. (2017). Ecological characterization of Aedes aegypti larval habitats (Diptera: Culicidae) in artificial water containers in Girardot, Colombia. J. Vector Ecol. 42, 289–297. doi: 10.1111/jvec.12269
Garrett-Jones, C. (1964). the Human Blood Index of Malaria Vectors in Relation To Epidemiological Assessment. Bull. World Health Organ. 30, 241–261.
Gnanasoundari, A, Thangamathi, P, and Ananth, S. (2017). Physico-Chemical Characteristics of Breeding Habitats and its Influence in Larval Population Density of Dengue Vector Aedes Aegypti in Thanjavur, Tamilnadu, India. Int. J. Res. Appl. Nat. Soc. Sci. 7, 69–76.
González, M. A., Sosa, M. A. R., Bautista, Y. E. V., Fernández, L. D., de Prada, M. B., Guerrero, K. A., et al. (2019). Micro-environmental features associated to container-dwelling mosquitoes (Diptera: Culicidae) in an urban cemetery of the Dominican republic. Rev. Biol. Trop. 67, 132–145. doi: 10.15517/RBT.V67I1.33158
Harbach, R., and Knight, K. (1980). Taxonomists’ Glossary of Mosquito Anatomy. Marlton, New Jersey: Plexus Publishing.
Harrington, L. C., Edman, J. D., and Scott, T. W. (2001). Why do female Aedes aegypti (Diptera: Culicidae) feed preferentially and frequently on human blood? J. Med. Entomol. 38, 411–22.
Harrington, L. C., Ponlawat, A., Edman, J. D., Scott, T. W., and Vermeylen, F. (2008). Influence of Container Size, Location, and Time of Day on Oviposition Patterns of the Dengue Vector, Aedes aegypti, in Thailand. Vect. Borne Zoonotic Dis. 8, 415–424. doi: 10.1089/vbz.2007.0203
Hartig, F. (2020). DHARMa: Residual Diagnostics for Hierarchical (Multi-Level / Mixed) Regression Models. URL: Available online at: https://cran.r-project.org/package=DHARMa.
Hemme, R. R., Tank, J. L., Chadee, D. D., and Severson, D. W. (2009). Environmental conditions in water storage drums and influences on Aedes aegypti in Trinidad, West Indies. Acta Trop. 112, 59–66. doi: 10.1016/j.actatropica.2009.06.008
Hery, L., Guidez, A., Durand, A. A., Delannay, C., Normandeau-Guimond, J., Reynaud, Y., et al. (2020). Natural Variation in Physicochemical Profiles and Bacterial Communities Associated with Aedes aegypti Breeding Sites and Larvae on Guadeloupe and French Guiana. Microb. Ecol. 81, 93–109. doi: 10.1007/s00248-020-01544-3
Hilbe, J., and Robinson, A. (2013). Methods of Statistical Model Estimation. 1st ed. New York: Chapman and Hall/CRC.
Islam, S., Haque, C. E., Hossain, S., and Rochon, K. (2019). Role of container type, behavioural, and ecological factors in Aedes pupal production in Dhaka, Bangladesh: An application of zero-inflated negative binomial model. Acta Trop. 193, 50–59. doi: 10.1016/j.actatropica.2019.02.019
Juliano, S. A. (2009). Species Interactions Among Larval Mosquitoes: Context Dependence Across Habitat Gradients. Annu. Rev. Entomol. 54, 37–56. doi: 10.1146/annurev.ento.54.110807.090611
Juliano, S. A., Ribeiro, G. S., Maciel-de-Freitas, R., Castro, M. G., Codeço, C., Lourenço-de-Oliveira, R., et al. (2014). She’s a femme fatale: Low-density larval development produces good disease vectors. Mem. Inst. Oswaldo Cruz. 109, 1070–1077. doi: 10.1590/0074-02760140455
Kraemer, M. U. G., Sinka, M. E., Duda, K. A., Mylne, A. Q. N., Shearer, F. M., Barker, C. M., et al. (2015). The global distribution of the arbovirus vectors Aedes aegypti and Ae. Albopictus. Elife. 4:e08347. doi: 10.7554/eLife.08347
Loeb, S. L. (1981). An in situ method for measuring the primary productivity and standing crop of the epilithic periphyton community in lentic systems. Limnol. Oceanogr. 26, 394–399. doi: 10.4319/lo.1981.26.2.0394
Lourenço-de-Oliveira, R., Castro, M. G., Braks, M. A. H., and Lounibos, L. P. (2004). The invasion of urban forest by dengue vectors in Rio de Janeiro. J. Vector Ecol. 29, 94–100. Available online at: http://www.ncbi.nlm.nih.gov/pubmed/15266746.
Macdonald, W. W. (1956). Aedes aegypti in Malaya. II. Larval and adult biology. Ann. Trop. Med. Parasitol. 50, 399–414.
Maciel-De-Freitas, R., Codeço, C. T., and Lourenço-De-Oliveira, R. (2007a). Body size-associated survival and dispersal rates of Aedes aegypti in Rio de Janeiro. Med. Vet. Entomol. 21, 284–292. doi: 10.1111/j.1365-2915.2007.00694.x
Maciel-de-Freitas, R., Marques, W. A, Peres, R. C., Cunha, S. P., and de Oliveira, R. L. (2007b). Variation in Aedes aegypti (Diptera: Culicidae) container productivity in a slum and a suburban district of Rio de Janeiro during dry and wet seasons. Mem. Inst. Oswaldo Cruz 102, 489–96.
Mazerolle, M. (2019). Model selection and multimodel inference based on (Q)AIC(c). R package version 2.2-2. Available from https://cran.r-project.org/package=AICcmodavg. R Packag.
Medeiros-Sousa, A. R., de Oliveira-Christe, R., Camargo, A. A., Scinachi, C. A., Milani, G. M., Urbinatti, P. R., et al. (2020). Influence of water’s physical and chemical parameters on mosquito (Diptera: Culicidae) assemblages in larval habitats in urban parks of São Paulo, Brazil. Acta Trop. 205:105394. doi: 10.1016/j.actatropica.2020.105394
Messina, J. P., Kraemer, M. U., Brady, O. J., Pigott, D. M., Shearer, F. M., Weiss, D. J., et al. (2016). Mapping global environmental suitability for Zika virus. Elife 5:15272. doi: 10.7554/eLife.15272
Meyer, D., Zeileis, A., and Hornik, K. (2020). vcd: Visualizing Categorical Data. Available online at: https://cran.r-project.org/package=vcd.
Mohammed, A., and Chadee, D. D. (2011). Effects of different temperature regimens on the development of Aedes aegypti (L.) (Diptera: Culicidae) mosquitoes. Acta Trop. 119, 38–43. doi: 10.1016/j.actatropica.2011.04.004
Nasci, R. S. (1991). Influence of larval and adult nutrition on biting persistence in Aedes aegypti (Diptera: Culicidae). J. Med. Entomol. 28, 522–526. doi: 10.1093/jmedent/28.4.522
Nsoesie, E. O., Kraemer, M. U., Golding, N., Pigott, D. M., Brady, O. J., Moyes, C. L., et al. (2016). Global distribution and environmental suitability for chikungunya virus, 1952 to 2015. Eurosurveillance 21:30234. doi: 10.2807/1560-7917.ES.2016.21.20.30234
Panigrahi, S. K., Barik, T. K., Mohanty, S., and Tripathy, N. K. (2014). Laboratory Evaluation of Oviposition Behavior of Field Collected Aedes Mosquitoes. J. Insects 2014, 1–8. doi: 10.1155/2014/207489
R Development Core Team. (2011). R: A Language and Environment for Statistical Computing. R Found. Stat. Comput. 1:409. doi: 10.1007/978-3-540-74686-7
Reiskin, M. H., and Lounibos, L. P. (2009). Effects of intraspecific larval competition on adult longevity in the mosquitoes Aedes aegypti and Aedes albopictus. Med. Vet. Entomol. 23, 62–68. doi: 10.1111/j.1365-2915.2008.00782.x
Rueda, L. M., Patel, K. J., Axtell, R. C., and Stinner, R. E. (1990). Temperature-dependent development and survival rates of Culex quinquefasciatus and Aedes aegypti (Diptera: Culicidae). J. Med. Entomol. 27, 892–898. doi: 10.1093/jmedent/27.5.892
Sasmita, H. I., Tu, W.-C., Bong, L.-J., and Neoh, K.-B. (2019). Effects of larval diets and temperature regimes on life history traits, energy reserves and temperature tolerance of male Aedes aegypti (Diptera: Culicidae): optimizing rearing techniques for the sterile insect programmes. Parasit. Vectors 12:578. doi: 10.1186/s13071-019-3830-z
Schneider, J. R., Morrison, A. C., Astete, H., Scott, T. W., and Wilson, M. L. (2004). Adult Size and Distribution of Aedes aegypti (Diptera: Culicidae) Associated with Larval Habitats in Iquitos, Peru. J. Med. Entomol. 41, 634–642. doi: 10.1603/0022-2585-41.4.634
Scott, T. W., Amerasinghe, P. H., Morrison, A. C., Lorenz, L. H., Clark, G. G., Strickman, D., et al. (2000). Longitudinal Studies of Aedes aegypti (Diptera: Culicidae) in Thailand and Puerto Rico: Blood Feeding Frequency. J. Med. Entomol. 37, 89–101. doi: 10.1603/0022-2585-37.1.89
Strickman, D., and Kittayapong, P. (2003). Dengue and its vectors in Thailand: Calculated transmission risk from total pupal counts of Aedes aegypti and association of wing-length measurements with aspects of the larval habitat. Am. J. Trop. Med. Hyg. 68, 209–217. doi: 10.4269/ajtmh.2003.68.209
Tun-Lin, W., Burkot, T. R., and Kay, B. H. (2000). Effects of temperature and larval diet on development rates and survival of the dengue vector Aedes aegypti in north Queensland, Australia. Med. Vet. Entomol. 14, 31–7.
Valderrama, J. C. (1981). The simultaneous analysis of total nitrogen and total phosphorus in natural waters. Mar. Chem. 10, 109–122. doi: 10.1016/0304-4203(81)90027-X
Walsh, R. K., Aguilar, C. L., Facchinelli, L., Valerio, L., Ramsey, J. M., Scott, T. W., et al. (2013). Regulation of aedes aegypti population dynamics in field systems: Quantifying direct and delayed density dependence. Am. J. Trop. Med. Hyg. 89, 68–77. doi: 10.4269/ajtmh.12-0378
Wong, J., Morrison, A. C., Stoddard, S. T., Astete, H., Chu, Y. Y., Baseer, I., et al. (2012). Linking oviposition site choice to offspring fitness in Aedes aegypti: Consequences for targeted larval control of dengue vectors. PLoS Negl. Trop. Dis. 6:e1632. doi: 10.1371/journal.pntd.0001632
Yee, D. A., Kesavaraju, B., and Juliano, S. A. (2006). Interspecific Differences in Feeding Behavior and Survival Under Food-Limited Conditions for Larval Aedes albopictus and Aedes aegypti (Diptera: Culicidae). Ann. Entomol. Soc. Am. 97, 720–728. doi: 10.1603/0013-87462004097
Keywords: Aedes aegypti, larval habitat, larval density, body size, field ecology
Citation: David MR, Dantas ES, Maciel-de-Freitas R, Codeço CT, Prast AE and Lourenço-de-Oliveira R (2021) Influence of Larval Habitat Environmental Characteristics on Culicidae Immature Abundance and Body Size of Adult Aedes aegypti. Front. Ecol. Evol. 9:626757. doi: 10.3389/fevo.2021.626757
Received: 06 November 2020; Accepted: 28 January 2021;
Published: 19 February 2021.
Edited by:
Andre Barretto Bruno Wilke, University of Miami, United StatesReviewed by:
Shannon L. LaDeau, Cary Institute of Ecosystem Studies, United StatesGabriel L. Hamer, Texas A&M University, United States
Copyright © 2021 David, Dantas, Maciel-de-Freitas, Codeço, Prast and Lourenço-de-Oliveira. This is an open-access article distributed under the terms of the Creative Commons Attribution License (CC BY). The use, distribution or reproduction in other forums is permitted, provided the original author(s) and the copyright owner(s) are credited and that the original publication in this journal is cited, in accordance with accepted academic practice. No use, distribution or reproduction is permitted which does not comply with these terms.
*Correspondence: Mariana Rocha David, bWFyaWRhdmlkQGlvYy5maW9jcnV6LmJy
†These authors have contributed equally to this work