- 1Changjiang River Scientific Research Institute, Wuhan, China
- 2Key Laboratory of River Regulation and Flood Control of MWR, Wuhan, China
After the construction of the Three Gorges Dam (TGD) in China, the downstream has been affected by the reduction in sediment discharge and regulation of flow processes, which have resulted in severe scouring and changes hydrological regime. Consequently, the spawning ground of Chinese sturgeon distributed along the downstream Yichang reach could be affected. This study examined the effects of TGD on the streamflow, sediment load and channel morphology downstream based on in situ measured data. Results showed that, after the impoundment of the TGD, sediment load at the downstream Yichang hydrological station decreased significantly, and the Yichang reach continued to be scoured. The distribution of erosion was uneven, and the scouring mainly occurred in the branching channels. The channel gradient and riverbed roughness increased with the erosion of the river cross section. After more than 10 years of erosion, the riverbed scouring and armouring in the Yichang reach was basically completed, thus we expected that the spawning grounds of Chinese sturgeon could be retain as the riverbed tends to be stable. The findings in this work have implications in the protection of the critically endangered Chinese sturgeon.
Introduction
The Three Gorges Reservoir (TGR) is one of the largest water projects in the world. While it plays an important role in flood control and water utilisation, the TGR dramatically changes the incoming water and sediment conditions in the downstream reaches (Yang et al., 2007a; Zhang et al., 2017; Guo et al., 2019). Owing to the storage and regulation performed by the reservoir, extensive sediment is deposited in the reservoir, thereby significantly reducing the sediment concentration of the flow discharged downstream. The sediment carrying capacity of the water flow is severely sub-saturated. Riverbed scouring and armouring critically impacts the flood control, navigation, ecology, and environment of the lower reaches (Friedman et al., 1998; Yang et al., 2014; Zhou et al., 2014).
Global researches demonstrated that about 30% of fish species in freshwater have become endangered, threatened, or extinct in the past few decades, which is much worse than in marine ecosystems (Liermann et al., 2012). Chinese sturgeon Acipenser sinensis, mainly lives in the mainstream of Yangtze river, the continental shelf of East China Sea and Yellow Sea. It is one of the first-class national protected animals in China, and has been classified as Critically Endangered in the IUCN Red List of Threatened Species. The spawning grounds of Chinese sturgeon were in the upper Yangtze river and the Jinshajiang river, covering a stretch of at least 600 km of the river length. However, after the construction of Gezhouba Dam (GD) in January 1981, Chinese sturgeon could not go to the previous spawning grounds and fish alternatively spawned within a 30 km section below the GD, which is much smaller than the original spawning ground in the upper reach of the Yangtze river (Chang, 1999; Wang et al., 2013). In 2003, the TGR (∼38 km upstream of Gezhouba Dam) began to operate, resulting in a large change in the downstream hydrological regime (Guo et al., 2012, 2020). It is urgent to protect Chinese sturgeon from extinction as the population of this endangered species in the Yangtze river has declined remarkably in recent decades due to the influences of habitat loss, overfishing, and hydrologic regime changes (Zhuang et al., 2016; Shen et al., 2018). The new spawning ground plays a crucial role in preserving Chinese sturgeon species. Thus, the conservation of this area is crucial.
Because of its importance, many researchers have studied the evolution and hydrodynamic characteristics of this reach. Li et al. (2011) analysed its flow-sediment conditions. Zhou et al. (2017) studied the shape of its cross section and variation in flat discharge and considered that the cross section tends to be narrower and deeper. However, other researchers (Zhang et al., 2013; Han et al., 2017; Xia et al., 2017) focused on the entire middle and lower reaches of the Yangtze River. Most of the riverbed in the middle and lower reaches of the Yangtze River have sandy materials, which are easy to deform, while only the Yichang reach has a sandy pebble riverbed in the middle reaches. In addition to morphological changes, bed sediment armouring often occurs in the scouring state (Doyle and Harbor, 2003; Yuan et al., 2012; Li et al., 2019). However, the impacts of the specific variations of hydraulic condition and channel morphology on the spawning ground of Chinese sturgeon just a few 10 km downstream of the GD remain unclear. Although the spawning behaviour in the downstream site next to GD has been detected after the impoundment of TGD, the spawning has been lowered in quantity and quality, moreover, the natural spawning activities of Chinese sturgeon has been interrupted for 3 consecutive years from 2017 to 2019. Therefore, it is necessary to figure out the effects of the newest changes in the river regime, riverbed materials, and response to flow conditions in the suitability of the only existed spawning ground for Chinese sturgeon. Our objectives were to analyse the impact of the impoundment of TGD on the water-sediment conditions and riverbed evolution of the Yichang reach and their influences on the spawning ground of Chinese sturgeon recently based on measured data from 2003 to 2018. It is expected to provide reference for the protection of Chinese sturgeon spawning grounds and the evolution of sandy pebble riverbed downstream of other dams.
Study Area and Methods
Study Area
The Yangtze river is the longest river in China with a total length of ∼6,300 km, and it provides essential habitat for many fish species. According to the geographic and hydrological properties, the Yangtze river is usually divided into upper, middle and lower reaches with divisions at Yichang and Hukou (Figure 1A).
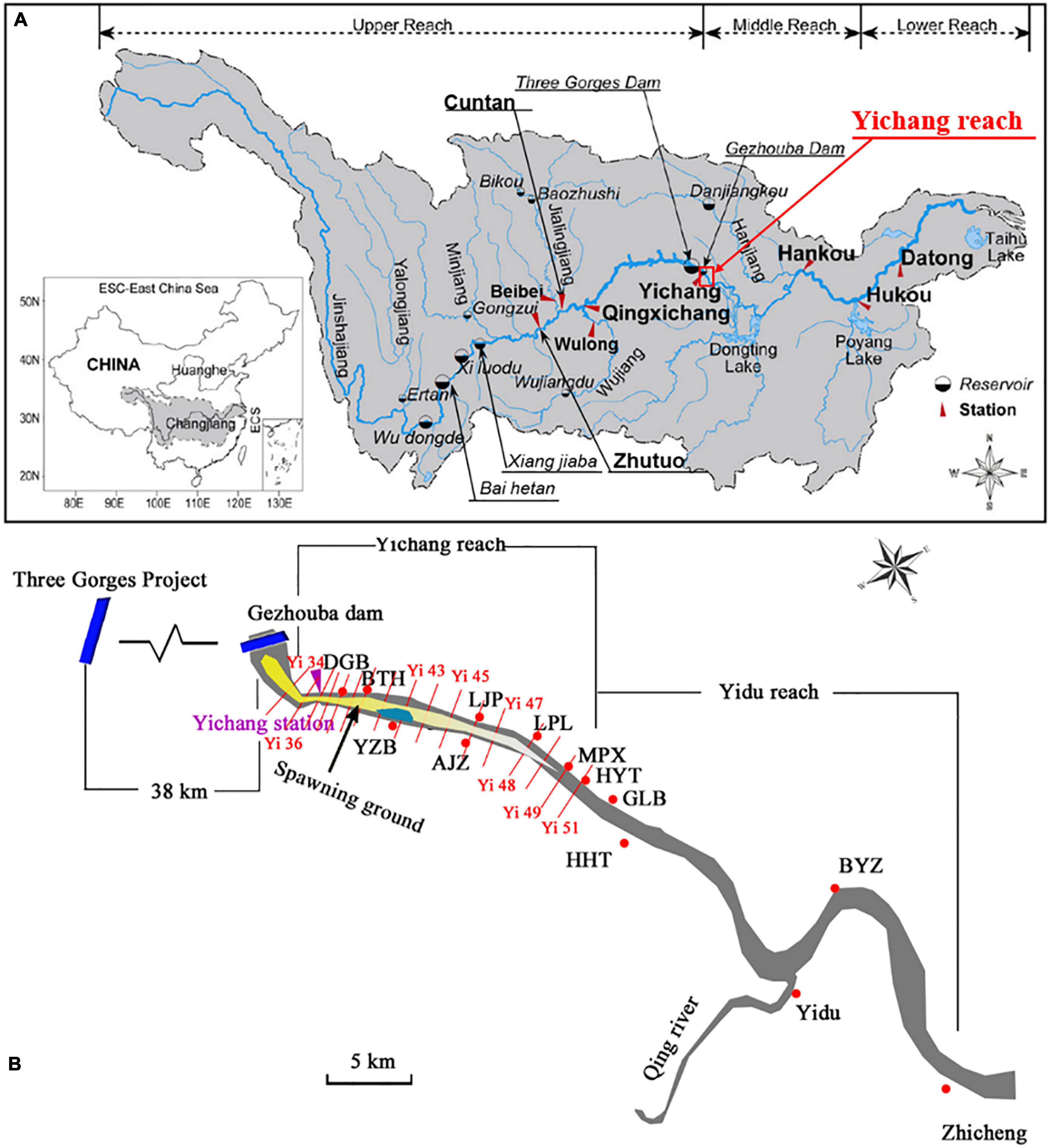
Figure 1. (A) Boundaries of the Yangtze River Basin. (B) Map of the Yichang reach. The yellow area in (B) shows the spawning ground of Chinese sturgeon, and red lines indicate the cross sections from Yi34-Yi51 without Yi35. DGB, Dagong Bridge; BTH, Baotahe; YZB, Yanzhiba; AJZ, Aijiazhen; LPL, Longpan Lake; MPX, Mopanxi; HYT, Huyatan; GLB, Gulaobei; HHT, Honghuatao.
The Three Gorges Dam (TGD) locates at the outlet of the upper Yangtze river, approximately 40 km upstream of the Yichang hydrological station. The storage capacity of TGR is about 393 × 108 m3, corresponding to its normal impoundment water level of 175 m. The GD locates about 38 km downstream of the TGD, and it is the largest runoff hydropower station with low water head and large flow discharge in the world. The GD was China’s largest hydroelectric facility with a total storage capacity of 15.8 × 108 m3 until the completion of the TGR project.
As the spawning ground of Chinese sturgeon have been changed to downstream of GD since 1982, the Yichang reach was selected as the study area.
The Yichang reach is located at the entrance of the middle reaches of the Yangtze river, which is a transition section from a mountainous river to a plain river. Restricted by the low mountains and hills on both sides of the river, the trend of the entire reach is from northwest to southeast. This reach starts at the GD and ends near Gulaobei (GLB), with a length of ∼30 km. The Yichang reach is generally straight, with Yanzhiba (YZB) forming a river island. The deep channel is close to the left bank, which is submerged and exposed to water in normal water periods. Hence, the Yangtze river is divided into left and right branches. The left branch is the main branch, whereas the right branch is cut off in dry seasons (Figure 1B).
Data Acquisition
Streamflow and sediment in the Yichang reach mainly come from the upper Yangtze river. Annual hydrological data including flow discharge, sediment load, and median particle size since 1950 were collected at the hydrometric station of Yichang, which is located just a few kilometers downstream of the GD (Figure 1B). The data used in the present study were obtained from the Changjiang Water Resources Commission (CWRC)1, and their consistency was verified.
In order to calculate the cumulative volume of channel deformation and analysis the plane change, the post-flood cross-sectional profiles surveyed at 17 fixed locations annually since 2002 were collected in the study reach, with the section number ranged from Yi34- Yi51 (without Yi35). The distance between two consecutive sections varies from about 1–3 km, with a mean spacing of around 2 km.
Methods
This study analysed the erosion and deposition volume and morphological changes of the river channel, riverbed armouring, gradient changes, and resistance changes.
The cross-section morphology method could accurately reflect the volume changes and distribution circumstances along the main channel of the rivers, as well as the beach and whole reach area on the cross-sections. Thus, the volume of erosion and deposition was calculated using the cross-section morphology method (Xia et al., 2017; Yuan et al., 2018). By cutting out several cross sections of the river channel, assuming that the changes between two adjacent sections are gradual. The storage capacity between sections Vi can be calculated using a trapezoidal formula as:
where Ai and Ai + 1 indicate the areas of the ith and i + 1th section, respectively. ΔL is the distance between the ith and i + 1th sections. Differences between Vi under varied years show the volume of erosion (negative) or deposition (positive).
When the circumstances of water and sediment changed, the river channel would usually gradually reach a new balance state through continuous self-adjustments. The fluvial facies relationship is known as a quantitative relationship between the section morphology and longitudinal profile (Yuan et al., 2018). In this study, the fluvial facies coefficient ζ was used to represent the morphological variation, which was expressed using the width to depth ratio as:
where B is the river width, h is the water depth.
Riverbed armouring is reflected by changes in the characteristic particle size of the riverbed materials (Doyle and Harbor, 2003).
The change in roughness was back-calculated using the Manning model:
where = the mean area river of import and export cross section in the channel, m2; = the mean discharge of import and export cross section, m3/s; = the mean hydraulic radius of import and export cross section, m; and J = the flow surface slope.
Results
Streamflow and Sediment Changes
The Yichang hydrological station is the control station. Figure 2 shows the variation in annual runoff and sediment load over time at Yichang station. The annual runoff at Yichang station fluctuated yearly with no clear trend, but the annual sediment load has been decreasing significantly since around 2001 when the TGD was closed to operation.
Before the impoundment of the GD (1950–1980), the multi-year average runoff and sediment load at Yichang station was 4,518 × 108 m3and 5.15 × 108 t, respectively. After the impoundment of the GD and before the impoundment of the TGR (1981–2002), the multi-year average runoff at Yichang station was 4,348 × 108 m3, and the mean annual sediment load was 4.59 × 108 t. Before the impoundment of the TGR, the annual runoff and sediment load at Yichang station underwent minor changes. Since the operation of the TGR (2003–2018), the annual runoff at Yichang station fluctuated around the average value over many years with minor changes, while the annual sediment load declined sharply down to about 0.36 × 108 t.
The variations of impoundment phases of the TGR was as follows: the storage level was raised to 135 m in 2003, and the cofferdam power generation period was initiated (period A). Then in October 2006, the storage level reached 156 m, marking the start of the initial storage period (period B). Two years later, the TGR began to impound water to the normal level of 175 m on September, 2008, known as the 175 m trial storage period (period C) (Ren et al., 2021). From 2003 to 2018, the outflow sediment became thinned. As shown in Figure 3, during the power generation period at the cofferdam, the mean particle size of the outflow sediment decreased sharply from 0.044 to 0.014 mm. The proportion of particles finer than 0.031 mm increased from 75.3 to 86.7%, and the proportion of particles larger than 0.125 mm decreased from 14 to 5.4%. The mean and median particle sizes of the outflow sediment had minor changes in the period B of the initial storage period from 2006 to 2008, and the proportion of coarse sediment continued to decrease. While during the trial storage period from October 2008 to December 2018, the mean and median particle sizes of suspended sediment slightly increased. In 2018, the median particle size at Yichang station was 0.009 mm, and the coarse-grained sediment content was 0.5%.
Since the TGR has been in operation, the changes in median and mean particle sizes of the suspended sediment during different periods basically demonstrated the same patterns. In other words, the discharged sediment became finer. During the power generation period at the cofferdam, the difference between the median and mean particle sizes of the suspended sediment discharged from the reservoir was large, indicating that the degree of non-uniformity of sediment gradation was large. However, in both initial and trial storage periods, the difference between the median and mean particle sizes decreased, indicating that, owing to the reservoir regulation, the non-uniformity of the suspended sediment discharged from the reservoir was reduced. From January 2013 to December 2018, the mean particle size of suspended sediment increased, but it was notably smaller than that during the cofferdam impoundment period. Further, the median particle size exhibited no significant changes.
Riverbed Erosion and Deposition
The shoreline of the Yichang reach is composed of hilly terraces with strong impact resistance. With the control of bedrock nodes along the course, the overall river regime has been stable over the years, and the main stream has been relatively stable.
Since the impoundment of the TGR, the channel was mainly in the scouring state. Analysis of the main flow line changes demonstrated that the flow in this section was relatively smooth, and no large backflow areas were observed. During the high water periods, the main stream was in the middle of the channel and could submerge the YZB. The maximum flow velocity was observed at the tail of YZB. During the normal and low water periods, branching flow was formed at the head of YZB, and the main stream flowed to the left channel. The maximum velocity happened at the head of YZB. During the low water period, the main stream started from the GD, and turned right to the head of YZB. After flowing out of YZB, the main stream slightly tended to the right bank during the low water period and tended toward the centre of the channel during medium and high water periods.
With the normal operation of the TGR, the scouring intensity of the riverbed in this section of the river gradually decreased, and the scouring slowly moved downstream. The scouring in the YZB reach was the most evident from 2002 to 2004, and the scouring intensity of the entire reach decreased slightly after 2004. Since the initial storage period of the TGR, the riverbed between the Yichang station and YZB presented a state of alternation of scouring and deposition until 2016, with a small change range. From YZB to Huyatan (HYT), the erosion remained dominant, and the location of erosion was significant in the YZB section. Table 1 shows the calculations of sectional erosion and deposition amount in the Yichang reach. It can be seen that from 2002 to 2016, the Yichang reach presented a scouring state, and the cumulative amount of erosion was 1,565 × 104 m3. Among them, the erosion in the YZB section was the most severe, and the total amount of erosion attained 47.5% of the entire Yichang reach (Table 1).
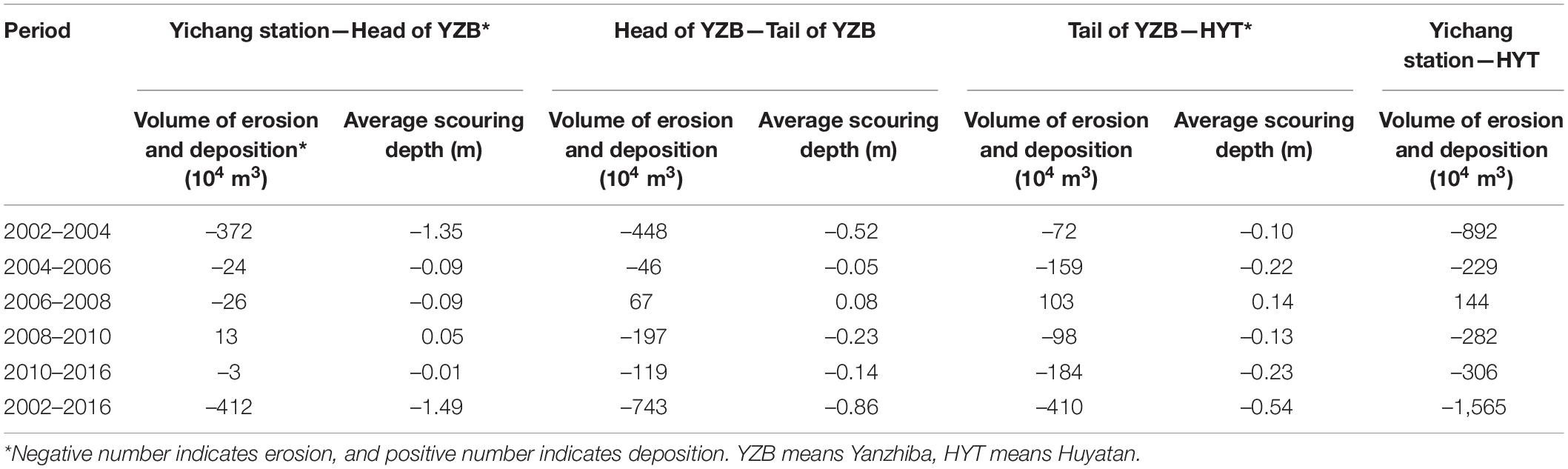
Table 1. Statistics of the erosion and deposition volume in the Yichang reach at the water level of 43.3 m in different periods.
Plane Changes
Thalweg
The thalweg line of the Yichang reach started from the GD, and it went down along the left trough of YZB and then gradually transited to the right bank after leaving YZB. In the vicinity of Longpan Lake (LPL), the thalweg line transited to the left bank and then turned to the right bank. It gradually transited to the left bank until reaching the Mopanxi (MPX). This trend was basically consistent with that of the main stream and underwent minor changes over the years (Figure 4). According to the change of main stream and thalweg, the interannual oscillation of the thalweg was small.
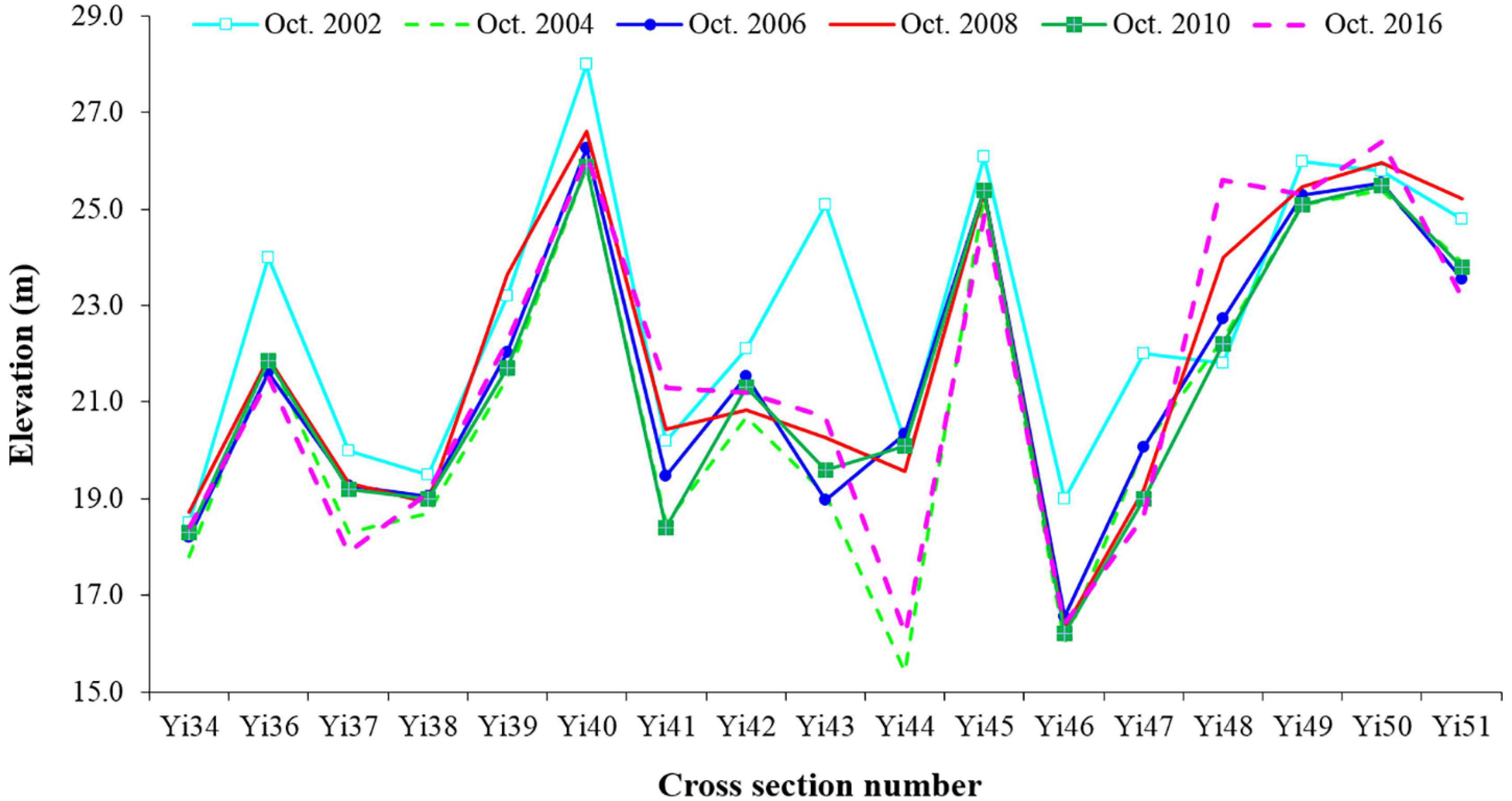
Figure 4. Thalweg variations along the Yichang reach before and after the impoundment of the Three Gorges Reservoir.
It was found that the river reach just downstream of the GD (sections Yi 34∼Yi 39) almost reached the bedrock, and the riverbed exhibited strong anti-scouring capability. The elevation variation range over the years was small, from 18 to 22 m. The changes of the thalweg were relatively large in the YZB reach (sections Yi 42∼Yi 44). From 2002 to 2008, the elevation of thalweg generally decreased by 2–5 m, while the descending rate of the thalweg slowed significantly from 2008 to 2010. In the reach below YZB, the thalweg descent slowed down after 2002, and the change of thalweg elevation was not significant from 2008 to 2010. From 2010 to 2016, the interannual variations of scouring and deposition of the reach increased, as well as the changes of longitudinal section of the thalweg.
Typical Marshland
The main river island of the Yichang reach is YZB, which is about 10.2 km away from the GD. YZB is a river island near the right bank, which stretches from Baotahe (BTH) down to Aijiazhen (AJZ). During both normal and high water periods, the YZB is submerged, whereas it is exposed during the low water period. After the normal impoundment of the GD and before the impoundment of the TGD, the YZB was basically stable, but the erosion and deposition processes were stronger than that of the natural river channel before the construction of the GD and TGD. In 2002, the 25 m contour lines of the upper and lower sections of the left branch channel were connected to form a complete 25 m deep channel. After 2002, the dam body of YZB was slightly scoured, and its area had been reduced, but the maximum length and width increased slightly. The maximum elevation of dam crest also increased slightly. After the impoundment of the TGD, the YZB was still generally stable, and its dam body erosion and shape change were relatively slow (Table 2).
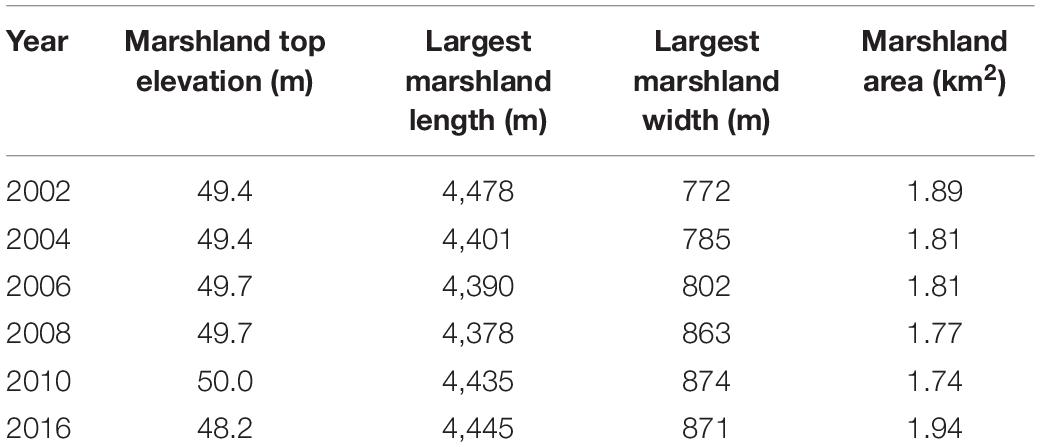
Table 2. Statistics of the characteristic values of Yanzhiba marshlands under the water depth of 39 m from 2002 to 2016.
Cross-Sectional Morphology
The cross-sectional morphology of the Yichang reach can be divided into three types: V shape for the curved section, U shape for the straight section, and W shape for the branching section. The main parts of erosion and deposition of each type of cross-section were the normal and low water parts of the main channel and side beach of the river bed, and the change of the part above the high water level is relatively small.
According to the analysis of the topographic data of the fixed section over time, the cross-sectional morphology of this reach changed greatly, and the fluvial facies coefficient was around 2–5. The width of the river changed from approximately 900 to 1,500 m, and the cross-sectional change was mainly reflected in the erosion and deposition in the deep channel. The statistics of cross-sectional elements of the normal-water channel in some sections of the Yichang reach showed that the fluvial facies coefficient in this section had a large change along the course and that of the YZB branching channel was large as the channel was wide and shallow. From 2002 to 2016, fluvial facies coefficient showed a decreasing trend, indicating that the river channel in this section tended to become narrower and deeper as the riverbed was undercut (Table 3).
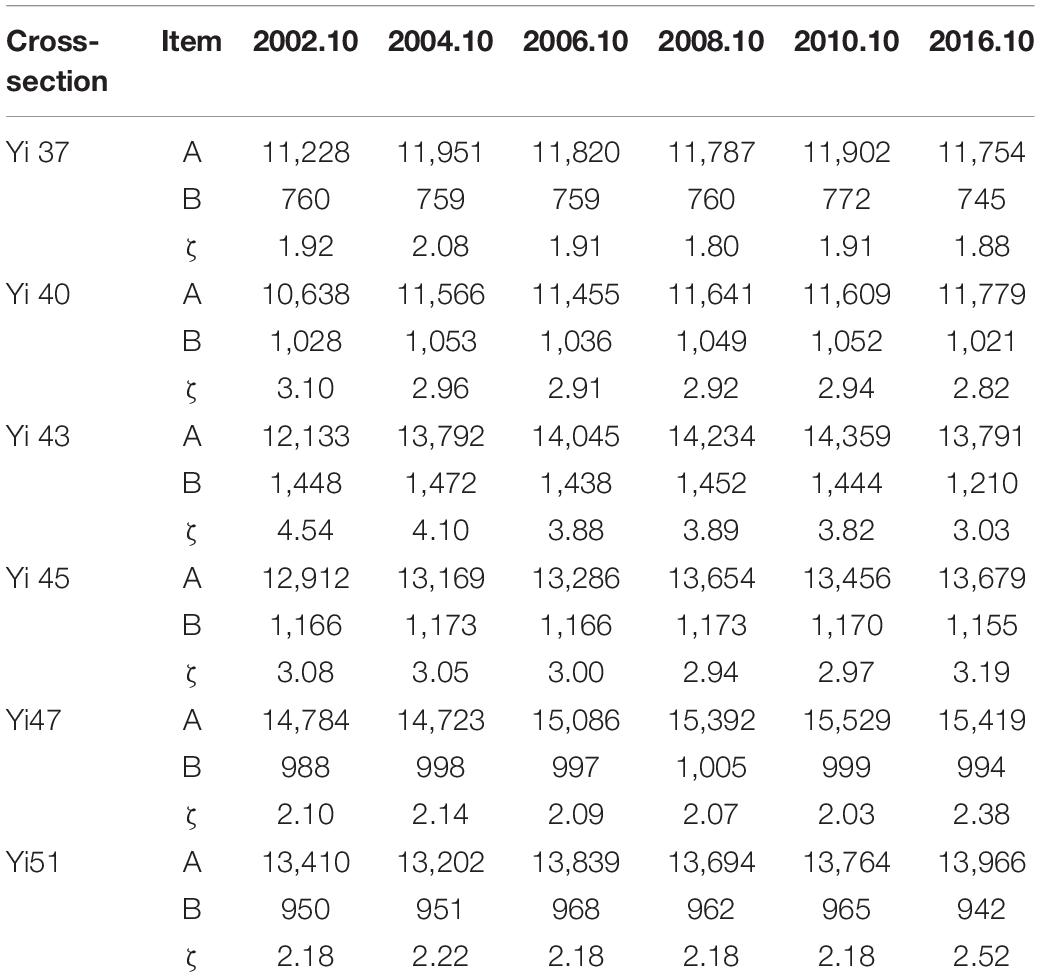
Table 3. Statistics of some cross-section elements of the Yichang reach under the water depth of 43.3 m.
As shown in Figure 5, the left and right shorelines of the Yichang reach were relatively stable, and the riverbed evolution trend was dominated by deep channel scouring. The erosion range was large. The deep groove expanded yearly and became deeper and longer, while the horizontal dimension remained unchanged overall. The shoals in the whole reach shrank to varying degrees, and the shrinkage amplitude of the river island was slightly less than that of the side beach. With the operation of the TGR, the scouring intensity of the reach was gradually weakened.
Discussion
Riverbed Armouring
Since the operation of the TGR, an overall temporal fining trend of the suspended sediment was found, which was in consistent with the decreasing sediment load tendency (Yang et al., 2014; Guo et al., 2020). In contrast, the riverbed materials coarsened significantly at Yichang station (Figure 6). The median size of riverbed materials increased from 0.638 mm in November 2003 to 23.59 mm in October 2012. The riverbed composition gradually evolved from sand or sandy pebble before impoundment to pebble with sand. Before the impoundment of the TGR, 99% of riverbed materials ranged from 0.062 mm to 0.5 mm. From 2003 to 2005, 99% of riverbed material sizes were between 0.125 and 1 mm, approximately twice as large as before impoundment. After 2006, the trend of riverbed armouring was more obvious, and the proportion of coarser particles increased annually. The median size of riverbed materials coarsened to 10 mm with annual fluctuations. The coarsening of riverbed sediment at Yichang reach was ascribed to erosion, which tends to resuspend the finer grains and leave the coarser particles on the riverbed (Yang et al., 2018). It was observed that the maximum coarsening of surficial sediment was immediately downstream of the TGD, and riverbed erosion has become the dominant source of suspended sediment in the middle and lower Yangtze reaches since the beginning of the TGD (Yang et al., 2018; Guo et al., 2019). The size of riverbed materials at Yichang station increased to the maximum value in 2009 due to the 175 m experimental impoundment of the TGR in 2008, with pebbles as the main component. After 2010, there was more sediment deposition in the Yichang reach, with a slight increase in the content of fine-grained materials. From 2012 to 2014, the riverbed materials changed with the erosion and deposition conditions in the river reach. Gravel and pebbles were still the main components of the riverbed materials. The content of sand particles less than 2 mm was low. In 2015, due to the reduction in incoming sand from upstream, weak scouring occurred in the Yichang reach, and the proportion of gravel and pebbles increased. Thus, the riverbed at Yichang station coarsened significantly. The median post-flood particle size of riverbed materials at Yichang station in 2017 was 43.1 mm. Although the surficial sediment at Yichang reach has been coarsening significantly, it is expected that armouring of the coarsening reach will prevent further erosion.
Changes in Gradient and Roughness
Changes in Gradient
The common result of riverbed undercutting erosion is water level drop. Since the GD has been in operation, low water levels at stations along the reach (Q: 5,000 m3/s) demonstrated a cumulative downward trend (Table 4).
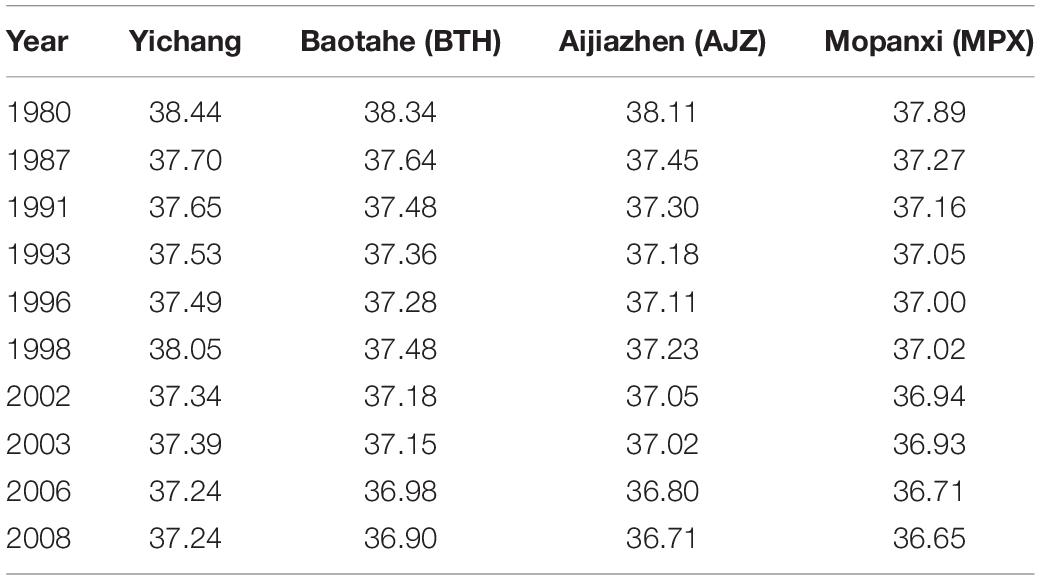
Table 4. Statistics of low water levels at each water level station along the Yichang reach under the flow discharge of 5,000 m3/s.
Figure 7 shows the water surface gradient of each section of the Yichang reach under different flow rates in 2012 after the impoundment of the TGR. The figure demonstrates that as flow rate increased, the overall gradient also increased. Gradients around YZB and MPX were small, which could control the low water level. For intermediate flood discharges, the control of YZB on the gradient is clearly weakened, while MPX has certain control at all discharge levels.
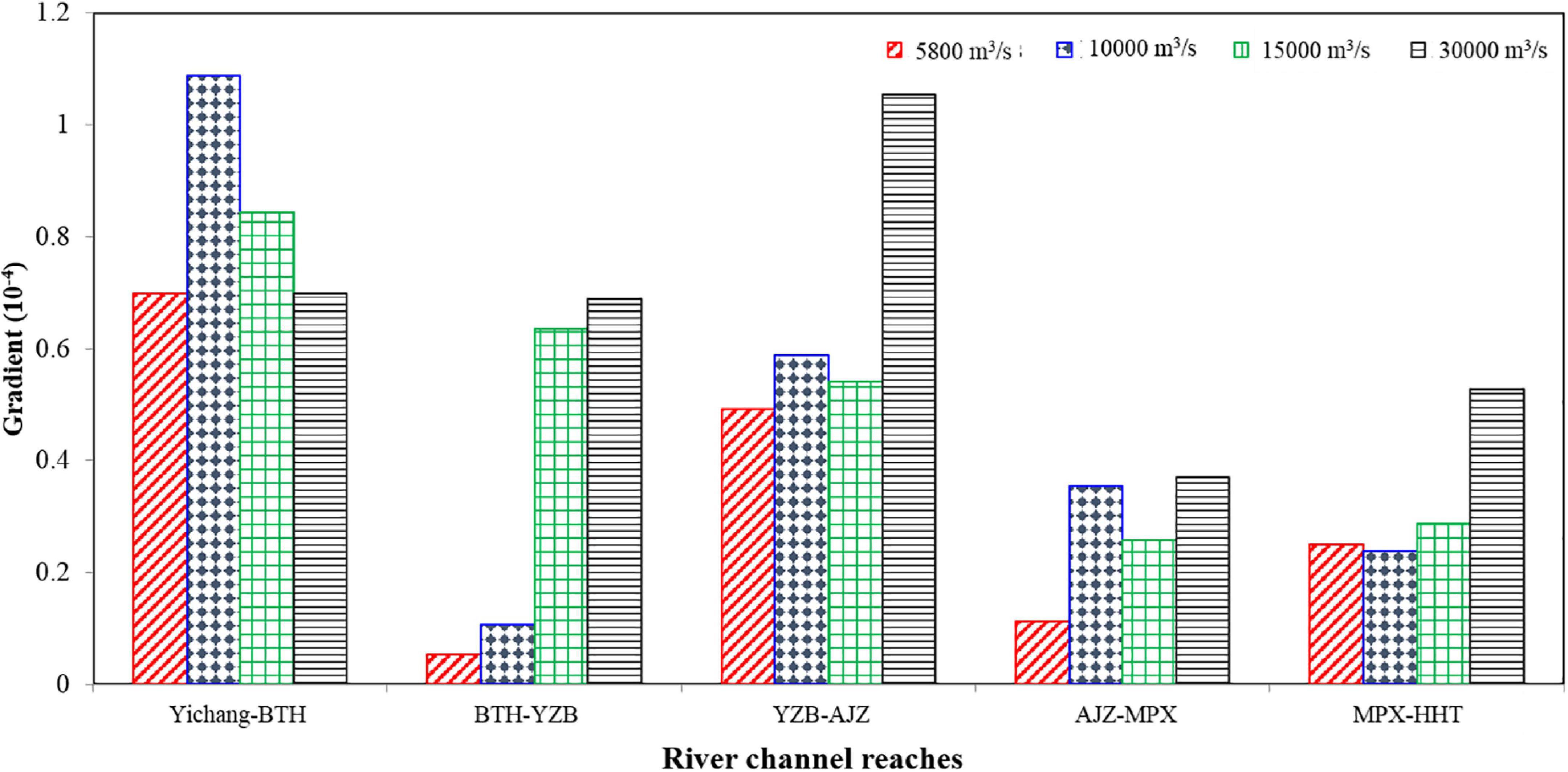
Figure 7. Water surface gradient of each section of the Yichang reach at different flow discharges in 2012.
Since the impoundment of the TGR, there has been a certain degree of adjustment in the water surface gradient in the Yichang reach (Figure 8). In general, the gradient of the downstream reach of the dam is lower, however, the gradient of the Yichang reach increased, especially in the section from the GD to the BTH. A larger gradient indicates that the roughness of the section will increase. Accordingly, the gradient of the section from YZB to AJZ slightly increased.
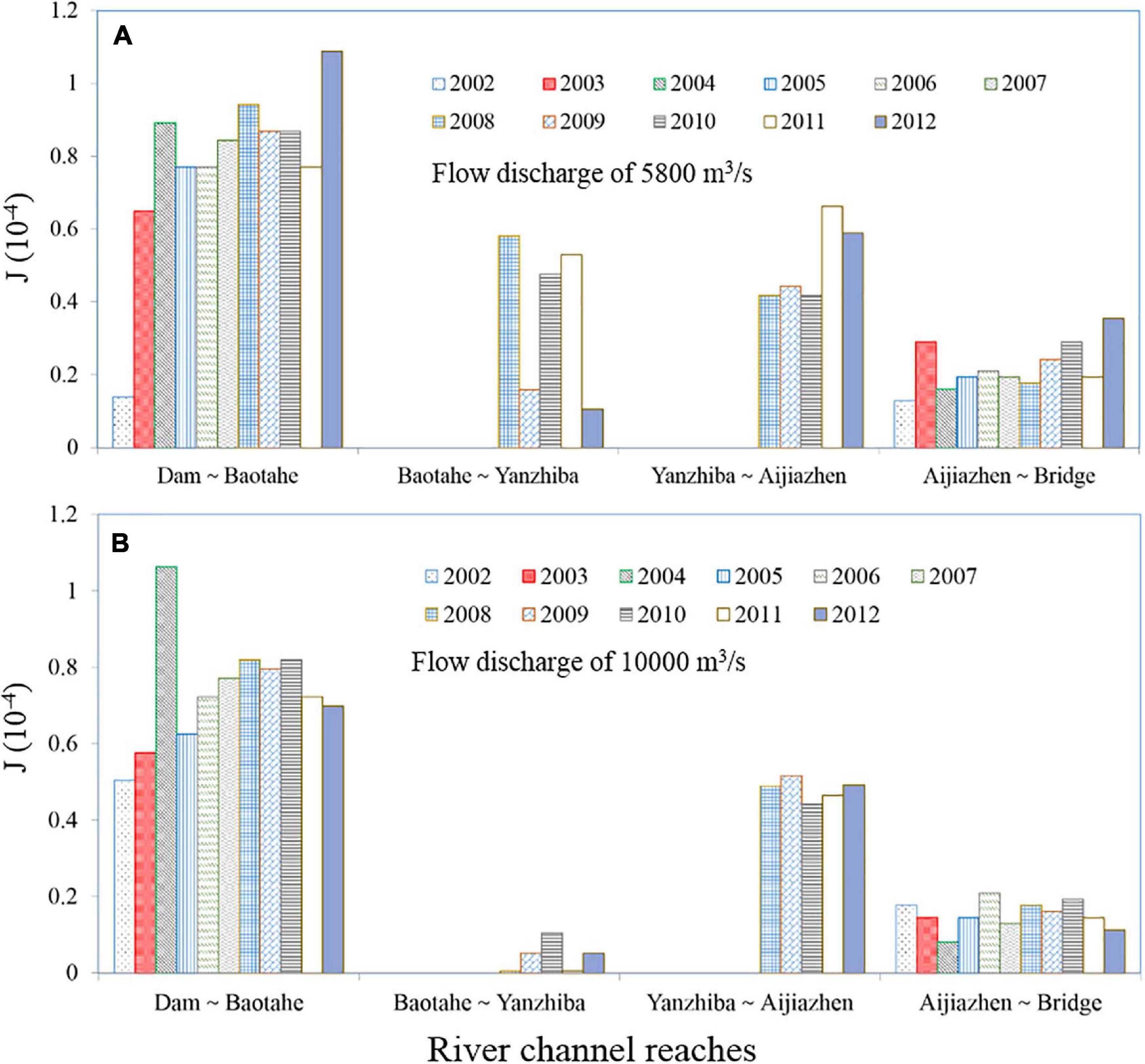
Figure 8. Water surface gradient variation of each section in the Yichang reach at two different flow discharges: (A) 5,800 m3/s; (B) 10,000 m3/s.
Changes in Resistance After Riverbed Armouring
For sandy pebble reaches, the resistance of sand particles on the riverbed surface is a major source of resistance. Downstream of the TGD, the differences in grain size after bed surface armouring increased 100-fold, which inevitably led to a significant change in riverbed surface resistance (Rinaldi and Simon, 1998).
Resistance adjustment can be visualized by the changes in the waterlines (Zhou et al., 2018). Using the measured topographic and water level data of 2003 and 2012, the changes in channel roughness were analysed. The results showed that the channel roughness in 2012 increased significantly compared to that in 2003 (Table 5), which was consistent with the riverbed surface armouring. The erosion of the cross section increased, and thus the hydrodynamic force was reduced. The roughness increase was beneficial to restrain the drawdown of normal and low water levels and reduced the flow rate. However, it was also likely to increase the turbulence near the riverbed, especially at the normal and low flow rates.
Effects on the Spawning Ground of Chinese Sturgeon
After the construction of GD, the spawning ground of Chinese sturgeon located just downstream of the GD (Yichang reach) was the only natural one that had been found (Tao et al., 2009). Thus it is crucial to retain the availability of this spawning ground for the sustainability of this critically endangered species.
Researches showed that sturgeons were selective in their spawning ground, and the specific spawning locations were generally around the sharp bends of river with complex flow patterns, with hard bed materials, and with water depth ranging from a few meters to 20–30 m (Paragamian and Wakkinen, 2002). Some biologists held that there was a certain relationship between the spawning of Chinese sturgeon and the hydrology as well as the substrate types of the river bottom (Chang, 1999; Zhang et al., 2011; Zhou et al., 2014). The spawn of Chinese sturgeon requires a series of certain environmental conditions, including water temperature, riverbed topography, substrate, hydrological and hydraulic conditions etc. (Zhang et al., 2011; Shen et al., 2018). It was found that the preferred flow velocity and suspended sediment concentration for the spawning of Chinese sturgeon were in the range of 1.0–1.7 m s–1 and 0.2–0.3 kg m–3, respectively (Yang et al., 2007b). Considering the water depth, the Chinese sturgeon appears more frequently in 6–15 m, regardless of whether it is male or female (Yang, 2007).
Among the influencing factors, the topography of the riverbed plays a key role, as the change of it could lead to the variations of substrate, hydrological and hydraulic conditions to a certain extent. According to the results of filed surveys, it was found that the morphology, gradient, and roughness of the Yichang reach had suffered from significant changes due to the erosion by the clean water from the TGR. The channel gradient increased, especially in the upstream section of YZB. The riverbed roughness also increased accordingly. With the erosion of the river cross section, the hydrodynamic force of the reach was weakened, which brought new impacts on the spawning ground of Chinese sturgeon. Good news is that except for the branched section, the adjustment range of riverbed scouring in the Yichang reach was small and stable. Thus, it is expected that as the riverbed topography tends to be stable after more than 10 years of erosion since 2003, the spawning grounds of Chinese sturgeon could also be retained. Thoroughly evaluation of the ecological effects is required in future studies, and necessary measures should be taken to rehabilitate the spawning ground of Chinese sturgeon if the adverse effects on the survival and development of Chinese sturgeon continues.
Conclusion
This work examined the construction of GD and TGD and their impacts on the streamflow, sediment load and channel morphology downstream based on in situ measured data. The operation of the GD reduced sediment inflow to Yichang station and triggered the prevailing scouring of the Yichang reach, which is the only regular spawning ground of Chinese sturgeon after the construction of GD. The impoundment of TGD greatly altered the water-sediment conditions of Yichang reach, which is manifested in the flow process regulation, sharp sediment content reduction, and particle size decrease etc. Thus, the river channel was further eroded, especially in the form of undercutting.
In recent years, channel erosion has been uneven as there are some scour resistant nodes in the reach, such as YZB and MPX. The water surface gradient is controlled by such nodes, and the water level of each section drops unevenly. Overall, the low water surface gradient has decreased. According to the grading of riverbed sediment, the riverbed scouring and armouring in the Yichang reach was basically completed, and the riverbed material has been transformed from sandy gravel to pebble. The channel gradient and riverbed roughness increased with the erosion of the river cross section, especially in the upstream section of YZB. The hydrodynamic force of the Yichang reach was weakened, while the adjustment range of riverbed scouring was small and stable. It is expected that the spawning grounds of Chinese sturgeon could be retained as the riverbed tends to be stable. Further evaluation and necessary steps should be taken considering the influences of clean water erosion and related problems in the downstream ecological.
Data Availability Statement
The original contributions presented in the study are included in the article/supplementary material, further inquiries can be directed to the corresponding author/s.
Author Contributions
YZ was responsible for the writing and design of data analysis. ZL, SY, MS, and CG were responsible for data analysis and discussion. All authors contributed to the article and approved the submitted version.
Funding
This research was funded by the National Key Research and Development Program of China (2019YFC1510704 and 2016YFC0402300), the Fundamental Research Funds for Central Public Welfare Research Institutes (CKSF2019246/HL and CKSF2019171/HL), Natural Science Foundation of China (Nos. 51579014, 41906149, and 51609013), and the National Major Hydraulic Engineering Construction Funds “Research Program on Key Sediment Problems of the Three Gorges Project” (12610100000018J129-05).
Conflict of Interest
The authors declare that the research was conducted in the absence of any commercial or financial relationships that could be construed as a potential conflict of interest.
Footnotes
References
Chang, J. (1999). Structure and dynamics of the spawning stock of Chinese sturgeon, Acipenser sinensis, in the Yangtze River. Doctoral dissertation. Wuhan: Institute of Hydrobiology, Chinese Academy of Sciences, 149.
Doyle, M. W., and Harbor, J. M. (2003). A scaling approximation of equilibrium timescales forsand-bed and gravel-bed rivers responding to base-level lowering. Geomorphology 54, 217–223. doi: 10.1016/s0169-555x(02)00357-4
Friedman, W. R., Osterkamp, M. L., and Scott, G. T. A. (1998). Downstream effects of dams on channel geometry and bottomland vegetation: regional patterns in the great plains. Wetlands 18, 619–633. doi: 10.1007/bf03161677
Guo, C., Jin, Z., Guo, L., Lu, J., and Zhou, Y. (2020). On the cumulative dam impact in the upper Changjiang river: streamflow and sediment load changes. Catena 184, 104250. doi: 10.1016/j.catena.2019.104250
Guo, H., Hu, Q., Zhang, Q., and Feng, S. (2012). Effects of the three gorges dam on yangtze river flow and river interaction with poyang lake, china: 2003-2008. J. Hydrol. 416, 19–27. doi: 10.1016/j.jhydrol.2011.11.027
Guo, L., Su, N., Townend, I., Wang, Z., and He, Q. (2019). From the headwater to the delta: a synthesis of the basin-scale sediment load regime in the changjiang river. Earth Sci. Rev. 197:102900. doi: 10.1016/j.earscirev.2019.102900
Han, J., Sun, Z., Li, Y., and Yang, Y. (2017). Combined effects of multiple large-scale hydraulic engineering on water stages in the middle yangtze river. Geomorphology 298, 31–40. doi: 10.1016/j.geomorph.2017.09.034
Li, D., Lu, X., Chen, L., and Wasson, R. J. (2019). Downstream geomorphic impact of the three gorges dam: with special reference to the channel bars in the middle yangtze river. Earth Surface Proc. Landforms 44, 2660–2670. doi: 10.1002/esp.4691
Li, Q., Yu, M., Lu, G., Cai, T., Bai, X., and Xia, Z. (2011). Impacts of the Gezhouba and Three Gorges reservoirs on the sediment regime in the yangtze river, china. J. Hydrol. 403, 224–233. doi: 10.1016/j.jhydrol.2011.03.043
Liermann, C., Nilsson, C., Robertson, J., and Ng, R. Y. (2012). Implications of dam obstruction for global freshwater fish diversity. Bioscience 62, 539–548. doi: 10.1525/bio.2012.62.6.5
Paragamian, V., and Wakkinen, V. (2002). Temporal distribution of kootenai river white sturgeon spawning events and the effect of flow and temperature. J. Appl. Ichthyol. 18, 542–549. doi: 10.1046/j.1439-0426.2002.00391.x
Ren, S., Zhang, B., Wang, W., Yuan, Y., and Guo, C. (2021). Sedimentation and its response to management strategies of the three gorges reservoir, yangtze river, china. Catena 199:105096. doi: 10.1016/j.catena.2020.105096
Rinaldi, M., and Simon, A. (1998). Bed-level adjustments in the arno river, central italy. Geomorphology 22, 57–71. doi: 10.1016/s0169-555x(97)00054-8
Shen, Y., Wang, P., Wang, C., Yu, Y., and Kong, N. (2018). Potential causes of habitat degradation and spawning time delay of the chinese sturgeon (acipenser sinensis). Ecol. Infor. 43, 96–105. doi: 10.1016/j.ecoinf.2017.11.002
Tao, J., Qiao, Y., Tan, X., and Chang, J. (2009). Species identification of chinese sturgeon using acoustic descriptors and ascertaining their spatial distribution in the spawning ground of Gezhouba Dam. Chinese Sci. Bull. 54, 3972–3980. doi: 10.1007/s11434-009-0557-9
Wang, C., Kynard, B., Wei, Q., Du, H., and Zhang, H. (2013). Spatial distribution and habitat suitability indices for non-spawning and spawning adult chinese sturgeons below gezhouba dam, yangtze river: effects of river alterations. J. Appl. Ichthyol. 29, 31–40. doi: 10.1111/jai.12094
Xia, J., Deng, S., Zhou, M., Lu, J., and Xu, Q. (2017). Geomorphic response of the Jingjiang Reach to the Three Gorges Project operation. Earth Surface Proc. Landforms 42, 866–876. doi: 10.1002/esp.4043
Yang, H., Yang, S., Xu, K., Milliman, J., Wang, H., Yang, Z., et al. (2018). Human impacts on sediment in the Yangtze river: a review and new perspectives. Global Planetary Change 162, 8–17. doi: 10.1016/j.gloplacha.2018.01.001
Yang, S., Milliman, J., Xu, K., Deng, B., Zhang, X., and Luo, X. (2014). Downstream sedimentary and geomorphic impacts of the three gorges dam on the yangtze river. Earth Sci. Rev. 138, 470–486.
Yang, S., Zhang, J., and Xu, X. (2007a). Influence of the three gorges dam on downstream delivery of sediment and its environmental implications, yangtze river. Geophy. Res. Lett. 34:L10401.
Yang, D., Wei, Q., Chen, X., Liu, J., Zhu, Y., and Wang, K. (2007b). Hydrology status on the spawning ground of Acipenser sinensis below the Gezhouba Dam and its relation to the spawning run. Acta Ecologica Sinica 27, 862–869. doi: 10.1016/s1872-2032(07)60023-7
Yang, Y. (2007). Study on hydraulic characteristics of Chinese sturgeon habitat in Yangtze river. Nanjing: Hohai University.
Yuan, W., Yin, D., Finlayson, B., and Chen, Z. (2012). Assessing the potential for change in the middle Yangtze River channel following impoundment of the three gorges dam. Geomorphology. 147, 27–34. doi: 10.1016/j.geomorph.2011.06.039
Yuan, X., Tian, F., Wang, X., Liu, Y., and Chen, M. (2018). Small-scale sediment scouring and siltation laws in the evolution trends of fluvial facies in the ningxia plain reaches of the yellow river (NPRYR). Quat. Int. 476, 14–25. doi: 10.1016/j.quaint.2018.03.034
Zhang, H., Wei, Q., Kynard, B., Du, H., Yang, D., and Chen, X. (2011). Spatial structure and bottom characteristics of the only remaining spawning area of chinese sturgeon in the yangtze river. J. Appl. Ichthyol. 27, 251–256. doi: 10.1111/j.1439-0426.2011.01708.x
Zhang, Q., Singh, V. P., Xu, C., and Chen, X. (2013). Abrupt behaviours of streamflow and sediment load variations of the yangtze river basin, china. Hydrol. Process 27, 444–452. doi: 10.1002/hyp.9278
Zhang, W., Yang, Y., Zhang, M., Li, Y., Zhu, L., You, X., et al. (2017). Mechanisms of suspended sediment restoration and bed level compensation in downstream reaches of the Three gorges projects (TGP). J. Geographic Sci. 27, 463–480. doi: 10.1007/s11442-017-1387-3
Zhou, J., Zhang, H., Song, L., Bi, S., and Zhao, Y. (2014). Assessing the effect of the three gorges reservoir impoundment on spawning habitat suitability of chinese sturgeon (acipenser sinensis) in yangtze river, china. Ecol. Infor. 20, 33–46. doi: 10.1016/j.ecoinf.2014.01.008
Zhou, M., Xia, J., Lu, J., Deng, S., and Lin, F. (2017). Morphological adjustments in a meandering reach of the middle yangtze river caused by severe human activities. Geomorphology 285, 325–332. doi: 10.1016/j.geomorph.2017.02.022
Zhou, Y., Lu, J., Chen, L., and Ren, J. (2018). Bed roughness adjustments determined from fractal measurements of river-bed morphology. J. Hydrodynamics 30, 882–889. doi: 10.1007/s42241-018-0101-y
Keywords: Three Gorges Reservoir, Chinese sturgeon, spawning grounds, riverbed evolution, Yangtze river
Citation: Zhou Y, Li Z, Yao S, Shan M and Guo C (2021) Case Study: Influence of Three Gorges Reservoir Impoundment on Hydrological Regime of the Acipenser sinensis Spawning Ground, Yangtze River, China. Front. Ecol. Evol. 9:624447. doi: 10.3389/fevo.2021.624447
Received: 31 October 2020; Accepted: 22 January 2021;
Published: 11 February 2021.
Edited by:
Paul Robbins, University of Wisconsin-Madison, United StatesReviewed by:
Swapan Talukdar, University of Gour Banga, IndiaMatthew McCartney, International Water Management Institute, Sri Lanka
Copyright © 2021 Zhou, Li, Yao, Shan and Guo. This is an open-access article distributed under the terms of the Creative Commons Attribution License (CC BY). The use, distribution or reproduction in other forums is permitted, provided the original author(s) and the copyright owner(s) are credited and that the original publication in this journal is cited, in accordance with accepted academic practice. No use, distribution or reproduction is permitted which does not comply with these terms.
*Correspondence: Zhijing Li, bHpqa2V0dHlAMTI2LmNvbQ==; Chao Guo, Z3VvY0BtYWlsLmNyc3JpLmNu; NjAzMTEwNjQyQHFxLmNvbQ==